Community Disassembly in Ephemeral Ecosystems
VerifiedAdded on 2023/01/19
|26
|7503
|40
AI Summary
This article discusses the process of community disassembly in ephemeral ecosystems, focusing on the mechanisms and patterns of disassembly. It explores how decreasing habitat size, harshening habitat conditions, ultraviolet light exposure, and the collapse of the entire metacommunity contribute to the disassembly process. The article also highlights the importance of understanding natural disassembly in shaping ecosystem structure and function.
Contribute Materials
Your contribution can guide someone’s learning journey. Share your
documents today.
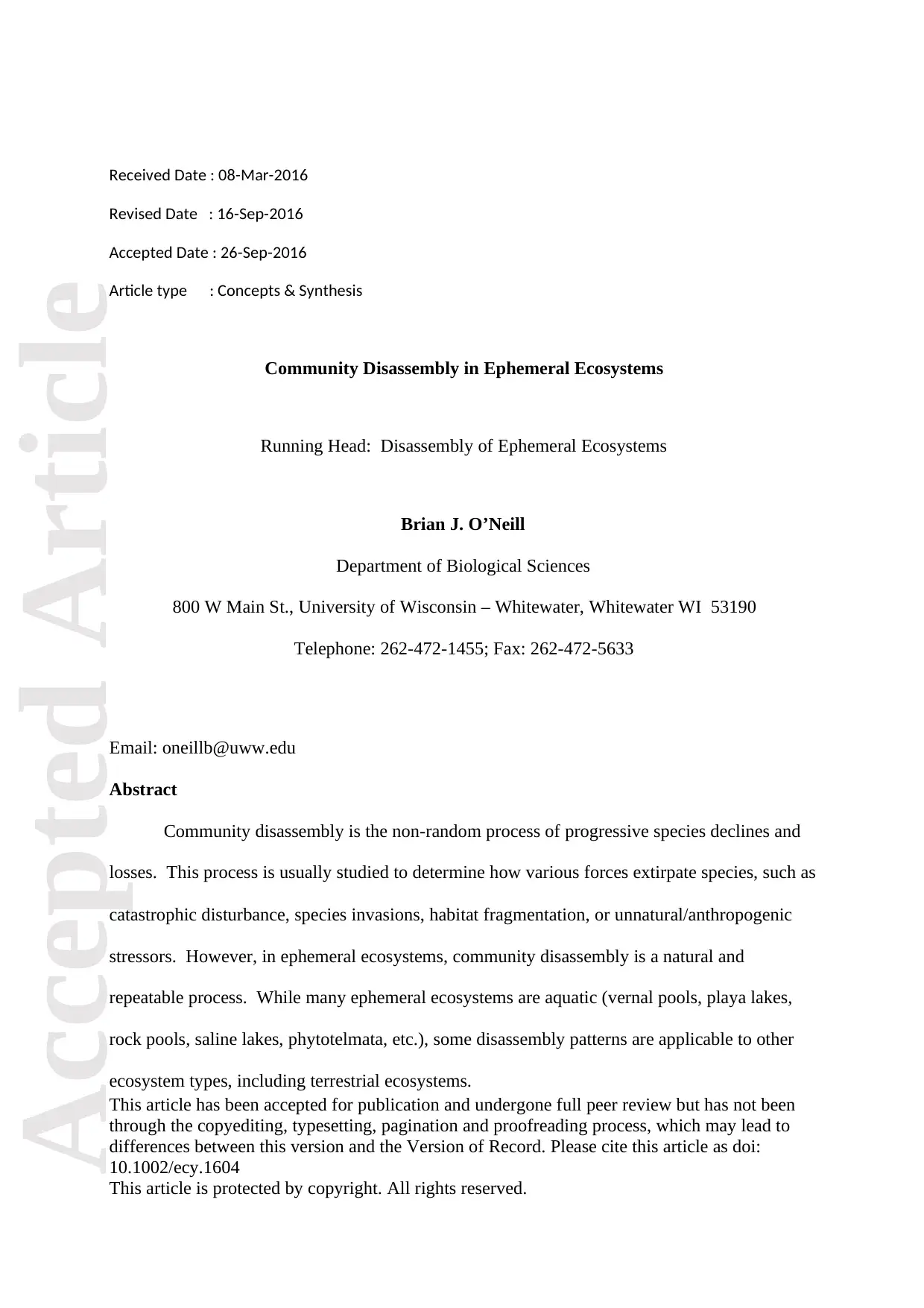
Accepted ArticleAccepted Article
This article has been accepted for publication and undergone full peer review but has not been
through the copyediting, typesetting, pagination and proofreading process, which may lead to
differences between this version and the Version of Record. Please cite this article as doi:
10.1002/ecy.1604
This article is protected by copyright. All rights reserved.
Received Date : 08-Mar-2016
Revised Date : 16-Sep-2016
Accepted Date : 26-Sep-2016
Article type : Concepts & Synthesis
Community Disassembly in Ephemeral Ecosystems
Running Head: Disassembly of Ephemeral Ecosystems
Brian J. O’Neill
Department of Biological Sciences
800 W Main St., University of Wisconsin – Whitewater, Whitewater WI 53190
Telephone: 262-472-1455; Fax: 262-472-5633
Email: oneillb@uww.edu
Abstract
Community disassembly is the non-random process of progressive species declines and
losses. This process is usually studied to determine how various forces extirpate species, such as
catastrophic disturbance, species invasions, habitat fragmentation, or unnatural/anthropogenic
stressors. However, in ephemeral ecosystems, community disassembly is a natural and
repeatable process. While many ephemeral ecosystems are aquatic (vernal pools, playa lakes,
rock pools, saline lakes, phytotelmata, etc.), some disassembly patterns are applicable to other
ecosystem types, including terrestrial ecosystems.
This article has been accepted for publication and undergone full peer review but has not been
through the copyediting, typesetting, pagination and proofreading process, which may lead to
differences between this version and the Version of Record. Please cite this article as doi:
10.1002/ecy.1604
This article is protected by copyright. All rights reserved.
Received Date : 08-Mar-2016
Revised Date : 16-Sep-2016
Accepted Date : 26-Sep-2016
Article type : Concepts & Synthesis
Community Disassembly in Ephemeral Ecosystems
Running Head: Disassembly of Ephemeral Ecosystems
Brian J. O’Neill
Department of Biological Sciences
800 W Main St., University of Wisconsin – Whitewater, Whitewater WI 53190
Telephone: 262-472-1455; Fax: 262-472-5633
Email: oneillb@uww.edu
Abstract
Community disassembly is the non-random process of progressive species declines and
losses. This process is usually studied to determine how various forces extirpate species, such as
catastrophic disturbance, species invasions, habitat fragmentation, or unnatural/anthropogenic
stressors. However, in ephemeral ecosystems, community disassembly is a natural and
repeatable process. While many ephemeral ecosystems are aquatic (vernal pools, playa lakes,
rock pools, saline lakes, phytotelmata, etc.), some disassembly patterns are applicable to other
ecosystem types, including terrestrial ecosystems.
Secure Best Marks with AI Grader
Need help grading? Try our AI Grader for instant feedback on your assignments.
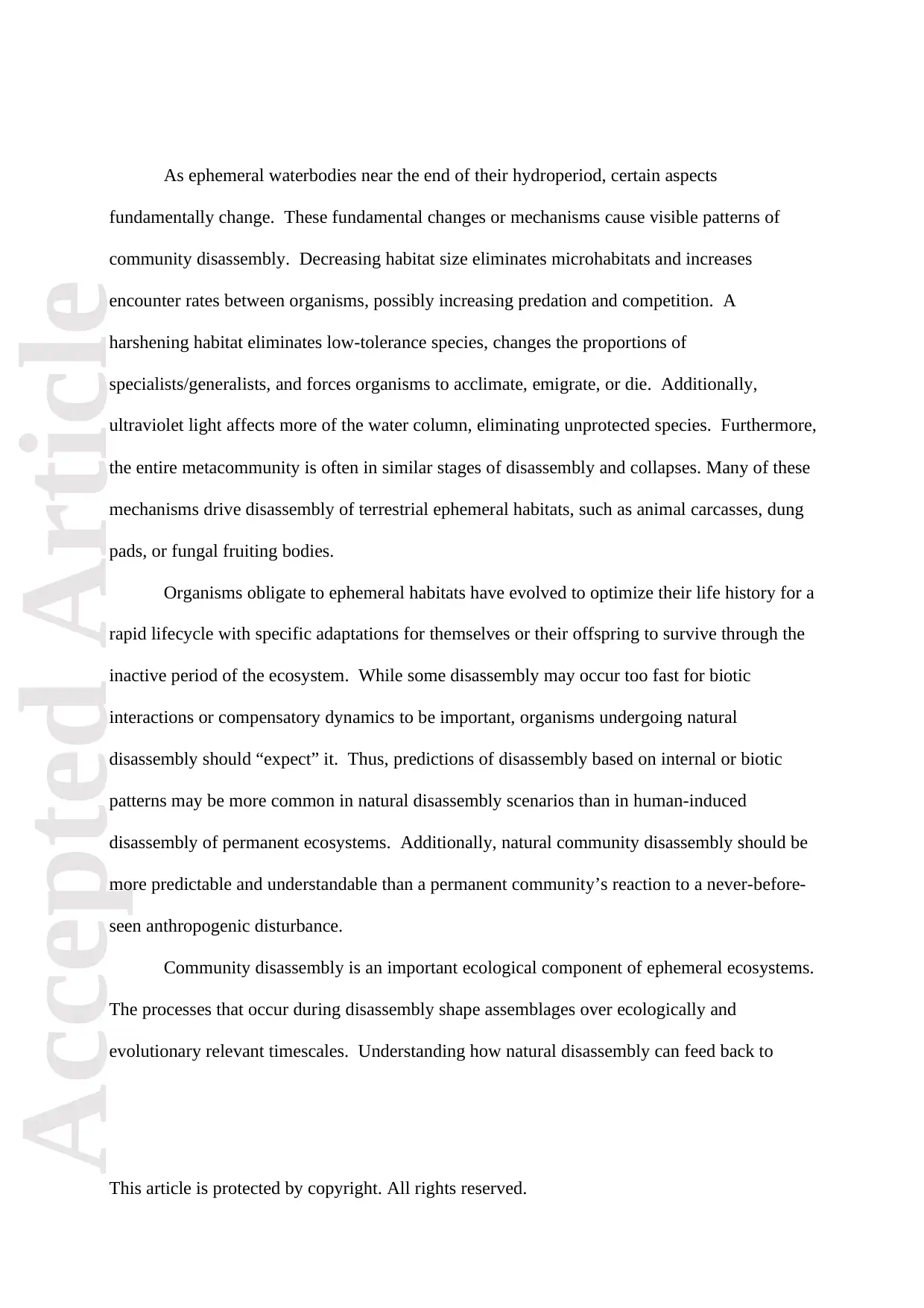
Accepted ArticleAccepted Article
This article is protected by copyright. All rights reserved.
As ephemeral waterbodies near the end of their hydroperiod, certain aspects
fundamentally change. These fundamental changes or mechanisms cause visible patterns of
community disassembly. Decreasing habitat size eliminates microhabitats and increases
encounter rates between organisms, possibly increasing predation and competition. A
harshening habitat eliminates low-tolerance species, changes the proportions of
specialists/generalists, and forces organisms to acclimate, emigrate, or die. Additionally,
ultraviolet light affects more of the water column, eliminating unprotected species. Furthermore,
the entire metacommunity is often in similar stages of disassembly and collapses. Many of these
mechanisms drive disassembly of terrestrial ephemeral habitats, such as animal carcasses, dung
pads, or fungal fruiting bodies.
Organisms obligate to ephemeral habitats have evolved to optimize their life history for a
rapid lifecycle with specific adaptations for themselves or their offspring to survive through the
inactive period of the ecosystem. While some disassembly may occur too fast for biotic
interactions or compensatory dynamics to be important, organisms undergoing natural
disassembly should “expect” it. Thus, predictions of disassembly based on internal or biotic
patterns may be more common in natural disassembly scenarios than in human-induced
disassembly of permanent ecosystems. Additionally, natural community disassembly should be
more predictable and understandable than a permanent community’s reaction to a never-before-
seen anthropogenic disturbance.
Community disassembly is an important ecological component of ephemeral ecosystems.
The processes that occur during disassembly shape assemblages over ecologically and
evolutionary relevant timescales. Understanding how natural disassembly can feed back to
This article is protected by copyright. All rights reserved.
As ephemeral waterbodies near the end of their hydroperiod, certain aspects
fundamentally change. These fundamental changes or mechanisms cause visible patterns of
community disassembly. Decreasing habitat size eliminates microhabitats and increases
encounter rates between organisms, possibly increasing predation and competition. A
harshening habitat eliminates low-tolerance species, changes the proportions of
specialists/generalists, and forces organisms to acclimate, emigrate, or die. Additionally,
ultraviolet light affects more of the water column, eliminating unprotected species. Furthermore,
the entire metacommunity is often in similar stages of disassembly and collapses. Many of these
mechanisms drive disassembly of terrestrial ephemeral habitats, such as animal carcasses, dung
pads, or fungal fruiting bodies.
Organisms obligate to ephemeral habitats have evolved to optimize their life history for a
rapid lifecycle with specific adaptations for themselves or their offspring to survive through the
inactive period of the ecosystem. While some disassembly may occur too fast for biotic
interactions or compensatory dynamics to be important, organisms undergoing natural
disassembly should “expect” it. Thus, predictions of disassembly based on internal or biotic
patterns may be more common in natural disassembly scenarios than in human-induced
disassembly of permanent ecosystems. Additionally, natural community disassembly should be
more predictable and understandable than a permanent community’s reaction to a never-before-
seen anthropogenic disturbance.
Community disassembly is an important ecological component of ephemeral ecosystems.
The processes that occur during disassembly shape assemblages over ecologically and
evolutionary relevant timescales. Understanding how natural disassembly can feed back to
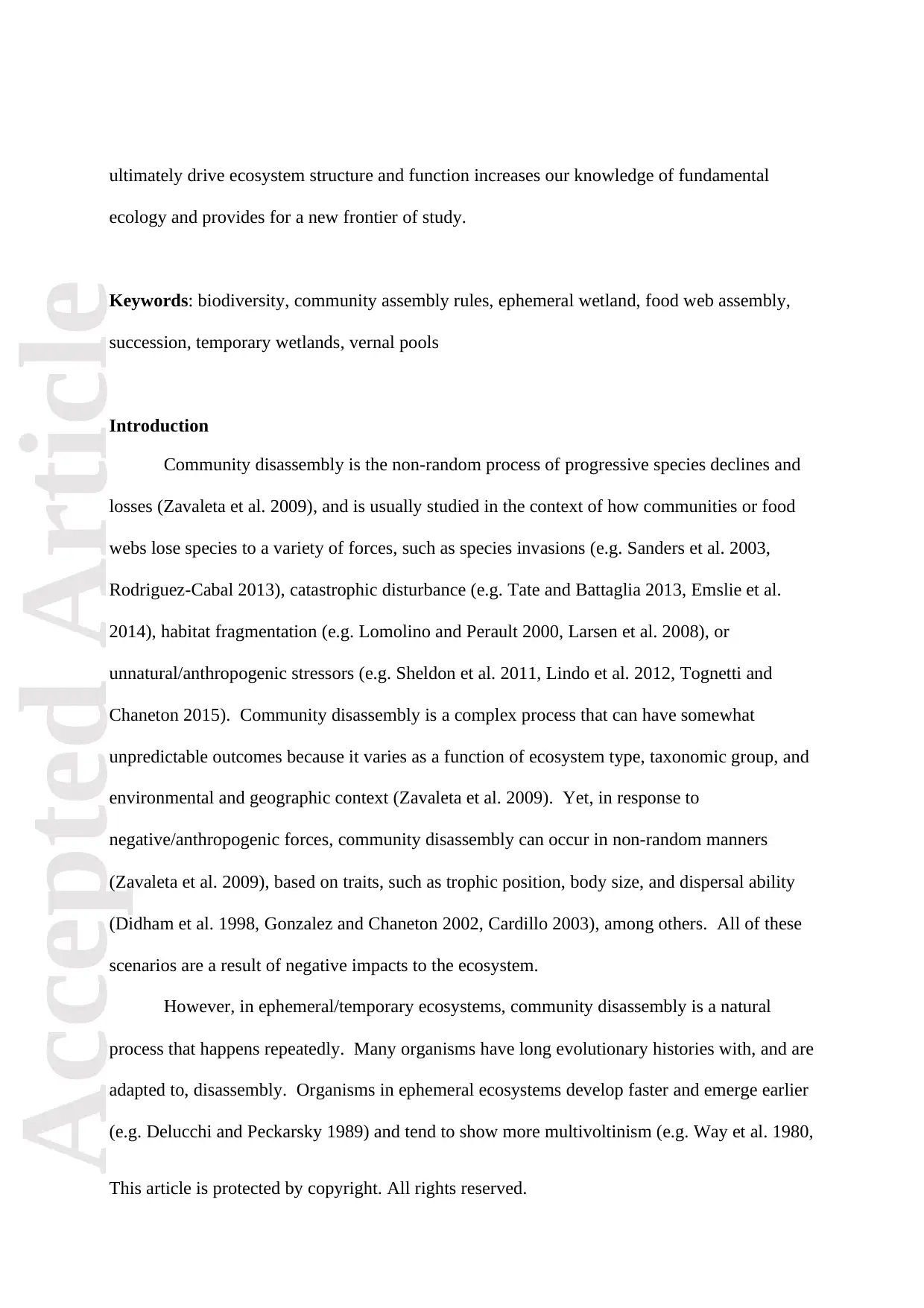
Accepted ArticleAccepted Article
This article is protected by copyright. All rights reserved.
ultimately drive ecosystem structure and function increases our knowledge of fundamental
ecology and provides for a new frontier of study.
Keywords: biodiversity, community assembly rules, ephemeral wetland, food web assembly,
succession, temporary wetlands, vernal pools
Introduction
Community disassembly is the non-random process of progressive species declines and
losses (Zavaleta et al. 2009), and is usually studied in the context of how communities or food
webs lose species to a variety of forces, such as species invasions (e.g. Sanders et al. 2003,
Rodriguez-Cabal 2013), catastrophic disturbance (e.g. Tate and Battaglia 2013, Emslie et al.
2014), habitat fragmentation (e.g. Lomolino and Perault 2000, Larsen et al. 2008), or
unnatural/anthropogenic stressors (e.g. Sheldon et al. 2011, Lindo et al. 2012, Tognetti and
Chaneton 2015). Community disassembly is a complex process that can have somewhat
unpredictable outcomes because it varies as a function of ecosystem type, taxonomic group, and
environmental and geographic context (Zavaleta et al. 2009). Yet, in response to
negative/anthropogenic forces, community disassembly can occur in non-random manners
(Zavaleta et al. 2009), based on traits, such as trophic position, body size, and dispersal ability
(Didham et al. 1998, Gonzalez and Chaneton 2002, Cardillo 2003), among others. All of these
scenarios are a result of negative impacts to the ecosystem.
However, in ephemeral/temporary ecosystems, community disassembly is a natural
process that happens repeatedly. Many organisms have long evolutionary histories with, and are
adapted to, disassembly. Organisms in ephemeral ecosystems develop faster and emerge earlier
(e.g. Delucchi and Peckarsky 1989) and tend to show more multivoltinism (e.g. Way et al. 1980,
This article is protected by copyright. All rights reserved.
ultimately drive ecosystem structure and function increases our knowledge of fundamental
ecology and provides for a new frontier of study.
Keywords: biodiversity, community assembly rules, ephemeral wetland, food web assembly,
succession, temporary wetlands, vernal pools
Introduction
Community disassembly is the non-random process of progressive species declines and
losses (Zavaleta et al. 2009), and is usually studied in the context of how communities or food
webs lose species to a variety of forces, such as species invasions (e.g. Sanders et al. 2003,
Rodriguez-Cabal 2013), catastrophic disturbance (e.g. Tate and Battaglia 2013, Emslie et al.
2014), habitat fragmentation (e.g. Lomolino and Perault 2000, Larsen et al. 2008), or
unnatural/anthropogenic stressors (e.g. Sheldon et al. 2011, Lindo et al. 2012, Tognetti and
Chaneton 2015). Community disassembly is a complex process that can have somewhat
unpredictable outcomes because it varies as a function of ecosystem type, taxonomic group, and
environmental and geographic context (Zavaleta et al. 2009). Yet, in response to
negative/anthropogenic forces, community disassembly can occur in non-random manners
(Zavaleta et al. 2009), based on traits, such as trophic position, body size, and dispersal ability
(Didham et al. 1998, Gonzalez and Chaneton 2002, Cardillo 2003), among others. All of these
scenarios are a result of negative impacts to the ecosystem.
However, in ephemeral/temporary ecosystems, community disassembly is a natural
process that happens repeatedly. Many organisms have long evolutionary histories with, and are
adapted to, disassembly. Organisms in ephemeral ecosystems develop faster and emerge earlier
(e.g. Delucchi and Peckarsky 1989) and tend to show more multivoltinism (e.g. Way et al. 1980,
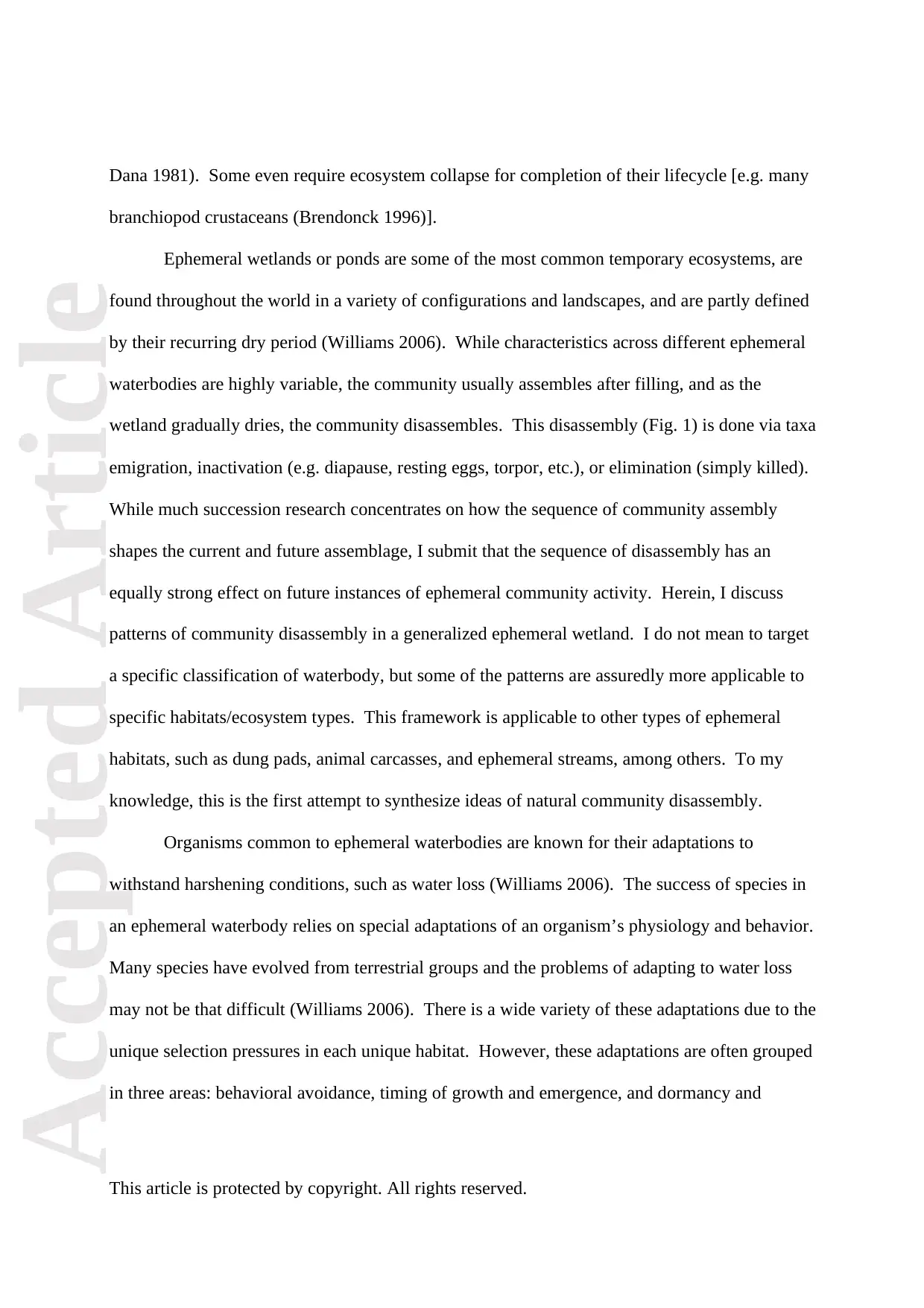
Accepted ArticleAccepted Article
This article is protected by copyright. All rights reserved.
Dana 1981). Some even require ecosystem collapse for completion of their lifecycle [e.g. many
branchiopod crustaceans (Brendonck 1996)].
Ephemeral wetlands or ponds are some of the most common temporary ecosystems, are
found throughout the world in a variety of configurations and landscapes, and are partly defined
by their recurring dry period (Williams 2006). While characteristics across different ephemeral
waterbodies are highly variable, the community usually assembles after filling, and as the
wetland gradually dries, the community disassembles. This disassembly (Fig. 1) is done via taxa
emigration, inactivation (e.g. diapause, resting eggs, torpor, etc.), or elimination (simply killed).
While much succession research concentrates on how the sequence of community assembly
shapes the current and future assemblage, I submit that the sequence of disassembly has an
equally strong effect on future instances of ephemeral community activity. Herein, I discuss
patterns of community disassembly in a generalized ephemeral wetland. I do not mean to target
a specific classification of waterbody, but some of the patterns are assuredly more applicable to
specific habitats/ecosystem types. This framework is applicable to other types of ephemeral
habitats, such as dung pads, animal carcasses, and ephemeral streams, among others. To my
knowledge, this is the first attempt to synthesize ideas of natural community disassembly.
Organisms common to ephemeral waterbodies are known for their adaptations to
withstand harshening conditions, such as water loss (Williams 2006). The success of species in
an ephemeral waterbody relies on special adaptations of an organism’s physiology and behavior.
Many species have evolved from terrestrial groups and the problems of adapting to water loss
may not be that difficult (Williams 2006). There is a wide variety of these adaptations due to the
unique selection pressures in each unique habitat. However, these adaptations are often grouped
in three areas: behavioral avoidance, timing of growth and emergence, and dormancy and
This article is protected by copyright. All rights reserved.
Dana 1981). Some even require ecosystem collapse for completion of their lifecycle [e.g. many
branchiopod crustaceans (Brendonck 1996)].
Ephemeral wetlands or ponds are some of the most common temporary ecosystems, are
found throughout the world in a variety of configurations and landscapes, and are partly defined
by their recurring dry period (Williams 2006). While characteristics across different ephemeral
waterbodies are highly variable, the community usually assembles after filling, and as the
wetland gradually dries, the community disassembles. This disassembly (Fig. 1) is done via taxa
emigration, inactivation (e.g. diapause, resting eggs, torpor, etc.), or elimination (simply killed).
While much succession research concentrates on how the sequence of community assembly
shapes the current and future assemblage, I submit that the sequence of disassembly has an
equally strong effect on future instances of ephemeral community activity. Herein, I discuss
patterns of community disassembly in a generalized ephemeral wetland. I do not mean to target
a specific classification of waterbody, but some of the patterns are assuredly more applicable to
specific habitats/ecosystem types. This framework is applicable to other types of ephemeral
habitats, such as dung pads, animal carcasses, and ephemeral streams, among others. To my
knowledge, this is the first attempt to synthesize ideas of natural community disassembly.
Organisms common to ephemeral waterbodies are known for their adaptations to
withstand harshening conditions, such as water loss (Williams 2006). The success of species in
an ephemeral waterbody relies on special adaptations of an organism’s physiology and behavior.
Many species have evolved from terrestrial groups and the problems of adapting to water loss
may not be that difficult (Williams 2006). There is a wide variety of these adaptations due to the
unique selection pressures in each unique habitat. However, these adaptations are often grouped
in three areas: behavioral avoidance, timing of growth and emergence, and dormancy and
Secure Best Marks with AI Grader
Need help grading? Try our AI Grader for instant feedback on your assignments.
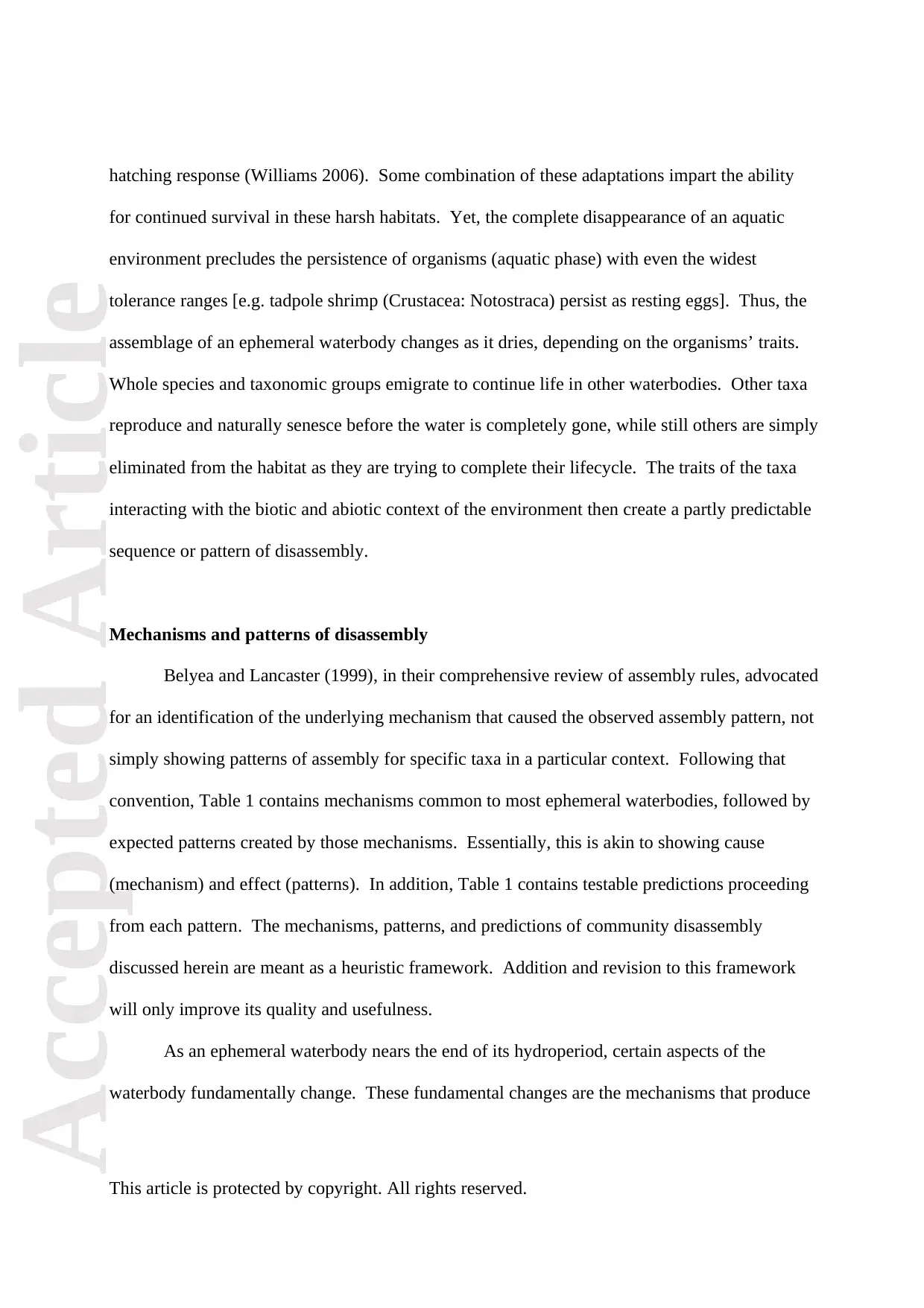
Accepted ArticleAccepted Article
This article is protected by copyright. All rights reserved.
hatching response (Williams 2006). Some combination of these adaptations impart the ability
for continued survival in these harsh habitats. Yet, the complete disappearance of an aquatic
environment precludes the persistence of organisms (aquatic phase) with even the widest
tolerance ranges [e.g. tadpole shrimp (Crustacea: Notostraca) persist as resting eggs]. Thus, the
assemblage of an ephemeral waterbody changes as it dries, depending on the organisms’ traits.
Whole species and taxonomic groups emigrate to continue life in other waterbodies. Other taxa
reproduce and naturally senesce before the water is completely gone, while still others are simply
eliminated from the habitat as they are trying to complete their lifecycle. The traits of the taxa
interacting with the biotic and abiotic context of the environment then create a partly predictable
sequence or pattern of disassembly.
Mechanisms and patterns of disassembly
Belyea and Lancaster (1999), in their comprehensive review of assembly rules, advocated
for an identification of the underlying mechanism that caused the observed assembly pattern, not
simply showing patterns of assembly for specific taxa in a particular context. Following that
convention, Table 1 contains mechanisms common to most ephemeral waterbodies, followed by
expected patterns created by those mechanisms. Essentially, this is akin to showing cause
(mechanism) and effect (patterns). In addition, Table 1 contains testable predictions proceeding
from each pattern. The mechanisms, patterns, and predictions of community disassembly
discussed herein are meant as a heuristic framework. Addition and revision to this framework
will only improve its quality and usefulness.
As an ephemeral waterbody nears the end of its hydroperiod, certain aspects of the
waterbody fundamentally change. These fundamental changes are the mechanisms that produce
This article is protected by copyright. All rights reserved.
hatching response (Williams 2006). Some combination of these adaptations impart the ability
for continued survival in these harsh habitats. Yet, the complete disappearance of an aquatic
environment precludes the persistence of organisms (aquatic phase) with even the widest
tolerance ranges [e.g. tadpole shrimp (Crustacea: Notostraca) persist as resting eggs]. Thus, the
assemblage of an ephemeral waterbody changes as it dries, depending on the organisms’ traits.
Whole species and taxonomic groups emigrate to continue life in other waterbodies. Other taxa
reproduce and naturally senesce before the water is completely gone, while still others are simply
eliminated from the habitat as they are trying to complete their lifecycle. The traits of the taxa
interacting with the biotic and abiotic context of the environment then create a partly predictable
sequence or pattern of disassembly.
Mechanisms and patterns of disassembly
Belyea and Lancaster (1999), in their comprehensive review of assembly rules, advocated
for an identification of the underlying mechanism that caused the observed assembly pattern, not
simply showing patterns of assembly for specific taxa in a particular context. Following that
convention, Table 1 contains mechanisms common to most ephemeral waterbodies, followed by
expected patterns created by those mechanisms. Essentially, this is akin to showing cause
(mechanism) and effect (patterns). In addition, Table 1 contains testable predictions proceeding
from each pattern. The mechanisms, patterns, and predictions of community disassembly
discussed herein are meant as a heuristic framework. Addition and revision to this framework
will only improve its quality and usefulness.
As an ephemeral waterbody nears the end of its hydroperiod, certain aspects of the
waterbody fundamentally change. These fundamental changes are the mechanisms that produce
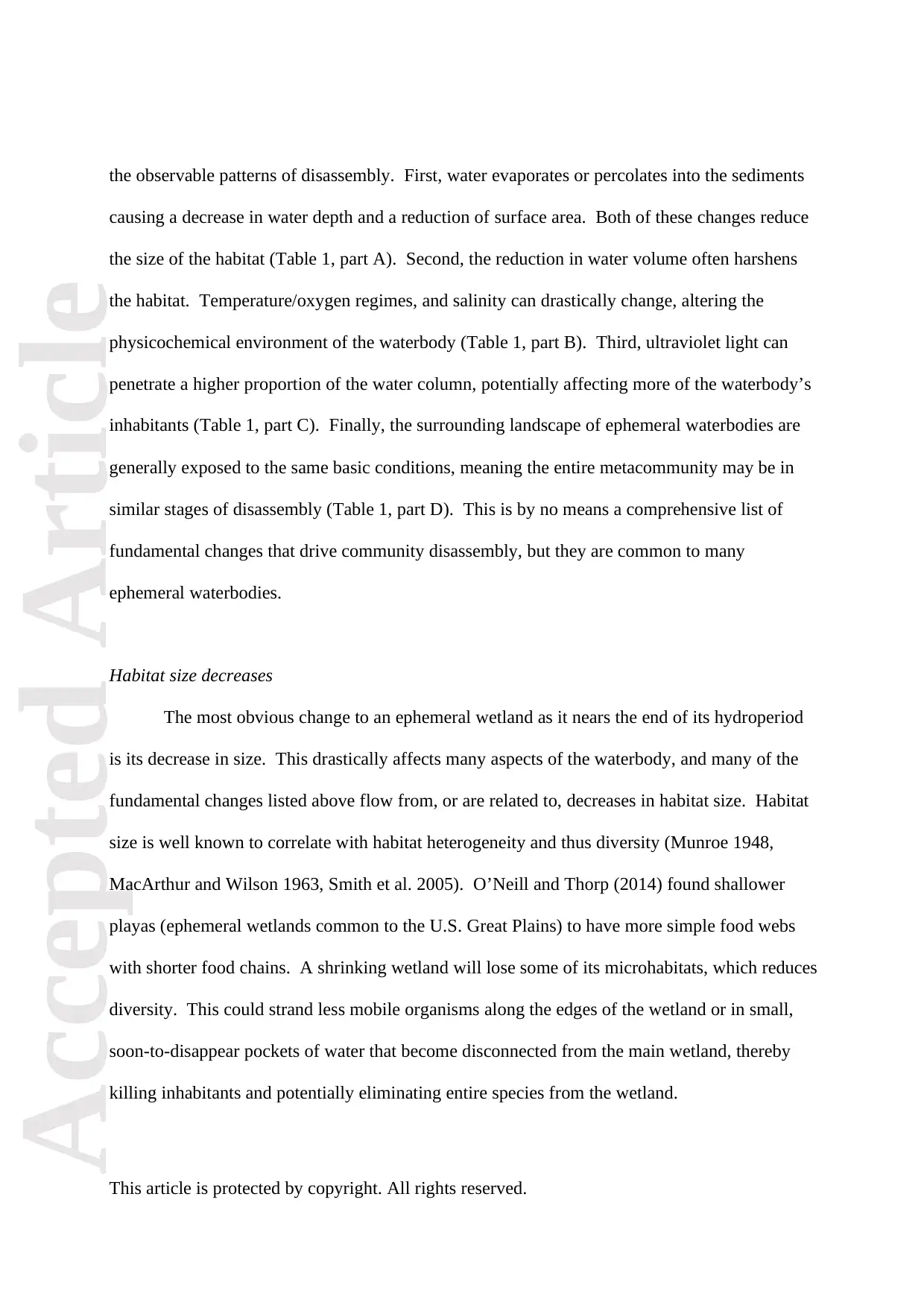
Accepted ArticleAccepted Article
This article is protected by copyright. All rights reserved.
the observable patterns of disassembly. First, water evaporates or percolates into the sediments
causing a decrease in water depth and a reduction of surface area. Both of these changes reduce
the size of the habitat (Table 1, part A). Second, the reduction in water volume often harshens
the habitat. Temperature/oxygen regimes, and salinity can drastically change, altering the
physicochemical environment of the waterbody (Table 1, part B). Third, ultraviolet light can
penetrate a higher proportion of the water column, potentially affecting more of the waterbody’s
inhabitants (Table 1, part C). Finally, the surrounding landscape of ephemeral waterbodies are
generally exposed to the same basic conditions, meaning the entire metacommunity may be in
similar stages of disassembly (Table 1, part D). This is by no means a comprehensive list of
fundamental changes that drive community disassembly, but they are common to many
ephemeral waterbodies.
Habitat size decreases
The most obvious change to an ephemeral wetland as it nears the end of its hydroperiod
is its decrease in size. This drastically affects many aspects of the waterbody, and many of the
fundamental changes listed above flow from, or are related to, decreases in habitat size. Habitat
size is well known to correlate with habitat heterogeneity and thus diversity (Munroe 1948,
MacArthur and Wilson 1963, Smith et al. 2005). O’Neill and Thorp (2014) found shallower
playas (ephemeral wetlands common to the U.S. Great Plains) to have more simple food webs
with shorter food chains. A shrinking wetland will lose some of its microhabitats, which reduces
diversity. This could strand less mobile organisms along the edges of the wetland or in small,
soon-to-disappear pockets of water that become disconnected from the main wetland, thereby
killing inhabitants and potentially eliminating entire species from the wetland.
This article is protected by copyright. All rights reserved.
the observable patterns of disassembly. First, water evaporates or percolates into the sediments
causing a decrease in water depth and a reduction of surface area. Both of these changes reduce
the size of the habitat (Table 1, part A). Second, the reduction in water volume often harshens
the habitat. Temperature/oxygen regimes, and salinity can drastically change, altering the
physicochemical environment of the waterbody (Table 1, part B). Third, ultraviolet light can
penetrate a higher proportion of the water column, potentially affecting more of the waterbody’s
inhabitants (Table 1, part C). Finally, the surrounding landscape of ephemeral waterbodies are
generally exposed to the same basic conditions, meaning the entire metacommunity may be in
similar stages of disassembly (Table 1, part D). This is by no means a comprehensive list of
fundamental changes that drive community disassembly, but they are common to many
ephemeral waterbodies.
Habitat size decreases
The most obvious change to an ephemeral wetland as it nears the end of its hydroperiod
is its decrease in size. This drastically affects many aspects of the waterbody, and many of the
fundamental changes listed above flow from, or are related to, decreases in habitat size. Habitat
size is well known to correlate with habitat heterogeneity and thus diversity (Munroe 1948,
MacArthur and Wilson 1963, Smith et al. 2005). O’Neill and Thorp (2014) found shallower
playas (ephemeral wetlands common to the U.S. Great Plains) to have more simple food webs
with shorter food chains. A shrinking wetland will lose some of its microhabitats, which reduces
diversity. This could strand less mobile organisms along the edges of the wetland or in small,
soon-to-disappear pockets of water that become disconnected from the main wetland, thereby
killing inhabitants and potentially eliminating entire species from the wetland.
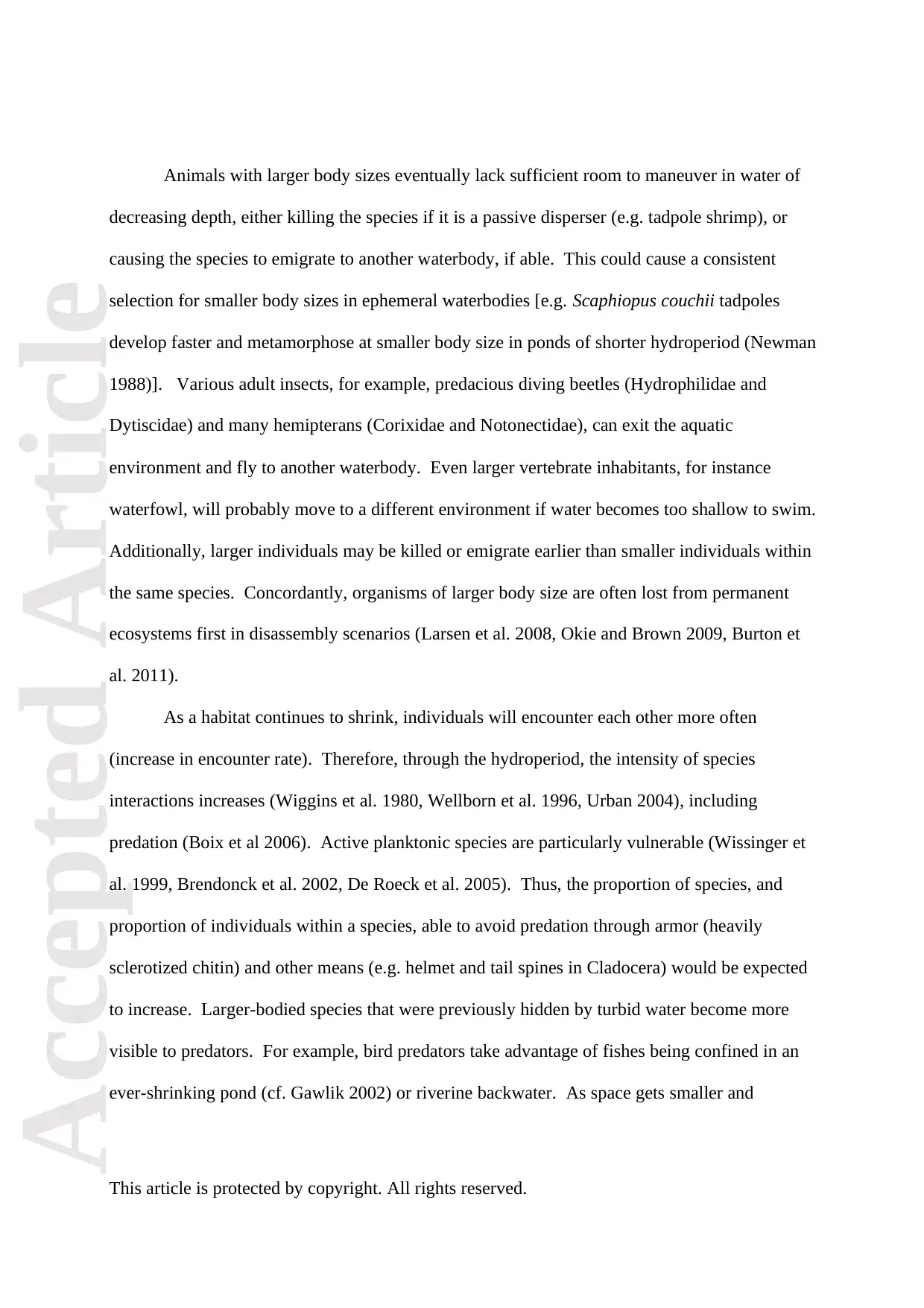
Accepted ArticleAccepted Article
This article is protected by copyright. All rights reserved.
Animals with larger body sizes eventually lack sufficient room to maneuver in water of
decreasing depth, either killing the species if it is a passive disperser (e.g. tadpole shrimp), or
causing the species to emigrate to another waterbody, if able. This could cause a consistent
selection for smaller body sizes in ephemeral waterbodies [e.g. Scaphiopus couchii tadpoles
develop faster and metamorphose at smaller body size in ponds of shorter hydroperiod (Newman
1988)]. Various adult insects, for example, predacious diving beetles (Hydrophilidae and
Dytiscidae) and many hemipterans (Corixidae and Notonectidae), can exit the aquatic
environment and fly to another waterbody. Even larger vertebrate inhabitants, for instance
waterfowl, will probably move to a different environment if water becomes too shallow to swim.
Additionally, larger individuals may be killed or emigrate earlier than smaller individuals within
the same species. Concordantly, organisms of larger body size are often lost from permanent
ecosystems first in disassembly scenarios (Larsen et al. 2008, Okie and Brown 2009, Burton et
al. 2011).
As a habitat continues to shrink, individuals will encounter each other more often
(increase in encounter rate). Therefore, through the hydroperiod, the intensity of species
interactions increases (Wiggins et al. 1980, Wellborn et al. 1996, Urban 2004), including
predation (Boix et al 2006). Active planktonic species are particularly vulnerable (Wissinger et
al. 1999, Brendonck et al. 2002, De Roeck et al. 2005). Thus, the proportion of species, and
proportion of individuals within a species, able to avoid predation through armor (heavily
sclerotized chitin) and other means (e.g. helmet and tail spines in Cladocera) would be expected
to increase. Larger-bodied species that were previously hidden by turbid water become more
visible to predators. For example, bird predators take advantage of fishes being confined in an
ever-shrinking pond (cf. Gawlik 2002) or riverine backwater. As space gets smaller and
This article is protected by copyright. All rights reserved.
Animals with larger body sizes eventually lack sufficient room to maneuver in water of
decreasing depth, either killing the species if it is a passive disperser (e.g. tadpole shrimp), or
causing the species to emigrate to another waterbody, if able. This could cause a consistent
selection for smaller body sizes in ephemeral waterbodies [e.g. Scaphiopus couchii tadpoles
develop faster and metamorphose at smaller body size in ponds of shorter hydroperiod (Newman
1988)]. Various adult insects, for example, predacious diving beetles (Hydrophilidae and
Dytiscidae) and many hemipterans (Corixidae and Notonectidae), can exit the aquatic
environment and fly to another waterbody. Even larger vertebrate inhabitants, for instance
waterfowl, will probably move to a different environment if water becomes too shallow to swim.
Additionally, larger individuals may be killed or emigrate earlier than smaller individuals within
the same species. Concordantly, organisms of larger body size are often lost from permanent
ecosystems first in disassembly scenarios (Larsen et al. 2008, Okie and Brown 2009, Burton et
al. 2011).
As a habitat continues to shrink, individuals will encounter each other more often
(increase in encounter rate). Therefore, through the hydroperiod, the intensity of species
interactions increases (Wiggins et al. 1980, Wellborn et al. 1996, Urban 2004), including
predation (Boix et al 2006). Active planktonic species are particularly vulnerable (Wissinger et
al. 1999, Brendonck et al. 2002, De Roeck et al. 2005). Thus, the proportion of species, and
proportion of individuals within a species, able to avoid predation through armor (heavily
sclerotized chitin) and other means (e.g. helmet and tail spines in Cladocera) would be expected
to increase. Larger-bodied species that were previously hidden by turbid water become more
visible to predators. For example, bird predators take advantage of fishes being confined in an
ever-shrinking pond (cf. Gawlik 2002) or riverine backwater. As space gets smaller and
Paraphrase This Document
Need a fresh take? Get an instant paraphrase of this document with our AI Paraphraser
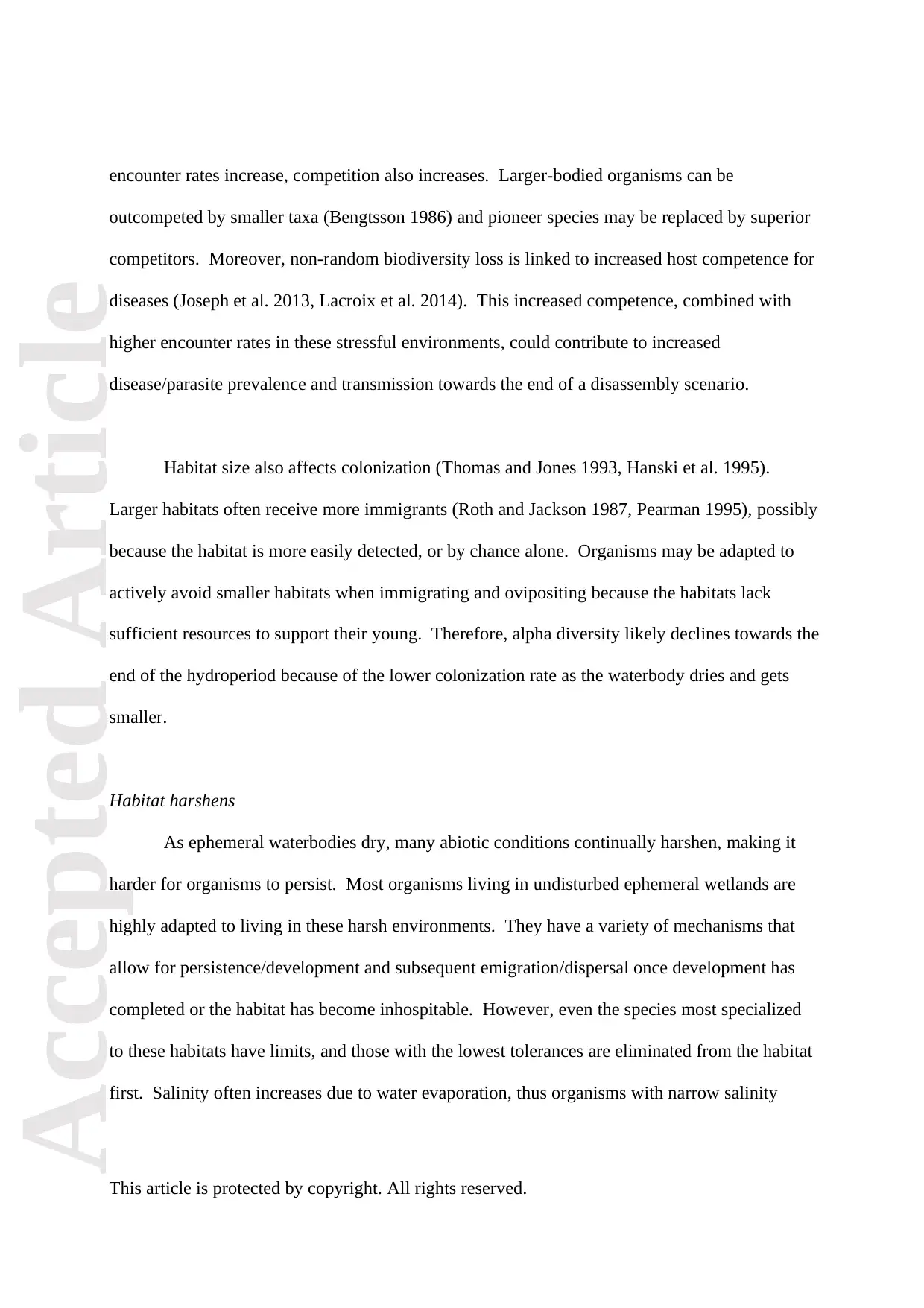
Accepted ArticleAccepted Article
This article is protected by copyright. All rights reserved.
encounter rates increase, competition also increases. Larger-bodied organisms can be
outcompeted by smaller taxa (Bengtsson 1986) and pioneer species may be replaced by superior
competitors. Moreover, non-random biodiversity loss is linked to increased host competence for
diseases (Joseph et al. 2013, Lacroix et al. 2014). This increased competence, combined with
higher encounter rates in these stressful environments, could contribute to increased
disease/parasite prevalence and transmission towards the end of a disassembly scenario.
Habitat size also affects colonization (Thomas and Jones 1993, Hanski et al. 1995).
Larger habitats often receive more immigrants (Roth and Jackson 1987, Pearman 1995), possibly
because the habitat is more easily detected, or by chance alone. Organisms may be adapted to
actively avoid smaller habitats when immigrating and ovipositing because the habitats lack
sufficient resources to support their young. Therefore, alpha diversity likely declines towards the
end of the hydroperiod because of the lower colonization rate as the waterbody dries and gets
smaller.
Habitat harshens
As ephemeral waterbodies dry, many abiotic conditions continually harshen, making it
harder for organisms to persist. Most organisms living in undisturbed ephemeral wetlands are
highly adapted to living in these harsh environments. They have a variety of mechanisms that
allow for persistence/development and subsequent emigration/dispersal once development has
completed or the habitat has become inhospitable. However, even the species most specialized
to these habitats have limits, and those with the lowest tolerances are eliminated from the habitat
first. Salinity often increases due to water evaporation, thus organisms with narrow salinity
This article is protected by copyright. All rights reserved.
encounter rates increase, competition also increases. Larger-bodied organisms can be
outcompeted by smaller taxa (Bengtsson 1986) and pioneer species may be replaced by superior
competitors. Moreover, non-random biodiversity loss is linked to increased host competence for
diseases (Joseph et al. 2013, Lacroix et al. 2014). This increased competence, combined with
higher encounter rates in these stressful environments, could contribute to increased
disease/parasite prevalence and transmission towards the end of a disassembly scenario.
Habitat size also affects colonization (Thomas and Jones 1993, Hanski et al. 1995).
Larger habitats often receive more immigrants (Roth and Jackson 1987, Pearman 1995), possibly
because the habitat is more easily detected, or by chance alone. Organisms may be adapted to
actively avoid smaller habitats when immigrating and ovipositing because the habitats lack
sufficient resources to support their young. Therefore, alpha diversity likely declines towards the
end of the hydroperiod because of the lower colonization rate as the waterbody dries and gets
smaller.
Habitat harshens
As ephemeral waterbodies dry, many abiotic conditions continually harshen, making it
harder for organisms to persist. Most organisms living in undisturbed ephemeral wetlands are
highly adapted to living in these harsh environments. They have a variety of mechanisms that
allow for persistence/development and subsequent emigration/dispersal once development has
completed or the habitat has become inhospitable. However, even the species most specialized
to these habitats have limits, and those with the lowest tolerances are eliminated from the habitat
first. Salinity often increases due to water evaporation, thus organisms with narrow salinity
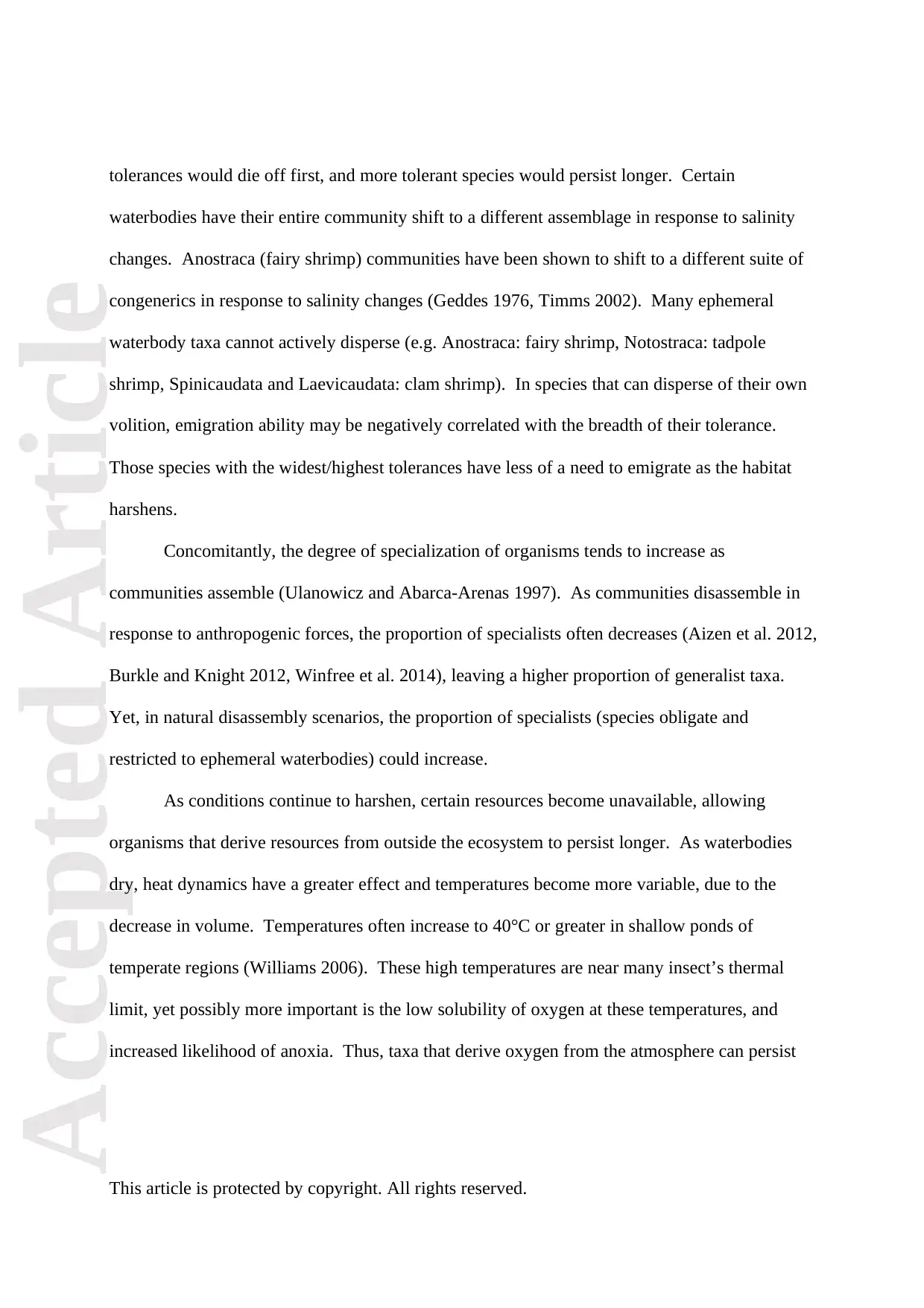
Accepted ArticleAccepted Article
This article is protected by copyright. All rights reserved.
tolerances would die off first, and more tolerant species would persist longer. Certain
waterbodies have their entire community shift to a different assemblage in response to salinity
changes. Anostraca (fairy shrimp) communities have been shown to shift to a different suite of
congenerics in response to salinity changes (Geddes 1976, Timms 2002). Many ephemeral
waterbody taxa cannot actively disperse (e.g. Anostraca: fairy shrimp, Notostraca: tadpole
shrimp, Spinicaudata and Laevicaudata: clam shrimp). In species that can disperse of their own
volition, emigration ability may be negatively correlated with the breadth of their tolerance.
Those species with the widest/highest tolerances have less of a need to emigrate as the habitat
harshens.
Concomitantly, the degree of specialization of organisms tends to increase as
communities assemble (Ulanowicz and Abarca-Arenas 1997). As communities disassemble in
response to anthropogenic forces, the proportion of specialists often decreases (Aizen et al. 2012,
Burkle and Knight 2012, Winfree et al. 2014), leaving a higher proportion of generalist taxa.
Yet, in natural disassembly scenarios, the proportion of specialists (species obligate and
restricted to ephemeral waterbodies) could increase.
As conditions continue to harshen, certain resources become unavailable, allowing
organisms that derive resources from outside the ecosystem to persist longer. As waterbodies
dry, heat dynamics have a greater effect and temperatures become more variable, due to the
decrease in volume. Temperatures often increase to 40°C or greater in shallow ponds of
temperate regions (Williams 2006). These high temperatures are near many insect’s thermal
limit, yet possibly more important is the low solubility of oxygen at these temperatures, and
increased likelihood of anoxia. Thus, taxa that derive oxygen from the atmosphere can persist
This article is protected by copyright. All rights reserved.
tolerances would die off first, and more tolerant species would persist longer. Certain
waterbodies have their entire community shift to a different assemblage in response to salinity
changes. Anostraca (fairy shrimp) communities have been shown to shift to a different suite of
congenerics in response to salinity changes (Geddes 1976, Timms 2002). Many ephemeral
waterbody taxa cannot actively disperse (e.g. Anostraca: fairy shrimp, Notostraca: tadpole
shrimp, Spinicaudata and Laevicaudata: clam shrimp). In species that can disperse of their own
volition, emigration ability may be negatively correlated with the breadth of their tolerance.
Those species with the widest/highest tolerances have less of a need to emigrate as the habitat
harshens.
Concomitantly, the degree of specialization of organisms tends to increase as
communities assemble (Ulanowicz and Abarca-Arenas 1997). As communities disassemble in
response to anthropogenic forces, the proportion of specialists often decreases (Aizen et al. 2012,
Burkle and Knight 2012, Winfree et al. 2014), leaving a higher proportion of generalist taxa.
Yet, in natural disassembly scenarios, the proportion of specialists (species obligate and
restricted to ephemeral waterbodies) could increase.
As conditions continue to harshen, certain resources become unavailable, allowing
organisms that derive resources from outside the ecosystem to persist longer. As waterbodies
dry, heat dynamics have a greater effect and temperatures become more variable, due to the
decrease in volume. Temperatures often increase to 40°C or greater in shallow ponds of
temperate regions (Williams 2006). These high temperatures are near many insect’s thermal
limit, yet possibly more important is the low solubility of oxygen at these temperatures, and
increased likelihood of anoxia. Thus, taxa that derive oxygen from the atmosphere can persist
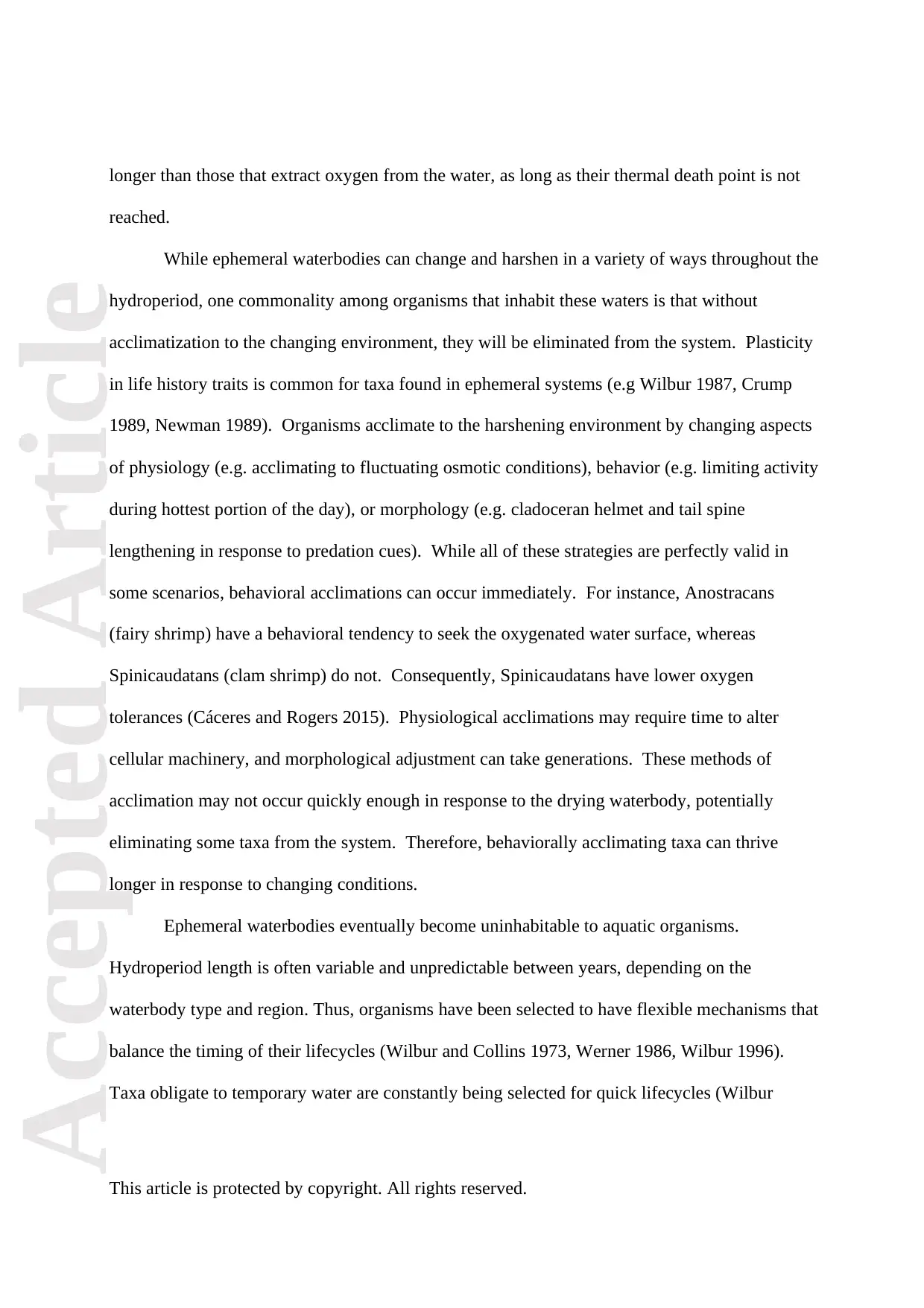
Accepted ArticleAccepted Article
This article is protected by copyright. All rights reserved.
longer than those that extract oxygen from the water, as long as their thermal death point is not
reached.
While ephemeral waterbodies can change and harshen in a variety of ways throughout the
hydroperiod, one commonality among organisms that inhabit these waters is that without
acclimatization to the changing environment, they will be eliminated from the system. Plasticity
in life history traits is common for taxa found in ephemeral systems (e.g Wilbur 1987, Crump
1989, Newman 1989). Organisms acclimate to the harshening environment by changing aspects
of physiology (e.g. acclimating to fluctuating osmotic conditions), behavior (e.g. limiting activity
during hottest portion of the day), or morphology (e.g. cladoceran helmet and tail spine
lengthening in response to predation cues). While all of these strategies are perfectly valid in
some scenarios, behavioral acclimations can occur immediately. For instance, Anostracans
(fairy shrimp) have a behavioral tendency to seek the oxygenated water surface, whereas
Spinicaudatans (clam shrimp) do not. Consequently, Spinicaudatans have lower oxygen
tolerances (Cáceres and Rogers 2015). Physiological acclimations may require time to alter
cellular machinery, and morphological adjustment can take generations. These methods of
acclimation may not occur quickly enough in response to the drying waterbody, potentially
eliminating some taxa from the system. Therefore, behaviorally acclimating taxa can thrive
longer in response to changing conditions.
Ephemeral waterbodies eventually become uninhabitable to aquatic organisms.
Hydroperiod length is often variable and unpredictable between years, depending on the
waterbody type and region. Thus, organisms have been selected to have flexible mechanisms that
balance the timing of their lifecycles (Wilbur and Collins 1973, Werner 1986, Wilbur 1996).
Taxa obligate to temporary water are constantly being selected for quick lifecycles (Wilbur
This article is protected by copyright. All rights reserved.
longer than those that extract oxygen from the water, as long as their thermal death point is not
reached.
While ephemeral waterbodies can change and harshen in a variety of ways throughout the
hydroperiod, one commonality among organisms that inhabit these waters is that without
acclimatization to the changing environment, they will be eliminated from the system. Plasticity
in life history traits is common for taxa found in ephemeral systems (e.g Wilbur 1987, Crump
1989, Newman 1989). Organisms acclimate to the harshening environment by changing aspects
of physiology (e.g. acclimating to fluctuating osmotic conditions), behavior (e.g. limiting activity
during hottest portion of the day), or morphology (e.g. cladoceran helmet and tail spine
lengthening in response to predation cues). While all of these strategies are perfectly valid in
some scenarios, behavioral acclimations can occur immediately. For instance, Anostracans
(fairy shrimp) have a behavioral tendency to seek the oxygenated water surface, whereas
Spinicaudatans (clam shrimp) do not. Consequently, Spinicaudatans have lower oxygen
tolerances (Cáceres and Rogers 2015). Physiological acclimations may require time to alter
cellular machinery, and morphological adjustment can take generations. These methods of
acclimation may not occur quickly enough in response to the drying waterbody, potentially
eliminating some taxa from the system. Therefore, behaviorally acclimating taxa can thrive
longer in response to changing conditions.
Ephemeral waterbodies eventually become uninhabitable to aquatic organisms.
Hydroperiod length is often variable and unpredictable between years, depending on the
waterbody type and region. Thus, organisms have been selected to have flexible mechanisms that
balance the timing of their lifecycles (Wilbur and Collins 1973, Werner 1986, Wilbur 1996).
Taxa obligate to temporary water are constantly being selected for quick lifecycles (Wilbur
Secure Best Marks with AI Grader
Need help grading? Try our AI Grader for instant feedback on your assignments.
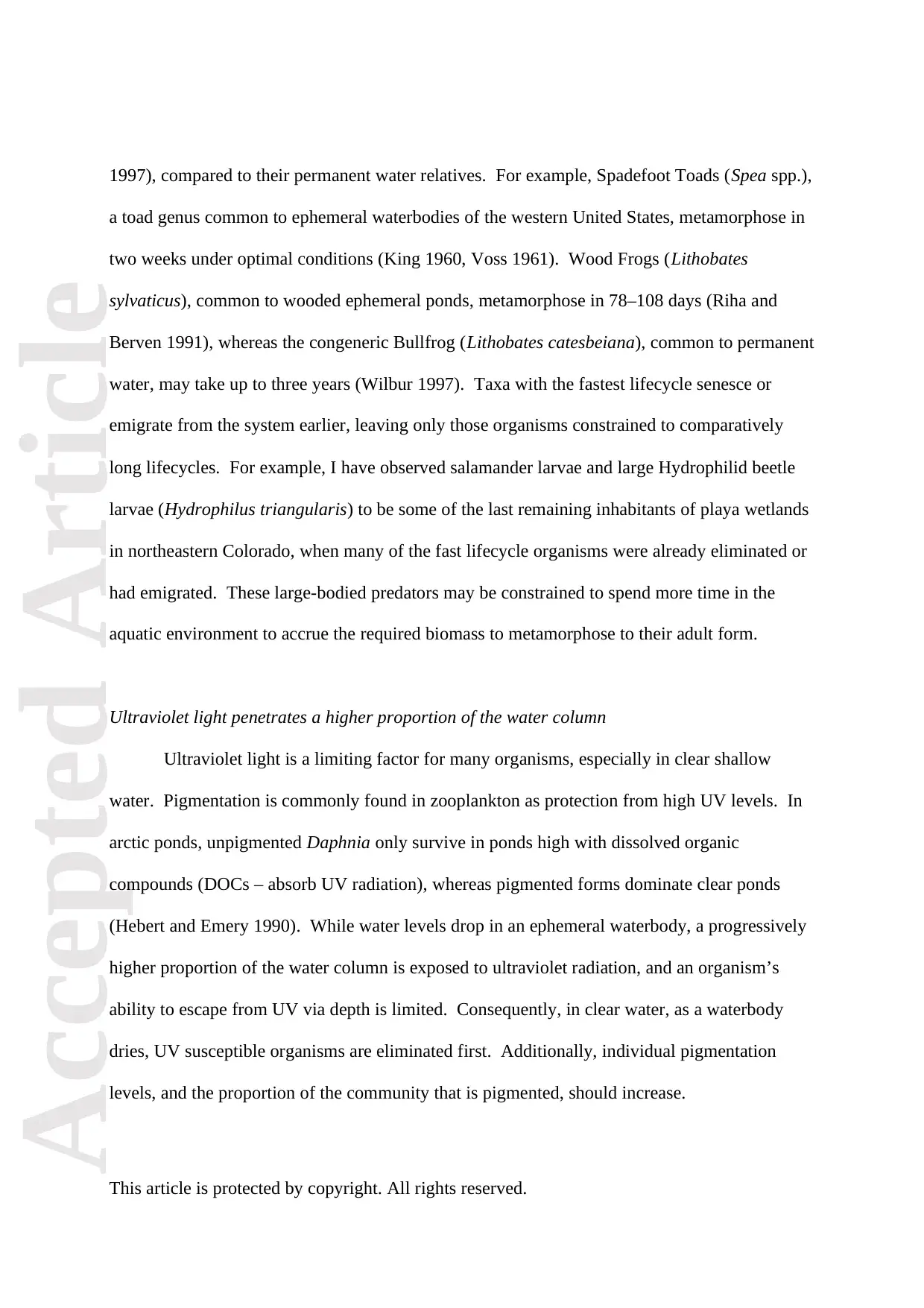
Accepted ArticleAccepted Article
This article is protected by copyright. All rights reserved.
1997), compared to their permanent water relatives. For example, Spadefoot Toads (Spea spp.),
a toad genus common to ephemeral waterbodies of the western United States, metamorphose in
two weeks under optimal conditions (King 1960, Voss 1961). Wood Frogs (Lithobates
sylvaticus), common to wooded ephemeral ponds, metamorphose in 78–108 days (Riha and
Berven 1991), whereas the congeneric Bullfrog (Lithobates catesbeiana), common to permanent
water, may take up to three years (Wilbur 1997). Taxa with the fastest lifecycle senesce or
emigrate from the system earlier, leaving only those organisms constrained to comparatively
long lifecycles. For example, I have observed salamander larvae and large Hydrophilid beetle
larvae (Hydrophilus triangularis) to be some of the last remaining inhabitants of playa wetlands
in northeastern Colorado, when many of the fast lifecycle organisms were already eliminated or
had emigrated. These large-bodied predators may be constrained to spend more time in the
aquatic environment to accrue the required biomass to metamorphose to their adult form.
Ultraviolet light penetrates a higher proportion of the water column
Ultraviolet light is a limiting factor for many organisms, especially in clear shallow
water. Pigmentation is commonly found in zooplankton as protection from high UV levels. In
arctic ponds, unpigmented Daphnia only survive in ponds high with dissolved organic
compounds (DOCs – absorb UV radiation), whereas pigmented forms dominate clear ponds
(Hebert and Emery 1990). While water levels drop in an ephemeral waterbody, a progressively
higher proportion of the water column is exposed to ultraviolet radiation, and an organism’s
ability to escape from UV via depth is limited. Consequently, in clear water, as a waterbody
dries, UV susceptible organisms are eliminated first. Additionally, individual pigmentation
levels, and the proportion of the community that is pigmented, should increase.
This article is protected by copyright. All rights reserved.
1997), compared to their permanent water relatives. For example, Spadefoot Toads (Spea spp.),
a toad genus common to ephemeral waterbodies of the western United States, metamorphose in
two weeks under optimal conditions (King 1960, Voss 1961). Wood Frogs (Lithobates
sylvaticus), common to wooded ephemeral ponds, metamorphose in 78–108 days (Riha and
Berven 1991), whereas the congeneric Bullfrog (Lithobates catesbeiana), common to permanent
water, may take up to three years (Wilbur 1997). Taxa with the fastest lifecycle senesce or
emigrate from the system earlier, leaving only those organisms constrained to comparatively
long lifecycles. For example, I have observed salamander larvae and large Hydrophilid beetle
larvae (Hydrophilus triangularis) to be some of the last remaining inhabitants of playa wetlands
in northeastern Colorado, when many of the fast lifecycle organisms were already eliminated or
had emigrated. These large-bodied predators may be constrained to spend more time in the
aquatic environment to accrue the required biomass to metamorphose to their adult form.
Ultraviolet light penetrates a higher proportion of the water column
Ultraviolet light is a limiting factor for many organisms, especially in clear shallow
water. Pigmentation is commonly found in zooplankton as protection from high UV levels. In
arctic ponds, unpigmented Daphnia only survive in ponds high with dissolved organic
compounds (DOCs – absorb UV radiation), whereas pigmented forms dominate clear ponds
(Hebert and Emery 1990). While water levels drop in an ephemeral waterbody, a progressively
higher proportion of the water column is exposed to ultraviolet radiation, and an organism’s
ability to escape from UV via depth is limited. Consequently, in clear water, as a waterbody
dries, UV susceptible organisms are eliminated first. Additionally, individual pigmentation
levels, and the proportion of the community that is pigmented, should increase.
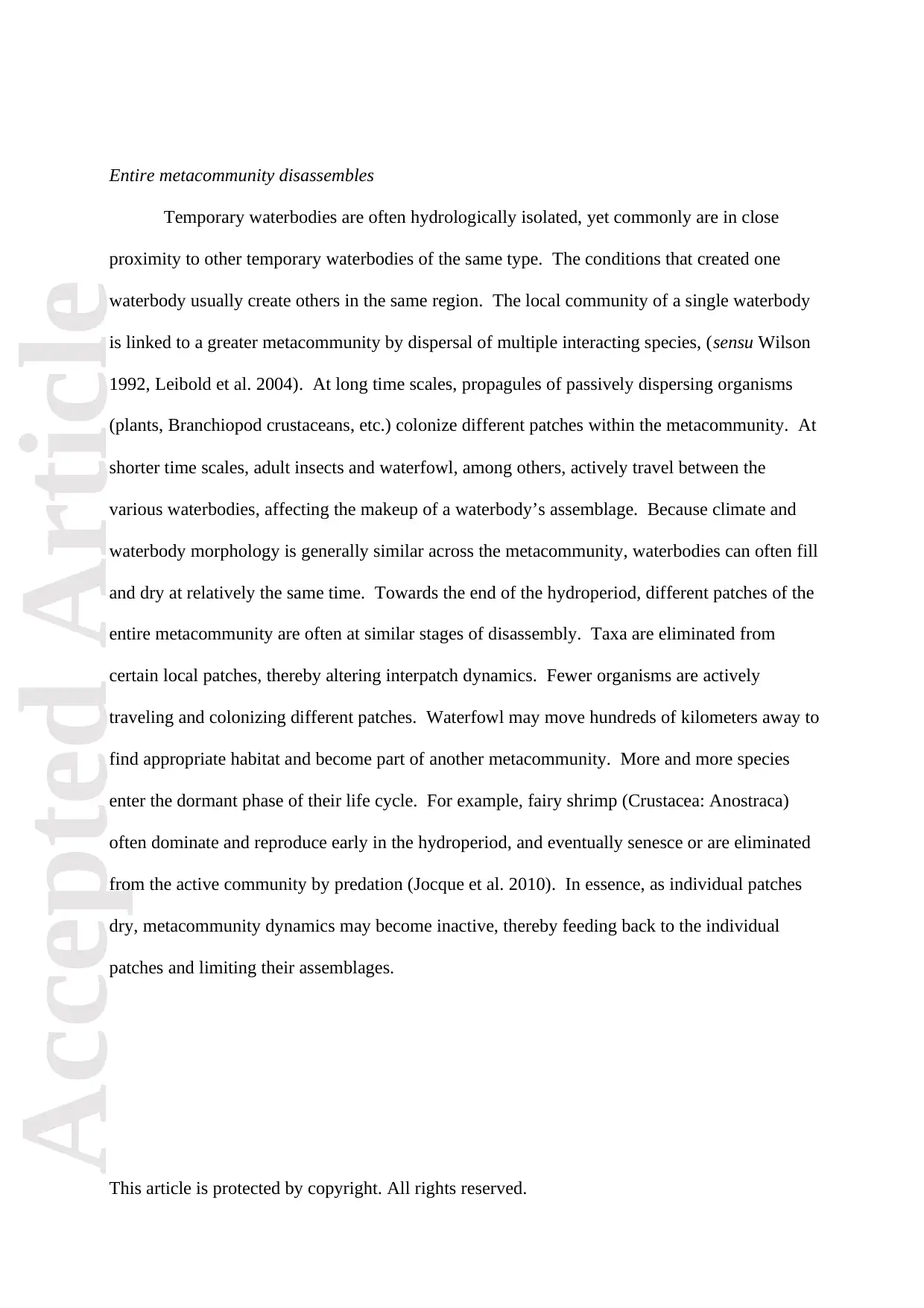
Accepted ArticleAccepted Article
This article is protected by copyright. All rights reserved.
Entire metacommunity disassembles
Temporary waterbodies are often hydrologically isolated, yet commonly are in close
proximity to other temporary waterbodies of the same type. The conditions that created one
waterbody usually create others in the same region. The local community of a single waterbody
is linked to a greater metacommunity by dispersal of multiple interacting species, (sensu Wilson
1992, Leibold et al. 2004). At long time scales, propagules of passively dispersing organisms
(plants, Branchiopod crustaceans, etc.) colonize different patches within the metacommunity. At
shorter time scales, adult insects and waterfowl, among others, actively travel between the
various waterbodies, affecting the makeup of a waterbody’s assemblage. Because climate and
waterbody morphology is generally similar across the metacommunity, waterbodies can often fill
and dry at relatively the same time. Towards the end of the hydroperiod, different patches of the
entire metacommunity are often at similar stages of disassembly. Taxa are eliminated from
certain local patches, thereby altering interpatch dynamics. Fewer organisms are actively
traveling and colonizing different patches. Waterfowl may move hundreds of kilometers away to
find appropriate habitat and become part of another metacommunity. More and more species
enter the dormant phase of their life cycle. For example, fairy shrimp (Crustacea: Anostraca)
often dominate and reproduce early in the hydroperiod, and eventually senesce or are eliminated
from the active community by predation (Jocque et al. 2010). In essence, as individual patches
dry, metacommunity dynamics may become inactive, thereby feeding back to the individual
patches and limiting their assemblages.
This article is protected by copyright. All rights reserved.
Entire metacommunity disassembles
Temporary waterbodies are often hydrologically isolated, yet commonly are in close
proximity to other temporary waterbodies of the same type. The conditions that created one
waterbody usually create others in the same region. The local community of a single waterbody
is linked to a greater metacommunity by dispersal of multiple interacting species, (sensu Wilson
1992, Leibold et al. 2004). At long time scales, propagules of passively dispersing organisms
(plants, Branchiopod crustaceans, etc.) colonize different patches within the metacommunity. At
shorter time scales, adult insects and waterfowl, among others, actively travel between the
various waterbodies, affecting the makeup of a waterbody’s assemblage. Because climate and
waterbody morphology is generally similar across the metacommunity, waterbodies can often fill
and dry at relatively the same time. Towards the end of the hydroperiod, different patches of the
entire metacommunity are often at similar stages of disassembly. Taxa are eliminated from
certain local patches, thereby altering interpatch dynamics. Fewer organisms are actively
traveling and colonizing different patches. Waterfowl may move hundreds of kilometers away to
find appropriate habitat and become part of another metacommunity. More and more species
enter the dormant phase of their life cycle. For example, fairy shrimp (Crustacea: Anostraca)
often dominate and reproduce early in the hydroperiod, and eventually senesce or are eliminated
from the active community by predation (Jocque et al. 2010). In essence, as individual patches
dry, metacommunity dynamics may become inactive, thereby feeding back to the individual
patches and limiting their assemblages.
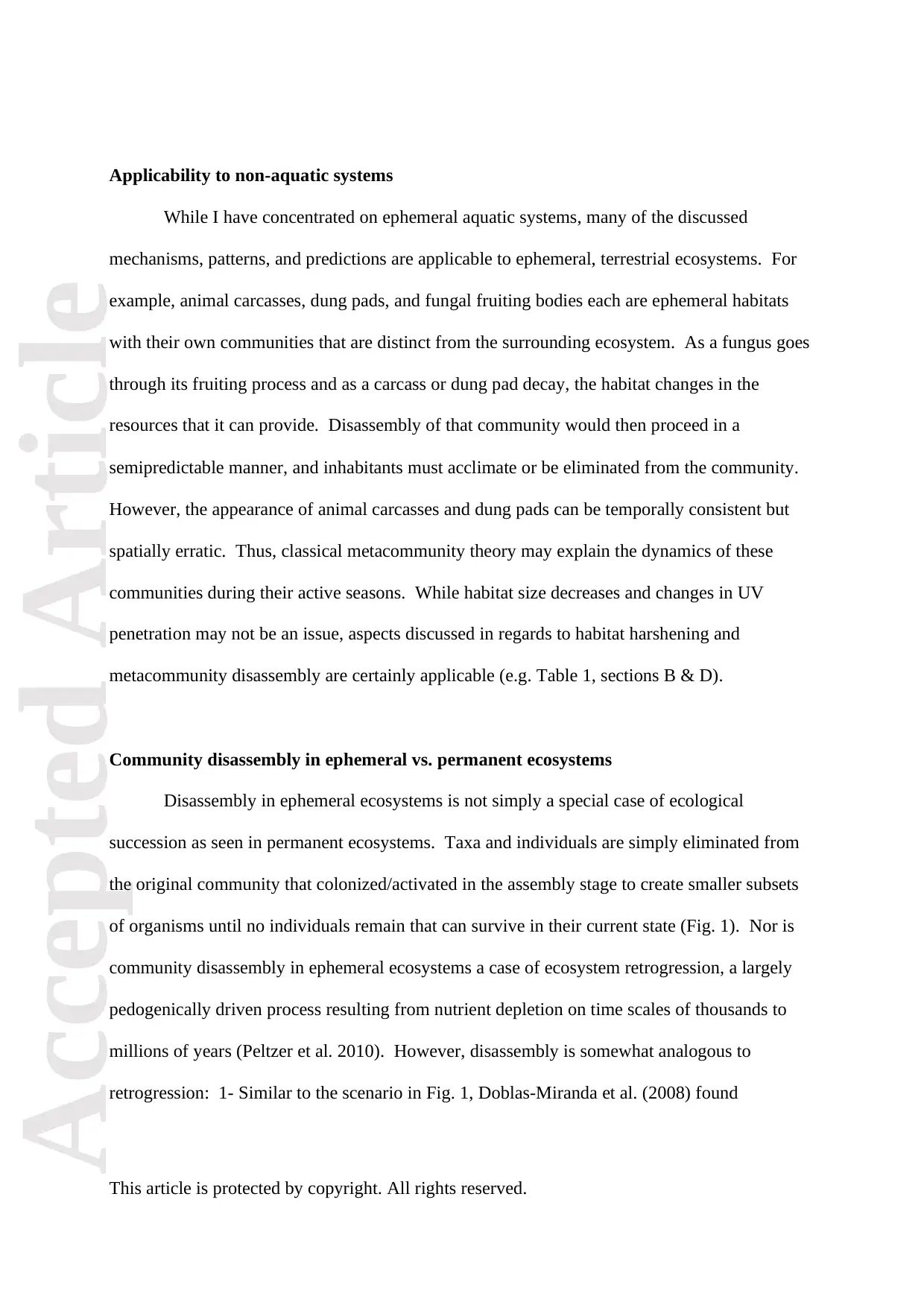
Accepted ArticleAccepted Article
This article is protected by copyright. All rights reserved.
Applicability to non-aquatic systems
While I have concentrated on ephemeral aquatic systems, many of the discussed
mechanisms, patterns, and predictions are applicable to ephemeral, terrestrial ecosystems. For
example, animal carcasses, dung pads, and fungal fruiting bodies each are ephemeral habitats
with their own communities that are distinct from the surrounding ecosystem. As a fungus goes
through its fruiting process and as a carcass or dung pad decay, the habitat changes in the
resources that it can provide. Disassembly of that community would then proceed in a
semipredictable manner, and inhabitants must acclimate or be eliminated from the community.
However, the appearance of animal carcasses and dung pads can be temporally consistent but
spatially erratic. Thus, classical metacommunity theory may explain the dynamics of these
communities during their active seasons. While habitat size decreases and changes in UV
penetration may not be an issue, aspects discussed in regards to habitat harshening and
metacommunity disassembly are certainly applicable (e.g. Table 1, sections B & D).
Community disassembly in ephemeral vs. permanent ecosystems
Disassembly in ephemeral ecosystems is not simply a special case of ecological
succession as seen in permanent ecosystems. Taxa and individuals are simply eliminated from
the original community that colonized/activated in the assembly stage to create smaller subsets
of organisms until no individuals remain that can survive in their current state (Fig. 1). Nor is
community disassembly in ephemeral ecosystems a case of ecosystem retrogression, a largely
pedogenically driven process resulting from nutrient depletion on time scales of thousands to
millions of years (Peltzer et al. 2010). However, disassembly is somewhat analogous to
retrogression: 1- Similar to the scenario in Fig. 1, Doblas-Miranda et al. (2008) found
This article is protected by copyright. All rights reserved.
Applicability to non-aquatic systems
While I have concentrated on ephemeral aquatic systems, many of the discussed
mechanisms, patterns, and predictions are applicable to ephemeral, terrestrial ecosystems. For
example, animal carcasses, dung pads, and fungal fruiting bodies each are ephemeral habitats
with their own communities that are distinct from the surrounding ecosystem. As a fungus goes
through its fruiting process and as a carcass or dung pad decay, the habitat changes in the
resources that it can provide. Disassembly of that community would then proceed in a
semipredictable manner, and inhabitants must acclimate or be eliminated from the community.
However, the appearance of animal carcasses and dung pads can be temporally consistent but
spatially erratic. Thus, classical metacommunity theory may explain the dynamics of these
communities during their active seasons. While habitat size decreases and changes in UV
penetration may not be an issue, aspects discussed in regards to habitat harshening and
metacommunity disassembly are certainly applicable (e.g. Table 1, sections B & D).
Community disassembly in ephemeral vs. permanent ecosystems
Disassembly in ephemeral ecosystems is not simply a special case of ecological
succession as seen in permanent ecosystems. Taxa and individuals are simply eliminated from
the original community that colonized/activated in the assembly stage to create smaller subsets
of organisms until no individuals remain that can survive in their current state (Fig. 1). Nor is
community disassembly in ephemeral ecosystems a case of ecosystem retrogression, a largely
pedogenically driven process resulting from nutrient depletion on time scales of thousands to
millions of years (Peltzer et al. 2010). However, disassembly is somewhat analogous to
retrogression: 1- Similar to the scenario in Fig. 1, Doblas-Miranda et al. (2008) found
Paraphrase This Document
Need a fresh take? Get an instant paraphrase of this document with our AI Paraphraser
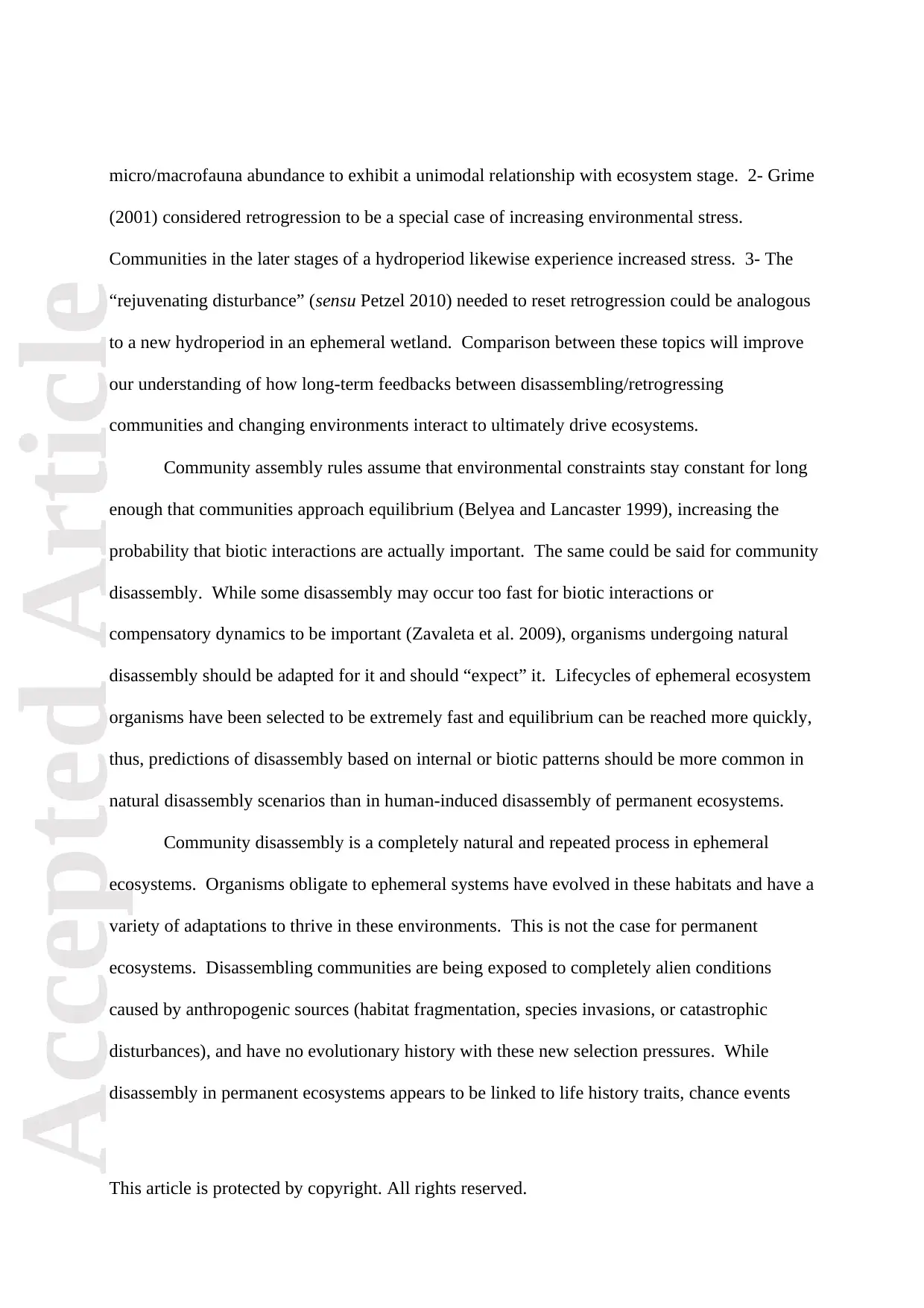
Accepted ArticleAccepted Article
This article is protected by copyright. All rights reserved.
micro/macrofauna abundance to exhibit a unimodal relationship with ecosystem stage. 2- Grime
(2001) considered retrogression to be a special case of increasing environmental stress.
Communities in the later stages of a hydroperiod likewise experience increased stress. 3- The
“rejuvenating disturbance” (sensu Petzel 2010) needed to reset retrogression could be analogous
to a new hydroperiod in an ephemeral wetland. Comparison between these topics will improve
our understanding of how long-term feedbacks between disassembling/retrogressing
communities and changing environments interact to ultimately drive ecosystems.
Community assembly rules assume that environmental constraints stay constant for long
enough that communities approach equilibrium (Belyea and Lancaster 1999), increasing the
probability that biotic interactions are actually important. The same could be said for community
disassembly. While some disassembly may occur too fast for biotic interactions or
compensatory dynamics to be important (Zavaleta et al. 2009), organisms undergoing natural
disassembly should be adapted for it and should “expect” it. Lifecycles of ephemeral ecosystem
organisms have been selected to be extremely fast and equilibrium can be reached more quickly,
thus, predictions of disassembly based on internal or biotic patterns should be more common in
natural disassembly scenarios than in human-induced disassembly of permanent ecosystems.
Community disassembly is a completely natural and repeated process in ephemeral
ecosystems. Organisms obligate to ephemeral systems have evolved in these habitats and have a
variety of adaptations to thrive in these environments. This is not the case for permanent
ecosystems. Disassembling communities are being exposed to completely alien conditions
caused by anthropogenic sources (habitat fragmentation, species invasions, or catastrophic
disturbances), and have no evolutionary history with these new selection pressures. While
disassembly in permanent ecosystems appears to be linked to life history traits, chance events
This article is protected by copyright. All rights reserved.
micro/macrofauna abundance to exhibit a unimodal relationship with ecosystem stage. 2- Grime
(2001) considered retrogression to be a special case of increasing environmental stress.
Communities in the later stages of a hydroperiod likewise experience increased stress. 3- The
“rejuvenating disturbance” (sensu Petzel 2010) needed to reset retrogression could be analogous
to a new hydroperiod in an ephemeral wetland. Comparison between these topics will improve
our understanding of how long-term feedbacks between disassembling/retrogressing
communities and changing environments interact to ultimately drive ecosystems.
Community assembly rules assume that environmental constraints stay constant for long
enough that communities approach equilibrium (Belyea and Lancaster 1999), increasing the
probability that biotic interactions are actually important. The same could be said for community
disassembly. While some disassembly may occur too fast for biotic interactions or
compensatory dynamics to be important (Zavaleta et al. 2009), organisms undergoing natural
disassembly should be adapted for it and should “expect” it. Lifecycles of ephemeral ecosystem
organisms have been selected to be extremely fast and equilibrium can be reached more quickly,
thus, predictions of disassembly based on internal or biotic patterns should be more common in
natural disassembly scenarios than in human-induced disassembly of permanent ecosystems.
Community disassembly is a completely natural and repeated process in ephemeral
ecosystems. Organisms obligate to ephemeral systems have evolved in these habitats and have a
variety of adaptations to thrive in these environments. This is not the case for permanent
ecosystems. Disassembling communities are being exposed to completely alien conditions
caused by anthropogenic sources (habitat fragmentation, species invasions, or catastrophic
disturbances), and have no evolutionary history with these new selection pressures. While
disassembly in permanent ecosystems appears to be linked to life history traits, chance events
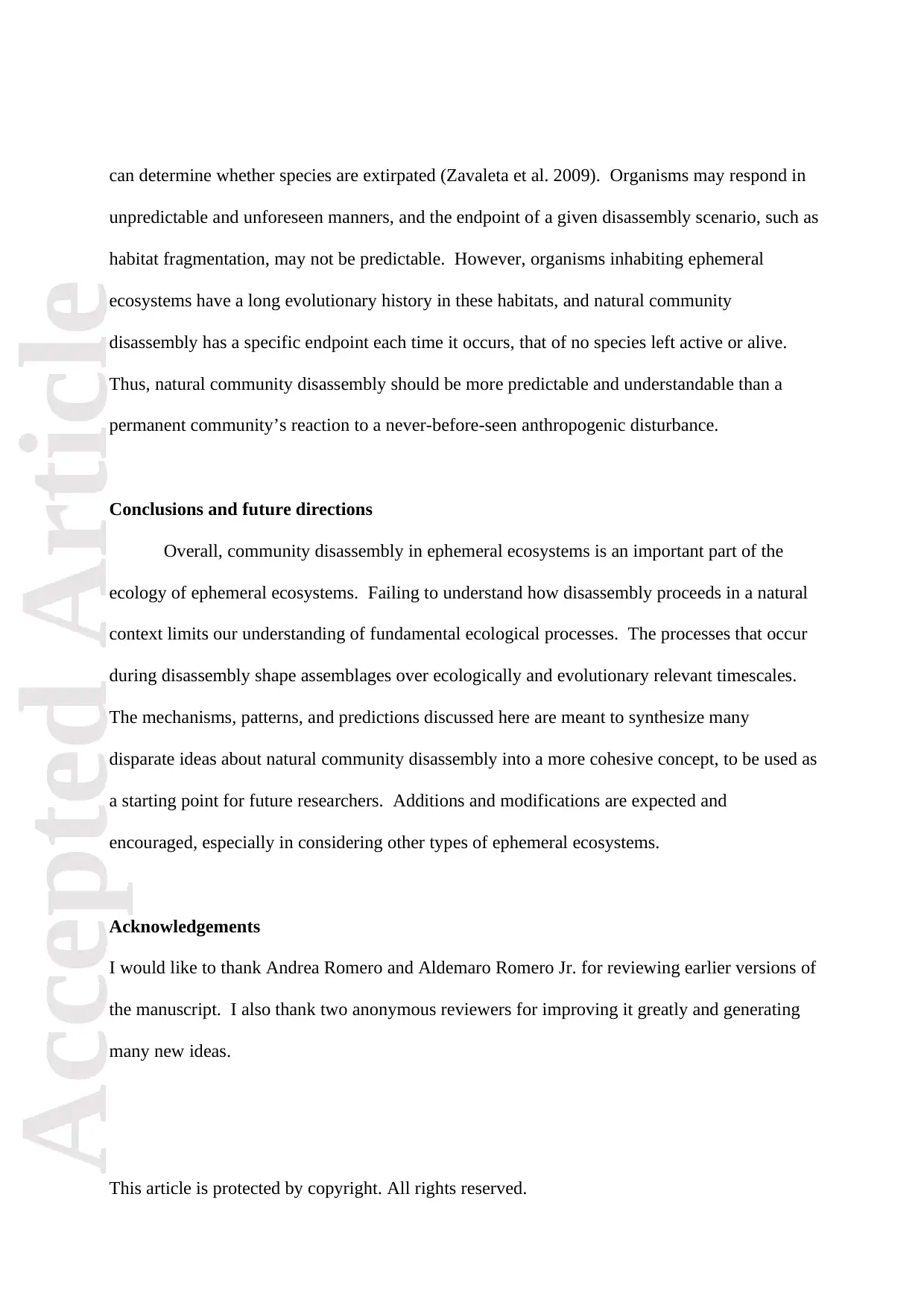
Accepted ArticleAccepted Article
This article is protected by copyright. All rights reserved.
can determine whether species are extirpated (Zavaleta et al. 2009). Organisms may respond in
unpredictable and unforeseen manners, and the endpoint of a given disassembly scenario, such as
habitat fragmentation, may not be predictable. However, organisms inhabiting ephemeral
ecosystems have a long evolutionary history in these habitats, and natural community
disassembly has a specific endpoint each time it occurs, that of no species left active or alive.
Thus, natural community disassembly should be more predictable and understandable than a
permanent community’s reaction to a never-before-seen anthropogenic disturbance.
Conclusions and future directions
Overall, community disassembly in ephemeral ecosystems is an important part of the
ecology of ephemeral ecosystems. Failing to understand how disassembly proceeds in a natural
context limits our understanding of fundamental ecological processes. The processes that occur
during disassembly shape assemblages over ecologically and evolutionary relevant timescales.
The mechanisms, patterns, and predictions discussed here are meant to synthesize many
disparate ideas about natural community disassembly into a more cohesive concept, to be used as
a starting point for future researchers. Additions and modifications are expected and
encouraged, especially in considering other types of ephemeral ecosystems.
Acknowledgements
I would like to thank Andrea Romero and Aldemaro Romero Jr. for reviewing earlier versions of
the manuscript. I also thank two anonymous reviewers for improving it greatly and generating
many new ideas.
This article is protected by copyright. All rights reserved.
can determine whether species are extirpated (Zavaleta et al. 2009). Organisms may respond in
unpredictable and unforeseen manners, and the endpoint of a given disassembly scenario, such as
habitat fragmentation, may not be predictable. However, organisms inhabiting ephemeral
ecosystems have a long evolutionary history in these habitats, and natural community
disassembly has a specific endpoint each time it occurs, that of no species left active or alive.
Thus, natural community disassembly should be more predictable and understandable than a
permanent community’s reaction to a never-before-seen anthropogenic disturbance.
Conclusions and future directions
Overall, community disassembly in ephemeral ecosystems is an important part of the
ecology of ephemeral ecosystems. Failing to understand how disassembly proceeds in a natural
context limits our understanding of fundamental ecological processes. The processes that occur
during disassembly shape assemblages over ecologically and evolutionary relevant timescales.
The mechanisms, patterns, and predictions discussed here are meant to synthesize many
disparate ideas about natural community disassembly into a more cohesive concept, to be used as
a starting point for future researchers. Additions and modifications are expected and
encouraged, especially in considering other types of ephemeral ecosystems.
Acknowledgements
I would like to thank Andrea Romero and Aldemaro Romero Jr. for reviewing earlier versions of
the manuscript. I also thank two anonymous reviewers for improving it greatly and generating
many new ideas.
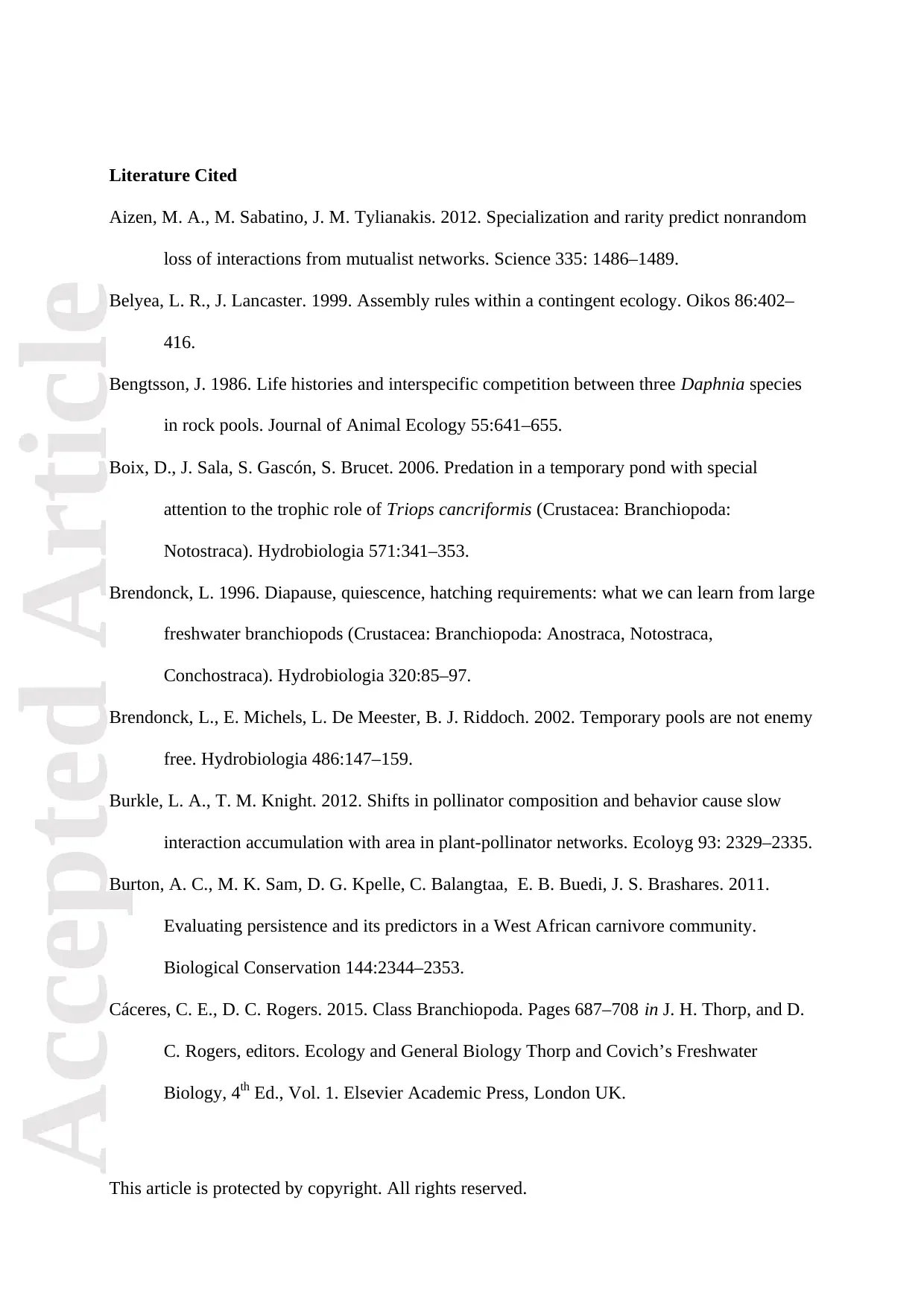
Accepted ArticleAccepted Article
This article is protected by copyright. All rights reserved.
Literature Cited
Aizen, M. A., M. Sabatino, J. M. Tylianakis. 2012. Specialization and rarity predict nonrandom
loss of interactions from mutualist networks. Science 335: 1486–1489.
Belyea, L. R., J. Lancaster. 1999. Assembly rules within a contingent ecology. Oikos 86:402–
416.
Bengtsson, J. 1986. Life histories and interspecific competition between three Daphnia species
in rock pools. Journal of Animal Ecology 55:641–655.
Boix, D., J. Sala, S. Gascón, S. Brucet. 2006. Predation in a temporary pond with special
attention to the trophic role of Triops cancriformis (Crustacea: Branchiopoda:
Notostraca). Hydrobiologia 571:341–353.
Brendonck, L. 1996. Diapause, quiescence, hatching requirements: what we can learn from large
freshwater branchiopods (Crustacea: Branchiopoda: Anostraca, Notostraca,
Conchostraca). Hydrobiologia 320:85–97.
Brendonck, L., E. Michels, L. De Meester, B. J. Riddoch. 2002. Temporary pools are not enemy
free. Hydrobiologia 486:147–159.
Burkle, L. A., T. M. Knight. 2012. Shifts in pollinator composition and behavior cause slow
interaction accumulation with area in plant-pollinator networks. Ecoloyg 93: 2329–2335.
Burton, A. C., M. K. Sam, D. G. Kpelle, C. Balangtaa, E. B. Buedi, J. S. Brashares. 2011.
Evaluating persistence and its predictors in a West African carnivore community.
Biological Conservation 144:2344–2353.
Cáceres, C. E., D. C. Rogers. 2015. Class Branchiopoda. Pages 687–708 in J. H. Thorp, and D.
C. Rogers, editors. Ecology and General Biology Thorp and Covich’s Freshwater
Biology, 4th Ed., Vol. 1. Elsevier Academic Press, London UK.
This article is protected by copyright. All rights reserved.
Literature Cited
Aizen, M. A., M. Sabatino, J. M. Tylianakis. 2012. Specialization and rarity predict nonrandom
loss of interactions from mutualist networks. Science 335: 1486–1489.
Belyea, L. R., J. Lancaster. 1999. Assembly rules within a contingent ecology. Oikos 86:402–
416.
Bengtsson, J. 1986. Life histories and interspecific competition between three Daphnia species
in rock pools. Journal of Animal Ecology 55:641–655.
Boix, D., J. Sala, S. Gascón, S. Brucet. 2006. Predation in a temporary pond with special
attention to the trophic role of Triops cancriformis (Crustacea: Branchiopoda:
Notostraca). Hydrobiologia 571:341–353.
Brendonck, L. 1996. Diapause, quiescence, hatching requirements: what we can learn from large
freshwater branchiopods (Crustacea: Branchiopoda: Anostraca, Notostraca,
Conchostraca). Hydrobiologia 320:85–97.
Brendonck, L., E. Michels, L. De Meester, B. J. Riddoch. 2002. Temporary pools are not enemy
free. Hydrobiologia 486:147–159.
Burkle, L. A., T. M. Knight. 2012. Shifts in pollinator composition and behavior cause slow
interaction accumulation with area in plant-pollinator networks. Ecoloyg 93: 2329–2335.
Burton, A. C., M. K. Sam, D. G. Kpelle, C. Balangtaa, E. B. Buedi, J. S. Brashares. 2011.
Evaluating persistence and its predictors in a West African carnivore community.
Biological Conservation 144:2344–2353.
Cáceres, C. E., D. C. Rogers. 2015. Class Branchiopoda. Pages 687–708 in J. H. Thorp, and D.
C. Rogers, editors. Ecology and General Biology Thorp and Covich’s Freshwater
Biology, 4th Ed., Vol. 1. Elsevier Academic Press, London UK.
Secure Best Marks with AI Grader
Need help grading? Try our AI Grader for instant feedback on your assignments.
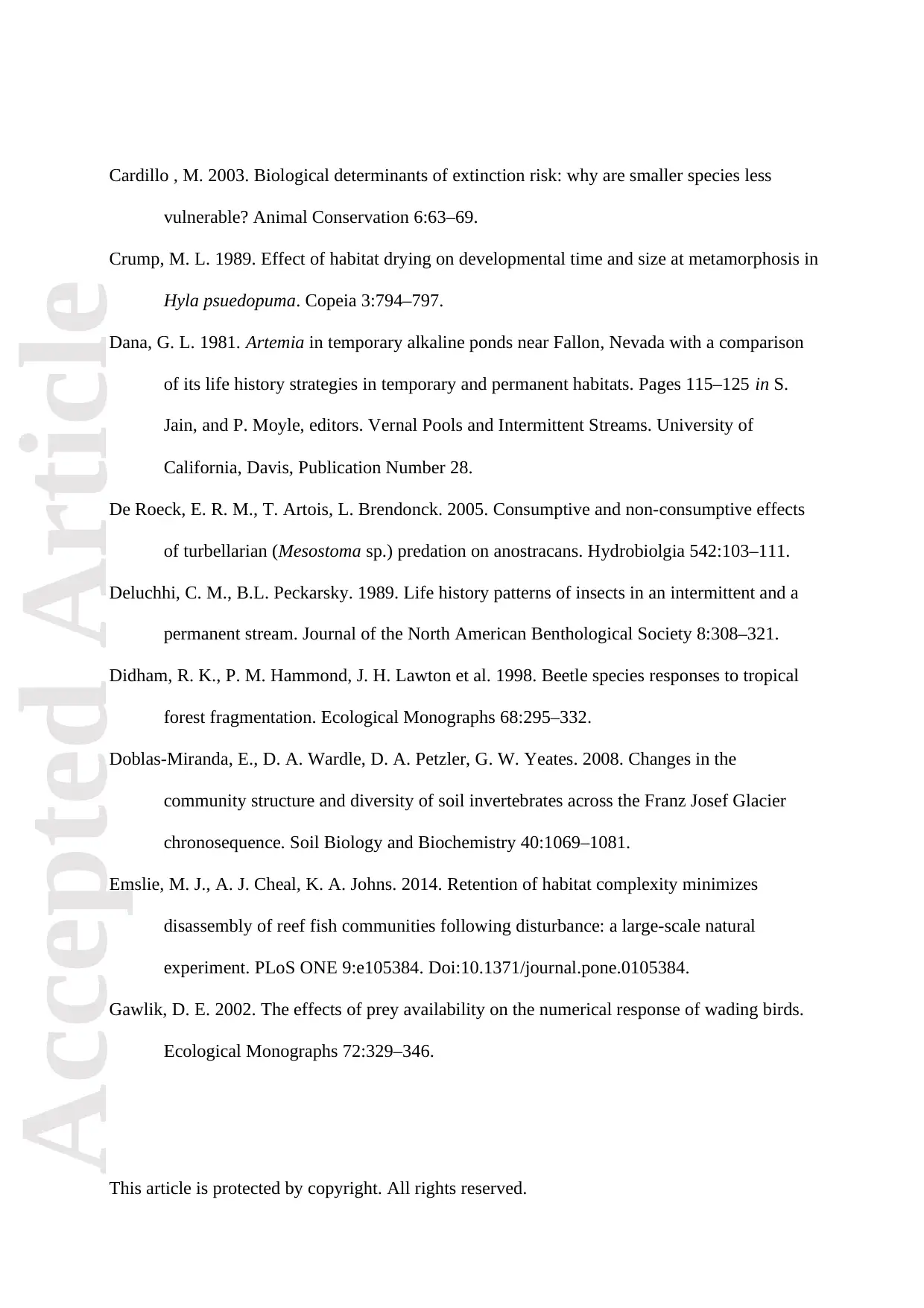
Accepted ArticleAccepted Article
This article is protected by copyright. All rights reserved.
Cardillo , M. 2003. Biological determinants of extinction risk: why are smaller species less
vulnerable? Animal Conservation 6:63–69.
Crump, M. L. 1989. Effect of habitat drying on developmental time and size at metamorphosis in
Hyla psuedopuma. Copeia 3:794–797.
Dana, G. L. 1981. Artemia in temporary alkaline ponds near Fallon, Nevada with a comparison
of its life history strategies in temporary and permanent habitats. Pages 115–125 in S.
Jain, and P. Moyle, editors. Vernal Pools and Intermittent Streams. University of
California, Davis, Publication Number 28.
De Roeck, E. R. M., T. Artois, L. Brendonck. 2005. Consumptive and non-consumptive effects
of turbellarian (Mesostoma sp.) predation on anostracans. Hydrobiolgia 542:103–111.
Deluchhi, C. M., B.L. Peckarsky. 1989. Life history patterns of insects in an intermittent and a
permanent stream. Journal of the North American Benthological Society 8:308–321.
Didham, R. K., P. M. Hammond, J. H. Lawton et al. 1998. Beetle species responses to tropical
forest fragmentation. Ecological Monographs 68:295–332.
Doblas-Miranda, E., D. A. Wardle, D. A. Petzler, G. W. Yeates. 2008. Changes in the
community structure and diversity of soil invertebrates across the Franz Josef Glacier
chronosequence. Soil Biology and Biochemistry 40:1069–1081.
Emslie, M. J., A. J. Cheal, K. A. Johns. 2014. Retention of habitat complexity minimizes
disassembly of reef fish communities following disturbance: a large-scale natural
experiment. PLoS ONE 9:e105384. Doi:10.1371/journal.pone.0105384.
Gawlik, D. E. 2002. The effects of prey availability on the numerical response of wading birds.
Ecological Monographs 72:329–346.
This article is protected by copyright. All rights reserved.
Cardillo , M. 2003. Biological determinants of extinction risk: why are smaller species less
vulnerable? Animal Conservation 6:63–69.
Crump, M. L. 1989. Effect of habitat drying on developmental time and size at metamorphosis in
Hyla psuedopuma. Copeia 3:794–797.
Dana, G. L. 1981. Artemia in temporary alkaline ponds near Fallon, Nevada with a comparison
of its life history strategies in temporary and permanent habitats. Pages 115–125 in S.
Jain, and P. Moyle, editors. Vernal Pools and Intermittent Streams. University of
California, Davis, Publication Number 28.
De Roeck, E. R. M., T. Artois, L. Brendonck. 2005. Consumptive and non-consumptive effects
of turbellarian (Mesostoma sp.) predation on anostracans. Hydrobiolgia 542:103–111.
Deluchhi, C. M., B.L. Peckarsky. 1989. Life history patterns of insects in an intermittent and a
permanent stream. Journal of the North American Benthological Society 8:308–321.
Didham, R. K., P. M. Hammond, J. H. Lawton et al. 1998. Beetle species responses to tropical
forest fragmentation. Ecological Monographs 68:295–332.
Doblas-Miranda, E., D. A. Wardle, D. A. Petzler, G. W. Yeates. 2008. Changes in the
community structure and diversity of soil invertebrates across the Franz Josef Glacier
chronosequence. Soil Biology and Biochemistry 40:1069–1081.
Emslie, M. J., A. J. Cheal, K. A. Johns. 2014. Retention of habitat complexity minimizes
disassembly of reef fish communities following disturbance: a large-scale natural
experiment. PLoS ONE 9:e105384. Doi:10.1371/journal.pone.0105384.
Gawlik, D. E. 2002. The effects of prey availability on the numerical response of wading birds.
Ecological Monographs 72:329–346.
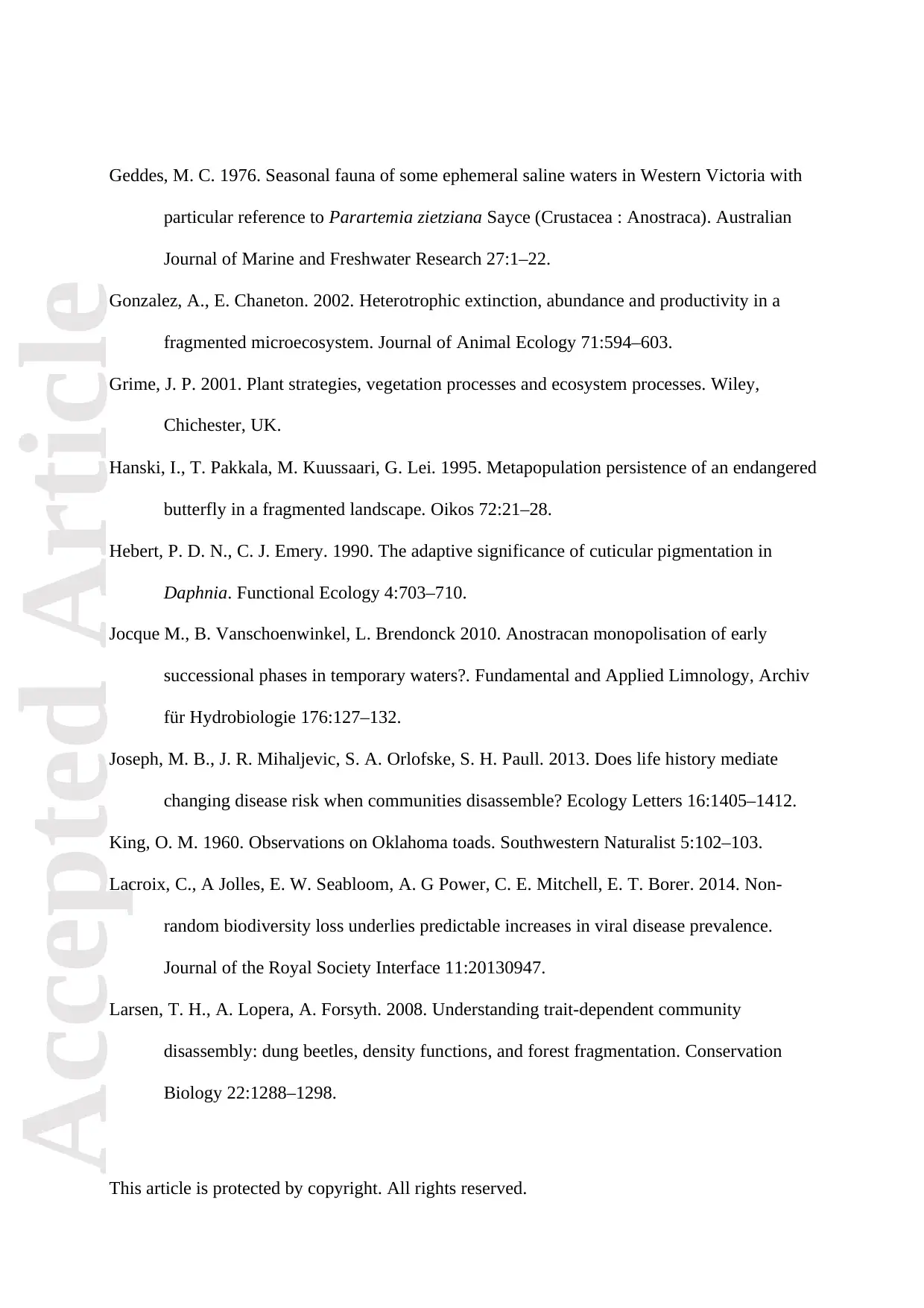
Accepted ArticleAccepted Article
This article is protected by copyright. All rights reserved.
Geddes, M. C. 1976. Seasonal fauna of some ephemeral saline waters in Western Victoria with
particular reference to Parartemia zietziana Sayce (Crustacea : Anostraca). Australian
Journal of Marine and Freshwater Research 27:1–22.
Gonzalez, A., E. Chaneton. 2002. Heterotrophic extinction, abundance and productivity in a
fragmented microecosystem. Journal of Animal Ecology 71:594–603.
Grime, J. P. 2001. Plant strategies, vegetation processes and ecosystem processes. Wiley,
Chichester, UK.
Hanski, I., T. Pakkala, M. Kuussaari, G. Lei. 1995. Metapopulation persistence of an endangered
butterfly in a fragmented landscape. Oikos 72:21–28.
Hebert, P. D. N., C. J. Emery. 1990. The adaptive significance of cuticular pigmentation in
Daphnia. Functional Ecology 4:703–710.
Jocque M., B. Vanschoenwinkel, L. Brendonck 2010. Anostracan monopolisation of early
successional phases in temporary waters?. Fundamental and Applied Limnology, Archiv
für Hydrobiologie 176:127–132.
Joseph, M. B., J. R. Mihaljevic, S. A. Orlofske, S. H. Paull. 2013. Does life history mediate
changing disease risk when communities disassemble? Ecology Letters 16:1405–1412.
King, O. M. 1960. Observations on Oklahoma toads. Southwestern Naturalist 5:102–103.
Lacroix, C., A Jolles, E. W. Seabloom, A. G Power, C. E. Mitchell, E. T. Borer. 2014. Non-
random biodiversity loss underlies predictable increases in viral disease prevalence.
Journal of the Royal Society Interface 11:20130947.
Larsen, T. H., A. Lopera, A. Forsyth. 2008. Understanding trait-dependent community
disassembly: dung beetles, density functions, and forest fragmentation. Conservation
Biology 22:1288–1298.
This article is protected by copyright. All rights reserved.
Geddes, M. C. 1976. Seasonal fauna of some ephemeral saline waters in Western Victoria with
particular reference to Parartemia zietziana Sayce (Crustacea : Anostraca). Australian
Journal of Marine and Freshwater Research 27:1–22.
Gonzalez, A., E. Chaneton. 2002. Heterotrophic extinction, abundance and productivity in a
fragmented microecosystem. Journal of Animal Ecology 71:594–603.
Grime, J. P. 2001. Plant strategies, vegetation processes and ecosystem processes. Wiley,
Chichester, UK.
Hanski, I., T. Pakkala, M. Kuussaari, G. Lei. 1995. Metapopulation persistence of an endangered
butterfly in a fragmented landscape. Oikos 72:21–28.
Hebert, P. D. N., C. J. Emery. 1990. The adaptive significance of cuticular pigmentation in
Daphnia. Functional Ecology 4:703–710.
Jocque M., B. Vanschoenwinkel, L. Brendonck 2010. Anostracan monopolisation of early
successional phases in temporary waters?. Fundamental and Applied Limnology, Archiv
für Hydrobiologie 176:127–132.
Joseph, M. B., J. R. Mihaljevic, S. A. Orlofske, S. H. Paull. 2013. Does life history mediate
changing disease risk when communities disassemble? Ecology Letters 16:1405–1412.
King, O. M. 1960. Observations on Oklahoma toads. Southwestern Naturalist 5:102–103.
Lacroix, C., A Jolles, E. W. Seabloom, A. G Power, C. E. Mitchell, E. T. Borer. 2014. Non-
random biodiversity loss underlies predictable increases in viral disease prevalence.
Journal of the Royal Society Interface 11:20130947.
Larsen, T. H., A. Lopera, A. Forsyth. 2008. Understanding trait-dependent community
disassembly: dung beetles, density functions, and forest fragmentation. Conservation
Biology 22:1288–1298.
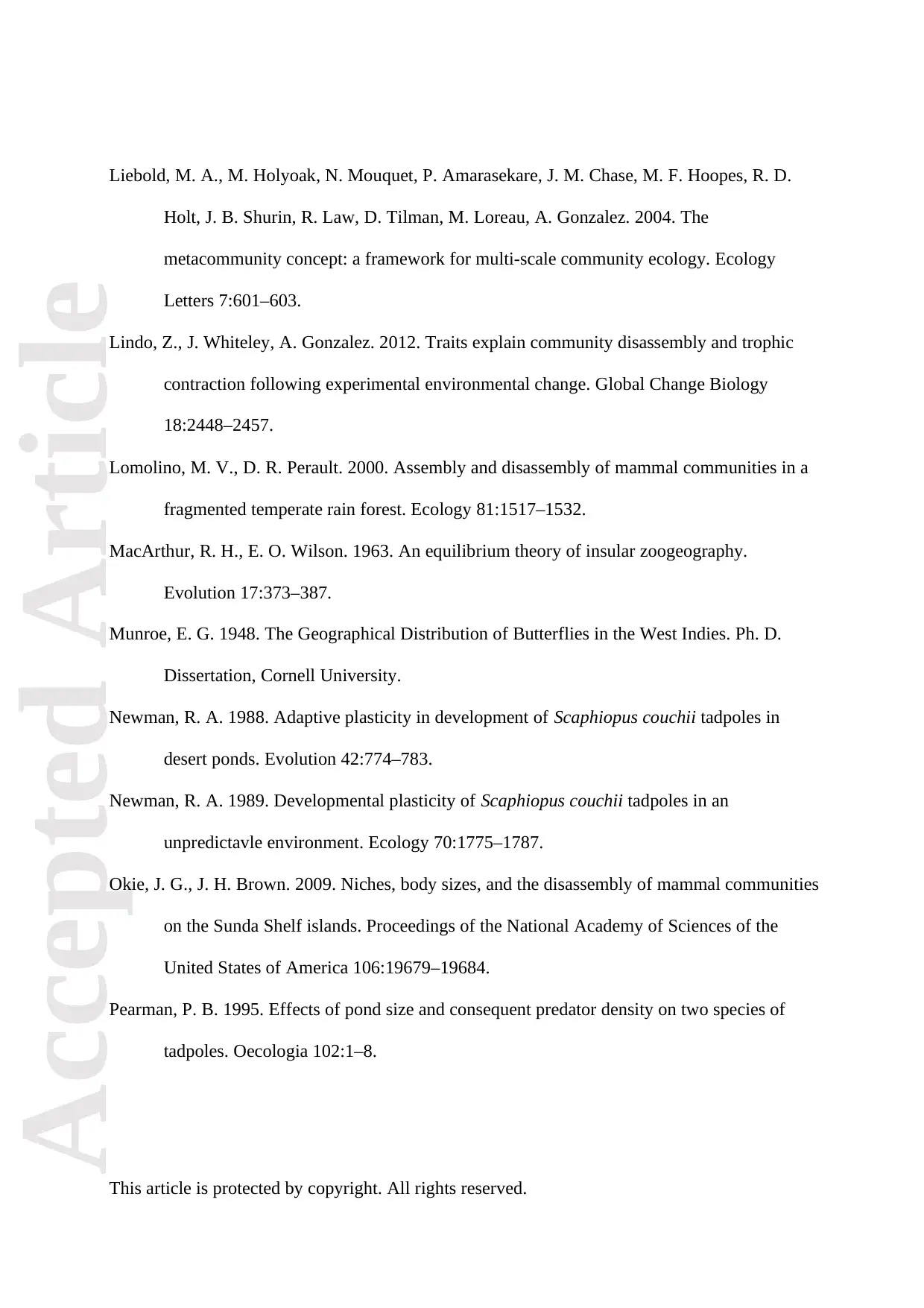
Accepted ArticleAccepted Article
This article is protected by copyright. All rights reserved.
Liebold, M. A., M. Holyoak, N. Mouquet, P. Amarasekare, J. M. Chase, M. F. Hoopes, R. D.
Holt, J. B. Shurin, R. Law, D. Tilman, M. Loreau, A. Gonzalez. 2004. The
metacommunity concept: a framework for multi-scale community ecology. Ecology
Letters 7:601–603.
Lindo, Z., J. Whiteley, A. Gonzalez. 2012. Traits explain community disassembly and trophic
contraction following experimental environmental change. Global Change Biology
18:2448–2457.
Lomolino, M. V., D. R. Perault. 2000. Assembly and disassembly of mammal communities in a
fragmented temperate rain forest. Ecology 81:1517–1532.
MacArthur, R. H., E. O. Wilson. 1963. An equilibrium theory of insular zoogeography.
Evolution 17:373–387.
Munroe, E. G. 1948. The Geographical Distribution of Butterflies in the West Indies. Ph. D.
Dissertation, Cornell University.
Newman, R. A. 1988. Adaptive plasticity in development of Scaphiopus couchii tadpoles in
desert ponds. Evolution 42:774–783.
Newman, R. A. 1989. Developmental plasticity of Scaphiopus couchii tadpoles in an
unpredictavle environment. Ecology 70:1775–1787.
Okie, J. G., J. H. Brown. 2009. Niches, body sizes, and the disassembly of mammal communities
on the Sunda Shelf islands. Proceedings of the National Academy of Sciences of the
United States of America 106:19679–19684.
Pearman, P. B. 1995. Effects of pond size and consequent predator density on two species of
tadpoles. Oecologia 102:1–8.
This article is protected by copyright. All rights reserved.
Liebold, M. A., M. Holyoak, N. Mouquet, P. Amarasekare, J. M. Chase, M. F. Hoopes, R. D.
Holt, J. B. Shurin, R. Law, D. Tilman, M. Loreau, A. Gonzalez. 2004. The
metacommunity concept: a framework for multi-scale community ecology. Ecology
Letters 7:601–603.
Lindo, Z., J. Whiteley, A. Gonzalez. 2012. Traits explain community disassembly and trophic
contraction following experimental environmental change. Global Change Biology
18:2448–2457.
Lomolino, M. V., D. R. Perault. 2000. Assembly and disassembly of mammal communities in a
fragmented temperate rain forest. Ecology 81:1517–1532.
MacArthur, R. H., E. O. Wilson. 1963. An equilibrium theory of insular zoogeography.
Evolution 17:373–387.
Munroe, E. G. 1948. The Geographical Distribution of Butterflies in the West Indies. Ph. D.
Dissertation, Cornell University.
Newman, R. A. 1988. Adaptive plasticity in development of Scaphiopus couchii tadpoles in
desert ponds. Evolution 42:774–783.
Newman, R. A. 1989. Developmental plasticity of Scaphiopus couchii tadpoles in an
unpredictavle environment. Ecology 70:1775–1787.
Okie, J. G., J. H. Brown. 2009. Niches, body sizes, and the disassembly of mammal communities
on the Sunda Shelf islands. Proceedings of the National Academy of Sciences of the
United States of America 106:19679–19684.
Pearman, P. B. 1995. Effects of pond size and consequent predator density on two species of
tadpoles. Oecologia 102:1–8.
Paraphrase This Document
Need a fresh take? Get an instant paraphrase of this document with our AI Paraphraser
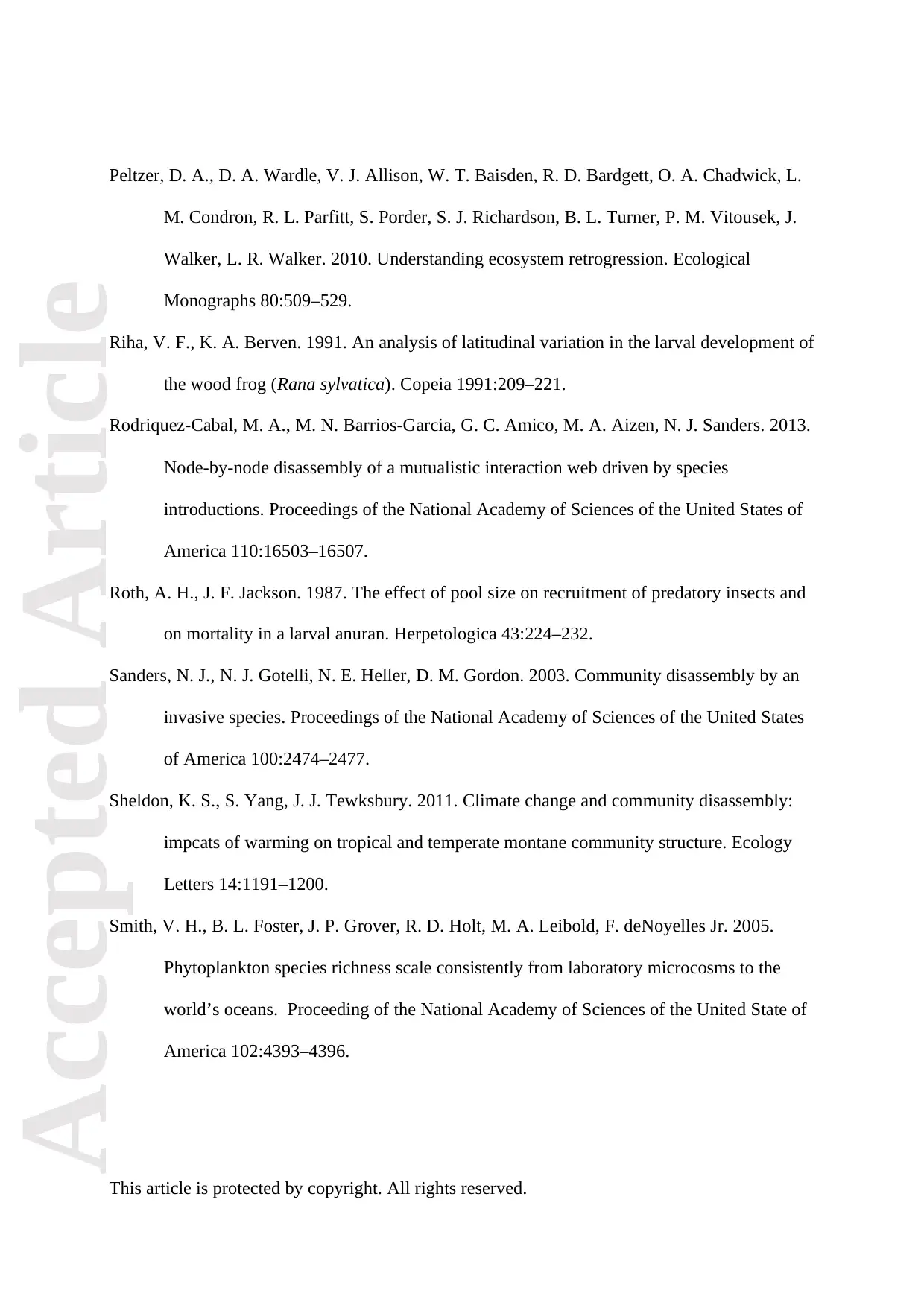
Accepted ArticleAccepted Article
This article is protected by copyright. All rights reserved.
Peltzer, D. A., D. A. Wardle, V. J. Allison, W. T. Baisden, R. D. Bardgett, O. A. Chadwick, L.
M. Condron, R. L. Parfitt, S. Porder, S. J. Richardson, B. L. Turner, P. M. Vitousek, J.
Walker, L. R. Walker. 2010. Understanding ecosystem retrogression. Ecological
Monographs 80:509–529.
Riha, V. F., K. A. Berven. 1991. An analysis of latitudinal variation in the larval development of
the wood frog (Rana sylvatica). Copeia 1991:209–221.
Rodriquez-Cabal, M. A., M. N. Barrios-Garcia, G. C. Amico, M. A. Aizen, N. J. Sanders. 2013.
Node-by-node disassembly of a mutualistic interaction web driven by species
introductions. Proceedings of the National Academy of Sciences of the United States of
America 110:16503–16507.
Roth, A. H., J. F. Jackson. 1987. The effect of pool size on recruitment of predatory insects and
on mortality in a larval anuran. Herpetologica 43:224–232.
Sanders, N. J., N. J. Gotelli, N. E. Heller, D. M. Gordon. 2003. Community disassembly by an
invasive species. Proceedings of the National Academy of Sciences of the United States
of America 100:2474–2477.
Sheldon, K. S., S. Yang, J. J. Tewksbury. 2011. Climate change and community disassembly:
impcats of warming on tropical and temperate montane community structure. Ecology
Letters 14:1191–1200.
Smith, V. H., B. L. Foster, J. P. Grover, R. D. Holt, M. A. Leibold, F. deNoyelles Jr. 2005.
Phytoplankton species richness scale consistently from laboratory microcosms to the
world’s oceans. Proceeding of the National Academy of Sciences of the United State of
America 102:4393–4396.
This article is protected by copyright. All rights reserved.
Peltzer, D. A., D. A. Wardle, V. J. Allison, W. T. Baisden, R. D. Bardgett, O. A. Chadwick, L.
M. Condron, R. L. Parfitt, S. Porder, S. J. Richardson, B. L. Turner, P. M. Vitousek, J.
Walker, L. R. Walker. 2010. Understanding ecosystem retrogression. Ecological
Monographs 80:509–529.
Riha, V. F., K. A. Berven. 1991. An analysis of latitudinal variation in the larval development of
the wood frog (Rana sylvatica). Copeia 1991:209–221.
Rodriquez-Cabal, M. A., M. N. Barrios-Garcia, G. C. Amico, M. A. Aizen, N. J. Sanders. 2013.
Node-by-node disassembly of a mutualistic interaction web driven by species
introductions. Proceedings of the National Academy of Sciences of the United States of
America 110:16503–16507.
Roth, A. H., J. F. Jackson. 1987. The effect of pool size on recruitment of predatory insects and
on mortality in a larval anuran. Herpetologica 43:224–232.
Sanders, N. J., N. J. Gotelli, N. E. Heller, D. M. Gordon. 2003. Community disassembly by an
invasive species. Proceedings of the National Academy of Sciences of the United States
of America 100:2474–2477.
Sheldon, K. S., S. Yang, J. J. Tewksbury. 2011. Climate change and community disassembly:
impcats of warming on tropical and temperate montane community structure. Ecology
Letters 14:1191–1200.
Smith, V. H., B. L. Foster, J. P. Grover, R. D. Holt, M. A. Leibold, F. deNoyelles Jr. 2005.
Phytoplankton species richness scale consistently from laboratory microcosms to the
world’s oceans. Proceeding of the National Academy of Sciences of the United State of
America 102:4393–4396.
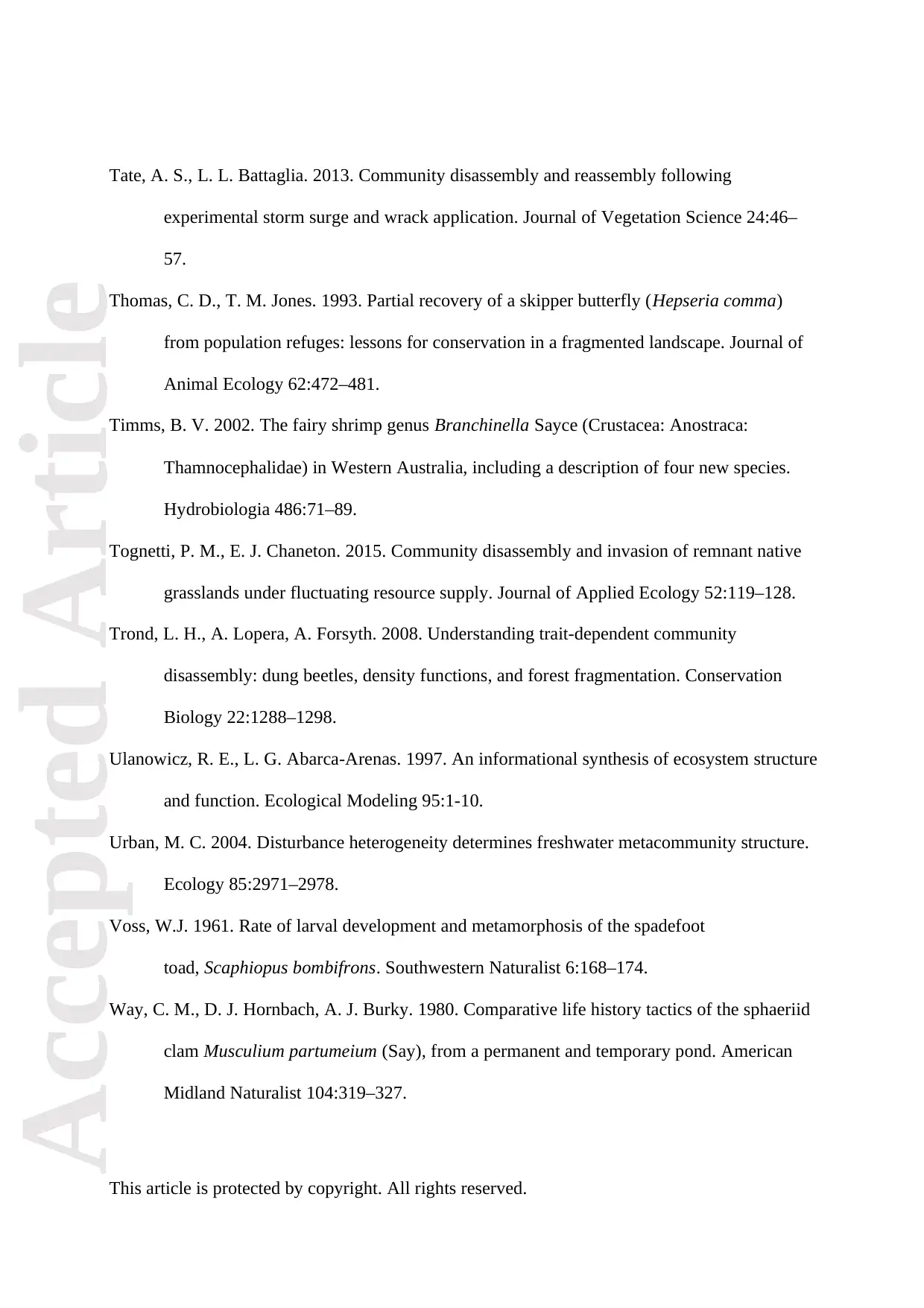
Accepted ArticleAccepted Article
This article is protected by copyright. All rights reserved.
Tate, A. S., L. L. Battaglia. 2013. Community disassembly and reassembly following
experimental storm surge and wrack application. Journal of Vegetation Science 24:46–
57.
Thomas, C. D., T. M. Jones. 1993. Partial recovery of a skipper butterfly (Hepseria comma)
from population refuges: lessons for conservation in a fragmented landscape. Journal of
Animal Ecology 62:472–481.
Timms, B. V. 2002. The fairy shrimp genus Branchinella Sayce (Crustacea: Anostraca:
Thamnocephalidae) in Western Australia, including a description of four new species.
Hydrobiologia 486:71–89.
Tognetti, P. M., E. J. Chaneton. 2015. Community disassembly and invasion of remnant native
grasslands under fluctuating resource supply. Journal of Applied Ecology 52:119–128.
Trond, L. H., A. Lopera, A. Forsyth. 2008. Understanding trait-dependent community
disassembly: dung beetles, density functions, and forest fragmentation. Conservation
Biology 22:1288–1298.
Ulanowicz, R. E., L. G. Abarca-Arenas. 1997. An informational synthesis of ecosystem structure
and function. Ecological Modeling 95:1-10.
Urban, M. C. 2004. Disturbance heterogeneity determines freshwater metacommunity structure.
Ecology 85:2971–2978.
Voss, W.J. 1961. Rate of larval development and metamorphosis of the spadefoot
toad, Scaphiopus bombifrons. Southwestern Naturalist 6:168–174.
Way, C. M., D. J. Hornbach, A. J. Burky. 1980. Comparative life history tactics of the sphaeriid
clam Musculium partumeium (Say), from a permanent and temporary pond. American
Midland Naturalist 104:319–327.
This article is protected by copyright. All rights reserved.
Tate, A. S., L. L. Battaglia. 2013. Community disassembly and reassembly following
experimental storm surge and wrack application. Journal of Vegetation Science 24:46–
57.
Thomas, C. D., T. M. Jones. 1993. Partial recovery of a skipper butterfly (Hepseria comma)
from population refuges: lessons for conservation in a fragmented landscape. Journal of
Animal Ecology 62:472–481.
Timms, B. V. 2002. The fairy shrimp genus Branchinella Sayce (Crustacea: Anostraca:
Thamnocephalidae) in Western Australia, including a description of four new species.
Hydrobiologia 486:71–89.
Tognetti, P. M., E. J. Chaneton. 2015. Community disassembly and invasion of remnant native
grasslands under fluctuating resource supply. Journal of Applied Ecology 52:119–128.
Trond, L. H., A. Lopera, A. Forsyth. 2008. Understanding trait-dependent community
disassembly: dung beetles, density functions, and forest fragmentation. Conservation
Biology 22:1288–1298.
Ulanowicz, R. E., L. G. Abarca-Arenas. 1997. An informational synthesis of ecosystem structure
and function. Ecological Modeling 95:1-10.
Urban, M. C. 2004. Disturbance heterogeneity determines freshwater metacommunity structure.
Ecology 85:2971–2978.
Voss, W.J. 1961. Rate of larval development and metamorphosis of the spadefoot
toad, Scaphiopus bombifrons. Southwestern Naturalist 6:168–174.
Way, C. M., D. J. Hornbach, A. J. Burky. 1980. Comparative life history tactics of the sphaeriid
clam Musculium partumeium (Say), from a permanent and temporary pond. American
Midland Naturalist 104:319–327.
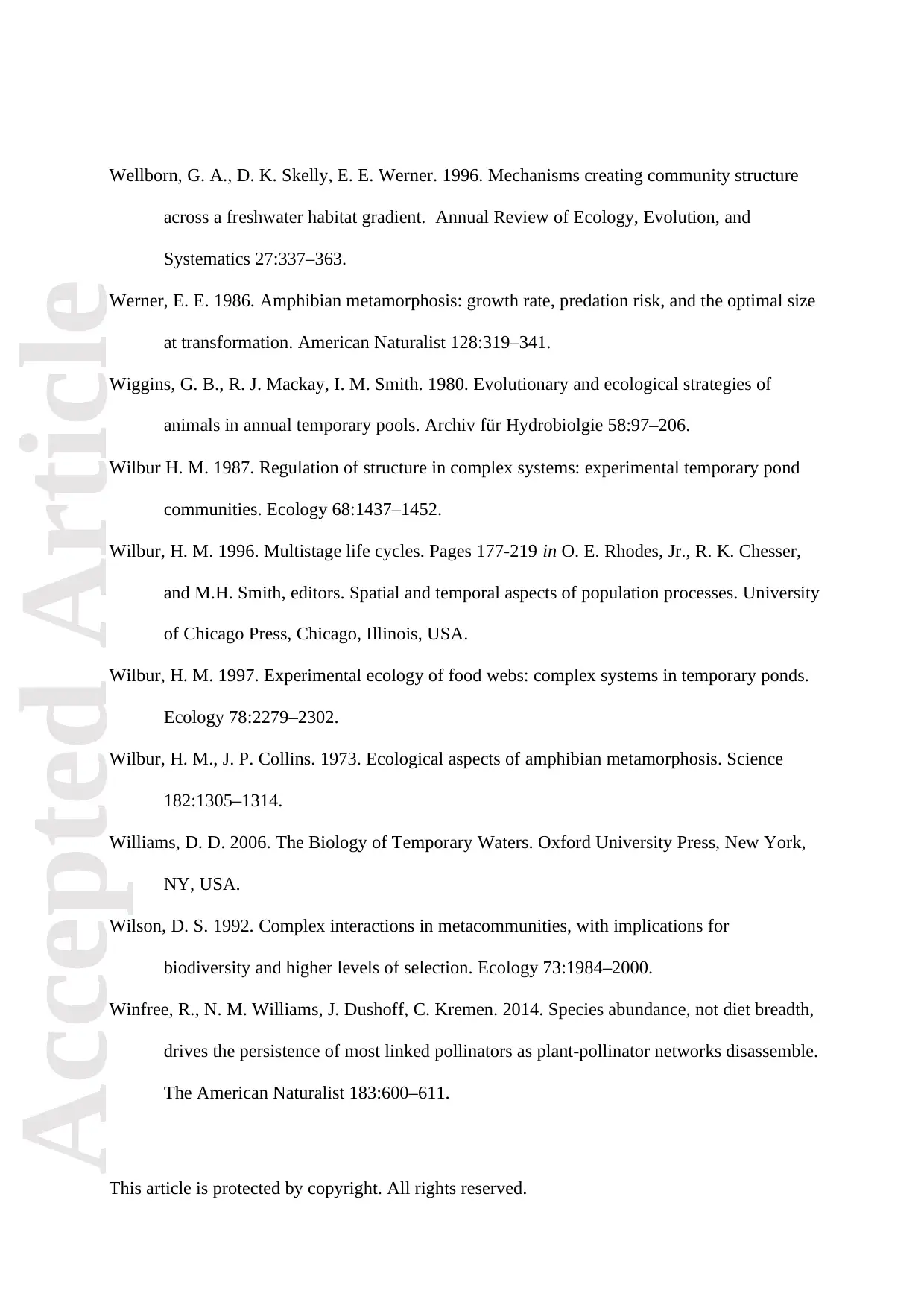
Accepted ArticleAccepted Article
This article is protected by copyright. All rights reserved.
Wellborn, G. A., D. K. Skelly, E. E. Werner. 1996. Mechanisms creating community structure
across a freshwater habitat gradient. Annual Review of Ecology, Evolution, and
Systematics 27:337–363.
Werner, E. E. 1986. Amphibian metamorphosis: growth rate, predation risk, and the optimal size
at transformation. American Naturalist 128:319–341.
Wiggins, G. B., R. J. Mackay, I. M. Smith. 1980. Evolutionary and ecological strategies of
animals in annual temporary pools. Archiv für Hydrobiolgie 58:97–206.
Wilbur H. M. 1987. Regulation of structure in complex systems: experimental temporary pond
communities. Ecology 68:1437–1452.
Wilbur, H. M. 1996. Multistage life cycles. Pages 177-219 in O. E. Rhodes, Jr., R. K. Chesser,
and M.H. Smith, editors. Spatial and temporal aspects of population processes. University
of Chicago Press, Chicago, Illinois, USA.
Wilbur, H. M. 1997. Experimental ecology of food webs: complex systems in temporary ponds.
Ecology 78:2279–2302.
Wilbur, H. M., J. P. Collins. 1973. Ecological aspects of amphibian metamorphosis. Science
182:1305–1314.
Williams, D. D. 2006. The Biology of Temporary Waters. Oxford University Press, New York,
NY, USA.
Wilson, D. S. 1992. Complex interactions in metacommunities, with implications for
biodiversity and higher levels of selection. Ecology 73:1984–2000.
Winfree, R., N. M. Williams, J. Dushoff, C. Kremen. 2014. Species abundance, not diet breadth,
drives the persistence of most linked pollinators as plant-pollinator networks disassemble.
The American Naturalist 183:600–611.
This article is protected by copyright. All rights reserved.
Wellborn, G. A., D. K. Skelly, E. E. Werner. 1996. Mechanisms creating community structure
across a freshwater habitat gradient. Annual Review of Ecology, Evolution, and
Systematics 27:337–363.
Werner, E. E. 1986. Amphibian metamorphosis: growth rate, predation risk, and the optimal size
at transformation. American Naturalist 128:319–341.
Wiggins, G. B., R. J. Mackay, I. M. Smith. 1980. Evolutionary and ecological strategies of
animals in annual temporary pools. Archiv für Hydrobiolgie 58:97–206.
Wilbur H. M. 1987. Regulation of structure in complex systems: experimental temporary pond
communities. Ecology 68:1437–1452.
Wilbur, H. M. 1996. Multistage life cycles. Pages 177-219 in O. E. Rhodes, Jr., R. K. Chesser,
and M.H. Smith, editors. Spatial and temporal aspects of population processes. University
of Chicago Press, Chicago, Illinois, USA.
Wilbur, H. M. 1997. Experimental ecology of food webs: complex systems in temporary ponds.
Ecology 78:2279–2302.
Wilbur, H. M., J. P. Collins. 1973. Ecological aspects of amphibian metamorphosis. Science
182:1305–1314.
Williams, D. D. 2006. The Biology of Temporary Waters. Oxford University Press, New York,
NY, USA.
Wilson, D. S. 1992. Complex interactions in metacommunities, with implications for
biodiversity and higher levels of selection. Ecology 73:1984–2000.
Winfree, R., N. M. Williams, J. Dushoff, C. Kremen. 2014. Species abundance, not diet breadth,
drives the persistence of most linked pollinators as plant-pollinator networks disassemble.
The American Naturalist 183:600–611.
Secure Best Marks with AI Grader
Need help grading? Try our AI Grader for instant feedback on your assignments.
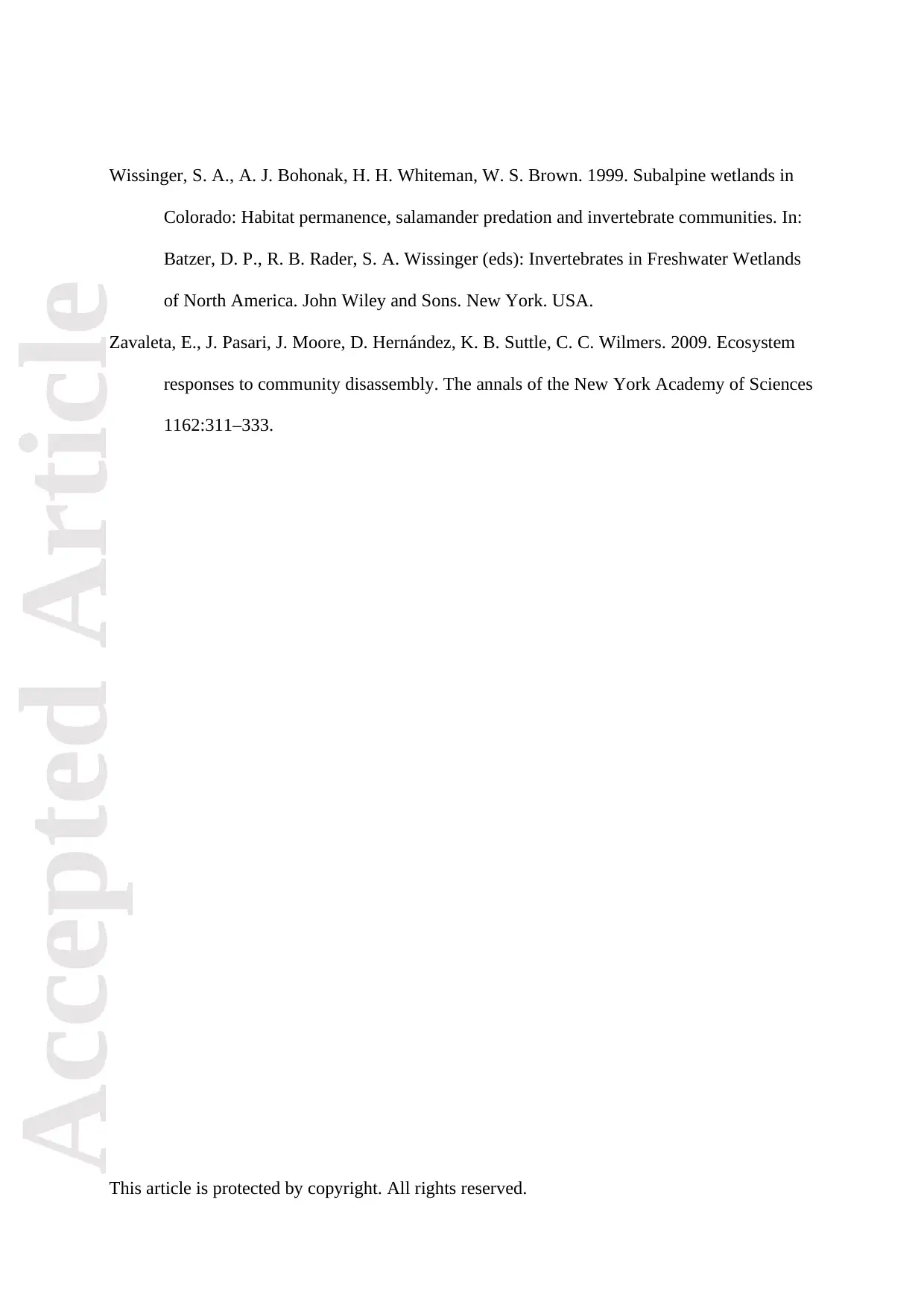
Accepted ArticleAccepted Article
This article is protected by copyright. All rights reserved.
Wissinger, S. A., A. J. Bohonak, H. H. Whiteman, W. S. Brown. 1999. Subalpine wetlands in
Colorado: Habitat permanence, salamander predation and invertebrate communities. In:
Batzer, D. P., R. B. Rader, S. A. Wissinger (eds): Invertebrates in Freshwater Wetlands
of North America. John Wiley and Sons. New York. USA.
Zavaleta, E., J. Pasari, J. Moore, D. Hernández, K. B. Suttle, C. C. Wilmers. 2009. Ecosystem
responses to community disassembly. The annals of the New York Academy of Sciences
1162:311–333.
This article is protected by copyright. All rights reserved.
Wissinger, S. A., A. J. Bohonak, H. H. Whiteman, W. S. Brown. 1999. Subalpine wetlands in
Colorado: Habitat permanence, salamander predation and invertebrate communities. In:
Batzer, D. P., R. B. Rader, S. A. Wissinger (eds): Invertebrates in Freshwater Wetlands
of North America. John Wiley and Sons. New York. USA.
Zavaleta, E., J. Pasari, J. Moore, D. Hernández, K. B. Suttle, C. C. Wilmers. 2009. Ecosystem
responses to community disassembly. The annals of the New York Academy of Sciences
1162:311–333.
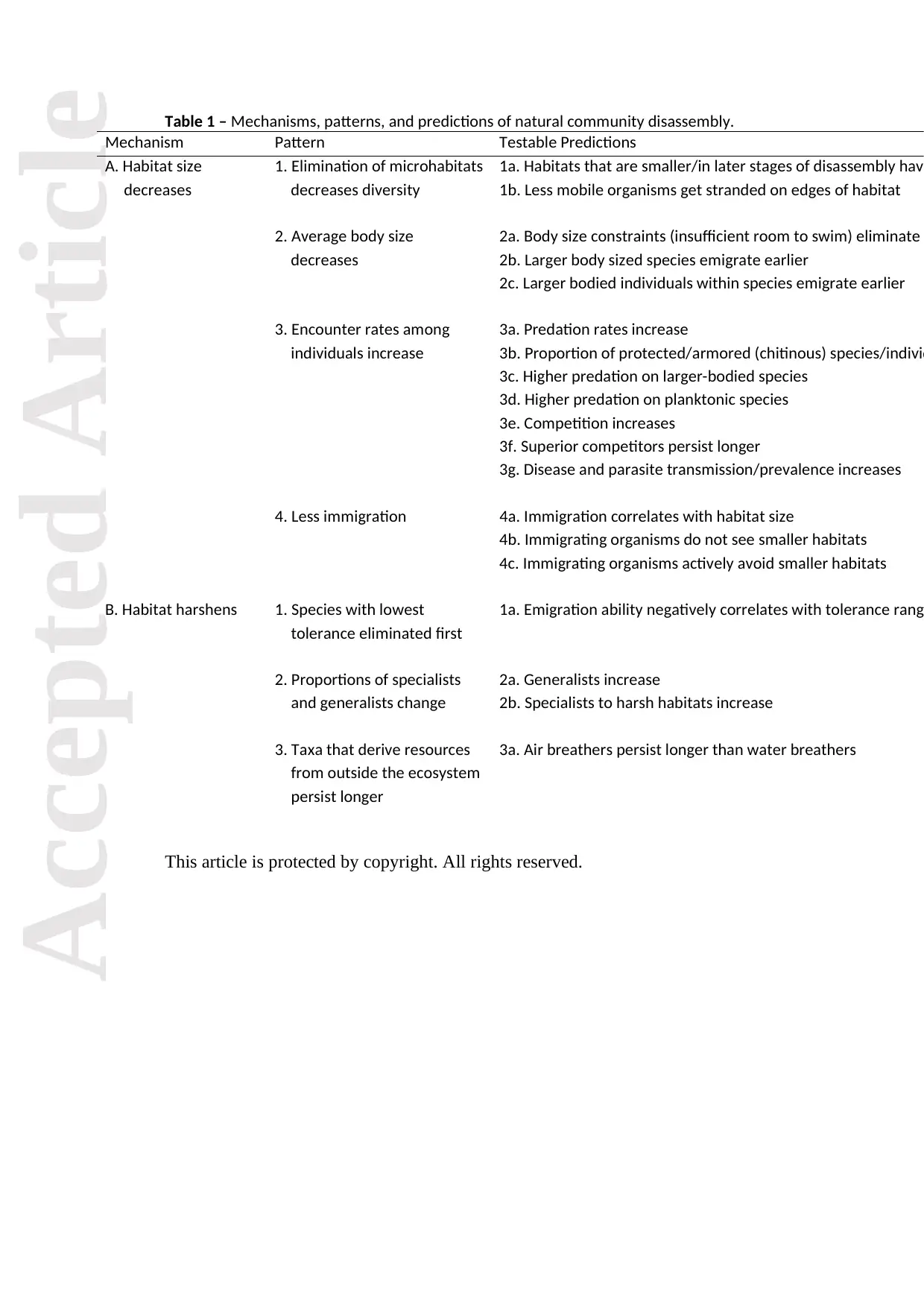
Accepted Article
This article is protected by copyright. All rights reserved.
Table 1 – Mechanisms, patterns, and predictions of natural community disassembly.
Mechanism Pattern Testable Predictions
A. Habitat size
decreases
1. Elimination of microhabitats
decreases diversity
1a. Habitats that are smaller/in later stages of disassembly have
1b. Less mobile organisms get stranded on edges of habitat
2. Average body size
decreases
2a. Body size constraints (insufficient room to swim) eliminate
2b. Larger body sized species emigrate earlier
2c. Larger bodied individuals within species emigrate earlier
3. Encounter rates among
individuals increase
3a. Predation rates increase
3b. Proportion of protected/armored (chitinous) species/individ
3c. Higher predation on larger-bodied species
3d. Higher predation on planktonic species
3e. Competition increases
3f. Superior competitors persist longer
3g. Disease and parasite transmission/prevalence increases
4. Less immigration 4a. Immigration correlates with habitat size
4b. Immigrating organisms do not see smaller habitats
4c. Immigrating organisms actively avoid smaller habitats
B. Habitat harshens 1. Species with lowest
tolerance eliminated first
1a. Emigration ability negatively correlates with tolerance rang
2. Proportions of specialists
and generalists change
2a. Generalists increase
2b. Specialists to harsh habitats increase
3. Taxa that derive resources
from outside the ecosystem
persist longer
3a. Air breathers persist longer than water breathers
This article is protected by copyright. All rights reserved.
Table 1 – Mechanisms, patterns, and predictions of natural community disassembly.
Mechanism Pattern Testable Predictions
A. Habitat size
decreases
1. Elimination of microhabitats
decreases diversity
1a. Habitats that are smaller/in later stages of disassembly have
1b. Less mobile organisms get stranded on edges of habitat
2. Average body size
decreases
2a. Body size constraints (insufficient room to swim) eliminate
2b. Larger body sized species emigrate earlier
2c. Larger bodied individuals within species emigrate earlier
3. Encounter rates among
individuals increase
3a. Predation rates increase
3b. Proportion of protected/armored (chitinous) species/individ
3c. Higher predation on larger-bodied species
3d. Higher predation on planktonic species
3e. Competition increases
3f. Superior competitors persist longer
3g. Disease and parasite transmission/prevalence increases
4. Less immigration 4a. Immigration correlates with habitat size
4b. Immigrating organisms do not see smaller habitats
4c. Immigrating organisms actively avoid smaller habitats
B. Habitat harshens 1. Species with lowest
tolerance eliminated first
1a. Emigration ability negatively correlates with tolerance rang
2. Proportions of specialists
and generalists change
2a. Generalists increase
2b. Specialists to harsh habitats increase
3. Taxa that derive resources
from outside the ecosystem
persist longer
3a. Air breathers persist longer than water breathers
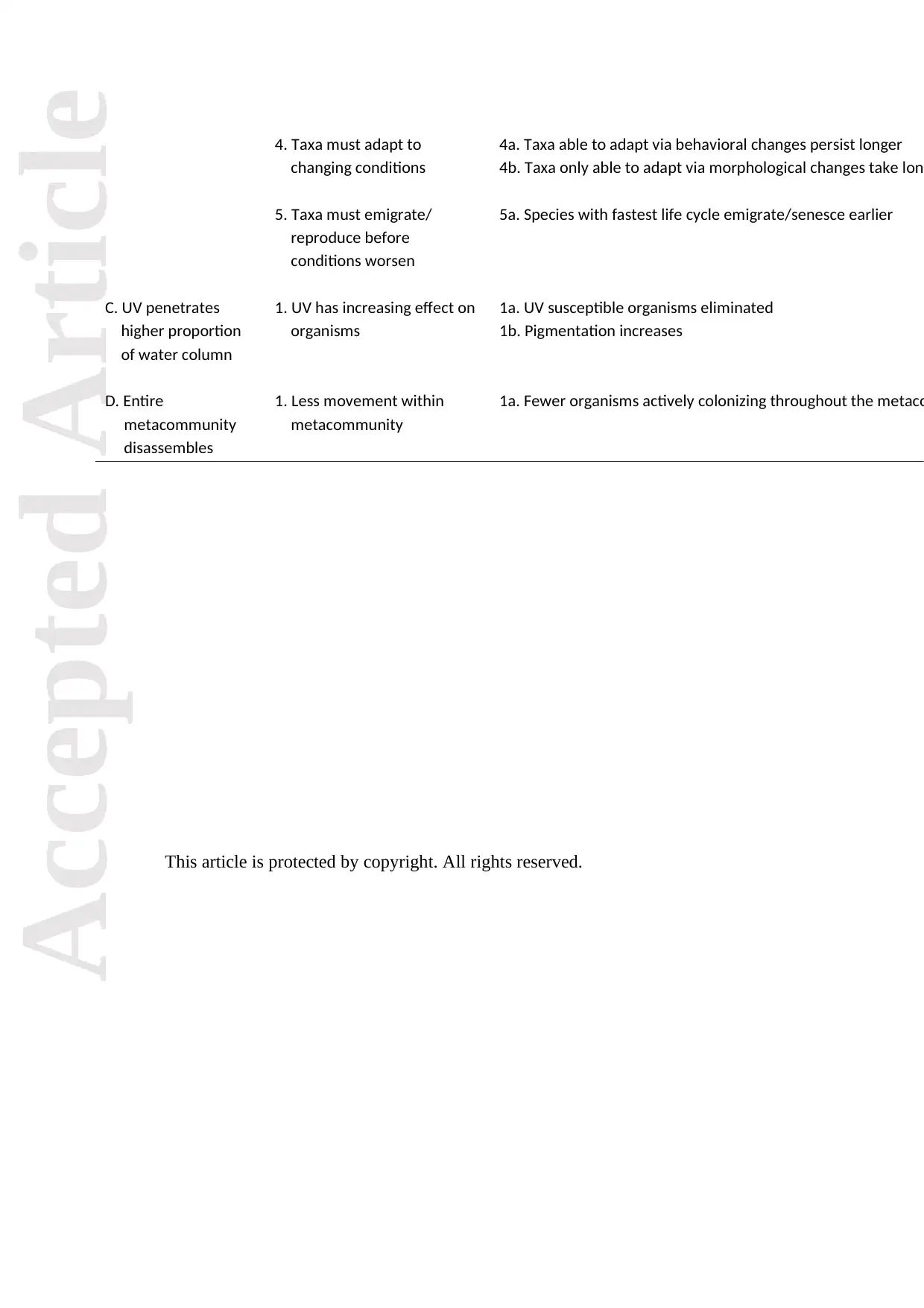
Accepted Article
This article is protected by copyright. All rights reserved.
4. Taxa must adapt to
changing conditions
4a. Taxa able to adapt via behavioral changes persist longer
4b. Taxa only able to adapt via morphological changes take long
5. Taxa must emigrate/
reproduce before
conditions worsen
5a. Species with fastest life cycle emigrate/senesce earlier
C. UV penetrates
higher proportion
of water column
1. UV has increasing effect on
organisms
1a. UV susceptible organisms eliminated
1b. Pigmentation increases
D. Entire
metacommunity
disassembles
1. Less movement within
metacommunity
1a. Fewer organisms actively colonizing throughout the metaco
This article is protected by copyright. All rights reserved.
4. Taxa must adapt to
changing conditions
4a. Taxa able to adapt via behavioral changes persist longer
4b. Taxa only able to adapt via morphological changes take long
5. Taxa must emigrate/
reproduce before
conditions worsen
5a. Species with fastest life cycle emigrate/senesce earlier
C. UV penetrates
higher proportion
of water column
1. UV has increasing effect on
organisms
1a. UV susceptible organisms eliminated
1b. Pigmentation increases
D. Entire
metacommunity
disassembles
1. Less movement within
metacommunity
1a. Fewer organisms actively colonizing throughout the metaco
Paraphrase This Document
Need a fresh take? Get an instant paraphrase of this document with our AI Paraphraser
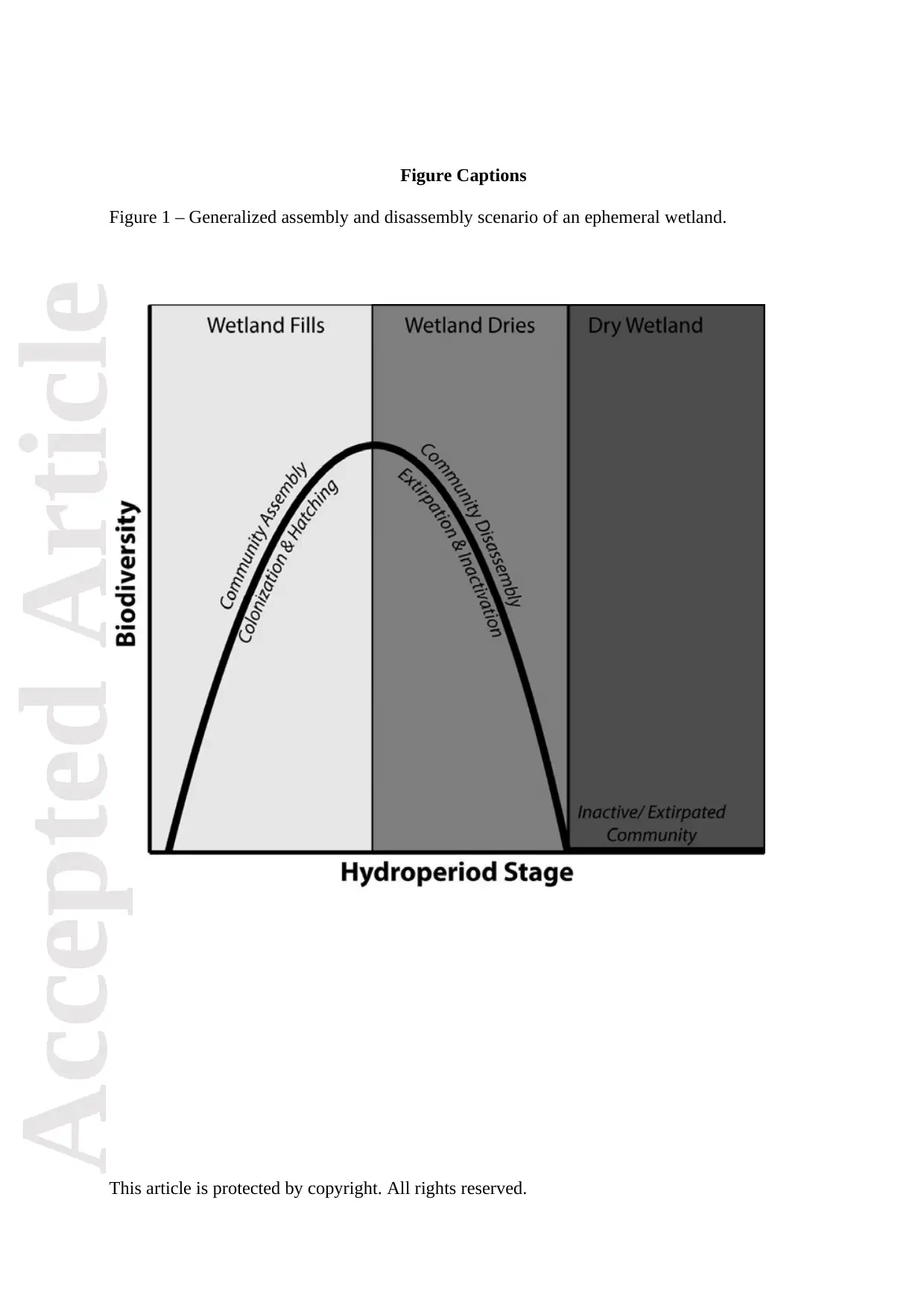
Accepted ArticleAccepted Article
This article is protected by copyright. All rights reserved.
Figure Captions
Figure 1 – Generalized assembly and disassembly scenario of an ephemeral wetland.
This article is protected by copyright. All rights reserved.
Figure Captions
Figure 1 – Generalized assembly and disassembly scenario of an ephemeral wetland.
1 out of 26
![[object Object]](/_next/image/?url=%2F_next%2Fstatic%2Fmedia%2Flogo.6d15ce61.png&w=640&q=75)
Your All-in-One AI-Powered Toolkit for Academic Success.
+13062052269
info@desklib.com
Available 24*7 on WhatsApp / Email
Unlock your academic potential
© 2024 | Zucol Services PVT LTD | All rights reserved.