Dye Sensitized Solar Cells: History, Principle, and Efficiency Parameters
VerifiedAdded on 2023/05/29
|6
|1798
|122
AI Summary
This literature review provides an overview of the history, principle, and efficiency parameters of dye sensitized solar cells (DSSCs). It explains the components of DSSCs, including the cathode, anode, oxide layer, dye, and electrolyte, and how they work together to convert sunlight into electricity. The review also discusses the efficiency parameters of DSSCs, including the quantum yield for process of injection and the Incident Photon to Electrical Conversion Efficiency (IPCE).
Contribute Materials
Your contribution can guide someone’s learning journey. Share your
documents today.
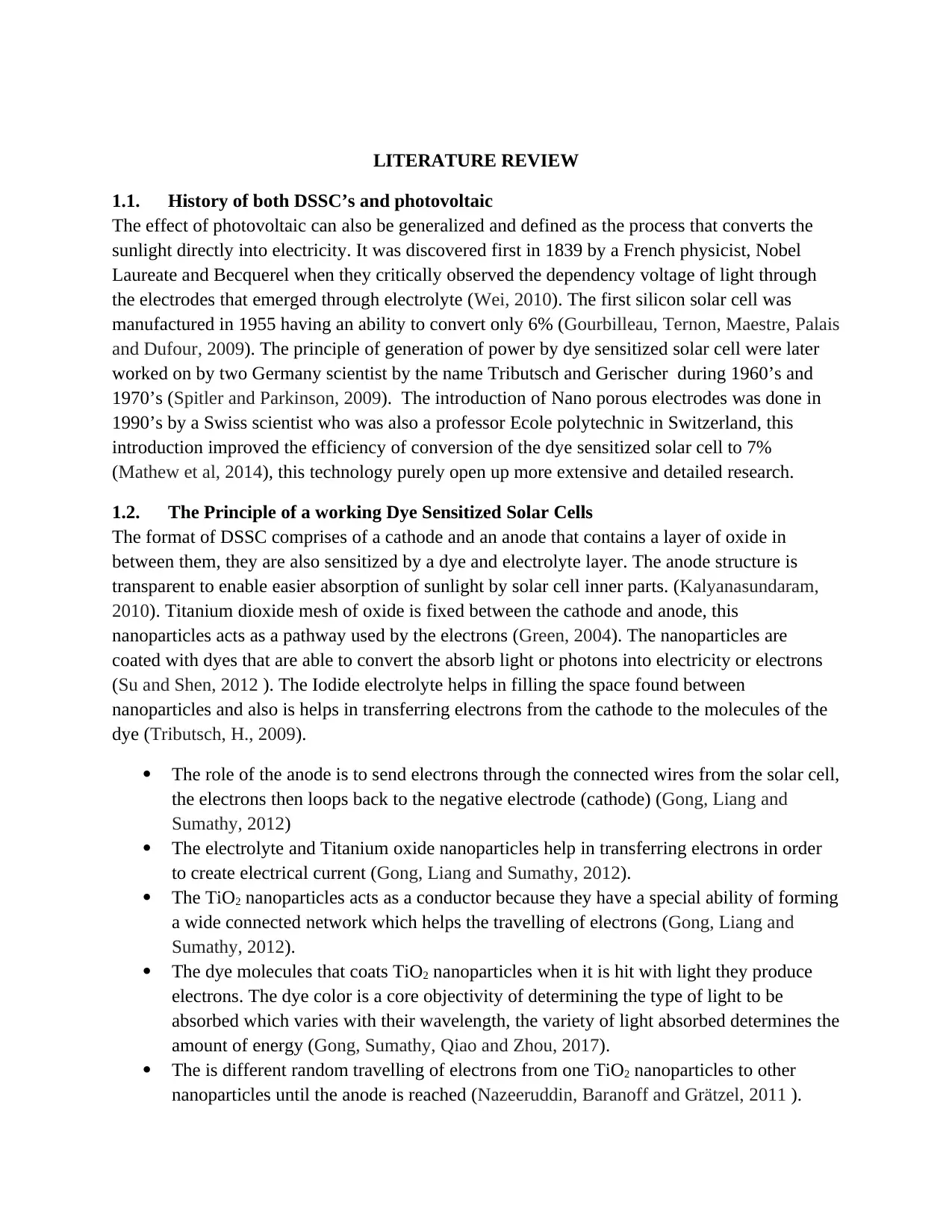
LITERATURE REVIEW
1.1. History of both DSSC’s and photovoltaic
The effect of photovoltaic can also be generalized and defined as the process that converts the
sunlight directly into electricity. It was discovered first in 1839 by a French physicist, Nobel
Laureate and Becquerel when they critically observed the dependency voltage of light through
the electrodes that emerged through electrolyte (Wei, 2010). The first silicon solar cell was
manufactured in 1955 having an ability to convert only 6% (Gourbilleau, Ternon, Maestre, Palais
and Dufour, 2009). The principle of generation of power by dye sensitized solar cell were later
worked on by two Germany scientist by the name Tributsch and Gerischer during 1960’s and
1970’s (Spitler and Parkinson, 2009). The introduction of Nano porous electrodes was done in
1990’s by a Swiss scientist who was also a professor Ecole polytechnic in Switzerland, this
introduction improved the efficiency of conversion of the dye sensitized solar cell to 7%
(Mathew et al, 2014), this technology purely open up more extensive and detailed research.
1.2. The Principle of a working Dye Sensitized Solar Cells
The format of DSSC comprises of a cathode and an anode that contains a layer of oxide in
between them, they are also sensitized by a dye and electrolyte layer. The anode structure is
transparent to enable easier absorption of sunlight by solar cell inner parts. (Kalyanasundaram,
2010). Titanium dioxide mesh of oxide is fixed between the cathode and anode, this
nanoparticles acts as a pathway used by the electrons (Green, 2004). The nanoparticles are
coated with dyes that are able to convert the absorb light or photons into electricity or electrons
(Su and Shen, 2012 ). The Iodide electrolyte helps in filling the space found between
nanoparticles and also is helps in transferring electrons from the cathode to the molecules of the
dye (Tributsch, H., 2009).
The role of the anode is to send electrons through the connected wires from the solar cell,
the electrons then loops back to the negative electrode (cathode) (Gong, Liang and
Sumathy, 2012)
The electrolyte and Titanium oxide nanoparticles help in transferring electrons in order
to create electrical current (Gong, Liang and Sumathy, 2012).
The TiO2 nanoparticles acts as a conductor because they have a special ability of forming
a wide connected network which helps the travelling of electrons (Gong, Liang and
Sumathy, 2012).
The dye molecules that coats TiO2 nanoparticles when it is hit with light they produce
electrons. The dye color is a core objectivity of determining the type of light to be
absorbed which varies with their wavelength, the variety of light absorbed determines the
amount of energy (Gong, Sumathy, Qiao and Zhou, 2017).
The is different random travelling of electrons from one TiO2 nanoparticles to other
nanoparticles until the anode is reached (Nazeeruddin, Baranoff and Grätzel, 2011 ).
1.1. History of both DSSC’s and photovoltaic
The effect of photovoltaic can also be generalized and defined as the process that converts the
sunlight directly into electricity. It was discovered first in 1839 by a French physicist, Nobel
Laureate and Becquerel when they critically observed the dependency voltage of light through
the electrodes that emerged through electrolyte (Wei, 2010). The first silicon solar cell was
manufactured in 1955 having an ability to convert only 6% (Gourbilleau, Ternon, Maestre, Palais
and Dufour, 2009). The principle of generation of power by dye sensitized solar cell were later
worked on by two Germany scientist by the name Tributsch and Gerischer during 1960’s and
1970’s (Spitler and Parkinson, 2009). The introduction of Nano porous electrodes was done in
1990’s by a Swiss scientist who was also a professor Ecole polytechnic in Switzerland, this
introduction improved the efficiency of conversion of the dye sensitized solar cell to 7%
(Mathew et al, 2014), this technology purely open up more extensive and detailed research.
1.2. The Principle of a working Dye Sensitized Solar Cells
The format of DSSC comprises of a cathode and an anode that contains a layer of oxide in
between them, they are also sensitized by a dye and electrolyte layer. The anode structure is
transparent to enable easier absorption of sunlight by solar cell inner parts. (Kalyanasundaram,
2010). Titanium dioxide mesh of oxide is fixed between the cathode and anode, this
nanoparticles acts as a pathway used by the electrons (Green, 2004). The nanoparticles are
coated with dyes that are able to convert the absorb light or photons into electricity or electrons
(Su and Shen, 2012 ). The Iodide electrolyte helps in filling the space found between
nanoparticles and also is helps in transferring electrons from the cathode to the molecules of the
dye (Tributsch, H., 2009).
The role of the anode is to send electrons through the connected wires from the solar cell,
the electrons then loops back to the negative electrode (cathode) (Gong, Liang and
Sumathy, 2012)
The electrolyte and Titanium oxide nanoparticles help in transferring electrons in order
to create electrical current (Gong, Liang and Sumathy, 2012).
The TiO2 nanoparticles acts as a conductor because they have a special ability of forming
a wide connected network which helps the travelling of electrons (Gong, Liang and
Sumathy, 2012).
The dye molecules that coats TiO2 nanoparticles when it is hit with light they produce
electrons. The dye color is a core objectivity of determining the type of light to be
absorbed which varies with their wavelength, the variety of light absorbed determines the
amount of energy (Gong, Sumathy, Qiao and Zhou, 2017).
The is different random travelling of electrons from one TiO2 nanoparticles to other
nanoparticles until the anode is reached (Nazeeruddin, Baranoff and Grätzel, 2011 ).
Secure Best Marks with AI Grader
Need help grading? Try our AI Grader for instant feedback on your assignments.
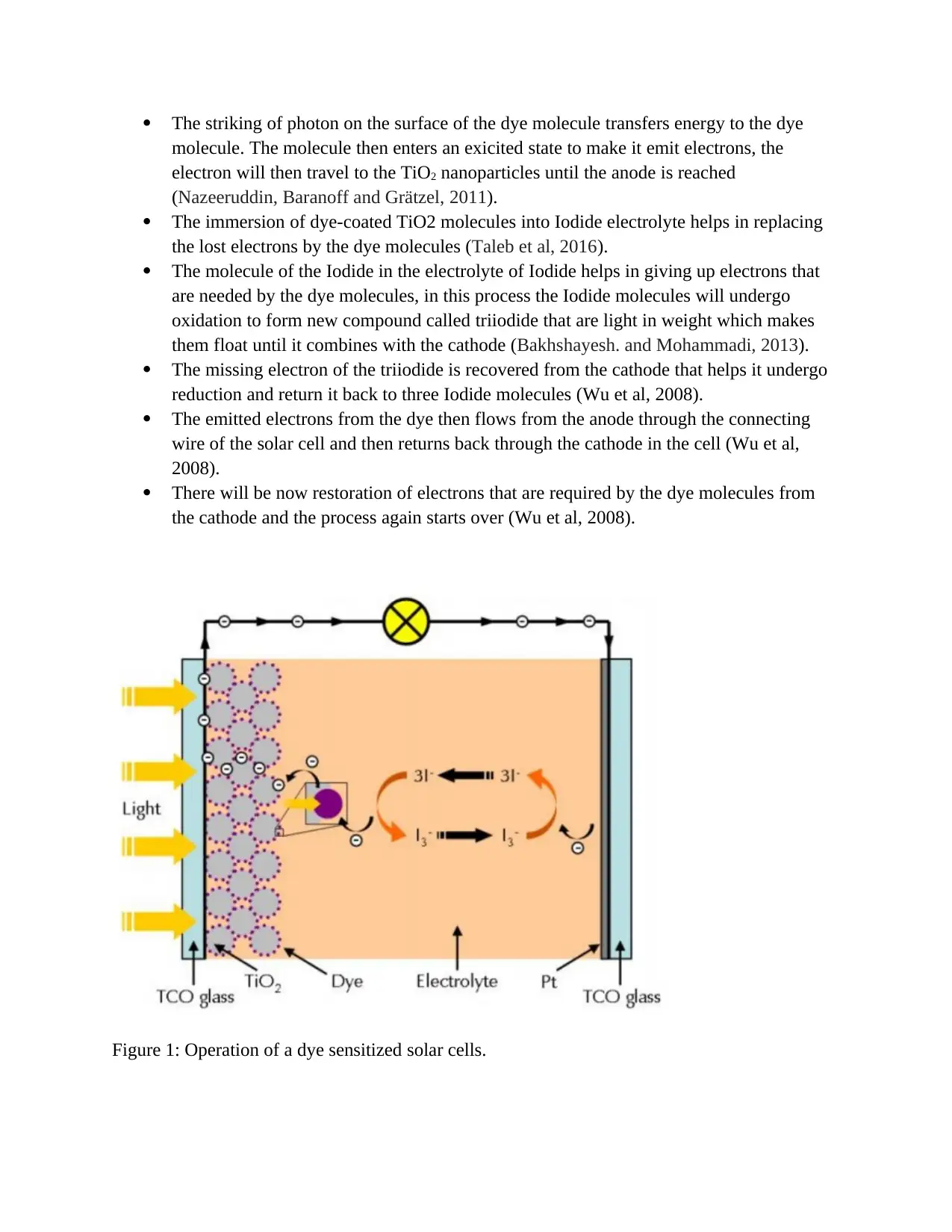
The striking of photon on the surface of the dye molecule transfers energy to the dye
molecule. The molecule then enters an exicited state to make it emit electrons, the
electron will then travel to the TiO2 nanoparticles until the anode is reached
(Nazeeruddin, Baranoff and Grätzel, 2011).
The immersion of dye-coated TiO2 molecules into Iodide electrolyte helps in replacing
the lost electrons by the dye molecules (Taleb et al, 2016).
The molecule of the Iodide in the electrolyte of Iodide helps in giving up electrons that
are needed by the dye molecules, in this process the Iodide molecules will undergo
oxidation to form new compound called triiodide that are light in weight which makes
them float until it combines with the cathode (Bakhshayesh. and Mohammadi, 2013).
The missing electron of the triiodide is recovered from the cathode that helps it undergo
reduction and return it back to three Iodide molecules (Wu et al, 2008).
The emitted electrons from the dye then flows from the anode through the connecting
wire of the solar cell and then returns back through the cathode in the cell (Wu et al,
2008).
There will be now restoration of electrons that are required by the dye molecules from
the cathode and the process again starts over (Wu et al, 2008).
Figure 1: Operation of a dye sensitized solar cells.
molecule. The molecule then enters an exicited state to make it emit electrons, the
electron will then travel to the TiO2 nanoparticles until the anode is reached
(Nazeeruddin, Baranoff and Grätzel, 2011).
The immersion of dye-coated TiO2 molecules into Iodide electrolyte helps in replacing
the lost electrons by the dye molecules (Taleb et al, 2016).
The molecule of the Iodide in the electrolyte of Iodide helps in giving up electrons that
are needed by the dye molecules, in this process the Iodide molecules will undergo
oxidation to form new compound called triiodide that are light in weight which makes
them float until it combines with the cathode (Bakhshayesh. and Mohammadi, 2013).
The missing electron of the triiodide is recovered from the cathode that helps it undergo
reduction and return it back to three Iodide molecules (Wu et al, 2008).
The emitted electrons from the dye then flows from the anode through the connecting
wire of the solar cell and then returns back through the cathode in the cell (Wu et al,
2008).
There will be now restoration of electrons that are required by the dye molecules from
the cathode and the process again starts over (Wu et al, 2008).
Figure 1: Operation of a dye sensitized solar cells.
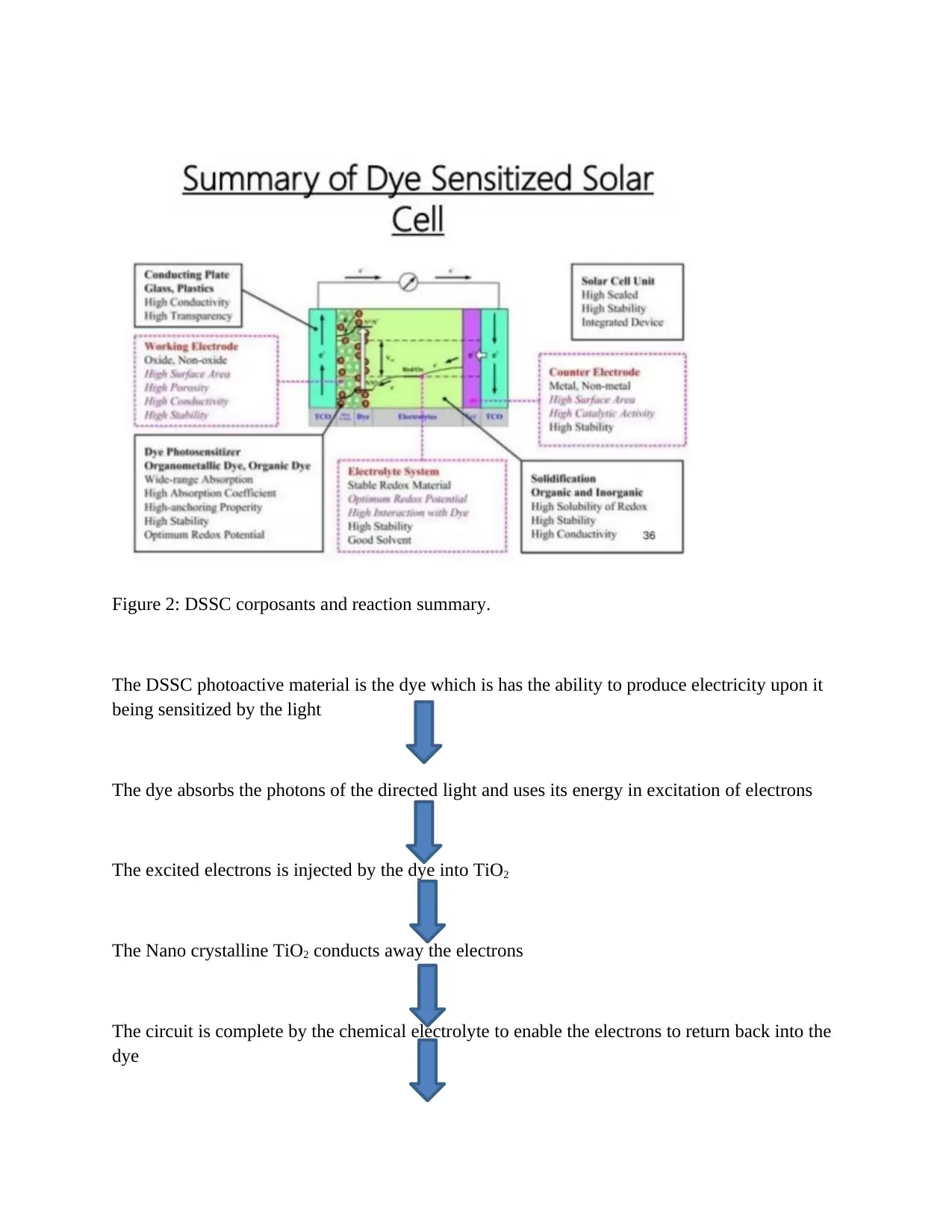
Figure 2: DSSC corposants and reaction summary.
The DSSC photoactive material is the dye which is has the ability to produce electricity upon it
being sensitized by the light
The dye absorbs the photons of the directed light and uses its energy in excitation of electrons
The excited electrons is injected by the dye into TiO2
The Nano crystalline TiO2 conducts away the electrons
The circuit is complete by the chemical electrolyte to enable the electrons to return back into the
dye
The DSSC photoactive material is the dye which is has the ability to produce electricity upon it
being sensitized by the light
The dye absorbs the photons of the directed light and uses its energy in excitation of electrons
The excited electrons is injected by the dye into TiO2
The Nano crystalline TiO2 conducts away the electrons
The circuit is complete by the chemical electrolyte to enable the electrons to return back into the
dye
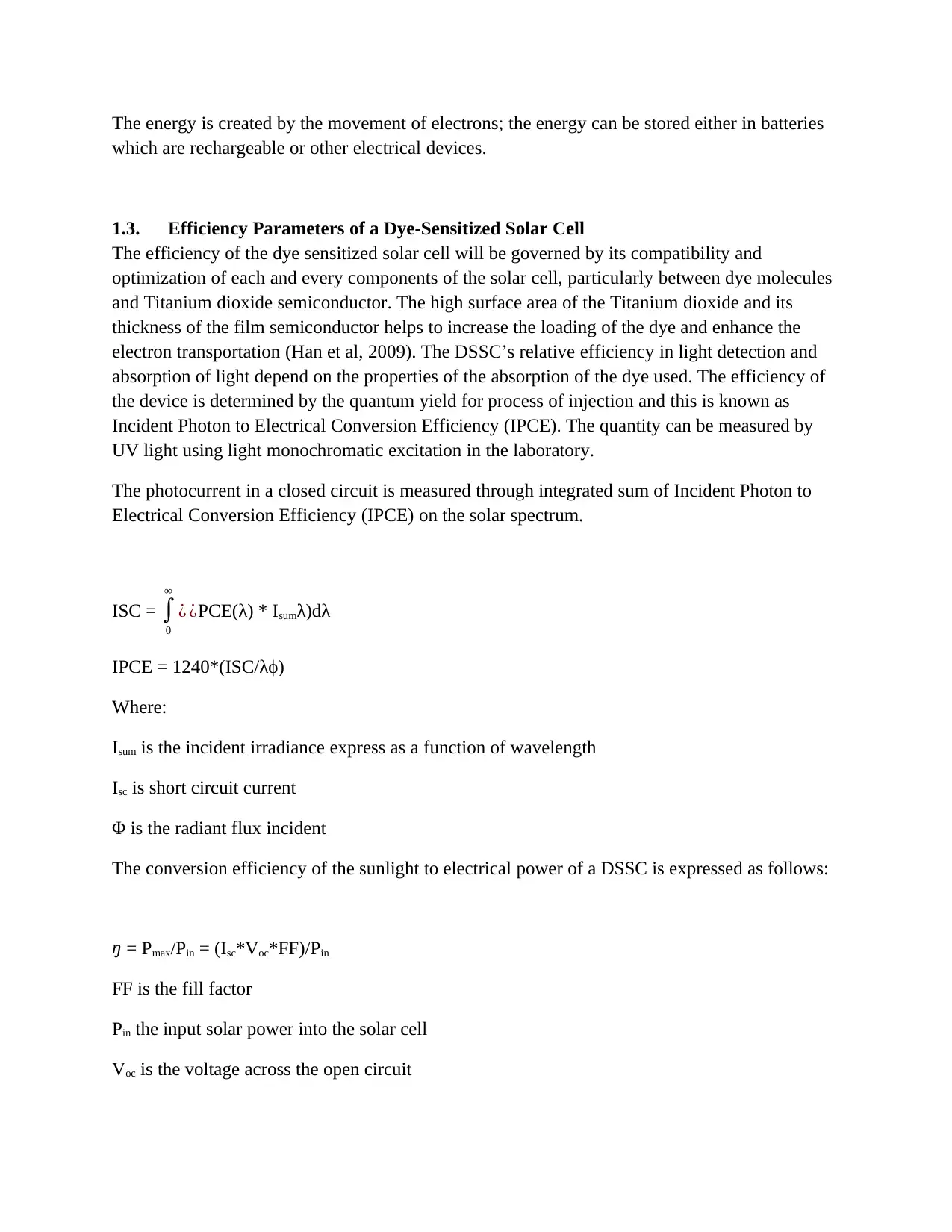
The energy is created by the movement of electrons; the energy can be stored either in batteries
which are rechargeable or other electrical devices.
1.3. Efficiency Parameters of a Dye-Sensitized Solar Cell
The efficiency of the dye sensitized solar cell will be governed by its compatibility and
optimization of each and every components of the solar cell, particularly between dye molecules
and Titanium dioxide semiconductor. The high surface area of the Titanium dioxide and its
thickness of the film semiconductor helps to increase the loading of the dye and enhance the
electron transportation (Han et al, 2009). The DSSC’s relative efficiency in light detection and
absorption of light depend on the properties of the absorption of the dye used. The efficiency of
the device is determined by the quantum yield for process of injection and this is known as
Incident Photon to Electrical Conversion Efficiency (IPCE). The quantity can be measured by
UV light using light monochromatic excitation in the laboratory.
The photocurrent in a closed circuit is measured through integrated sum of Incident Photon to
Electrical Conversion Efficiency (IPCE) on the solar spectrum.
ISC = ∫
0
∞
¿ ¿PCE(λ) * Isumλ)dλ
IPCE = 1240*(ISC/λϕ)
Where:
Isum is the incident irradiance express as a function of wavelength
Isc is short circuit current
Φ is the radiant flux incident
The conversion efficiency of the sunlight to electrical power of a DSSC is expressed as follows:
ŋ = Pmax/Pin = (Isc*Voc*FF)/Pin
FF is the fill factor
Pin the input solar power into the solar cell
Voc is the voltage across the open circuit
which are rechargeable or other electrical devices.
1.3. Efficiency Parameters of a Dye-Sensitized Solar Cell
The efficiency of the dye sensitized solar cell will be governed by its compatibility and
optimization of each and every components of the solar cell, particularly between dye molecules
and Titanium dioxide semiconductor. The high surface area of the Titanium dioxide and its
thickness of the film semiconductor helps to increase the loading of the dye and enhance the
electron transportation (Han et al, 2009). The DSSC’s relative efficiency in light detection and
absorption of light depend on the properties of the absorption of the dye used. The efficiency of
the device is determined by the quantum yield for process of injection and this is known as
Incident Photon to Electrical Conversion Efficiency (IPCE). The quantity can be measured by
UV light using light monochromatic excitation in the laboratory.
The photocurrent in a closed circuit is measured through integrated sum of Incident Photon to
Electrical Conversion Efficiency (IPCE) on the solar spectrum.
ISC = ∫
0
∞
¿ ¿PCE(λ) * Isumλ)dλ
IPCE = 1240*(ISC/λϕ)
Where:
Isum is the incident irradiance express as a function of wavelength
Isc is short circuit current
Φ is the radiant flux incident
The conversion efficiency of the sunlight to electrical power of a DSSC is expressed as follows:
ŋ = Pmax/Pin = (Isc*Voc*FF)/Pin
FF is the fill factor
Pin the input solar power into the solar cell
Voc is the voltage across the open circuit
Secure Best Marks with AI Grader
Need help grading? Try our AI Grader for instant feedback on your assignments.
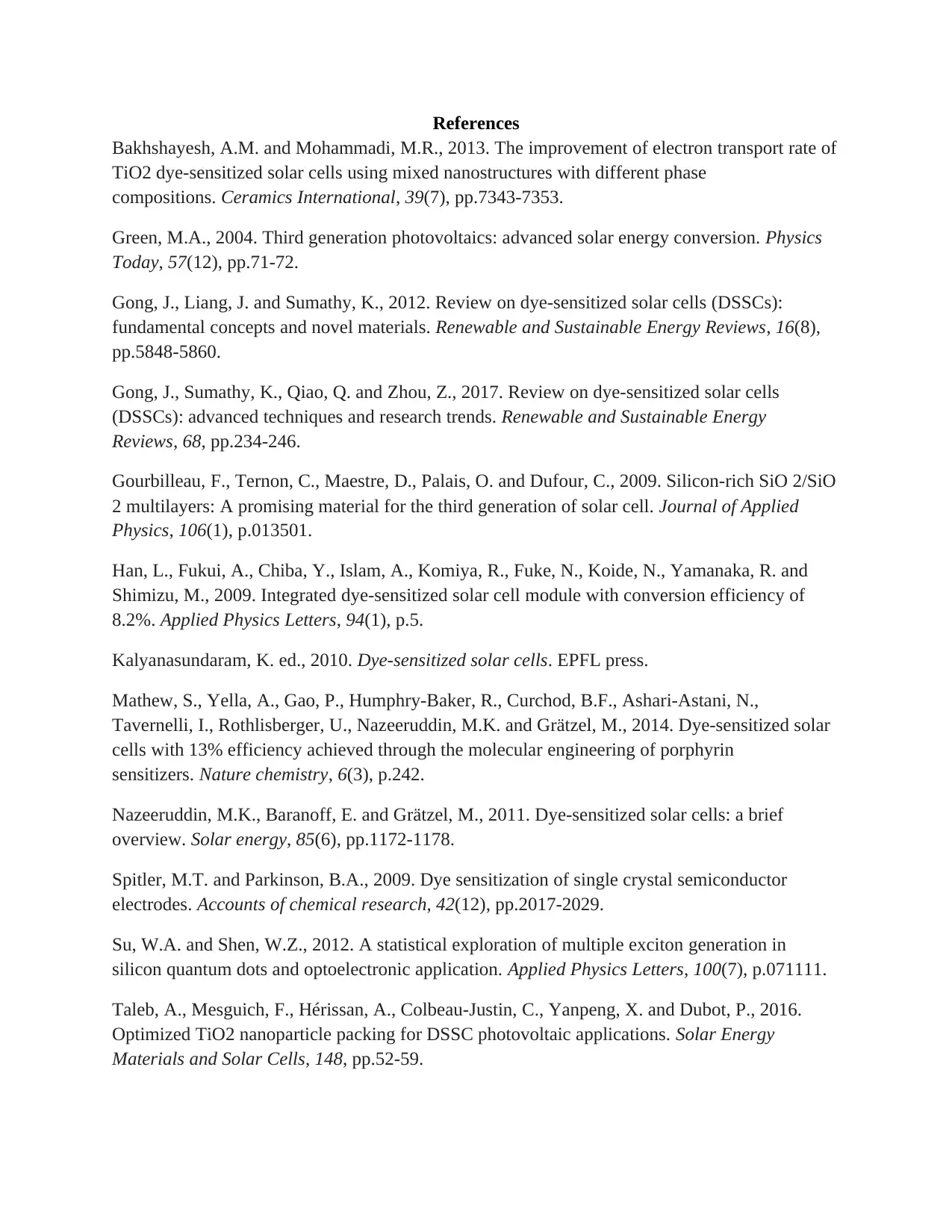
References
Bakhshayesh, A.M. and Mohammadi, M.R., 2013. The improvement of electron transport rate of
TiO2 dye-sensitized solar cells using mixed nanostructures with different phase
compositions. Ceramics International, 39(7), pp.7343-7353.
Green, M.A., 2004. Third generation photovoltaics: advanced solar energy conversion. Physics
Today, 57(12), pp.71-72.
Gong, J., Liang, J. and Sumathy, K., 2012. Review on dye-sensitized solar cells (DSSCs):
fundamental concepts and novel materials. Renewable and Sustainable Energy Reviews, 16(8),
pp.5848-5860.
Gong, J., Sumathy, K., Qiao, Q. and Zhou, Z., 2017. Review on dye-sensitized solar cells
(DSSCs): advanced techniques and research trends. Renewable and Sustainable Energy
Reviews, 68, pp.234-246.
Gourbilleau, F., Ternon, C., Maestre, D., Palais, O. and Dufour, C., 2009. Silicon-rich SiO 2/SiO
2 multilayers: A promising material for the third generation of solar cell. Journal of Applied
Physics, 106(1), p.013501.
Han, L., Fukui, A., Chiba, Y., Islam, A., Komiya, R., Fuke, N., Koide, N., Yamanaka, R. and
Shimizu, M., 2009. Integrated dye-sensitized solar cell module with conversion efficiency of
8.2%. Applied Physics Letters, 94(1), p.5.
Kalyanasundaram, K. ed., 2010. Dye-sensitized solar cells. EPFL press.
Mathew, S., Yella, A., Gao, P., Humphry-Baker, R., Curchod, B.F., Ashari-Astani, N.,
Tavernelli, I., Rothlisberger, U., Nazeeruddin, M.K. and Grätzel, M., 2014. Dye-sensitized solar
cells with 13% efficiency achieved through the molecular engineering of porphyrin
sensitizers. Nature chemistry, 6(3), p.242.
Nazeeruddin, M.K., Baranoff, E. and Grätzel, M., 2011. Dye-sensitized solar cells: a brief
overview. Solar energy, 85(6), pp.1172-1178.
Spitler, M.T. and Parkinson, B.A., 2009. Dye sensitization of single crystal semiconductor
electrodes. Accounts of chemical research, 42(12), pp.2017-2029.
Su, W.A. and Shen, W.Z., 2012. A statistical exploration of multiple exciton generation in
silicon quantum dots and optoelectronic application. Applied Physics Letters, 100(7), p.071111.
Taleb, A., Mesguich, F., Hérissan, A., Colbeau-Justin, C., Yanpeng, X. and Dubot, P., 2016.
Optimized TiO2 nanoparticle packing for DSSC photovoltaic applications. Solar Energy
Materials and Solar Cells, 148, pp.52-59.
Bakhshayesh, A.M. and Mohammadi, M.R., 2013. The improvement of electron transport rate of
TiO2 dye-sensitized solar cells using mixed nanostructures with different phase
compositions. Ceramics International, 39(7), pp.7343-7353.
Green, M.A., 2004. Third generation photovoltaics: advanced solar energy conversion. Physics
Today, 57(12), pp.71-72.
Gong, J., Liang, J. and Sumathy, K., 2012. Review on dye-sensitized solar cells (DSSCs):
fundamental concepts and novel materials. Renewable and Sustainable Energy Reviews, 16(8),
pp.5848-5860.
Gong, J., Sumathy, K., Qiao, Q. and Zhou, Z., 2017. Review on dye-sensitized solar cells
(DSSCs): advanced techniques and research trends. Renewable and Sustainable Energy
Reviews, 68, pp.234-246.
Gourbilleau, F., Ternon, C., Maestre, D., Palais, O. and Dufour, C., 2009. Silicon-rich SiO 2/SiO
2 multilayers: A promising material for the third generation of solar cell. Journal of Applied
Physics, 106(1), p.013501.
Han, L., Fukui, A., Chiba, Y., Islam, A., Komiya, R., Fuke, N., Koide, N., Yamanaka, R. and
Shimizu, M., 2009. Integrated dye-sensitized solar cell module with conversion efficiency of
8.2%. Applied Physics Letters, 94(1), p.5.
Kalyanasundaram, K. ed., 2010. Dye-sensitized solar cells. EPFL press.
Mathew, S., Yella, A., Gao, P., Humphry-Baker, R., Curchod, B.F., Ashari-Astani, N.,
Tavernelli, I., Rothlisberger, U., Nazeeruddin, M.K. and Grätzel, M., 2014. Dye-sensitized solar
cells with 13% efficiency achieved through the molecular engineering of porphyrin
sensitizers. Nature chemistry, 6(3), p.242.
Nazeeruddin, M.K., Baranoff, E. and Grätzel, M., 2011. Dye-sensitized solar cells: a brief
overview. Solar energy, 85(6), pp.1172-1178.
Spitler, M.T. and Parkinson, B.A., 2009. Dye sensitization of single crystal semiconductor
electrodes. Accounts of chemical research, 42(12), pp.2017-2029.
Su, W.A. and Shen, W.Z., 2012. A statistical exploration of multiple exciton generation in
silicon quantum dots and optoelectronic application. Applied Physics Letters, 100(7), p.071111.
Taleb, A., Mesguich, F., Hérissan, A., Colbeau-Justin, C., Yanpeng, X. and Dubot, P., 2016.
Optimized TiO2 nanoparticle packing for DSSC photovoltaic applications. Solar Energy
Materials and Solar Cells, 148, pp.52-59.
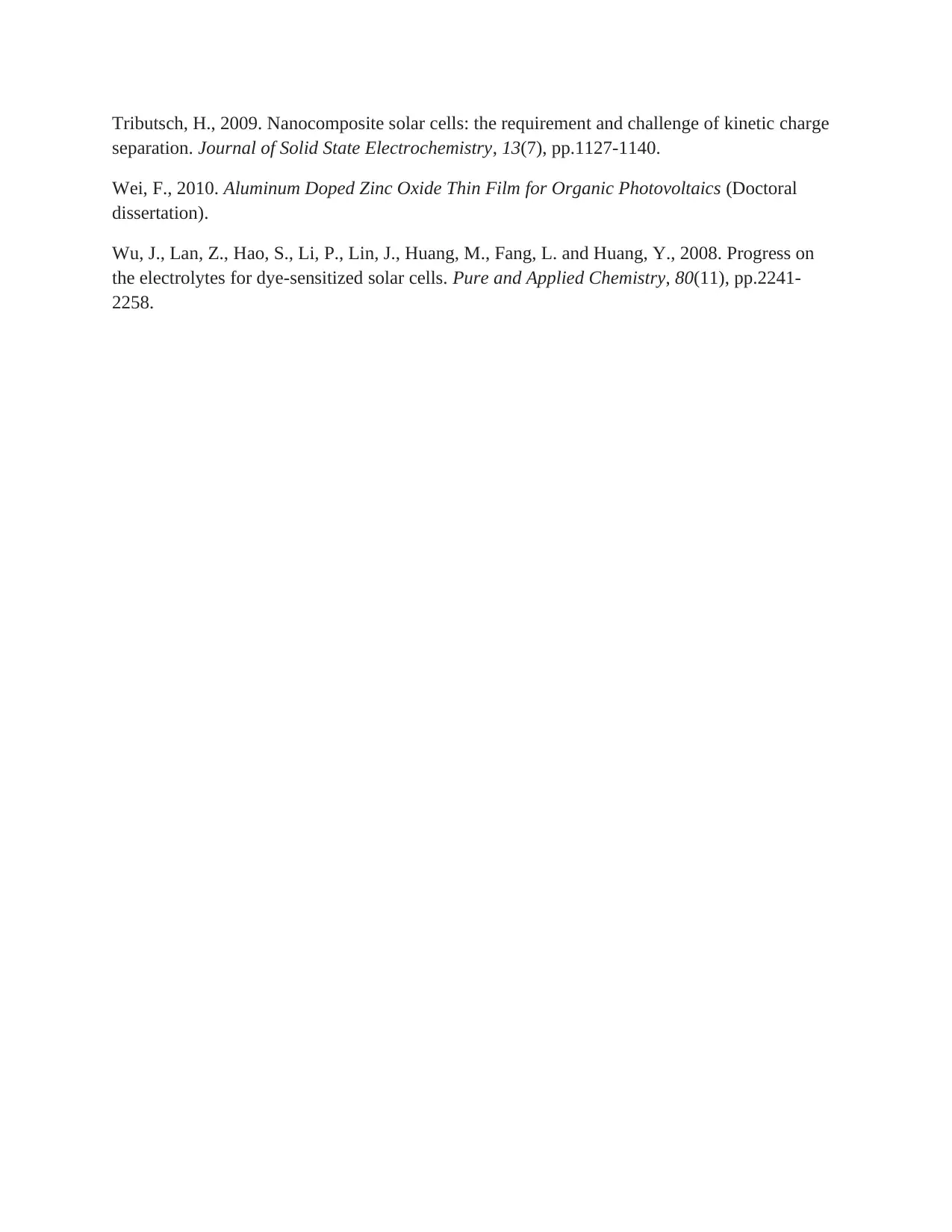
Tributsch, H., 2009. Nanocomposite solar cells: the requirement and challenge of kinetic charge
separation. Journal of Solid State Electrochemistry, 13(7), pp.1127-1140.
Wei, F., 2010. Aluminum Doped Zinc Oxide Thin Film for Organic Photovoltaics (Doctoral
dissertation).
Wu, J., Lan, Z., Hao, S., Li, P., Lin, J., Huang, M., Fang, L. and Huang, Y., 2008. Progress on
the electrolytes for dye-sensitized solar cells. Pure and Applied Chemistry, 80(11), pp.2241-
2258.
separation. Journal of Solid State Electrochemistry, 13(7), pp.1127-1140.
Wei, F., 2010. Aluminum Doped Zinc Oxide Thin Film for Organic Photovoltaics (Doctoral
dissertation).
Wu, J., Lan, Z., Hao, S., Li, P., Lin, J., Huang, M., Fang, L. and Huang, Y., 2008. Progress on
the electrolytes for dye-sensitized solar cells. Pure and Applied Chemistry, 80(11), pp.2241-
2258.
1 out of 6
![[object Object]](/_next/image/?url=%2F_next%2Fstatic%2Fmedia%2Flogo.6d15ce61.png&w=640&q=75)
Your All-in-One AI-Powered Toolkit for Academic Success.
+13062052269
info@desklib.com
Available 24*7 on WhatsApp / Email
Unlock your academic potential
© 2024 | Zucol Services PVT LTD | All rights reserved.