Ammonia Stress Corrosion Cracking: Mechanism, Materials and Prevention
VerifiedAdded on 2023/06/14
|14
|4189
|266
AI Summary
This report by Desklib delves into the mechanism, materials and prevention of ammonia stress corrosion cracking. It explains how stress corrosion cracking occurs, the affected materials and equipment, and the appearance and damage caused by this issue. The report also provides prevention and mitigation techniques for this problem.
Contribute Materials
Your contribution can guide someone’s learning journey. Share your
documents today.
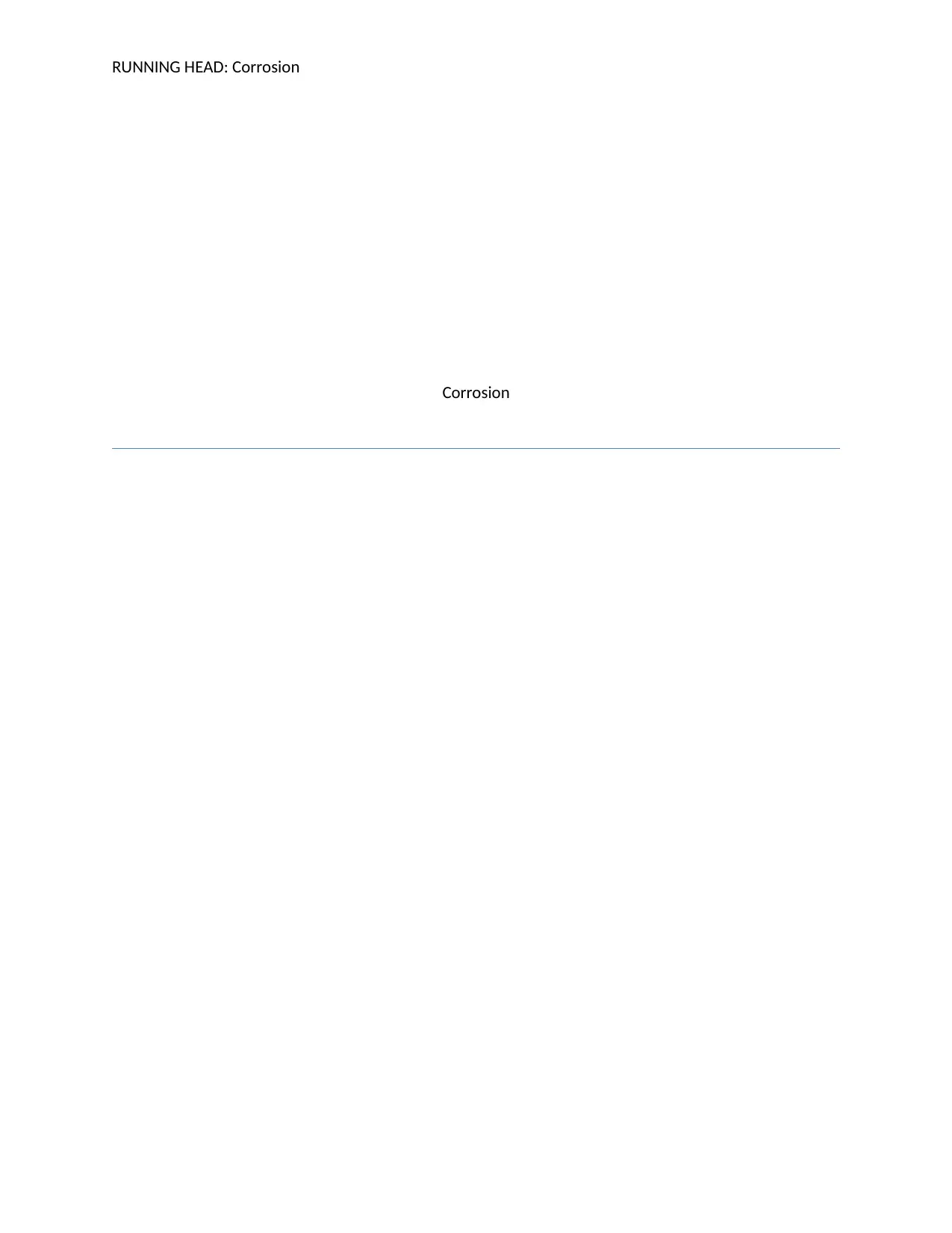
RUNNING HEAD: Corrosion
Corrosion
Corrosion
Secure Best Marks with AI Grader
Need help grading? Try our AI Grader for instant feedback on your assignments.
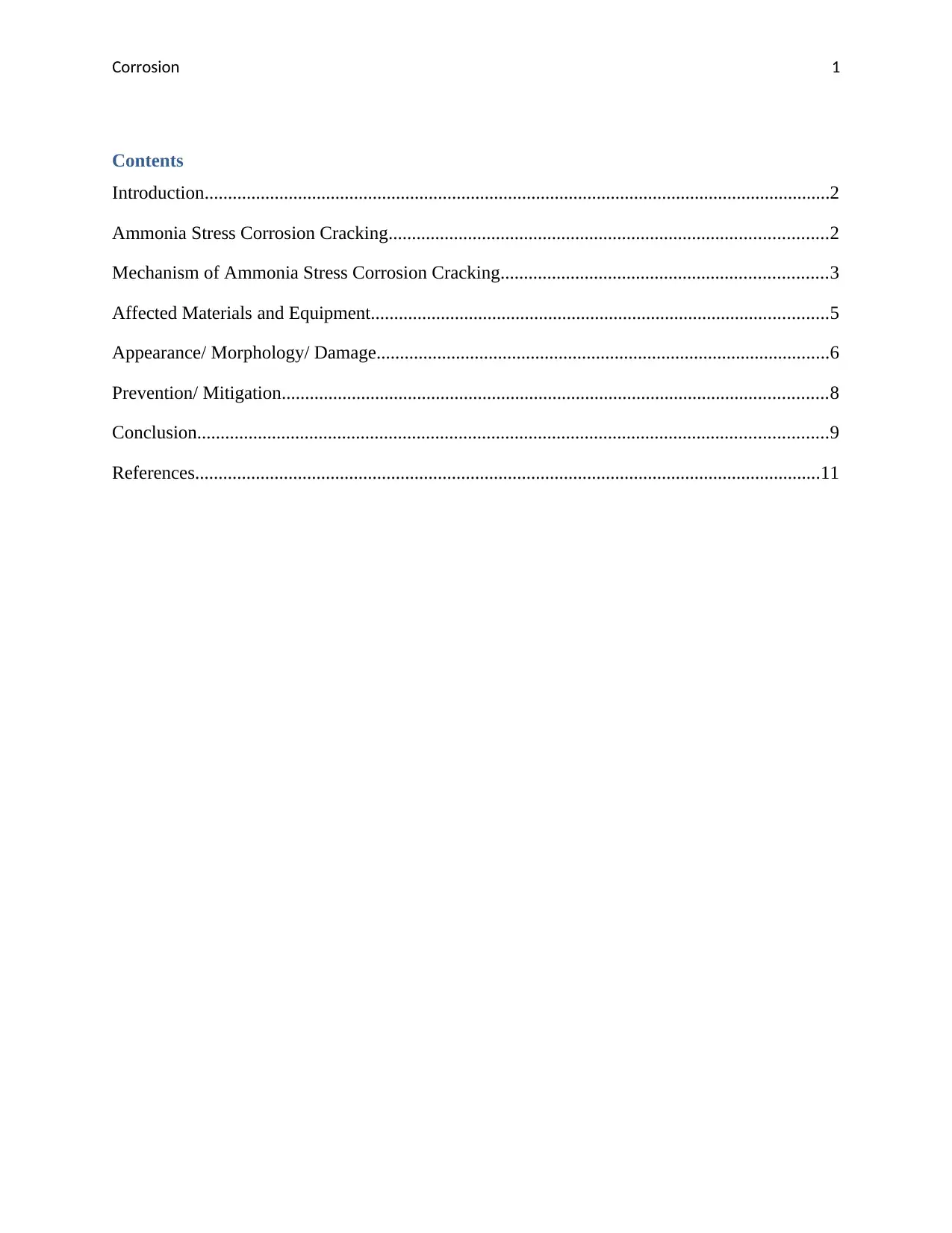
Corrosion 1
Contents
Introduction......................................................................................................................................2
Ammonia Stress Corrosion Cracking..............................................................................................2
Mechanism of Ammonia Stress Corrosion Cracking......................................................................3
Affected Materials and Equipment..................................................................................................5
Appearance/ Morphology/ Damage.................................................................................................6
Prevention/ Mitigation.....................................................................................................................8
Conclusion.......................................................................................................................................9
References......................................................................................................................................11
Contents
Introduction......................................................................................................................................2
Ammonia Stress Corrosion Cracking..............................................................................................2
Mechanism of Ammonia Stress Corrosion Cracking......................................................................3
Affected Materials and Equipment..................................................................................................5
Appearance/ Morphology/ Damage.................................................................................................6
Prevention/ Mitigation.....................................................................................................................8
Conclusion.......................................................................................................................................9
References......................................................................................................................................11
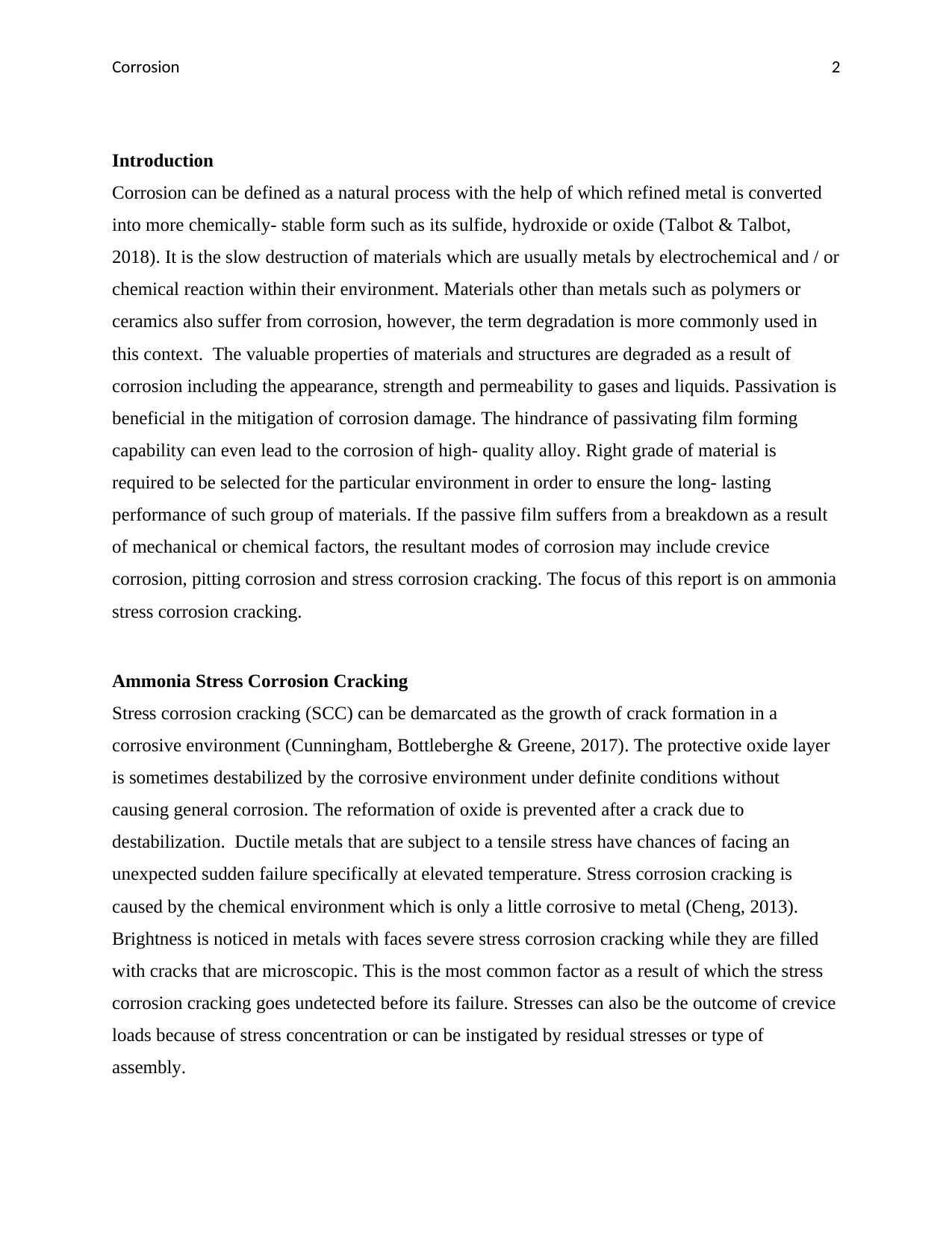
Corrosion 2
Introduction
Corrosion can be defined as a natural process with the help of which refined metal is converted
into more chemically- stable form such as its sulfide, hydroxide or oxide (Talbot & Talbot,
2018). It is the slow destruction of materials which are usually metals by electrochemical and / or
chemical reaction within their environment. Materials other than metals such as polymers or
ceramics also suffer from corrosion, however, the term degradation is more commonly used in
this context. The valuable properties of materials and structures are degraded as a result of
corrosion including the appearance, strength and permeability to gases and liquids. Passivation is
beneficial in the mitigation of corrosion damage. The hindrance of passivating film forming
capability can even lead to the corrosion of high- quality alloy. Right grade of material is
required to be selected for the particular environment in order to ensure the long- lasting
performance of such group of materials. If the passive film suffers from a breakdown as a result
of mechanical or chemical factors, the resultant modes of corrosion may include crevice
corrosion, pitting corrosion and stress corrosion cracking. The focus of this report is on ammonia
stress corrosion cracking.
Ammonia Stress Corrosion Cracking
Stress corrosion cracking (SCC) can be demarcated as the growth of crack formation in a
corrosive environment (Cunningham, Bottleberghe & Greene, 2017). The protective oxide layer
is sometimes destabilized by the corrosive environment under definite conditions without
causing general corrosion. The reformation of oxide is prevented after a crack due to
destabilization. Ductile metals that are subject to a tensile stress have chances of facing an
unexpected sudden failure specifically at elevated temperature. Stress corrosion cracking is
caused by the chemical environment which is only a little corrosive to metal (Cheng, 2013).
Brightness is noticed in metals with faces severe stress corrosion cracking while they are filled
with cracks that are microscopic. This is the most common factor as a result of which the stress
corrosion cracking goes undetected before its failure. Stresses can also be the outcome of crevice
loads because of stress concentration or can be instigated by residual stresses or type of
assembly.
Introduction
Corrosion can be defined as a natural process with the help of which refined metal is converted
into more chemically- stable form such as its sulfide, hydroxide or oxide (Talbot & Talbot,
2018). It is the slow destruction of materials which are usually metals by electrochemical and / or
chemical reaction within their environment. Materials other than metals such as polymers or
ceramics also suffer from corrosion, however, the term degradation is more commonly used in
this context. The valuable properties of materials and structures are degraded as a result of
corrosion including the appearance, strength and permeability to gases and liquids. Passivation is
beneficial in the mitigation of corrosion damage. The hindrance of passivating film forming
capability can even lead to the corrosion of high- quality alloy. Right grade of material is
required to be selected for the particular environment in order to ensure the long- lasting
performance of such group of materials. If the passive film suffers from a breakdown as a result
of mechanical or chemical factors, the resultant modes of corrosion may include crevice
corrosion, pitting corrosion and stress corrosion cracking. The focus of this report is on ammonia
stress corrosion cracking.
Ammonia Stress Corrosion Cracking
Stress corrosion cracking (SCC) can be demarcated as the growth of crack formation in a
corrosive environment (Cunningham, Bottleberghe & Greene, 2017). The protective oxide layer
is sometimes destabilized by the corrosive environment under definite conditions without
causing general corrosion. The reformation of oxide is prevented after a crack due to
destabilization. Ductile metals that are subject to a tensile stress have chances of facing an
unexpected sudden failure specifically at elevated temperature. Stress corrosion cracking is
caused by the chemical environment which is only a little corrosive to metal (Cheng, 2013).
Brightness is noticed in metals with faces severe stress corrosion cracking while they are filled
with cracks that are microscopic. This is the most common factor as a result of which the stress
corrosion cracking goes undetected before its failure. Stresses can also be the outcome of crevice
loads because of stress concentration or can be instigated by residual stresses or type of
assembly.
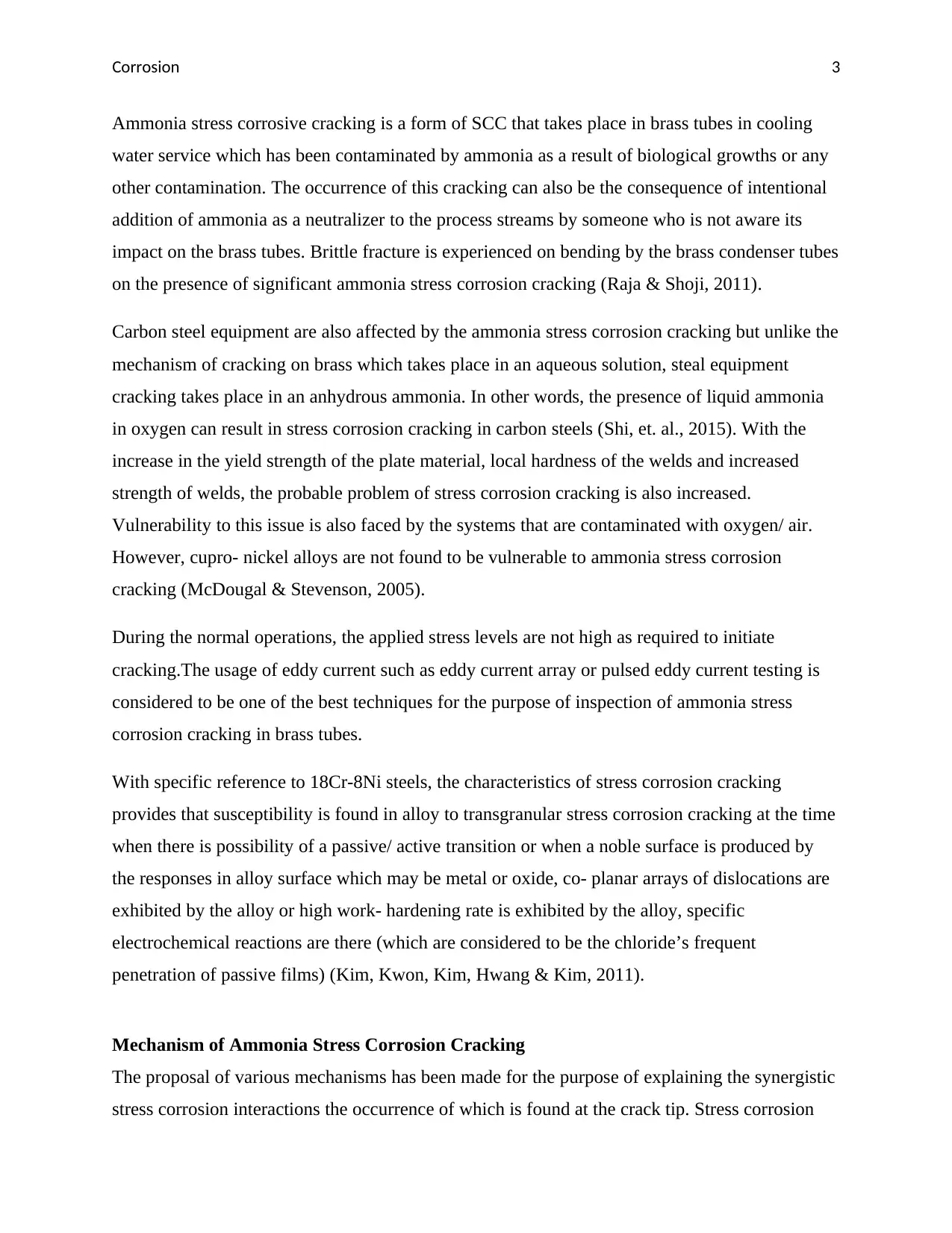
Corrosion 3
Ammonia stress corrosive cracking is a form of SCC that takes place in brass tubes in cooling
water service which has been contaminated by ammonia as a result of biological growths or any
other contamination. The occurrence of this cracking can also be the consequence of intentional
addition of ammonia as a neutralizer to the process streams by someone who is not aware its
impact on the brass tubes. Brittle fracture is experienced on bending by the brass condenser tubes
on the presence of significant ammonia stress corrosion cracking (Raja & Shoji, 2011).
Carbon steel equipment are also affected by the ammonia stress corrosion cracking but unlike the
mechanism of cracking on brass which takes place in an aqueous solution, steal equipment
cracking takes place in an anhydrous ammonia. In other words, the presence of liquid ammonia
in oxygen can result in stress corrosion cracking in carbon steels (Shi, et. al., 2015). With the
increase in the yield strength of the plate material, local hardness of the welds and increased
strength of welds, the probable problem of stress corrosion cracking is also increased.
Vulnerability to this issue is also faced by the systems that are contaminated with oxygen/ air.
However, cupro- nickel alloys are not found to be vulnerable to ammonia stress corrosion
cracking (McDougal & Stevenson, 2005).
During the normal operations, the applied stress levels are not high as required to initiate
cracking.The usage of eddy current such as eddy current array or pulsed eddy current testing is
considered to be one of the best techniques for the purpose of inspection of ammonia stress
corrosion cracking in brass tubes.
With specific reference to 18Cr-8Ni steels, the characteristics of stress corrosion cracking
provides that susceptibility is found in alloy to transgranular stress corrosion cracking at the time
when there is possibility of a passive/ active transition or when a noble surface is produced by
the responses in alloy surface which may be metal or oxide, co- planar arrays of dislocations are
exhibited by the alloy or high work- hardening rate is exhibited by the alloy, specific
electrochemical reactions are there (which are considered to be the chloride’s frequent
penetration of passive films) (Kim, Kwon, Kim, Hwang & Kim, 2011).
Mechanism of Ammonia Stress Corrosion Cracking
The proposal of various mechanisms has been made for the purpose of explaining the synergistic
stress corrosion interactions the occurrence of which is found at the crack tip. Stress corrosion
Ammonia stress corrosive cracking is a form of SCC that takes place in brass tubes in cooling
water service which has been contaminated by ammonia as a result of biological growths or any
other contamination. The occurrence of this cracking can also be the consequence of intentional
addition of ammonia as a neutralizer to the process streams by someone who is not aware its
impact on the brass tubes. Brittle fracture is experienced on bending by the brass condenser tubes
on the presence of significant ammonia stress corrosion cracking (Raja & Shoji, 2011).
Carbon steel equipment are also affected by the ammonia stress corrosion cracking but unlike the
mechanism of cracking on brass which takes place in an aqueous solution, steal equipment
cracking takes place in an anhydrous ammonia. In other words, the presence of liquid ammonia
in oxygen can result in stress corrosion cracking in carbon steels (Shi, et. al., 2015). With the
increase in the yield strength of the plate material, local hardness of the welds and increased
strength of welds, the probable problem of stress corrosion cracking is also increased.
Vulnerability to this issue is also faced by the systems that are contaminated with oxygen/ air.
However, cupro- nickel alloys are not found to be vulnerable to ammonia stress corrosion
cracking (McDougal & Stevenson, 2005).
During the normal operations, the applied stress levels are not high as required to initiate
cracking.The usage of eddy current such as eddy current array or pulsed eddy current testing is
considered to be one of the best techniques for the purpose of inspection of ammonia stress
corrosion cracking in brass tubes.
With specific reference to 18Cr-8Ni steels, the characteristics of stress corrosion cracking
provides that susceptibility is found in alloy to transgranular stress corrosion cracking at the time
when there is possibility of a passive/ active transition or when a noble surface is produced by
the responses in alloy surface which may be metal or oxide, co- planar arrays of dislocations are
exhibited by the alloy or high work- hardening rate is exhibited by the alloy, specific
electrochemical reactions are there (which are considered to be the chloride’s frequent
penetration of passive films) (Kim, Kwon, Kim, Hwang & Kim, 2011).
Mechanism of Ammonia Stress Corrosion Cracking
The proposal of various mechanisms has been made for the purpose of explaining the synergistic
stress corrosion interactions the occurrence of which is found at the crack tip. Stress corrosion
Paraphrase This Document
Need a fresh take? Get an instant paraphrase of this document with our AI Paraphraser
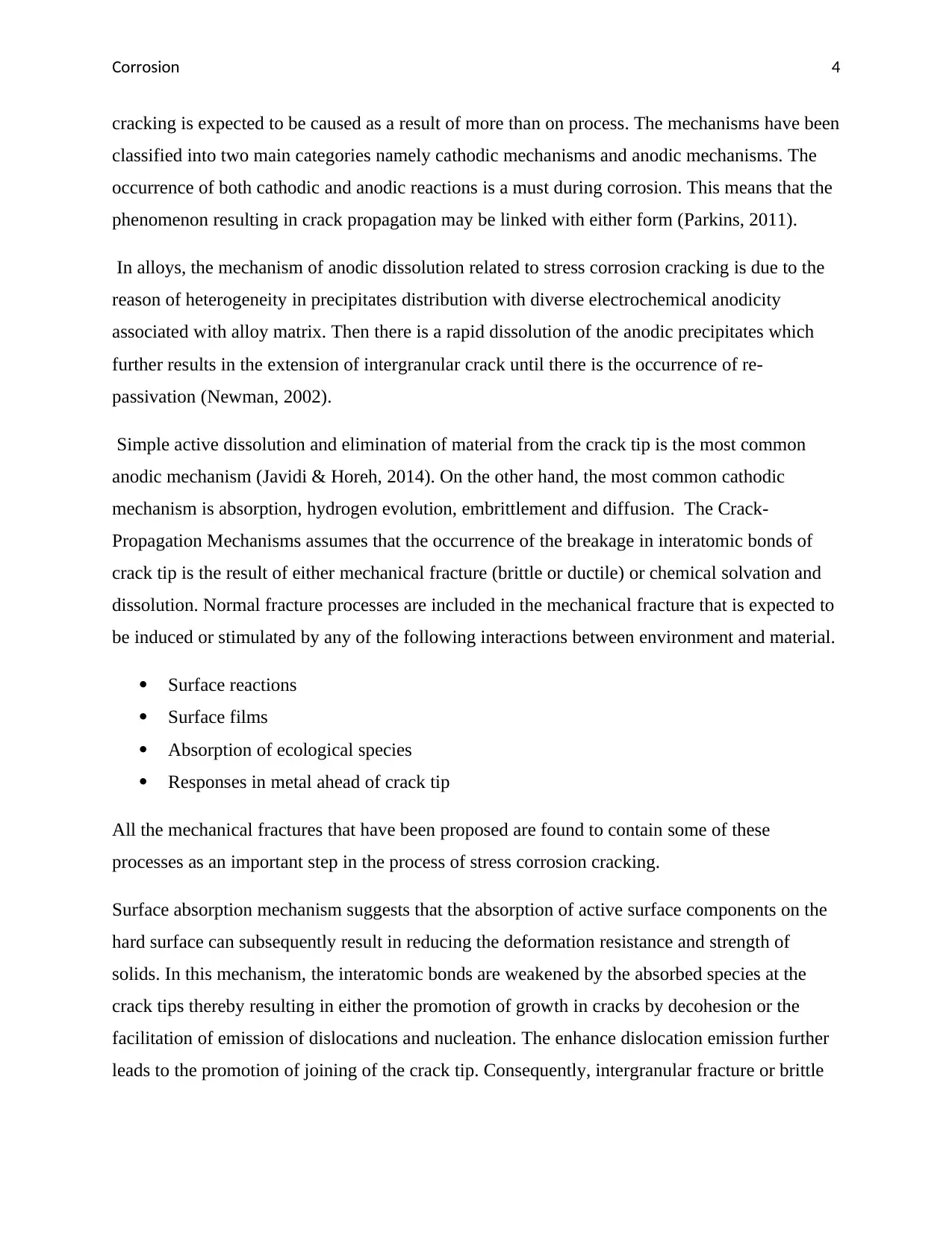
Corrosion 4
cracking is expected to be caused as a result of more than on process. The mechanisms have been
classified into two main categories namely cathodic mechanisms and anodic mechanisms. The
occurrence of both cathodic and anodic reactions is a must during corrosion. This means that the
phenomenon resulting in crack propagation may be linked with either form (Parkins, 2011).
In alloys, the mechanism of anodic dissolution related to stress corrosion cracking is due to the
reason of heterogeneity in precipitates distribution with diverse electrochemical anodicity
associated with alloy matrix. Then there is a rapid dissolution of the anodic precipitates which
further results in the extension of intergranular crack until there is the occurrence of re-
passivation (Newman, 2002).
Simple active dissolution and elimination of material from the crack tip is the most common
anodic mechanism (Javidi & Horeh, 2014). On the other hand, the most common cathodic
mechanism is absorption, hydrogen evolution, embrittlement and diffusion. The Crack-
Propagation Mechanisms assumes that the occurrence of the breakage in interatomic bonds of
crack tip is the result of either mechanical fracture (brittle or ductile) or chemical solvation and
dissolution. Normal fracture processes are included in the mechanical fracture that is expected to
be induced or stimulated by any of the following interactions between environment and material.
Surface reactions
Surface films
Absorption of ecological species
Responses in metal ahead of crack tip
All the mechanical fractures that have been proposed are found to contain some of these
processes as an important step in the process of stress corrosion cracking.
Surface absorption mechanism suggests that the absorption of active surface components on the
hard surface can subsequently result in reducing the deformation resistance and strength of
solids. In this mechanism, the interatomic bonds are weakened by the absorbed species at the
crack tips thereby resulting in either the promotion of growth in cracks by decohesion or the
facilitation of emission of dislocations and nucleation. The enhance dislocation emission further
leads to the promotion of joining of the crack tip. Consequently, intergranular fracture or brittle
cracking is expected to be caused as a result of more than on process. The mechanisms have been
classified into two main categories namely cathodic mechanisms and anodic mechanisms. The
occurrence of both cathodic and anodic reactions is a must during corrosion. This means that the
phenomenon resulting in crack propagation may be linked with either form (Parkins, 2011).
In alloys, the mechanism of anodic dissolution related to stress corrosion cracking is due to the
reason of heterogeneity in precipitates distribution with diverse electrochemical anodicity
associated with alloy matrix. Then there is a rapid dissolution of the anodic precipitates which
further results in the extension of intergranular crack until there is the occurrence of re-
passivation (Newman, 2002).
Simple active dissolution and elimination of material from the crack tip is the most common
anodic mechanism (Javidi & Horeh, 2014). On the other hand, the most common cathodic
mechanism is absorption, hydrogen evolution, embrittlement and diffusion. The Crack-
Propagation Mechanisms assumes that the occurrence of the breakage in interatomic bonds of
crack tip is the result of either mechanical fracture (brittle or ductile) or chemical solvation and
dissolution. Normal fracture processes are included in the mechanical fracture that is expected to
be induced or stimulated by any of the following interactions between environment and material.
Surface reactions
Surface films
Absorption of ecological species
Responses in metal ahead of crack tip
All the mechanical fractures that have been proposed are found to contain some of these
processes as an important step in the process of stress corrosion cracking.
Surface absorption mechanism suggests that the absorption of active surface components on the
hard surface can subsequently result in reducing the deformation resistance and strength of
solids. In this mechanism, the interatomic bonds are weakened by the absorbed species at the
crack tips thereby resulting in either the promotion of growth in cracks by decohesion or the
facilitation of emission of dislocations and nucleation. The enhance dislocation emission further
leads to the promotion of joining of the crack tip. Consequently, intergranular fracture or brittle
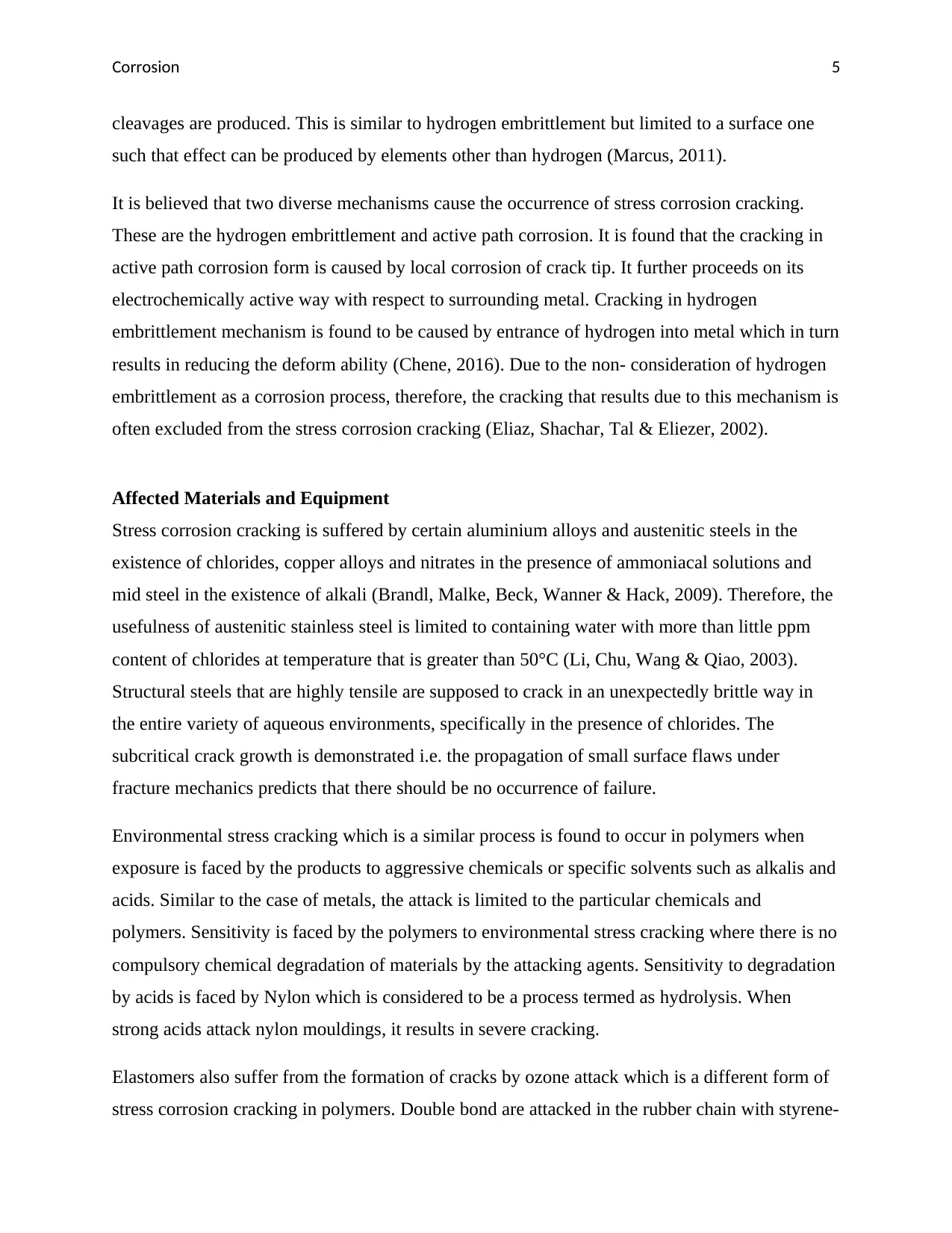
Corrosion 5
cleavages are produced. This is similar to hydrogen embrittlement but limited to a surface one
such that effect can be produced by elements other than hydrogen (Marcus, 2011).
It is believed that two diverse mechanisms cause the occurrence of stress corrosion cracking.
These are the hydrogen embrittlement and active path corrosion. It is found that the cracking in
active path corrosion form is caused by local corrosion of crack tip. It further proceeds on its
electrochemically active way with respect to surrounding metal. Cracking in hydrogen
embrittlement mechanism is found to be caused by entrance of hydrogen into metal which in turn
results in reducing the deform ability (Chene, 2016). Due to the non- consideration of hydrogen
embrittlement as a corrosion process, therefore, the cracking that results due to this mechanism is
often excluded from the stress corrosion cracking (Eliaz, Shachar, Tal & Eliezer, 2002).
Affected Materials and Equipment
Stress corrosion cracking is suffered by certain aluminium alloys and austenitic steels in the
existence of chlorides, copper alloys and nitrates in the presence of ammoniacal solutions and
mid steel in the existence of alkali (Brandl, Malke, Beck, Wanner & Hack, 2009). Therefore, the
usefulness of austenitic stainless steel is limited to containing water with more than little ppm
content of chlorides at temperature that is greater than 50°C (Li, Chu, Wang & Qiao, 2003).
Structural steels that are highly tensile are supposed to crack in an unexpectedly brittle way in
the entire variety of aqueous environments, specifically in the presence of chlorides. The
subcritical crack growth is demonstrated i.e. the propagation of small surface flaws under
fracture mechanics predicts that there should be no occurrence of failure.
Environmental stress cracking which is a similar process is found to occur in polymers when
exposure is faced by the products to aggressive chemicals or specific solvents such as alkalis and
acids. Similar to the case of metals, the attack is limited to the particular chemicals and
polymers. Sensitivity is faced by the polymers to environmental stress cracking where there is no
compulsory chemical degradation of materials by the attacking agents. Sensitivity to degradation
by acids is faced by Nylon which is considered to be a process termed as hydrolysis. When
strong acids attack nylon mouldings, it results in severe cracking.
Elastomers also suffer from the formation of cracks by ozone attack which is a different form of
stress corrosion cracking in polymers. Double bond are attacked in the rubber chain with styrene-
cleavages are produced. This is similar to hydrogen embrittlement but limited to a surface one
such that effect can be produced by elements other than hydrogen (Marcus, 2011).
It is believed that two diverse mechanisms cause the occurrence of stress corrosion cracking.
These are the hydrogen embrittlement and active path corrosion. It is found that the cracking in
active path corrosion form is caused by local corrosion of crack tip. It further proceeds on its
electrochemically active way with respect to surrounding metal. Cracking in hydrogen
embrittlement mechanism is found to be caused by entrance of hydrogen into metal which in turn
results in reducing the deform ability (Chene, 2016). Due to the non- consideration of hydrogen
embrittlement as a corrosion process, therefore, the cracking that results due to this mechanism is
often excluded from the stress corrosion cracking (Eliaz, Shachar, Tal & Eliezer, 2002).
Affected Materials and Equipment
Stress corrosion cracking is suffered by certain aluminium alloys and austenitic steels in the
existence of chlorides, copper alloys and nitrates in the presence of ammoniacal solutions and
mid steel in the existence of alkali (Brandl, Malke, Beck, Wanner & Hack, 2009). Therefore, the
usefulness of austenitic stainless steel is limited to containing water with more than little ppm
content of chlorides at temperature that is greater than 50°C (Li, Chu, Wang & Qiao, 2003).
Structural steels that are highly tensile are supposed to crack in an unexpectedly brittle way in
the entire variety of aqueous environments, specifically in the presence of chlorides. The
subcritical crack growth is demonstrated i.e. the propagation of small surface flaws under
fracture mechanics predicts that there should be no occurrence of failure.
Environmental stress cracking which is a similar process is found to occur in polymers when
exposure is faced by the products to aggressive chemicals or specific solvents such as alkalis and
acids. Similar to the case of metals, the attack is limited to the particular chemicals and
polymers. Sensitivity is faced by the polymers to environmental stress cracking where there is no
compulsory chemical degradation of materials by the attacking agents. Sensitivity to degradation
by acids is faced by Nylon which is considered to be a process termed as hydrolysis. When
strong acids attack nylon mouldings, it results in severe cracking.
Elastomers also suffer from the formation of cracks by ozone attack which is a different form of
stress corrosion cracking in polymers. Double bond are attacked in the rubber chain with styrene-
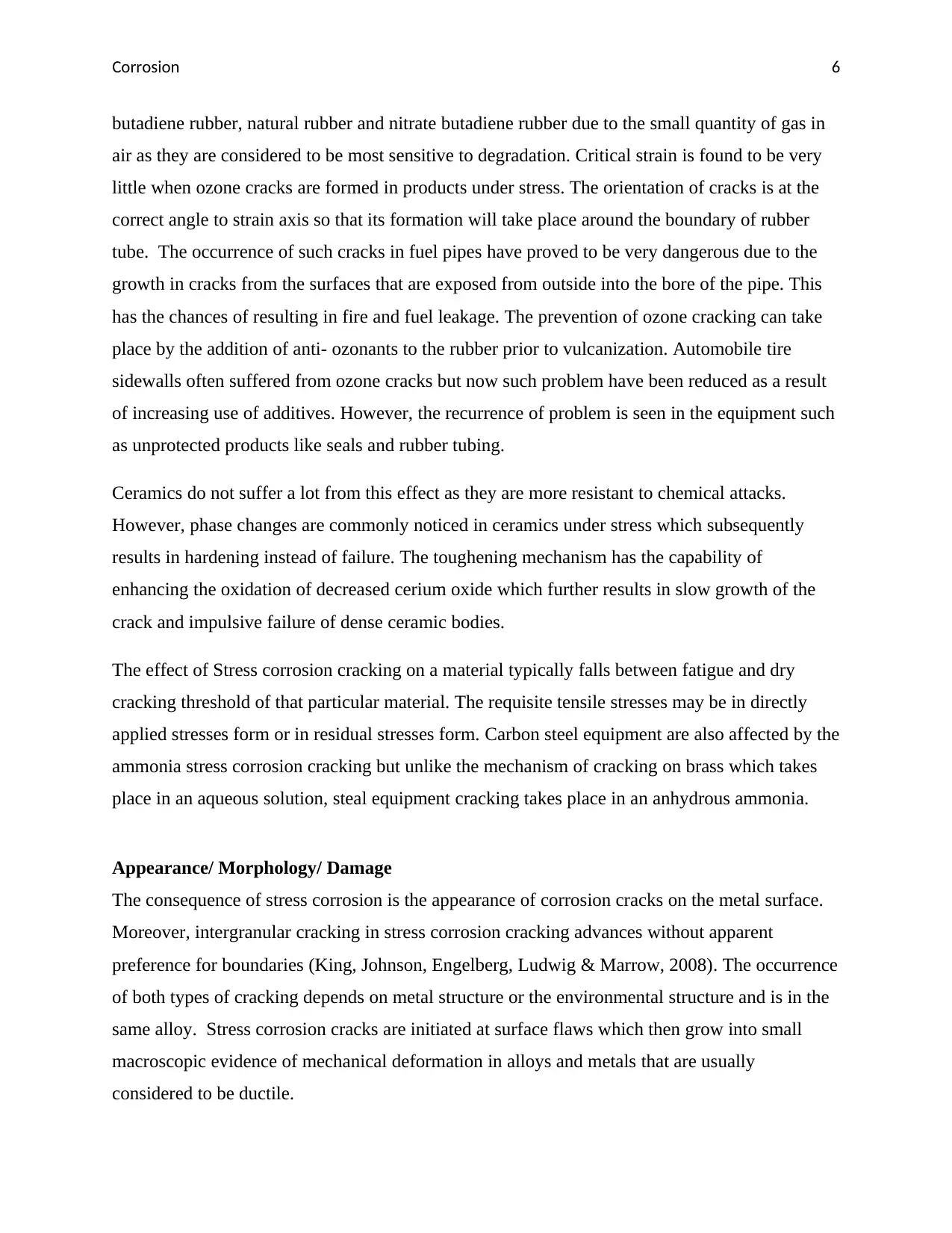
Corrosion 6
butadiene rubber, natural rubber and nitrate butadiene rubber due to the small quantity of gas in
air as they are considered to be most sensitive to degradation. Critical strain is found to be very
little when ozone cracks are formed in products under stress. The orientation of cracks is at the
correct angle to strain axis so that its formation will take place around the boundary of rubber
tube. The occurrence of such cracks in fuel pipes have proved to be very dangerous due to the
growth in cracks from the surfaces that are exposed from outside into the bore of the pipe. This
has the chances of resulting in fire and fuel leakage. The prevention of ozone cracking can take
place by the addition of anti- ozonants to the rubber prior to vulcanization. Automobile tire
sidewalls often suffered from ozone cracks but now such problem have been reduced as a result
of increasing use of additives. However, the recurrence of problem is seen in the equipment such
as unprotected products like seals and rubber tubing.
Ceramics do not suffer a lot from this effect as they are more resistant to chemical attacks.
However, phase changes are commonly noticed in ceramics under stress which subsequently
results in hardening instead of failure. The toughening mechanism has the capability of
enhancing the oxidation of decreased cerium oxide which further results in slow growth of the
crack and impulsive failure of dense ceramic bodies.
The effect of Stress corrosion cracking on a material typically falls between fatigue and dry
cracking threshold of that particular material. The requisite tensile stresses may be in directly
applied stresses form or in residual stresses form. Carbon steel equipment are also affected by the
ammonia stress corrosion cracking but unlike the mechanism of cracking on brass which takes
place in an aqueous solution, steal equipment cracking takes place in an anhydrous ammonia.
Appearance/ Morphology/ Damage
The consequence of stress corrosion is the appearance of corrosion cracks on the metal surface.
Moreover, intergranular cracking in stress corrosion cracking advances without apparent
preference for boundaries (King, Johnson, Engelberg, Ludwig & Marrow, 2008). The occurrence
of both types of cracking depends on metal structure or the environmental structure and is in the
same alloy. Stress corrosion cracks are initiated at surface flaws which then grow into small
macroscopic evidence of mechanical deformation in alloys and metals that are usually
considered to be ductile.
butadiene rubber, natural rubber and nitrate butadiene rubber due to the small quantity of gas in
air as they are considered to be most sensitive to degradation. Critical strain is found to be very
little when ozone cracks are formed in products under stress. The orientation of cracks is at the
correct angle to strain axis so that its formation will take place around the boundary of rubber
tube. The occurrence of such cracks in fuel pipes have proved to be very dangerous due to the
growth in cracks from the surfaces that are exposed from outside into the bore of the pipe. This
has the chances of resulting in fire and fuel leakage. The prevention of ozone cracking can take
place by the addition of anti- ozonants to the rubber prior to vulcanization. Automobile tire
sidewalls often suffered from ozone cracks but now such problem have been reduced as a result
of increasing use of additives. However, the recurrence of problem is seen in the equipment such
as unprotected products like seals and rubber tubing.
Ceramics do not suffer a lot from this effect as they are more resistant to chemical attacks.
However, phase changes are commonly noticed in ceramics under stress which subsequently
results in hardening instead of failure. The toughening mechanism has the capability of
enhancing the oxidation of decreased cerium oxide which further results in slow growth of the
crack and impulsive failure of dense ceramic bodies.
The effect of Stress corrosion cracking on a material typically falls between fatigue and dry
cracking threshold of that particular material. The requisite tensile stresses may be in directly
applied stresses form or in residual stresses form. Carbon steel equipment are also affected by the
ammonia stress corrosion cracking but unlike the mechanism of cracking on brass which takes
place in an aqueous solution, steal equipment cracking takes place in an anhydrous ammonia.
Appearance/ Morphology/ Damage
The consequence of stress corrosion is the appearance of corrosion cracks on the metal surface.
Moreover, intergranular cracking in stress corrosion cracking advances without apparent
preference for boundaries (King, Johnson, Engelberg, Ludwig & Marrow, 2008). The occurrence
of both types of cracking depends on metal structure or the environmental structure and is in the
same alloy. Stress corrosion cracks are initiated at surface flaws which then grow into small
macroscopic evidence of mechanical deformation in alloys and metals that are usually
considered to be ductile.
Secure Best Marks with AI Grader
Need help grading? Try our AI Grader for instant feedback on your assignments.
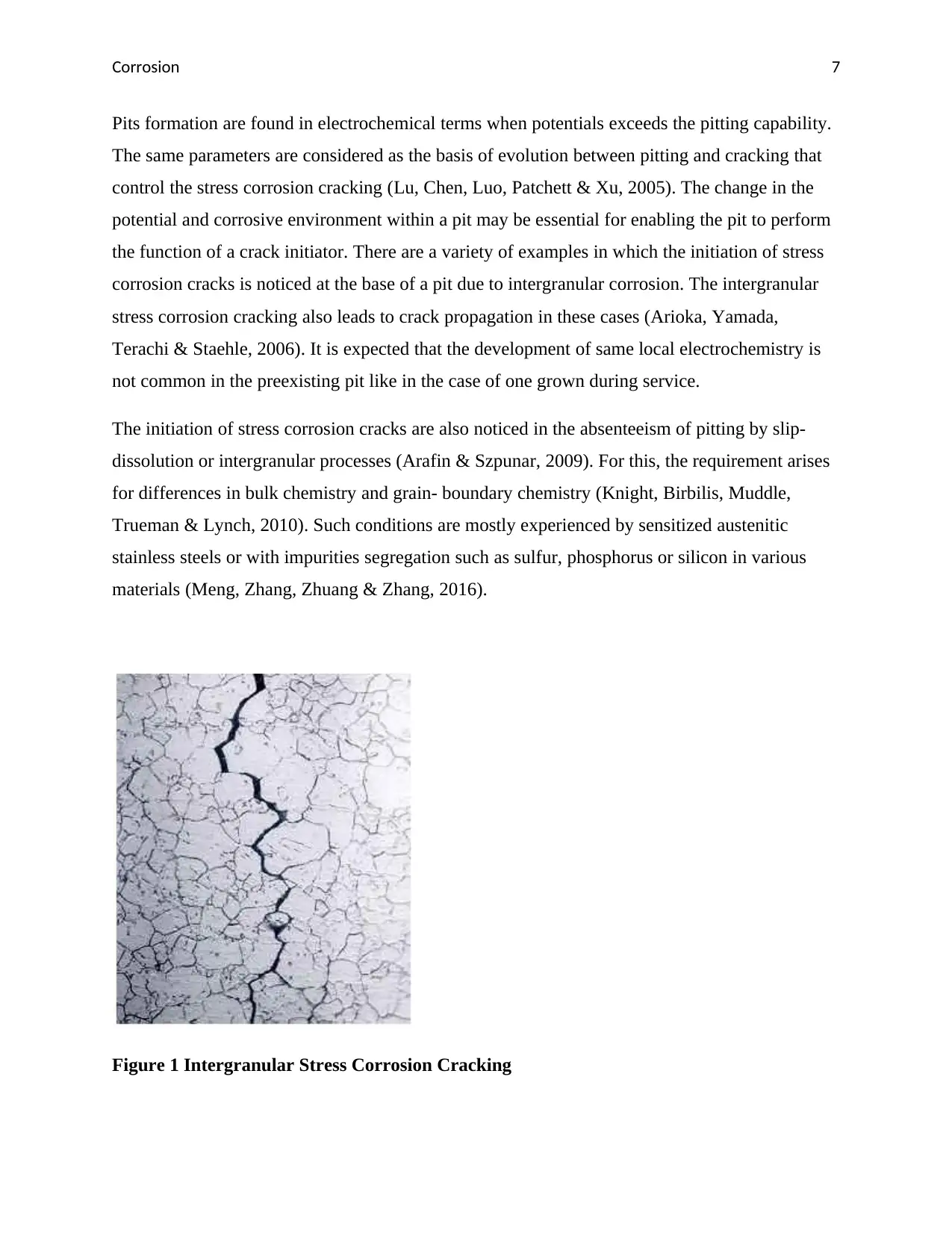
Corrosion 7
Pits formation are found in electrochemical terms when potentials exceeds the pitting capability.
The same parameters are considered as the basis of evolution between pitting and cracking that
control the stress corrosion cracking (Lu, Chen, Luo, Patchett & Xu, 2005). The change in the
potential and corrosive environment within a pit may be essential for enabling the pit to perform
the function of a crack initiator. There are a variety of examples in which the initiation of stress
corrosion cracks is noticed at the base of a pit due to intergranular corrosion. The intergranular
stress corrosion cracking also leads to crack propagation in these cases (Arioka, Yamada,
Terachi & Staehle, 2006). It is expected that the development of same local electrochemistry is
not common in the preexisting pit like in the case of one grown during service.
The initiation of stress corrosion cracks are also noticed in the absenteeism of pitting by slip-
dissolution or intergranular processes (Arafin & Szpunar, 2009). For this, the requirement arises
for differences in bulk chemistry and grain- boundary chemistry (Knight, Birbilis, Muddle,
Trueman & Lynch, 2010). Such conditions are mostly experienced by sensitized austenitic
stainless steels or with impurities segregation such as sulfur, phosphorus or silicon in various
materials (Meng, Zhang, Zhuang & Zhang, 2016).
Figure 1 Intergranular Stress Corrosion Cracking
Pits formation are found in electrochemical terms when potentials exceeds the pitting capability.
The same parameters are considered as the basis of evolution between pitting and cracking that
control the stress corrosion cracking (Lu, Chen, Luo, Patchett & Xu, 2005). The change in the
potential and corrosive environment within a pit may be essential for enabling the pit to perform
the function of a crack initiator. There are a variety of examples in which the initiation of stress
corrosion cracks is noticed at the base of a pit due to intergranular corrosion. The intergranular
stress corrosion cracking also leads to crack propagation in these cases (Arioka, Yamada,
Terachi & Staehle, 2006). It is expected that the development of same local electrochemistry is
not common in the preexisting pit like in the case of one grown during service.
The initiation of stress corrosion cracks are also noticed in the absenteeism of pitting by slip-
dissolution or intergranular processes (Arafin & Szpunar, 2009). For this, the requirement arises
for differences in bulk chemistry and grain- boundary chemistry (Knight, Birbilis, Muddle,
Trueman & Lynch, 2010). Such conditions are mostly experienced by sensitized austenitic
stainless steels or with impurities segregation such as sulfur, phosphorus or silicon in various
materials (Meng, Zhang, Zhuang & Zhang, 2016).
Figure 1 Intergranular Stress Corrosion Cracking
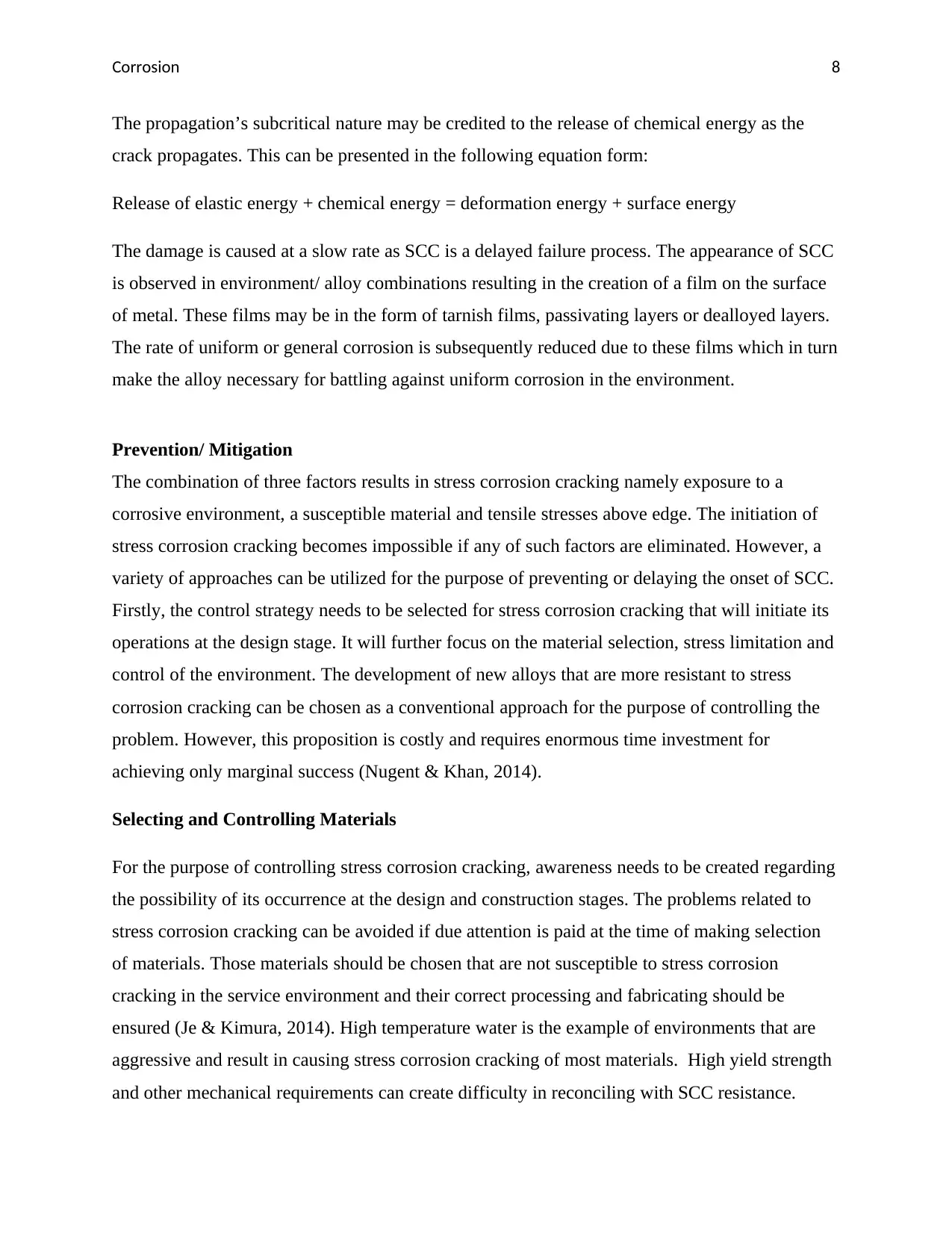
Corrosion 8
The propagation’s subcritical nature may be credited to the release of chemical energy as the
crack propagates. This can be presented in the following equation form:
Release of elastic energy + chemical energy = deformation energy + surface energy
The damage is caused at a slow rate as SCC is a delayed failure process. The appearance of SCC
is observed in environment/ alloy combinations resulting in the creation of a film on the surface
of metal. These films may be in the form of tarnish films, passivating layers or dealloyed layers.
The rate of uniform or general corrosion is subsequently reduced due to these films which in turn
make the alloy necessary for battling against uniform corrosion in the environment.
Prevention/ Mitigation
The combination of three factors results in stress corrosion cracking namely exposure to a
corrosive environment, a susceptible material and tensile stresses above edge. The initiation of
stress corrosion cracking becomes impossible if any of such factors are eliminated. However, a
variety of approaches can be utilized for the purpose of preventing or delaying the onset of SCC.
Firstly, the control strategy needs to be selected for stress corrosion cracking that will initiate its
operations at the design stage. It will further focus on the material selection, stress limitation and
control of the environment. The development of new alloys that are more resistant to stress
corrosion cracking can be chosen as a conventional approach for the purpose of controlling the
problem. However, this proposition is costly and requires enormous time investment for
achieving only marginal success (Nugent & Khan, 2014).
Selecting and Controlling Materials
For the purpose of controlling stress corrosion cracking, awareness needs to be created regarding
the possibility of its occurrence at the design and construction stages. The problems related to
stress corrosion cracking can be avoided if due attention is paid at the time of making selection
of materials. Those materials should be chosen that are not susceptible to stress corrosion
cracking in the service environment and their correct processing and fabricating should be
ensured (Je & Kimura, 2014). High temperature water is the example of environments that are
aggressive and result in causing stress corrosion cracking of most materials. High yield strength
and other mechanical requirements can create difficulty in reconciling with SCC resistance.
The propagation’s subcritical nature may be credited to the release of chemical energy as the
crack propagates. This can be presented in the following equation form:
Release of elastic energy + chemical energy = deformation energy + surface energy
The damage is caused at a slow rate as SCC is a delayed failure process. The appearance of SCC
is observed in environment/ alloy combinations resulting in the creation of a film on the surface
of metal. These films may be in the form of tarnish films, passivating layers or dealloyed layers.
The rate of uniform or general corrosion is subsequently reduced due to these films which in turn
make the alloy necessary for battling against uniform corrosion in the environment.
Prevention/ Mitigation
The combination of three factors results in stress corrosion cracking namely exposure to a
corrosive environment, a susceptible material and tensile stresses above edge. The initiation of
stress corrosion cracking becomes impossible if any of such factors are eliminated. However, a
variety of approaches can be utilized for the purpose of preventing or delaying the onset of SCC.
Firstly, the control strategy needs to be selected for stress corrosion cracking that will initiate its
operations at the design stage. It will further focus on the material selection, stress limitation and
control of the environment. The development of new alloys that are more resistant to stress
corrosion cracking can be chosen as a conventional approach for the purpose of controlling the
problem. However, this proposition is costly and requires enormous time investment for
achieving only marginal success (Nugent & Khan, 2014).
Selecting and Controlling Materials
For the purpose of controlling stress corrosion cracking, awareness needs to be created regarding
the possibility of its occurrence at the design and construction stages. The problems related to
stress corrosion cracking can be avoided if due attention is paid at the time of making selection
of materials. Those materials should be chosen that are not susceptible to stress corrosion
cracking in the service environment and their correct processing and fabricating should be
ensured (Je & Kimura, 2014). High temperature water is the example of environments that are
aggressive and result in causing stress corrosion cracking of most materials. High yield strength
and other mechanical requirements can create difficulty in reconciling with SCC resistance.
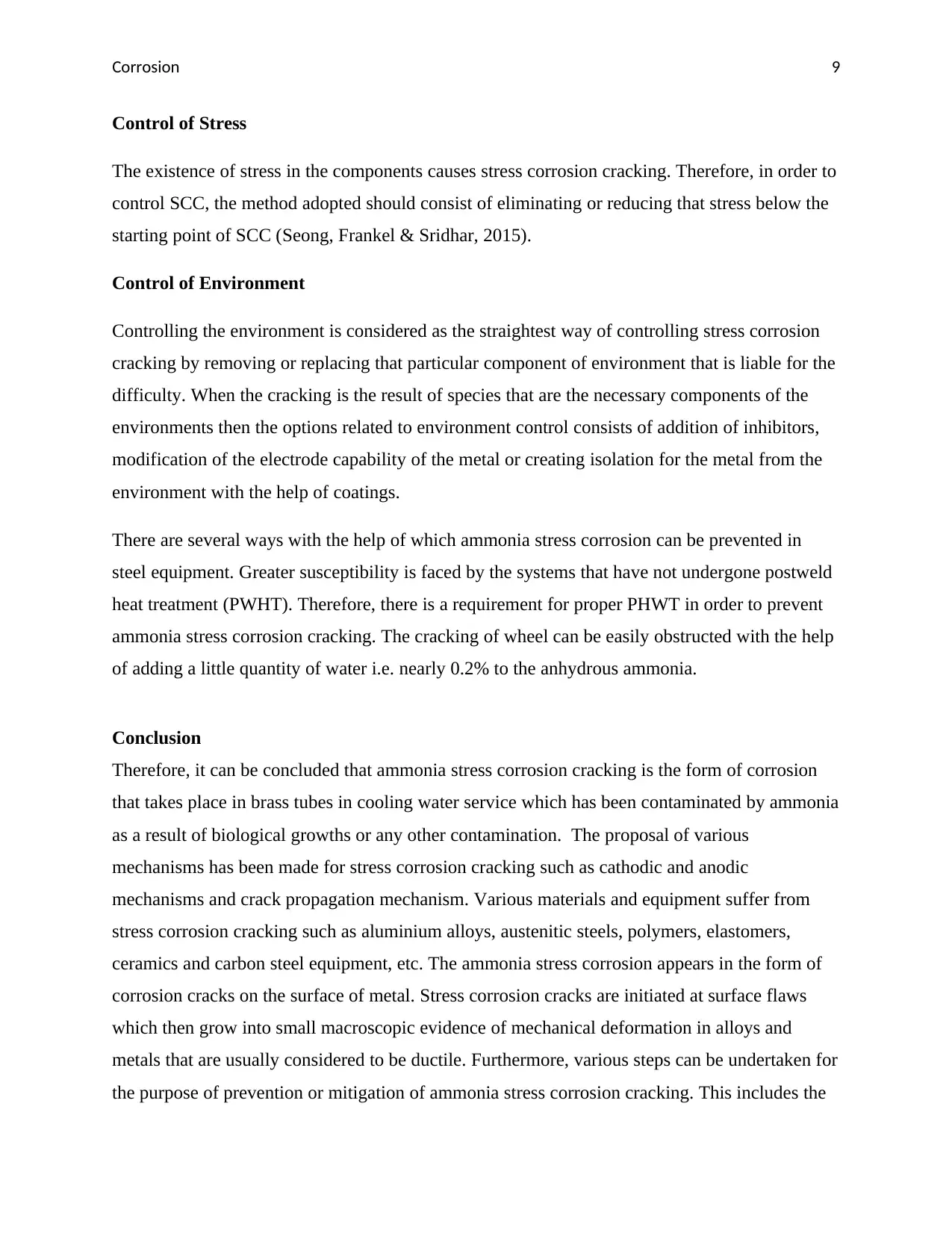
Corrosion 9
Control of Stress
The existence of stress in the components causes stress corrosion cracking. Therefore, in order to
control SCC, the method adopted should consist of eliminating or reducing that stress below the
starting point of SCC (Seong, Frankel & Sridhar, 2015).
Control of Environment
Controlling the environment is considered as the straightest way of controlling stress corrosion
cracking by removing or replacing that particular component of environment that is liable for the
difficulty. When the cracking is the result of species that are the necessary components of the
environments then the options related to environment control consists of addition of inhibitors,
modification of the electrode capability of the metal or creating isolation for the metal from the
environment with the help of coatings.
There are several ways with the help of which ammonia stress corrosion can be prevented in
steel equipment. Greater susceptibility is faced by the systems that have not undergone postweld
heat treatment (PWHT). Therefore, there is a requirement for proper PHWT in order to prevent
ammonia stress corrosion cracking. The cracking of wheel can be easily obstructed with the help
of adding a little quantity of water i.e. nearly 0.2% to the anhydrous ammonia.
Conclusion
Therefore, it can be concluded that ammonia stress corrosion cracking is the form of corrosion
that takes place in brass tubes in cooling water service which has been contaminated by ammonia
as a result of biological growths or any other contamination. The proposal of various
mechanisms has been made for stress corrosion cracking such as cathodic and anodic
mechanisms and crack propagation mechanism. Various materials and equipment suffer from
stress corrosion cracking such as aluminium alloys, austenitic steels, polymers, elastomers,
ceramics and carbon steel equipment, etc. The ammonia stress corrosion appears in the form of
corrosion cracks on the surface of metal. Stress corrosion cracks are initiated at surface flaws
which then grow into small macroscopic evidence of mechanical deformation in alloys and
metals that are usually considered to be ductile. Furthermore, various steps can be undertaken for
the purpose of prevention or mitigation of ammonia stress corrosion cracking. This includes the
Control of Stress
The existence of stress in the components causes stress corrosion cracking. Therefore, in order to
control SCC, the method adopted should consist of eliminating or reducing that stress below the
starting point of SCC (Seong, Frankel & Sridhar, 2015).
Control of Environment
Controlling the environment is considered as the straightest way of controlling stress corrosion
cracking by removing or replacing that particular component of environment that is liable for the
difficulty. When the cracking is the result of species that are the necessary components of the
environments then the options related to environment control consists of addition of inhibitors,
modification of the electrode capability of the metal or creating isolation for the metal from the
environment with the help of coatings.
There are several ways with the help of which ammonia stress corrosion can be prevented in
steel equipment. Greater susceptibility is faced by the systems that have not undergone postweld
heat treatment (PWHT). Therefore, there is a requirement for proper PHWT in order to prevent
ammonia stress corrosion cracking. The cracking of wheel can be easily obstructed with the help
of adding a little quantity of water i.e. nearly 0.2% to the anhydrous ammonia.
Conclusion
Therefore, it can be concluded that ammonia stress corrosion cracking is the form of corrosion
that takes place in brass tubes in cooling water service which has been contaminated by ammonia
as a result of biological growths or any other contamination. The proposal of various
mechanisms has been made for stress corrosion cracking such as cathodic and anodic
mechanisms and crack propagation mechanism. Various materials and equipment suffer from
stress corrosion cracking such as aluminium alloys, austenitic steels, polymers, elastomers,
ceramics and carbon steel equipment, etc. The ammonia stress corrosion appears in the form of
corrosion cracks on the surface of metal. Stress corrosion cracks are initiated at surface flaws
which then grow into small macroscopic evidence of mechanical deformation in alloys and
metals that are usually considered to be ductile. Furthermore, various steps can be undertaken for
the purpose of prevention or mitigation of ammonia stress corrosion cracking. This includes the
Paraphrase This Document
Need a fresh take? Get an instant paraphrase of this document with our AI Paraphraser
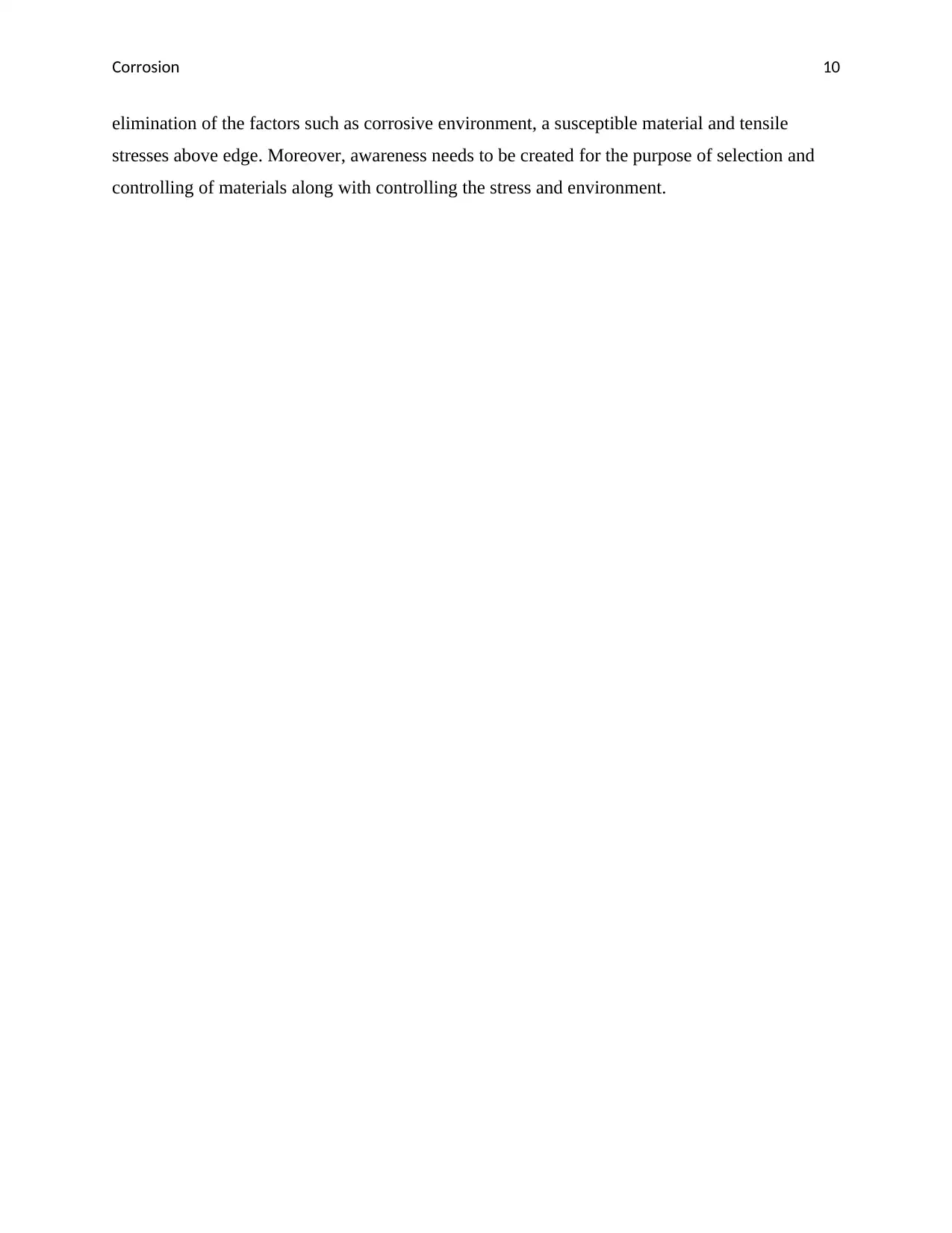
Corrosion 10
elimination of the factors such as corrosive environment, a susceptible material and tensile
stresses above edge. Moreover, awareness needs to be created for the purpose of selection and
controlling of materials along with controlling the stress and environment.
elimination of the factors such as corrosive environment, a susceptible material and tensile
stresses above edge. Moreover, awareness needs to be created for the purpose of selection and
controlling of materials along with controlling the stress and environment.
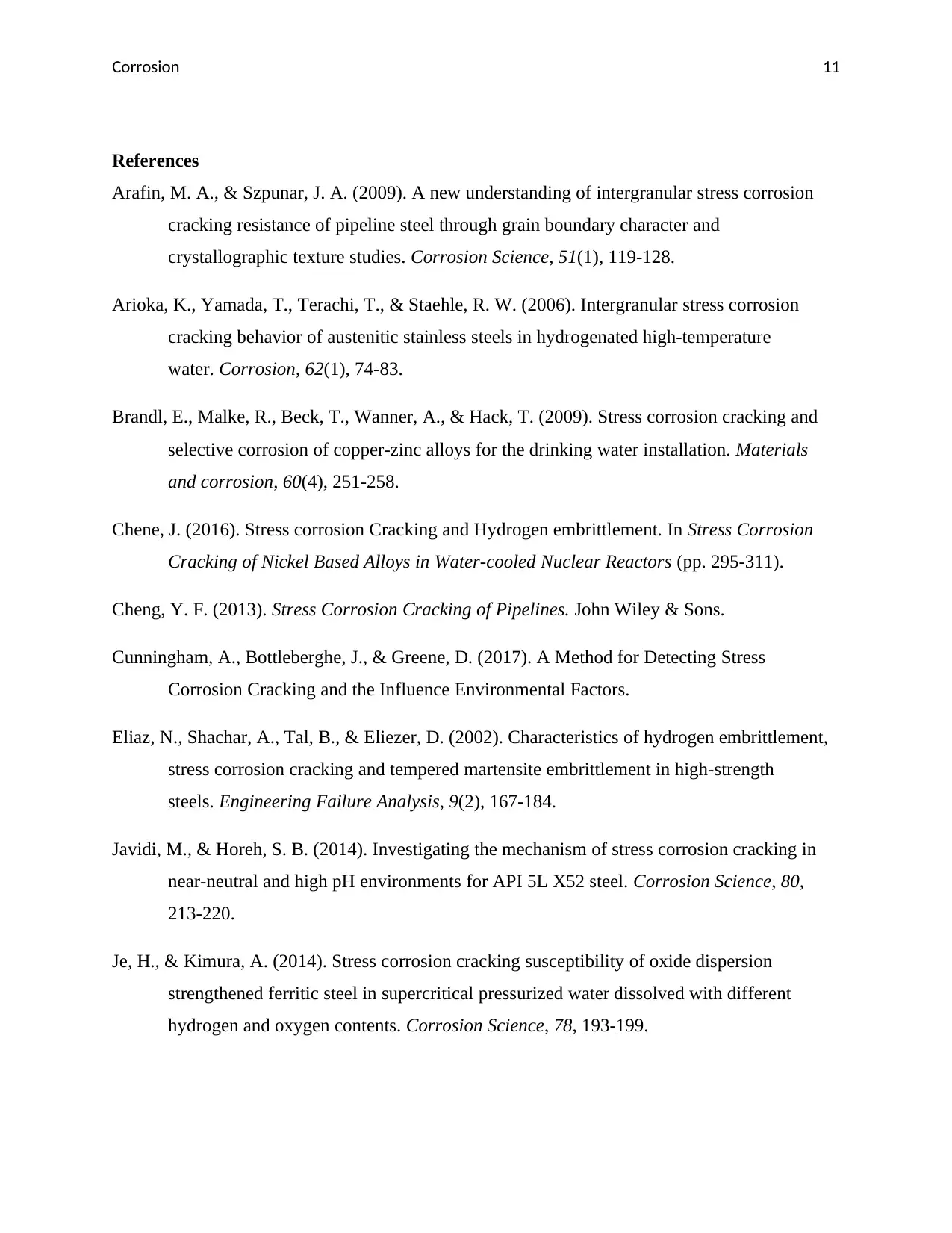
Corrosion 11
References
Arafin, M. A., & Szpunar, J. A. (2009). A new understanding of intergranular stress corrosion
cracking resistance of pipeline steel through grain boundary character and
crystallographic texture studies. Corrosion Science, 51(1), 119-128.
Arioka, K., Yamada, T., Terachi, T., & Staehle, R. W. (2006). Intergranular stress corrosion
cracking behavior of austenitic stainless steels in hydrogenated high-temperature
water. Corrosion, 62(1), 74-83.
Brandl, E., Malke, R., Beck, T., Wanner, A., & Hack, T. (2009). Stress corrosion cracking and
selective corrosion of copper‐zinc alloys for the drinking water installation. Materials
and corrosion, 60(4), 251-258.
Chene, J. (2016). Stress corrosion Cracking and Hydrogen embrittlement. In Stress Corrosion
Cracking of Nickel Based Alloys in Water-cooled Nuclear Reactors (pp. 295-311).
Cheng, Y. F. (2013). Stress Corrosion Cracking of Pipelines. John Wiley & Sons.
Cunningham, A., Bottleberghe, J., & Greene, D. (2017). A Method for Detecting Stress
Corrosion Cracking and the Influence Environmental Factors.
Eliaz, N., Shachar, A., Tal, B., & Eliezer, D. (2002). Characteristics of hydrogen embrittlement,
stress corrosion cracking and tempered martensite embrittlement in high-strength
steels. Engineering Failure Analysis, 9(2), 167-184.
Javidi, M., & Horeh, S. B. (2014). Investigating the mechanism of stress corrosion cracking in
near-neutral and high pH environments for API 5L X52 steel. Corrosion Science, 80,
213-220.
Je, H., & Kimura, A. (2014). Stress corrosion cracking susceptibility of oxide dispersion
strengthened ferritic steel in supercritical pressurized water dissolved with different
hydrogen and oxygen contents. Corrosion Science, 78, 193-199.
References
Arafin, M. A., & Szpunar, J. A. (2009). A new understanding of intergranular stress corrosion
cracking resistance of pipeline steel through grain boundary character and
crystallographic texture studies. Corrosion Science, 51(1), 119-128.
Arioka, K., Yamada, T., Terachi, T., & Staehle, R. W. (2006). Intergranular stress corrosion
cracking behavior of austenitic stainless steels in hydrogenated high-temperature
water. Corrosion, 62(1), 74-83.
Brandl, E., Malke, R., Beck, T., Wanner, A., & Hack, T. (2009). Stress corrosion cracking and
selective corrosion of copper‐zinc alloys for the drinking water installation. Materials
and corrosion, 60(4), 251-258.
Chene, J. (2016). Stress corrosion Cracking and Hydrogen embrittlement. In Stress Corrosion
Cracking of Nickel Based Alloys in Water-cooled Nuclear Reactors (pp. 295-311).
Cheng, Y. F. (2013). Stress Corrosion Cracking of Pipelines. John Wiley & Sons.
Cunningham, A., Bottleberghe, J., & Greene, D. (2017). A Method for Detecting Stress
Corrosion Cracking and the Influence Environmental Factors.
Eliaz, N., Shachar, A., Tal, B., & Eliezer, D. (2002). Characteristics of hydrogen embrittlement,
stress corrosion cracking and tempered martensite embrittlement in high-strength
steels. Engineering Failure Analysis, 9(2), 167-184.
Javidi, M., & Horeh, S. B. (2014). Investigating the mechanism of stress corrosion cracking in
near-neutral and high pH environments for API 5L X52 steel. Corrosion Science, 80,
213-220.
Je, H., & Kimura, A. (2014). Stress corrosion cracking susceptibility of oxide dispersion
strengthened ferritic steel in supercritical pressurized water dissolved with different
hydrogen and oxygen contents. Corrosion Science, 78, 193-199.
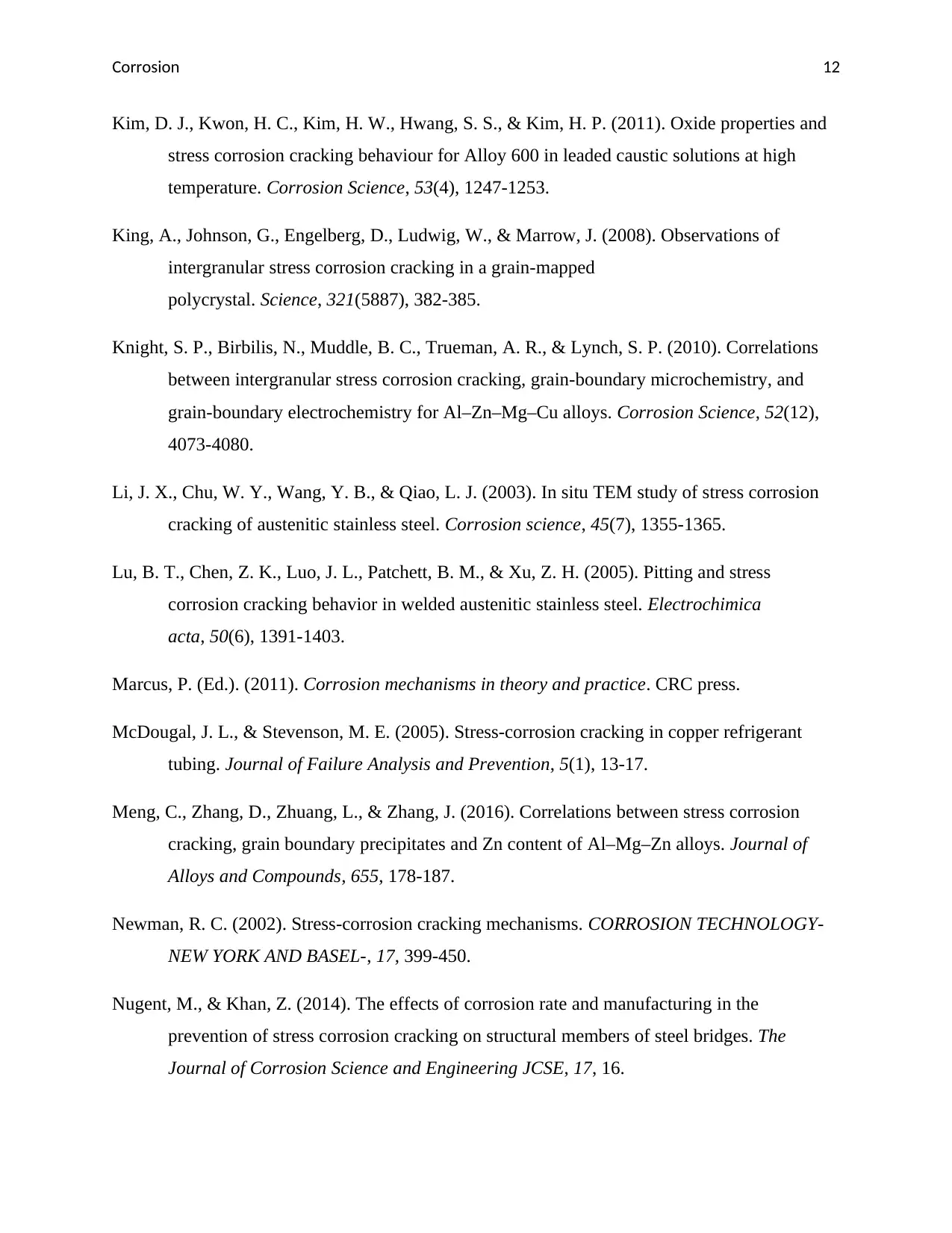
Corrosion 12
Kim, D. J., Kwon, H. C., Kim, H. W., Hwang, S. S., & Kim, H. P. (2011). Oxide properties and
stress corrosion cracking behaviour for Alloy 600 in leaded caustic solutions at high
temperature. Corrosion Science, 53(4), 1247-1253.
King, A., Johnson, G., Engelberg, D., Ludwig, W., & Marrow, J. (2008). Observations of
intergranular stress corrosion cracking in a grain-mapped
polycrystal. Science, 321(5887), 382-385.
Knight, S. P., Birbilis, N., Muddle, B. C., Trueman, A. R., & Lynch, S. P. (2010). Correlations
between intergranular stress corrosion cracking, grain-boundary microchemistry, and
grain-boundary electrochemistry for Al–Zn–Mg–Cu alloys. Corrosion Science, 52(12),
4073-4080.
Li, J. X., Chu, W. Y., Wang, Y. B., & Qiao, L. J. (2003). In situ TEM study of stress corrosion
cracking of austenitic stainless steel. Corrosion science, 45(7), 1355-1365.
Lu, B. T., Chen, Z. K., Luo, J. L., Patchett, B. M., & Xu, Z. H. (2005). Pitting and stress
corrosion cracking behavior in welded austenitic stainless steel. Electrochimica
acta, 50(6), 1391-1403.
Marcus, P. (Ed.). (2011). Corrosion mechanisms in theory and practice. CRC press.
McDougal, J. L., & Stevenson, M. E. (2005). Stress-corrosion cracking in copper refrigerant
tubing. Journal of Failure Analysis and Prevention, 5(1), 13-17.
Meng, C., Zhang, D., Zhuang, L., & Zhang, J. (2016). Correlations between stress corrosion
cracking, grain boundary precipitates and Zn content of Al–Mg–Zn alloys. Journal of
Alloys and Compounds, 655, 178-187.
Newman, R. C. (2002). Stress-corrosion cracking mechanisms. CORROSION TECHNOLOGY-
NEW YORK AND BASEL-, 17, 399-450.
Nugent, M., & Khan, Z. (2014). The effects of corrosion rate and manufacturing in the
prevention of stress corrosion cracking on structural members of steel bridges. The
Journal of Corrosion Science and Engineering JCSE, 17, 16.
Kim, D. J., Kwon, H. C., Kim, H. W., Hwang, S. S., & Kim, H. P. (2011). Oxide properties and
stress corrosion cracking behaviour for Alloy 600 in leaded caustic solutions at high
temperature. Corrosion Science, 53(4), 1247-1253.
King, A., Johnson, G., Engelberg, D., Ludwig, W., & Marrow, J. (2008). Observations of
intergranular stress corrosion cracking in a grain-mapped
polycrystal. Science, 321(5887), 382-385.
Knight, S. P., Birbilis, N., Muddle, B. C., Trueman, A. R., & Lynch, S. P. (2010). Correlations
between intergranular stress corrosion cracking, grain-boundary microchemistry, and
grain-boundary electrochemistry for Al–Zn–Mg–Cu alloys. Corrosion Science, 52(12),
4073-4080.
Li, J. X., Chu, W. Y., Wang, Y. B., & Qiao, L. J. (2003). In situ TEM study of stress corrosion
cracking of austenitic stainless steel. Corrosion science, 45(7), 1355-1365.
Lu, B. T., Chen, Z. K., Luo, J. L., Patchett, B. M., & Xu, Z. H. (2005). Pitting and stress
corrosion cracking behavior in welded austenitic stainless steel. Electrochimica
acta, 50(6), 1391-1403.
Marcus, P. (Ed.). (2011). Corrosion mechanisms in theory and practice. CRC press.
McDougal, J. L., & Stevenson, M. E. (2005). Stress-corrosion cracking in copper refrigerant
tubing. Journal of Failure Analysis and Prevention, 5(1), 13-17.
Meng, C., Zhang, D., Zhuang, L., & Zhang, J. (2016). Correlations between stress corrosion
cracking, grain boundary precipitates and Zn content of Al–Mg–Zn alloys. Journal of
Alloys and Compounds, 655, 178-187.
Newman, R. C. (2002). Stress-corrosion cracking mechanisms. CORROSION TECHNOLOGY-
NEW YORK AND BASEL-, 17, 399-450.
Nugent, M., & Khan, Z. (2014). The effects of corrosion rate and manufacturing in the
prevention of stress corrosion cracking on structural members of steel bridges. The
Journal of Corrosion Science and Engineering JCSE, 17, 16.
Secure Best Marks with AI Grader
Need help grading? Try our AI Grader for instant feedback on your assignments.
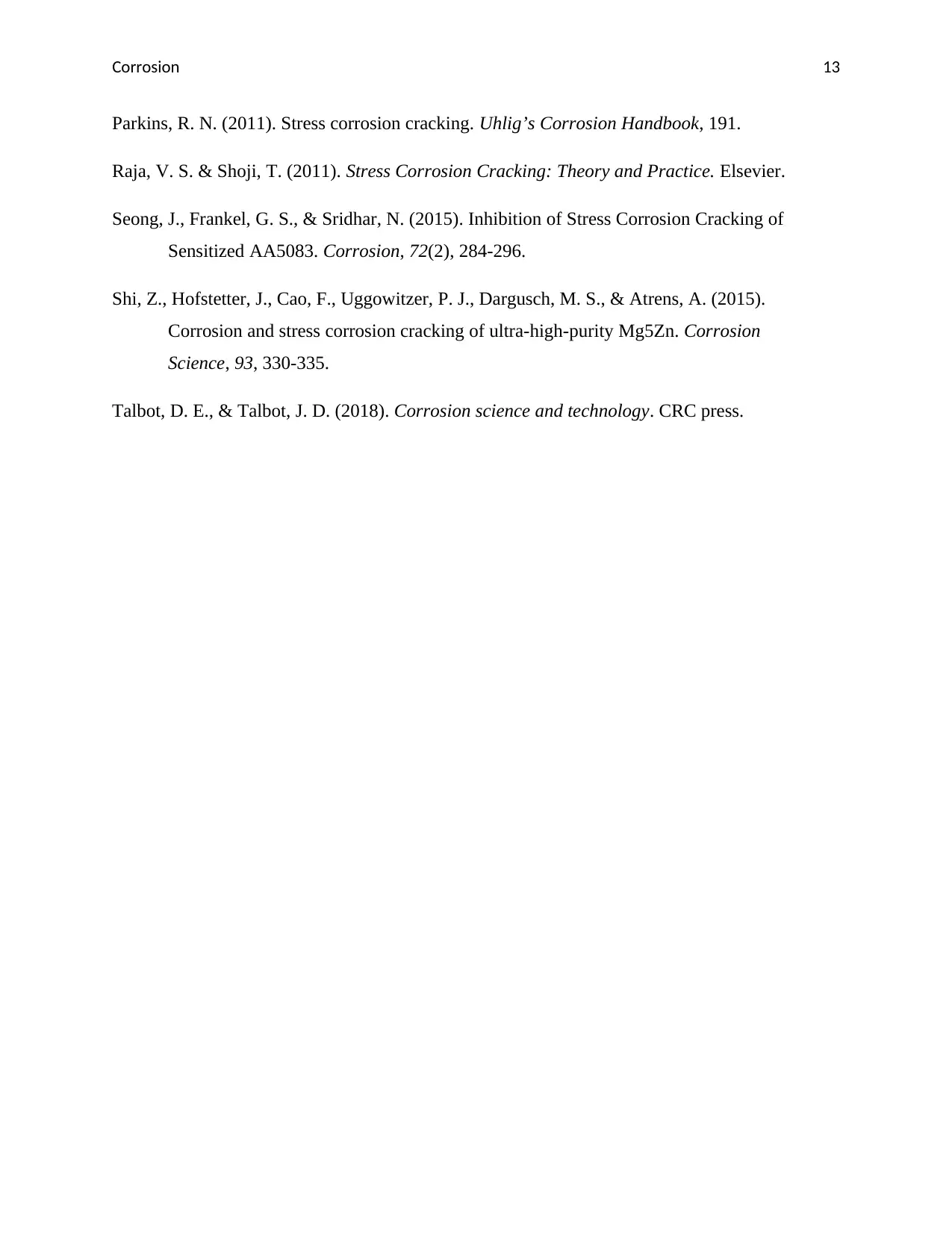
Corrosion 13
Parkins, R. N. (2011). Stress corrosion cracking. Uhlig’s Corrosion Handbook, 191.
Raja, V. S. & Shoji, T. (2011). Stress Corrosion Cracking: Theory and Practice. Elsevier.
Seong, J., Frankel, G. S., & Sridhar, N. (2015). Inhibition of Stress Corrosion Cracking of
Sensitized AA5083. Corrosion, 72(2), 284-296.
Shi, Z., Hofstetter, J., Cao, F., Uggowitzer, P. J., Dargusch, M. S., & Atrens, A. (2015).
Corrosion and stress corrosion cracking of ultra-high-purity Mg5Zn. Corrosion
Science, 93, 330-335.
Talbot, D. E., & Talbot, J. D. (2018). Corrosion science and technology. CRC press.
Parkins, R. N. (2011). Stress corrosion cracking. Uhlig’s Corrosion Handbook, 191.
Raja, V. S. & Shoji, T. (2011). Stress Corrosion Cracking: Theory and Practice. Elsevier.
Seong, J., Frankel, G. S., & Sridhar, N. (2015). Inhibition of Stress Corrosion Cracking of
Sensitized AA5083. Corrosion, 72(2), 284-296.
Shi, Z., Hofstetter, J., Cao, F., Uggowitzer, P. J., Dargusch, M. S., & Atrens, A. (2015).
Corrosion and stress corrosion cracking of ultra-high-purity Mg5Zn. Corrosion
Science, 93, 330-335.
Talbot, D. E., & Talbot, J. D. (2018). Corrosion science and technology. CRC press.
1 out of 14
![[object Object]](/_next/image/?url=%2F_next%2Fstatic%2Fmedia%2Flogo.6d15ce61.png&w=640&q=75)
Your All-in-One AI-Powered Toolkit for Academic Success.
+13062052269
info@desklib.com
Available 24*7 on WhatsApp / Email
Unlock your academic potential
© 2024 | Zucol Services PVT LTD | All rights reserved.