Assignment on Industry Affiliates Program
VerifiedAdded on 2022/09/28
|54
|18940
|17
Assignment
AI Summary
Contribute Materials
Your contribution can guide someone’s learning journey. Share your
documents today.
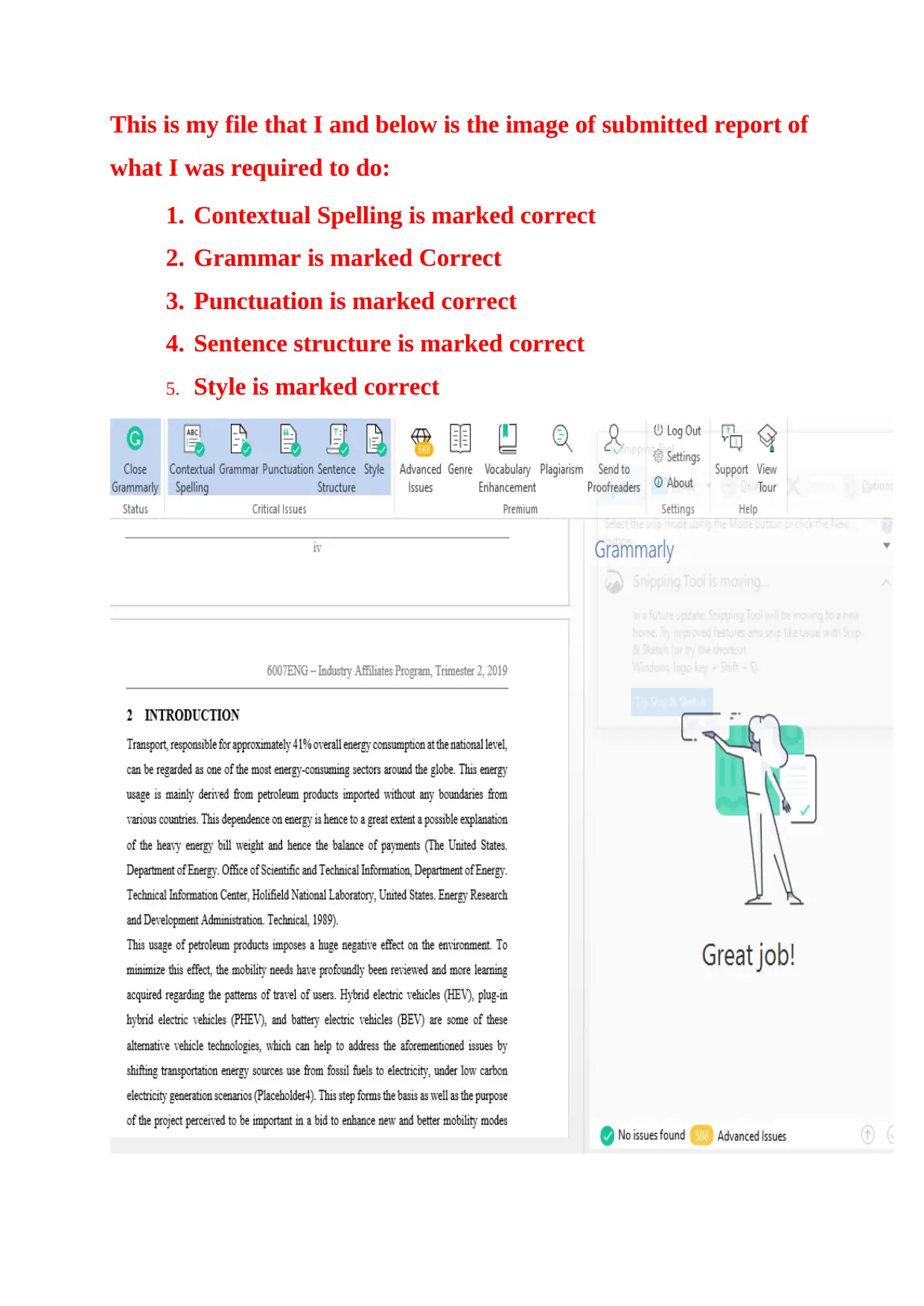
This is my file that I and below is the image of submitted report of
what I was required to do:
1. Contextual Spelling is marked correct
2. Grammar is marked Correct
3. Punctuation is marked correct
4. Sentence structure is marked correct
5. Style is marked correct
what I was required to do:
1. Contextual Spelling is marked correct
2. Grammar is marked Correct
3. Punctuation is marked correct
4. Sentence structure is marked correct
5. Style is marked correct
Secure Best Marks with AI Grader
Need help grading? Try our AI Grader for instant feedback on your assignments.
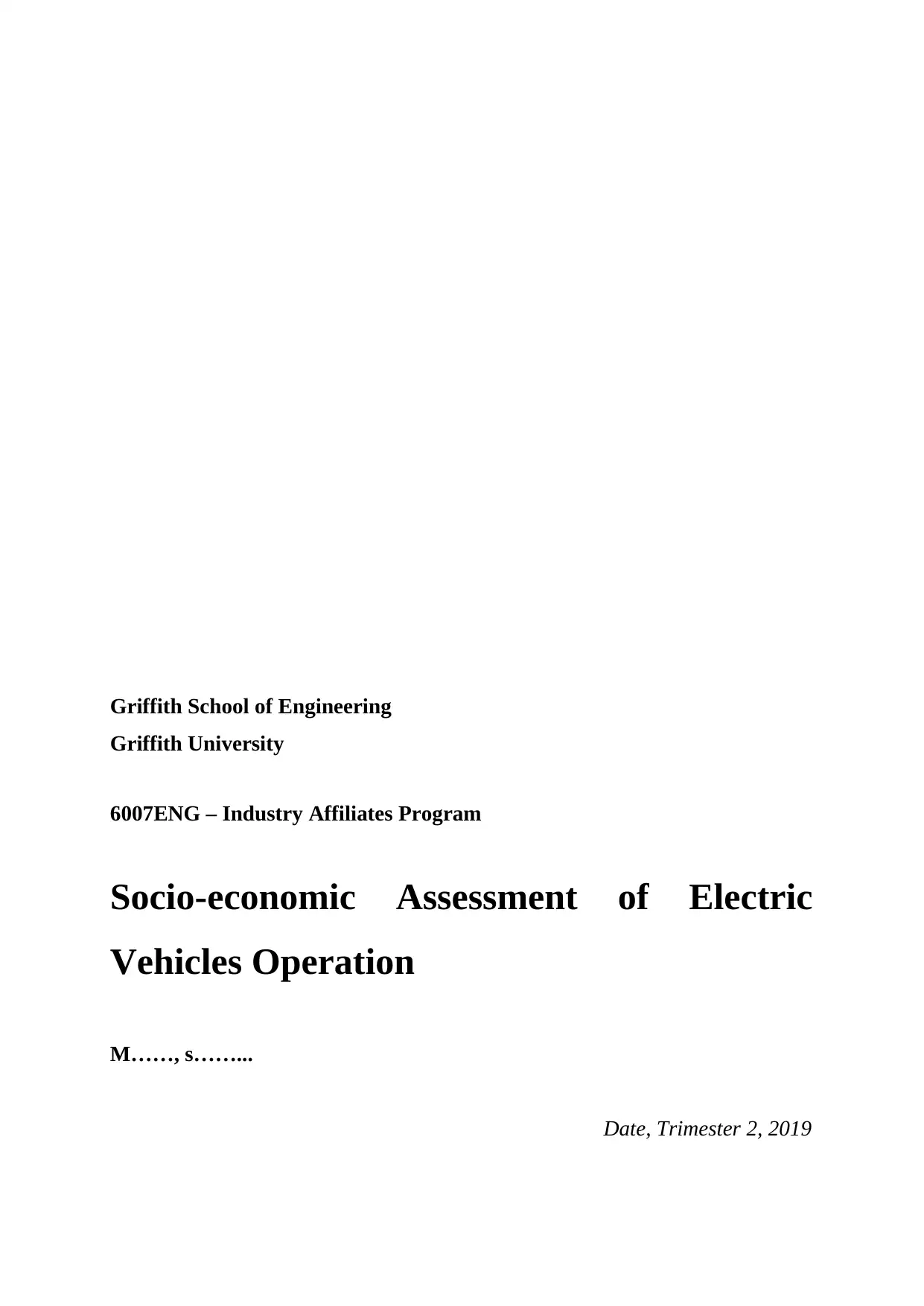
Griffith School of Engineering
Griffith University
6007ENG – Industry Affiliates Program
Socio-economic Assessment of Electric
Vehicles Operation
M……, s……...
Date, Trimester 2, 2019
Griffith University
6007ENG – Industry Affiliates Program
Socio-economic Assessment of Electric
Vehicles Operation
M……, s……...
Date, Trimester 2, 2019
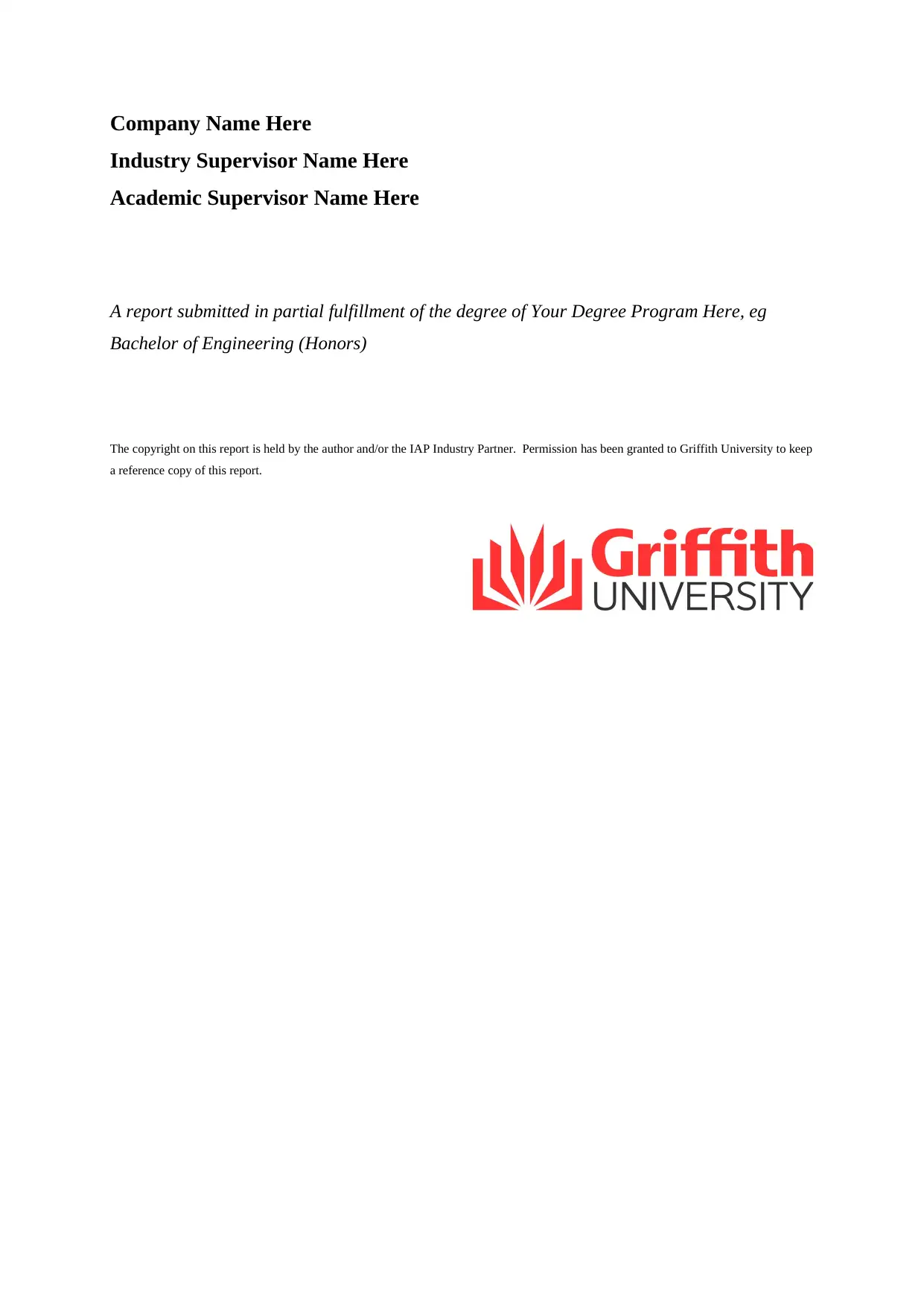
Company Name Here
Industry Supervisor Name Here
Academic Supervisor Name Here
A report submitted in partial fulfillment of the degree of Your Degree Program Here, eg
Bachelor of Engineering (Honors)
The copyright on this report is held by the author and/or the IAP Industry Partner. Permission has been granted to Griffith University to keep
a reference copy of this report.
Industry Supervisor Name Here
Academic Supervisor Name Here
A report submitted in partial fulfillment of the degree of Your Degree Program Here, eg
Bachelor of Engineering (Honors)
The copyright on this report is held by the author and/or the IAP Industry Partner. Permission has been granted to Griffith University to keep
a reference copy of this report.
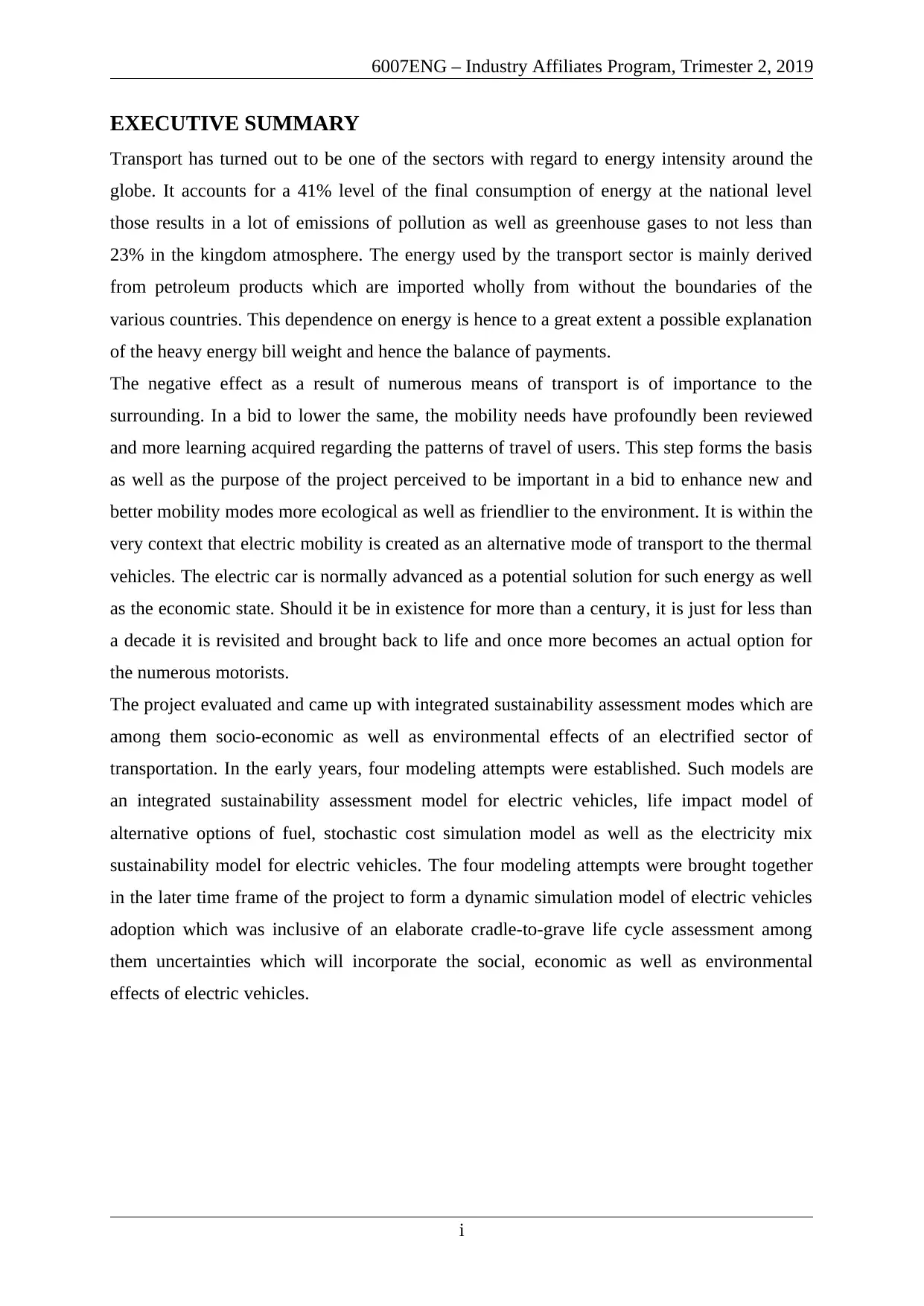
6007ENG – Industry Affiliates Program, Trimester 2, 2019
EXECUTIVE SUMMARY
Transport has turned out to be one of the sectors with regard to energy intensity around the
globe. It accounts for a 41% level of the final consumption of energy at the national level
those results in a lot of emissions of pollution as well as greenhouse gases to not less than
23% in the kingdom atmosphere. The energy used by the transport sector is mainly derived
from petroleum products which are imported wholly from without the boundaries of the
various countries. This dependence on energy is hence to a great extent a possible explanation
of the heavy energy bill weight and hence the balance of payments.
The negative effect as a result of numerous means of transport is of importance to the
surrounding. In a bid to lower the same, the mobility needs have profoundly been reviewed
and more learning acquired regarding the patterns of travel of users. This step forms the basis
as well as the purpose of the project perceived to be important in a bid to enhance new and
better mobility modes more ecological as well as friendlier to the environment. It is within the
very context that electric mobility is created as an alternative mode of transport to the thermal
vehicles. The electric car is normally advanced as a potential solution for such energy as well
as the economic state. Should it be in existence for more than a century, it is just for less than
a decade it is revisited and brought back to life and once more becomes an actual option for
the numerous motorists.
The project evaluated and came up with integrated sustainability assessment modes which are
among them socio-economic as well as environmental effects of an electrified sector of
transportation. In the early years, four modeling attempts were established. Such models are
an integrated sustainability assessment model for electric vehicles, life impact model of
alternative options of fuel, stochastic cost simulation model as well as the electricity mix
sustainability model for electric vehicles. The four modeling attempts were brought together
in the later time frame of the project to form a dynamic simulation model of electric vehicles
adoption which was inclusive of an elaborate cradle-to-grave life cycle assessment among
them uncertainties which will incorporate the social, economic as well as environmental
effects of electric vehicles.
i
EXECUTIVE SUMMARY
Transport has turned out to be one of the sectors with regard to energy intensity around the
globe. It accounts for a 41% level of the final consumption of energy at the national level
those results in a lot of emissions of pollution as well as greenhouse gases to not less than
23% in the kingdom atmosphere. The energy used by the transport sector is mainly derived
from petroleum products which are imported wholly from without the boundaries of the
various countries. This dependence on energy is hence to a great extent a possible explanation
of the heavy energy bill weight and hence the balance of payments.
The negative effect as a result of numerous means of transport is of importance to the
surrounding. In a bid to lower the same, the mobility needs have profoundly been reviewed
and more learning acquired regarding the patterns of travel of users. This step forms the basis
as well as the purpose of the project perceived to be important in a bid to enhance new and
better mobility modes more ecological as well as friendlier to the environment. It is within the
very context that electric mobility is created as an alternative mode of transport to the thermal
vehicles. The electric car is normally advanced as a potential solution for such energy as well
as the economic state. Should it be in existence for more than a century, it is just for less than
a decade it is revisited and brought back to life and once more becomes an actual option for
the numerous motorists.
The project evaluated and came up with integrated sustainability assessment modes which are
among them socio-economic as well as environmental effects of an electrified sector of
transportation. In the early years, four modeling attempts were established. Such models are
an integrated sustainability assessment model for electric vehicles, life impact model of
alternative options of fuel, stochastic cost simulation model as well as the electricity mix
sustainability model for electric vehicles. The four modeling attempts were brought together
in the later time frame of the project to form a dynamic simulation model of electric vehicles
adoption which was inclusive of an elaborate cradle-to-grave life cycle assessment among
them uncertainties which will incorporate the social, economic as well as environmental
effects of electric vehicles.
i
Secure Best Marks with AI Grader
Need help grading? Try our AI Grader for instant feedback on your assignments.
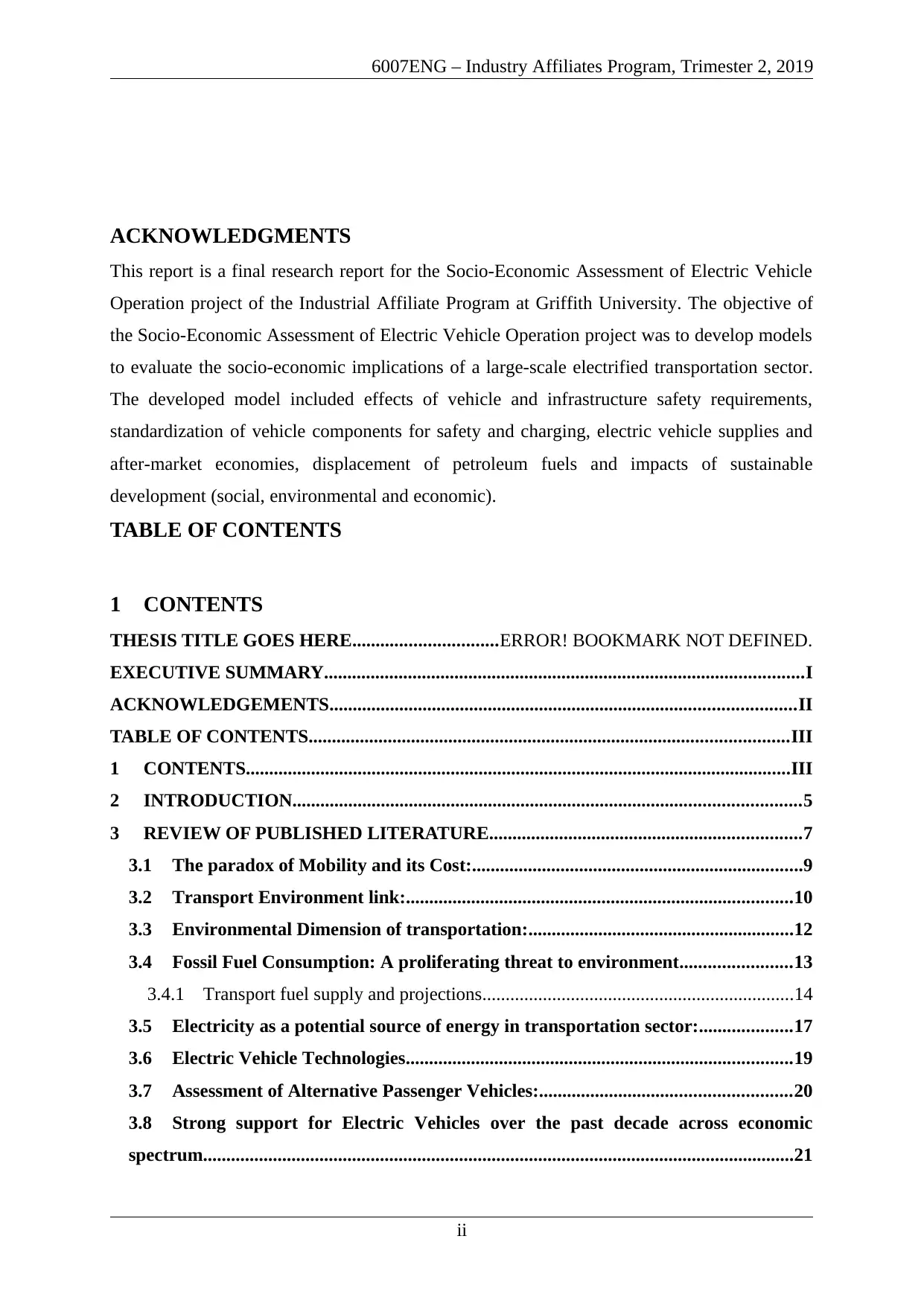
6007ENG – Industry Affiliates Program, Trimester 2, 2019
ACKNOWLEDGMENTS
This report is a final research report for the Socio-Economic Assessment of Electric Vehicle
Operation project of the Industrial Affiliate Program at Griffith University. The objective of
the Socio-Economic Assessment of Electric Vehicle Operation project was to develop models
to evaluate the socio-economic implications of a large-scale electrified transportation sector.
The developed model included effects of vehicle and infrastructure safety requirements,
standardization of vehicle components for safety and charging, electric vehicle supplies and
after-market economies, displacement of petroleum fuels and impacts of sustainable
development (social, environmental and economic).
TABLE OF CONTENTS
1 CONTENTS
THESIS TITLE GOES HERE...............................ERROR! BOOKMARK NOT DEFINED.
EXECUTIVE SUMMARY.......................................................................................................I
ACKNOWLEDGEMENTS....................................................................................................II
TABLE OF CONTENTS.......................................................................................................III
1 CONTENTS.....................................................................................................................III
2 INTRODUCTION.............................................................................................................5
3 REVIEW OF PUBLISHED LITERATURE...................................................................7
3.1 The paradox of Mobility and its Cost:.......................................................................9
3.2 Transport Environment link:...................................................................................10
3.3 Environmental Dimension of transportation:.........................................................12
3.4 Fossil Fuel Consumption: A proliferating threat to environment........................13
3.4.1 Transport fuel supply and projections...................................................................14
3.5 Electricity as a potential source of energy in transportation sector:....................17
3.6 Electric Vehicle Technologies...................................................................................19
3.7 Assessment of Alternative Passenger Vehicles:......................................................20
3.8 Strong support for Electric Vehicles over the past decade across economic
spectrum...............................................................................................................................21
ii
ACKNOWLEDGMENTS
This report is a final research report for the Socio-Economic Assessment of Electric Vehicle
Operation project of the Industrial Affiliate Program at Griffith University. The objective of
the Socio-Economic Assessment of Electric Vehicle Operation project was to develop models
to evaluate the socio-economic implications of a large-scale electrified transportation sector.
The developed model included effects of vehicle and infrastructure safety requirements,
standardization of vehicle components for safety and charging, electric vehicle supplies and
after-market economies, displacement of petroleum fuels and impacts of sustainable
development (social, environmental and economic).
TABLE OF CONTENTS
1 CONTENTS
THESIS TITLE GOES HERE...............................ERROR! BOOKMARK NOT DEFINED.
EXECUTIVE SUMMARY.......................................................................................................I
ACKNOWLEDGEMENTS....................................................................................................II
TABLE OF CONTENTS.......................................................................................................III
1 CONTENTS.....................................................................................................................III
2 INTRODUCTION.............................................................................................................5
3 REVIEW OF PUBLISHED LITERATURE...................................................................7
3.1 The paradox of Mobility and its Cost:.......................................................................9
3.2 Transport Environment link:...................................................................................10
3.3 Environmental Dimension of transportation:.........................................................12
3.4 Fossil Fuel Consumption: A proliferating threat to environment........................13
3.4.1 Transport fuel supply and projections...................................................................14
3.5 Electricity as a potential source of energy in transportation sector:....................17
3.6 Electric Vehicle Technologies...................................................................................19
3.7 Assessment of Alternative Passenger Vehicles:......................................................20
3.8 Strong support for Electric Vehicles over the past decade across economic
spectrum...............................................................................................................................21
ii
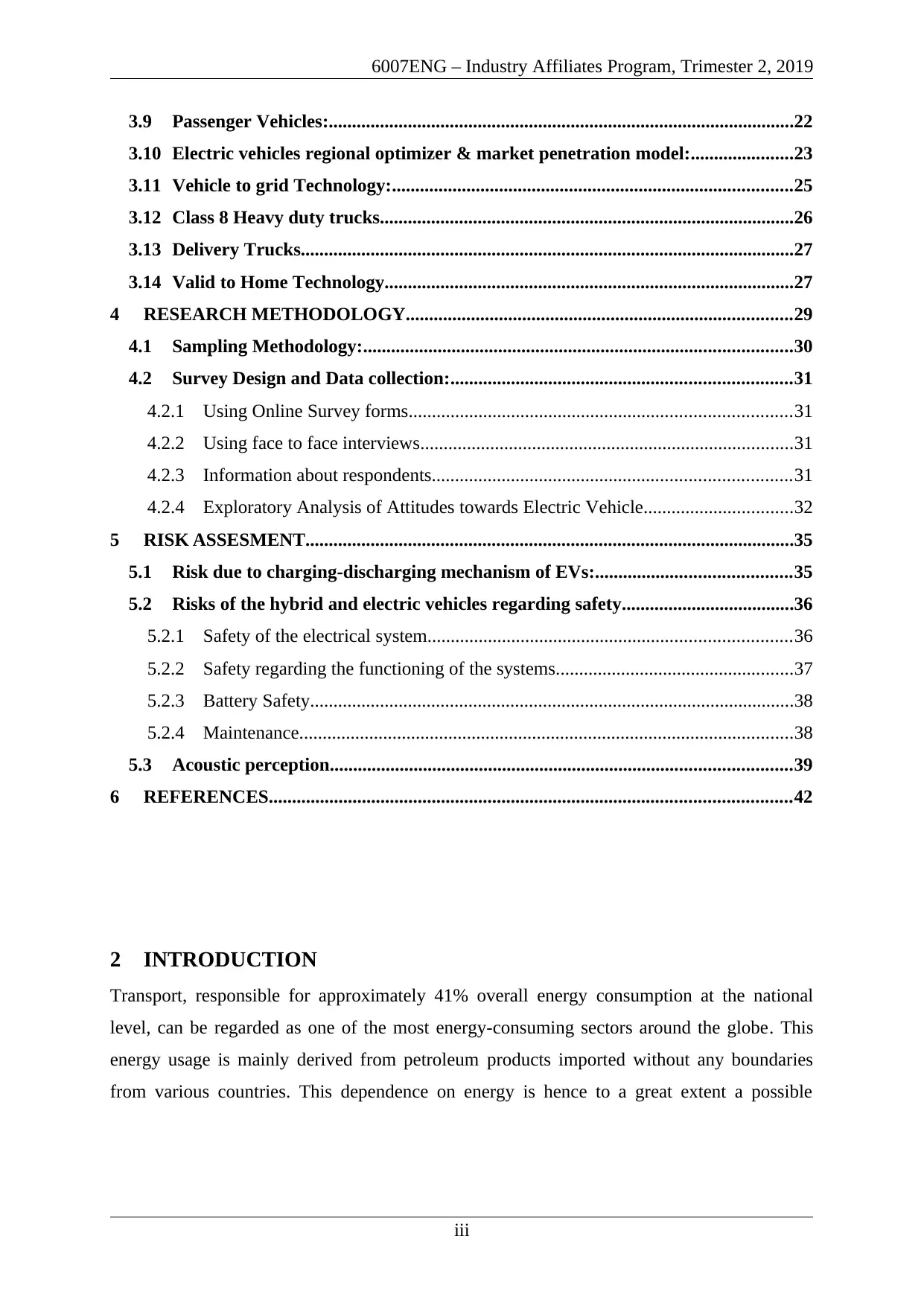
6007ENG – Industry Affiliates Program, Trimester 2, 2019
3.9 Passenger Vehicles:....................................................................................................22
3.10 Electric vehicles regional optimizer & market penetration model:......................23
3.11 Vehicle to grid Technology:......................................................................................25
3.12 Class 8 Heavy duty trucks.........................................................................................26
3.13 Delivery Trucks..........................................................................................................27
3.14 Valid to Home Technology........................................................................................27
4 RESEARCH METHODOLOGY...................................................................................29
4.1 Sampling Methodology:............................................................................................30
4.2 Survey Design and Data collection:.........................................................................31
4.2.1 Using Online Survey forms..................................................................................31
4.2.2 Using face to face interviews................................................................................31
4.2.3 Information about respondents.............................................................................31
4.2.4 Exploratory Analysis of Attitudes towards Electric Vehicle................................32
5 RISK ASSESMENT.........................................................................................................35
5.1 Risk due to charging-discharging mechanism of EVs:..........................................35
5.2 Risks of the hybrid and electric vehicles regarding safety.....................................36
5.2.1 Safety of the electrical system..............................................................................36
5.2.2 Safety regarding the functioning of the systems...................................................37
5.2.3 Battery Safety........................................................................................................38
5.2.4 Maintenance..........................................................................................................38
5.3 Acoustic perception...................................................................................................39
6 REFERENCES................................................................................................................42
2 INTRODUCTION
Transport, responsible for approximately 41% overall energy consumption at the national
level, can be regarded as one of the most energy-consuming sectors around the globe. This
energy usage is mainly derived from petroleum products imported without any boundaries
from various countries. This dependence on energy is hence to a great extent a possible
iii
3.9 Passenger Vehicles:....................................................................................................22
3.10 Electric vehicles regional optimizer & market penetration model:......................23
3.11 Vehicle to grid Technology:......................................................................................25
3.12 Class 8 Heavy duty trucks.........................................................................................26
3.13 Delivery Trucks..........................................................................................................27
3.14 Valid to Home Technology........................................................................................27
4 RESEARCH METHODOLOGY...................................................................................29
4.1 Sampling Methodology:............................................................................................30
4.2 Survey Design and Data collection:.........................................................................31
4.2.1 Using Online Survey forms..................................................................................31
4.2.2 Using face to face interviews................................................................................31
4.2.3 Information about respondents.............................................................................31
4.2.4 Exploratory Analysis of Attitudes towards Electric Vehicle................................32
5 RISK ASSESMENT.........................................................................................................35
5.1 Risk due to charging-discharging mechanism of EVs:..........................................35
5.2 Risks of the hybrid and electric vehicles regarding safety.....................................36
5.2.1 Safety of the electrical system..............................................................................36
5.2.2 Safety regarding the functioning of the systems...................................................37
5.2.3 Battery Safety........................................................................................................38
5.2.4 Maintenance..........................................................................................................38
5.3 Acoustic perception...................................................................................................39
6 REFERENCES................................................................................................................42
2 INTRODUCTION
Transport, responsible for approximately 41% overall energy consumption at the national
level, can be regarded as one of the most energy-consuming sectors around the globe. This
energy usage is mainly derived from petroleum products imported without any boundaries
from various countries. This dependence on energy is hence to a great extent a possible
iii
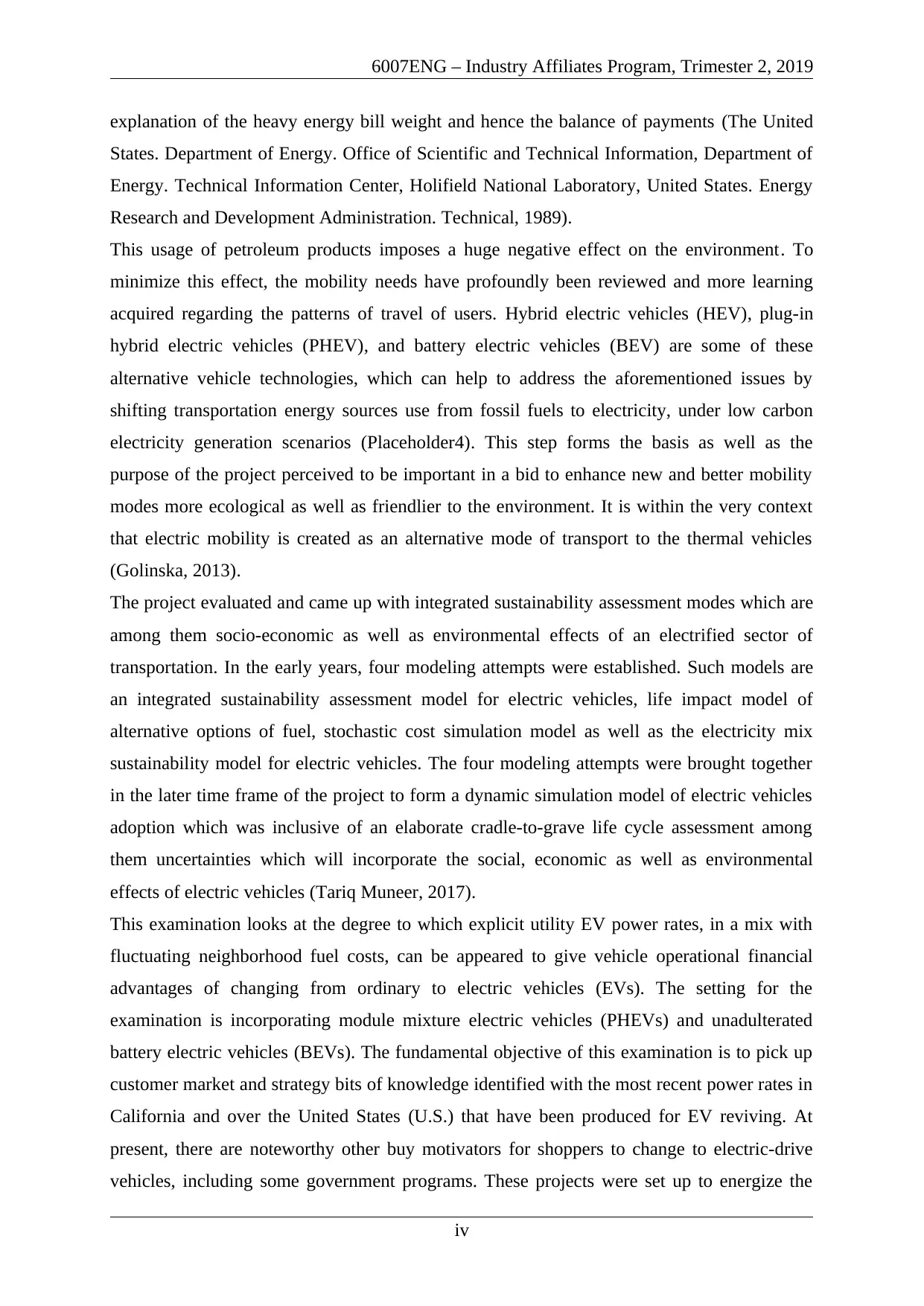
6007ENG – Industry Affiliates Program, Trimester 2, 2019
explanation of the heavy energy bill weight and hence the balance of payments (The United
States. Department of Energy. Office of Scientific and Technical Information, Department of
Energy. Technical Information Center, Holifield National Laboratory, United States. Energy
Research and Development Administration. Technical, 1989).
This usage of petroleum products imposes a huge negative effect on the environment. To
minimize this effect, the mobility needs have profoundly been reviewed and more learning
acquired regarding the patterns of travel of users. Hybrid electric vehicles (HEV), plug-in
hybrid electric vehicles (PHEV), and battery electric vehicles (BEV) are some of these
alternative vehicle technologies, which can help to address the aforementioned issues by
shifting transportation energy sources use from fossil fuels to electricity, under low carbon
electricity generation scenarios (Placeholder4). This step forms the basis as well as the
purpose of the project perceived to be important in a bid to enhance new and better mobility
modes more ecological as well as friendlier to the environment. It is within the very context
that electric mobility is created as an alternative mode of transport to the thermal vehicles
(Golinska, 2013).
The project evaluated and came up with integrated sustainability assessment modes which are
among them socio-economic as well as environmental effects of an electrified sector of
transportation. In the early years, four modeling attempts were established. Such models are
an integrated sustainability assessment model for electric vehicles, life impact model of
alternative options of fuel, stochastic cost simulation model as well as the electricity mix
sustainability model for electric vehicles. The four modeling attempts were brought together
in the later time frame of the project to form a dynamic simulation model of electric vehicles
adoption which was inclusive of an elaborate cradle-to-grave life cycle assessment among
them uncertainties which will incorporate the social, economic as well as environmental
effects of electric vehicles (Tariq Muneer, 2017).
This examination looks at the degree to which explicit utility EV power rates, in a mix with
fluctuating neighborhood fuel costs, can be appeared to give vehicle operational financial
advantages of changing from ordinary to electric vehicles (EVs). The setting for the
examination is incorporating module mixture electric vehicles (PHEVs) and unadulterated
battery electric vehicles (BEVs). The fundamental objective of this examination is to pick up
customer market and strategy bits of knowledge identified with the most recent power rates in
California and over the United States (U.S.) that have been produced for EV reviving. At
present, there are noteworthy other buy motivators for shoppers to change to electric-drive
vehicles, including some government programs. These projects were set up to energize the
iv
explanation of the heavy energy bill weight and hence the balance of payments (The United
States. Department of Energy. Office of Scientific and Technical Information, Department of
Energy. Technical Information Center, Holifield National Laboratory, United States. Energy
Research and Development Administration. Technical, 1989).
This usage of petroleum products imposes a huge negative effect on the environment. To
minimize this effect, the mobility needs have profoundly been reviewed and more learning
acquired regarding the patterns of travel of users. Hybrid electric vehicles (HEV), plug-in
hybrid electric vehicles (PHEV), and battery electric vehicles (BEV) are some of these
alternative vehicle technologies, which can help to address the aforementioned issues by
shifting transportation energy sources use from fossil fuels to electricity, under low carbon
electricity generation scenarios (Placeholder4). This step forms the basis as well as the
purpose of the project perceived to be important in a bid to enhance new and better mobility
modes more ecological as well as friendlier to the environment. It is within the very context
that electric mobility is created as an alternative mode of transport to the thermal vehicles
(Golinska, 2013).
The project evaluated and came up with integrated sustainability assessment modes which are
among them socio-economic as well as environmental effects of an electrified sector of
transportation. In the early years, four modeling attempts were established. Such models are
an integrated sustainability assessment model for electric vehicles, life impact model of
alternative options of fuel, stochastic cost simulation model as well as the electricity mix
sustainability model for electric vehicles. The four modeling attempts were brought together
in the later time frame of the project to form a dynamic simulation model of electric vehicles
adoption which was inclusive of an elaborate cradle-to-grave life cycle assessment among
them uncertainties which will incorporate the social, economic as well as environmental
effects of electric vehicles (Tariq Muneer, 2017).
This examination looks at the degree to which explicit utility EV power rates, in a mix with
fluctuating neighborhood fuel costs, can be appeared to give vehicle operational financial
advantages of changing from ordinary to electric vehicles (EVs). The setting for the
examination is incorporating module mixture electric vehicles (PHEVs) and unadulterated
battery electric vehicles (BEVs). The fundamental objective of this examination is to pick up
customer market and strategy bits of knowledge identified with the most recent power rates in
California and over the United States (U.S.) that have been produced for EV reviving. At
present, there are noteworthy other buy motivators for shoppers to change to electric-drive
vehicles, including some government programs. These projects were set up to energize the
iv
Paraphrase This Document
Need a fresh take? Get an instant paraphrase of this document with our AI Paraphraser
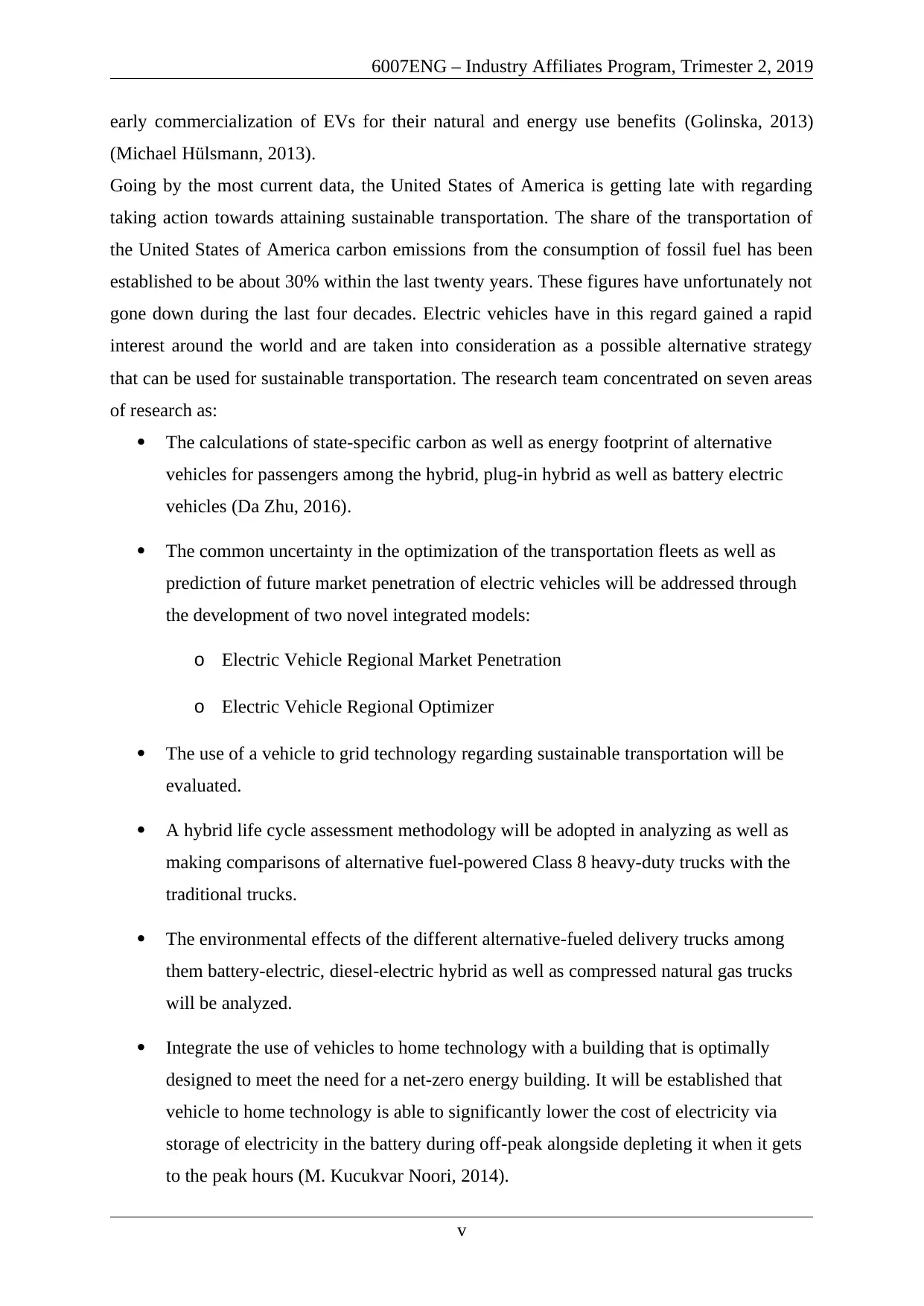
6007ENG – Industry Affiliates Program, Trimester 2, 2019
early commercialization of EVs for their natural and energy use benefits (Golinska, 2013)
(Michael Hülsmann, 2013).
Going by the most current data, the United States of America is getting late with regarding
taking action towards attaining sustainable transportation. The share of the transportation of
the United States of America carbon emissions from the consumption of fossil fuel has been
established to be about 30% within the last twenty years. These figures have unfortunately not
gone down during the last four decades. Electric vehicles have in this regard gained a rapid
interest around the world and are taken into consideration as a possible alternative strategy
that can be used for sustainable transportation. The research team concentrated on seven areas
of research as:
The calculations of state-specific carbon as well as energy footprint of alternative
vehicles for passengers among the hybrid, plug-in hybrid as well as battery electric
vehicles (Da Zhu, 2016).
The common uncertainty in the optimization of the transportation fleets as well as
prediction of future market penetration of electric vehicles will be addressed through
the development of two novel integrated models:
o Electric Vehicle Regional Market Penetration
o Electric Vehicle Regional Optimizer
The use of a vehicle to grid technology regarding sustainable transportation will be
evaluated.
A hybrid life cycle assessment methodology will be adopted in analyzing as well as
making comparisons of alternative fuel-powered Class 8 heavy-duty trucks with the
traditional trucks.
The environmental effects of the different alternative-fueled delivery trucks among
them battery-electric, diesel-electric hybrid as well as compressed natural gas trucks
will be analyzed.
Integrate the use of vehicles to home technology with a building that is optimally
designed to meet the need for a net-zero energy building. It will be established that
vehicle to home technology is able to significantly lower the cost of electricity via
storage of electricity in the battery during off-peak alongside depleting it when it gets
to the peak hours (M. Kucukvar Noori, 2014).
v
early commercialization of EVs for their natural and energy use benefits (Golinska, 2013)
(Michael Hülsmann, 2013).
Going by the most current data, the United States of America is getting late with regarding
taking action towards attaining sustainable transportation. The share of the transportation of
the United States of America carbon emissions from the consumption of fossil fuel has been
established to be about 30% within the last twenty years. These figures have unfortunately not
gone down during the last four decades. Electric vehicles have in this regard gained a rapid
interest around the world and are taken into consideration as a possible alternative strategy
that can be used for sustainable transportation. The research team concentrated on seven areas
of research as:
The calculations of state-specific carbon as well as energy footprint of alternative
vehicles for passengers among the hybrid, plug-in hybrid as well as battery electric
vehicles (Da Zhu, 2016).
The common uncertainty in the optimization of the transportation fleets as well as
prediction of future market penetration of electric vehicles will be addressed through
the development of two novel integrated models:
o Electric Vehicle Regional Market Penetration
o Electric Vehicle Regional Optimizer
The use of a vehicle to grid technology regarding sustainable transportation will be
evaluated.
A hybrid life cycle assessment methodology will be adopted in analyzing as well as
making comparisons of alternative fuel-powered Class 8 heavy-duty trucks with the
traditional trucks.
The environmental effects of the different alternative-fueled delivery trucks among
them battery-electric, diesel-electric hybrid as well as compressed natural gas trucks
will be analyzed.
Integrate the use of vehicles to home technology with a building that is optimally
designed to meet the need for a net-zero energy building. It will be established that
vehicle to home technology is able to significantly lower the cost of electricity via
storage of electricity in the battery during off-peak alongside depleting it when it gets
to the peak hours (M. Kucukvar Noori, 2014).
v
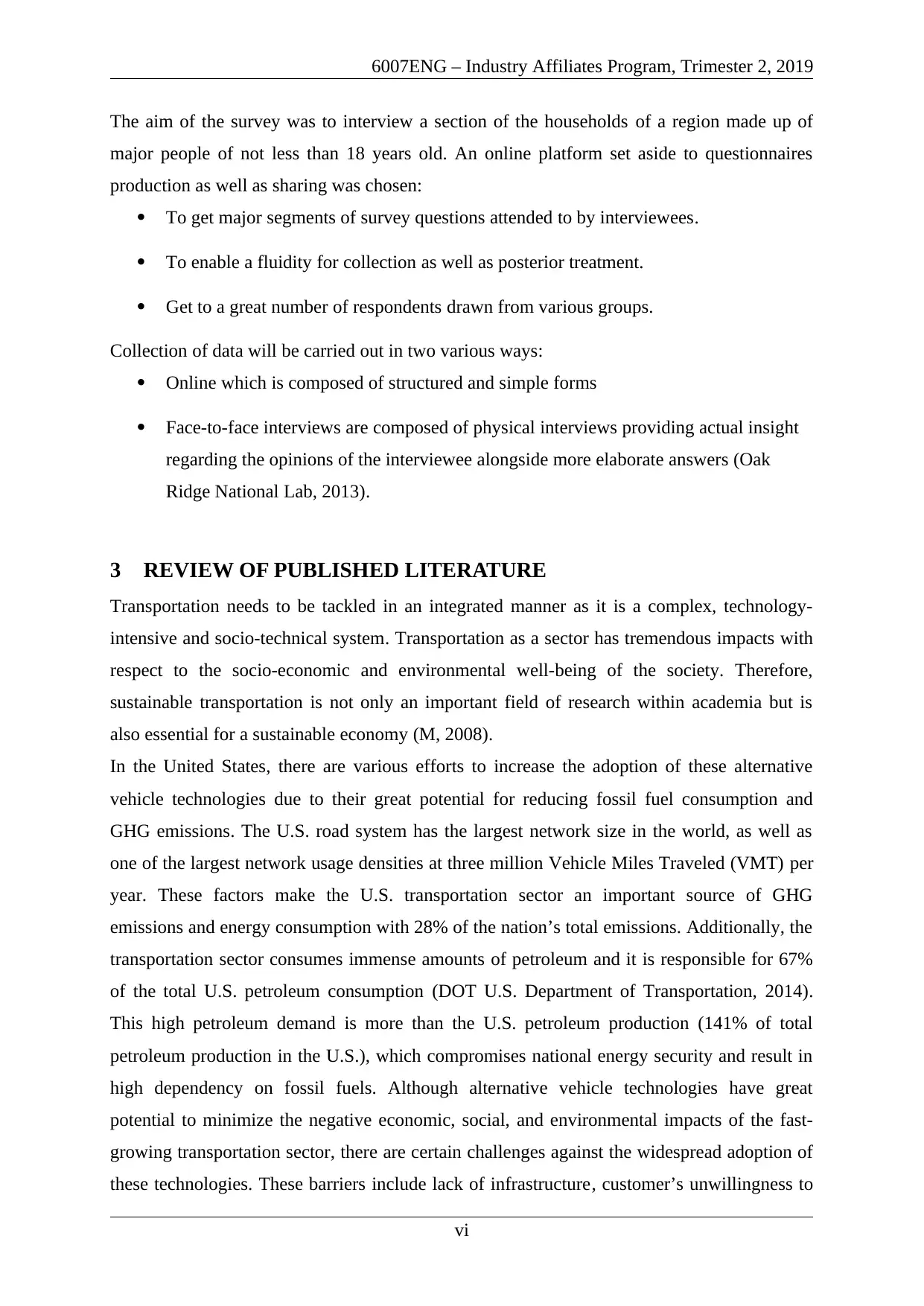
6007ENG – Industry Affiliates Program, Trimester 2, 2019
The aim of the survey was to interview a section of the households of a region made up of
major people of not less than 18 years old. An online platform set aside to questionnaires
production as well as sharing was chosen:
To get major segments of survey questions attended to by interviewees.
To enable a fluidity for collection as well as posterior treatment.
Get to a great number of respondents drawn from various groups.
Collection of data will be carried out in two various ways:
Online which is composed of structured and simple forms
Face-to-face interviews are composed of physical interviews providing actual insight
regarding the opinions of the interviewee alongside more elaborate answers (Oak
Ridge National Lab, 2013).
3 REVIEW OF PUBLISHED LITERATURE
Transportation needs to be tackled in an integrated manner as it is a complex, technology-
intensive and socio-technical system. Transportation as a sector has tremendous impacts with
respect to the socio-economic and environmental well-being of the society. Therefore,
sustainable transportation is not only an important field of research within academia but is
also essential for a sustainable economy (M, 2008).
In the United States, there are various efforts to increase the adoption of these alternative
vehicle technologies due to their great potential for reducing fossil fuel consumption and
GHG emissions. The U.S. road system has the largest network size in the world, as well as
one of the largest network usage densities at three million Vehicle Miles Traveled (VMT) per
year. These factors make the U.S. transportation sector an important source of GHG
emissions and energy consumption with 28% of the nation’s total emissions. Additionally, the
transportation sector consumes immense amounts of petroleum and it is responsible for 67%
of the total U.S. petroleum consumption (DOT U.S. Department of Transportation, 2014).
This high petroleum demand is more than the U.S. petroleum production (141% of total
petroleum production in the U.S.), which compromises national energy security and result in
high dependency on fossil fuels. Although alternative vehicle technologies have great
potential to minimize the negative economic, social, and environmental impacts of the fast-
growing transportation sector, there are certain challenges against the widespread adoption of
these technologies. These barriers include lack of infrastructure, customer’s unwillingness to
vi
The aim of the survey was to interview a section of the households of a region made up of
major people of not less than 18 years old. An online platform set aside to questionnaires
production as well as sharing was chosen:
To get major segments of survey questions attended to by interviewees.
To enable a fluidity for collection as well as posterior treatment.
Get to a great number of respondents drawn from various groups.
Collection of data will be carried out in two various ways:
Online which is composed of structured and simple forms
Face-to-face interviews are composed of physical interviews providing actual insight
regarding the opinions of the interviewee alongside more elaborate answers (Oak
Ridge National Lab, 2013).
3 REVIEW OF PUBLISHED LITERATURE
Transportation needs to be tackled in an integrated manner as it is a complex, technology-
intensive and socio-technical system. Transportation as a sector has tremendous impacts with
respect to the socio-economic and environmental well-being of the society. Therefore,
sustainable transportation is not only an important field of research within academia but is
also essential for a sustainable economy (M, 2008).
In the United States, there are various efforts to increase the adoption of these alternative
vehicle technologies due to their great potential for reducing fossil fuel consumption and
GHG emissions. The U.S. road system has the largest network size in the world, as well as
one of the largest network usage densities at three million Vehicle Miles Traveled (VMT) per
year. These factors make the U.S. transportation sector an important source of GHG
emissions and energy consumption with 28% of the nation’s total emissions. Additionally, the
transportation sector consumes immense amounts of petroleum and it is responsible for 67%
of the total U.S. petroleum consumption (DOT U.S. Department of Transportation, 2014).
This high petroleum demand is more than the U.S. petroleum production (141% of total
petroleum production in the U.S.), which compromises national energy security and result in
high dependency on fossil fuels. Although alternative vehicle technologies have great
potential to minimize the negative economic, social, and environmental impacts of the fast-
growing transportation sector, there are certain challenges against the widespread adoption of
these technologies. These barriers include lack of infrastructure, customer’s unwillingness to
vi
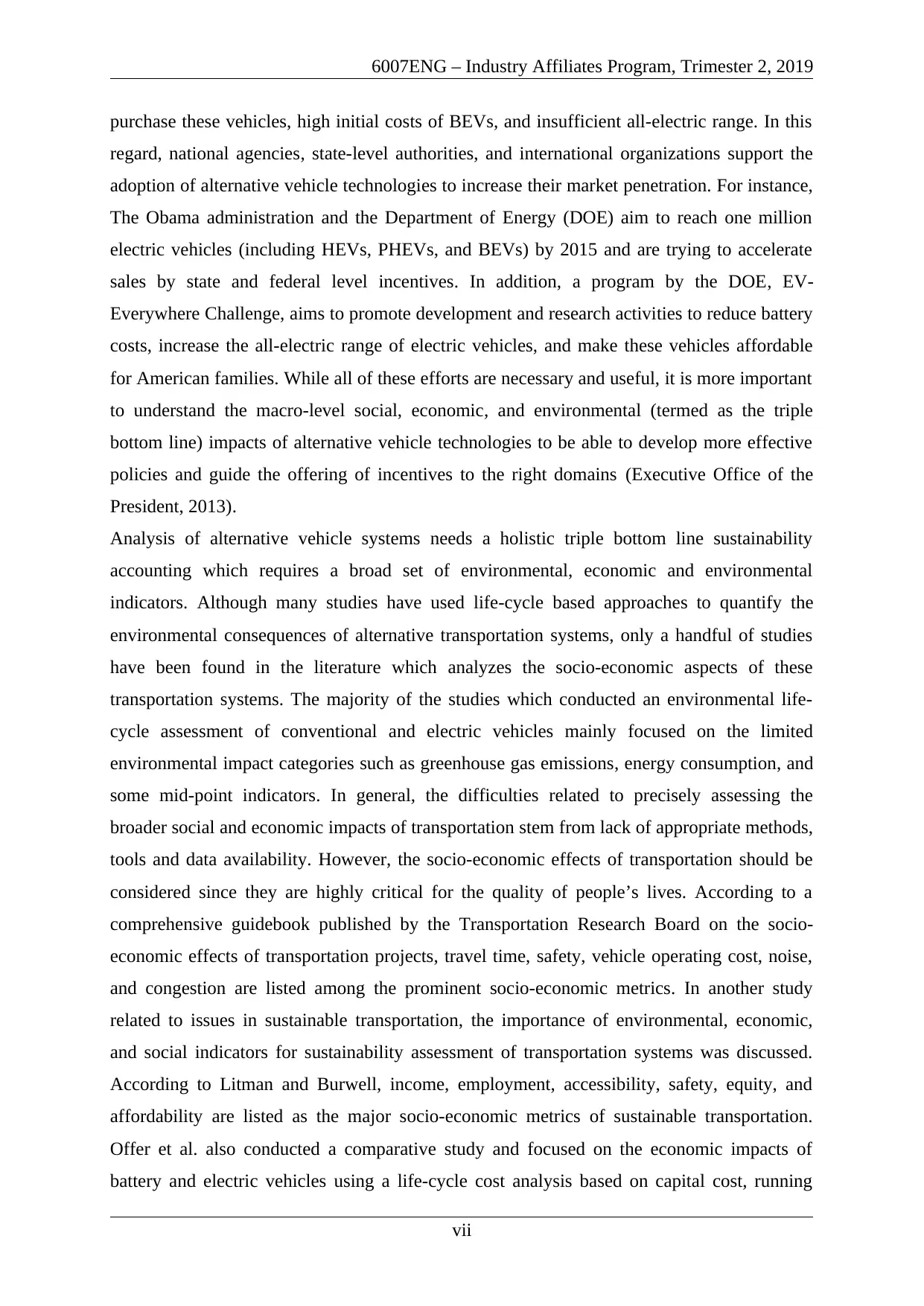
6007ENG – Industry Affiliates Program, Trimester 2, 2019
purchase these vehicles, high initial costs of BEVs, and insufficient all-electric range. In this
regard, national agencies, state-level authorities, and international organizations support the
adoption of alternative vehicle technologies to increase their market penetration. For instance,
The Obama administration and the Department of Energy (DOE) aim to reach one million
electric vehicles (including HEVs, PHEVs, and BEVs) by 2015 and are trying to accelerate
sales by state and federal level incentives. In addition, a program by the DOE, EV-
Everywhere Challenge, aims to promote development and research activities to reduce battery
costs, increase the all-electric range of electric vehicles, and make these vehicles affordable
for American families. While all of these efforts are necessary and useful, it is more important
to understand the macro-level social, economic, and environmental (termed as the triple
bottom line) impacts of alternative vehicle technologies to be able to develop more effective
policies and guide the offering of incentives to the right domains (Executive Office of the
President, 2013).
Analysis of alternative vehicle systems needs a holistic triple bottom line sustainability
accounting which requires a broad set of environmental, economic and environmental
indicators. Although many studies have used life-cycle based approaches to quantify the
environmental consequences of alternative transportation systems, only a handful of studies
have been found in the literature which analyzes the socio-economic aspects of these
transportation systems. The majority of the studies which conducted an environmental life-
cycle assessment of conventional and electric vehicles mainly focused on the limited
environmental impact categories such as greenhouse gas emissions, energy consumption, and
some mid-point indicators. In general, the difficulties related to precisely assessing the
broader social and economic impacts of transportation stem from lack of appropriate methods,
tools and data availability. However, the socio-economic effects of transportation should be
considered since they are highly critical for the quality of people’s lives. According to a
comprehensive guidebook published by the Transportation Research Board on the socio-
economic effects of transportation projects, travel time, safety, vehicle operating cost, noise,
and congestion are listed among the prominent socio-economic metrics. In another study
related to issues in sustainable transportation, the importance of environmental, economic,
and social indicators for sustainability assessment of transportation systems was discussed.
According to Litman and Burwell, income, employment, accessibility, safety, equity, and
affordability are listed as the major socio-economic metrics of sustainable transportation.
Offer et al. also conducted a comparative study and focused on the economic impacts of
battery and electric vehicles using a life-cycle cost analysis based on capital cost, running
vii
purchase these vehicles, high initial costs of BEVs, and insufficient all-electric range. In this
regard, national agencies, state-level authorities, and international organizations support the
adoption of alternative vehicle technologies to increase their market penetration. For instance,
The Obama administration and the Department of Energy (DOE) aim to reach one million
electric vehicles (including HEVs, PHEVs, and BEVs) by 2015 and are trying to accelerate
sales by state and federal level incentives. In addition, a program by the DOE, EV-
Everywhere Challenge, aims to promote development and research activities to reduce battery
costs, increase the all-electric range of electric vehicles, and make these vehicles affordable
for American families. While all of these efforts are necessary and useful, it is more important
to understand the macro-level social, economic, and environmental (termed as the triple
bottom line) impacts of alternative vehicle technologies to be able to develop more effective
policies and guide the offering of incentives to the right domains (Executive Office of the
President, 2013).
Analysis of alternative vehicle systems needs a holistic triple bottom line sustainability
accounting which requires a broad set of environmental, economic and environmental
indicators. Although many studies have used life-cycle based approaches to quantify the
environmental consequences of alternative transportation systems, only a handful of studies
have been found in the literature which analyzes the socio-economic aspects of these
transportation systems. The majority of the studies which conducted an environmental life-
cycle assessment of conventional and electric vehicles mainly focused on the limited
environmental impact categories such as greenhouse gas emissions, energy consumption, and
some mid-point indicators. In general, the difficulties related to precisely assessing the
broader social and economic impacts of transportation stem from lack of appropriate methods,
tools and data availability. However, the socio-economic effects of transportation should be
considered since they are highly critical for the quality of people’s lives. According to a
comprehensive guidebook published by the Transportation Research Board on the socio-
economic effects of transportation projects, travel time, safety, vehicle operating cost, noise,
and congestion are listed among the prominent socio-economic metrics. In another study
related to issues in sustainable transportation, the importance of environmental, economic,
and social indicators for sustainability assessment of transportation systems was discussed.
According to Litman and Burwell, income, employment, accessibility, safety, equity, and
affordability are listed as the major socio-economic metrics of sustainable transportation.
Offer et al. also conducted a comparative study and focused on the economic impacts of
battery and electric vehicles using a life-cycle cost analysis based on capital cost, running
vii
Secure Best Marks with AI Grader
Need help grading? Try our AI Grader for instant feedback on your assignments.
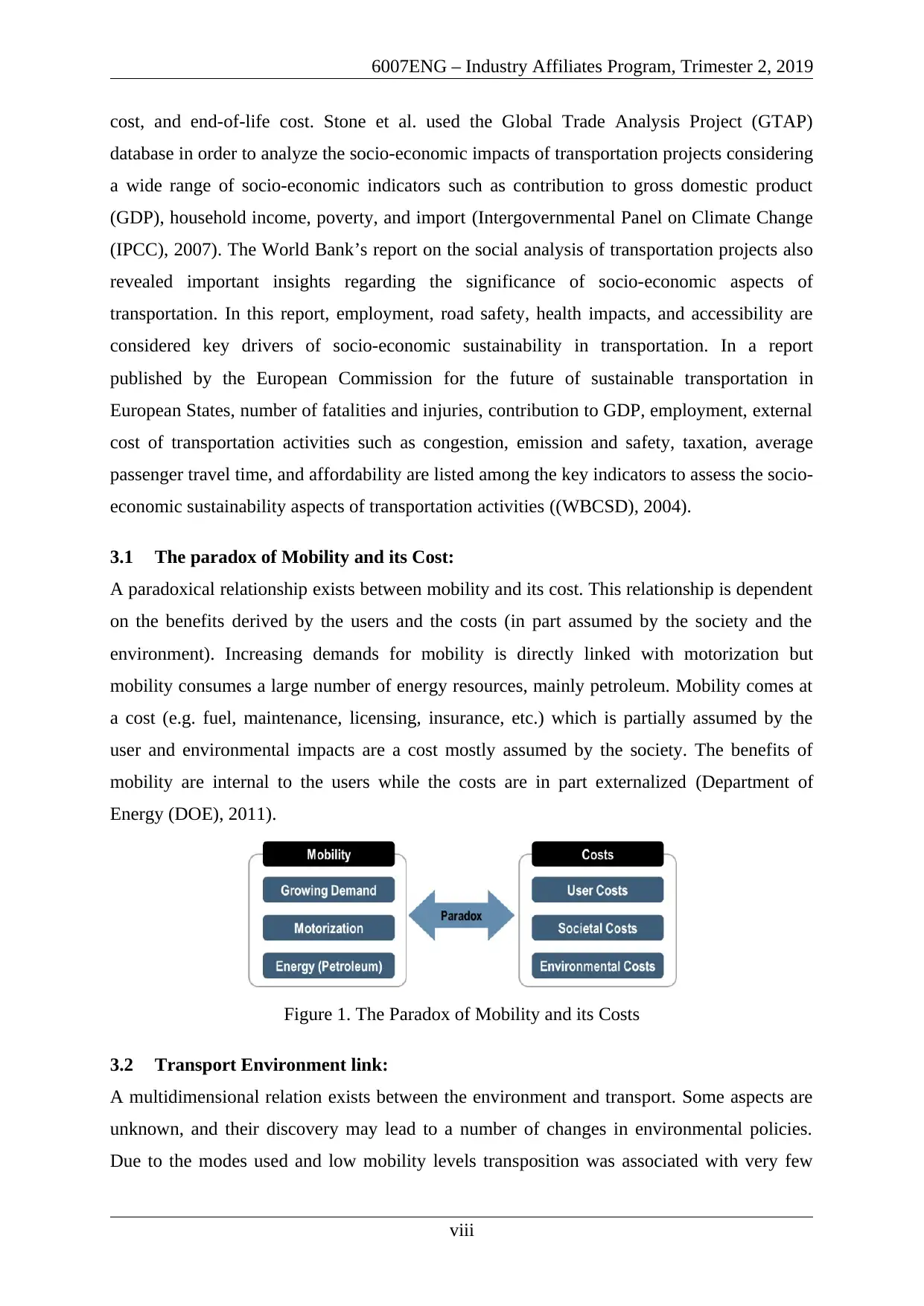
6007ENG – Industry Affiliates Program, Trimester 2, 2019
cost, and end-of-life cost. Stone et al. used the Global Trade Analysis Project (GTAP)
database in order to analyze the socio-economic impacts of transportation projects considering
a wide range of socio-economic indicators such as contribution to gross domestic product
(GDP), household income, poverty, and import (Intergovernmental Panel on Climate Change
(IPCC), 2007). The World Bank’s report on the social analysis of transportation projects also
revealed important insights regarding the significance of socio-economic aspects of
transportation. In this report, employment, road safety, health impacts, and accessibility are
considered key drivers of socio-economic sustainability in transportation. In a report
published by the European Commission for the future of sustainable transportation in
European States, number of fatalities and injuries, contribution to GDP, employment, external
cost of transportation activities such as congestion, emission and safety, taxation, average
passenger travel time, and affordability are listed among the key indicators to assess the socio-
economic sustainability aspects of transportation activities ((WBCSD), 2004).
3.1 The paradox of Mobility and its Cost:
A paradoxical relationship exists between mobility and its cost. This relationship is dependent
on the benefits derived by the users and the costs (in part assumed by the society and the
environment). Increasing demands for mobility is directly linked with motorization but
mobility consumes a large number of energy resources, mainly petroleum. Mobility comes at
a cost (e.g. fuel, maintenance, licensing, insurance, etc.) which is partially assumed by the
user and environmental impacts are a cost mostly assumed by the society. The benefits of
mobility are internal to the users while the costs are in part externalized (Department of
Energy (DOE), 2011).
Figure 1. The Paradox of Mobility and its Costs
3.2 Transport Environment link:
A multidimensional relation exists between the environment and transport. Some aspects are
unknown, and their discovery may lead to a number of changes in environmental policies.
Due to the modes used and low mobility levels transposition was associated with very few
viii
cost, and end-of-life cost. Stone et al. used the Global Trade Analysis Project (GTAP)
database in order to analyze the socio-economic impacts of transportation projects considering
a wide range of socio-economic indicators such as contribution to gross domestic product
(GDP), household income, poverty, and import (Intergovernmental Panel on Climate Change
(IPCC), 2007). The World Bank’s report on the social analysis of transportation projects also
revealed important insights regarding the significance of socio-economic aspects of
transportation. In this report, employment, road safety, health impacts, and accessibility are
considered key drivers of socio-economic sustainability in transportation. In a report
published by the European Commission for the future of sustainable transportation in
European States, number of fatalities and injuries, contribution to GDP, employment, external
cost of transportation activities such as congestion, emission and safety, taxation, average
passenger travel time, and affordability are listed among the key indicators to assess the socio-
economic sustainability aspects of transportation activities ((WBCSD), 2004).
3.1 The paradox of Mobility and its Cost:
A paradoxical relationship exists between mobility and its cost. This relationship is dependent
on the benefits derived by the users and the costs (in part assumed by the society and the
environment). Increasing demands for mobility is directly linked with motorization but
mobility consumes a large number of energy resources, mainly petroleum. Mobility comes at
a cost (e.g. fuel, maintenance, licensing, insurance, etc.) which is partially assumed by the
user and environmental impacts are a cost mostly assumed by the society. The benefits of
mobility are internal to the users while the costs are in part externalized (Department of
Energy (DOE), 2011).
Figure 1. The Paradox of Mobility and its Costs
3.2 Transport Environment link:
A multidimensional relation exists between the environment and transport. Some aspects are
unknown, and their discovery may lead to a number of changes in environmental policies.
Due to the modes used and low mobility levels transposition was associated with very few
viii
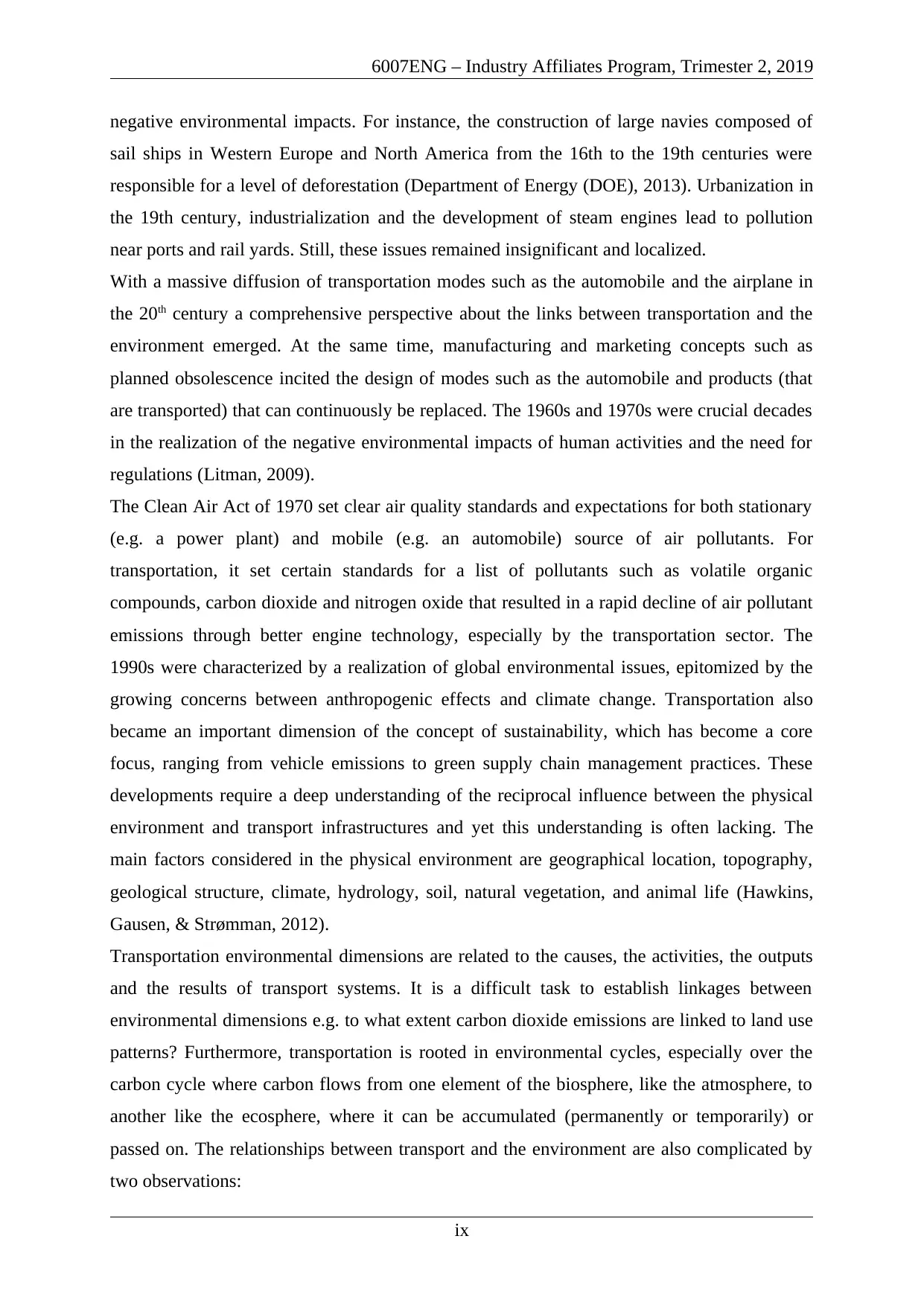
6007ENG – Industry Affiliates Program, Trimester 2, 2019
negative environmental impacts. For instance, the construction of large navies composed of
sail ships in Western Europe and North America from the 16th to the 19th centuries were
responsible for a level of deforestation (Department of Energy (DOE), 2013). Urbanization in
the 19th century, industrialization and the development of steam engines lead to pollution
near ports and rail yards. Still, these issues remained insignificant and localized.
With a massive diffusion of transportation modes such as the automobile and the airplane in
the 20th century a comprehensive perspective about the links between transportation and the
environment emerged. At the same time, manufacturing and marketing concepts such as
planned obsolescence incited the design of modes such as the automobile and products (that
are transported) that can continuously be replaced. The 1960s and 1970s were crucial decades
in the realization of the negative environmental impacts of human activities and the need for
regulations (Litman, 2009).
The Clean Air Act of 1970 set clear air quality standards and expectations for both stationary
(e.g. a power plant) and mobile (e.g. an automobile) source of air pollutants. For
transportation, it set certain standards for a list of pollutants such as volatile organic
compounds, carbon dioxide and nitrogen oxide that resulted in a rapid decline of air pollutant
emissions through better engine technology, especially by the transportation sector. The
1990s were characterized by a realization of global environmental issues, epitomized by the
growing concerns between anthropogenic effects and climate change. Transportation also
became an important dimension of the concept of sustainability, which has become a core
focus, ranging from vehicle emissions to green supply chain management practices. These
developments require a deep understanding of the reciprocal influence between the physical
environment and transport infrastructures and yet this understanding is often lacking. The
main factors considered in the physical environment are geographical location, topography,
geological structure, climate, hydrology, soil, natural vegetation, and animal life (Hawkins,
Gausen, & Strømman, 2012).
Transportation environmental dimensions are related to the causes, the activities, the outputs
and the results of transport systems. It is a difficult task to establish linkages between
environmental dimensions e.g. to what extent carbon dioxide emissions are linked to land use
patterns? Furthermore, transportation is rooted in environmental cycles, especially over the
carbon cycle where carbon flows from one element of the biosphere, like the atmosphere, to
another like the ecosphere, where it can be accumulated (permanently or temporarily) or
passed on. The relationships between transport and the environment are also complicated by
two observations:
ix
negative environmental impacts. For instance, the construction of large navies composed of
sail ships in Western Europe and North America from the 16th to the 19th centuries were
responsible for a level of deforestation (Department of Energy (DOE), 2013). Urbanization in
the 19th century, industrialization and the development of steam engines lead to pollution
near ports and rail yards. Still, these issues remained insignificant and localized.
With a massive diffusion of transportation modes such as the automobile and the airplane in
the 20th century a comprehensive perspective about the links between transportation and the
environment emerged. At the same time, manufacturing and marketing concepts such as
planned obsolescence incited the design of modes such as the automobile and products (that
are transported) that can continuously be replaced. The 1960s and 1970s were crucial decades
in the realization of the negative environmental impacts of human activities and the need for
regulations (Litman, 2009).
The Clean Air Act of 1970 set clear air quality standards and expectations for both stationary
(e.g. a power plant) and mobile (e.g. an automobile) source of air pollutants. For
transportation, it set certain standards for a list of pollutants such as volatile organic
compounds, carbon dioxide and nitrogen oxide that resulted in a rapid decline of air pollutant
emissions through better engine technology, especially by the transportation sector. The
1990s were characterized by a realization of global environmental issues, epitomized by the
growing concerns between anthropogenic effects and climate change. Transportation also
became an important dimension of the concept of sustainability, which has become a core
focus, ranging from vehicle emissions to green supply chain management practices. These
developments require a deep understanding of the reciprocal influence between the physical
environment and transport infrastructures and yet this understanding is often lacking. The
main factors considered in the physical environment are geographical location, topography,
geological structure, climate, hydrology, soil, natural vegetation, and animal life (Hawkins,
Gausen, & Strømman, 2012).
Transportation environmental dimensions are related to the causes, the activities, the outputs
and the results of transport systems. It is a difficult task to establish linkages between
environmental dimensions e.g. to what extent carbon dioxide emissions are linked to land use
patterns? Furthermore, transportation is rooted in environmental cycles, especially over the
carbon cycle where carbon flows from one element of the biosphere, like the atmosphere, to
another like the ecosphere, where it can be accumulated (permanently or temporarily) or
passed on. The relationships between transport and the environment are also complicated by
two observations:
ix
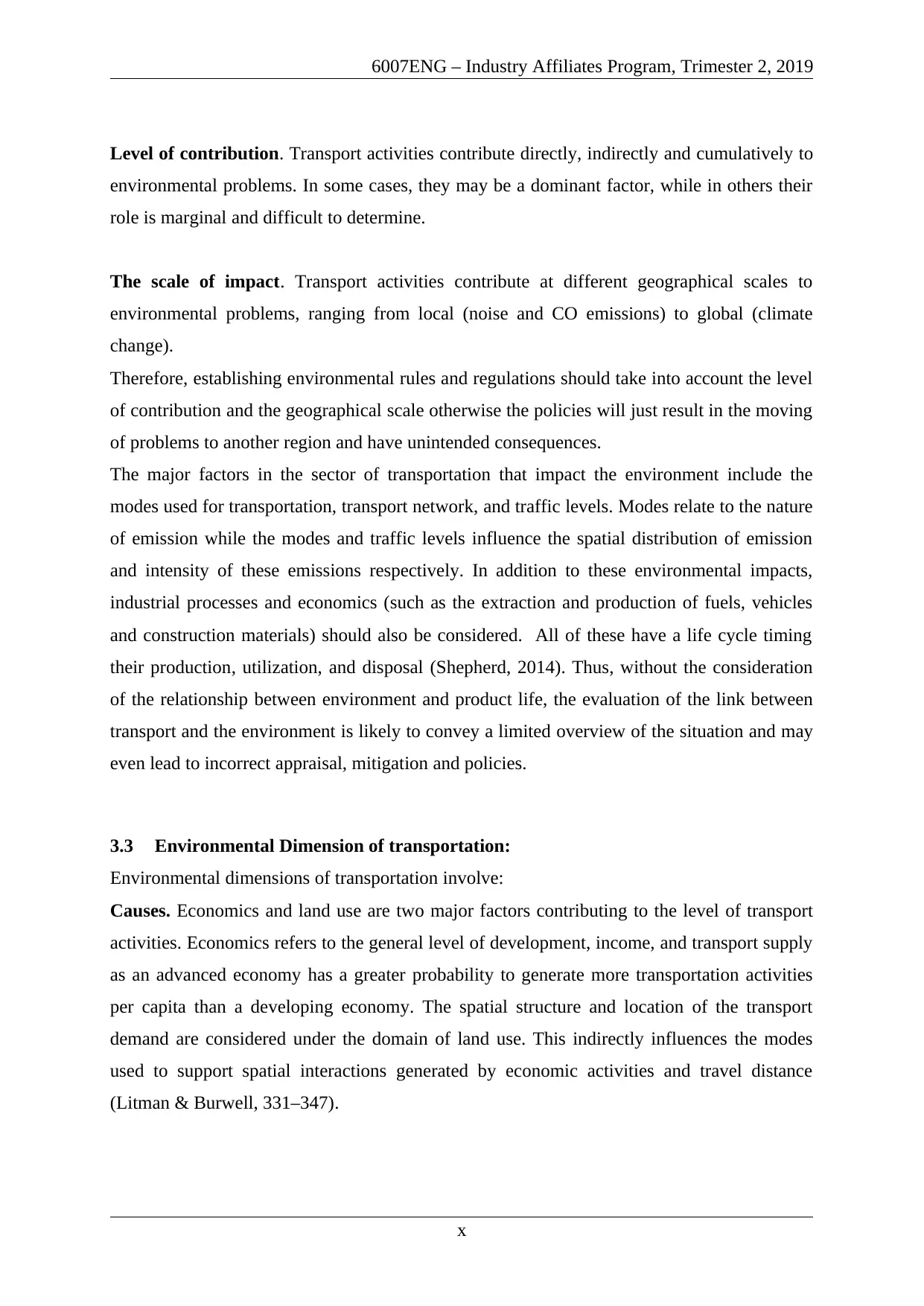
6007ENG – Industry Affiliates Program, Trimester 2, 2019
Level of contribution. Transport activities contribute directly, indirectly and cumulatively to
environmental problems. In some cases, they may be a dominant factor, while in others their
role is marginal and difficult to determine.
The scale of impact. Transport activities contribute at different geographical scales to
environmental problems, ranging from local (noise and CO emissions) to global (climate
change).
Therefore, establishing environmental rules and regulations should take into account the level
of contribution and the geographical scale otherwise the policies will just result in the moving
of problems to another region and have unintended consequences.
The major factors in the sector of transportation that impact the environment include the
modes used for transportation, transport network, and traffic levels. Modes relate to the nature
of emission while the modes and traffic levels influence the spatial distribution of emission
and intensity of these emissions respectively. In addition to these environmental impacts,
industrial processes and economics (such as the extraction and production of fuels, vehicles
and construction materials) should also be considered. All of these have a life cycle timing
their production, utilization, and disposal (Shepherd, 2014). Thus, without the consideration
of the relationship between environment and product life, the evaluation of the link between
transport and the environment is likely to convey a limited overview of the situation and may
even lead to incorrect appraisal, mitigation and policies.
3.3 Environmental Dimension of transportation:
Environmental dimensions of transportation involve:
Causes. Economics and land use are two major factors contributing to the level of transport
activities. Economics refers to the general level of development, income, and transport supply
as an advanced economy has a greater probability to generate more transportation activities
per capita than a developing economy. The spatial structure and location of the transport
demand are considered under the domain of land use. This indirectly influences the modes
used to support spatial interactions generated by economic activities and travel distance
(Litman & Burwell, 331–347).
x
Level of contribution. Transport activities contribute directly, indirectly and cumulatively to
environmental problems. In some cases, they may be a dominant factor, while in others their
role is marginal and difficult to determine.
The scale of impact. Transport activities contribute at different geographical scales to
environmental problems, ranging from local (noise and CO emissions) to global (climate
change).
Therefore, establishing environmental rules and regulations should take into account the level
of contribution and the geographical scale otherwise the policies will just result in the moving
of problems to another region and have unintended consequences.
The major factors in the sector of transportation that impact the environment include the
modes used for transportation, transport network, and traffic levels. Modes relate to the nature
of emission while the modes and traffic levels influence the spatial distribution of emission
and intensity of these emissions respectively. In addition to these environmental impacts,
industrial processes and economics (such as the extraction and production of fuels, vehicles
and construction materials) should also be considered. All of these have a life cycle timing
their production, utilization, and disposal (Shepherd, 2014). Thus, without the consideration
of the relationship between environment and product life, the evaluation of the link between
transport and the environment is likely to convey a limited overview of the situation and may
even lead to incorrect appraisal, mitigation and policies.
3.3 Environmental Dimension of transportation:
Environmental dimensions of transportation involve:
Causes. Economics and land use are two major factors contributing to the level of transport
activities. Economics refers to the general level of development, income, and transport supply
as an advanced economy has a greater probability to generate more transportation activities
per capita than a developing economy. The spatial structure and location of the transport
demand are considered under the domain of land use. This indirectly influences the modes
used to support spatial interactions generated by economic activities and travel distance
(Litman & Burwell, 331–347).
x
Paraphrase This Document
Need a fresh take? Get an instant paraphrase of this document with our AI Paraphraser
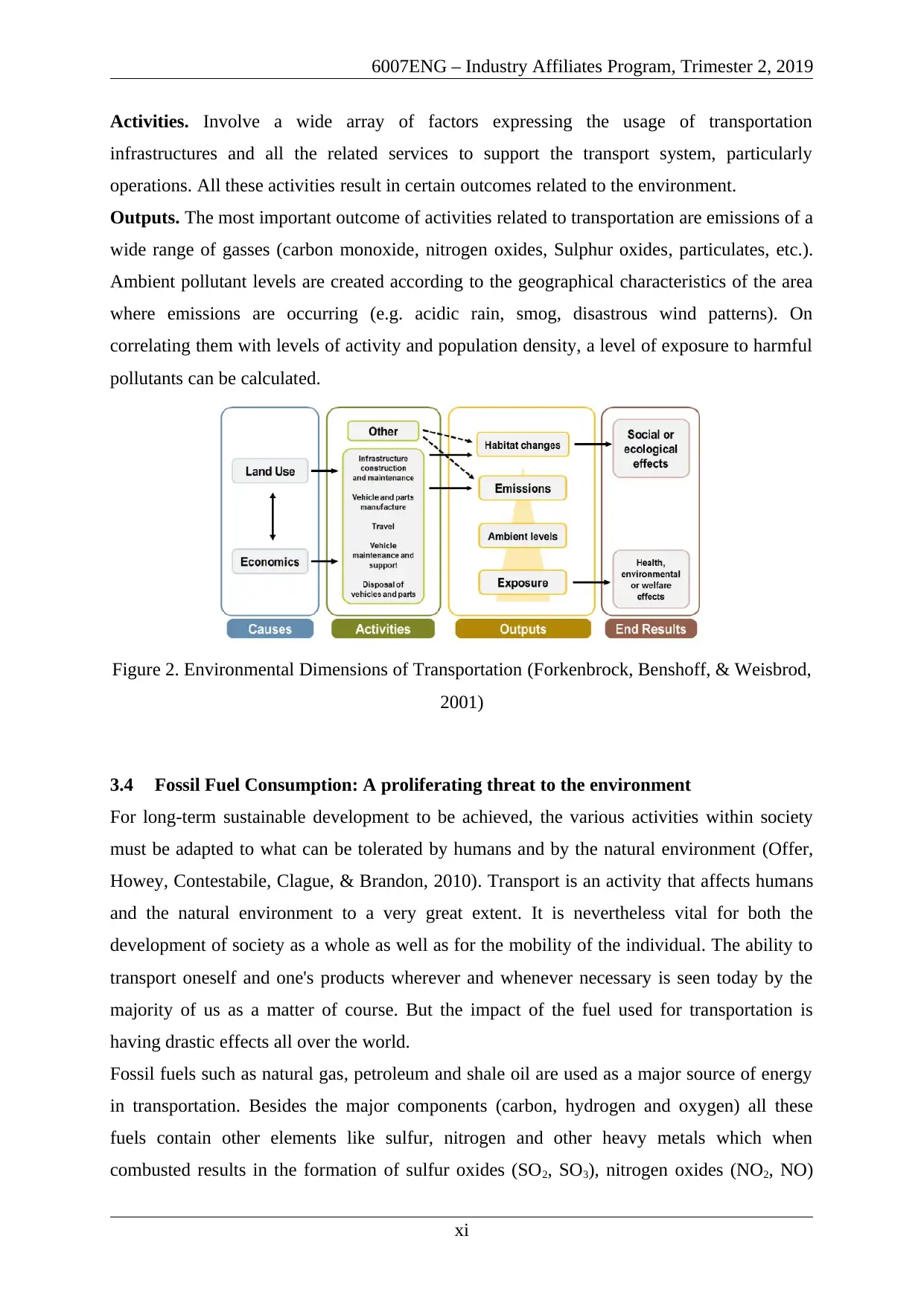
6007ENG – Industry Affiliates Program, Trimester 2, 2019
Activities. Involve a wide array of factors expressing the usage of transportation
infrastructures and all the related services to support the transport system, particularly
operations. All these activities result in certain outcomes related to the environment.
Outputs. The most important outcome of activities related to transportation are emissions of a
wide range of gasses (carbon monoxide, nitrogen oxides, Sulphur oxides, particulates, etc.).
Ambient pollutant levels are created according to the geographical characteristics of the area
where emissions are occurring (e.g. acidic rain, smog, disastrous wind patterns). On
correlating them with levels of activity and population density, a level of exposure to harmful
pollutants can be calculated.
Figure 2. Environmental Dimensions of Transportation (Forkenbrock, Benshoff, & Weisbrod,
2001)
3.4 Fossil Fuel Consumption: A proliferating threat to the environment
For long-term sustainable development to be achieved, the various activities within society
must be adapted to what can be tolerated by humans and by the natural environment (Offer,
Howey, Contestabile, Clague, & Brandon, 2010). Transport is an activity that affects humans
and the natural environment to a very great extent. It is nevertheless vital for both the
development of society as a whole as well as for the mobility of the individual. The ability to
transport oneself and one's products wherever and whenever necessary is seen today by the
majority of us as a matter of course. But the impact of the fuel used for transportation is
having drastic effects all over the world.
Fossil fuels such as natural gas, petroleum and shale oil are used as a major source of energy
in transportation. Besides the major components (carbon, hydrogen and oxygen) all these
fuels contain other elements like sulfur, nitrogen and other heavy metals which when
combusted results in the formation of sulfur oxides (SO2, SO3), nitrogen oxides (NO2, NO)
xi
Activities. Involve a wide array of factors expressing the usage of transportation
infrastructures and all the related services to support the transport system, particularly
operations. All these activities result in certain outcomes related to the environment.
Outputs. The most important outcome of activities related to transportation are emissions of a
wide range of gasses (carbon monoxide, nitrogen oxides, Sulphur oxides, particulates, etc.).
Ambient pollutant levels are created according to the geographical characteristics of the area
where emissions are occurring (e.g. acidic rain, smog, disastrous wind patterns). On
correlating them with levels of activity and population density, a level of exposure to harmful
pollutants can be calculated.
Figure 2. Environmental Dimensions of Transportation (Forkenbrock, Benshoff, & Weisbrod,
2001)
3.4 Fossil Fuel Consumption: A proliferating threat to the environment
For long-term sustainable development to be achieved, the various activities within society
must be adapted to what can be tolerated by humans and by the natural environment (Offer,
Howey, Contestabile, Clague, & Brandon, 2010). Transport is an activity that affects humans
and the natural environment to a very great extent. It is nevertheless vital for both the
development of society as a whole as well as for the mobility of the individual. The ability to
transport oneself and one's products wherever and whenever necessary is seen today by the
majority of us as a matter of course. But the impact of the fuel used for transportation is
having drastic effects all over the world.
Fossil fuels such as natural gas, petroleum and shale oil are used as a major source of energy
in transportation. Besides the major components (carbon, hydrogen and oxygen) all these
fuels contain other elements like sulfur, nitrogen and other heavy metals which when
combusted results in the formation of sulfur oxides (SO2, SO3), nitrogen oxides (NO2, NO)
xi
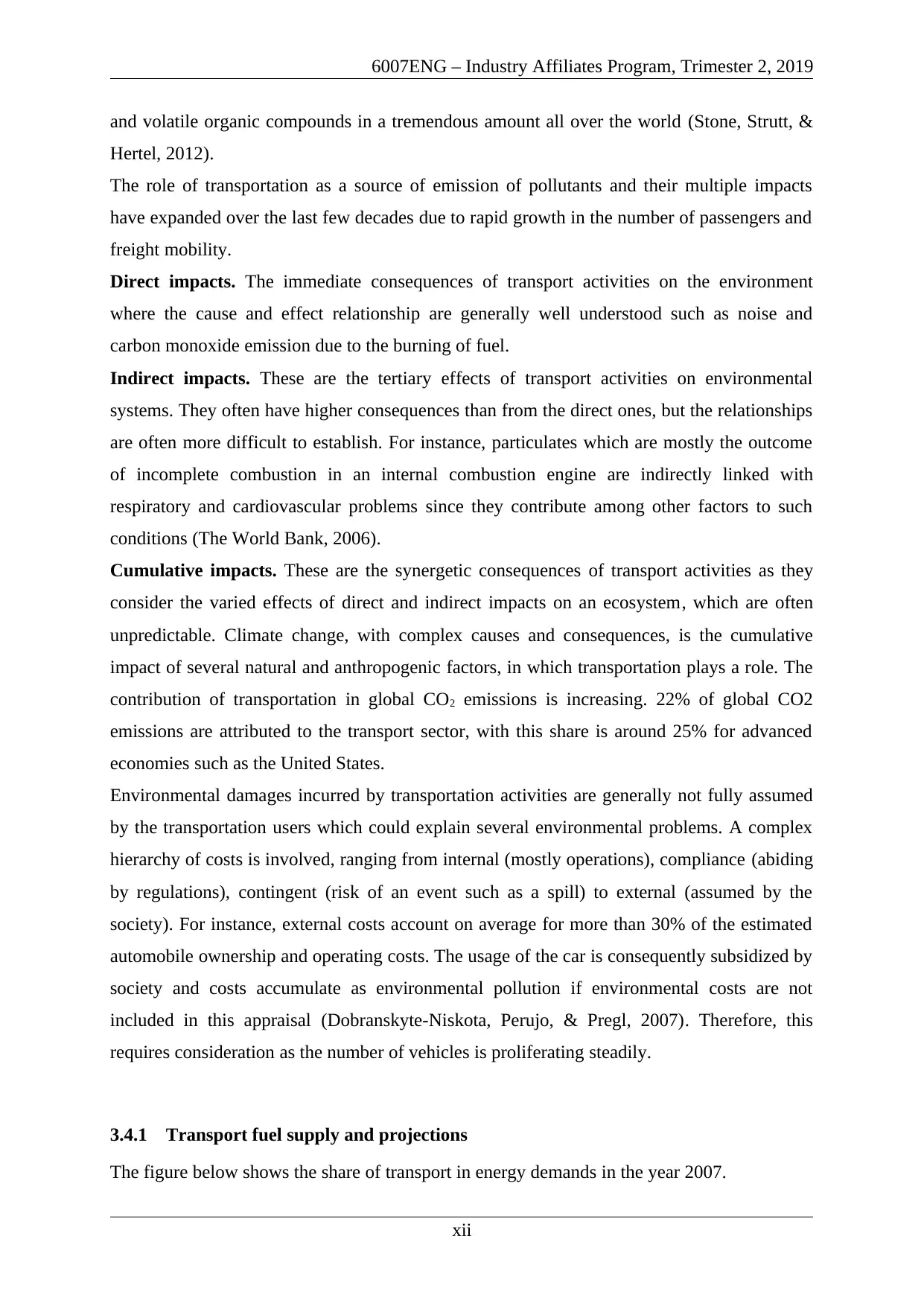
6007ENG – Industry Affiliates Program, Trimester 2, 2019
and volatile organic compounds in a tremendous amount all over the world (Stone, Strutt, &
Hertel, 2012).
The role of transportation as a source of emission of pollutants and their multiple impacts
have expanded over the last few decades due to rapid growth in the number of passengers and
freight mobility.
Direct impacts. The immediate consequences of transport activities on the environment
where the cause and effect relationship are generally well understood such as noise and
carbon monoxide emission due to the burning of fuel.
Indirect impacts. These are the tertiary effects of transport activities on environmental
systems. They often have higher consequences than from the direct ones, but the relationships
are often more difficult to establish. For instance, particulates which are mostly the outcome
of incomplete combustion in an internal combustion engine are indirectly linked with
respiratory and cardiovascular problems since they contribute among other factors to such
conditions (The World Bank, 2006).
Cumulative impacts. These are the synergetic consequences of transport activities as they
consider the varied effects of direct and indirect impacts on an ecosystem, which are often
unpredictable. Climate change, with complex causes and consequences, is the cumulative
impact of several natural and anthropogenic factors, in which transportation plays a role. The
contribution of transportation in global CO2 emissions is increasing. 22% of global CO2
emissions are attributed to the transport sector, with this share is around 25% for advanced
economies such as the United States.
Environmental damages incurred by transportation activities are generally not fully assumed
by the transportation users which could explain several environmental problems. A complex
hierarchy of costs is involved, ranging from internal (mostly operations), compliance (abiding
by regulations), contingent (risk of an event such as a spill) to external (assumed by the
society). For instance, external costs account on average for more than 30% of the estimated
automobile ownership and operating costs. The usage of the car is consequently subsidized by
society and costs accumulate as environmental pollution if environmental costs are not
included in this appraisal (Dobranskyte-Niskota, Perujo, & Pregl, 2007). Therefore, this
requires consideration as the number of vehicles is proliferating steadily.
3.4.1 Transport fuel supply and projections
The figure below shows the share of transport in energy demands in the year 2007.
xii
and volatile organic compounds in a tremendous amount all over the world (Stone, Strutt, &
Hertel, 2012).
The role of transportation as a source of emission of pollutants and their multiple impacts
have expanded over the last few decades due to rapid growth in the number of passengers and
freight mobility.
Direct impacts. The immediate consequences of transport activities on the environment
where the cause and effect relationship are generally well understood such as noise and
carbon monoxide emission due to the burning of fuel.
Indirect impacts. These are the tertiary effects of transport activities on environmental
systems. They often have higher consequences than from the direct ones, but the relationships
are often more difficult to establish. For instance, particulates which are mostly the outcome
of incomplete combustion in an internal combustion engine are indirectly linked with
respiratory and cardiovascular problems since they contribute among other factors to such
conditions (The World Bank, 2006).
Cumulative impacts. These are the synergetic consequences of transport activities as they
consider the varied effects of direct and indirect impacts on an ecosystem, which are often
unpredictable. Climate change, with complex causes and consequences, is the cumulative
impact of several natural and anthropogenic factors, in which transportation plays a role. The
contribution of transportation in global CO2 emissions is increasing. 22% of global CO2
emissions are attributed to the transport sector, with this share is around 25% for advanced
economies such as the United States.
Environmental damages incurred by transportation activities are generally not fully assumed
by the transportation users which could explain several environmental problems. A complex
hierarchy of costs is involved, ranging from internal (mostly operations), compliance (abiding
by regulations), contingent (risk of an event such as a spill) to external (assumed by the
society). For instance, external costs account on average for more than 30% of the estimated
automobile ownership and operating costs. The usage of the car is consequently subsidized by
society and costs accumulate as environmental pollution if environmental costs are not
included in this appraisal (Dobranskyte-Niskota, Perujo, & Pregl, 2007). Therefore, this
requires consideration as the number of vehicles is proliferating steadily.
3.4.1 Transport fuel supply and projections
The figure below shows the share of transport in energy demands in the year 2007.
xii
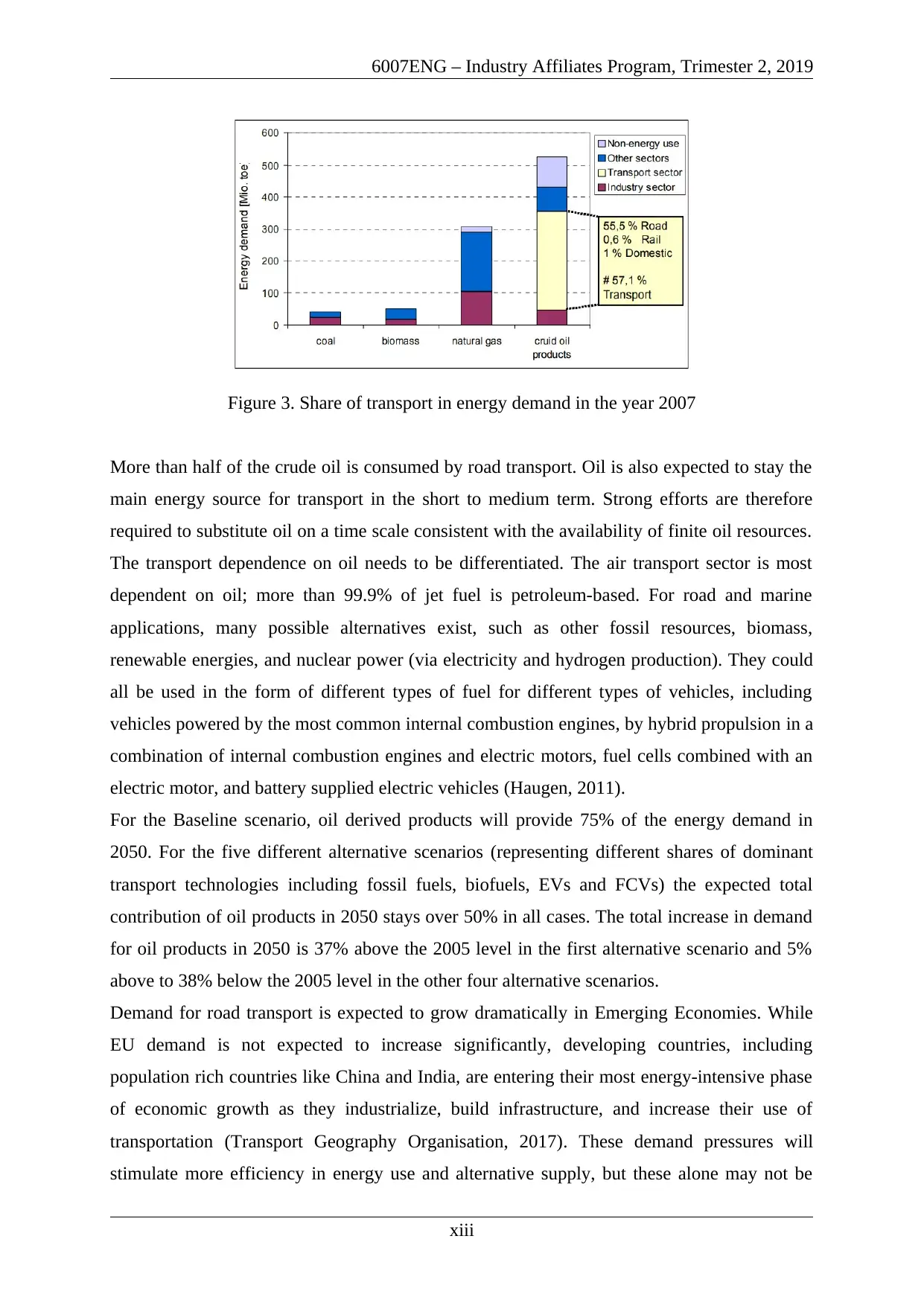
6007ENG – Industry Affiliates Program, Trimester 2, 2019
Figure 3. Share of transport in energy demand in the year 2007
More than half of the crude oil is consumed by road transport. Oil is also expected to stay the
main energy source for transport in the short to medium term. Strong efforts are therefore
required to substitute oil on a time scale consistent with the availability of finite oil resources.
The transport dependence on oil needs to be differentiated. The air transport sector is most
dependent on oil; more than 99.9% of jet fuel is petroleum-based. For road and marine
applications, many possible alternatives exist, such as other fossil resources, biomass,
renewable energies, and nuclear power (via electricity and hydrogen production). They could
all be used in the form of different types of fuel for different types of vehicles, including
vehicles powered by the most common internal combustion engines, by hybrid propulsion in a
combination of internal combustion engines and electric motors, fuel cells combined with an
electric motor, and battery supplied electric vehicles (Haugen, 2011).
For the Baseline scenario, oil derived products will provide 75% of the energy demand in
2050. For the five different alternative scenarios (representing different shares of dominant
transport technologies including fossil fuels, biofuels, EVs and FCVs) the expected total
contribution of oil products in 2050 stays over 50% in all cases. The total increase in demand
for oil products in 2050 is 37% above the 2005 level in the first alternative scenario and 5%
above to 38% below the 2005 level in the other four alternative scenarios.
Demand for road transport is expected to grow dramatically in Emerging Economies. While
EU demand is not expected to increase significantly, developing countries, including
population rich countries like China and India, are entering their most energy-intensive phase
of economic growth as they industrialize, build infrastructure, and increase their use of
transportation (Transport Geography Organisation, 2017). These demand pressures will
stimulate more efficiency in energy use and alternative supply, but these alone may not be
xiii
Figure 3. Share of transport in energy demand in the year 2007
More than half of the crude oil is consumed by road transport. Oil is also expected to stay the
main energy source for transport in the short to medium term. Strong efforts are therefore
required to substitute oil on a time scale consistent with the availability of finite oil resources.
The transport dependence on oil needs to be differentiated. The air transport sector is most
dependent on oil; more than 99.9% of jet fuel is petroleum-based. For road and marine
applications, many possible alternatives exist, such as other fossil resources, biomass,
renewable energies, and nuclear power (via electricity and hydrogen production). They could
all be used in the form of different types of fuel for different types of vehicles, including
vehicles powered by the most common internal combustion engines, by hybrid propulsion in a
combination of internal combustion engines and electric motors, fuel cells combined with an
electric motor, and battery supplied electric vehicles (Haugen, 2011).
For the Baseline scenario, oil derived products will provide 75% of the energy demand in
2050. For the five different alternative scenarios (representing different shares of dominant
transport technologies including fossil fuels, biofuels, EVs and FCVs) the expected total
contribution of oil products in 2050 stays over 50% in all cases. The total increase in demand
for oil products in 2050 is 37% above the 2005 level in the first alternative scenario and 5%
above to 38% below the 2005 level in the other four alternative scenarios.
Demand for road transport is expected to grow dramatically in Emerging Economies. While
EU demand is not expected to increase significantly, developing countries, including
population rich countries like China and India, are entering their most energy-intensive phase
of economic growth as they industrialize, build infrastructure, and increase their use of
transportation (Transport Geography Organisation, 2017). These demand pressures will
stimulate more efficiency in energy use and alternative supply, but these alone may not be
xiii
Secure Best Marks with AI Grader
Need help grading? Try our AI Grader for instant feedback on your assignments.
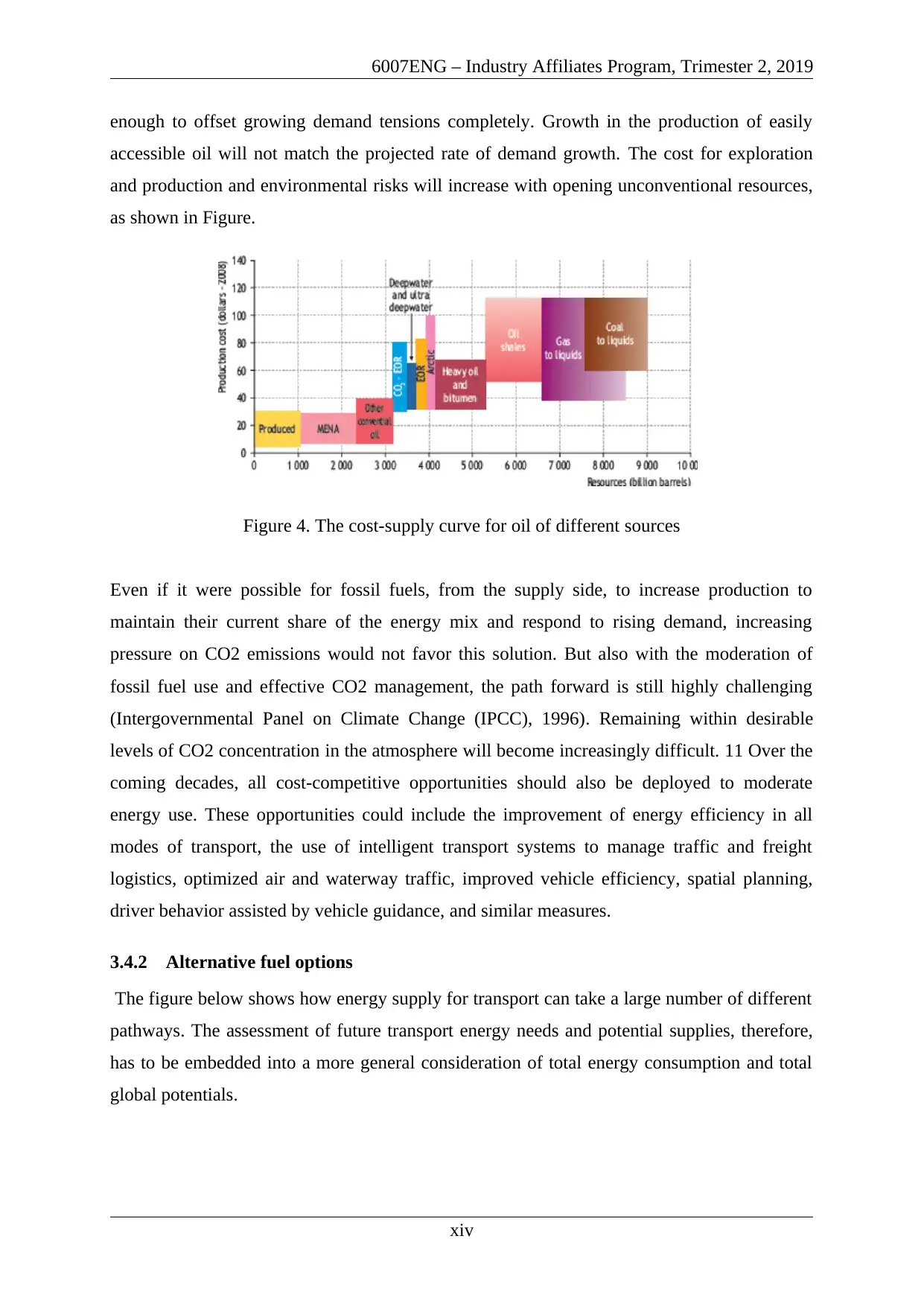
6007ENG – Industry Affiliates Program, Trimester 2, 2019
enough to offset growing demand tensions completely. Growth in the production of easily
accessible oil will not match the projected rate of demand growth. The cost for exploration
and production and environmental risks will increase with opening unconventional resources,
as shown in Figure.
Figure 4. The cost-supply curve for oil of different sources
Even if it were possible for fossil fuels, from the supply side, to increase production to
maintain their current share of the energy mix and respond to rising demand, increasing
pressure on CO2 emissions would not favor this solution. But also with the moderation of
fossil fuel use and effective CO2 management, the path forward is still highly challenging
(Intergovernmental Panel on Climate Change (IPCC), 1996). Remaining within desirable
levels of CO2 concentration in the atmosphere will become increasingly difficult. 11 Over the
coming decades, all cost-competitive opportunities should also be deployed to moderate
energy use. These opportunities could include the improvement of energy efficiency in all
modes of transport, the use of intelligent transport systems to manage traffic and freight
logistics, optimized air and waterway traffic, improved vehicle efficiency, spatial planning,
driver behavior assisted by vehicle guidance, and similar measures.
3.4.2 Alternative fuel options
The figure below shows how energy supply for transport can take a large number of different
pathways. The assessment of future transport energy needs and potential supplies, therefore,
has to be embedded into a more general consideration of total energy consumption and total
global potentials.
xiv
enough to offset growing demand tensions completely. Growth in the production of easily
accessible oil will not match the projected rate of demand growth. The cost for exploration
and production and environmental risks will increase with opening unconventional resources,
as shown in Figure.
Figure 4. The cost-supply curve for oil of different sources
Even if it were possible for fossil fuels, from the supply side, to increase production to
maintain their current share of the energy mix and respond to rising demand, increasing
pressure on CO2 emissions would not favor this solution. But also with the moderation of
fossil fuel use and effective CO2 management, the path forward is still highly challenging
(Intergovernmental Panel on Climate Change (IPCC), 1996). Remaining within desirable
levels of CO2 concentration in the atmosphere will become increasingly difficult. 11 Over the
coming decades, all cost-competitive opportunities should also be deployed to moderate
energy use. These opportunities could include the improvement of energy efficiency in all
modes of transport, the use of intelligent transport systems to manage traffic and freight
logistics, optimized air and waterway traffic, improved vehicle efficiency, spatial planning,
driver behavior assisted by vehicle guidance, and similar measures.
3.4.2 Alternative fuel options
The figure below shows how energy supply for transport can take a large number of different
pathways. The assessment of future transport energy needs and potential supplies, therefore,
has to be embedded into a more general consideration of total energy consumption and total
global potentials.
xiv
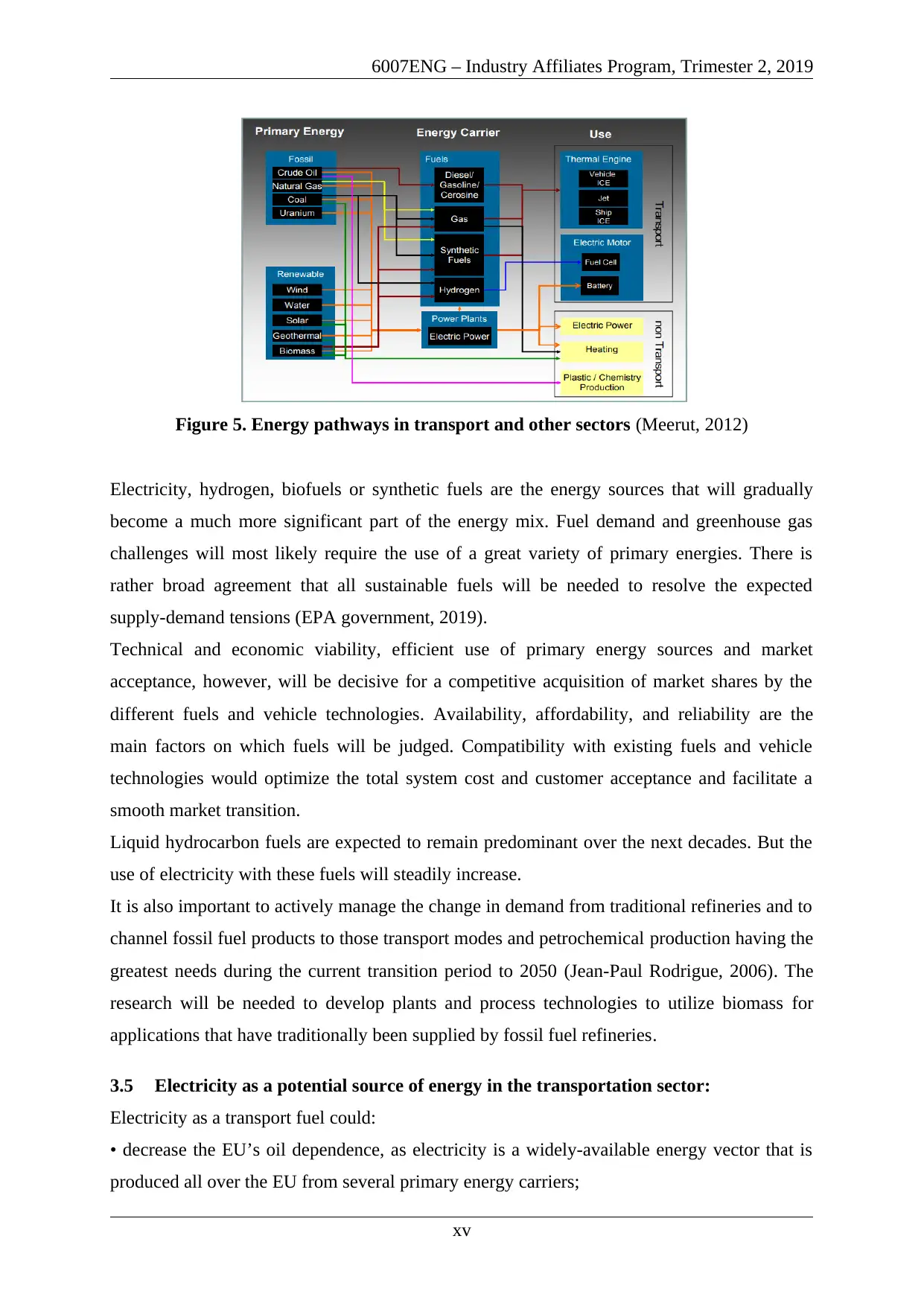
6007ENG – Industry Affiliates Program, Trimester 2, 2019
Figure 5. Energy pathways in transport and other sectors (Meerut, 2012)
Electricity, hydrogen, biofuels or synthetic fuels are the energy sources that will gradually
become a much more significant part of the energy mix. Fuel demand and greenhouse gas
challenges will most likely require the use of a great variety of primary energies. There is
rather broad agreement that all sustainable fuels will be needed to resolve the expected
supply-demand tensions (EPA government, 2019).
Technical and economic viability, efficient use of primary energy sources and market
acceptance, however, will be decisive for a competitive acquisition of market shares by the
different fuels and vehicle technologies. Availability, affordability, and reliability are the
main factors on which fuels will be judged. Compatibility with existing fuels and vehicle
technologies would optimize the total system cost and customer acceptance and facilitate a
smooth market transition.
Liquid hydrocarbon fuels are expected to remain predominant over the next decades. But the
use of electricity with these fuels will steadily increase.
It is also important to actively manage the change in demand from traditional refineries and to
channel fossil fuel products to those transport modes and petrochemical production having the
greatest needs during the current transition period to 2050 (Jean-Paul Rodrigue, 2006). The
research will be needed to develop plants and process technologies to utilize biomass for
applications that have traditionally been supplied by fossil fuel refineries.
3.5 Electricity as a potential source of energy in the transportation sector:
Electricity as a transport fuel could:
• decrease the EU’s oil dependence, as electricity is a widely‐available energy vector that is
produced all over the EU from several primary energy carriers;
xv
Figure 5. Energy pathways in transport and other sectors (Meerut, 2012)
Electricity, hydrogen, biofuels or synthetic fuels are the energy sources that will gradually
become a much more significant part of the energy mix. Fuel demand and greenhouse gas
challenges will most likely require the use of a great variety of primary energies. There is
rather broad agreement that all sustainable fuels will be needed to resolve the expected
supply-demand tensions (EPA government, 2019).
Technical and economic viability, efficient use of primary energy sources and market
acceptance, however, will be decisive for a competitive acquisition of market shares by the
different fuels and vehicle technologies. Availability, affordability, and reliability are the
main factors on which fuels will be judged. Compatibility with existing fuels and vehicle
technologies would optimize the total system cost and customer acceptance and facilitate a
smooth market transition.
Liquid hydrocarbon fuels are expected to remain predominant over the next decades. But the
use of electricity with these fuels will steadily increase.
It is also important to actively manage the change in demand from traditional refineries and to
channel fossil fuel products to those transport modes and petrochemical production having the
greatest needs during the current transition period to 2050 (Jean-Paul Rodrigue, 2006). The
research will be needed to develop plants and process technologies to utilize biomass for
applications that have traditionally been supplied by fossil fuel refineries.
3.5 Electricity as a potential source of energy in the transportation sector:
Electricity as a transport fuel could:
• decrease the EU’s oil dependence, as electricity is a widely‐available energy vector that is
produced all over the EU from several primary energy carriers;
xv
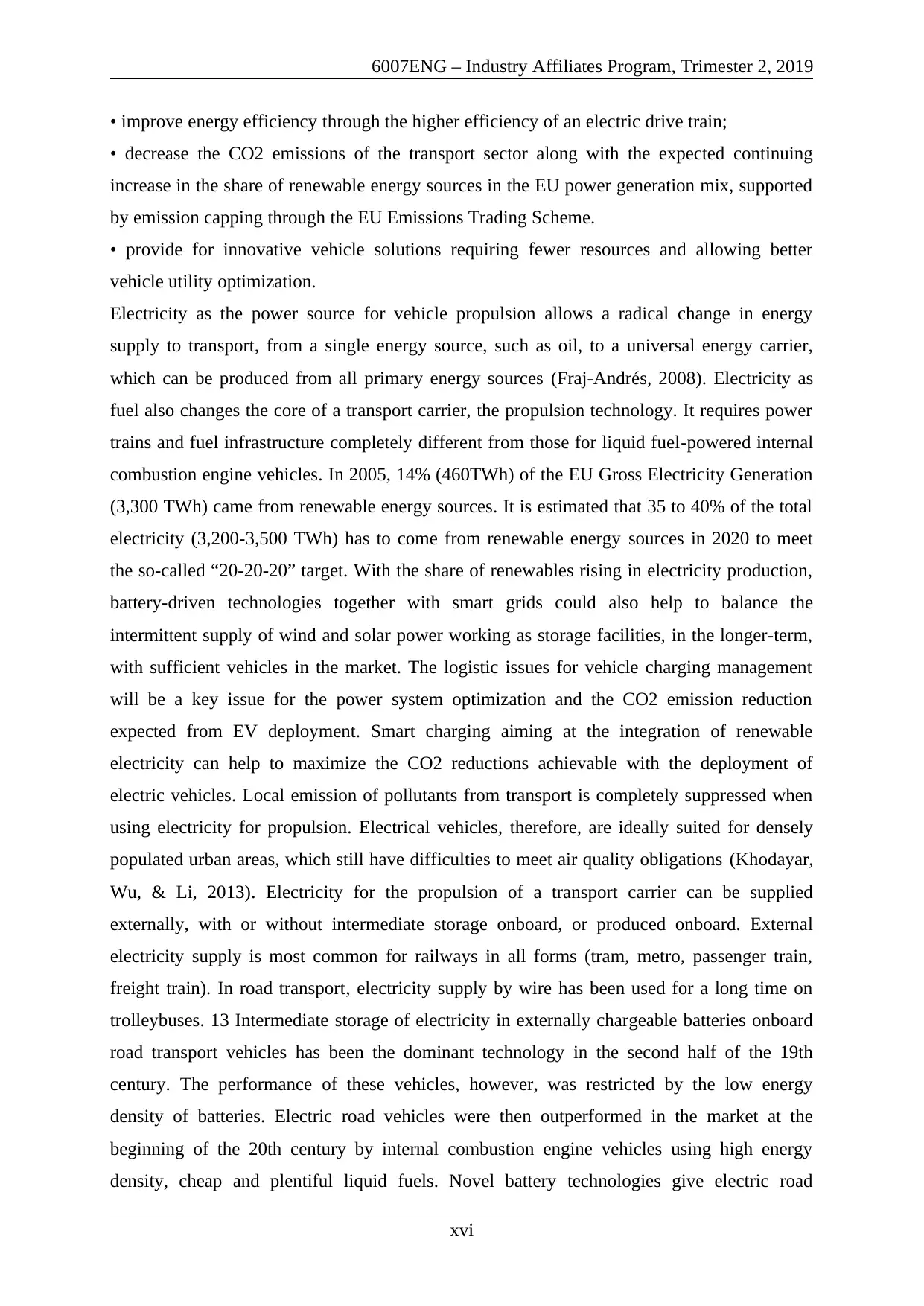
6007ENG – Industry Affiliates Program, Trimester 2, 2019
• improve energy efficiency through the higher efficiency of an electric drive train;
• decrease the CO2 emissions of the transport sector along with the expected continuing
increase in the share of renewable energy sources in the EU power generation mix, supported
by emission capping through the EU Emissions Trading Scheme.
• provide for innovative vehicle solutions requiring fewer resources and allowing better
vehicle utility optimization.
Electricity as the power source for vehicle propulsion allows a radical change in energy
supply to transport, from a single energy source, such as oil, to a universal energy carrier,
which can be produced from all primary energy sources (Fraj-Andrés, 2008). Electricity as
fuel also changes the core of a transport carrier, the propulsion technology. It requires power
trains and fuel infrastructure completely different from those for liquid fuel-powered internal
combustion engine vehicles. In 2005, 14% (460TWh) of the EU Gross Electricity Generation
(3,300 TWh) came from renewable energy sources. It is estimated that 35 to 40% of the total
electricity (3,200-3,500 TWh) has to come from renewable energy sources in 2020 to meet
the so-called “20-20-20” target. With the share of renewables rising in electricity production,
battery-driven technologies together with smart grids could also help to balance the
intermittent supply of wind and solar power working as storage facilities, in the longer-term,
with sufficient vehicles in the market. The logistic issues for vehicle charging management
will be a key issue for the power system optimization and the CO2 emission reduction
expected from EV deployment. Smart charging aiming at the integration of renewable
electricity can help to maximize the CO2 reductions achievable with the deployment of
electric vehicles. Local emission of pollutants from transport is completely suppressed when
using electricity for propulsion. Electrical vehicles, therefore, are ideally suited for densely
populated urban areas, which still have difficulties to meet air quality obligations (Khodayar,
Wu, & Li, 2013). Electricity for the propulsion of a transport carrier can be supplied
externally, with or without intermediate storage onboard, or produced onboard. External
electricity supply is most common for railways in all forms (tram, metro, passenger train,
freight train). In road transport, electricity supply by wire has been used for a long time on
trolleybuses. 13 Intermediate storage of electricity in externally chargeable batteries onboard
road transport vehicles has been the dominant technology in the second half of the 19th
century. The performance of these vehicles, however, was restricted by the low energy
density of batteries. Electric road vehicles were then outperformed in the market at the
beginning of the 20th century by internal combustion engine vehicles using high energy
density, cheap and plentiful liquid fuels. Novel battery technologies give electric road
xvi
• improve energy efficiency through the higher efficiency of an electric drive train;
• decrease the CO2 emissions of the transport sector along with the expected continuing
increase in the share of renewable energy sources in the EU power generation mix, supported
by emission capping through the EU Emissions Trading Scheme.
• provide for innovative vehicle solutions requiring fewer resources and allowing better
vehicle utility optimization.
Electricity as the power source for vehicle propulsion allows a radical change in energy
supply to transport, from a single energy source, such as oil, to a universal energy carrier,
which can be produced from all primary energy sources (Fraj-Andrés, 2008). Electricity as
fuel also changes the core of a transport carrier, the propulsion technology. It requires power
trains and fuel infrastructure completely different from those for liquid fuel-powered internal
combustion engine vehicles. In 2005, 14% (460TWh) of the EU Gross Electricity Generation
(3,300 TWh) came from renewable energy sources. It is estimated that 35 to 40% of the total
electricity (3,200-3,500 TWh) has to come from renewable energy sources in 2020 to meet
the so-called “20-20-20” target. With the share of renewables rising in electricity production,
battery-driven technologies together with smart grids could also help to balance the
intermittent supply of wind and solar power working as storage facilities, in the longer-term,
with sufficient vehicles in the market. The logistic issues for vehicle charging management
will be a key issue for the power system optimization and the CO2 emission reduction
expected from EV deployment. Smart charging aiming at the integration of renewable
electricity can help to maximize the CO2 reductions achievable with the deployment of
electric vehicles. Local emission of pollutants from transport is completely suppressed when
using electricity for propulsion. Electrical vehicles, therefore, are ideally suited for densely
populated urban areas, which still have difficulties to meet air quality obligations (Khodayar,
Wu, & Li, 2013). Electricity for the propulsion of a transport carrier can be supplied
externally, with or without intermediate storage onboard, or produced onboard. External
electricity supply is most common for railways in all forms (tram, metro, passenger train,
freight train). In road transport, electricity supply by wire has been used for a long time on
trolleybuses. 13 Intermediate storage of electricity in externally chargeable batteries onboard
road transport vehicles has been the dominant technology in the second half of the 19th
century. The performance of these vehicles, however, was restricted by the low energy
density of batteries. Electric road vehicles were then outperformed in the market at the
beginning of the 20th century by internal combustion engine vehicles using high energy
density, cheap and plentiful liquid fuels. Novel battery technologies give electric road
xvi
Paraphrase This Document
Need a fresh take? Get an instant paraphrase of this document with our AI Paraphraser
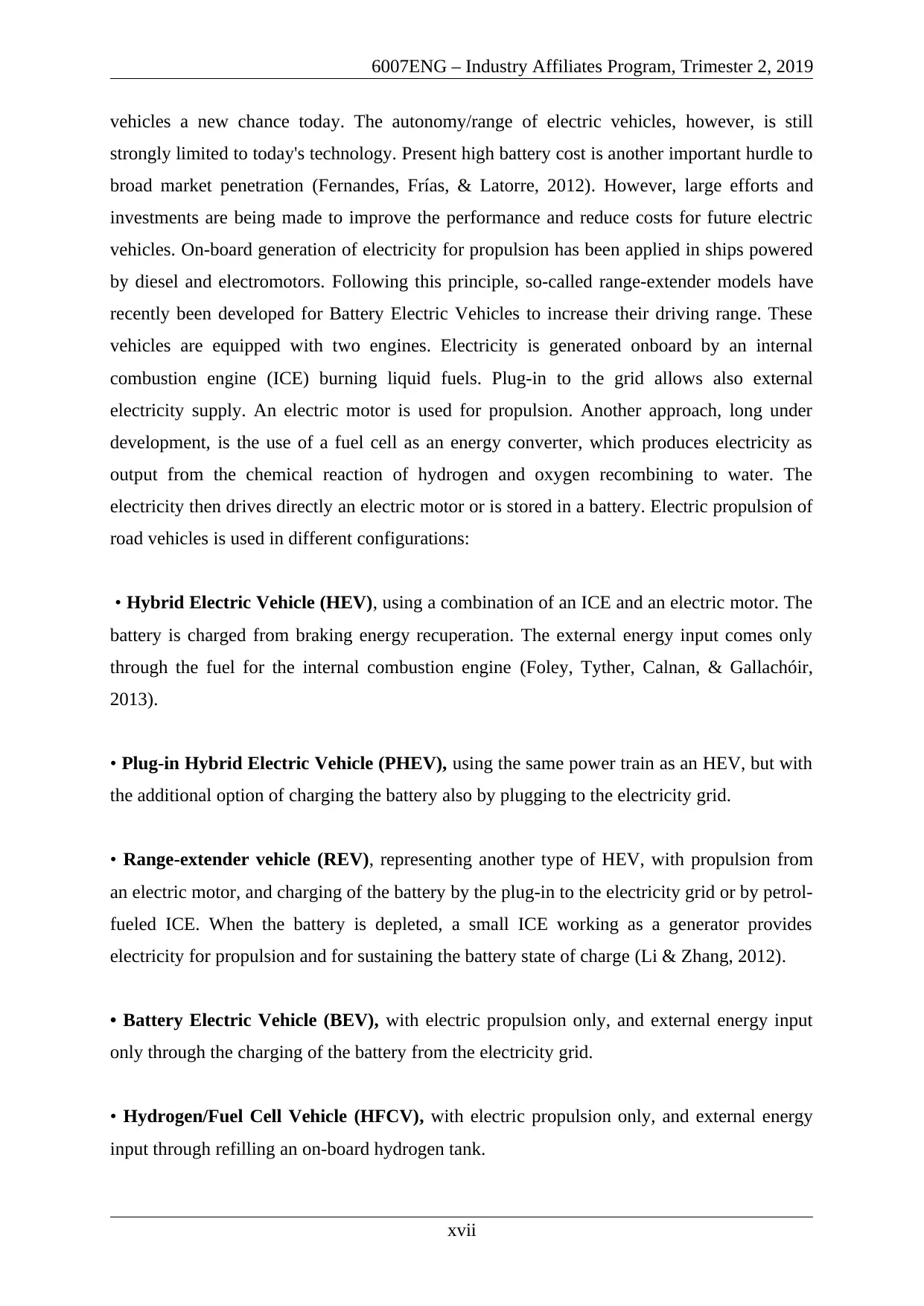
6007ENG – Industry Affiliates Program, Trimester 2, 2019
vehicles a new chance today. The autonomy/range of electric vehicles, however, is still
strongly limited to today's technology. Present high battery cost is another important hurdle to
broad market penetration (Fernandes, Frías, & Latorre, 2012). However, large efforts and
investments are being made to improve the performance and reduce costs for future electric
vehicles. On-board generation of electricity for propulsion has been applied in ships powered
by diesel and electromotors. Following this principle, so-called range-extender models have
recently been developed for Battery Electric Vehicles to increase their driving range. These
vehicles are equipped with two engines. Electricity is generated onboard by an internal
combustion engine (ICE) burning liquid fuels. Plug-in to the grid allows also external
electricity supply. An electric motor is used for propulsion. Another approach, long under
development, is the use of a fuel cell as an energy converter, which produces electricity as
output from the chemical reaction of hydrogen and oxygen recombining to water. The
electricity then drives directly an electric motor or is stored in a battery. Electric propulsion of
road vehicles is used in different configurations:
• Hybrid Electric Vehicle (HEV), using a combination of an ICE and an electric motor. The
battery is charged from braking energy recuperation. The external energy input comes only
through the fuel for the internal combustion engine (Foley, Tyther, Calnan, & Gallachóir,
2013).
• Plug-in Hybrid Electric Vehicle (PHEV), using the same power train as an HEV, but with
the additional option of charging the battery also by plugging to the electricity grid.
• Range-extender vehicle (REV), representing another type of HEV, with propulsion from
an electric motor, and charging of the battery by the plug-in to the electricity grid or by petrol-
fueled ICE. When the battery is depleted, a small ICE working as a generator provides
electricity for propulsion and for sustaining the battery state of charge (Li & Zhang, 2012).
• Battery Electric Vehicle (BEV), with electric propulsion only, and external energy input
only through the charging of the battery from the electricity grid.
• Hydrogen/Fuel Cell Vehicle (HFCV), with electric propulsion only, and external energy
input through refilling an on-board hydrogen tank.
xvii
vehicles a new chance today. The autonomy/range of electric vehicles, however, is still
strongly limited to today's technology. Present high battery cost is another important hurdle to
broad market penetration (Fernandes, Frías, & Latorre, 2012). However, large efforts and
investments are being made to improve the performance and reduce costs for future electric
vehicles. On-board generation of electricity for propulsion has been applied in ships powered
by diesel and electromotors. Following this principle, so-called range-extender models have
recently been developed for Battery Electric Vehicles to increase their driving range. These
vehicles are equipped with two engines. Electricity is generated onboard by an internal
combustion engine (ICE) burning liquid fuels. Plug-in to the grid allows also external
electricity supply. An electric motor is used for propulsion. Another approach, long under
development, is the use of a fuel cell as an energy converter, which produces electricity as
output from the chemical reaction of hydrogen and oxygen recombining to water. The
electricity then drives directly an electric motor or is stored in a battery. Electric propulsion of
road vehicles is used in different configurations:
• Hybrid Electric Vehicle (HEV), using a combination of an ICE and an electric motor. The
battery is charged from braking energy recuperation. The external energy input comes only
through the fuel for the internal combustion engine (Foley, Tyther, Calnan, & Gallachóir,
2013).
• Plug-in Hybrid Electric Vehicle (PHEV), using the same power train as an HEV, but with
the additional option of charging the battery also by plugging to the electricity grid.
• Range-extender vehicle (REV), representing another type of HEV, with propulsion from
an electric motor, and charging of the battery by the plug-in to the electricity grid or by petrol-
fueled ICE. When the battery is depleted, a small ICE working as a generator provides
electricity for propulsion and for sustaining the battery state of charge (Li & Zhang, 2012).
• Battery Electric Vehicle (BEV), with electric propulsion only, and external energy input
only through the charging of the battery from the electricity grid.
• Hydrogen/Fuel Cell Vehicle (HFCV), with electric propulsion only, and external energy
input through refilling an on-board hydrogen tank.
xvii
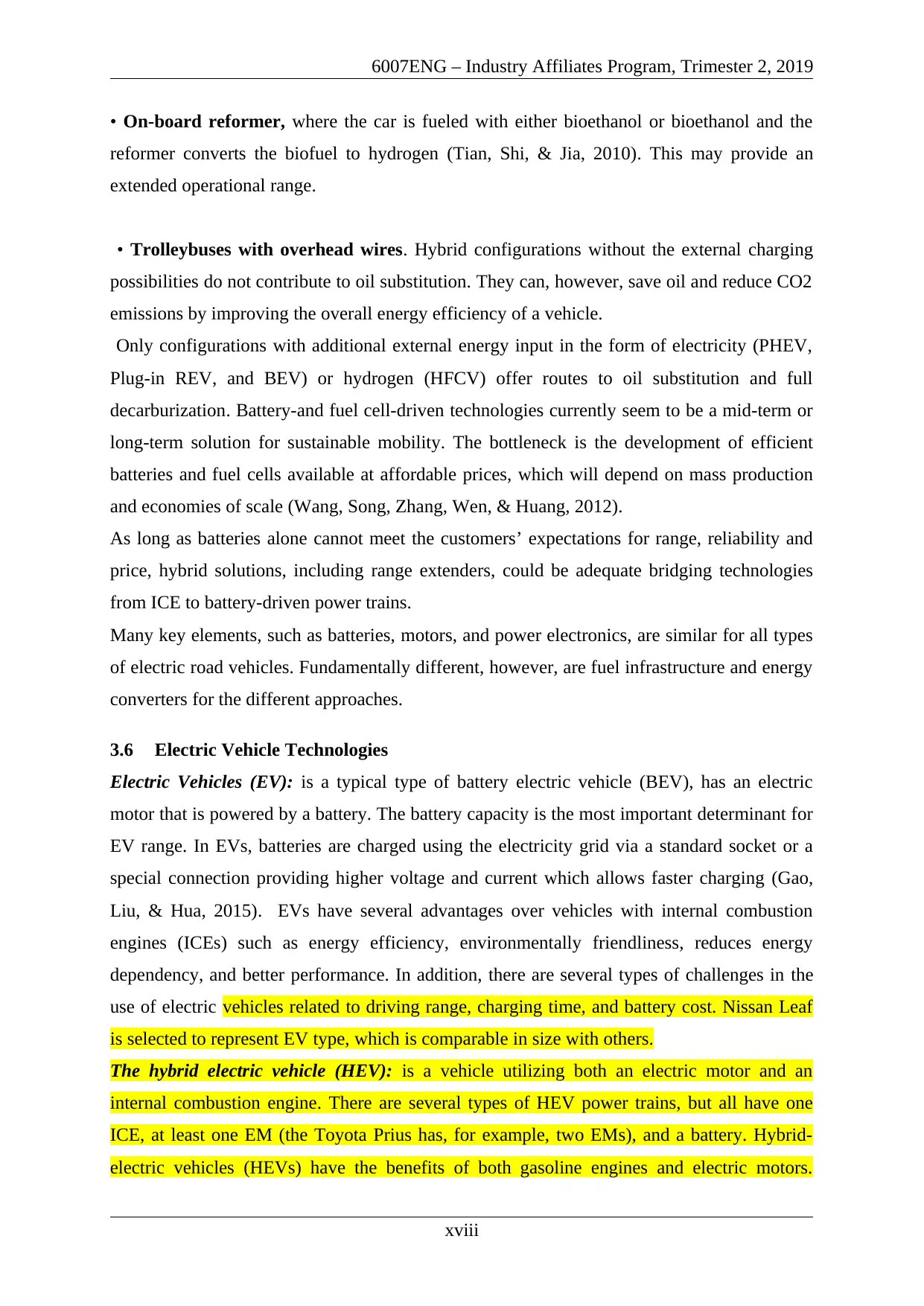
6007ENG – Industry Affiliates Program, Trimester 2, 2019
• On-board reformer, where the car is fueled with either bioethanol or bioethanol and the
reformer converts the biofuel to hydrogen (Tian, Shi, & Jia, 2010). This may provide an
extended operational range.
• Trolleybuses with overhead wires. Hybrid configurations without the external charging
possibilities do not contribute to oil substitution. They can, however, save oil and reduce CO2
emissions by improving the overall energy efficiency of a vehicle.
Only configurations with additional external energy input in the form of electricity (PHEV,
Plug-in REV, and BEV) or hydrogen (HFCV) offer routes to oil substitution and full
decarburization. Battery-and fuel cell-driven technologies currently seem to be a mid-term or
long-term solution for sustainable mobility. The bottleneck is the development of efficient
batteries and fuel cells available at affordable prices, which will depend on mass production
and economies of scale (Wang, Song, Zhang, Wen, & Huang, 2012).
As long as batteries alone cannot meet the customers’ expectations for range, reliability and
price, hybrid solutions, including range extenders, could be adequate bridging technologies
from ICE to battery-driven power trains.
Many key elements, such as batteries, motors, and power electronics, are similar for all types
of electric road vehicles. Fundamentally different, however, are fuel infrastructure and energy
converters for the different approaches.
3.6 Electric Vehicle Technologies
Electric Vehicles (EV): is a typical type of battery electric vehicle (BEV), has an electric
motor that is powered by a battery. The battery capacity is the most important determinant for
EV range. In EVs, batteries are charged using the electricity grid via a standard socket or a
special connection providing higher voltage and current which allows faster charging (Gao,
Liu, & Hua, 2015). EVs have several advantages over vehicles with internal combustion
engines (ICEs) such as energy efficiency, environmentally friendliness, reduces energy
dependency, and better performance. In addition, there are several types of challenges in the
use of electric vehicles related to driving range, charging time, and battery cost. Nissan Leaf
is selected to represent EV type, which is comparable in size with others.
The hybrid electric vehicle (HEV): is a vehicle utilizing both an electric motor and an
internal combustion engine. There are several types of HEV power trains, but all have one
ICE, at least one EM (the Toyota Prius has, for example, two EMs), and a battery. Hybrid-
electric vehicles (HEVs) have the benefits of both gasoline engines and electric motors.
xviii
• On-board reformer, where the car is fueled with either bioethanol or bioethanol and the
reformer converts the biofuel to hydrogen (Tian, Shi, & Jia, 2010). This may provide an
extended operational range.
• Trolleybuses with overhead wires. Hybrid configurations without the external charging
possibilities do not contribute to oil substitution. They can, however, save oil and reduce CO2
emissions by improving the overall energy efficiency of a vehicle.
Only configurations with additional external energy input in the form of electricity (PHEV,
Plug-in REV, and BEV) or hydrogen (HFCV) offer routes to oil substitution and full
decarburization. Battery-and fuel cell-driven technologies currently seem to be a mid-term or
long-term solution for sustainable mobility. The bottleneck is the development of efficient
batteries and fuel cells available at affordable prices, which will depend on mass production
and economies of scale (Wang, Song, Zhang, Wen, & Huang, 2012).
As long as batteries alone cannot meet the customers’ expectations for range, reliability and
price, hybrid solutions, including range extenders, could be adequate bridging technologies
from ICE to battery-driven power trains.
Many key elements, such as batteries, motors, and power electronics, are similar for all types
of electric road vehicles. Fundamentally different, however, are fuel infrastructure and energy
converters for the different approaches.
3.6 Electric Vehicle Technologies
Electric Vehicles (EV): is a typical type of battery electric vehicle (BEV), has an electric
motor that is powered by a battery. The battery capacity is the most important determinant for
EV range. In EVs, batteries are charged using the electricity grid via a standard socket or a
special connection providing higher voltage and current which allows faster charging (Gao,
Liu, & Hua, 2015). EVs have several advantages over vehicles with internal combustion
engines (ICEs) such as energy efficiency, environmentally friendliness, reduces energy
dependency, and better performance. In addition, there are several types of challenges in the
use of electric vehicles related to driving range, charging time, and battery cost. Nissan Leaf
is selected to represent EV type, which is comparable in size with others.
The hybrid electric vehicle (HEV): is a vehicle utilizing both an electric motor and an
internal combustion engine. There are several types of HEV power trains, but all have one
ICE, at least one EM (the Toyota Prius has, for example, two EMs), and a battery. Hybrid-
electric vehicles (HEVs) have the benefits of both gasoline engines and electric motors.
xviii
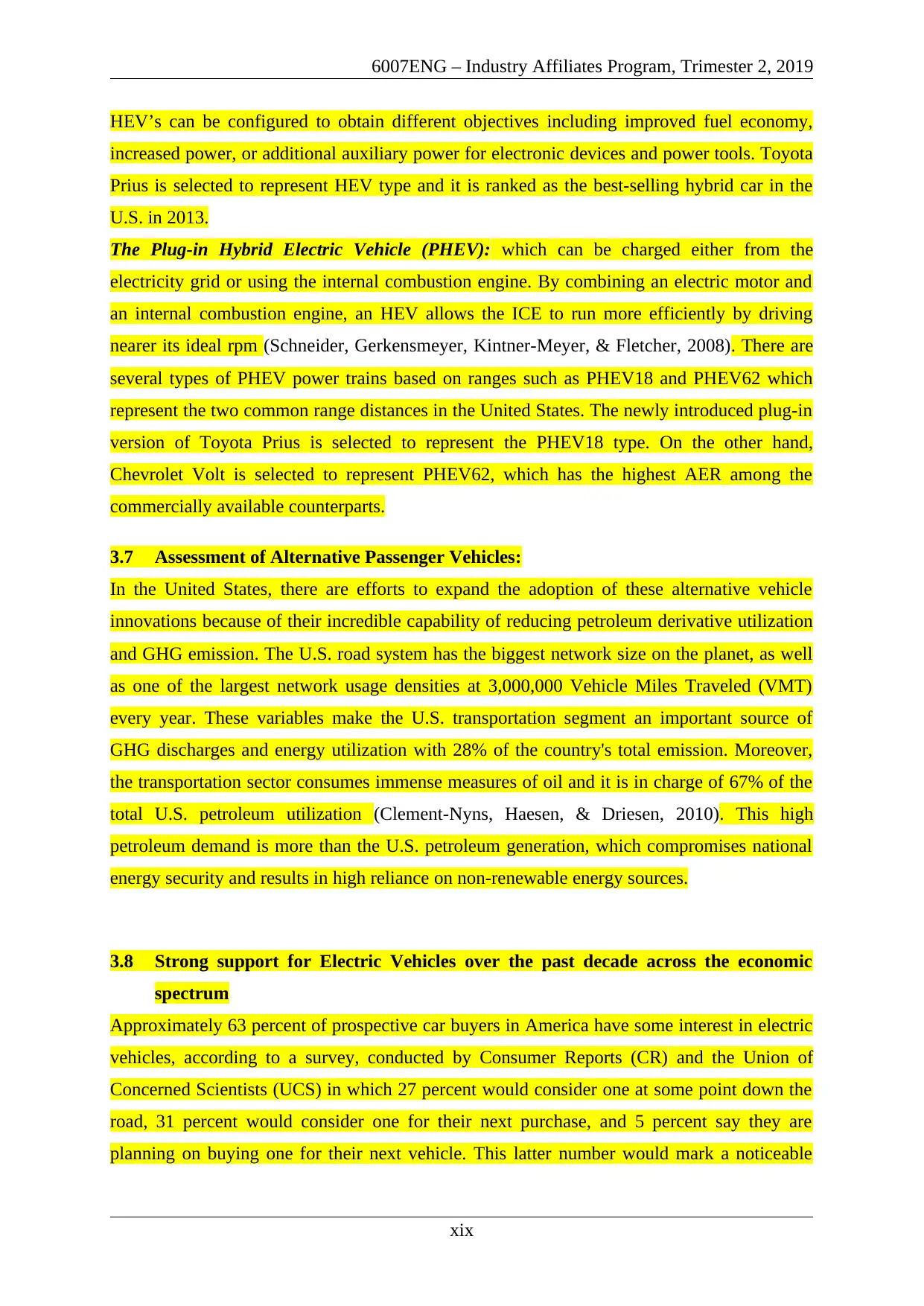
6007ENG – Industry Affiliates Program, Trimester 2, 2019
HEV’s can be configured to obtain different objectives including improved fuel economy,
increased power, or additional auxiliary power for electronic devices and power tools. Toyota
Prius is selected to represent HEV type and it is ranked as the best-selling hybrid car in the
U.S. in 2013.
The Plug-in Hybrid Electric Vehicle (PHEV): which can be charged either from the
electricity grid or using the internal combustion engine. By combining an electric motor and
an internal combustion engine, an HEV allows the ICE to run more efficiently by driving
nearer its ideal rpm (Schneider, Gerkensmeyer, Kintner-Meyer, & Fletcher, 2008). There are
several types of PHEV power trains based on ranges such as PHEV18 and PHEV62 which
represent the two common range distances in the United States. The newly introduced plug-in
version of Toyota Prius is selected to represent the PHEV18 type. On the other hand,
Chevrolet Volt is selected to represent PHEV62, which has the highest AER among the
commercially available counterparts.
3.7 Assessment of Alternative Passenger Vehicles:
In the United States, there are efforts to expand the adoption of these alternative vehicle
innovations because of their incredible capability of reducing petroleum derivative utilization
and GHG emission. The U.S. road system has the biggest network size on the planet, as well
as one of the largest network usage densities at 3,000,000 Vehicle Miles Traveled (VMT)
every year. These variables make the U.S. transportation segment an important source of
GHG discharges and energy utilization with 28% of the country's total emission. Moreover,
the transportation sector consumes immense measures of oil and it is in charge of 67% of the
total U.S. petroleum utilization (Clement-Nyns, Haesen, & Driesen, 2010). This high
petroleum demand is more than the U.S. petroleum generation, which compromises national
energy security and results in high reliance on non-renewable energy sources.
3.8 Strong support for Electric Vehicles over the past decade across the economic
spectrum
Approximately 63 percent of prospective car buyers in America have some interest in electric
vehicles, according to a survey, conducted by Consumer Reports (CR) and the Union of
Concerned Scientists (UCS) in which 27 percent would consider one at some point down the
road, 31 percent would consider one for their next purchase, and 5 percent say they are
planning on buying one for their next vehicle. This latter number would mark a noticeable
xix
HEV’s can be configured to obtain different objectives including improved fuel economy,
increased power, or additional auxiliary power for electronic devices and power tools. Toyota
Prius is selected to represent HEV type and it is ranked as the best-selling hybrid car in the
U.S. in 2013.
The Plug-in Hybrid Electric Vehicle (PHEV): which can be charged either from the
electricity grid or using the internal combustion engine. By combining an electric motor and
an internal combustion engine, an HEV allows the ICE to run more efficiently by driving
nearer its ideal rpm (Schneider, Gerkensmeyer, Kintner-Meyer, & Fletcher, 2008). There are
several types of PHEV power trains based on ranges such as PHEV18 and PHEV62 which
represent the two common range distances in the United States. The newly introduced plug-in
version of Toyota Prius is selected to represent the PHEV18 type. On the other hand,
Chevrolet Volt is selected to represent PHEV62, which has the highest AER among the
commercially available counterparts.
3.7 Assessment of Alternative Passenger Vehicles:
In the United States, there are efforts to expand the adoption of these alternative vehicle
innovations because of their incredible capability of reducing petroleum derivative utilization
and GHG emission. The U.S. road system has the biggest network size on the planet, as well
as one of the largest network usage densities at 3,000,000 Vehicle Miles Traveled (VMT)
every year. These variables make the U.S. transportation segment an important source of
GHG discharges and energy utilization with 28% of the country's total emission. Moreover,
the transportation sector consumes immense measures of oil and it is in charge of 67% of the
total U.S. petroleum utilization (Clement-Nyns, Haesen, & Driesen, 2010). This high
petroleum demand is more than the U.S. petroleum generation, which compromises national
energy security and results in high reliance on non-renewable energy sources.
3.8 Strong support for Electric Vehicles over the past decade across the economic
spectrum
Approximately 63 percent of prospective car buyers in America have some interest in electric
vehicles, according to a survey, conducted by Consumer Reports (CR) and the Union of
Concerned Scientists (UCS) in which 27 percent would consider one at some point down the
road, 31 percent would consider one for their next purchase, and 5 percent say they are
planning on buying one for their next vehicle. This latter number would mark a noticeable
xix
Secure Best Marks with AI Grader
Need help grading? Try our AI Grader for instant feedback on your assignments.
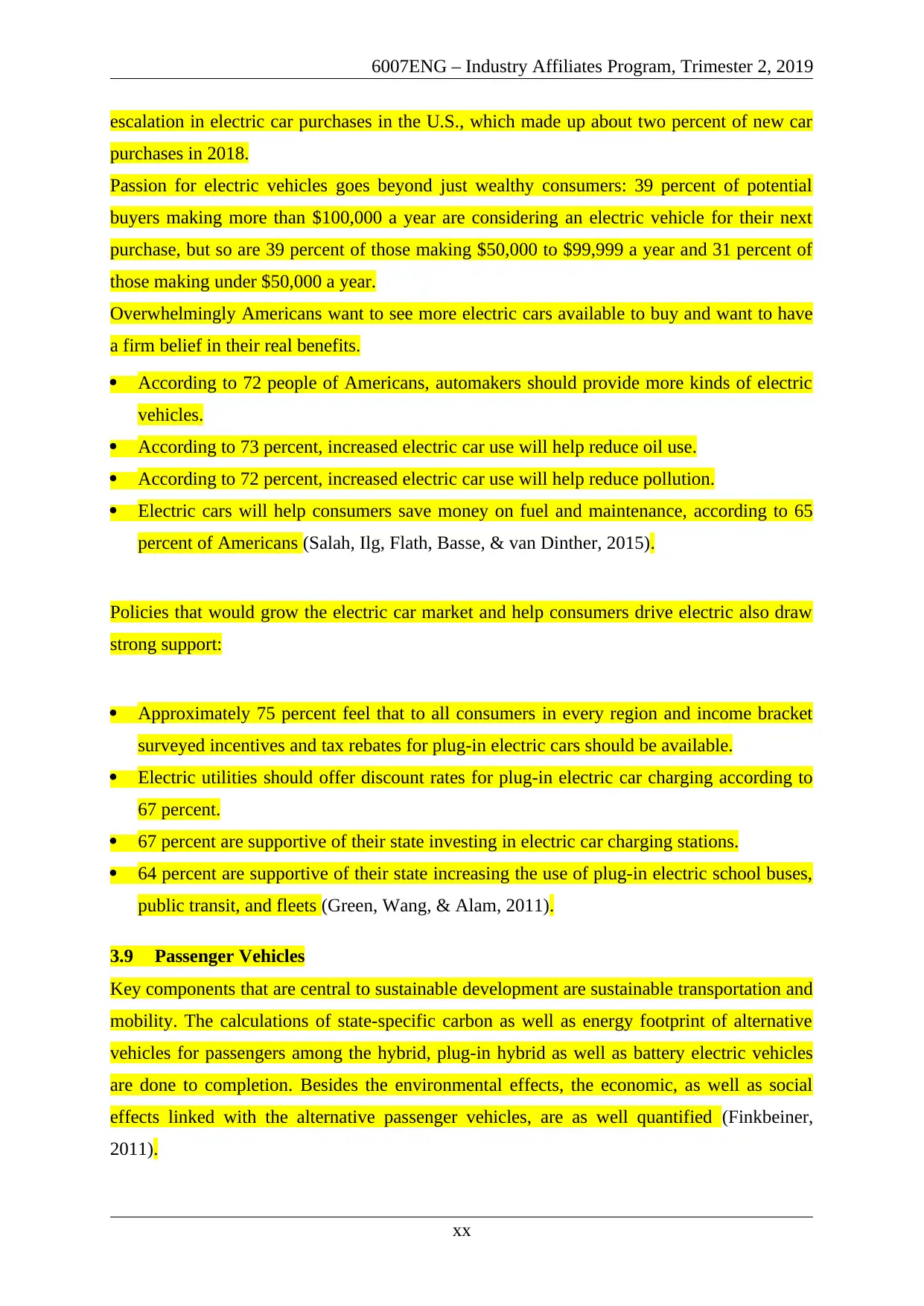
6007ENG – Industry Affiliates Program, Trimester 2, 2019
escalation in electric car purchases in the U.S., which made up about two percent of new car
purchases in 2018.
Passion for electric vehicles goes beyond just wealthy consumers: 39 percent of potential
buyers making more than $100,000 a year are considering an electric vehicle for their next
purchase, but so are 39 percent of those making $50,000 to $99,999 a year and 31 percent of
those making under $50,000 a year.
Overwhelmingly Americans want to see more electric cars available to buy and want to have
a firm belief in their real benefits.
According to 72 people of Americans, automakers should provide more kinds of electric
vehicles.
According to 73 percent, increased electric car use will help reduce oil use.
According to 72 percent, increased electric car use will help reduce pollution.
Electric cars will help consumers save money on fuel and maintenance, according to 65
percent of Americans (Salah, Ilg, Flath, Basse, & van Dinther, 2015).
Policies that would grow the electric car market and help consumers drive electric also draw
strong support:
Approximately 75 percent feel that to all consumers in every region and income bracket
surveyed incentives and tax rebates for plug-in electric cars should be available.
Electric utilities should offer discount rates for plug-in electric car charging according to
67 percent.
67 percent are supportive of their state investing in electric car charging stations.
64 percent are supportive of their state increasing the use of plug-in electric school buses,
public transit, and fleets (Green, Wang, & Alam, 2011).
3.9 Passenger Vehicles
Key components that are central to sustainable development are sustainable transportation and
mobility. The calculations of state-specific carbon as well as energy footprint of alternative
vehicles for passengers among the hybrid, plug-in hybrid as well as battery electric vehicles
are done to completion. Besides the environmental effects, the economic, as well as social
effects linked with the alternative passenger vehicles, are as well quantified (Finkbeiner,
2011).
xx
escalation in electric car purchases in the U.S., which made up about two percent of new car
purchases in 2018.
Passion for electric vehicles goes beyond just wealthy consumers: 39 percent of potential
buyers making more than $100,000 a year are considering an electric vehicle for their next
purchase, but so are 39 percent of those making $50,000 to $99,999 a year and 31 percent of
those making under $50,000 a year.
Overwhelmingly Americans want to see more electric cars available to buy and want to have
a firm belief in their real benefits.
According to 72 people of Americans, automakers should provide more kinds of electric
vehicles.
According to 73 percent, increased electric car use will help reduce oil use.
According to 72 percent, increased electric car use will help reduce pollution.
Electric cars will help consumers save money on fuel and maintenance, according to 65
percent of Americans (Salah, Ilg, Flath, Basse, & van Dinther, 2015).
Policies that would grow the electric car market and help consumers drive electric also draw
strong support:
Approximately 75 percent feel that to all consumers in every region and income bracket
surveyed incentives and tax rebates for plug-in electric cars should be available.
Electric utilities should offer discount rates for plug-in electric car charging according to
67 percent.
67 percent are supportive of their state investing in electric car charging stations.
64 percent are supportive of their state increasing the use of plug-in electric school buses,
public transit, and fleets (Green, Wang, & Alam, 2011).
3.9 Passenger Vehicles
Key components that are central to sustainable development are sustainable transportation and
mobility. The calculations of state-specific carbon as well as energy footprint of alternative
vehicles for passengers among the hybrid, plug-in hybrid as well as battery electric vehicles
are done to completion. Besides the environmental effects, the economic, as well as social
effects linked with the alternative passenger vehicles, are as well quantified (Finkbeiner,
2011).
xx
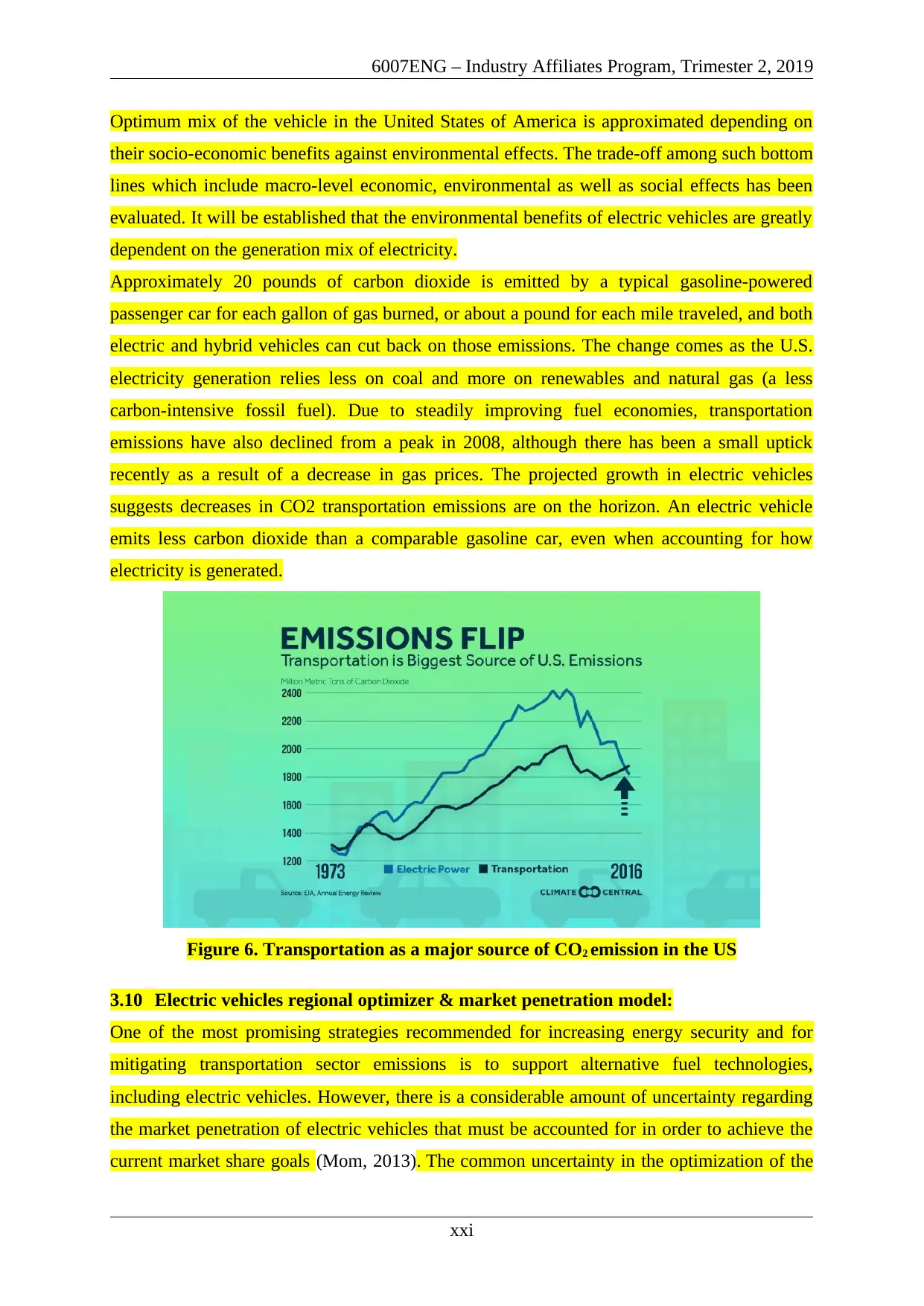
6007ENG – Industry Affiliates Program, Trimester 2, 2019
Optimum mix of the vehicle in the United States of America is approximated depending on
their socio-economic benefits against environmental effects. The trade-off among such bottom
lines which include macro-level economic, environmental as well as social effects has been
evaluated. It will be established that the environmental benefits of electric vehicles are greatly
dependent on the generation mix of electricity.
Approximately 20 pounds of carbon dioxide is emitted by a typical gasoline-powered
passenger car for each gallon of gas burned, or about a pound for each mile traveled, and both
electric and hybrid vehicles can cut back on those emissions. The change comes as the U.S.
electricity generation relies less on coal and more on renewables and natural gas (a less
carbon-intensive fossil fuel). Due to steadily improving fuel economies, transportation
emissions have also declined from a peak in 2008, although there has been a small uptick
recently as a result of a decrease in gas prices. The projected growth in electric vehicles
suggests decreases in CO2 transportation emissions are on the horizon. An electric vehicle
emits less carbon dioxide than a comparable gasoline car, even when accounting for how
electricity is generated.
Figure 6. Transportation as a major source of CO2 emission in the US
3.10 Electric vehicles regional optimizer & market penetration model:
One of the most promising strategies recommended for increasing energy security and for
mitigating transportation sector emissions is to support alternative fuel technologies,
including electric vehicles. However, there is a considerable amount of uncertainty regarding
the market penetration of electric vehicles that must be accounted for in order to achieve the
current market share goals (Mom, 2013). The common uncertainty in the optimization of the
xxi
Optimum mix of the vehicle in the United States of America is approximated depending on
their socio-economic benefits against environmental effects. The trade-off among such bottom
lines which include macro-level economic, environmental as well as social effects has been
evaluated. It will be established that the environmental benefits of electric vehicles are greatly
dependent on the generation mix of electricity.
Approximately 20 pounds of carbon dioxide is emitted by a typical gasoline-powered
passenger car for each gallon of gas burned, or about a pound for each mile traveled, and both
electric and hybrid vehicles can cut back on those emissions. The change comes as the U.S.
electricity generation relies less on coal and more on renewables and natural gas (a less
carbon-intensive fossil fuel). Due to steadily improving fuel economies, transportation
emissions have also declined from a peak in 2008, although there has been a small uptick
recently as a result of a decrease in gas prices. The projected growth in electric vehicles
suggests decreases in CO2 transportation emissions are on the horizon. An electric vehicle
emits less carbon dioxide than a comparable gasoline car, even when accounting for how
electricity is generated.
Figure 6. Transportation as a major source of CO2 emission in the US
3.10 Electric vehicles regional optimizer & market penetration model:
One of the most promising strategies recommended for increasing energy security and for
mitigating transportation sector emissions is to support alternative fuel technologies,
including electric vehicles. However, there is a considerable amount of uncertainty regarding
the market penetration of electric vehicles that must be accounted for in order to achieve the
current market share goals (Mom, 2013). The common uncertainty in the optimization of the
xxi
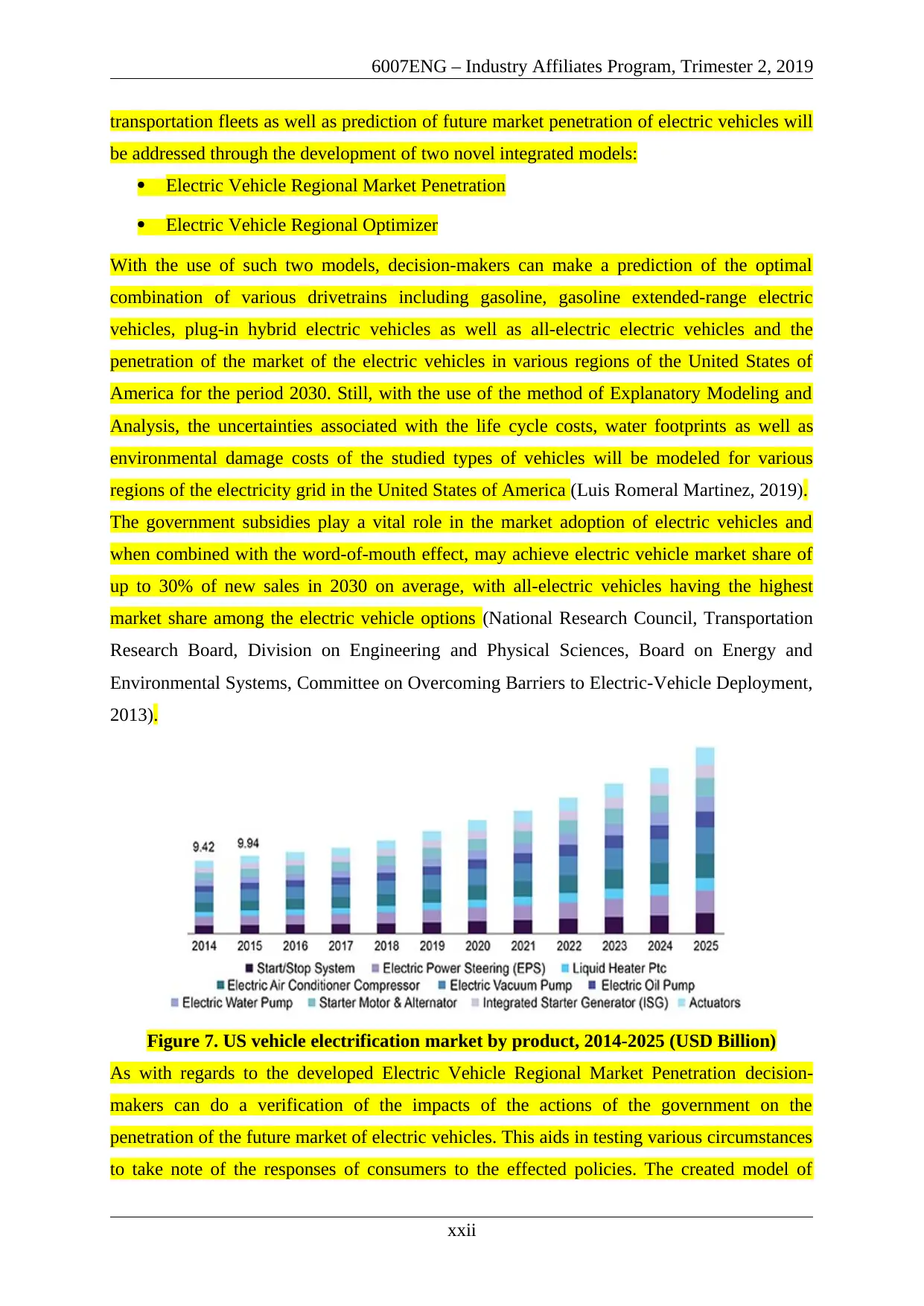
6007ENG – Industry Affiliates Program, Trimester 2, 2019
transportation fleets as well as prediction of future market penetration of electric vehicles will
be addressed through the development of two novel integrated models:
Electric Vehicle Regional Market Penetration
Electric Vehicle Regional Optimizer
With the use of such two models, decision-makers can make a prediction of the optimal
combination of various drivetrains including gasoline, gasoline extended-range electric
vehicles, plug-in hybrid electric vehicles as well as all-electric electric vehicles and the
penetration of the market of the electric vehicles in various regions of the United States of
America for the period 2030. Still, with the use of the method of Explanatory Modeling and
Analysis, the uncertainties associated with the life cycle costs, water footprints as well as
environmental damage costs of the studied types of vehicles will be modeled for various
regions of the electricity grid in the United States of America (Luis Romeral Martinez, 2019).
The government subsidies play a vital role in the market adoption of electric vehicles and
when combined with the word-of-mouth effect, may achieve electric vehicle market share of
up to 30% of new sales in 2030 on average, with all-electric vehicles having the highest
market share among the electric vehicle options (National Research Council, Transportation
Research Board, Division on Engineering and Physical Sciences, Board on Energy and
Environmental Systems, Committee on Overcoming Barriers to Electric-Vehicle Deployment,
2013).
Figure 7. US vehicle electrification market by product, 2014-2025 (USD Billion)
As with regards to the developed Electric Vehicle Regional Market Penetration decision-
makers can do a verification of the impacts of the actions of the government on the
penetration of the future market of electric vehicles. This aids in testing various circumstances
to take note of the responses of consumers to the effected policies. The created model of
xxii
transportation fleets as well as prediction of future market penetration of electric vehicles will
be addressed through the development of two novel integrated models:
Electric Vehicle Regional Market Penetration
Electric Vehicle Regional Optimizer
With the use of such two models, decision-makers can make a prediction of the optimal
combination of various drivetrains including gasoline, gasoline extended-range electric
vehicles, plug-in hybrid electric vehicles as well as all-electric electric vehicles and the
penetration of the market of the electric vehicles in various regions of the United States of
America for the period 2030. Still, with the use of the method of Explanatory Modeling and
Analysis, the uncertainties associated with the life cycle costs, water footprints as well as
environmental damage costs of the studied types of vehicles will be modeled for various
regions of the electricity grid in the United States of America (Luis Romeral Martinez, 2019).
The government subsidies play a vital role in the market adoption of electric vehicles and
when combined with the word-of-mouth effect, may achieve electric vehicle market share of
up to 30% of new sales in 2030 on average, with all-electric vehicles having the highest
market share among the electric vehicle options (National Research Council, Transportation
Research Board, Division on Engineering and Physical Sciences, Board on Energy and
Environmental Systems, Committee on Overcoming Barriers to Electric-Vehicle Deployment,
2013).
Figure 7. US vehicle electrification market by product, 2014-2025 (USD Billion)
As with regards to the developed Electric Vehicle Regional Market Penetration decision-
makers can do a verification of the impacts of the actions of the government on the
penetration of the future market of electric vehicles. This aids in testing various circumstances
to take note of the responses of consumers to the effected policies. The created model of
xxii
Paraphrase This Document
Need a fresh take? Get an instant paraphrase of this document with our AI Paraphraser
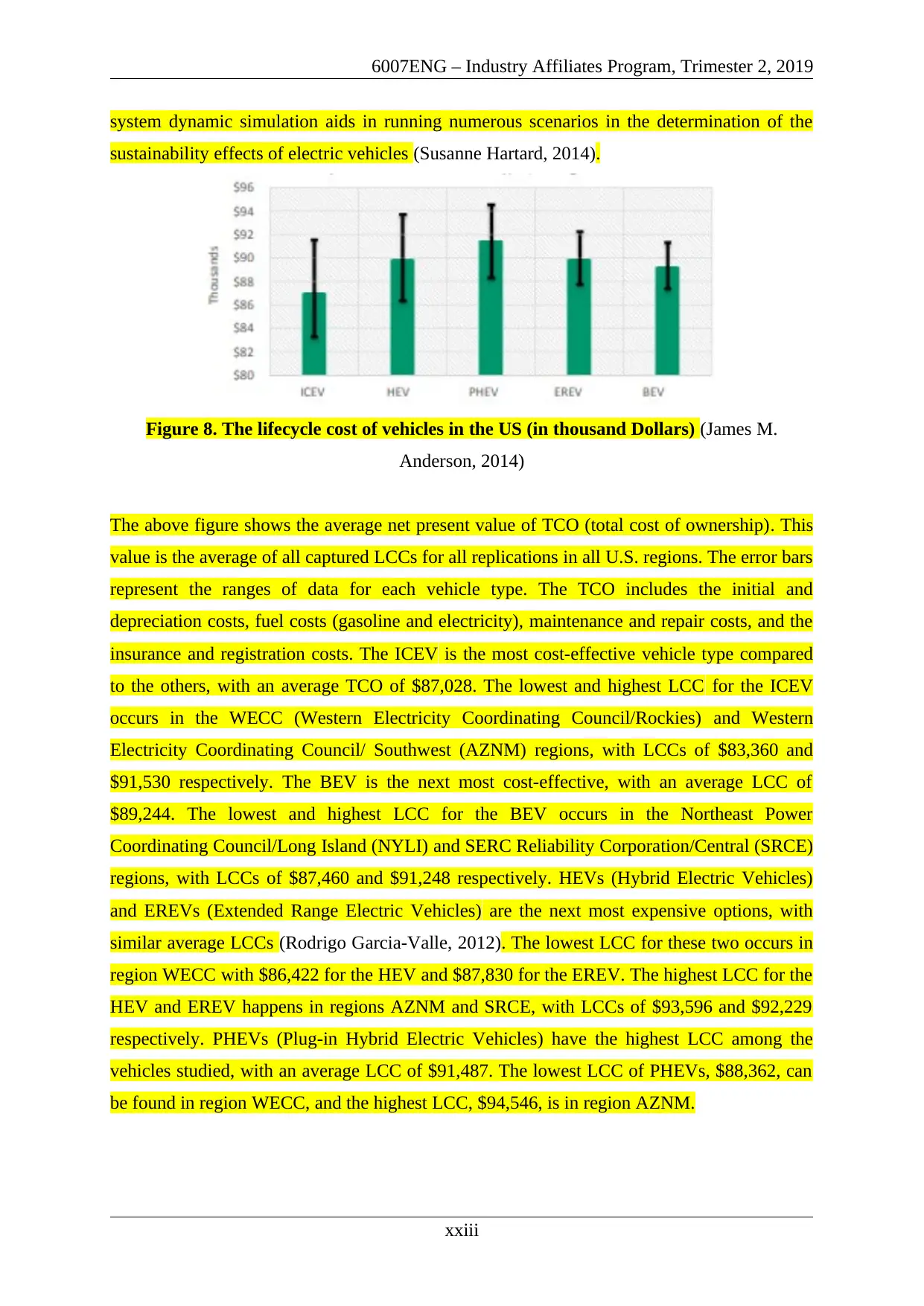
6007ENG – Industry Affiliates Program, Trimester 2, 2019
system dynamic simulation aids in running numerous scenarios in the determination of the
sustainability effects of electric vehicles (Susanne Hartard, 2014).
Figure 8. The lifecycle cost of vehicles in the US (in thousand Dollars) (James M.
Anderson, 2014)
The above figure shows the average net present value of TCO (total cost of ownership). This
value is the average of all captured LCCs for all replications in all U.S. regions. The error bars
represent the ranges of data for each vehicle type. The TCO includes the initial and
depreciation costs, fuel costs (gasoline and electricity), maintenance and repair costs, and the
insurance and registration costs. The ICEV is the most cost-effective vehicle type compared
to the others, with an average TCO of $87,028. The lowest and highest LCC for the ICEV
occurs in the WECC (Western Electricity Coordinating Council/Rockies) and Western
Electricity Coordinating Council/ Southwest (AZNM) regions, with LCCs of $83,360 and
$91,530 respectively. The BEV is the next most cost-effective, with an average LCC of
$89,244. The lowest and highest LCC for the BEV occurs in the Northeast Power
Coordinating Council/Long Island (NYLI) and SERC Reliability Corporation/Central (SRCE)
regions, with LCCs of $87,460 and $91,248 respectively. HEVs (Hybrid Electric Vehicles)
and EREVs (Extended Range Electric Vehicles) are the next most expensive options, with
similar average LCCs (Rodrigo Garcia-Valle, 2012). The lowest LCC for these two occurs in
region WECC with $86,422 for the HEV and $87,830 for the EREV. The highest LCC for the
HEV and EREV happens in regions AZNM and SRCE, with LCCs of $93,596 and $92,229
respectively. PHEVs (Plug-in Hybrid Electric Vehicles) have the highest LCC among the
vehicles studied, with an average LCC of $91,487. The lowest LCC of PHEVs, $88,362, can
be found in region WECC, and the highest LCC, $94,546, is in region AZNM.
xxiii
system dynamic simulation aids in running numerous scenarios in the determination of the
sustainability effects of electric vehicles (Susanne Hartard, 2014).
Figure 8. The lifecycle cost of vehicles in the US (in thousand Dollars) (James M.
Anderson, 2014)
The above figure shows the average net present value of TCO (total cost of ownership). This
value is the average of all captured LCCs for all replications in all U.S. regions. The error bars
represent the ranges of data for each vehicle type. The TCO includes the initial and
depreciation costs, fuel costs (gasoline and electricity), maintenance and repair costs, and the
insurance and registration costs. The ICEV is the most cost-effective vehicle type compared
to the others, with an average TCO of $87,028. The lowest and highest LCC for the ICEV
occurs in the WECC (Western Electricity Coordinating Council/Rockies) and Western
Electricity Coordinating Council/ Southwest (AZNM) regions, with LCCs of $83,360 and
$91,530 respectively. The BEV is the next most cost-effective, with an average LCC of
$89,244. The lowest and highest LCC for the BEV occurs in the Northeast Power
Coordinating Council/Long Island (NYLI) and SERC Reliability Corporation/Central (SRCE)
regions, with LCCs of $87,460 and $91,248 respectively. HEVs (Hybrid Electric Vehicles)
and EREVs (Extended Range Electric Vehicles) are the next most expensive options, with
similar average LCCs (Rodrigo Garcia-Valle, 2012). The lowest LCC for these two occurs in
region WECC with $86,422 for the HEV and $87,830 for the EREV. The highest LCC for the
HEV and EREV happens in regions AZNM and SRCE, with LCCs of $93,596 and $92,229
respectively. PHEVs (Plug-in Hybrid Electric Vehicles) have the highest LCC among the
vehicles studied, with an average LCC of $91,487. The lowest LCC of PHEVs, $88,362, can
be found in region WECC, and the highest LCC, $94,546, is in region AZNM.
xxiii
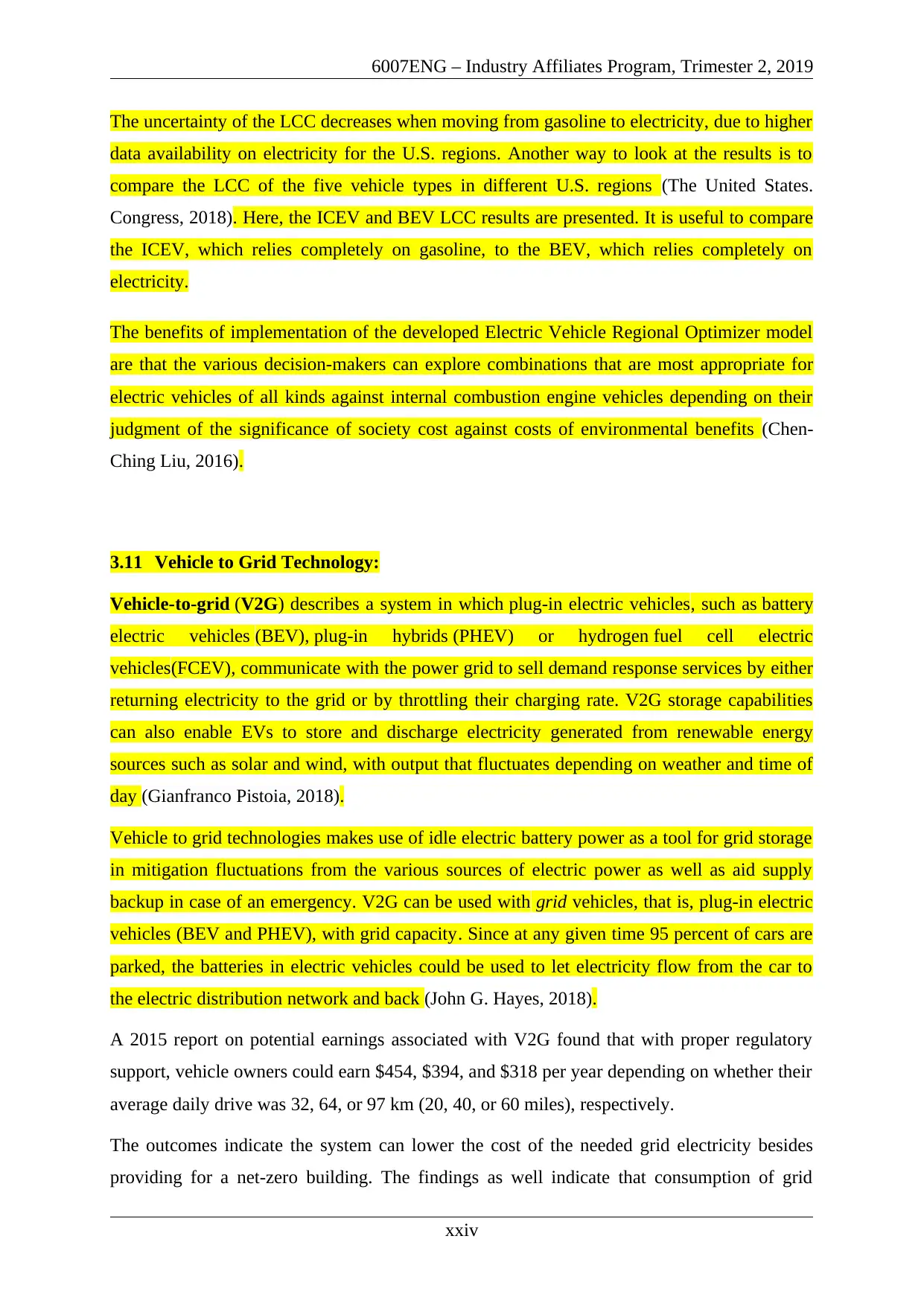
6007ENG – Industry Affiliates Program, Trimester 2, 2019
The uncertainty of the LCC decreases when moving from gasoline to electricity, due to higher
data availability on electricity for the U.S. regions. Another way to look at the results is to
compare the LCC of the five vehicle types in different U.S. regions (The United States.
Congress, 2018). Here, the ICEV and BEV LCC results are presented. It is useful to compare
the ICEV, which relies completely on gasoline, to the BEV, which relies completely on
electricity.
The benefits of implementation of the developed Electric Vehicle Regional Optimizer model
are that the various decision-makers can explore combinations that are most appropriate for
electric vehicles of all kinds against internal combustion engine vehicles depending on their
judgment of the significance of society cost against costs of environmental benefits (Chen-
Ching Liu, 2016).
3.11 Vehicle to Grid Technology:
Vehicle-to-grid (V2G) describes a system in which plug-in electric vehicles, such as battery
electric vehicles (BEV), plug-in hybrids (PHEV) or hydrogen fuel cell electric
vehicles(FCEV), communicate with the power grid to sell demand response services by either
returning electricity to the grid or by throttling their charging rate. V2G storage capabilities
can also enable EVs to store and discharge electricity generated from renewable energy
sources such as solar and wind, with output that fluctuates depending on weather and time of
day (Gianfranco Pistoia, 2018).
Vehicle to grid technologies makes use of idle electric battery power as a tool for grid storage
in mitigation fluctuations from the various sources of electric power as well as aid supply
backup in case of an emergency. V2G can be used with grid vehicles, that is, plug-in electric
vehicles (BEV and PHEV), with grid capacity. Since at any given time 95 percent of cars are
parked, the batteries in electric vehicles could be used to let electricity flow from the car to
the electric distribution network and back (John G. Hayes, 2018).
A 2015 report on potential earnings associated with V2G found that with proper regulatory
support, vehicle owners could earn $454, $394, and $318 per year depending on whether their
average daily drive was 32, 64, or 97 km (20, 40, or 60 miles), respectively.
The outcomes indicate the system can lower the cost of the needed grid electricity besides
providing for a net-zero building. The findings as well indicate that consumption of grid
xxiv
The uncertainty of the LCC decreases when moving from gasoline to electricity, due to higher
data availability on electricity for the U.S. regions. Another way to look at the results is to
compare the LCC of the five vehicle types in different U.S. regions (The United States.
Congress, 2018). Here, the ICEV and BEV LCC results are presented. It is useful to compare
the ICEV, which relies completely on gasoline, to the BEV, which relies completely on
electricity.
The benefits of implementation of the developed Electric Vehicle Regional Optimizer model
are that the various decision-makers can explore combinations that are most appropriate for
electric vehicles of all kinds against internal combustion engine vehicles depending on their
judgment of the significance of society cost against costs of environmental benefits (Chen-
Ching Liu, 2016).
3.11 Vehicle to Grid Technology:
Vehicle-to-grid (V2G) describes a system in which plug-in electric vehicles, such as battery
electric vehicles (BEV), plug-in hybrids (PHEV) or hydrogen fuel cell electric
vehicles(FCEV), communicate with the power grid to sell demand response services by either
returning electricity to the grid or by throttling their charging rate. V2G storage capabilities
can also enable EVs to store and discharge electricity generated from renewable energy
sources such as solar and wind, with output that fluctuates depending on weather and time of
day (Gianfranco Pistoia, 2018).
Vehicle to grid technologies makes use of idle electric battery power as a tool for grid storage
in mitigation fluctuations from the various sources of electric power as well as aid supply
backup in case of an emergency. V2G can be used with grid vehicles, that is, plug-in electric
vehicles (BEV and PHEV), with grid capacity. Since at any given time 95 percent of cars are
parked, the batteries in electric vehicles could be used to let electricity flow from the car to
the electric distribution network and back (John G. Hayes, 2018).
A 2015 report on potential earnings associated with V2G found that with proper regulatory
support, vehicle owners could earn $454, $394, and $318 per year depending on whether their
average daily drive was 32, 64, or 97 km (20, 40, or 60 miles), respectively.
The outcomes indicate the system can lower the cost of the needed grid electricity besides
providing for a net-zero building. The findings as well indicate that consumption of grid
xxiv
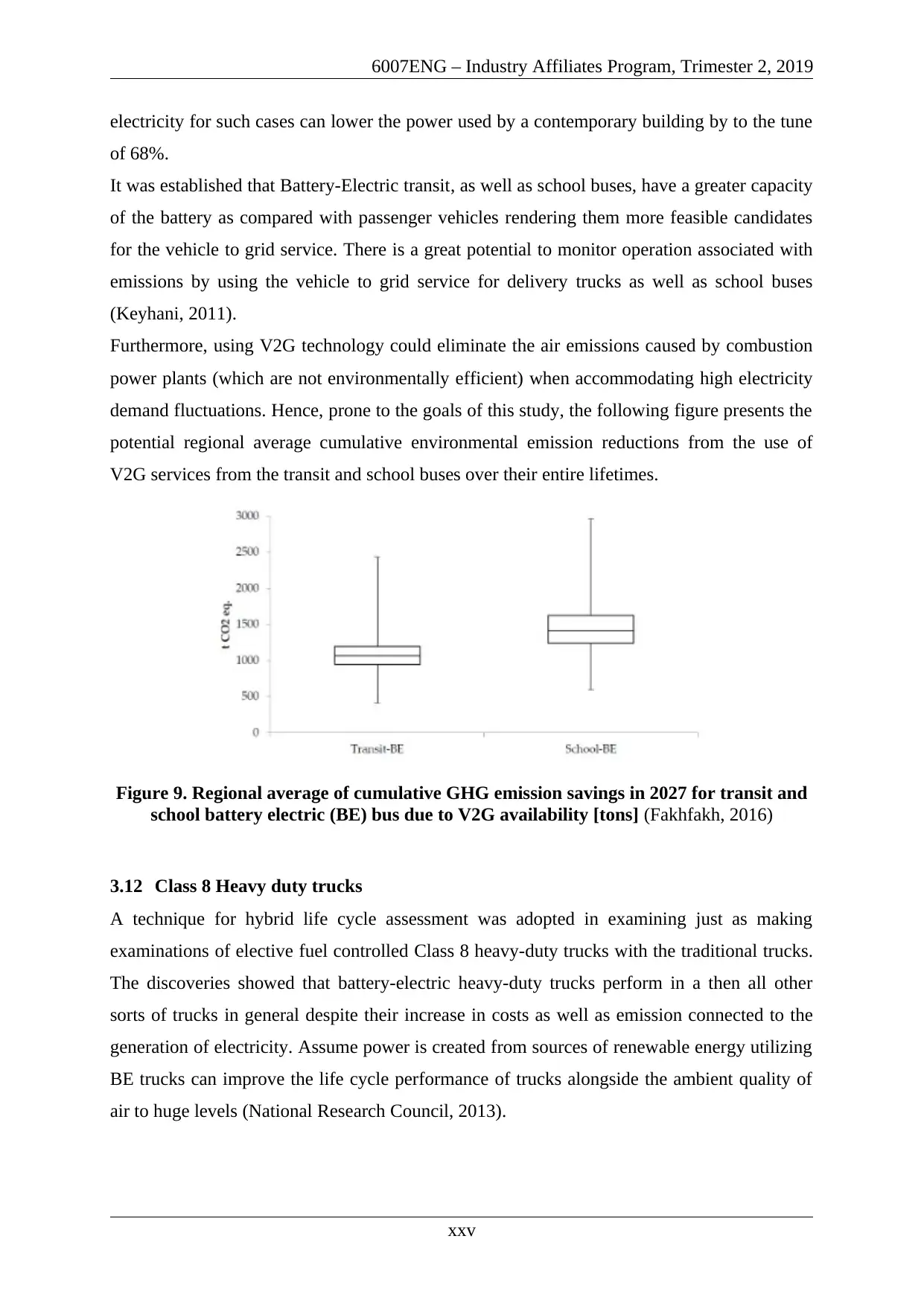
6007ENG – Industry Affiliates Program, Trimester 2, 2019
electricity for such cases can lower the power used by a contemporary building by to the tune
of 68%.
It was established that Battery-Electric transit, as well as school buses, have a greater capacity
of the battery as compared with passenger vehicles rendering them more feasible candidates
for the vehicle to grid service. There is a great potential to monitor operation associated with
emissions by using the vehicle to grid service for delivery trucks as well as school buses
(Keyhani, 2011).
Furthermore, using V2G technology could eliminate the air emissions caused by combustion
power plants (which are not environmentally efficient) when accommodating high electricity
demand fluctuations. Hence, prone to the goals of this study, the following figure presents the
potential regional average cumulative environmental emission reductions from the use of
V2G services from the transit and school buses over their entire lifetimes.
Figure 9. Regional average of cumulative GHG emission savings in 2027 for transit and
school battery electric (BE) bus due to V2G availability [tons] (Fakhfakh, 2016)
3.12 Class 8 Heavy duty trucks
A technique for hybrid life cycle assessment was adopted in examining just as making
examinations of elective fuel controlled Class 8 heavy-duty trucks with the traditional trucks.
The discoveries showed that battery-electric heavy-duty trucks perform in a then all other
sorts of trucks in general despite their increase in costs as well as emission connected to the
generation of electricity. Assume power is created from sources of renewable energy utilizing
BE trucks can improve the life cycle performance of trucks alongside the ambient quality of
air to huge levels (National Research Council, 2013).
xxv
electricity for such cases can lower the power used by a contemporary building by to the tune
of 68%.
It was established that Battery-Electric transit, as well as school buses, have a greater capacity
of the battery as compared with passenger vehicles rendering them more feasible candidates
for the vehicle to grid service. There is a great potential to monitor operation associated with
emissions by using the vehicle to grid service for delivery trucks as well as school buses
(Keyhani, 2011).
Furthermore, using V2G technology could eliminate the air emissions caused by combustion
power plants (which are not environmentally efficient) when accommodating high electricity
demand fluctuations. Hence, prone to the goals of this study, the following figure presents the
potential regional average cumulative environmental emission reductions from the use of
V2G services from the transit and school buses over their entire lifetimes.
Figure 9. Regional average of cumulative GHG emission savings in 2027 for transit and
school battery electric (BE) bus due to V2G availability [tons] (Fakhfakh, 2016)
3.12 Class 8 Heavy duty trucks
A technique for hybrid life cycle assessment was adopted in examining just as making
examinations of elective fuel controlled Class 8 heavy-duty trucks with the traditional trucks.
The discoveries showed that battery-electric heavy-duty trucks perform in a then all other
sorts of trucks in general despite their increase in costs as well as emission connected to the
generation of electricity. Assume power is created from sources of renewable energy utilizing
BE trucks can improve the life cycle performance of trucks alongside the ambient quality of
air to huge levels (National Research Council, 2013).
xxv
Secure Best Marks with AI Grader
Need help grading? Try our AI Grader for instant feedback on your assignments.
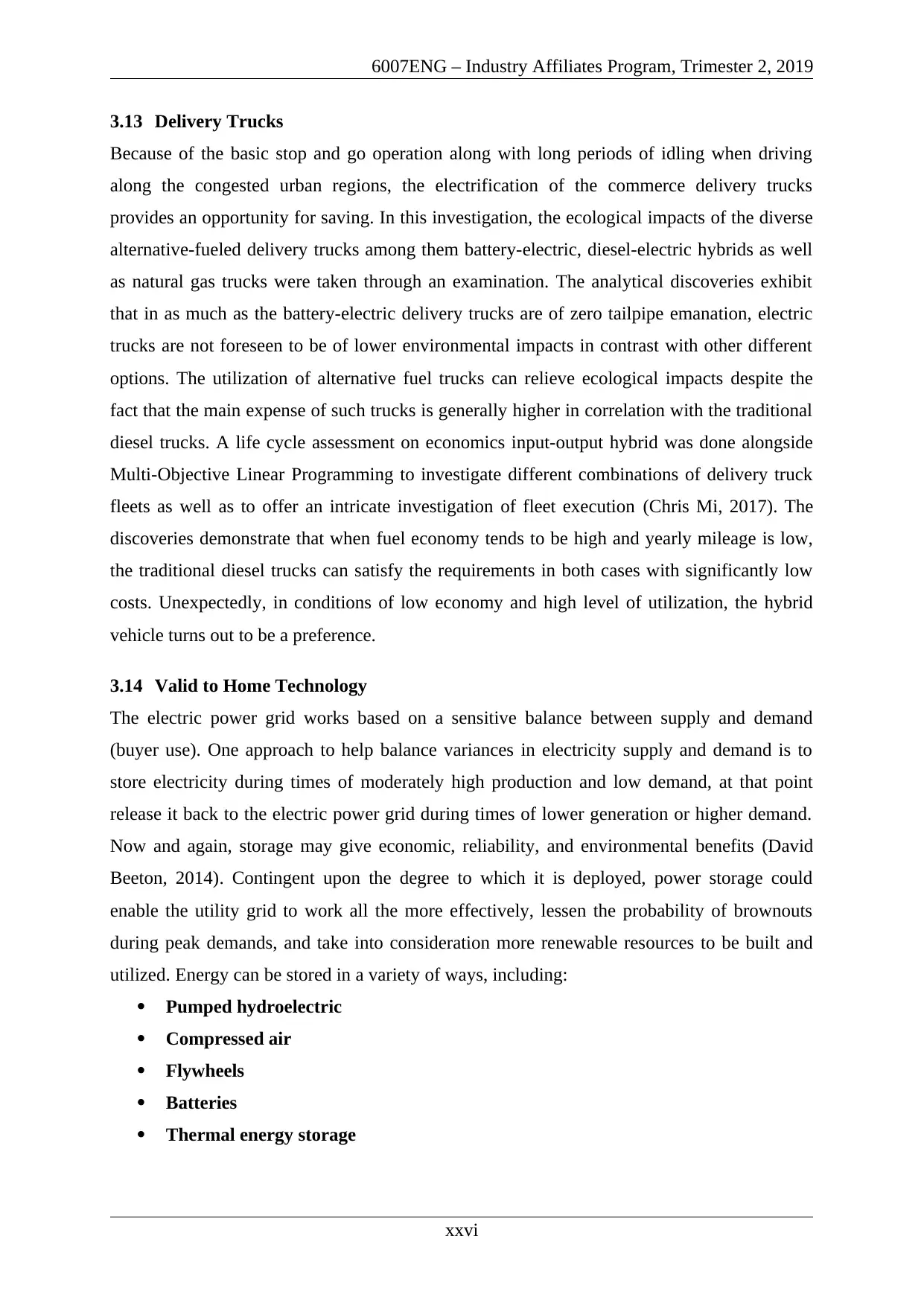
6007ENG – Industry Affiliates Program, Trimester 2, 2019
3.13 Delivery Trucks
Because of the basic stop and go operation along with long periods of idling when driving
along the congested urban regions, the electrification of the commerce delivery trucks
provides an opportunity for saving. In this investigation, the ecological impacts of the diverse
alternative-fueled delivery trucks among them battery-electric, diesel-electric hybrids as well
as natural gas trucks were taken through an examination. The analytical discoveries exhibit
that in as much as the battery-electric delivery trucks are of zero tailpipe emanation, electric
trucks are not foreseen to be of lower environmental impacts in contrast with other different
options. The utilization of alternative fuel trucks can relieve ecological impacts despite the
fact that the main expense of such trucks is generally higher in correlation with the traditional
diesel trucks. A life cycle assessment on economics input-output hybrid was done alongside
Multi-Objective Linear Programming to investigate different combinations of delivery truck
fleets as well as to offer an intricate investigation of fleet execution (Chris Mi, 2017). The
discoveries demonstrate that when fuel economy tends to be high and yearly mileage is low,
the traditional diesel trucks can satisfy the requirements in both cases with significantly low
costs. Unexpectedly, in conditions of low economy and high level of utilization, the hybrid
vehicle turns out to be a preference.
3.14 Valid to Home Technology
The electric power grid works based on a sensitive balance between supply and demand
(buyer use). One approach to help balance variances in electricity supply and demand is to
store electricity during times of moderately high production and low demand, at that point
release it back to the electric power grid during times of lower generation or higher demand.
Now and again, storage may give economic, reliability, and environmental benefits (David
Beeton, 2014). Contingent upon the degree to which it is deployed, power storage could
enable the utility grid to work all the more effectively, lessen the probability of brownouts
during peak demands, and take into consideration more renewable resources to be built and
utilized. Energy can be stored in a variety of ways, including:
Pumped hydroelectric
Compressed air
Flywheels
Batteries
Thermal energy storage
xxvi
3.13 Delivery Trucks
Because of the basic stop and go operation along with long periods of idling when driving
along the congested urban regions, the electrification of the commerce delivery trucks
provides an opportunity for saving. In this investigation, the ecological impacts of the diverse
alternative-fueled delivery trucks among them battery-electric, diesel-electric hybrids as well
as natural gas trucks were taken through an examination. The analytical discoveries exhibit
that in as much as the battery-electric delivery trucks are of zero tailpipe emanation, electric
trucks are not foreseen to be of lower environmental impacts in contrast with other different
options. The utilization of alternative fuel trucks can relieve ecological impacts despite the
fact that the main expense of such trucks is generally higher in correlation with the traditional
diesel trucks. A life cycle assessment on economics input-output hybrid was done alongside
Multi-Objective Linear Programming to investigate different combinations of delivery truck
fleets as well as to offer an intricate investigation of fleet execution (Chris Mi, 2017). The
discoveries demonstrate that when fuel economy tends to be high and yearly mileage is low,
the traditional diesel trucks can satisfy the requirements in both cases with significantly low
costs. Unexpectedly, in conditions of low economy and high level of utilization, the hybrid
vehicle turns out to be a preference.
3.14 Valid to Home Technology
The electric power grid works based on a sensitive balance between supply and demand
(buyer use). One approach to help balance variances in electricity supply and demand is to
store electricity during times of moderately high production and low demand, at that point
release it back to the electric power grid during times of lower generation or higher demand.
Now and again, storage may give economic, reliability, and environmental benefits (David
Beeton, 2014). Contingent upon the degree to which it is deployed, power storage could
enable the utility grid to work all the more effectively, lessen the probability of brownouts
during peak demands, and take into consideration more renewable resources to be built and
utilized. Energy can be stored in a variety of ways, including:
Pumped hydroelectric
Compressed air
Flywheels
Batteries
Thermal energy storage
xxvi
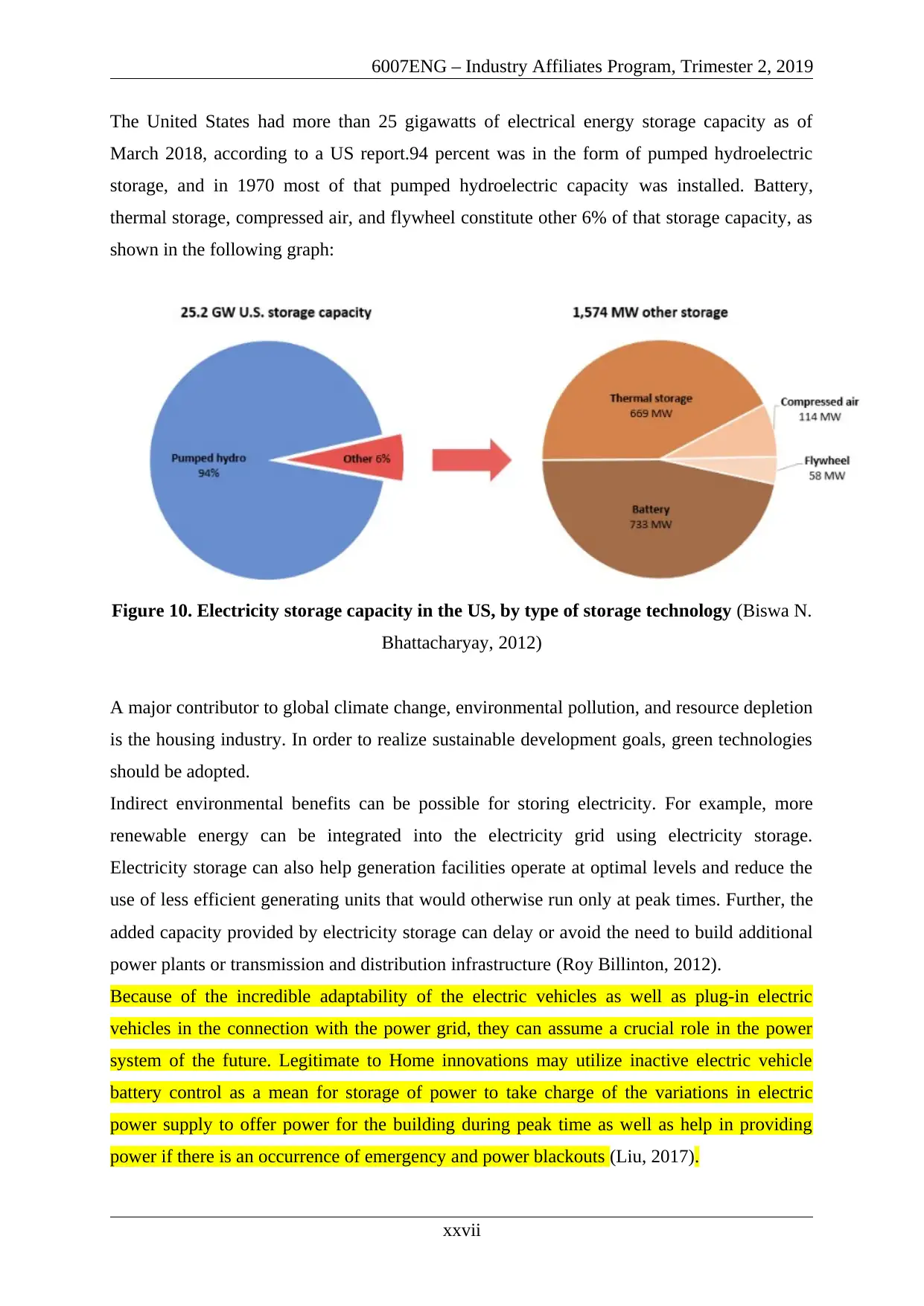
6007ENG – Industry Affiliates Program, Trimester 2, 2019
The United States had more than 25 gigawatts of electrical energy storage capacity as of
March 2018, according to a US report.94 percent was in the form of pumped hydroelectric
storage, and in 1970 most of that pumped hydroelectric capacity was installed. Battery,
thermal storage, compressed air, and flywheel constitute other 6% of that storage capacity, as
shown in the following graph:
Figure 10. Electricity storage capacity in the US, by type of storage technology (Biswa N.
Bhattacharyay, 2012)
A major contributor to global climate change, environmental pollution, and resource depletion
is the housing industry. In order to realize sustainable development goals, green technologies
should be adopted.
Indirect environmental benefits can be possible for storing electricity. For example, more
renewable energy can be integrated into the electricity grid using electricity storage.
Electricity storage can also help generation facilities operate at optimal levels and reduce the
use of less efficient generating units that would otherwise run only at peak times. Further, the
added capacity provided by electricity storage can delay or avoid the need to build additional
power plants or transmission and distribution infrastructure (Roy Billinton, 2012).
Because of the incredible adaptability of the electric vehicles as well as plug-in electric
vehicles in the connection with the power grid, they can assume a crucial role in the power
system of the future. Legitimate to Home innovations may utilize inactive electric vehicle
battery control as a mean for storage of power to take charge of the variations in electric
power supply to offer power for the building during peak time as well as help in providing
power if there is an occurrence of emergency and power blackouts (Liu, 2017).
xxvii
The United States had more than 25 gigawatts of electrical energy storage capacity as of
March 2018, according to a US report.94 percent was in the form of pumped hydroelectric
storage, and in 1970 most of that pumped hydroelectric capacity was installed. Battery,
thermal storage, compressed air, and flywheel constitute other 6% of that storage capacity, as
shown in the following graph:
Figure 10. Electricity storage capacity in the US, by type of storage technology (Biswa N.
Bhattacharyay, 2012)
A major contributor to global climate change, environmental pollution, and resource depletion
is the housing industry. In order to realize sustainable development goals, green technologies
should be adopted.
Indirect environmental benefits can be possible for storing electricity. For example, more
renewable energy can be integrated into the electricity grid using electricity storage.
Electricity storage can also help generation facilities operate at optimal levels and reduce the
use of less efficient generating units that would otherwise run only at peak times. Further, the
added capacity provided by electricity storage can delay or avoid the need to build additional
power plants or transmission and distribution infrastructure (Roy Billinton, 2012).
Because of the incredible adaptability of the electric vehicles as well as plug-in electric
vehicles in the connection with the power grid, they can assume a crucial role in the power
system of the future. Legitimate to Home innovations may utilize inactive electric vehicle
battery control as a mean for storage of power to take charge of the variations in electric
power supply to offer power for the building during peak time as well as help in providing
power if there is an occurrence of emergency and power blackouts (Liu, 2017).
xxvii
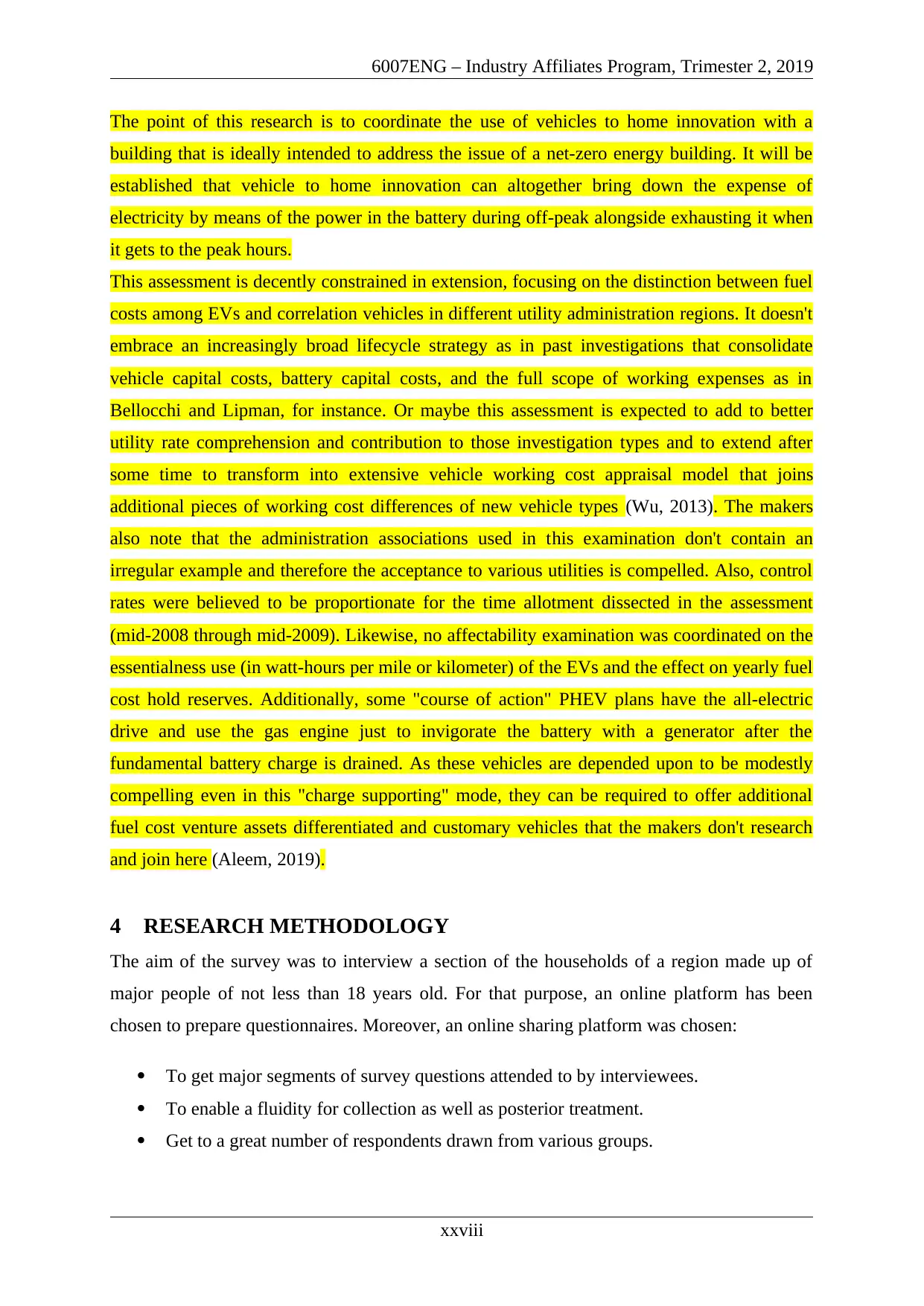
6007ENG – Industry Affiliates Program, Trimester 2, 2019
The point of this research is to coordinate the use of vehicles to home innovation with a
building that is ideally intended to address the issue of a net-zero energy building. It will be
established that vehicle to home innovation can altogether bring down the expense of
electricity by means of the power in the battery during off-peak alongside exhausting it when
it gets to the peak hours.
This assessment is decently constrained in extension, focusing on the distinction between fuel
costs among EVs and correlation vehicles in different utility administration regions. It doesn't
embrace an increasingly broad lifecycle strategy as in past investigations that consolidate
vehicle capital costs, battery capital costs, and the full scope of working expenses as in
Bellocchi and Lipman, for instance. Or maybe this assessment is expected to add to better
utility rate comprehension and contribution to those investigation types and to extend after
some time to transform into extensive vehicle working cost appraisal model that joins
additional pieces of working cost differences of new vehicle types (Wu, 2013). The makers
also note that the administration associations used in this examination don't contain an
irregular example and therefore the acceptance to various utilities is compelled. Also, control
rates were believed to be proportionate for the time allotment dissected in the assessment
(mid-2008 through mid-2009). Likewise, no affectability examination was coordinated on the
essentialness use (in watt-hours per mile or kilometer) of the EVs and the effect on yearly fuel
cost hold reserves. Additionally, some "course of action" PHEV plans have the all-electric
drive and use the gas engine just to invigorate the battery with a generator after the
fundamental battery charge is drained. As these vehicles are depended upon to be modestly
compelling even in this "charge supporting" mode, they can be required to offer additional
fuel cost venture assets differentiated and customary vehicles that the makers don't research
and join here (Aleem, 2019).
4 RESEARCH METHODOLOGY
The aim of the survey was to interview a section of the households of a region made up of
major people of not less than 18 years old. For that purpose, an online platform has been
chosen to prepare questionnaires. Moreover, an online sharing platform was chosen:
To get major segments of survey questions attended to by interviewees.
To enable a fluidity for collection as well as posterior treatment.
Get to a great number of respondents drawn from various groups.
xxviii
The point of this research is to coordinate the use of vehicles to home innovation with a
building that is ideally intended to address the issue of a net-zero energy building. It will be
established that vehicle to home innovation can altogether bring down the expense of
electricity by means of the power in the battery during off-peak alongside exhausting it when
it gets to the peak hours.
This assessment is decently constrained in extension, focusing on the distinction between fuel
costs among EVs and correlation vehicles in different utility administration regions. It doesn't
embrace an increasingly broad lifecycle strategy as in past investigations that consolidate
vehicle capital costs, battery capital costs, and the full scope of working expenses as in
Bellocchi and Lipman, for instance. Or maybe this assessment is expected to add to better
utility rate comprehension and contribution to those investigation types and to extend after
some time to transform into extensive vehicle working cost appraisal model that joins
additional pieces of working cost differences of new vehicle types (Wu, 2013). The makers
also note that the administration associations used in this examination don't contain an
irregular example and therefore the acceptance to various utilities is compelled. Also, control
rates were believed to be proportionate for the time allotment dissected in the assessment
(mid-2008 through mid-2009). Likewise, no affectability examination was coordinated on the
essentialness use (in watt-hours per mile or kilometer) of the EVs and the effect on yearly fuel
cost hold reserves. Additionally, some "course of action" PHEV plans have the all-electric
drive and use the gas engine just to invigorate the battery with a generator after the
fundamental battery charge is drained. As these vehicles are depended upon to be modestly
compelling even in this "charge supporting" mode, they can be required to offer additional
fuel cost venture assets differentiated and customary vehicles that the makers don't research
and join here (Aleem, 2019).
4 RESEARCH METHODOLOGY
The aim of the survey was to interview a section of the households of a region made up of
major people of not less than 18 years old. For that purpose, an online platform has been
chosen to prepare questionnaires. Moreover, an online sharing platform was chosen:
To get major segments of survey questions attended to by interviewees.
To enable a fluidity for collection as well as posterior treatment.
Get to a great number of respondents drawn from various groups.
xxviii
Paraphrase This Document
Need a fresh take? Get an instant paraphrase of this document with our AI Paraphraser
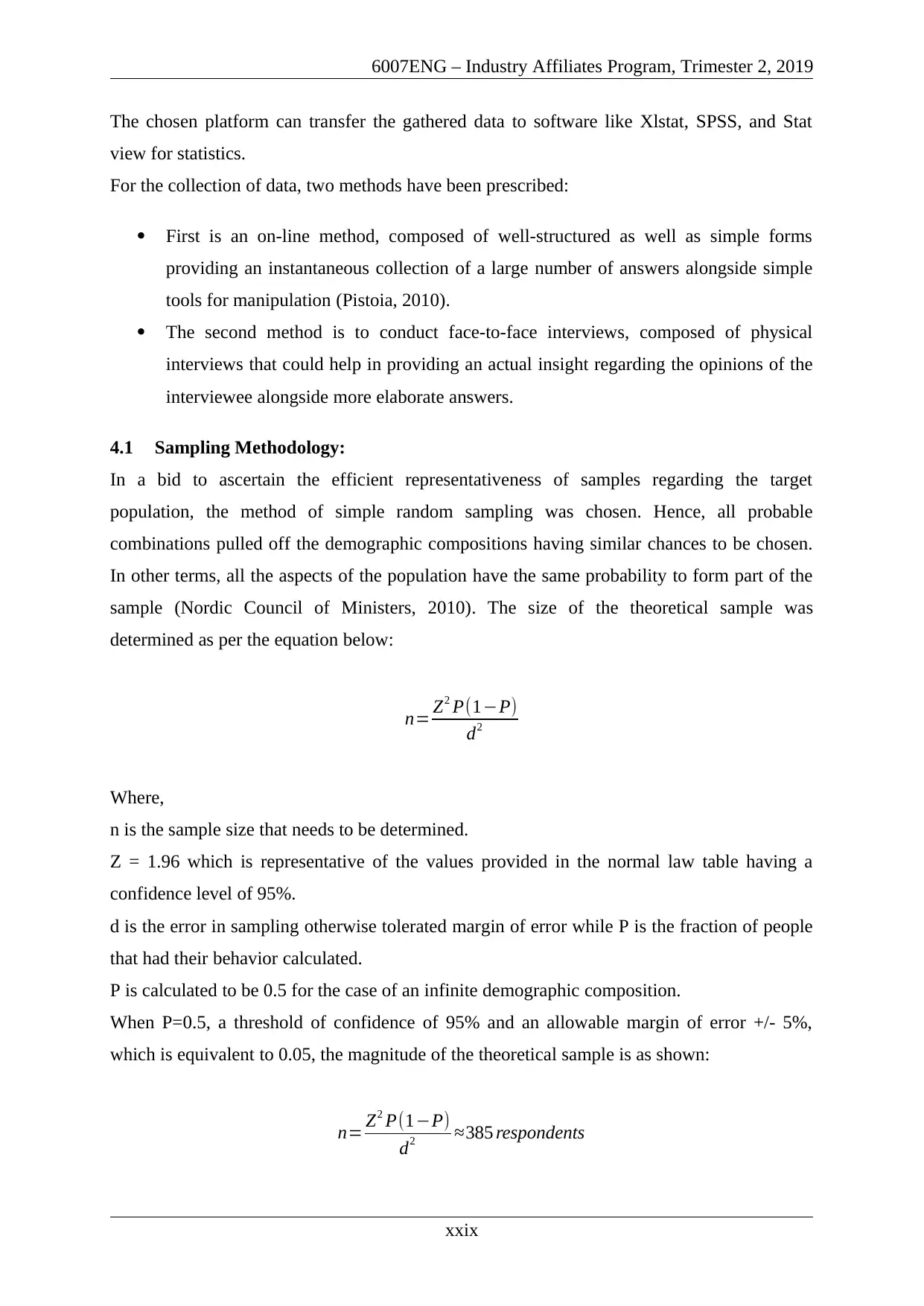
6007ENG – Industry Affiliates Program, Trimester 2, 2019
The chosen platform can transfer the gathered data to software like Xlstat, SPSS, and Stat
view for statistics.
For the collection of data, two methods have been prescribed:
First is an on-line method, composed of well-structured as well as simple forms
providing an instantaneous collection of a large number of answers alongside simple
tools for manipulation (Pistoia, 2010).
The second method is to conduct face-to-face interviews, composed of physical
interviews that could help in providing an actual insight regarding the opinions of the
interviewee alongside more elaborate answers.
4.1 Sampling Methodology:
In a bid to ascertain the efficient representativeness of samples regarding the target
population, the method of simple random sampling was chosen. Hence, all probable
combinations pulled off the demographic compositions having similar chances to be chosen.
In other terms, all the aspects of the population have the same probability to form part of the
sample (Nordic Council of Ministers, 2010). The size of the theoretical sample was
determined as per the equation below:
n= Z2 P(1−P)
d2
Where,
n is the sample size that needs to be determined.
Z = 1.96 which is representative of the values provided in the normal law table having a
confidence level of 95%.
d is the error in sampling otherwise tolerated margin of error while P is the fraction of people
that had their behavior calculated.
P is calculated to be 0.5 for the case of an infinite demographic composition.
When P=0.5, a threshold of confidence of 95% and an allowable margin of error +/- 5%,
which is equivalent to 0.05, the magnitude of the theoretical sample is as shown:
n= Z2 P(1−P)
d2 ≈385 respondents
xxix
The chosen platform can transfer the gathered data to software like Xlstat, SPSS, and Stat
view for statistics.
For the collection of data, two methods have been prescribed:
First is an on-line method, composed of well-structured as well as simple forms
providing an instantaneous collection of a large number of answers alongside simple
tools for manipulation (Pistoia, 2010).
The second method is to conduct face-to-face interviews, composed of physical
interviews that could help in providing an actual insight regarding the opinions of the
interviewee alongside more elaborate answers.
4.1 Sampling Methodology:
In a bid to ascertain the efficient representativeness of samples regarding the target
population, the method of simple random sampling was chosen. Hence, all probable
combinations pulled off the demographic compositions having similar chances to be chosen.
In other terms, all the aspects of the population have the same probability to form part of the
sample (Nordic Council of Ministers, 2010). The size of the theoretical sample was
determined as per the equation below:
n= Z2 P(1−P)
d2
Where,
n is the sample size that needs to be determined.
Z = 1.96 which is representative of the values provided in the normal law table having a
confidence level of 95%.
d is the error in sampling otherwise tolerated margin of error while P is the fraction of people
that had their behavior calculated.
P is calculated to be 0.5 for the case of an infinite demographic composition.
When P=0.5, a threshold of confidence of 95% and an allowable margin of error +/- 5%,
which is equivalent to 0.05, the magnitude of the theoretical sample is as shown:
n= Z2 P(1−P)
d2 ≈385 respondents
xxix
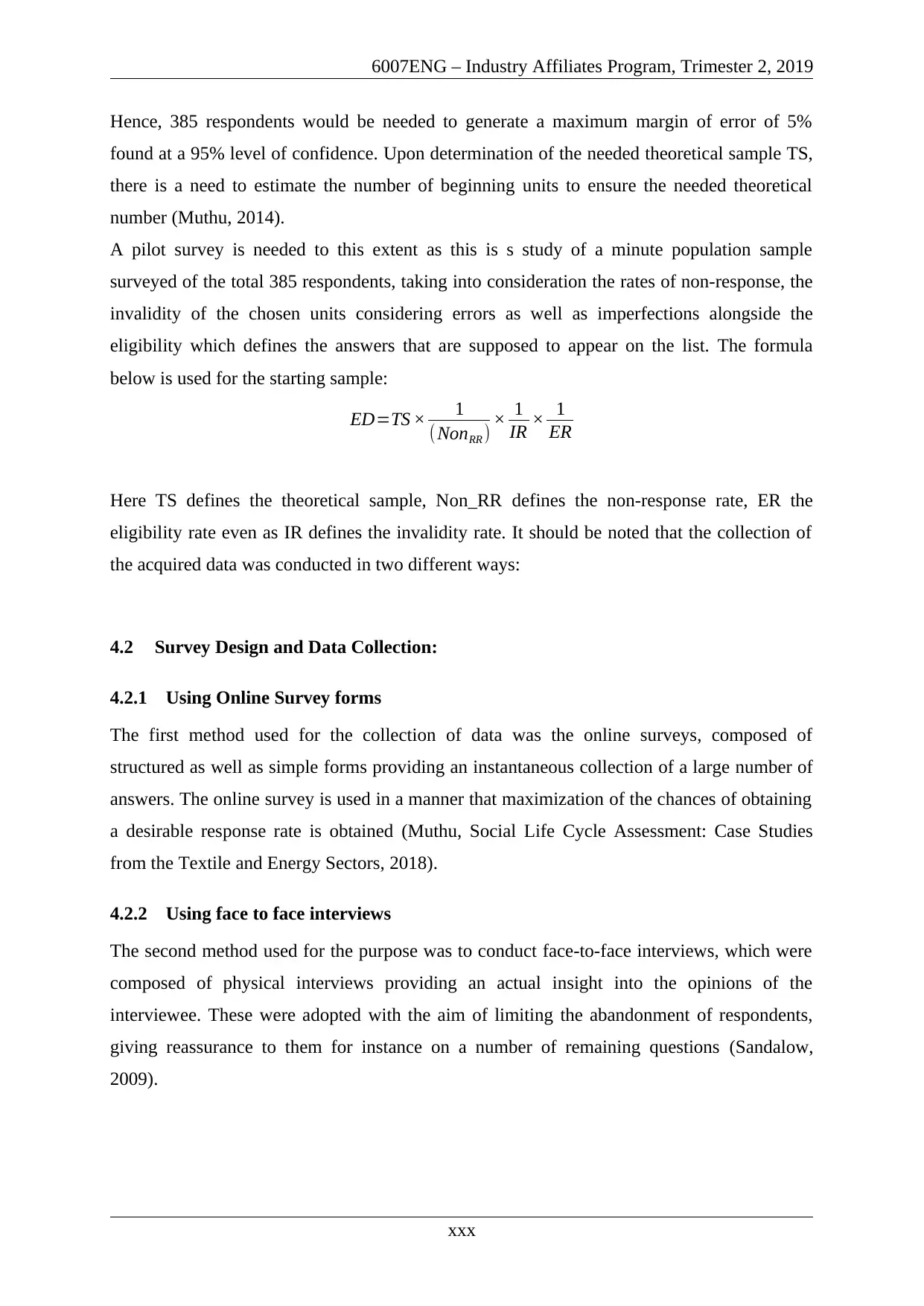
6007ENG – Industry Affiliates Program, Trimester 2, 2019
Hence, 385 respondents would be needed to generate a maximum margin of error of 5%
found at a 95% level of confidence. Upon determination of the needed theoretical sample TS,
there is a need to estimate the number of beginning units to ensure the needed theoretical
number (Muthu, 2014).
A pilot survey is needed to this extent as this is s study of a minute population sample
surveyed of the total 385 respondents, taking into consideration the rates of non-response, the
invalidity of the chosen units considering errors as well as imperfections alongside the
eligibility which defines the answers that are supposed to appear on the list. The formula
below is used for the starting sample:
ED=TS × 1
(NonRR ) × 1
IR × 1
ER
Here TS defines the theoretical sample, Non_RR defines the non-response rate, ER the
eligibility rate even as IR defines the invalidity rate. It should be noted that the collection of
the acquired data was conducted in two different ways:
4.2 Survey Design and Data Collection:
4.2.1 Using Online Survey forms
The first method used for the collection of data was the online surveys, composed of
structured as well as simple forms providing an instantaneous collection of a large number of
answers. The online survey is used in a manner that maximization of the chances of obtaining
a desirable response rate is obtained (Muthu, Social Life Cycle Assessment: Case Studies
from the Textile and Energy Sectors, 2018).
4.2.2 Using face to face interviews
The second method used for the purpose was to conduct face-to-face interviews, which were
composed of physical interviews providing an actual insight into the opinions of the
interviewee. These were adopted with the aim of limiting the abandonment of respondents,
giving reassurance to them for instance on a number of remaining questions (Sandalow,
2009).
xxx
Hence, 385 respondents would be needed to generate a maximum margin of error of 5%
found at a 95% level of confidence. Upon determination of the needed theoretical sample TS,
there is a need to estimate the number of beginning units to ensure the needed theoretical
number (Muthu, 2014).
A pilot survey is needed to this extent as this is s study of a minute population sample
surveyed of the total 385 respondents, taking into consideration the rates of non-response, the
invalidity of the chosen units considering errors as well as imperfections alongside the
eligibility which defines the answers that are supposed to appear on the list. The formula
below is used for the starting sample:
ED=TS × 1
(NonRR ) × 1
IR × 1
ER
Here TS defines the theoretical sample, Non_RR defines the non-response rate, ER the
eligibility rate even as IR defines the invalidity rate. It should be noted that the collection of
the acquired data was conducted in two different ways:
4.2 Survey Design and Data Collection:
4.2.1 Using Online Survey forms
The first method used for the collection of data was the online surveys, composed of
structured as well as simple forms providing an instantaneous collection of a large number of
answers. The online survey is used in a manner that maximization of the chances of obtaining
a desirable response rate is obtained (Muthu, Social Life Cycle Assessment: Case Studies
from the Textile and Energy Sectors, 2018).
4.2.2 Using face to face interviews
The second method used for the purpose was to conduct face-to-face interviews, which were
composed of physical interviews providing an actual insight into the opinions of the
interviewee. These were adopted with the aim of limiting the abandonment of respondents,
giving reassurance to them for instance on a number of remaining questions (Sandalow,
2009).
xxx
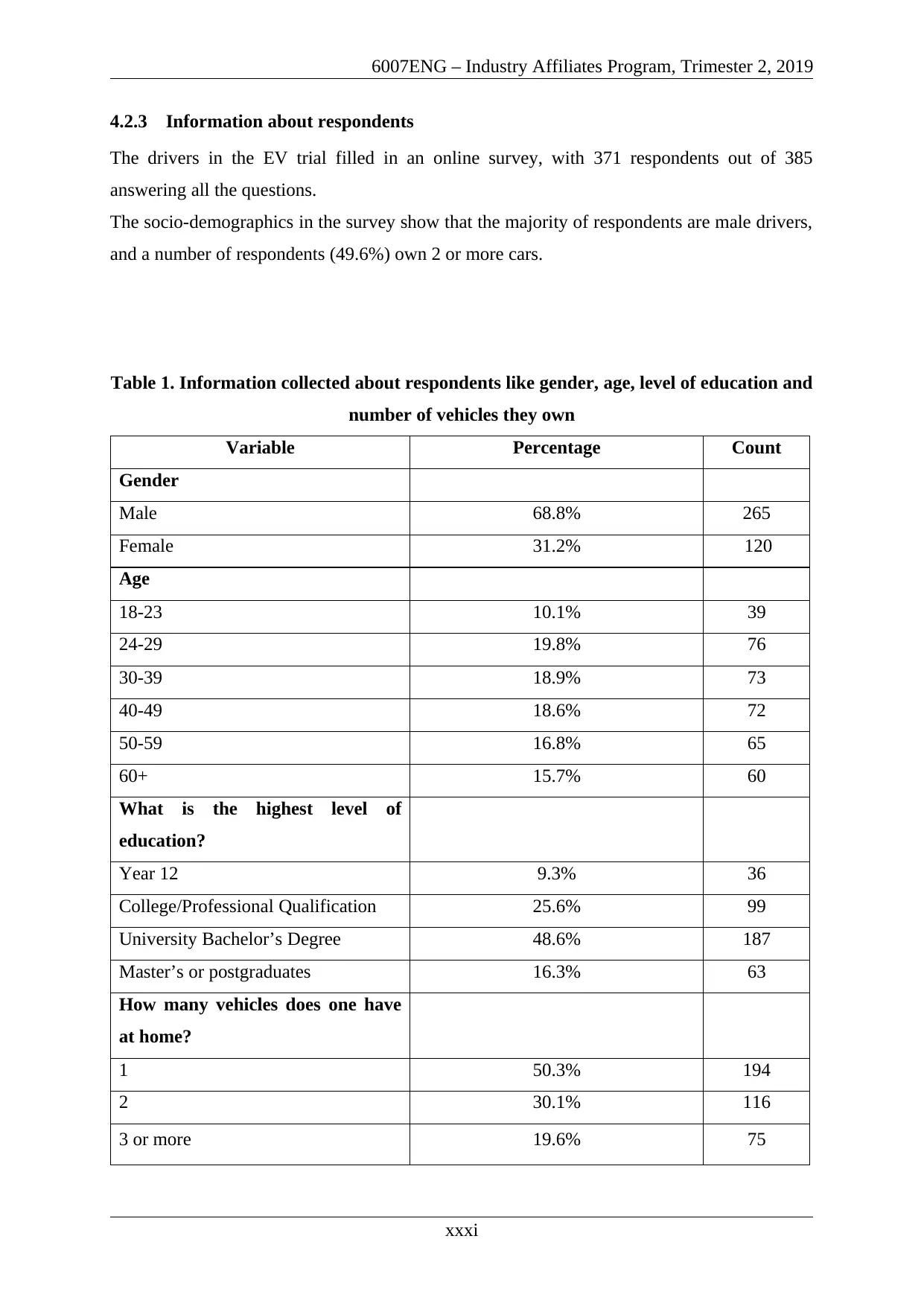
6007ENG – Industry Affiliates Program, Trimester 2, 2019
4.2.3 Information about respondents
The drivers in the EV trial filled in an online survey, with 371 respondents out of 385
answering all the questions.
The socio-demographics in the survey show that the majority of respondents are male drivers,
and a number of respondents (49.6%) own 2 or more cars.
Table 1. Information collected about respondents like gender, age, level of education and
number of vehicles they own
Variable Percentage Count
Gender
Male 68.8% 265
Female 31.2% 120
Age
18-23 10.1% 39
24-29 19.8% 76
30-39 18.9% 73
40-49 18.6% 72
50-59 16.8% 65
60+ 15.7% 60
What is the highest level of
education?
Year 12 9.3% 36
College/Professional Qualification 25.6% 99
University Bachelor’s Degree 48.6% 187
Master’s or postgraduates 16.3% 63
How many vehicles does one have
at home?
1 50.3% 194
2 30.1% 116
3 or more 19.6% 75
xxxi
4.2.3 Information about respondents
The drivers in the EV trial filled in an online survey, with 371 respondents out of 385
answering all the questions.
The socio-demographics in the survey show that the majority of respondents are male drivers,
and a number of respondents (49.6%) own 2 or more cars.
Table 1. Information collected about respondents like gender, age, level of education and
number of vehicles they own
Variable Percentage Count
Gender
Male 68.8% 265
Female 31.2% 120
Age
18-23 10.1% 39
24-29 19.8% 76
30-39 18.9% 73
40-49 18.6% 72
50-59 16.8% 65
60+ 15.7% 60
What is the highest level of
education?
Year 12 9.3% 36
College/Professional Qualification 25.6% 99
University Bachelor’s Degree 48.6% 187
Master’s or postgraduates 16.3% 63
How many vehicles does one have
at home?
1 50.3% 194
2 30.1% 116
3 or more 19.6% 75
xxxi
Secure Best Marks with AI Grader
Need help grading? Try our AI Grader for instant feedback on your assignments.
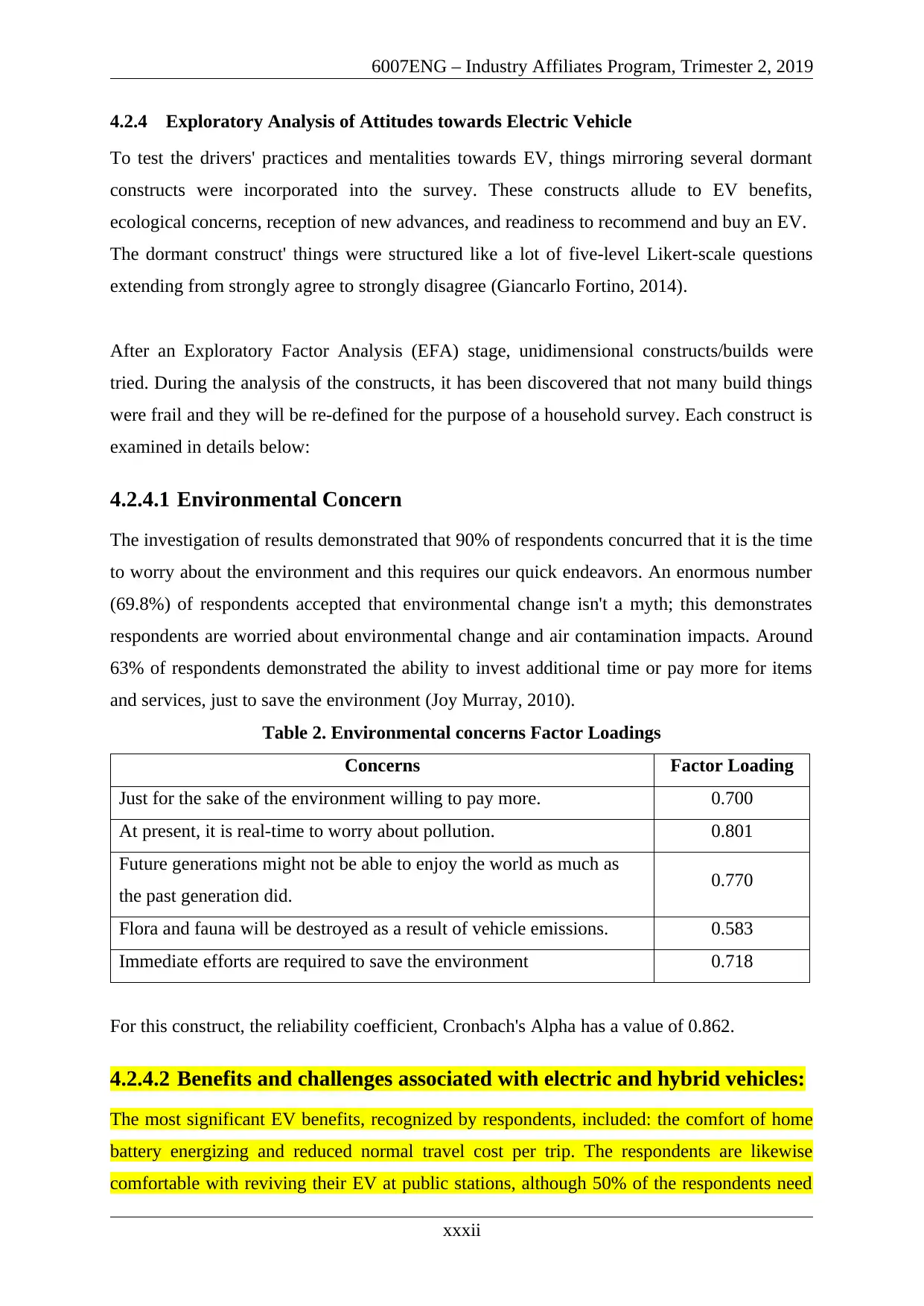
6007ENG – Industry Affiliates Program, Trimester 2, 2019
4.2.4 Exploratory Analysis of Attitudes towards Electric Vehicle
To test the drivers' practices and mentalities towards EV, things mirroring several dormant
constructs were incorporated into the survey. These constructs allude to EV benefits,
ecological concerns, reception of new advances, and readiness to recommend and buy an EV.
The dormant construct' things were structured like a lot of five-level Likert-scale questions
extending from strongly agree to strongly disagree (Giancarlo Fortino, 2014).
After an Exploratory Factor Analysis (EFA) stage, unidimensional constructs/builds were
tried. During the analysis of the constructs, it has been discovered that not many build things
were frail and they will be re-defined for the purpose of a household survey. Each construct is
examined in details below:
4.2.4.1 Environmental Concern
The investigation of results demonstrated that 90% of respondents concurred that it is the time
to worry about the environment and this requires our quick endeavors. An enormous number
(69.8%) of respondents accepted that environmental change isn't a myth; this demonstrates
respondents are worried about environmental change and air contamination impacts. Around
63% of respondents demonstrated the ability to invest additional time or pay more for items
and services, just to save the environment (Joy Murray, 2010).
Table 2. Environmental concerns Factor Loadings
Concerns Factor Loading
Just for the sake of the environment willing to pay more. 0.700
At present, it is real-time to worry about pollution. 0.801
Future generations might not be able to enjoy the world as much as
the past generation did. 0.770
Flora and fauna will be destroyed as a result of vehicle emissions. 0.583
Immediate efforts are required to save the environment 0.718
For this construct, the reliability coefficient, Cronbach's Alpha has a value of 0.862.
4.2.4.2 Benefits and challenges associated with electric and hybrid vehicles:
The most significant EV benefits, recognized by respondents, included: the comfort of home
battery energizing and reduced normal travel cost per trip. The respondents are likewise
comfortable with reviving their EV at public stations, although 50% of the respondents need
xxxii
4.2.4 Exploratory Analysis of Attitudes towards Electric Vehicle
To test the drivers' practices and mentalities towards EV, things mirroring several dormant
constructs were incorporated into the survey. These constructs allude to EV benefits,
ecological concerns, reception of new advances, and readiness to recommend and buy an EV.
The dormant construct' things were structured like a lot of five-level Likert-scale questions
extending from strongly agree to strongly disagree (Giancarlo Fortino, 2014).
After an Exploratory Factor Analysis (EFA) stage, unidimensional constructs/builds were
tried. During the analysis of the constructs, it has been discovered that not many build things
were frail and they will be re-defined for the purpose of a household survey. Each construct is
examined in details below:
4.2.4.1 Environmental Concern
The investigation of results demonstrated that 90% of respondents concurred that it is the time
to worry about the environment and this requires our quick endeavors. An enormous number
(69.8%) of respondents accepted that environmental change isn't a myth; this demonstrates
respondents are worried about environmental change and air contamination impacts. Around
63% of respondents demonstrated the ability to invest additional time or pay more for items
and services, just to save the environment (Joy Murray, 2010).
Table 2. Environmental concerns Factor Loadings
Concerns Factor Loading
Just for the sake of the environment willing to pay more. 0.700
At present, it is real-time to worry about pollution. 0.801
Future generations might not be able to enjoy the world as much as
the past generation did. 0.770
Flora and fauna will be destroyed as a result of vehicle emissions. 0.583
Immediate efforts are required to save the environment 0.718
For this construct, the reliability coefficient, Cronbach's Alpha has a value of 0.862.
4.2.4.2 Benefits and challenges associated with electric and hybrid vehicles:
The most significant EV benefits, recognized by respondents, included: the comfort of home
battery energizing and reduced normal travel cost per trip. The respondents are likewise
comfortable with reviving their EV at public stations, although 50% of the respondents need
xxxii
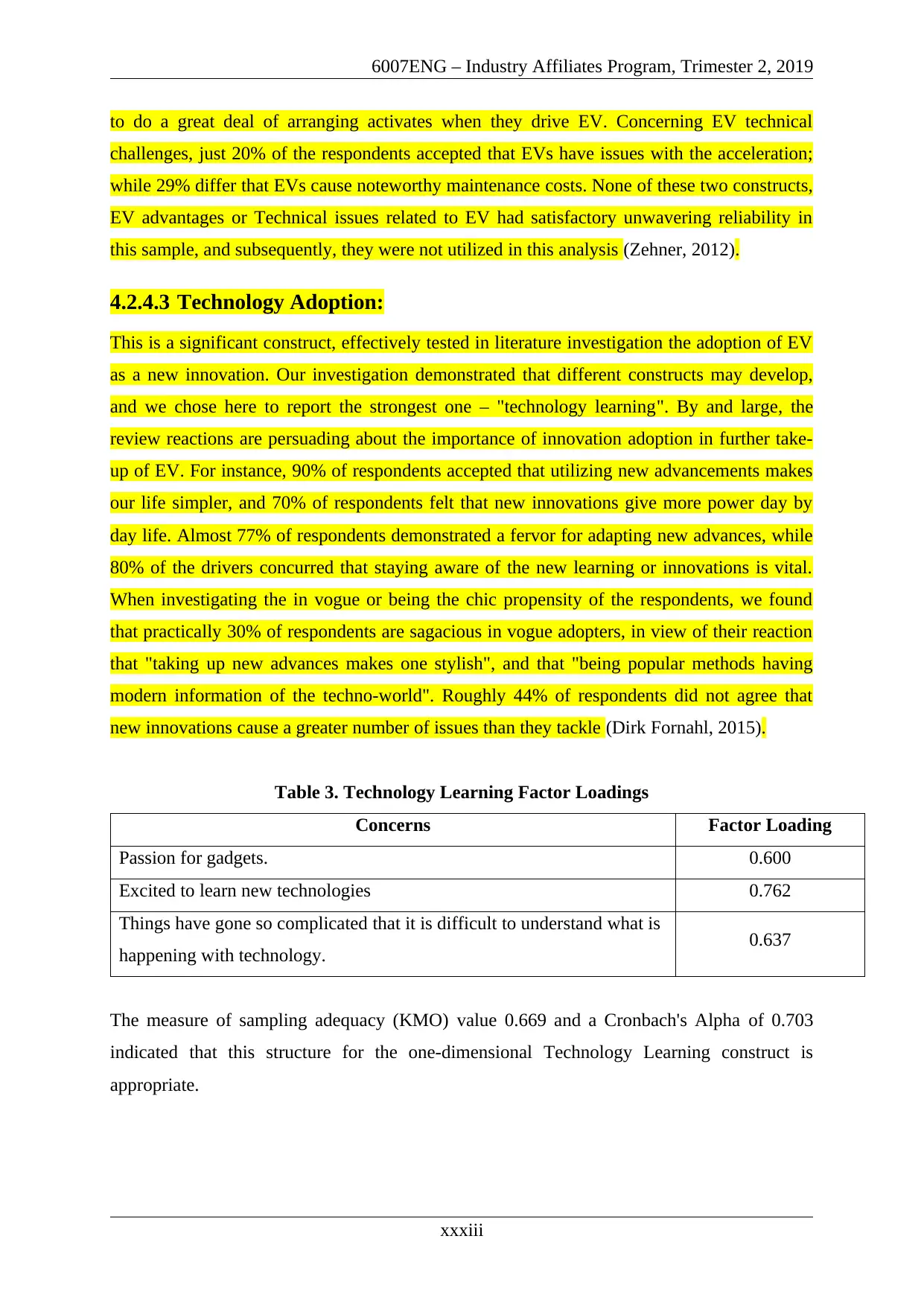
6007ENG – Industry Affiliates Program, Trimester 2, 2019
to do a great deal of arranging activates when they drive EV. Concerning EV technical
challenges, just 20% of the respondents accepted that EVs have issues with the acceleration;
while 29% differ that EVs cause noteworthy maintenance costs. None of these two constructs,
EV advantages or Technical issues related to EV had satisfactory unwavering reliability in
this sample, and subsequently, they were not utilized in this analysis (Zehner, 2012).
4.2.4.3 Technology Adoption:
This is a significant construct, effectively tested in literature investigation the adoption of EV
as a new innovation. Our investigation demonstrated that different constructs may develop,
and we chose here to report the strongest one – "technology learning". By and large, the
review reactions are persuading about the importance of innovation adoption in further take-
up of EV. For instance, 90% of respondents accepted that utilizing new advancements makes
our life simpler, and 70% of respondents felt that new innovations give more power day by
day life. Almost 77% of respondents demonstrated a fervor for adapting new advances, while
80% of the drivers concurred that staying aware of the new learning or innovations is vital.
When investigating the in vogue or being the chic propensity of the respondents, we found
that practically 30% of respondents are sagacious in vogue adopters, in view of their reaction
that "taking up new advances makes one stylish", and that "being popular methods having
modern information of the techno-world". Roughly 44% of respondents did not agree that
new innovations cause a greater number of issues than they tackle (Dirk Fornahl, 2015).
Table 3. Technology Learning Factor Loadings
Concerns Factor Loading
Passion for gadgets. 0.600
Excited to learn new technologies 0.762
Things have gone so complicated that it is difficult to understand what is
happening with technology. 0.637
The measure of sampling adequacy (KMO) value 0.669 and a Cronbach's Alpha of 0.703
indicated that this structure for the one-dimensional Technology Learning construct is
appropriate.
xxxiii
to do a great deal of arranging activates when they drive EV. Concerning EV technical
challenges, just 20% of the respondents accepted that EVs have issues with the acceleration;
while 29% differ that EVs cause noteworthy maintenance costs. None of these two constructs,
EV advantages or Technical issues related to EV had satisfactory unwavering reliability in
this sample, and subsequently, they were not utilized in this analysis (Zehner, 2012).
4.2.4.3 Technology Adoption:
This is a significant construct, effectively tested in literature investigation the adoption of EV
as a new innovation. Our investigation demonstrated that different constructs may develop,
and we chose here to report the strongest one – "technology learning". By and large, the
review reactions are persuading about the importance of innovation adoption in further take-
up of EV. For instance, 90% of respondents accepted that utilizing new advancements makes
our life simpler, and 70% of respondents felt that new innovations give more power day by
day life. Almost 77% of respondents demonstrated a fervor for adapting new advances, while
80% of the drivers concurred that staying aware of the new learning or innovations is vital.
When investigating the in vogue or being the chic propensity of the respondents, we found
that practically 30% of respondents are sagacious in vogue adopters, in view of their reaction
that "taking up new advances makes one stylish", and that "being popular methods having
modern information of the techno-world". Roughly 44% of respondents did not agree that
new innovations cause a greater number of issues than they tackle (Dirk Fornahl, 2015).
Table 3. Technology Learning Factor Loadings
Concerns Factor Loading
Passion for gadgets. 0.600
Excited to learn new technologies 0.762
Things have gone so complicated that it is difficult to understand what is
happening with technology. 0.637
The measure of sampling adequacy (KMO) value 0.669 and a Cronbach's Alpha of 0.703
indicated that this structure for the one-dimensional Technology Learning construct is
appropriate.
xxxiii
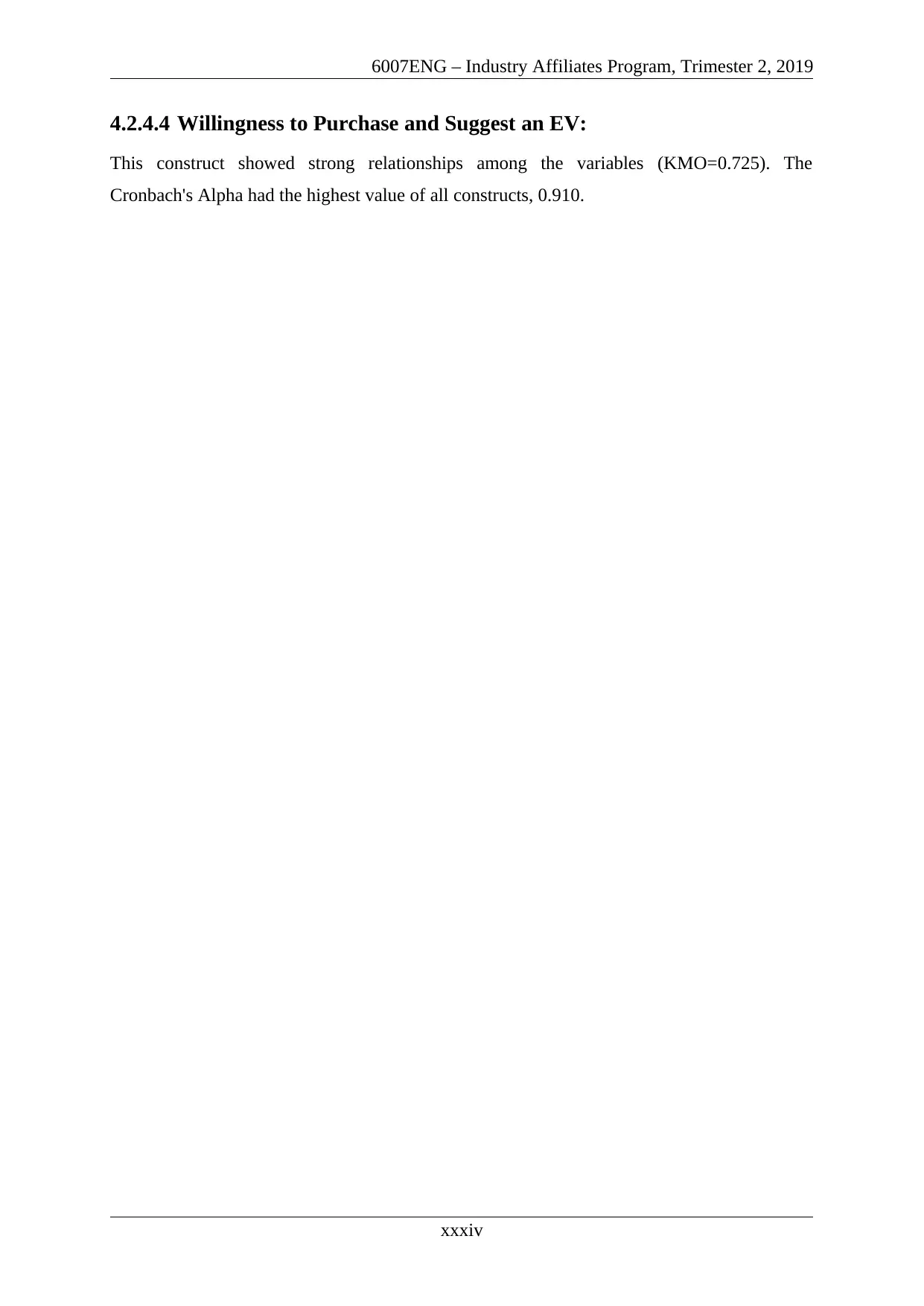
6007ENG – Industry Affiliates Program, Trimester 2, 2019
4.2.4.4 Willingness to Purchase and Suggest an EV:
This construct showed strong relationships among the variables (KMO=0.725). The
Cronbach's Alpha had the highest value of all constructs, 0.910.
xxxiv
4.2.4.4 Willingness to Purchase and Suggest an EV:
This construct showed strong relationships among the variables (KMO=0.725). The
Cronbach's Alpha had the highest value of all constructs, 0.910.
xxxiv
Paraphrase This Document
Need a fresh take? Get an instant paraphrase of this document with our AI Paraphraser
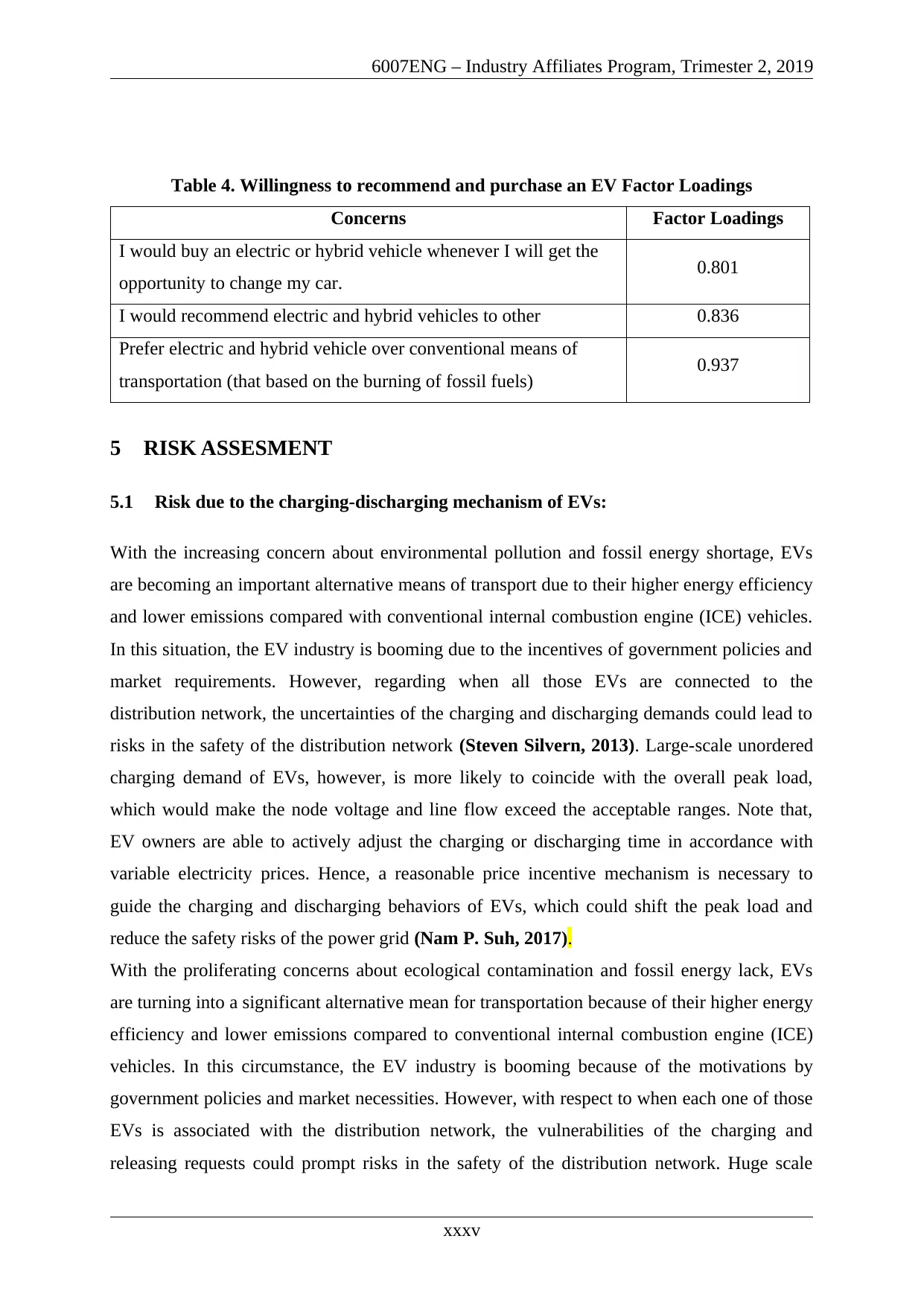
6007ENG – Industry Affiliates Program, Trimester 2, 2019
Table 4. Willingness to recommend and purchase an EV Factor Loadings
Concerns Factor Loadings
I would buy an electric or hybrid vehicle whenever I will get the
opportunity to change my car. 0.801
I would recommend electric and hybrid vehicles to other 0.836
Prefer electric and hybrid vehicle over conventional means of
transportation (that based on the burning of fossil fuels) 0.937
5 RISK ASSESMENT
5.1 Risk due to the charging-discharging mechanism of EVs:
With the increasing concern about environmental pollution and fossil energy shortage, EVs
are becoming an important alternative means of transport due to their higher energy efficiency
and lower emissions compared with conventional internal combustion engine (ICE) vehicles.
In this situation, the EV industry is booming due to the incentives of government policies and
market requirements. However, regarding when all those EVs are connected to the
distribution network, the uncertainties of the charging and discharging demands could lead to
risks in the safety of the distribution network (Steven Silvern, 2013). Large-scale unordered
charging demand of EVs, however, is more likely to coincide with the overall peak load,
which would make the node voltage and line flow exceed the acceptable ranges. Note that,
EV owners are able to actively adjust the charging or discharging time in accordance with
variable electricity prices. Hence, a reasonable price incentive mechanism is necessary to
guide the charging and discharging behaviors of EVs, which could shift the peak load and
reduce the safety risks of the power grid (Nam P. Suh, 2017).
With the proliferating concerns about ecological contamination and fossil energy lack, EVs
are turning into a significant alternative mean for transportation because of their higher energy
efficiency and lower emissions compared to conventional internal combustion engine (ICE)
vehicles. In this circumstance, the EV industry is booming because of the motivations by
government policies and market necessities. However, with respect to when each one of those
EVs is associated with the distribution network, the vulnerabilities of the charging and
releasing requests could prompt risks in the safety of the distribution network. Huge scale
xxxv
Table 4. Willingness to recommend and purchase an EV Factor Loadings
Concerns Factor Loadings
I would buy an electric or hybrid vehicle whenever I will get the
opportunity to change my car. 0.801
I would recommend electric and hybrid vehicles to other 0.836
Prefer electric and hybrid vehicle over conventional means of
transportation (that based on the burning of fossil fuels) 0.937
5 RISK ASSESMENT
5.1 Risk due to the charging-discharging mechanism of EVs:
With the increasing concern about environmental pollution and fossil energy shortage, EVs
are becoming an important alternative means of transport due to their higher energy efficiency
and lower emissions compared with conventional internal combustion engine (ICE) vehicles.
In this situation, the EV industry is booming due to the incentives of government policies and
market requirements. However, regarding when all those EVs are connected to the
distribution network, the uncertainties of the charging and discharging demands could lead to
risks in the safety of the distribution network (Steven Silvern, 2013). Large-scale unordered
charging demand of EVs, however, is more likely to coincide with the overall peak load,
which would make the node voltage and line flow exceed the acceptable ranges. Note that,
EV owners are able to actively adjust the charging or discharging time in accordance with
variable electricity prices. Hence, a reasonable price incentive mechanism is necessary to
guide the charging and discharging behaviors of EVs, which could shift the peak load and
reduce the safety risks of the power grid (Nam P. Suh, 2017).
With the proliferating concerns about ecological contamination and fossil energy lack, EVs
are turning into a significant alternative mean for transportation because of their higher energy
efficiency and lower emissions compared to conventional internal combustion engine (ICE)
vehicles. In this circumstance, the EV industry is booming because of the motivations by
government policies and market necessities. However, with respect to when each one of those
EVs is associated with the distribution network, the vulnerabilities of the charging and
releasing requests could prompt risks in the safety of the distribution network. Huge scale
xxxv
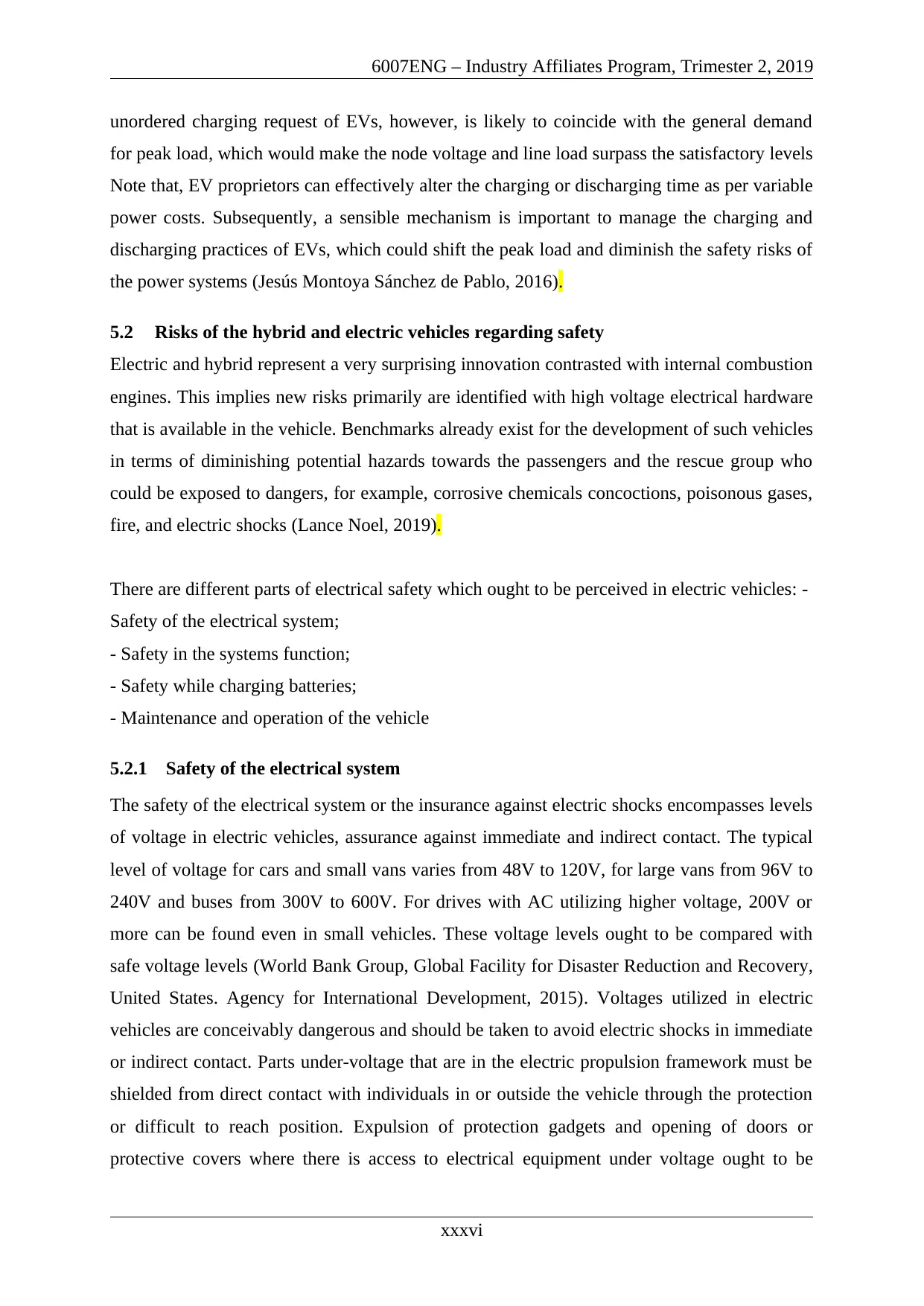
6007ENG – Industry Affiliates Program, Trimester 2, 2019
unordered charging request of EVs, however, is likely to coincide with the general demand
for peak load, which would make the node voltage and line load surpass the satisfactory levels
Note that, EV proprietors can effectively alter the charging or discharging time as per variable
power costs. Subsequently, a sensible mechanism is important to manage the charging and
discharging practices of EVs, which could shift the peak load and diminish the safety risks of
the power systems (Jesús Montoya Sánchez de Pablo, 2016).
5.2 Risks of the hybrid and electric vehicles regarding safety
Electric and hybrid represent a very surprising innovation contrasted with internal combustion
engines. This implies new risks primarily are identified with high voltage electrical hardware
that is available in the vehicle. Benchmarks already exist for the development of such vehicles
in terms of diminishing potential hazards towards the passengers and the rescue group who
could be exposed to dangers, for example, corrosive chemicals concoctions, poisonous gases,
fire, and electric shocks (Lance Noel, 2019).
There are different parts of electrical safety which ought to be perceived in electric vehicles: -
Safety of the electrical system;
- Safety in the systems function;
- Safety while charging batteries;
- Maintenance and operation of the vehicle
5.2.1 Safety of the electrical system
The safety of the electrical system or the insurance against electric shocks encompasses levels
of voltage in electric vehicles, assurance against immediate and indirect contact. The typical
level of voltage for cars and small vans varies from 48V to 120V, for large vans from 96V to
240V and buses from 300V to 600V. For drives with AC utilizing higher voltage, 200V or
more can be found even in small vehicles. These voltage levels ought to be compared with
safe voltage levels (World Bank Group, Global Facility for Disaster Reduction and Recovery,
United States. Agency for International Development, 2015). Voltages utilized in electric
vehicles are conceivably dangerous and should be taken to avoid electric shocks in immediate
or indirect contact. Parts under-voltage that are in the electric propulsion framework must be
shielded from direct contact with individuals in or outside the vehicle through the protection
or difficult to reach position. Expulsion of protection gadgets and opening of doors or
protective covers where there is access to electrical equipment under voltage ought to be
xxxvi
unordered charging request of EVs, however, is likely to coincide with the general demand
for peak load, which would make the node voltage and line load surpass the satisfactory levels
Note that, EV proprietors can effectively alter the charging or discharging time as per variable
power costs. Subsequently, a sensible mechanism is important to manage the charging and
discharging practices of EVs, which could shift the peak load and diminish the safety risks of
the power systems (Jesús Montoya Sánchez de Pablo, 2016).
5.2 Risks of the hybrid and electric vehicles regarding safety
Electric and hybrid represent a very surprising innovation contrasted with internal combustion
engines. This implies new risks primarily are identified with high voltage electrical hardware
that is available in the vehicle. Benchmarks already exist for the development of such vehicles
in terms of diminishing potential hazards towards the passengers and the rescue group who
could be exposed to dangers, for example, corrosive chemicals concoctions, poisonous gases,
fire, and electric shocks (Lance Noel, 2019).
There are different parts of electrical safety which ought to be perceived in electric vehicles: -
Safety of the electrical system;
- Safety in the systems function;
- Safety while charging batteries;
- Maintenance and operation of the vehicle
5.2.1 Safety of the electrical system
The safety of the electrical system or the insurance against electric shocks encompasses levels
of voltage in electric vehicles, assurance against immediate and indirect contact. The typical
level of voltage for cars and small vans varies from 48V to 120V, for large vans from 96V to
240V and buses from 300V to 600V. For drives with AC utilizing higher voltage, 200V or
more can be found even in small vehicles. These voltage levels ought to be compared with
safe voltage levels (World Bank Group, Global Facility for Disaster Reduction and Recovery,
United States. Agency for International Development, 2015). Voltages utilized in electric
vehicles are conceivably dangerous and should be taken to avoid electric shocks in immediate
or indirect contact. Parts under-voltage that are in the electric propulsion framework must be
shielded from direct contact with individuals in or outside the vehicle through the protection
or difficult to reach position. Expulsion of protection gadgets and opening of doors or
protective covers where there is access to electrical equipment under voltage ought to be
xxxvi
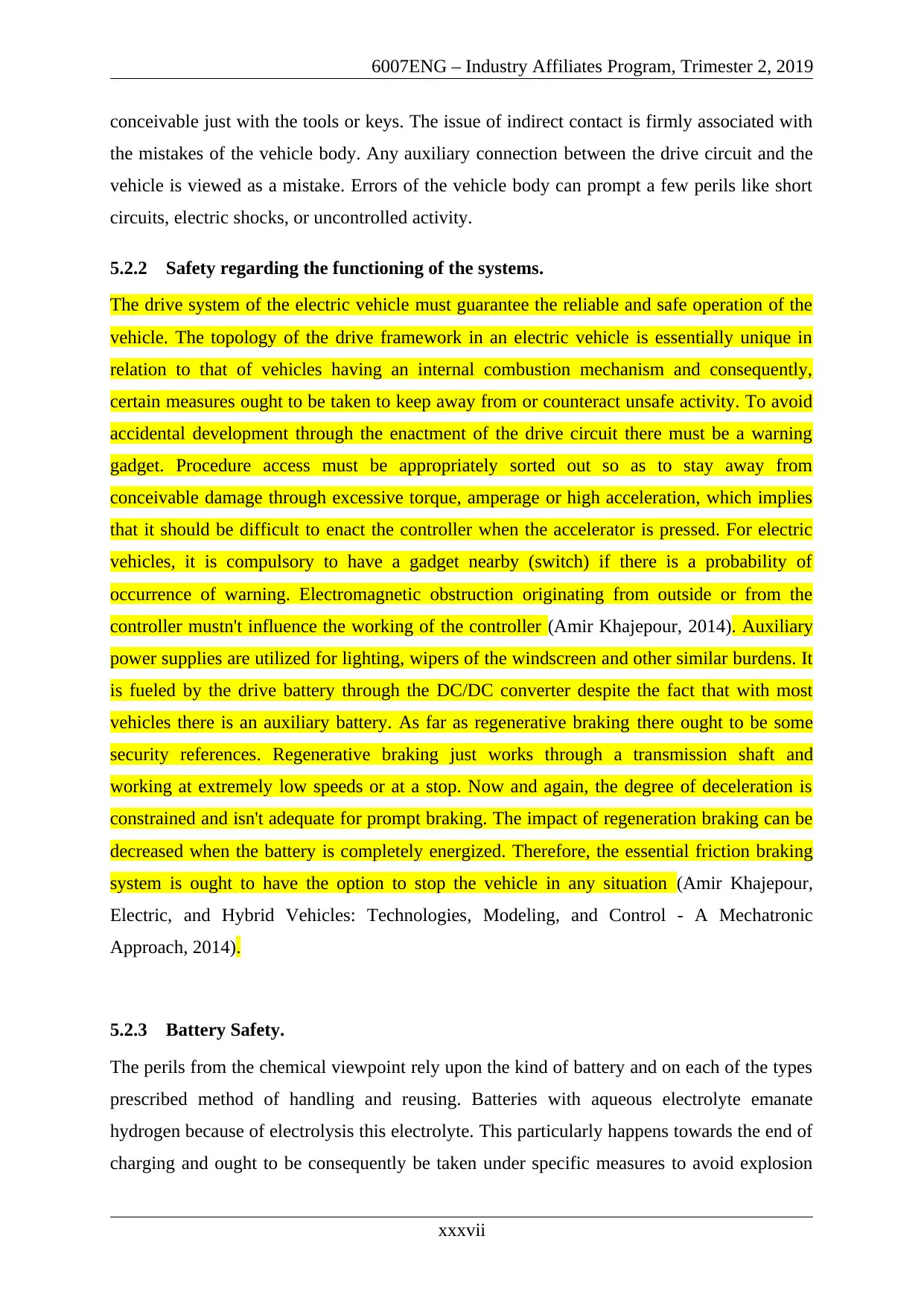
6007ENG – Industry Affiliates Program, Trimester 2, 2019
conceivable just with the tools or keys. The issue of indirect contact is firmly associated with
the mistakes of the vehicle body. Any auxiliary connection between the drive circuit and the
vehicle is viewed as a mistake. Errors of the vehicle body can prompt a few perils like short
circuits, electric shocks, or uncontrolled activity.
5.2.2 Safety regarding the functioning of the systems.
The drive system of the electric vehicle must guarantee the reliable and safe operation of the
vehicle. The topology of the drive framework in an electric vehicle is essentially unique in
relation to that of vehicles having an internal combustion mechanism and consequently,
certain measures ought to be taken to keep away from or counteract unsafe activity. To avoid
accidental development through the enactment of the drive circuit there must be a warning
gadget. Procedure access must be appropriately sorted out so as to stay away from
conceivable damage through excessive torque, amperage or high acceleration, which implies
that it should be difficult to enact the controller when the accelerator is pressed. For electric
vehicles, it is compulsory to have a gadget nearby (switch) if there is a probability of
occurrence of warning. Electromagnetic obstruction originating from outside or from the
controller mustn't influence the working of the controller (Amir Khajepour, 2014). Auxiliary
power supplies are utilized for lighting, wipers of the windscreen and other similar burdens. It
is fueled by the drive battery through the DC/DC converter despite the fact that with most
vehicles there is an auxiliary battery. As far as regenerative braking there ought to be some
security references. Regenerative braking just works through a transmission shaft and
working at extremely low speeds or at a stop. Now and again, the degree of deceleration is
constrained and isn't adequate for prompt braking. The impact of regeneration braking can be
decreased when the battery is completely energized. Therefore, the essential friction braking
system is ought to have the option to stop the vehicle in any situation (Amir Khajepour,
Electric, and Hybrid Vehicles: Technologies, Modeling, and Control - A Mechatronic
Approach, 2014).
5.2.3 Battery Safety.
The perils from the chemical viewpoint rely upon the kind of battery and on each of the types
prescribed method of handling and reusing. Batteries with aqueous electrolyte emanate
hydrogen because of electrolysis this electrolyte. This particularly happens towards the end of
charging and ought to be consequently be taken under specific measures to avoid explosion
xxxvii
conceivable just with the tools or keys. The issue of indirect contact is firmly associated with
the mistakes of the vehicle body. Any auxiliary connection between the drive circuit and the
vehicle is viewed as a mistake. Errors of the vehicle body can prompt a few perils like short
circuits, electric shocks, or uncontrolled activity.
5.2.2 Safety regarding the functioning of the systems.
The drive system of the electric vehicle must guarantee the reliable and safe operation of the
vehicle. The topology of the drive framework in an electric vehicle is essentially unique in
relation to that of vehicles having an internal combustion mechanism and consequently,
certain measures ought to be taken to keep away from or counteract unsafe activity. To avoid
accidental development through the enactment of the drive circuit there must be a warning
gadget. Procedure access must be appropriately sorted out so as to stay away from
conceivable damage through excessive torque, amperage or high acceleration, which implies
that it should be difficult to enact the controller when the accelerator is pressed. For electric
vehicles, it is compulsory to have a gadget nearby (switch) if there is a probability of
occurrence of warning. Electromagnetic obstruction originating from outside or from the
controller mustn't influence the working of the controller (Amir Khajepour, 2014). Auxiliary
power supplies are utilized for lighting, wipers of the windscreen and other similar burdens. It
is fueled by the drive battery through the DC/DC converter despite the fact that with most
vehicles there is an auxiliary battery. As far as regenerative braking there ought to be some
security references. Regenerative braking just works through a transmission shaft and
working at extremely low speeds or at a stop. Now and again, the degree of deceleration is
constrained and isn't adequate for prompt braking. The impact of regeneration braking can be
decreased when the battery is completely energized. Therefore, the essential friction braking
system is ought to have the option to stop the vehicle in any situation (Amir Khajepour,
Electric, and Hybrid Vehicles: Technologies, Modeling, and Control - A Mechatronic
Approach, 2014).
5.2.3 Battery Safety.
The perils from the chemical viewpoint rely upon the kind of battery and on each of the types
prescribed method of handling and reusing. Batteries with aqueous electrolyte emanate
hydrogen because of electrolysis this electrolyte. This particularly happens towards the end of
charging and ought to be consequently be taken under specific measures to avoid explosion
xxxvii
Secure Best Marks with AI Grader
Need help grading? Try our AI Grader for instant feedback on your assignments.
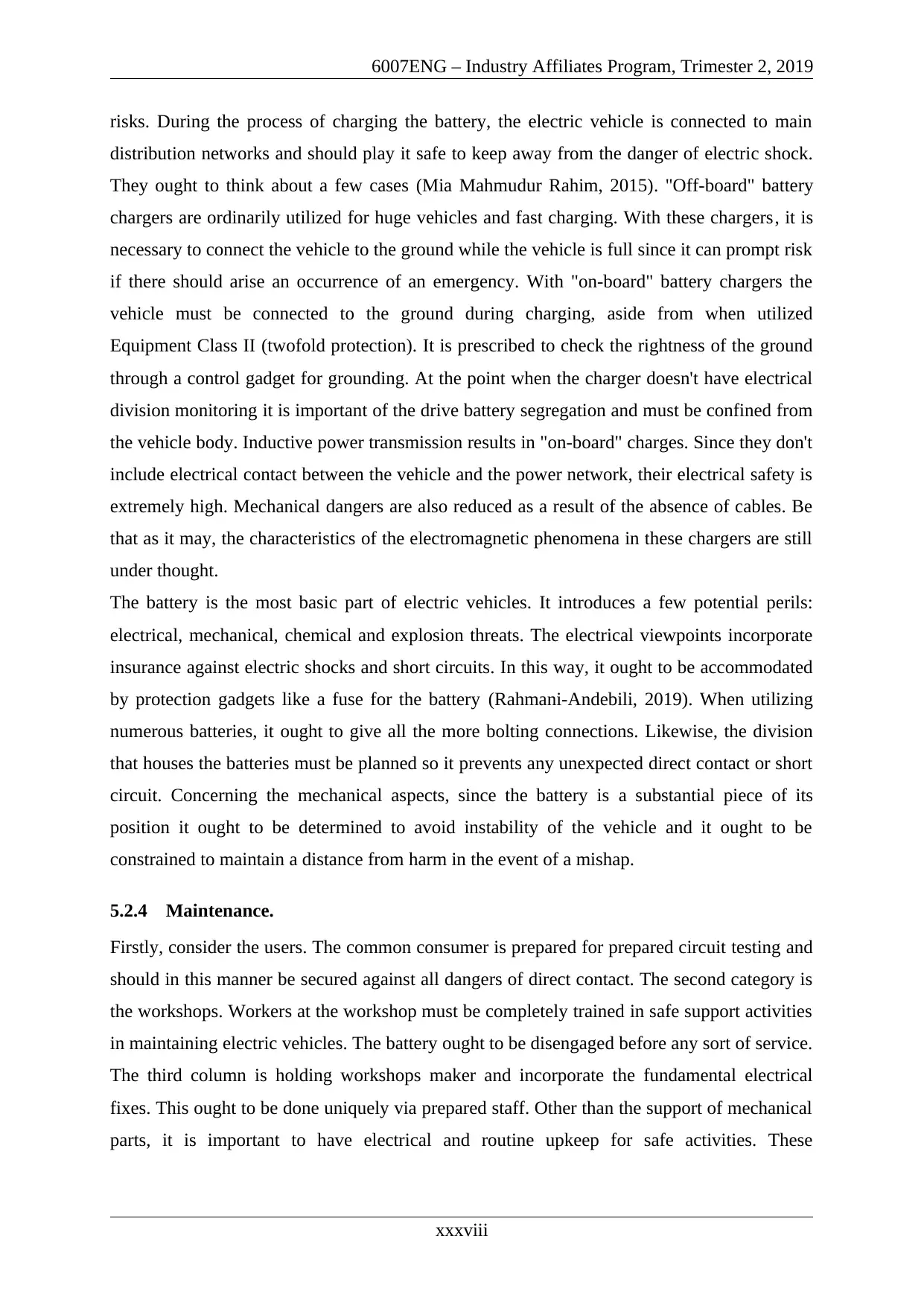
6007ENG – Industry Affiliates Program, Trimester 2, 2019
risks. During the process of charging the battery, the electric vehicle is connected to main
distribution networks and should play it safe to keep away from the danger of electric shock.
They ought to think about a few cases (Mia Mahmudur Rahim, 2015). "Off-board" battery
chargers are ordinarily utilized for huge vehicles and fast charging. With these chargers, it is
necessary to connect the vehicle to the ground while the vehicle is full since it can prompt risk
if there should arise an occurrence of an emergency. With "on-board" battery chargers the
vehicle must be connected to the ground during charging, aside from when utilized
Equipment Class II (twofold protection). It is prescribed to check the rightness of the ground
through a control gadget for grounding. At the point when the charger doesn't have electrical
division monitoring it is important of the drive battery segregation and must be confined from
the vehicle body. Inductive power transmission results in "on-board" charges. Since they don't
include electrical contact between the vehicle and the power network, their electrical safety is
extremely high. Mechanical dangers are also reduced as a result of the absence of cables. Be
that as it may, the characteristics of the electromagnetic phenomena in these chargers are still
under thought.
The battery is the most basic part of electric vehicles. It introduces a few potential perils:
electrical, mechanical, chemical and explosion threats. The electrical viewpoints incorporate
insurance against electric shocks and short circuits. In this way, it ought to be accommodated
by protection gadgets like a fuse for the battery (Rahmani-Andebili, 2019). When utilizing
numerous batteries, it ought to give all the more bolting connections. Likewise, the division
that houses the batteries must be planned so it prevents any unexpected direct contact or short
circuit. Concerning the mechanical aspects, since the battery is a substantial piece of its
position it ought to be determined to avoid instability of the vehicle and it ought to be
constrained to maintain a distance from harm in the event of a mishap.
5.2.4 Maintenance.
Firstly, consider the users. The common consumer is prepared for prepared circuit testing and
should in this manner be secured against all dangers of direct contact. The second category is
the workshops. Workers at the workshop must be completely trained in safe support activities
in maintaining electric vehicles. The battery ought to be disengaged before any sort of service.
The third column is holding workshops maker and incorporate the fundamental electrical
fixes. This ought to be done uniquely via prepared staff. Other than the support of mechanical
parts, it is important to have electrical and routine upkeep for safe activities. These
xxxviii
risks. During the process of charging the battery, the electric vehicle is connected to main
distribution networks and should play it safe to keep away from the danger of electric shock.
They ought to think about a few cases (Mia Mahmudur Rahim, 2015). "Off-board" battery
chargers are ordinarily utilized for huge vehicles and fast charging. With these chargers, it is
necessary to connect the vehicle to the ground while the vehicle is full since it can prompt risk
if there should arise an occurrence of an emergency. With "on-board" battery chargers the
vehicle must be connected to the ground during charging, aside from when utilized
Equipment Class II (twofold protection). It is prescribed to check the rightness of the ground
through a control gadget for grounding. At the point when the charger doesn't have electrical
division monitoring it is important of the drive battery segregation and must be confined from
the vehicle body. Inductive power transmission results in "on-board" charges. Since they don't
include electrical contact between the vehicle and the power network, their electrical safety is
extremely high. Mechanical dangers are also reduced as a result of the absence of cables. Be
that as it may, the characteristics of the electromagnetic phenomena in these chargers are still
under thought.
The battery is the most basic part of electric vehicles. It introduces a few potential perils:
electrical, mechanical, chemical and explosion threats. The electrical viewpoints incorporate
insurance against electric shocks and short circuits. In this way, it ought to be accommodated
by protection gadgets like a fuse for the battery (Rahmani-Andebili, 2019). When utilizing
numerous batteries, it ought to give all the more bolting connections. Likewise, the division
that houses the batteries must be planned so it prevents any unexpected direct contact or short
circuit. Concerning the mechanical aspects, since the battery is a substantial piece of its
position it ought to be determined to avoid instability of the vehicle and it ought to be
constrained to maintain a distance from harm in the event of a mishap.
5.2.4 Maintenance.
Firstly, consider the users. The common consumer is prepared for prepared circuit testing and
should in this manner be secured against all dangers of direct contact. The second category is
the workshops. Workers at the workshop must be completely trained in safe support activities
in maintaining electric vehicles. The battery ought to be disengaged before any sort of service.
The third column is holding workshops maker and incorporate the fundamental electrical
fixes. This ought to be done uniquely via prepared staff. Other than the support of mechanical
parts, it is important to have electrical and routine upkeep for safe activities. These
xxxviii
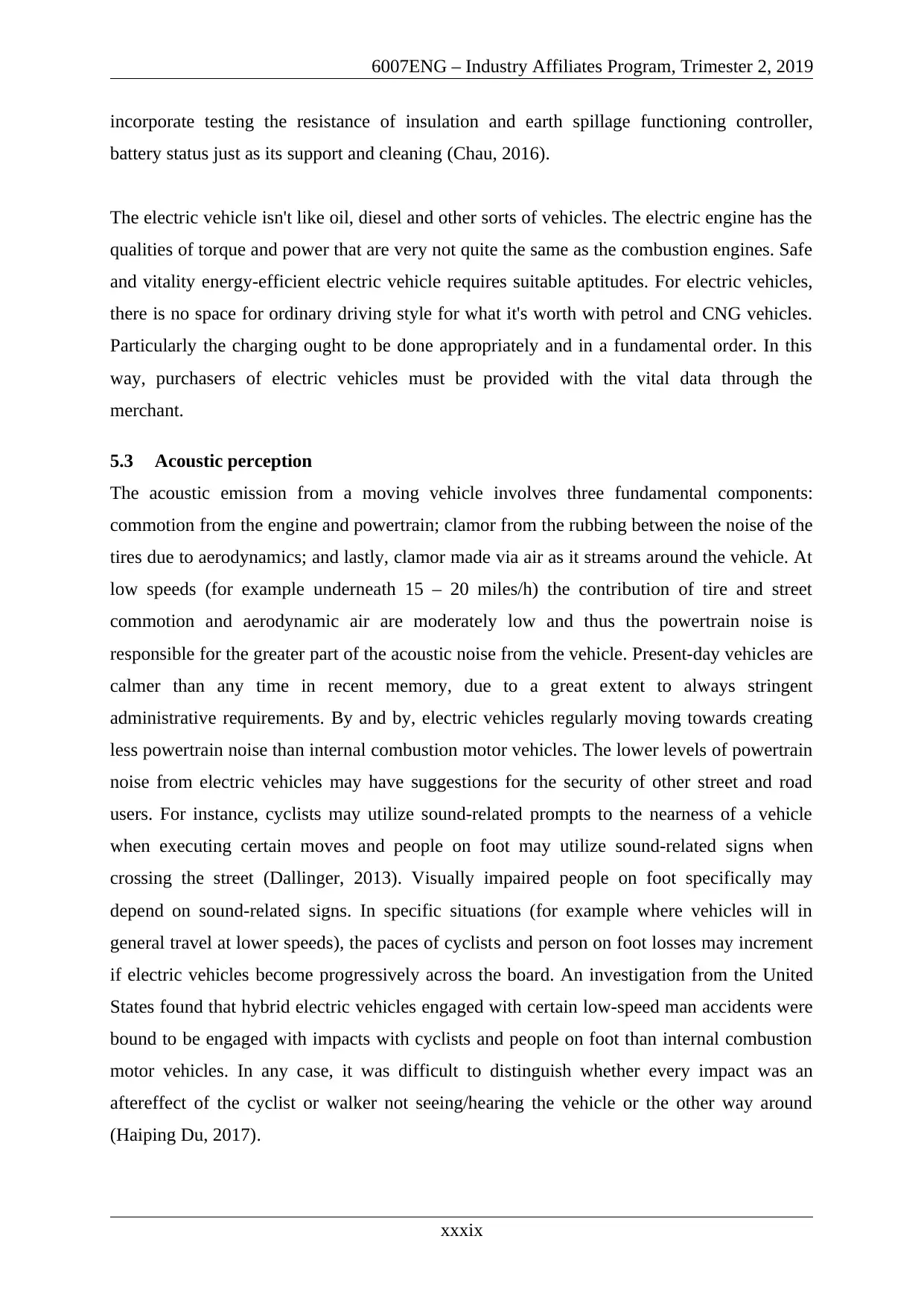
6007ENG – Industry Affiliates Program, Trimester 2, 2019
incorporate testing the resistance of insulation and earth spillage functioning controller,
battery status just as its support and cleaning (Chau, 2016).
The electric vehicle isn't like oil, diesel and other sorts of vehicles. The electric engine has the
qualities of torque and power that are very not quite the same as the combustion engines. Safe
and vitality energy-efficient electric vehicle requires suitable aptitudes. For electric vehicles,
there is no space for ordinary driving style for what it's worth with petrol and CNG vehicles.
Particularly the charging ought to be done appropriately and in a fundamental order. In this
way, purchasers of electric vehicles must be provided with the vital data through the
merchant.
5.3 Acoustic perception
The acoustic emission from a moving vehicle involves three fundamental components:
commotion from the engine and powertrain; clamor from the rubbing between the noise of the
tires due to aerodynamics; and lastly, clamor made via air as it streams around the vehicle. At
low speeds (for example underneath 15 – 20 miles/h) the contribution of tire and street
commotion and aerodynamic air are moderately low and thus the powertrain noise is
responsible for the greater part of the acoustic noise from the vehicle. Present-day vehicles are
calmer than any time in recent memory, due to a great extent to always stringent
administrative requirements. By and by, electric vehicles regularly moving towards creating
less powertrain noise than internal combustion motor vehicles. The lower levels of powertrain
noise from electric vehicles may have suggestions for the security of other street and road
users. For instance, cyclists may utilize sound-related prompts to the nearness of a vehicle
when executing certain moves and people on foot may utilize sound-related signs when
crossing the street (Dallinger, 2013). Visually impaired people on foot specifically may
depend on sound-related signs. In specific situations (for example where vehicles will in
general travel at lower speeds), the paces of cyclists and person on foot losses may increment
if electric vehicles become progressively across the board. An investigation from the United
States found that hybrid electric vehicles engaged with certain low-speed man accidents were
bound to be engaged with impacts with cyclists and people on foot than internal combustion
motor vehicles. In any case, it was difficult to distinguish whether every impact was an
aftereffect of the cyclist or walker not seeing/hearing the vehicle or the other way around
(Haiping Du, 2017).
xxxix
incorporate testing the resistance of insulation and earth spillage functioning controller,
battery status just as its support and cleaning (Chau, 2016).
The electric vehicle isn't like oil, diesel and other sorts of vehicles. The electric engine has the
qualities of torque and power that are very not quite the same as the combustion engines. Safe
and vitality energy-efficient electric vehicle requires suitable aptitudes. For electric vehicles,
there is no space for ordinary driving style for what it's worth with petrol and CNG vehicles.
Particularly the charging ought to be done appropriately and in a fundamental order. In this
way, purchasers of electric vehicles must be provided with the vital data through the
merchant.
5.3 Acoustic perception
The acoustic emission from a moving vehicle involves three fundamental components:
commotion from the engine and powertrain; clamor from the rubbing between the noise of the
tires due to aerodynamics; and lastly, clamor made via air as it streams around the vehicle. At
low speeds (for example underneath 15 – 20 miles/h) the contribution of tire and street
commotion and aerodynamic air are moderately low and thus the powertrain noise is
responsible for the greater part of the acoustic noise from the vehicle. Present-day vehicles are
calmer than any time in recent memory, due to a great extent to always stringent
administrative requirements. By and by, electric vehicles regularly moving towards creating
less powertrain noise than internal combustion motor vehicles. The lower levels of powertrain
noise from electric vehicles may have suggestions for the security of other street and road
users. For instance, cyclists may utilize sound-related prompts to the nearness of a vehicle
when executing certain moves and people on foot may utilize sound-related signs when
crossing the street (Dallinger, 2013). Visually impaired people on foot specifically may
depend on sound-related signs. In specific situations (for example where vehicles will in
general travel at lower speeds), the paces of cyclists and person on foot losses may increment
if electric vehicles become progressively across the board. An investigation from the United
States found that hybrid electric vehicles engaged with certain low-speed man accidents were
bound to be engaged with impacts with cyclists and people on foot than internal combustion
motor vehicles. In any case, it was difficult to distinguish whether every impact was an
aftereffect of the cyclist or walker not seeing/hearing the vehicle or the other way around
(Haiping Du, 2017).
xxxix
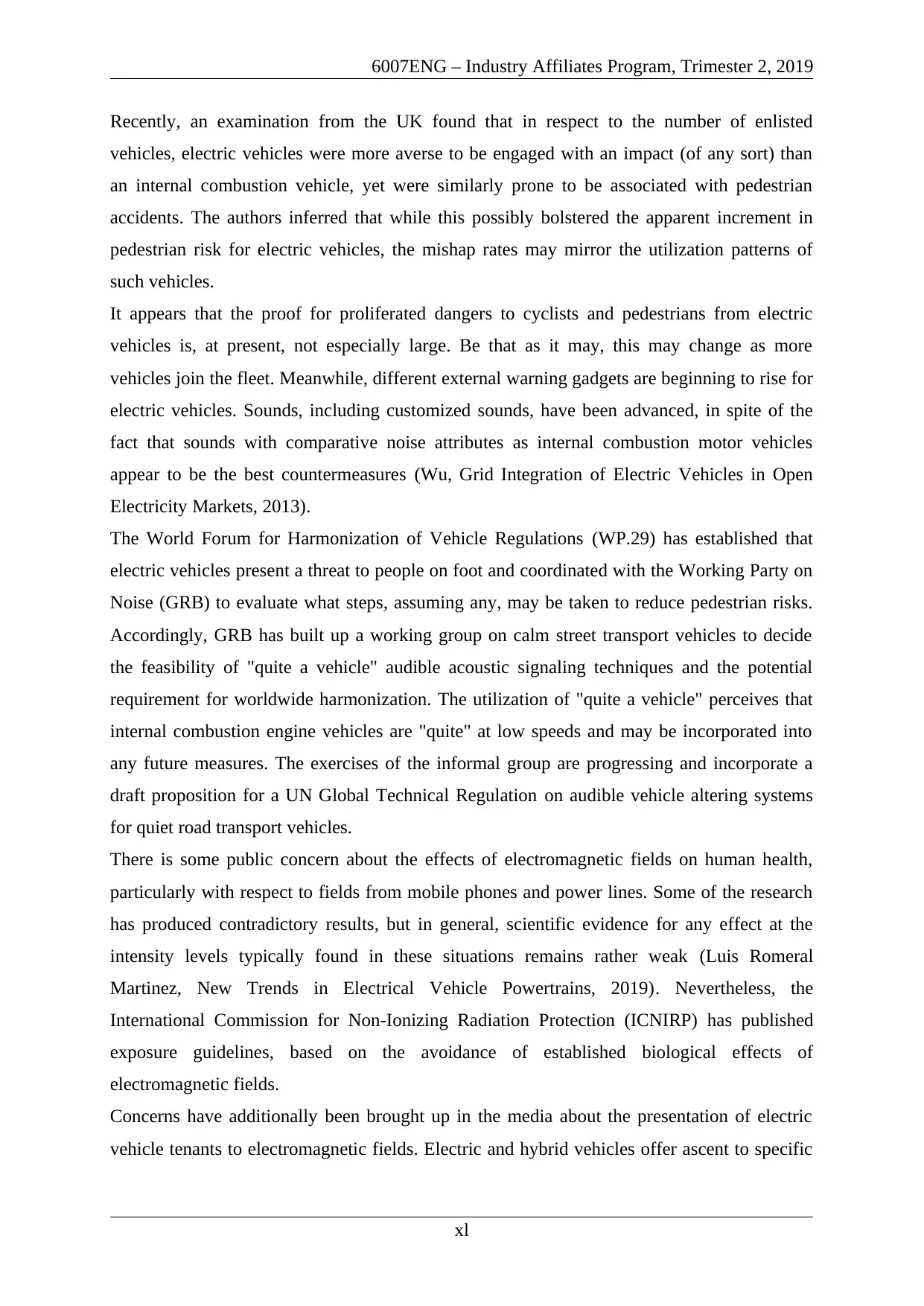
6007ENG – Industry Affiliates Program, Trimester 2, 2019
Recently, an examination from the UK found that in respect to the number of enlisted
vehicles, electric vehicles were more averse to be engaged with an impact (of any sort) than
an internal combustion vehicle, yet were similarly prone to be associated with pedestrian
accidents. The authors inferred that while this possibly bolstered the apparent increment in
pedestrian risk for electric vehicles, the mishap rates may mirror the utilization patterns of
such vehicles.
It appears that the proof for proliferated dangers to cyclists and pedestrians from electric
vehicles is, at present, not especially large. Be that as it may, this may change as more
vehicles join the fleet. Meanwhile, different external warning gadgets are beginning to rise for
electric vehicles. Sounds, including customized sounds, have been advanced, in spite of the
fact that sounds with comparative noise attributes as internal combustion motor vehicles
appear to be the best countermeasures (Wu, Grid Integration of Electric Vehicles in Open
Electricity Markets, 2013).
The World Forum for Harmonization of Vehicle Regulations (WP.29) has established that
electric vehicles present a threat to people on foot and coordinated with the Working Party on
Noise (GRB) to evaluate what steps, assuming any, may be taken to reduce pedestrian risks.
Accordingly, GRB has built up a working group on calm street transport vehicles to decide
the feasibility of "quite a vehicle" audible acoustic signaling techniques and the potential
requirement for worldwide harmonization. The utilization of "quite a vehicle" perceives that
internal combustion engine vehicles are "quite" at low speeds and may be incorporated into
any future measures. The exercises of the informal group are progressing and incorporate a
draft proposition for a UN Global Technical Regulation on audible vehicle altering systems
for quiet road transport vehicles.
There is some public concern about the effects of electromagnetic fields on human health,
particularly with respect to fields from mobile phones and power lines. Some of the research
has produced contradictory results, but in general, scientific evidence for any effect at the
intensity levels typically found in these situations remains rather weak (Luis Romeral
Martinez, New Trends in Electrical Vehicle Powertrains, 2019). Nevertheless, the
International Commission for Non-Ionizing Radiation Protection (ICNIRP) has published
exposure guidelines, based on the avoidance of established biological effects of
electromagnetic fields.
Concerns have additionally been brought up in the media about the presentation of electric
vehicle tenants to electromagnetic fields. Electric and hybrid vehicles offer ascent to specific
xl
Recently, an examination from the UK found that in respect to the number of enlisted
vehicles, electric vehicles were more averse to be engaged with an impact (of any sort) than
an internal combustion vehicle, yet were similarly prone to be associated with pedestrian
accidents. The authors inferred that while this possibly bolstered the apparent increment in
pedestrian risk for electric vehicles, the mishap rates may mirror the utilization patterns of
such vehicles.
It appears that the proof for proliferated dangers to cyclists and pedestrians from electric
vehicles is, at present, not especially large. Be that as it may, this may change as more
vehicles join the fleet. Meanwhile, different external warning gadgets are beginning to rise for
electric vehicles. Sounds, including customized sounds, have been advanced, in spite of the
fact that sounds with comparative noise attributes as internal combustion motor vehicles
appear to be the best countermeasures (Wu, Grid Integration of Electric Vehicles in Open
Electricity Markets, 2013).
The World Forum for Harmonization of Vehicle Regulations (WP.29) has established that
electric vehicles present a threat to people on foot and coordinated with the Working Party on
Noise (GRB) to evaluate what steps, assuming any, may be taken to reduce pedestrian risks.
Accordingly, GRB has built up a working group on calm street transport vehicles to decide
the feasibility of "quite a vehicle" audible acoustic signaling techniques and the potential
requirement for worldwide harmonization. The utilization of "quite a vehicle" perceives that
internal combustion engine vehicles are "quite" at low speeds and may be incorporated into
any future measures. The exercises of the informal group are progressing and incorporate a
draft proposition for a UN Global Technical Regulation on audible vehicle altering systems
for quiet road transport vehicles.
There is some public concern about the effects of electromagnetic fields on human health,
particularly with respect to fields from mobile phones and power lines. Some of the research
has produced contradictory results, but in general, scientific evidence for any effect at the
intensity levels typically found in these situations remains rather weak (Luis Romeral
Martinez, New Trends in Electrical Vehicle Powertrains, 2019). Nevertheless, the
International Commission for Non-Ionizing Radiation Protection (ICNIRP) has published
exposure guidelines, based on the avoidance of established biological effects of
electromagnetic fields.
Concerns have additionally been brought up in the media about the presentation of electric
vehicle tenants to electromagnetic fields. Electric and hybrid vehicles offer ascent to specific
xl
Paraphrase This Document
Need a fresh take? Get an instant paraphrase of this document with our AI Paraphraser
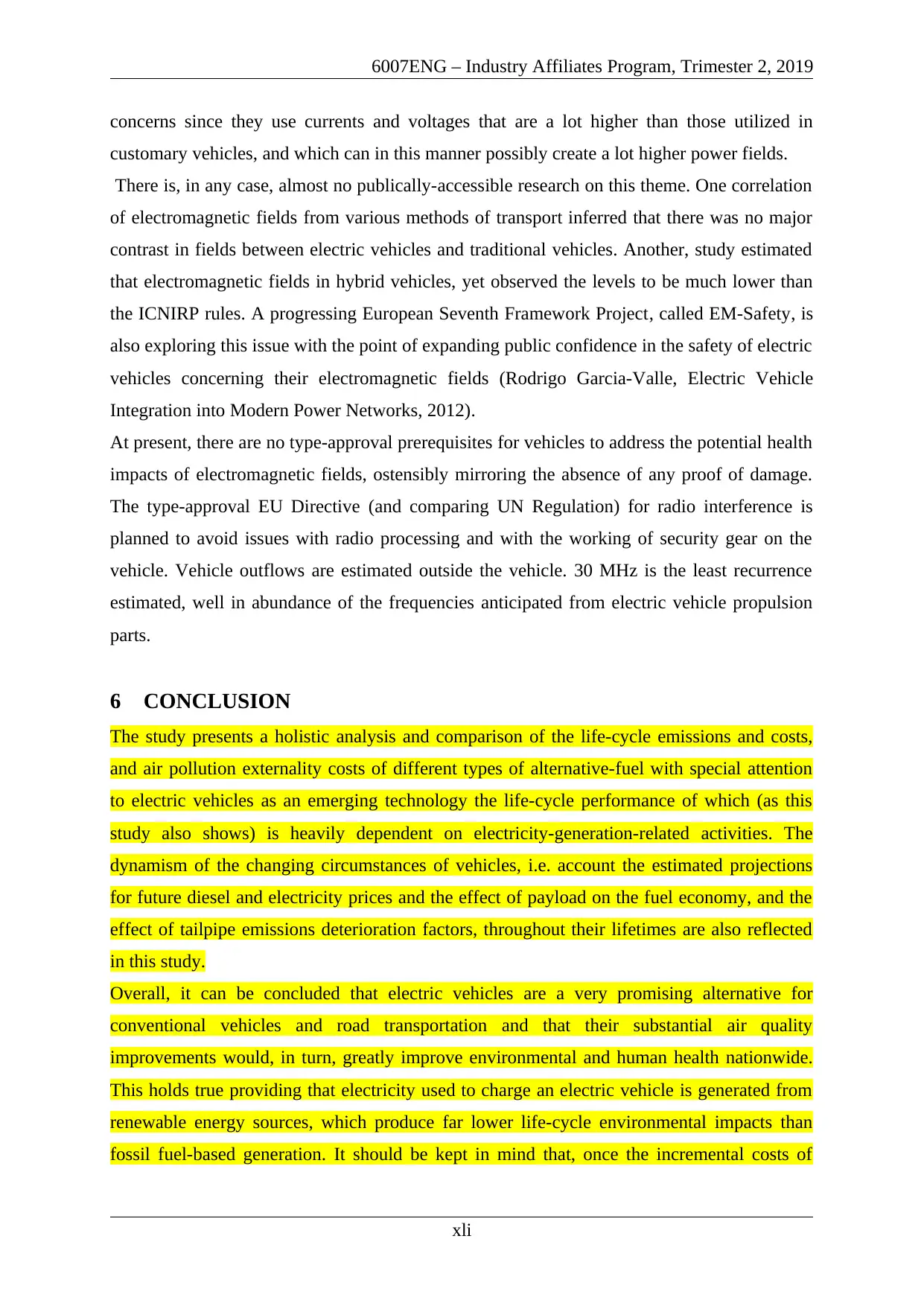
6007ENG – Industry Affiliates Program, Trimester 2, 2019
concerns since they use currents and voltages that are a lot higher than those utilized in
customary vehicles, and which can in this manner possibly create a lot higher power fields.
There is, in any case, almost no publically-accessible research on this theme. One correlation
of electromagnetic fields from various methods of transport inferred that there was no major
contrast in fields between electric vehicles and traditional vehicles. Another, study estimated
that electromagnetic fields in hybrid vehicles, yet observed the levels to be much lower than
the ICNIRP rules. A progressing European Seventh Framework Project, called EM-Safety, is
also exploring this issue with the point of expanding public confidence in the safety of electric
vehicles concerning their electromagnetic fields (Rodrigo Garcia-Valle, Electric Vehicle
Integration into Modern Power Networks, 2012).
At present, there are no type-approval prerequisites for vehicles to address the potential health
impacts of electromagnetic fields, ostensibly mirroring the absence of any proof of damage.
The type-approval EU Directive (and comparing UN Regulation) for radio interference is
planned to avoid issues with radio processing and with the working of security gear on the
vehicle. Vehicle outflows are estimated outside the vehicle. 30 MHz is the least recurrence
estimated, well in abundance of the frequencies anticipated from electric vehicle propulsion
parts.
6 CONCLUSION
The study presents a holistic analysis and comparison of the life-cycle emissions and costs,
and air pollution externality costs of different types of alternative-fuel with special attention
to electric vehicles as an emerging technology the life-cycle performance of which (as this
study also shows) is heavily dependent on electricity-generation-related activities. The
dynamism of the changing circumstances of vehicles, i.e. account the estimated projections
for future diesel and electricity prices and the effect of payload on the fuel economy, and the
effect of tailpipe emissions deterioration factors, throughout their lifetimes are also reflected
in this study.
Overall, it can be concluded that electric vehicles are a very promising alternative for
conventional vehicles and road transportation and that their substantial air quality
improvements would, in turn, greatly improve environmental and human health nationwide.
This holds true providing that electricity used to charge an electric vehicle is generated from
renewable energy sources, which produce far lower life-cycle environmental impacts than
fossil fuel-based generation. It should be kept in mind that, once the incremental costs of
xli
concerns since they use currents and voltages that are a lot higher than those utilized in
customary vehicles, and which can in this manner possibly create a lot higher power fields.
There is, in any case, almost no publically-accessible research on this theme. One correlation
of electromagnetic fields from various methods of transport inferred that there was no major
contrast in fields between electric vehicles and traditional vehicles. Another, study estimated
that electromagnetic fields in hybrid vehicles, yet observed the levels to be much lower than
the ICNIRP rules. A progressing European Seventh Framework Project, called EM-Safety, is
also exploring this issue with the point of expanding public confidence in the safety of electric
vehicles concerning their electromagnetic fields (Rodrigo Garcia-Valle, Electric Vehicle
Integration into Modern Power Networks, 2012).
At present, there are no type-approval prerequisites for vehicles to address the potential health
impacts of electromagnetic fields, ostensibly mirroring the absence of any proof of damage.
The type-approval EU Directive (and comparing UN Regulation) for radio interference is
planned to avoid issues with radio processing and with the working of security gear on the
vehicle. Vehicle outflows are estimated outside the vehicle. 30 MHz is the least recurrence
estimated, well in abundance of the frequencies anticipated from electric vehicle propulsion
parts.
6 CONCLUSION
The study presents a holistic analysis and comparison of the life-cycle emissions and costs,
and air pollution externality costs of different types of alternative-fuel with special attention
to electric vehicles as an emerging technology the life-cycle performance of which (as this
study also shows) is heavily dependent on electricity-generation-related activities. The
dynamism of the changing circumstances of vehicles, i.e. account the estimated projections
for future diesel and electricity prices and the effect of payload on the fuel economy, and the
effect of tailpipe emissions deterioration factors, throughout their lifetimes are also reflected
in this study.
Overall, it can be concluded that electric vehicles are a very promising alternative for
conventional vehicles and road transportation and that their substantial air quality
improvements would, in turn, greatly improve environmental and human health nationwide.
This holds true providing that electricity used to charge an electric vehicle is generated from
renewable energy sources, which produce far lower life-cycle environmental impacts than
fossil fuel-based generation. It should be kept in mind that, once the incremental costs of
xli
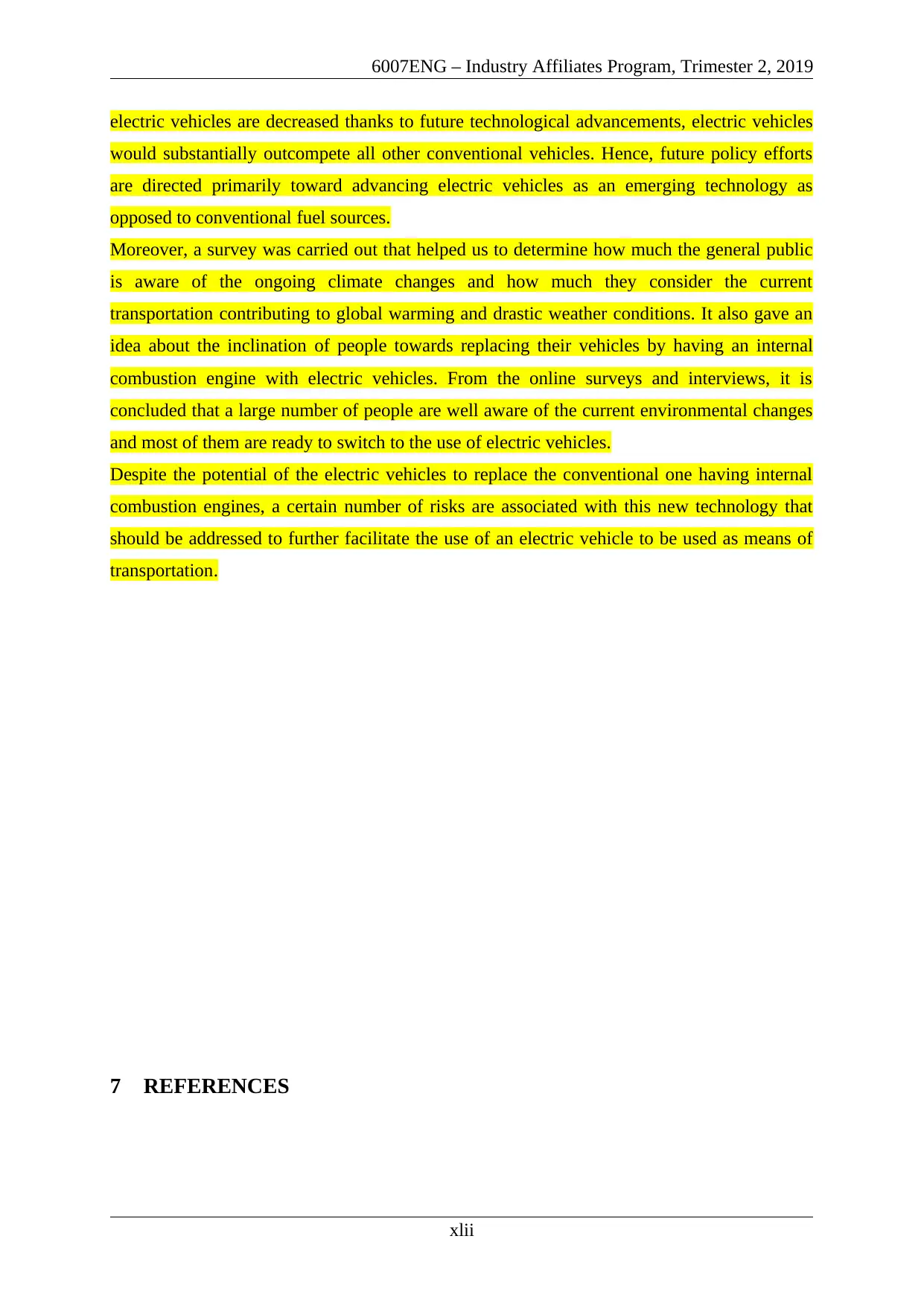
6007ENG – Industry Affiliates Program, Trimester 2, 2019
electric vehicles are decreased thanks to future technological advancements, electric vehicles
would substantially outcompete all other conventional vehicles. Hence, future policy efforts
are directed primarily toward advancing electric vehicles as an emerging technology as
opposed to conventional fuel sources.
Moreover, a survey was carried out that helped us to determine how much the general public
is aware of the ongoing climate changes and how much they consider the current
transportation contributing to global warming and drastic weather conditions. It also gave an
idea about the inclination of people towards replacing their vehicles by having an internal
combustion engine with electric vehicles. From the online surveys and interviews, it is
concluded that a large number of people are well aware of the current environmental changes
and most of them are ready to switch to the use of electric vehicles.
Despite the potential of the electric vehicles to replace the conventional one having internal
combustion engines, a certain number of risks are associated with this new technology that
should be addressed to further facilitate the use of an electric vehicle to be used as means of
transportation.
7 REFERENCES
xlii
electric vehicles are decreased thanks to future technological advancements, electric vehicles
would substantially outcompete all other conventional vehicles. Hence, future policy efforts
are directed primarily toward advancing electric vehicles as an emerging technology as
opposed to conventional fuel sources.
Moreover, a survey was carried out that helped us to determine how much the general public
is aware of the ongoing climate changes and how much they consider the current
transportation contributing to global warming and drastic weather conditions. It also gave an
idea about the inclination of people towards replacing their vehicles by having an internal
combustion engine with electric vehicles. From the online surveys and interviews, it is
concluded that a large number of people are well aware of the current environmental changes
and most of them are ready to switch to the use of electric vehicles.
Despite the potential of the electric vehicles to replace the conventional one having internal
combustion engines, a certain number of risks are associated with this new technology that
should be addressed to further facilitate the use of an electric vehicle to be used as means of
transportation.
7 REFERENCES
xlii
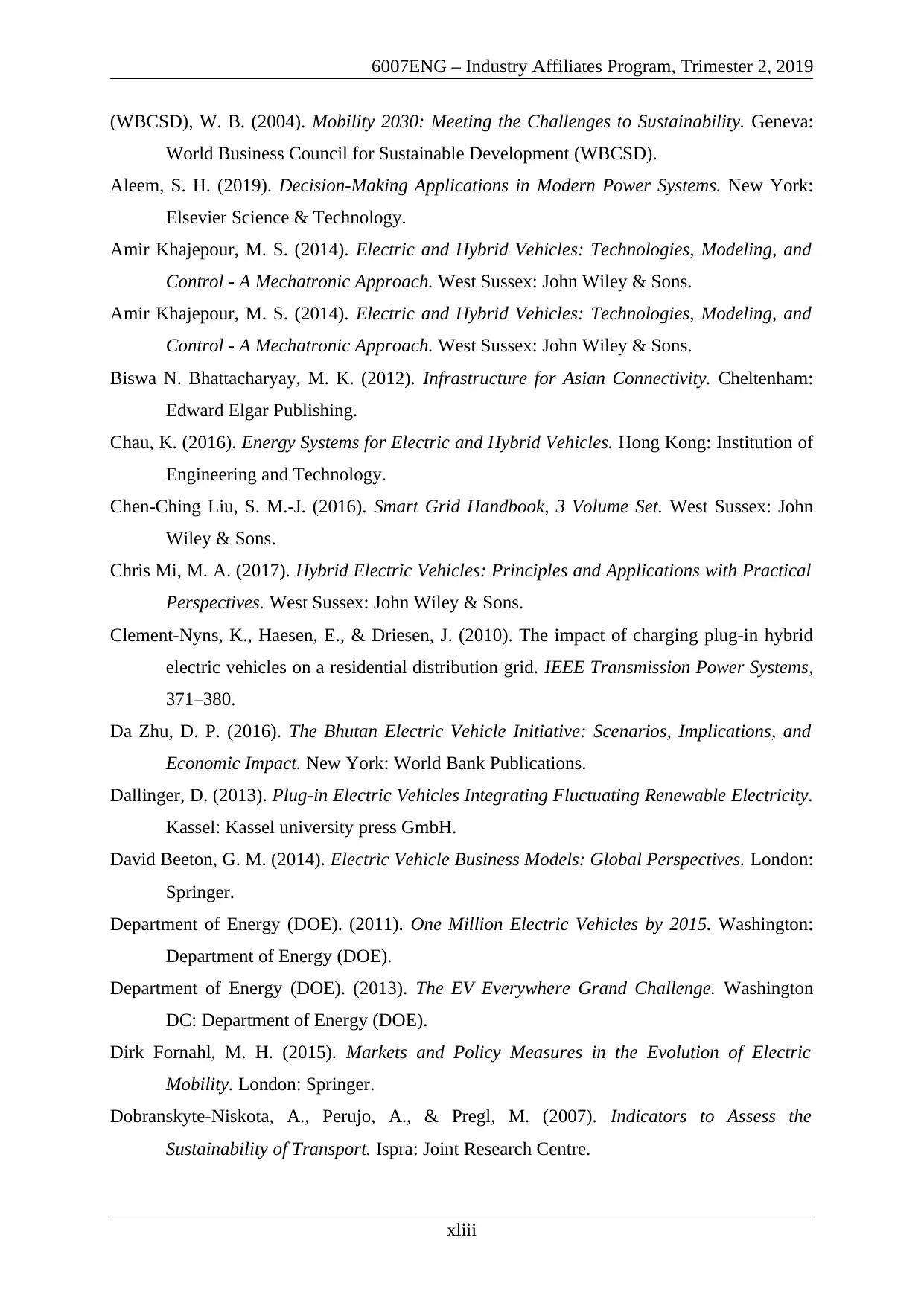
6007ENG – Industry Affiliates Program, Trimester 2, 2019
(WBCSD), W. B. (2004). Mobility 2030: Meeting the Challenges to Sustainability. Geneva:
World Business Council for Sustainable Development (WBCSD).
Aleem, S. H. (2019). Decision-Making Applications in Modern Power Systems. New York:
Elsevier Science & Technology.
Amir Khajepour, M. S. (2014). Electric and Hybrid Vehicles: Technologies, Modeling, and
Control - A Mechatronic Approach. West Sussex: John Wiley & Sons.
Amir Khajepour, M. S. (2014). Electric and Hybrid Vehicles: Technologies, Modeling, and
Control - A Mechatronic Approach. West Sussex: John Wiley & Sons.
Biswa N. Bhattacharyay, M. K. (2012). Infrastructure for Asian Connectivity. Cheltenham:
Edward Elgar Publishing.
Chau, K. (2016). Energy Systems for Electric and Hybrid Vehicles. Hong Kong: Institution of
Engineering and Technology.
Chen-Ching Liu, S. M.-J. (2016). Smart Grid Handbook, 3 Volume Set. West Sussex: John
Wiley & Sons.
Chris Mi, M. A. (2017). Hybrid Electric Vehicles: Principles and Applications with Practical
Perspectives. West Sussex: John Wiley & Sons.
Clement-Nyns, K., Haesen, E., & Driesen, J. (2010). The impact of charging plug-in hybrid
electric vehicles on a residential distribution grid. IEEE Transmission Power Systems,
371–380.
Da Zhu, D. P. (2016). The Bhutan Electric Vehicle Initiative: Scenarios, Implications, and
Economic Impact. New York: World Bank Publications.
Dallinger, D. (2013). Plug-in Electric Vehicles Integrating Fluctuating Renewable Electricity.
Kassel: Kassel university press GmbH.
David Beeton, G. M. (2014). Electric Vehicle Business Models: Global Perspectives. London:
Springer.
Department of Energy (DOE). (2011). One Million Electric Vehicles by 2015. Washington:
Department of Energy (DOE).
Department of Energy (DOE). (2013). The EV Everywhere Grand Challenge. Washington
DC: Department of Energy (DOE).
Dirk Fornahl, M. H. (2015). Markets and Policy Measures in the Evolution of Electric
Mobility. London: Springer.
Dobranskyte-Niskota, A., Perujo, A., & Pregl, M. (2007). Indicators to Assess the
Sustainability of Transport. Ispra: Joint Research Centre.
xliii
(WBCSD), W. B. (2004). Mobility 2030: Meeting the Challenges to Sustainability. Geneva:
World Business Council for Sustainable Development (WBCSD).
Aleem, S. H. (2019). Decision-Making Applications in Modern Power Systems. New York:
Elsevier Science & Technology.
Amir Khajepour, M. S. (2014). Electric and Hybrid Vehicles: Technologies, Modeling, and
Control - A Mechatronic Approach. West Sussex: John Wiley & Sons.
Amir Khajepour, M. S. (2014). Electric and Hybrid Vehicles: Technologies, Modeling, and
Control - A Mechatronic Approach. West Sussex: John Wiley & Sons.
Biswa N. Bhattacharyay, M. K. (2012). Infrastructure for Asian Connectivity. Cheltenham:
Edward Elgar Publishing.
Chau, K. (2016). Energy Systems for Electric and Hybrid Vehicles. Hong Kong: Institution of
Engineering and Technology.
Chen-Ching Liu, S. M.-J. (2016). Smart Grid Handbook, 3 Volume Set. West Sussex: John
Wiley & Sons.
Chris Mi, M. A. (2017). Hybrid Electric Vehicles: Principles and Applications with Practical
Perspectives. West Sussex: John Wiley & Sons.
Clement-Nyns, K., Haesen, E., & Driesen, J. (2010). The impact of charging plug-in hybrid
electric vehicles on a residential distribution grid. IEEE Transmission Power Systems,
371–380.
Da Zhu, D. P. (2016). The Bhutan Electric Vehicle Initiative: Scenarios, Implications, and
Economic Impact. New York: World Bank Publications.
Dallinger, D. (2013). Plug-in Electric Vehicles Integrating Fluctuating Renewable Electricity.
Kassel: Kassel university press GmbH.
David Beeton, G. M. (2014). Electric Vehicle Business Models: Global Perspectives. London:
Springer.
Department of Energy (DOE). (2011). One Million Electric Vehicles by 2015. Washington:
Department of Energy (DOE).
Department of Energy (DOE). (2013). The EV Everywhere Grand Challenge. Washington
DC: Department of Energy (DOE).
Dirk Fornahl, M. H. (2015). Markets and Policy Measures in the Evolution of Electric
Mobility. London: Springer.
Dobranskyte-Niskota, A., Perujo, A., & Pregl, M. (2007). Indicators to Assess the
Sustainability of Transport. Ispra: Joint Research Centre.
xliii
Secure Best Marks with AI Grader
Need help grading? Try our AI Grader for instant feedback on your assignments.
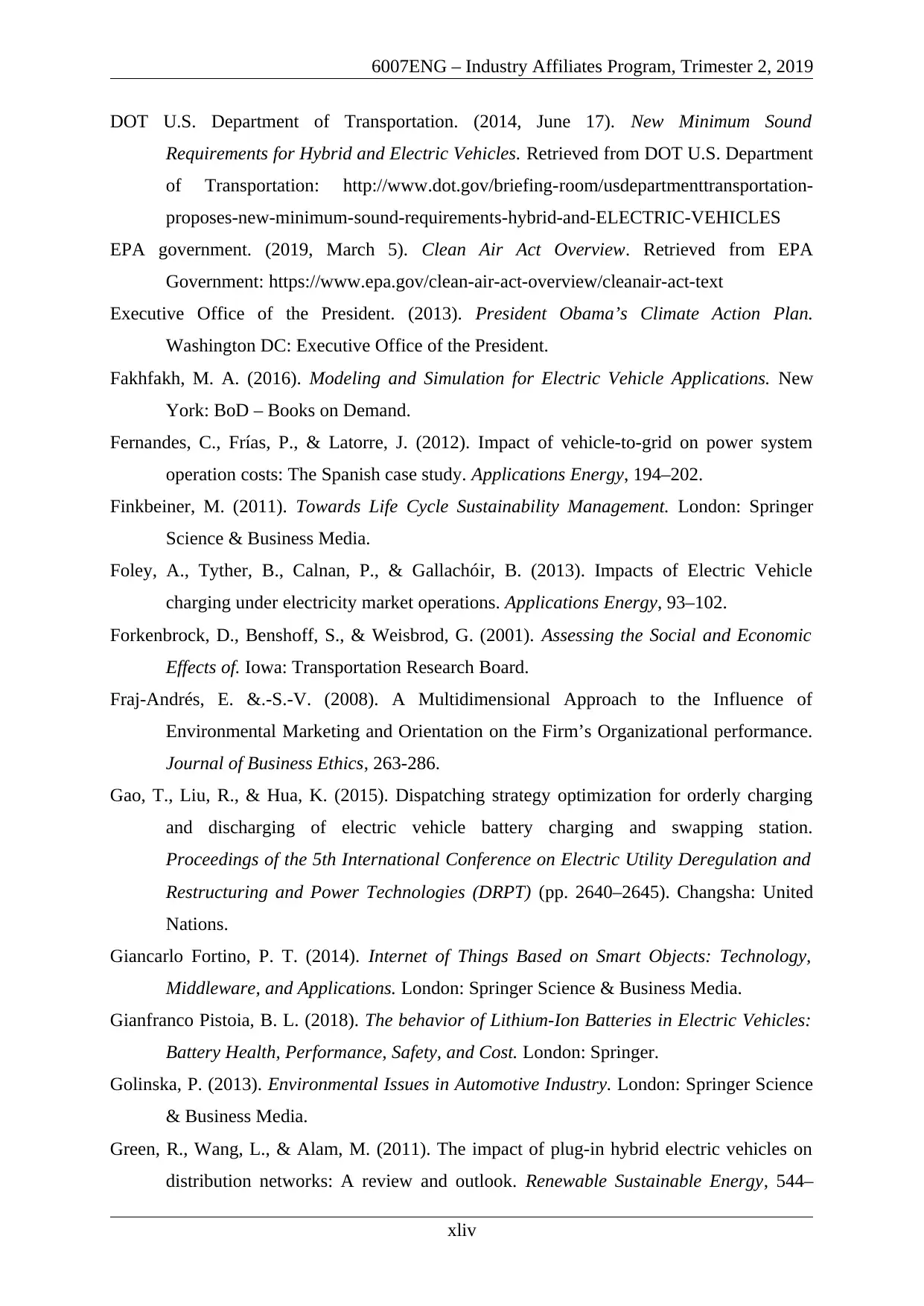
6007ENG – Industry Affiliates Program, Trimester 2, 2019
DOT U.S. Department of Transportation. (2014, June 17). New Minimum Sound
Requirements for Hybrid and Electric Vehicles. Retrieved from DOT U.S. Department
of Transportation: http://www.dot.gov/briefing-room/usdepartmenttransportation-
proposes-new-minimum-sound-requirements-hybrid-and-ELECTRIC-VEHICLES
EPA government. (2019, March 5). Clean Air Act Overview. Retrieved from EPA
Government: https://www.epa.gov/clean-air-act-overview/cleanair-act-text
Executive Office of the President. (2013). President Obama’s Climate Action Plan.
Washington DC: Executive Office of the President.
Fakhfakh, M. A. (2016). Modeling and Simulation for Electric Vehicle Applications. New
York: BoD – Books on Demand.
Fernandes, C., Frías, P., & Latorre, J. (2012). Impact of vehicle-to-grid on power system
operation costs: The Spanish case study. Applications Energy, 194–202.
Finkbeiner, M. (2011). Towards Life Cycle Sustainability Management. London: Springer
Science & Business Media.
Foley, A., Tyther, B., Calnan, P., & Gallachóir, B. (2013). Impacts of Electric Vehicle
charging under electricity market operations. Applications Energy, 93–102.
Forkenbrock, D., Benshoff, S., & Weisbrod, G. (2001). Assessing the Social and Economic
Effects of. Iowa: Transportation Research Board.
Fraj-Andrés, E. &.-S.-V. (2008). A Multidimensional Approach to the Influence of
Environmental Marketing and Orientation on the Firm’s Organizational performance.
Journal of Business Ethics, 263-286.
Gao, T., Liu, R., & Hua, K. (2015). Dispatching strategy optimization for orderly charging
and discharging of electric vehicle battery charging and swapping station.
Proceedings of the 5th International Conference on Electric Utility Deregulation and
Restructuring and Power Technologies (DRPT) (pp. 2640–2645). Changsha: United
Nations.
Giancarlo Fortino, P. T. (2014). Internet of Things Based on Smart Objects: Technology,
Middleware, and Applications. London: Springer Science & Business Media.
Gianfranco Pistoia, B. L. (2018). The behavior of Lithium-Ion Batteries in Electric Vehicles:
Battery Health, Performance, Safety, and Cost. London: Springer.
Golinska, P. (2013). Environmental Issues in Automotive Industry. London: Springer Science
& Business Media.
Green, R., Wang, L., & Alam, M. (2011). The impact of plug-in hybrid electric vehicles on
distribution networks: A review and outlook. Renewable Sustainable Energy, 544–
xliv
DOT U.S. Department of Transportation. (2014, June 17). New Minimum Sound
Requirements for Hybrid and Electric Vehicles. Retrieved from DOT U.S. Department
of Transportation: http://www.dot.gov/briefing-room/usdepartmenttransportation-
proposes-new-minimum-sound-requirements-hybrid-and-ELECTRIC-VEHICLES
EPA government. (2019, March 5). Clean Air Act Overview. Retrieved from EPA
Government: https://www.epa.gov/clean-air-act-overview/cleanair-act-text
Executive Office of the President. (2013). President Obama’s Climate Action Plan.
Washington DC: Executive Office of the President.
Fakhfakh, M. A. (2016). Modeling and Simulation for Electric Vehicle Applications. New
York: BoD – Books on Demand.
Fernandes, C., Frías, P., & Latorre, J. (2012). Impact of vehicle-to-grid on power system
operation costs: The Spanish case study. Applications Energy, 194–202.
Finkbeiner, M. (2011). Towards Life Cycle Sustainability Management. London: Springer
Science & Business Media.
Foley, A., Tyther, B., Calnan, P., & Gallachóir, B. (2013). Impacts of Electric Vehicle
charging under electricity market operations. Applications Energy, 93–102.
Forkenbrock, D., Benshoff, S., & Weisbrod, G. (2001). Assessing the Social and Economic
Effects of. Iowa: Transportation Research Board.
Fraj-Andrés, E. &.-S.-V. (2008). A Multidimensional Approach to the Influence of
Environmental Marketing and Orientation on the Firm’s Organizational performance.
Journal of Business Ethics, 263-286.
Gao, T., Liu, R., & Hua, K. (2015). Dispatching strategy optimization for orderly charging
and discharging of electric vehicle battery charging and swapping station.
Proceedings of the 5th International Conference on Electric Utility Deregulation and
Restructuring and Power Technologies (DRPT) (pp. 2640–2645). Changsha: United
Nations.
Giancarlo Fortino, P. T. (2014). Internet of Things Based on Smart Objects: Technology,
Middleware, and Applications. London: Springer Science & Business Media.
Gianfranco Pistoia, B. L. (2018). The behavior of Lithium-Ion Batteries in Electric Vehicles:
Battery Health, Performance, Safety, and Cost. London: Springer.
Golinska, P. (2013). Environmental Issues in Automotive Industry. London: Springer Science
& Business Media.
Green, R., Wang, L., & Alam, M. (2011). The impact of plug-in hybrid electric vehicles on
distribution networks: A review and outlook. Renewable Sustainable Energy, 544–
xliv
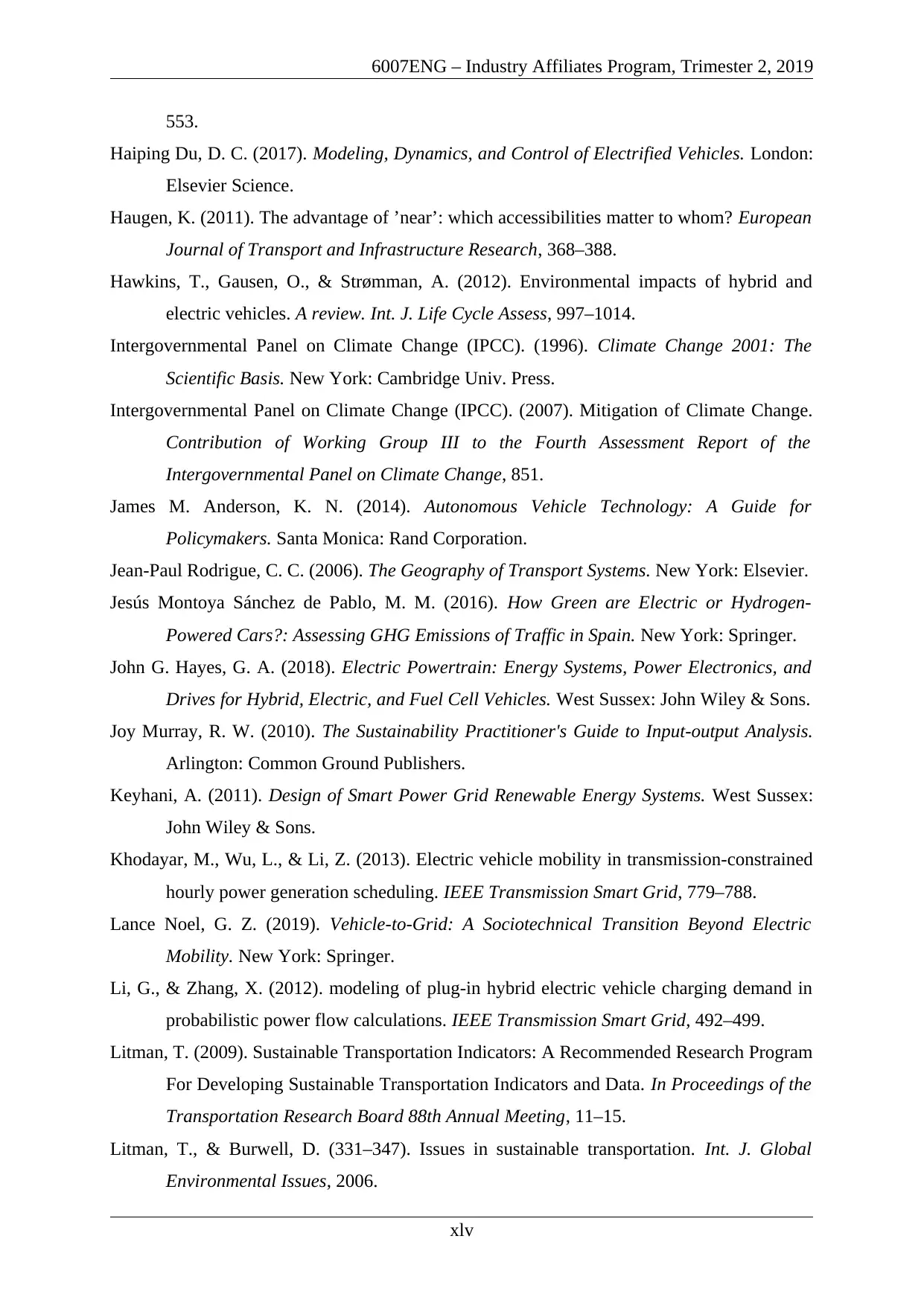
6007ENG – Industry Affiliates Program, Trimester 2, 2019
553.
Haiping Du, D. C. (2017). Modeling, Dynamics, and Control of Electrified Vehicles. London:
Elsevier Science.
Haugen, K. (2011). The advantage of ’near’: which accessibilities matter to whom? European
Journal of Transport and Infrastructure Research, 368–388.
Hawkins, T., Gausen, O., & Strømman, A. (2012). Environmental impacts of hybrid and
electric vehicles. A review. Int. J. Life Cycle Assess, 997–1014.
Intergovernmental Panel on Climate Change (IPCC). (1996). Climate Change 2001: The
Scientific Basis. New York: Cambridge Univ. Press.
Intergovernmental Panel on Climate Change (IPCC). (2007). Mitigation of Climate Change.
Contribution of Working Group III to the Fourth Assessment Report of the
Intergovernmental Panel on Climate Change, 851.
James M. Anderson, K. N. (2014). Autonomous Vehicle Technology: A Guide for
Policymakers. Santa Monica: Rand Corporation.
Jean-Paul Rodrigue, C. C. (2006). The Geography of Transport Systems. New York: Elsevier.
Jesús Montoya Sánchez de Pablo, M. M. (2016). How Green are Electric or Hydrogen-
Powered Cars?: Assessing GHG Emissions of Traffic in Spain. New York: Springer.
John G. Hayes, G. A. (2018). Electric Powertrain: Energy Systems, Power Electronics, and
Drives for Hybrid, Electric, and Fuel Cell Vehicles. West Sussex: John Wiley & Sons.
Joy Murray, R. W. (2010). The Sustainability Practitioner's Guide to Input-output Analysis.
Arlington: Common Ground Publishers.
Keyhani, A. (2011). Design of Smart Power Grid Renewable Energy Systems. West Sussex:
John Wiley & Sons.
Khodayar, M., Wu, L., & Li, Z. (2013). Electric vehicle mobility in transmission-constrained
hourly power generation scheduling. IEEE Transmission Smart Grid, 779–788.
Lance Noel, G. Z. (2019). Vehicle-to-Grid: A Sociotechnical Transition Beyond Electric
Mobility. New York: Springer.
Li, G., & Zhang, X. (2012). modeling of plug-in hybrid electric vehicle charging demand in
probabilistic power flow calculations. IEEE Transmission Smart Grid, 492–499.
Litman, T. (2009). Sustainable Transportation Indicators: A Recommended Research Program
For Developing Sustainable Transportation Indicators and Data. In Proceedings of the
Transportation Research Board 88th Annual Meeting, 11–15.
Litman, T., & Burwell, D. (331–347). Issues in sustainable transportation. Int. J. Global
Environmental Issues, 2006.
xlv
553.
Haiping Du, D. C. (2017). Modeling, Dynamics, and Control of Electrified Vehicles. London:
Elsevier Science.
Haugen, K. (2011). The advantage of ’near’: which accessibilities matter to whom? European
Journal of Transport and Infrastructure Research, 368–388.
Hawkins, T., Gausen, O., & Strømman, A. (2012). Environmental impacts of hybrid and
electric vehicles. A review. Int. J. Life Cycle Assess, 997–1014.
Intergovernmental Panel on Climate Change (IPCC). (1996). Climate Change 2001: The
Scientific Basis. New York: Cambridge Univ. Press.
Intergovernmental Panel on Climate Change (IPCC). (2007). Mitigation of Climate Change.
Contribution of Working Group III to the Fourth Assessment Report of the
Intergovernmental Panel on Climate Change, 851.
James M. Anderson, K. N. (2014). Autonomous Vehicle Technology: A Guide for
Policymakers. Santa Monica: Rand Corporation.
Jean-Paul Rodrigue, C. C. (2006). The Geography of Transport Systems. New York: Elsevier.
Jesús Montoya Sánchez de Pablo, M. M. (2016). How Green are Electric or Hydrogen-
Powered Cars?: Assessing GHG Emissions of Traffic in Spain. New York: Springer.
John G. Hayes, G. A. (2018). Electric Powertrain: Energy Systems, Power Electronics, and
Drives for Hybrid, Electric, and Fuel Cell Vehicles. West Sussex: John Wiley & Sons.
Joy Murray, R. W. (2010). The Sustainability Practitioner's Guide to Input-output Analysis.
Arlington: Common Ground Publishers.
Keyhani, A. (2011). Design of Smart Power Grid Renewable Energy Systems. West Sussex:
John Wiley & Sons.
Khodayar, M., Wu, L., & Li, Z. (2013). Electric vehicle mobility in transmission-constrained
hourly power generation scheduling. IEEE Transmission Smart Grid, 779–788.
Lance Noel, G. Z. (2019). Vehicle-to-Grid: A Sociotechnical Transition Beyond Electric
Mobility. New York: Springer.
Li, G., & Zhang, X. (2012). modeling of plug-in hybrid electric vehicle charging demand in
probabilistic power flow calculations. IEEE Transmission Smart Grid, 492–499.
Litman, T. (2009). Sustainable Transportation Indicators: A Recommended Research Program
For Developing Sustainable Transportation Indicators and Data. In Proceedings of the
Transportation Research Board 88th Annual Meeting, 11–15.
Litman, T., & Burwell, D. (331–347). Issues in sustainable transportation. Int. J. Global
Environmental Issues, 2006.
xlv
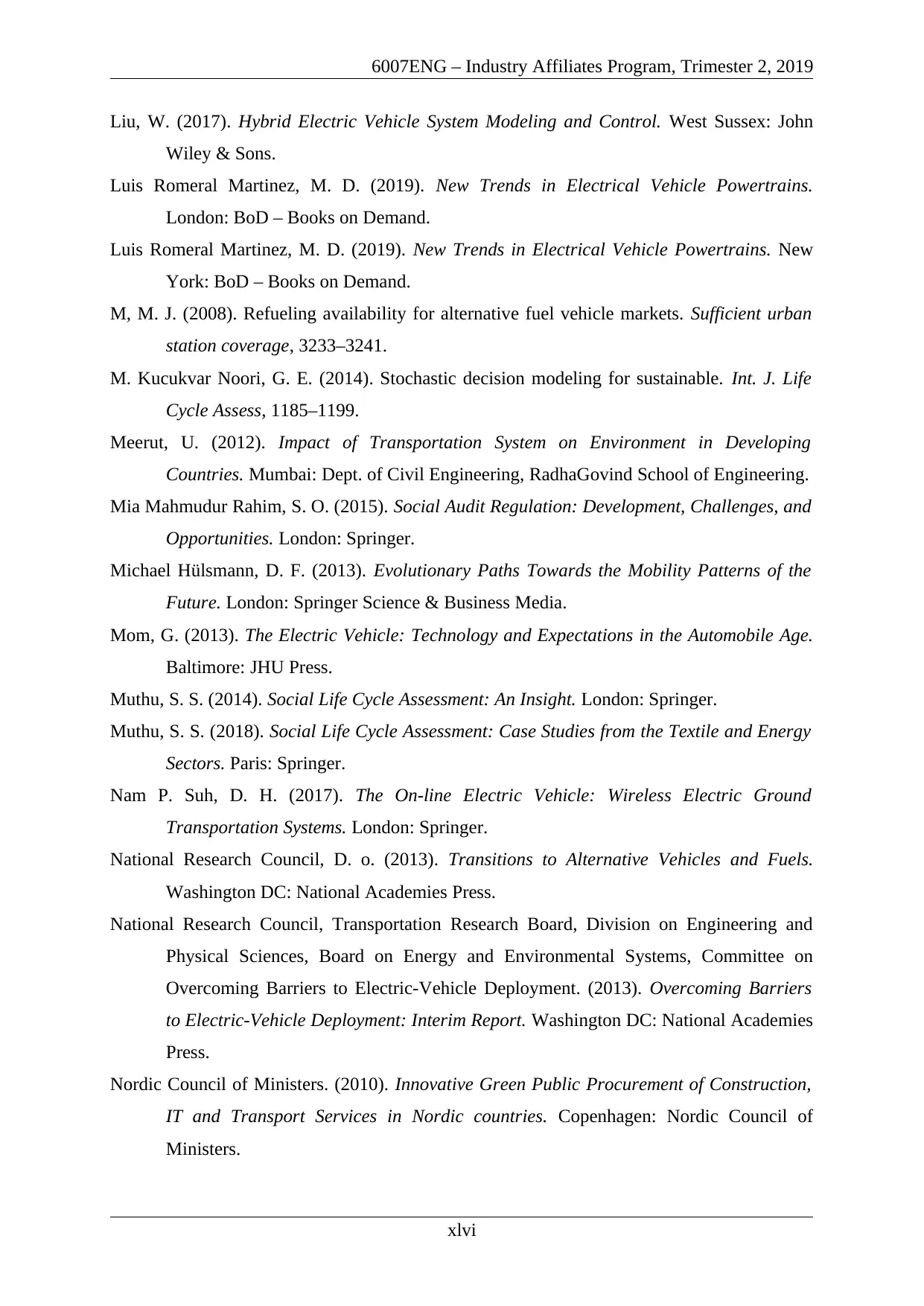
6007ENG – Industry Affiliates Program, Trimester 2, 2019
Liu, W. (2017). Hybrid Electric Vehicle System Modeling and Control. West Sussex: John
Wiley & Sons.
Luis Romeral Martinez, M. D. (2019). New Trends in Electrical Vehicle Powertrains.
London: BoD – Books on Demand.
Luis Romeral Martinez, M. D. (2019). New Trends in Electrical Vehicle Powertrains. New
York: BoD – Books on Demand.
M, M. J. (2008). Refueling availability for alternative fuel vehicle markets. Sufficient urban
station coverage, 3233–3241.
M. Kucukvar Noori, G. E. (2014). Stochastic decision modeling for sustainable. Int. J. Life
Cycle Assess, 1185–1199.
Meerut, U. (2012). Impact of Transportation System on Environment in Developing
Countries. Mumbai: Dept. of Civil Engineering, RadhaGovind School of Engineering.
Mia Mahmudur Rahim, S. O. (2015). Social Audit Regulation: Development, Challenges, and
Opportunities. London: Springer.
Michael Hülsmann, D. F. (2013). Evolutionary Paths Towards the Mobility Patterns of the
Future. London: Springer Science & Business Media.
Mom, G. (2013). The Electric Vehicle: Technology and Expectations in the Automobile Age.
Baltimore: JHU Press.
Muthu, S. S. (2014). Social Life Cycle Assessment: An Insight. London: Springer.
Muthu, S. S. (2018). Social Life Cycle Assessment: Case Studies from the Textile and Energy
Sectors. Paris: Springer.
Nam P. Suh, D. H. (2017). The On-line Electric Vehicle: Wireless Electric Ground
Transportation Systems. London: Springer.
National Research Council, D. o. (2013). Transitions to Alternative Vehicles and Fuels.
Washington DC: National Academies Press.
National Research Council, Transportation Research Board, Division on Engineering and
Physical Sciences, Board on Energy and Environmental Systems, Committee on
Overcoming Barriers to Electric-Vehicle Deployment. (2013). Overcoming Barriers
to Electric-Vehicle Deployment: Interim Report. Washington DC: National Academies
Press.
Nordic Council of Ministers. (2010). Innovative Green Public Procurement of Construction,
IT and Transport Services in Nordic countries. Copenhagen: Nordic Council of
Ministers.
xlvi
Liu, W. (2017). Hybrid Electric Vehicle System Modeling and Control. West Sussex: John
Wiley & Sons.
Luis Romeral Martinez, M. D. (2019). New Trends in Electrical Vehicle Powertrains.
London: BoD – Books on Demand.
Luis Romeral Martinez, M. D. (2019). New Trends in Electrical Vehicle Powertrains. New
York: BoD – Books on Demand.
M, M. J. (2008). Refueling availability for alternative fuel vehicle markets. Sufficient urban
station coverage, 3233–3241.
M. Kucukvar Noori, G. E. (2014). Stochastic decision modeling for sustainable. Int. J. Life
Cycle Assess, 1185–1199.
Meerut, U. (2012). Impact of Transportation System on Environment in Developing
Countries. Mumbai: Dept. of Civil Engineering, RadhaGovind School of Engineering.
Mia Mahmudur Rahim, S. O. (2015). Social Audit Regulation: Development, Challenges, and
Opportunities. London: Springer.
Michael Hülsmann, D. F. (2013). Evolutionary Paths Towards the Mobility Patterns of the
Future. London: Springer Science & Business Media.
Mom, G. (2013). The Electric Vehicle: Technology and Expectations in the Automobile Age.
Baltimore: JHU Press.
Muthu, S. S. (2014). Social Life Cycle Assessment: An Insight. London: Springer.
Muthu, S. S. (2018). Social Life Cycle Assessment: Case Studies from the Textile and Energy
Sectors. Paris: Springer.
Nam P. Suh, D. H. (2017). The On-line Electric Vehicle: Wireless Electric Ground
Transportation Systems. London: Springer.
National Research Council, D. o. (2013). Transitions to Alternative Vehicles and Fuels.
Washington DC: National Academies Press.
National Research Council, Transportation Research Board, Division on Engineering and
Physical Sciences, Board on Energy and Environmental Systems, Committee on
Overcoming Barriers to Electric-Vehicle Deployment. (2013). Overcoming Barriers
to Electric-Vehicle Deployment: Interim Report. Washington DC: National Academies
Press.
Nordic Council of Ministers. (2010). Innovative Green Public Procurement of Construction,
IT and Transport Services in Nordic countries. Copenhagen: Nordic Council of
Ministers.
xlvi
Paraphrase This Document
Need a fresh take? Get an instant paraphrase of this document with our AI Paraphraser
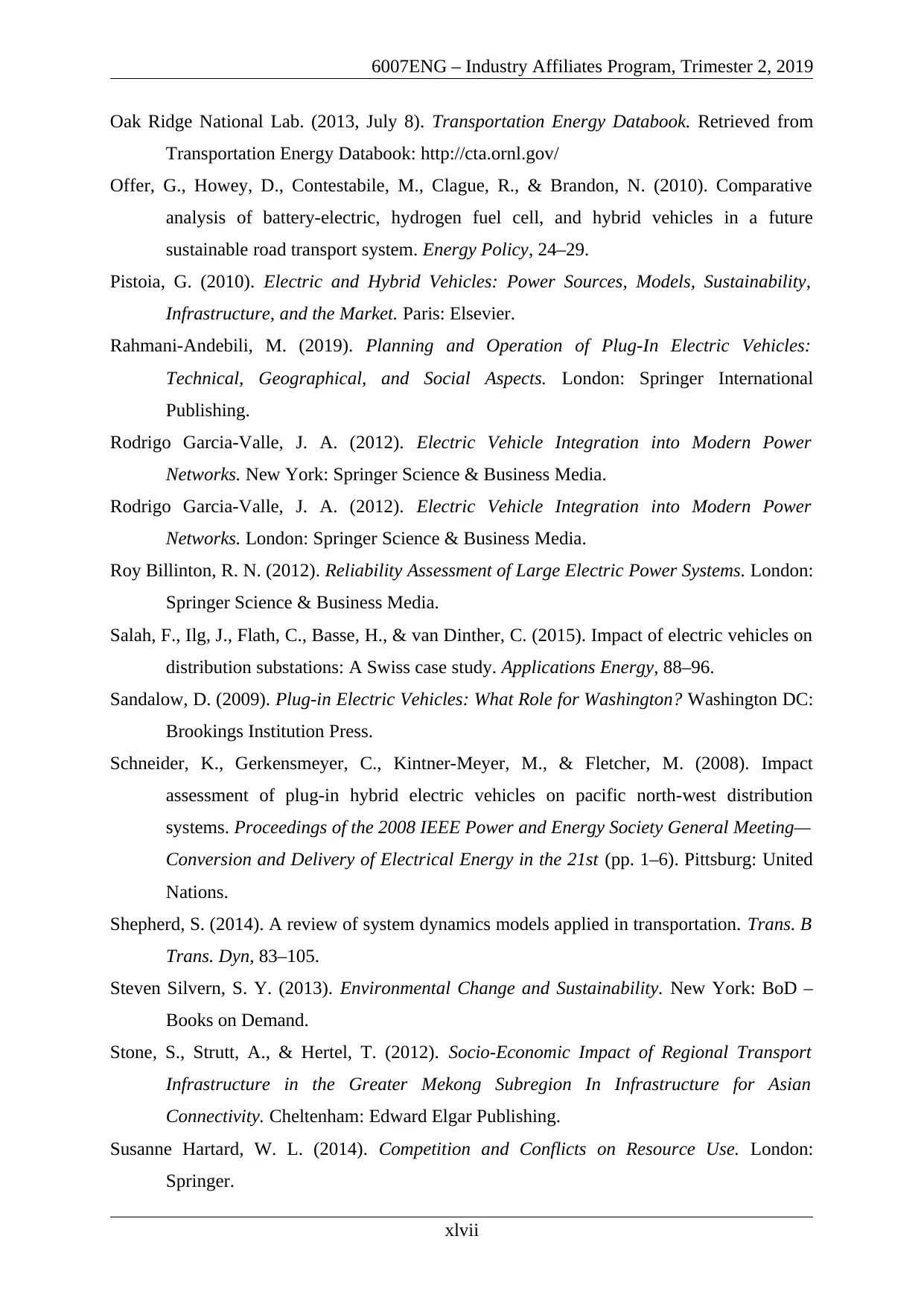
6007ENG – Industry Affiliates Program, Trimester 2, 2019
Oak Ridge National Lab. (2013, July 8). Transportation Energy Databook. Retrieved from
Transportation Energy Databook: http://cta.ornl.gov/
Offer, G., Howey, D., Contestabile, M., Clague, R., & Brandon, N. (2010). Comparative
analysis of battery-electric, hydrogen fuel cell, and hybrid vehicles in a future
sustainable road transport system. Energy Policy, 24–29.
Pistoia, G. (2010). Electric and Hybrid Vehicles: Power Sources, Models, Sustainability,
Infrastructure, and the Market. Paris: Elsevier.
Rahmani-Andebili, M. (2019). Planning and Operation of Plug-In Electric Vehicles:
Technical, Geographical, and Social Aspects. London: Springer International
Publishing.
Rodrigo Garcia-Valle, J. A. (2012). Electric Vehicle Integration into Modern Power
Networks. New York: Springer Science & Business Media.
Rodrigo Garcia-Valle, J. A. (2012). Electric Vehicle Integration into Modern Power
Networks. London: Springer Science & Business Media.
Roy Billinton, R. N. (2012). Reliability Assessment of Large Electric Power Systems. London:
Springer Science & Business Media.
Salah, F., Ilg, J., Flath, C., Basse, H., & van Dinther, C. (2015). Impact of electric vehicles on
distribution substations: A Swiss case study. Applications Energy, 88–96.
Sandalow, D. (2009). Plug-in Electric Vehicles: What Role for Washington? Washington DC:
Brookings Institution Press.
Schneider, K., Gerkensmeyer, C., Kintner-Meyer, M., & Fletcher, M. (2008). Impact
assessment of plug-in hybrid electric vehicles on pacific north-west distribution
systems. Proceedings of the 2008 IEEE Power and Energy Society General Meeting—
Conversion and Delivery of Electrical Energy in the 21st (pp. 1–6). Pittsburg: United
Nations.
Shepherd, S. (2014). A review of system dynamics models applied in transportation. Trans. B
Trans. Dyn, 83–105.
Steven Silvern, S. Y. (2013). Environmental Change and Sustainability. New York: BoD –
Books on Demand.
Stone, S., Strutt, A., & Hertel, T. (2012). Socio-Economic Impact of Regional Transport
Infrastructure in the Greater Mekong Subregion In Infrastructure for Asian
Connectivity. Cheltenham: Edward Elgar Publishing.
Susanne Hartard, W. L. (2014). Competition and Conflicts on Resource Use. London:
Springer.
xlvii
Oak Ridge National Lab. (2013, July 8). Transportation Energy Databook. Retrieved from
Transportation Energy Databook: http://cta.ornl.gov/
Offer, G., Howey, D., Contestabile, M., Clague, R., & Brandon, N. (2010). Comparative
analysis of battery-electric, hydrogen fuel cell, and hybrid vehicles in a future
sustainable road transport system. Energy Policy, 24–29.
Pistoia, G. (2010). Electric and Hybrid Vehicles: Power Sources, Models, Sustainability,
Infrastructure, and the Market. Paris: Elsevier.
Rahmani-Andebili, M. (2019). Planning and Operation of Plug-In Electric Vehicles:
Technical, Geographical, and Social Aspects. London: Springer International
Publishing.
Rodrigo Garcia-Valle, J. A. (2012). Electric Vehicle Integration into Modern Power
Networks. New York: Springer Science & Business Media.
Rodrigo Garcia-Valle, J. A. (2012). Electric Vehicle Integration into Modern Power
Networks. London: Springer Science & Business Media.
Roy Billinton, R. N. (2012). Reliability Assessment of Large Electric Power Systems. London:
Springer Science & Business Media.
Salah, F., Ilg, J., Flath, C., Basse, H., & van Dinther, C. (2015). Impact of electric vehicles on
distribution substations: A Swiss case study. Applications Energy, 88–96.
Sandalow, D. (2009). Plug-in Electric Vehicles: What Role for Washington? Washington DC:
Brookings Institution Press.
Schneider, K., Gerkensmeyer, C., Kintner-Meyer, M., & Fletcher, M. (2008). Impact
assessment of plug-in hybrid electric vehicles on pacific north-west distribution
systems. Proceedings of the 2008 IEEE Power and Energy Society General Meeting—
Conversion and Delivery of Electrical Energy in the 21st (pp. 1–6). Pittsburg: United
Nations.
Shepherd, S. (2014). A review of system dynamics models applied in transportation. Trans. B
Trans. Dyn, 83–105.
Steven Silvern, S. Y. (2013). Environmental Change and Sustainability. New York: BoD –
Books on Demand.
Stone, S., Strutt, A., & Hertel, T. (2012). Socio-Economic Impact of Regional Transport
Infrastructure in the Greater Mekong Subregion In Infrastructure for Asian
Connectivity. Cheltenham: Edward Elgar Publishing.
Susanne Hartard, W. L. (2014). Competition and Conflicts on Resource Use. London:
Springer.
xlvii
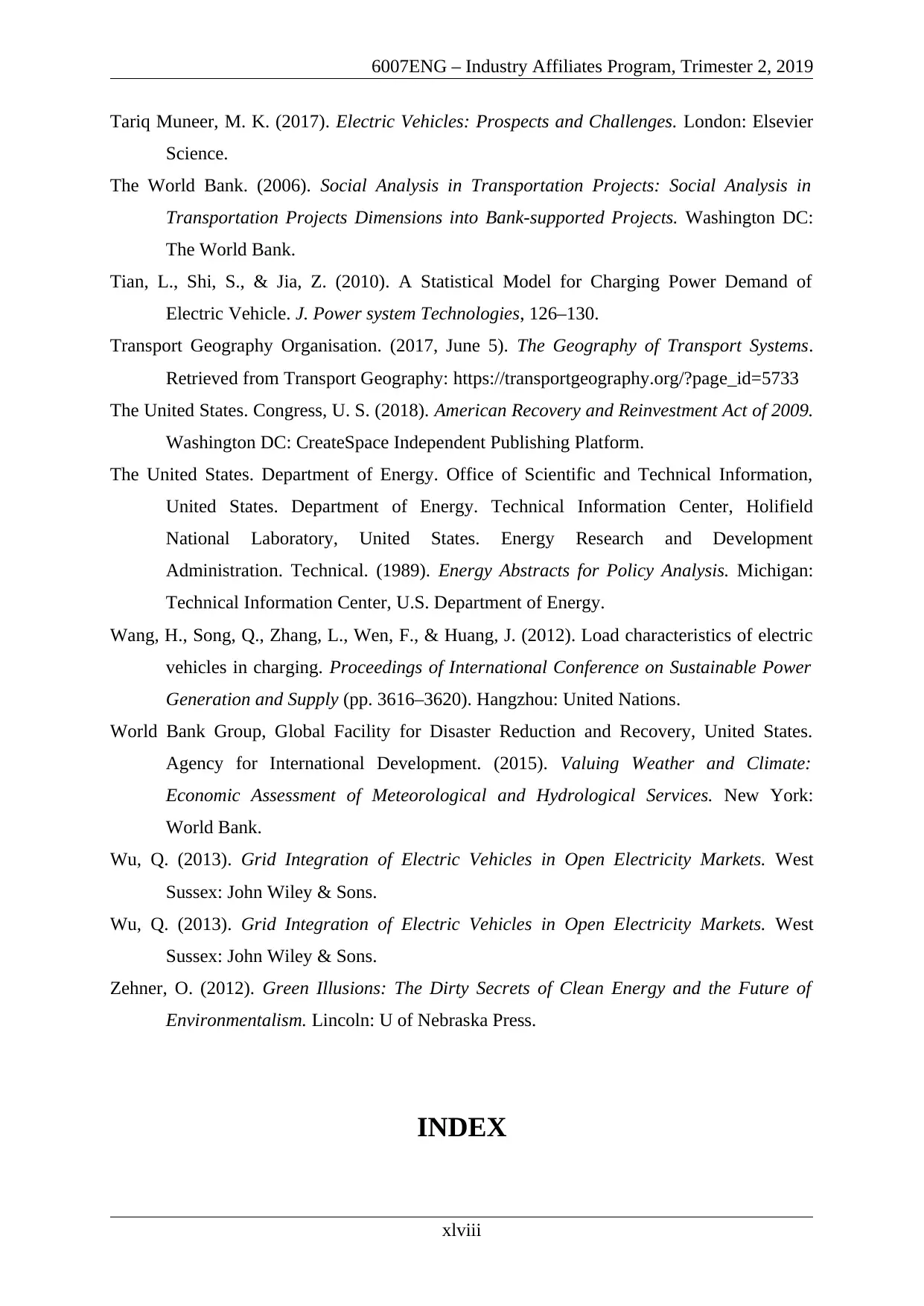
6007ENG – Industry Affiliates Program, Trimester 2, 2019
Tariq Muneer, M. K. (2017). Electric Vehicles: Prospects and Challenges. London: Elsevier
Science.
The World Bank. (2006). Social Analysis in Transportation Projects: Social Analysis in
Transportation Projects Dimensions into Bank-supported Projects. Washington DC:
The World Bank.
Tian, L., Shi, S., & Jia, Z. (2010). A Statistical Model for Charging Power Demand of
Electric Vehicle. J. Power system Technologies, 126–130.
Transport Geography Organisation. (2017, June 5). The Geography of Transport Systems.
Retrieved from Transport Geography: https://transportgeography.org/?page_id=5733
The United States. Congress, U. S. (2018). American Recovery and Reinvestment Act of 2009.
Washington DC: CreateSpace Independent Publishing Platform.
The United States. Department of Energy. Office of Scientific and Technical Information,
United States. Department of Energy. Technical Information Center, Holifield
National Laboratory, United States. Energy Research and Development
Administration. Technical. (1989). Energy Abstracts for Policy Analysis. Michigan:
Technical Information Center, U.S. Department of Energy.
Wang, H., Song, Q., Zhang, L., Wen, F., & Huang, J. (2012). Load characteristics of electric
vehicles in charging. Proceedings of International Conference on Sustainable Power
Generation and Supply (pp. 3616–3620). Hangzhou: United Nations.
World Bank Group, Global Facility for Disaster Reduction and Recovery, United States.
Agency for International Development. (2015). Valuing Weather and Climate:
Economic Assessment of Meteorological and Hydrological Services. New York:
World Bank.
Wu, Q. (2013). Grid Integration of Electric Vehicles in Open Electricity Markets. West
Sussex: John Wiley & Sons.
Wu, Q. (2013). Grid Integration of Electric Vehicles in Open Electricity Markets. West
Sussex: John Wiley & Sons.
Zehner, O. (2012). Green Illusions: The Dirty Secrets of Clean Energy and the Future of
Environmentalism. Lincoln: U of Nebraska Press.
INDEX
xlviii
Tariq Muneer, M. K. (2017). Electric Vehicles: Prospects and Challenges. London: Elsevier
Science.
The World Bank. (2006). Social Analysis in Transportation Projects: Social Analysis in
Transportation Projects Dimensions into Bank-supported Projects. Washington DC:
The World Bank.
Tian, L., Shi, S., & Jia, Z. (2010). A Statistical Model for Charging Power Demand of
Electric Vehicle. J. Power system Technologies, 126–130.
Transport Geography Organisation. (2017, June 5). The Geography of Transport Systems.
Retrieved from Transport Geography: https://transportgeography.org/?page_id=5733
The United States. Congress, U. S. (2018). American Recovery and Reinvestment Act of 2009.
Washington DC: CreateSpace Independent Publishing Platform.
The United States. Department of Energy. Office of Scientific and Technical Information,
United States. Department of Energy. Technical Information Center, Holifield
National Laboratory, United States. Energy Research and Development
Administration. Technical. (1989). Energy Abstracts for Policy Analysis. Michigan:
Technical Information Center, U.S. Department of Energy.
Wang, H., Song, Q., Zhang, L., Wen, F., & Huang, J. (2012). Load characteristics of electric
vehicles in charging. Proceedings of International Conference on Sustainable Power
Generation and Supply (pp. 3616–3620). Hangzhou: United Nations.
World Bank Group, Global Facility for Disaster Reduction and Recovery, United States.
Agency for International Development. (2015). Valuing Weather and Climate:
Economic Assessment of Meteorological and Hydrological Services. New York:
World Bank.
Wu, Q. (2013). Grid Integration of Electric Vehicles in Open Electricity Markets. West
Sussex: John Wiley & Sons.
Wu, Q. (2013). Grid Integration of Electric Vehicles in Open Electricity Markets. West
Sussex: John Wiley & Sons.
Zehner, O. (2012). Green Illusions: The Dirty Secrets of Clean Energy and the Future of
Environmentalism. Lincoln: U of Nebraska Press.
INDEX
xlviii
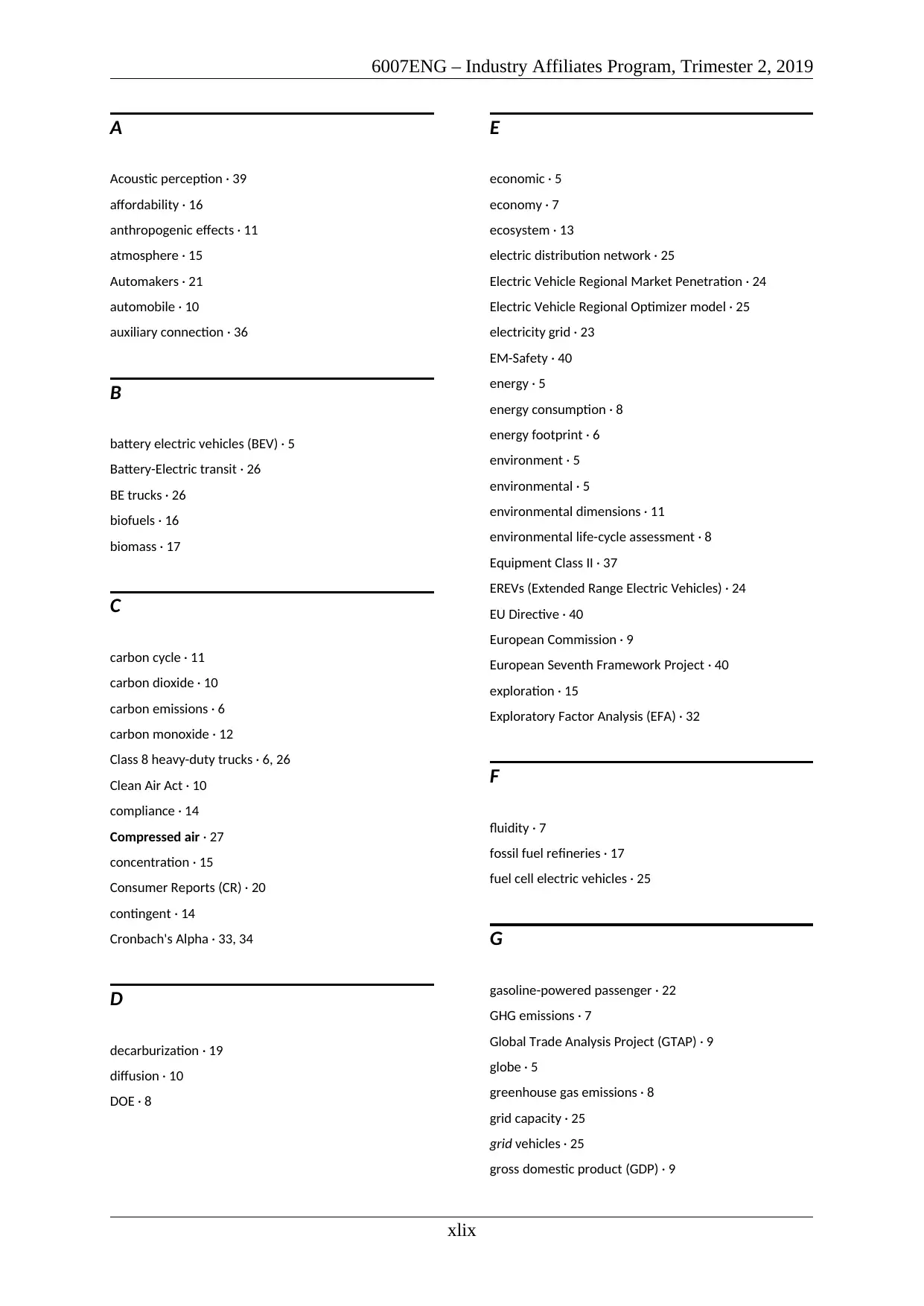
6007ENG – Industry Affiliates Program, Trimester 2, 2019
A
Acoustic perception · 39
affordability · 16
anthropogenic effects · 11
atmosphere · 15
Automakers · 21
automobile · 10
auxiliary connection · 36
B
battery electric vehicles (BEV) · 5
Battery-Electric transit · 26
BE trucks · 26
biofuels · 16
biomass · 17
C
carbon cycle · 11
carbon dioxide · 10
carbon emissions · 6
carbon monoxide · 12
Class 8 heavy-duty trucks · 6, 26
Clean Air Act · 10
compliance · 14
Compressed air · 27
concentration · 15
Consumer Reports (CR) · 20
contingent · 14
Cronbach's Alpha · 33, 34
D
decarburization · 19
diffusion · 10
DOE · 8
E
economic · 5
economy · 7
ecosystem · 13
electric distribution network · 25
Electric Vehicle Regional Market Penetration · 24
Electric Vehicle Regional Optimizer model · 25
electricity grid · 23
EM-Safety · 40
energy · 5
energy consumption · 8
energy footprint · 6
environment · 5
environmental · 5
environmental dimensions · 11
environmental life-cycle assessment · 8
Equipment Class II · 37
EREVs (Extended Range Electric Vehicles) · 24
EU Directive · 40
European Commission · 9
European Seventh Framework Project · 40
exploration · 15
Exploratory Factor Analysis (EFA) · 32
F
fluidity · 7
fossil fuel refineries · 17
fuel cell electric vehicles · 25
G
gasoline-powered passenger · 22
GHG emissions · 7
Global Trade Analysis Project (GTAP) · 9
globe · 5
greenhouse gas emissions · 8
grid capacity · 25
grid vehicles · 25
gross domestic product (GDP) · 9
xlix
A
Acoustic perception · 39
affordability · 16
anthropogenic effects · 11
atmosphere · 15
Automakers · 21
automobile · 10
auxiliary connection · 36
B
battery electric vehicles (BEV) · 5
Battery-Electric transit · 26
BE trucks · 26
biofuels · 16
biomass · 17
C
carbon cycle · 11
carbon dioxide · 10
carbon emissions · 6
carbon monoxide · 12
Class 8 heavy-duty trucks · 6, 26
Clean Air Act · 10
compliance · 14
Compressed air · 27
concentration · 15
Consumer Reports (CR) · 20
contingent · 14
Cronbach's Alpha · 33, 34
D
decarburization · 19
diffusion · 10
DOE · 8
E
economic · 5
economy · 7
ecosystem · 13
electric distribution network · 25
Electric Vehicle Regional Market Penetration · 24
Electric Vehicle Regional Optimizer model · 25
electricity grid · 23
EM-Safety · 40
energy · 5
energy consumption · 8
energy footprint · 6
environment · 5
environmental · 5
environmental dimensions · 11
environmental life-cycle assessment · 8
Equipment Class II · 37
EREVs (Extended Range Electric Vehicles) · 24
EU Directive · 40
European Commission · 9
European Seventh Framework Project · 40
exploration · 15
Exploratory Factor Analysis (EFA) · 32
F
fluidity · 7
fossil fuel refineries · 17
fuel cell electric vehicles · 25
G
gasoline-powered passenger · 22
GHG emissions · 7
Global Trade Analysis Project (GTAP) · 9
globe · 5
greenhouse gas emissions · 8
grid capacity · 25
grid vehicles · 25
gross domestic product (GDP) · 9
xlix
Secure Best Marks with AI Grader
Need help grading? Try our AI Grader for instant feedback on your assignments.
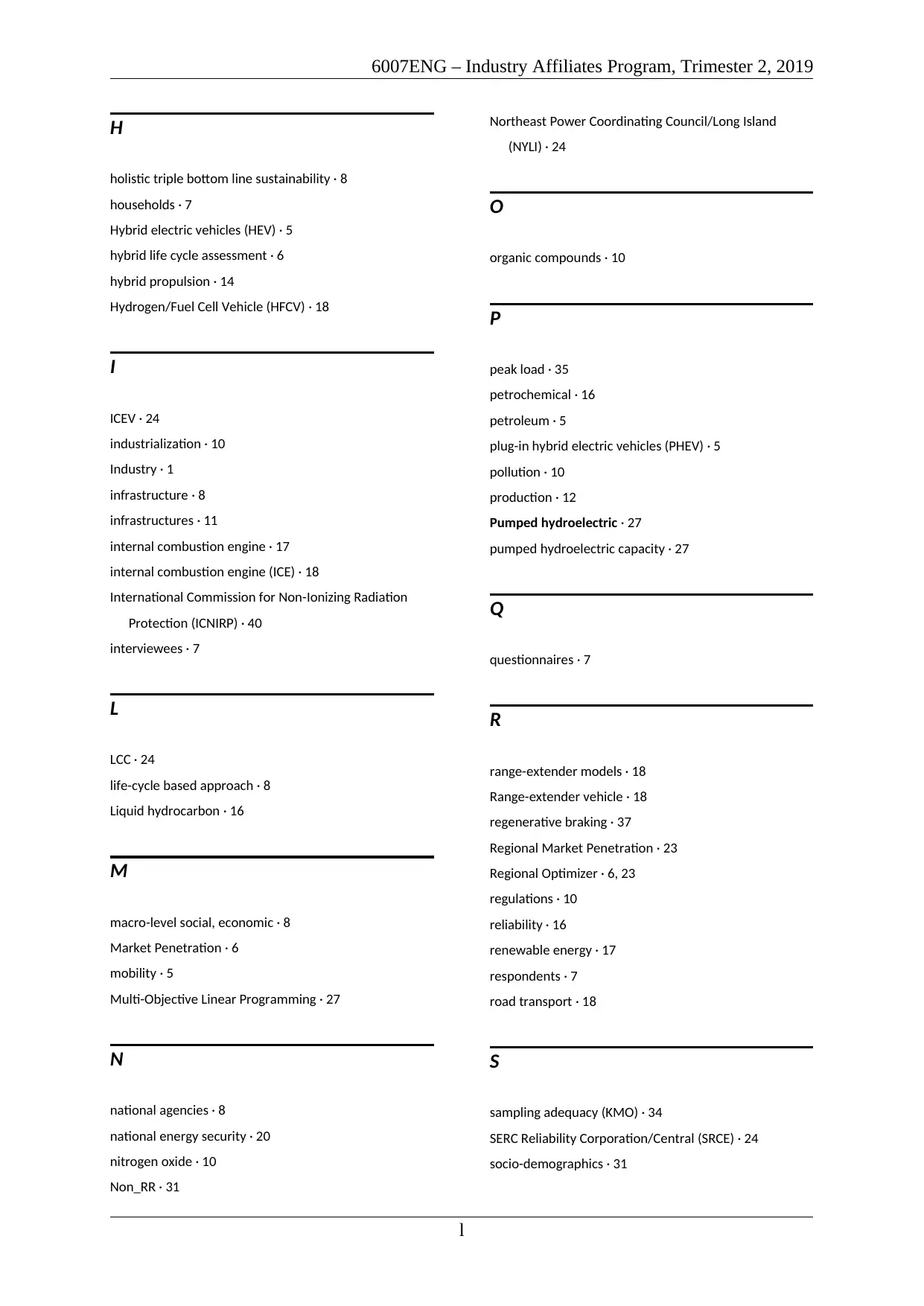
6007ENG – Industry Affiliates Program, Trimester 2, 2019
H
holistic triple bottom line sustainability · 8
households · 7
Hybrid electric vehicles (HEV) · 5
hybrid life cycle assessment · 6
hybrid propulsion · 14
Hydrogen/Fuel Cell Vehicle (HFCV) · 18
I
ICEV · 24
industrialization · 10
Industry · 1
infrastructure · 8
infrastructures · 11
internal combustion engine · 17
internal combustion engine (ICE) · 18
International Commission for Non-Ionizing Radiation
Protection (ICNIRP) · 40
interviewees · 7
L
LCC · 24
life-cycle based approach · 8
Liquid hydrocarbon · 16
M
macro-level social, economic · 8
Market Penetration · 6
mobility · 5
Multi-Objective Linear Programming · 27
N
national agencies · 8
national energy security · 20
nitrogen oxide · 10
Non_RR · 31
Northeast Power Coordinating Council/Long Island
(NYLI) · 24
O
organic compounds · 10
P
peak load · 35
petrochemical · 16
petroleum · 5
plug-in hybrid electric vehicles (PHEV) · 5
pollution · 10
production · 12
Pumped hydroelectric · 27
pumped hydroelectric capacity · 27
Q
questionnaires · 7
R
range-extender models · 18
Range-extender vehicle · 18
regenerative braking · 37
Regional Market Penetration · 23
Regional Optimizer · 6, 23
regulations · 10
reliability · 16
renewable energy · 17
respondents · 7
road transport · 18
S
sampling adequacy (KMO) · 34
SERC Reliability Corporation/Central (SRCE) · 24
socio-demographics · 31
l
H
holistic triple bottom line sustainability · 8
households · 7
Hybrid electric vehicles (HEV) · 5
hybrid life cycle assessment · 6
hybrid propulsion · 14
Hydrogen/Fuel Cell Vehicle (HFCV) · 18
I
ICEV · 24
industrialization · 10
Industry · 1
infrastructure · 8
infrastructures · 11
internal combustion engine · 17
internal combustion engine (ICE) · 18
International Commission for Non-Ionizing Radiation
Protection (ICNIRP) · 40
interviewees · 7
L
LCC · 24
life-cycle based approach · 8
Liquid hydrocarbon · 16
M
macro-level social, economic · 8
Market Penetration · 6
mobility · 5
Multi-Objective Linear Programming · 27
N
national agencies · 8
national energy security · 20
nitrogen oxide · 10
Non_RR · 31
Northeast Power Coordinating Council/Long Island
(NYLI) · 24
O
organic compounds · 10
P
peak load · 35
petrochemical · 16
petroleum · 5
plug-in hybrid electric vehicles (PHEV) · 5
pollution · 10
production · 12
Pumped hydroelectric · 27
pumped hydroelectric capacity · 27
Q
questionnaires · 7
R
range-extender models · 18
Range-extender vehicle · 18
regenerative braking · 37
Regional Market Penetration · 23
Regional Optimizer · 6, 23
regulations · 10
reliability · 16
renewable energy · 17
respondents · 7
road transport · 18
S
sampling adequacy (KMO) · 34
SERC Reliability Corporation/Central (SRCE) · 24
socio-demographics · 31
l
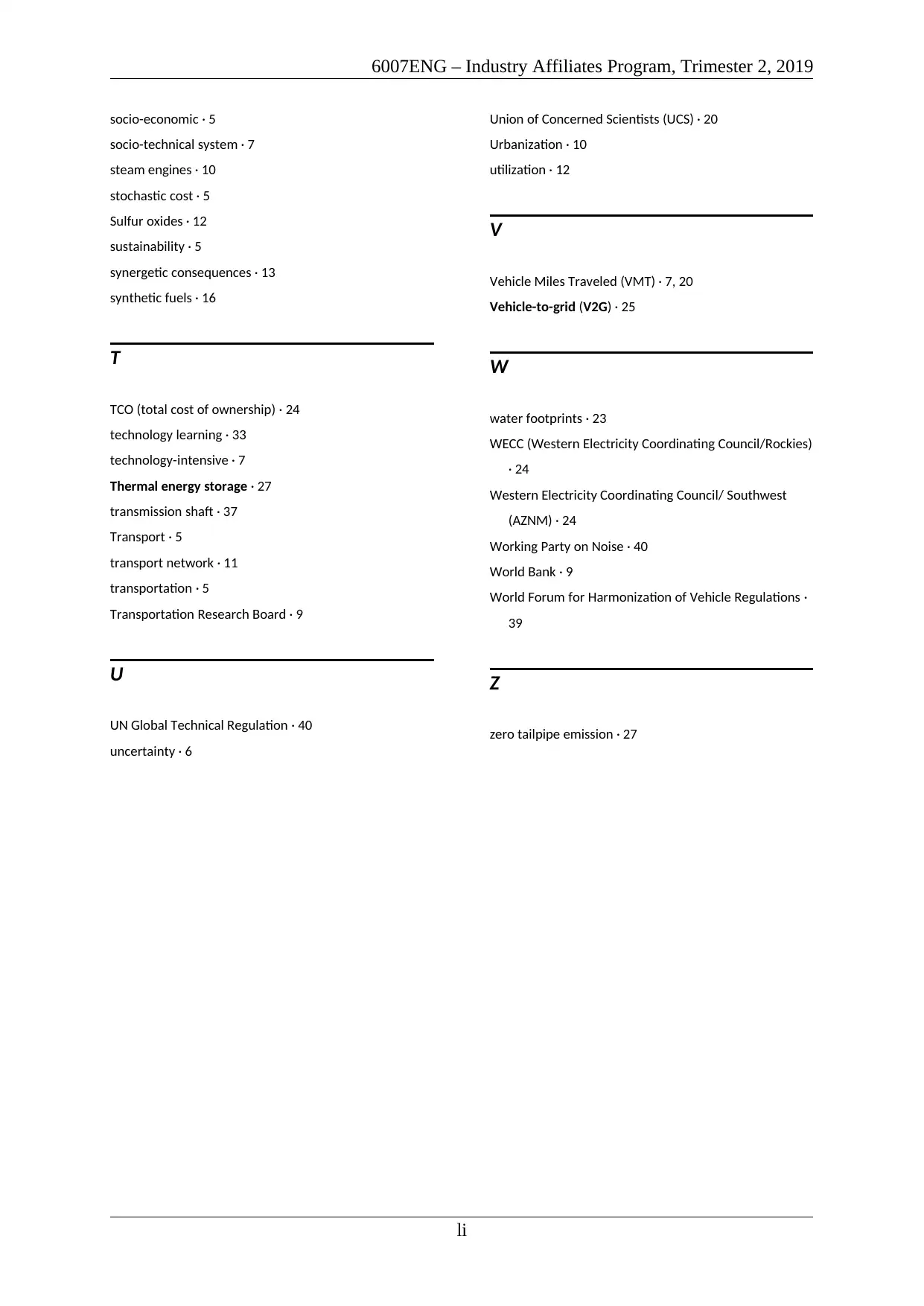
6007ENG – Industry Affiliates Program, Trimester 2, 2019
socio-economic · 5
socio-technical system · 7
steam engines · 10
stochastic cost · 5
Sulfur oxides · 12
sustainability · 5
synergetic consequences · 13
synthetic fuels · 16
T
TCO (total cost of ownership) · 24
technology learning · 33
technology-intensive · 7
Thermal energy storage · 27
transmission shaft · 37
Transport · 5
transport network · 11
transportation · 5
Transportation Research Board · 9
U
UN Global Technical Regulation · 40
uncertainty · 6
Union of Concerned Scientists (UCS) · 20
Urbanization · 10
utilization · 12
V
Vehicle Miles Traveled (VMT) · 7, 20
Vehicle-to-grid (V2G) · 25
W
water footprints · 23
WECC (Western Electricity Coordinating Council/Rockies)
· 24
Western Electricity Coordinating Council/ Southwest
(AZNM) · 24
Working Party on Noise · 40
World Bank · 9
World Forum for Harmonization of Vehicle Regulations ·
39
Z
zero tailpipe emission · 27
li
socio-economic · 5
socio-technical system · 7
steam engines · 10
stochastic cost · 5
Sulfur oxides · 12
sustainability · 5
synergetic consequences · 13
synthetic fuels · 16
T
TCO (total cost of ownership) · 24
technology learning · 33
technology-intensive · 7
Thermal energy storage · 27
transmission shaft · 37
Transport · 5
transport network · 11
transportation · 5
Transportation Research Board · 9
U
UN Global Technical Regulation · 40
uncertainty · 6
Union of Concerned Scientists (UCS) · 20
Urbanization · 10
utilization · 12
V
Vehicle Miles Traveled (VMT) · 7, 20
Vehicle-to-grid (V2G) · 25
W
water footprints · 23
WECC (Western Electricity Coordinating Council/Rockies)
· 24
Western Electricity Coordinating Council/ Southwest
(AZNM) · 24
Working Party on Noise · 40
World Bank · 9
World Forum for Harmonization of Vehicle Regulations ·
39
Z
zero tailpipe emission · 27
li
1 out of 54
![[object Object]](/_next/image/?url=%2F_next%2Fstatic%2Fmedia%2Flogo.6d15ce61.png&w=640&q=75)
Your All-in-One AI-Powered Toolkit for Academic Success.
+13062052269
info@desklib.com
Available 24*7 on WhatsApp / Email
Unlock your academic potential
© 2024 | Zucol Services PVT LTD | All rights reserved.