Microfluidics for Stem Cells Culture, Maintenance and Differentiation
VerifiedAdded on 2022/10/02
|11
|3466
|392
AI Summary
This article discusses the advantages and limitations of using microfluidics for stem cells culture, maintenance and differentiation. It explores the latest advancements in this field and provides future recommendations to address key concerns. The article also provides a literature review of studies related to microfluidics and stem cells culture.
Contribute Materials
Your contribution can guide someone’s learning journey. Share your
documents today.
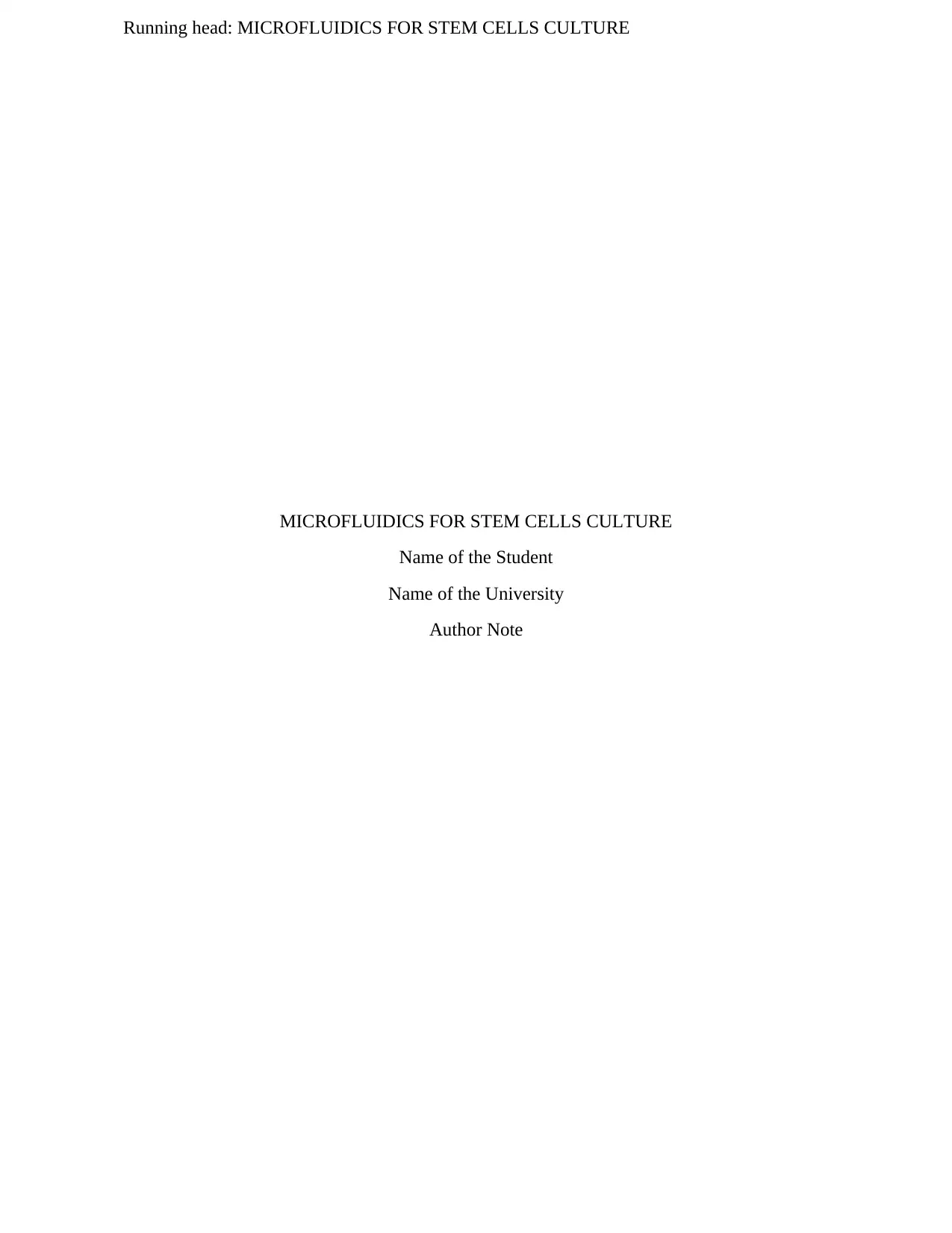
Running head: MICROFLUIDICS FOR STEM CELLS CULTURE
MICROFLUIDICS FOR STEM CELLS CULTURE
Name of the Student
Name of the University
Author Note
MICROFLUIDICS FOR STEM CELLS CULTURE
Name of the Student
Name of the University
Author Note
Secure Best Marks with AI Grader
Need help grading? Try our AI Grader for instant feedback on your assignments.
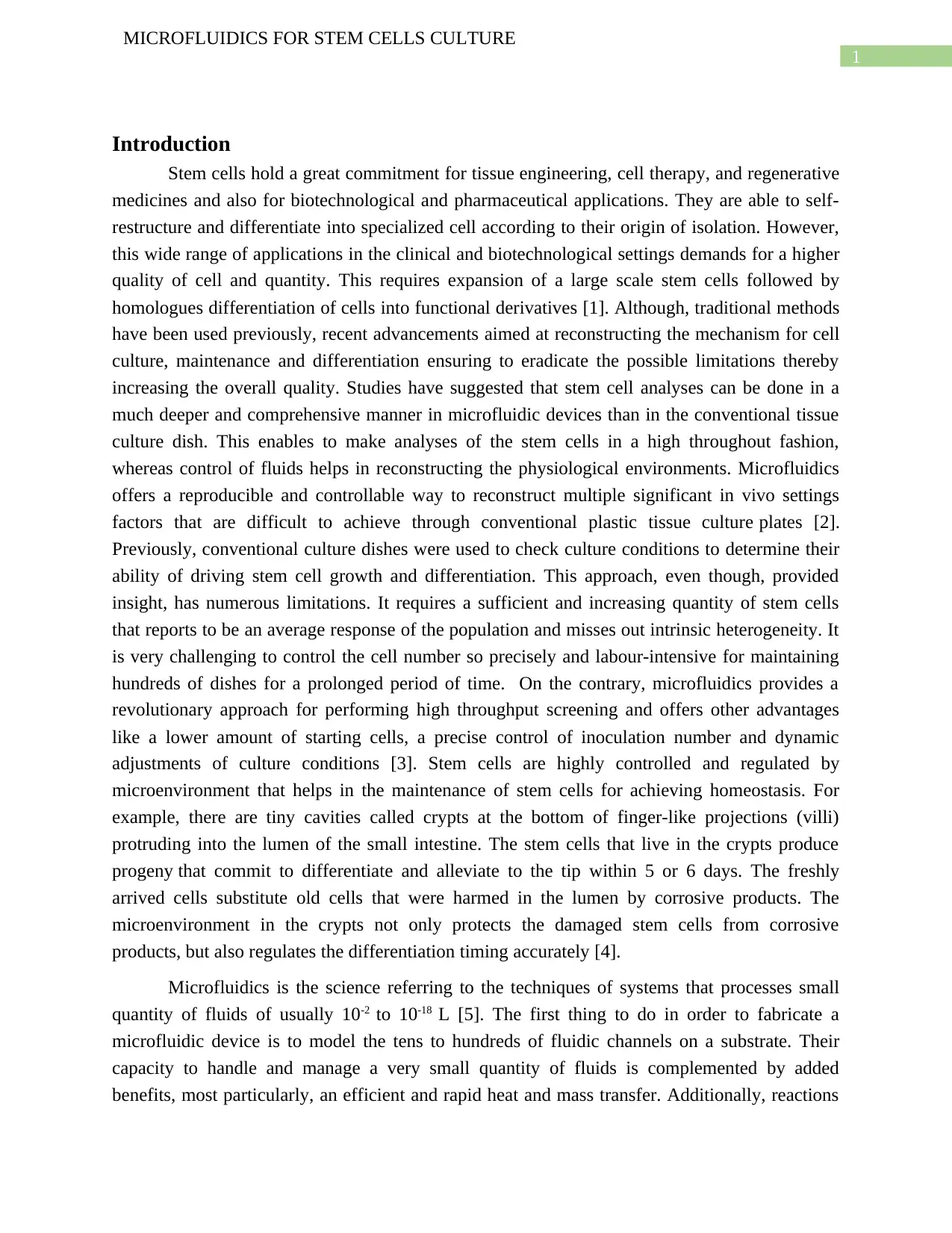
1
MICROFLUIDICS FOR STEM CELLS CULTURE
Introduction
Stem cells hold a great commitment for tissue engineering, cell therapy, and regenerative
medicines and also for biotechnological and pharmaceutical applications. They are able to self-
restructure and differentiate into specialized cell according to their origin of isolation. However,
this wide range of applications in the clinical and biotechnological settings demands for a higher
quality of cell and quantity. This requires expansion of a large scale stem cells followed by
homologues differentiation of cells into functional derivatives [1]. Although, traditional methods
have been used previously, recent advancements aimed at reconstructing the mechanism for cell
culture, maintenance and differentiation ensuring to eradicate the possible limitations thereby
increasing the overall quality. Studies have suggested that stem cell analyses can be done in a
much deeper and comprehensive manner in microfluidic devices than in the conventional tissue
culture dish. This enables to make analyses of the stem cells in a high throughout fashion,
whereas control of fluids helps in reconstructing the physiological environments. Microfluidics
offers a reproducible and controllable way to reconstruct multiple significant in vivo settings
factors that are difficult to achieve through conventional plastic tissue culture plates [2].
Previously, conventional culture dishes were used to check culture conditions to determine their
ability of driving stem cell growth and differentiation. This approach, even though, provided
insight, has numerous limitations. It requires a sufficient and increasing quantity of stem cells
that reports to be an average response of the population and misses out intrinsic heterogeneity. It
is very challenging to control the cell number so precisely and labour-intensive for maintaining
hundreds of dishes for a prolonged period of time. On the contrary, microfluidics provides a
revolutionary approach for performing high throughput screening and offers other advantages
like a lower amount of starting cells, a precise control of inoculation number and dynamic
adjustments of culture conditions [3]. Stem cells are highly controlled and regulated by
microenvironment that helps in the maintenance of stem cells for achieving homeostasis. For
example, there are tiny cavities called crypts at the bottom of finger-like projections (villi)
protruding into the lumen of the small intestine. The stem cells that live in the crypts produce
progeny that commit to differentiate and alleviate to the tip within 5 or 6 days. The freshly
arrived cells substitute old cells that were harmed in the lumen by corrosive products. The
microenvironment in the crypts not only protects the damaged stem cells from corrosive
products, but also regulates the differentiation timing accurately [4].
Microfluidics is the science referring to the techniques of systems that processes small
quantity of fluids of usually 10-2 to 10-18 L [5]. The first thing to do in order to fabricate a
microfluidic device is to model the tens to hundreds of fluidic channels on a substrate. Their
capacity to handle and manage a very small quantity of fluids is complemented by added
benefits, most particularly, an efficient and rapid heat and mass transfer. Additionally, reactions
MICROFLUIDICS FOR STEM CELLS CULTURE
Introduction
Stem cells hold a great commitment for tissue engineering, cell therapy, and regenerative
medicines and also for biotechnological and pharmaceutical applications. They are able to self-
restructure and differentiate into specialized cell according to their origin of isolation. However,
this wide range of applications in the clinical and biotechnological settings demands for a higher
quality of cell and quantity. This requires expansion of a large scale stem cells followed by
homologues differentiation of cells into functional derivatives [1]. Although, traditional methods
have been used previously, recent advancements aimed at reconstructing the mechanism for cell
culture, maintenance and differentiation ensuring to eradicate the possible limitations thereby
increasing the overall quality. Studies have suggested that stem cell analyses can be done in a
much deeper and comprehensive manner in microfluidic devices than in the conventional tissue
culture dish. This enables to make analyses of the stem cells in a high throughout fashion,
whereas control of fluids helps in reconstructing the physiological environments. Microfluidics
offers a reproducible and controllable way to reconstruct multiple significant in vivo settings
factors that are difficult to achieve through conventional plastic tissue culture plates [2].
Previously, conventional culture dishes were used to check culture conditions to determine their
ability of driving stem cell growth and differentiation. This approach, even though, provided
insight, has numerous limitations. It requires a sufficient and increasing quantity of stem cells
that reports to be an average response of the population and misses out intrinsic heterogeneity. It
is very challenging to control the cell number so precisely and labour-intensive for maintaining
hundreds of dishes for a prolonged period of time. On the contrary, microfluidics provides a
revolutionary approach for performing high throughput screening and offers other advantages
like a lower amount of starting cells, a precise control of inoculation number and dynamic
adjustments of culture conditions [3]. Stem cells are highly controlled and regulated by
microenvironment that helps in the maintenance of stem cells for achieving homeostasis. For
example, there are tiny cavities called crypts at the bottom of finger-like projections (villi)
protruding into the lumen of the small intestine. The stem cells that live in the crypts produce
progeny that commit to differentiate and alleviate to the tip within 5 or 6 days. The freshly
arrived cells substitute old cells that were harmed in the lumen by corrosive products. The
microenvironment in the crypts not only protects the damaged stem cells from corrosive
products, but also regulates the differentiation timing accurately [4].
Microfluidics is the science referring to the techniques of systems that processes small
quantity of fluids of usually 10-2 to 10-18 L [5]. The first thing to do in order to fabricate a
microfluidic device is to model the tens to hundreds of fluidic channels on a substrate. Their
capacity to handle and manage a very small quantity of fluids is complemented by added
benefits, most particularly, an efficient and rapid heat and mass transfer. Additionally, reactions
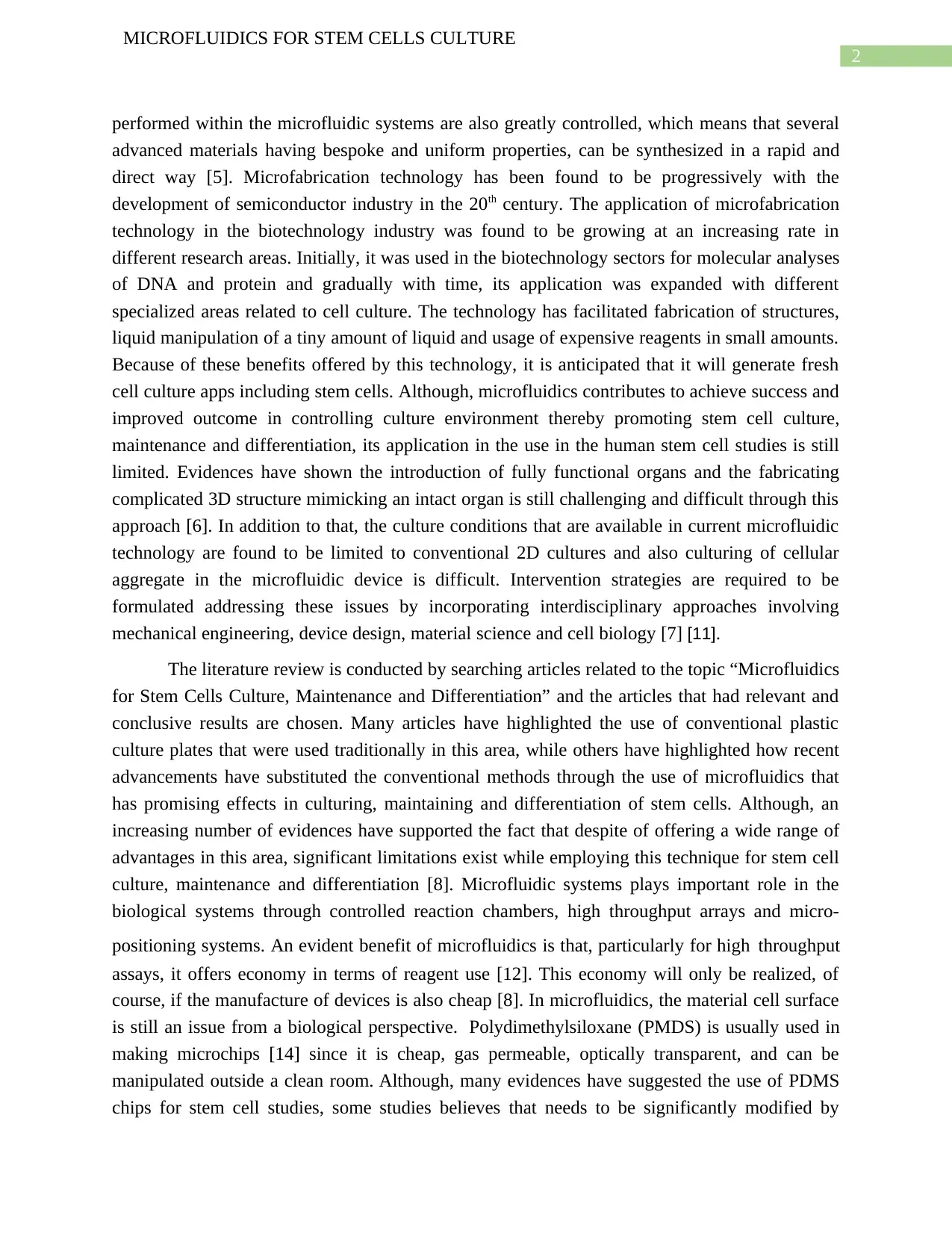
2
MICROFLUIDICS FOR STEM CELLS CULTURE
performed within the microfluidic systems are also greatly controlled, which means that several
advanced materials having bespoke and uniform properties, can be synthesized in a rapid and
direct way [5]. Microfabrication technology has been found to be progressively with the
development of semiconductor industry in the 20th century. The application of microfabrication
technology in the biotechnology industry was found to be growing at an increasing rate in
different research areas. Initially, it was used in the biotechnology sectors for molecular analyses
of DNA and protein and gradually with time, its application was expanded with different
specialized areas related to cell culture. The technology has facilitated fabrication of structures,
liquid manipulation of a tiny amount of liquid and usage of expensive reagents in small amounts.
Because of these benefits offered by this technology, it is anticipated that it will generate fresh
cell culture apps including stem cells. Although, microfluidics contributes to achieve success and
improved outcome in controlling culture environment thereby promoting stem cell culture,
maintenance and differentiation, its application in the use in the human stem cell studies is still
limited. Evidences have shown the introduction of fully functional organs and the fabricating
complicated 3D structure mimicking an intact organ is still challenging and difficult through this
approach [6]. In addition to that, the culture conditions that are available in current microfluidic
technology are found to be limited to conventional 2D cultures and also culturing of cellular
aggregate in the microfluidic device is difficult. Intervention strategies are required to be
formulated addressing these issues by incorporating interdisciplinary approaches involving
mechanical engineering, device design, material science and cell biology [7] [11].
The literature review is conducted by searching articles related to the topic “Microfluidics
for Stem Cells Culture, Maintenance and Differentiation” and the articles that had relevant and
conclusive results are chosen. Many articles have highlighted the use of conventional plastic
culture plates that were used traditionally in this area, while others have highlighted how recent
advancements have substituted the conventional methods through the use of microfluidics that
has promising effects in culturing, maintaining and differentiation of stem cells. Although, an
increasing number of evidences have supported the fact that despite of offering a wide range of
advantages in this area, significant limitations exist while employing this technique for stem cell
culture, maintenance and differentiation [8]. Microfluidic systems plays important role in the
biological systems through controlled reaction chambers, high throughput arrays and micro-
positioning systems. An evident benefit of microfluidics is that, particularly for high throughput
assays, it offers economy in terms of reagent use [12]. This economy will only be realized, of
course, if the manufacture of devices is also cheap [8]. In microfluidics, the material cell surface
is still an issue from a biological perspective. Polydimethylsiloxane (PMDS) is usually used in
making microchips [14] since it is cheap, gas permeable, optically transparent, and can be
manipulated outside a clean room. Although, many evidences have suggested the use of PDMS
chips for stem cell studies, some studies believes that needs to be significantly modified by
MICROFLUIDICS FOR STEM CELLS CULTURE
performed within the microfluidic systems are also greatly controlled, which means that several
advanced materials having bespoke and uniform properties, can be synthesized in a rapid and
direct way [5]. Microfabrication technology has been found to be progressively with the
development of semiconductor industry in the 20th century. The application of microfabrication
technology in the biotechnology industry was found to be growing at an increasing rate in
different research areas. Initially, it was used in the biotechnology sectors for molecular analyses
of DNA and protein and gradually with time, its application was expanded with different
specialized areas related to cell culture. The technology has facilitated fabrication of structures,
liquid manipulation of a tiny amount of liquid and usage of expensive reagents in small amounts.
Because of these benefits offered by this technology, it is anticipated that it will generate fresh
cell culture apps including stem cells. Although, microfluidics contributes to achieve success and
improved outcome in controlling culture environment thereby promoting stem cell culture,
maintenance and differentiation, its application in the use in the human stem cell studies is still
limited. Evidences have shown the introduction of fully functional organs and the fabricating
complicated 3D structure mimicking an intact organ is still challenging and difficult through this
approach [6]. In addition to that, the culture conditions that are available in current microfluidic
technology are found to be limited to conventional 2D cultures and also culturing of cellular
aggregate in the microfluidic device is difficult. Intervention strategies are required to be
formulated addressing these issues by incorporating interdisciplinary approaches involving
mechanical engineering, device design, material science and cell biology [7] [11].
The literature review is conducted by searching articles related to the topic “Microfluidics
for Stem Cells Culture, Maintenance and Differentiation” and the articles that had relevant and
conclusive results are chosen. Many articles have highlighted the use of conventional plastic
culture plates that were used traditionally in this area, while others have highlighted how recent
advancements have substituted the conventional methods through the use of microfluidics that
has promising effects in culturing, maintaining and differentiation of stem cells. Although, an
increasing number of evidences have supported the fact that despite of offering a wide range of
advantages in this area, significant limitations exist while employing this technique for stem cell
culture, maintenance and differentiation [8]. Microfluidic systems plays important role in the
biological systems through controlled reaction chambers, high throughput arrays and micro-
positioning systems. An evident benefit of microfluidics is that, particularly for high throughput
assays, it offers economy in terms of reagent use [12]. This economy will only be realized, of
course, if the manufacture of devices is also cheap [8]. In microfluidics, the material cell surface
is still an issue from a biological perspective. Polydimethylsiloxane (PMDS) is usually used in
making microchips [14] since it is cheap, gas permeable, optically transparent, and can be
manipulated outside a clean room. Although, many evidences have suggested the use of PDMS
chips for stem cell studies, some studies believes that needs to be significantly modified by
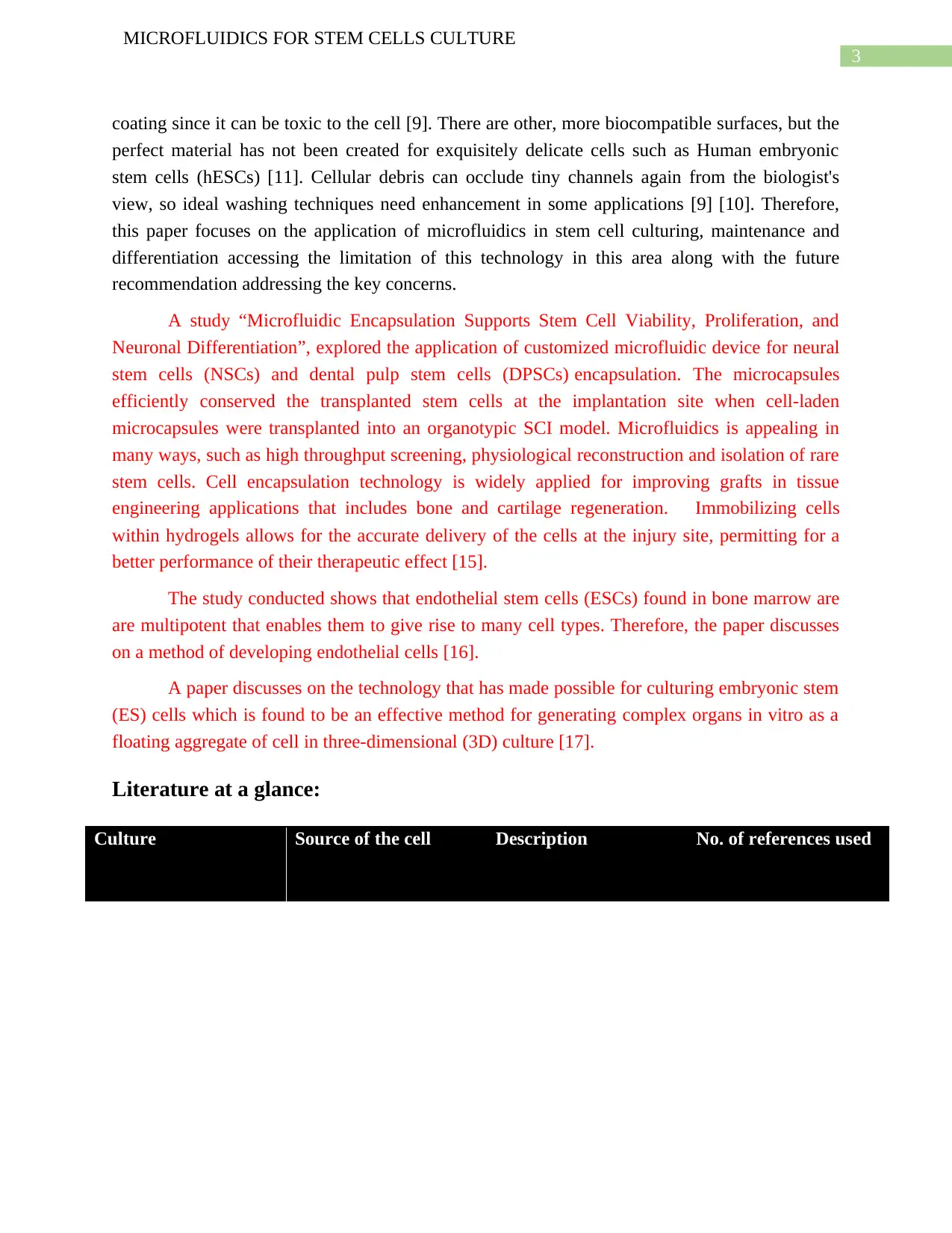
3
MICROFLUIDICS FOR STEM CELLS CULTURE
coating since it can be toxic to the cell [9]. There are other, more biocompatible surfaces, but the
perfect material has not been created for exquisitely delicate cells such as Human embryonic
stem cells (hESCs) [11]. Cellular debris can occlude tiny channels again from the biologist's
view, so ideal washing techniques need enhancement in some applications [9] [10]. Therefore,
this paper focuses on the application of microfluidics in stem cell culturing, maintenance and
differentiation accessing the limitation of this technology in this area along with the future
recommendation addressing the key concerns.
A study “Microfluidic Encapsulation Supports Stem Cell Viability, Proliferation, and
Neuronal Differentiation”, explored the application of customized microfluidic device for neural
stem cells (NSCs) and dental pulp stem cells (DPSCs) encapsulation. The microcapsules
efficiently conserved the transplanted stem cells at the implantation site when cell-laden
microcapsules were transplanted into an organotypic SCI model. Microfluidics is appealing in
many ways, such as high throughput screening, physiological reconstruction and isolation of rare
stem cells. Cell encapsulation technology is widely applied for improving grafts in tissue
engineering applications that includes bone and cartilage regeneration. Immobilizing cells
within hydrogels allows for the accurate delivery of the cells at the injury site, permitting for a
better performance of their therapeutic effect [15].
The study conducted shows that endothelial stem cells (ESCs) found in bone marrow are
are multipotent that enables them to give rise to many cell types. Therefore, the paper discusses
on a method of developing endothelial cells [16].
A paper discusses on the technology that has made possible for culturing embryonic stem
(ES) cells which is found to be an effective method for generating complex organs in vitro as a
floating aggregate of cell in three-dimensional (3D) culture [17].
Literature at a glance:
Culture Source of the cell Description No. of references used
MICROFLUIDICS FOR STEM CELLS CULTURE
coating since it can be toxic to the cell [9]. There are other, more biocompatible surfaces, but the
perfect material has not been created for exquisitely delicate cells such as Human embryonic
stem cells (hESCs) [11]. Cellular debris can occlude tiny channels again from the biologist's
view, so ideal washing techniques need enhancement in some applications [9] [10]. Therefore,
this paper focuses on the application of microfluidics in stem cell culturing, maintenance and
differentiation accessing the limitation of this technology in this area along with the future
recommendation addressing the key concerns.
A study “Microfluidic Encapsulation Supports Stem Cell Viability, Proliferation, and
Neuronal Differentiation”, explored the application of customized microfluidic device for neural
stem cells (NSCs) and dental pulp stem cells (DPSCs) encapsulation. The microcapsules
efficiently conserved the transplanted stem cells at the implantation site when cell-laden
microcapsules were transplanted into an organotypic SCI model. Microfluidics is appealing in
many ways, such as high throughput screening, physiological reconstruction and isolation of rare
stem cells. Cell encapsulation technology is widely applied for improving grafts in tissue
engineering applications that includes bone and cartilage regeneration. Immobilizing cells
within hydrogels allows for the accurate delivery of the cells at the injury site, permitting for a
better performance of their therapeutic effect [15].
The study conducted shows that endothelial stem cells (ESCs) found in bone marrow are
are multipotent that enables them to give rise to many cell types. Therefore, the paper discusses
on a method of developing endothelial cells [16].
A paper discusses on the technology that has made possible for culturing embryonic stem
(ES) cells which is found to be an effective method for generating complex organs in vitro as a
floating aggregate of cell in three-dimensional (3D) culture [17].
Literature at a glance:
Culture Source of the cell Description No. of references used
Secure Best Marks with AI Grader
Need help grading? Try our AI Grader for instant feedback on your assignments.
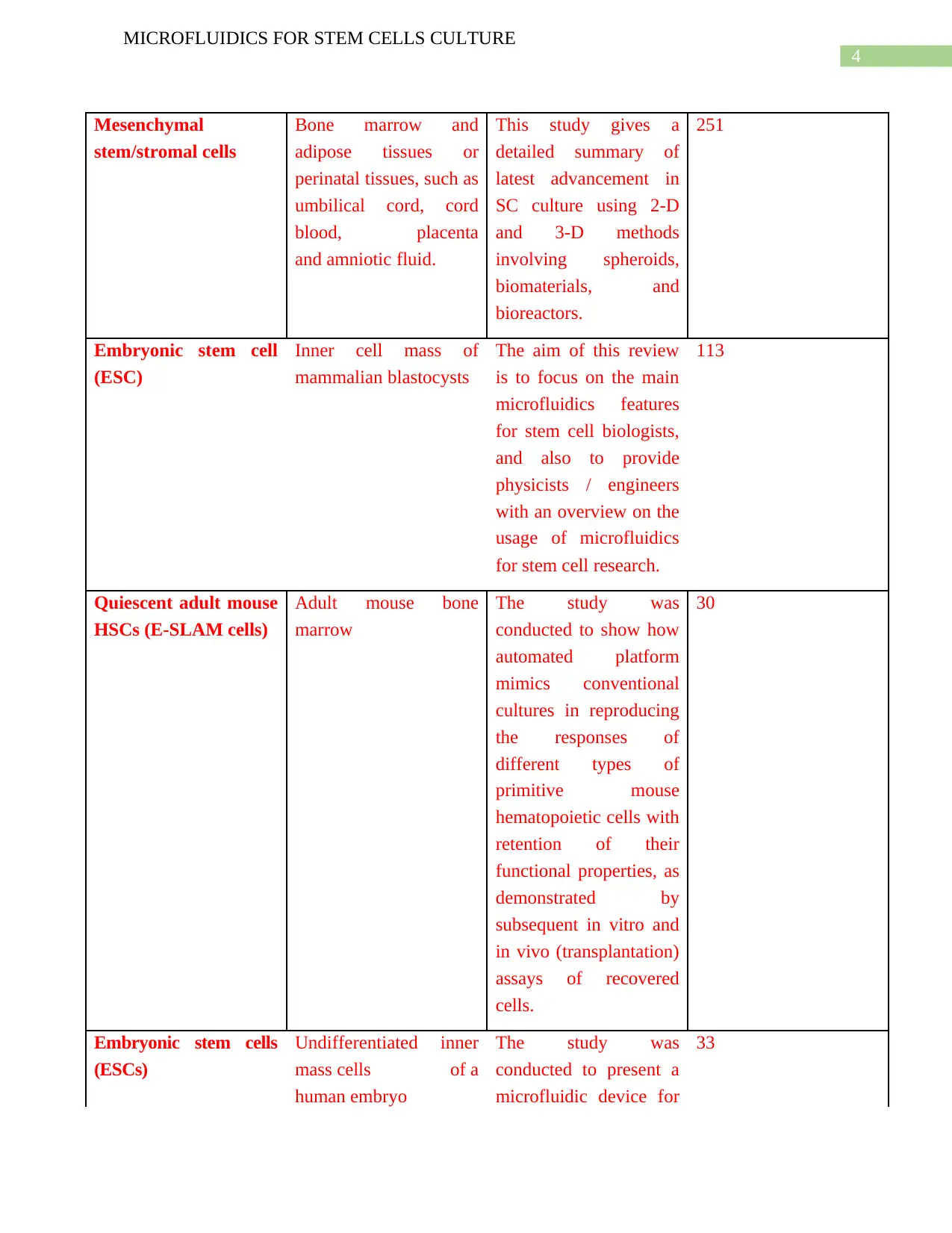
4
MICROFLUIDICS FOR STEM CELLS CULTURE
Mesenchymal
stem/stromal cells
Bone marrow and
adipose tissues or
perinatal tissues, such as
umbilical cord, cord
blood, placenta
and amniotic fluid.
This study gives a
detailed summary of
latest advancement in
SC culture using 2-D
and 3-D methods
involving spheroids,
biomaterials, and
bioreactors.
251
Embryonic stem cell
(ESC)
Inner cell mass of
mammalian blastocysts
The aim of this review
is to focus on the main
microfluidics features
for stem cell biologists,
and also to provide
physicists / engineers
with an overview on the
usage of microfluidics
for stem cell research.
113
Quiescent adult mouse
HSCs (E-SLAM cells)
Adult mouse bone
marrow
The study was
conducted to show how
automated platform
mimics conventional
cultures in reproducing
the responses of
different types of
primitive mouse
hematopoietic cells with
retention of their
functional properties, as
demonstrated by
subsequent in vitro and
in vivo (transplantation)
assays of recovered
cells.
30
Embryonic stem cells
(ESCs)
Undifferentiated inner
mass cells of a
human embryo
The study was
conducted to present a
microfluidic device for
33
MICROFLUIDICS FOR STEM CELLS CULTURE
Mesenchymal
stem/stromal cells
Bone marrow and
adipose tissues or
perinatal tissues, such as
umbilical cord, cord
blood, placenta
and amniotic fluid.
This study gives a
detailed summary of
latest advancement in
SC culture using 2-D
and 3-D methods
involving spheroids,
biomaterials, and
bioreactors.
251
Embryonic stem cell
(ESC)
Inner cell mass of
mammalian blastocysts
The aim of this review
is to focus on the main
microfluidics features
for stem cell biologists,
and also to provide
physicists / engineers
with an overview on the
usage of microfluidics
for stem cell research.
113
Quiescent adult mouse
HSCs (E-SLAM cells)
Adult mouse bone
marrow
The study was
conducted to show how
automated platform
mimics conventional
cultures in reproducing
the responses of
different types of
primitive mouse
hematopoietic cells with
retention of their
functional properties, as
demonstrated by
subsequent in vitro and
in vivo (transplantation)
assays of recovered
cells.
30
Embryonic stem cells
(ESCs)
Undifferentiated inner
mass cells of a
human embryo
The study was
conducted to present a
microfluidic device for
33
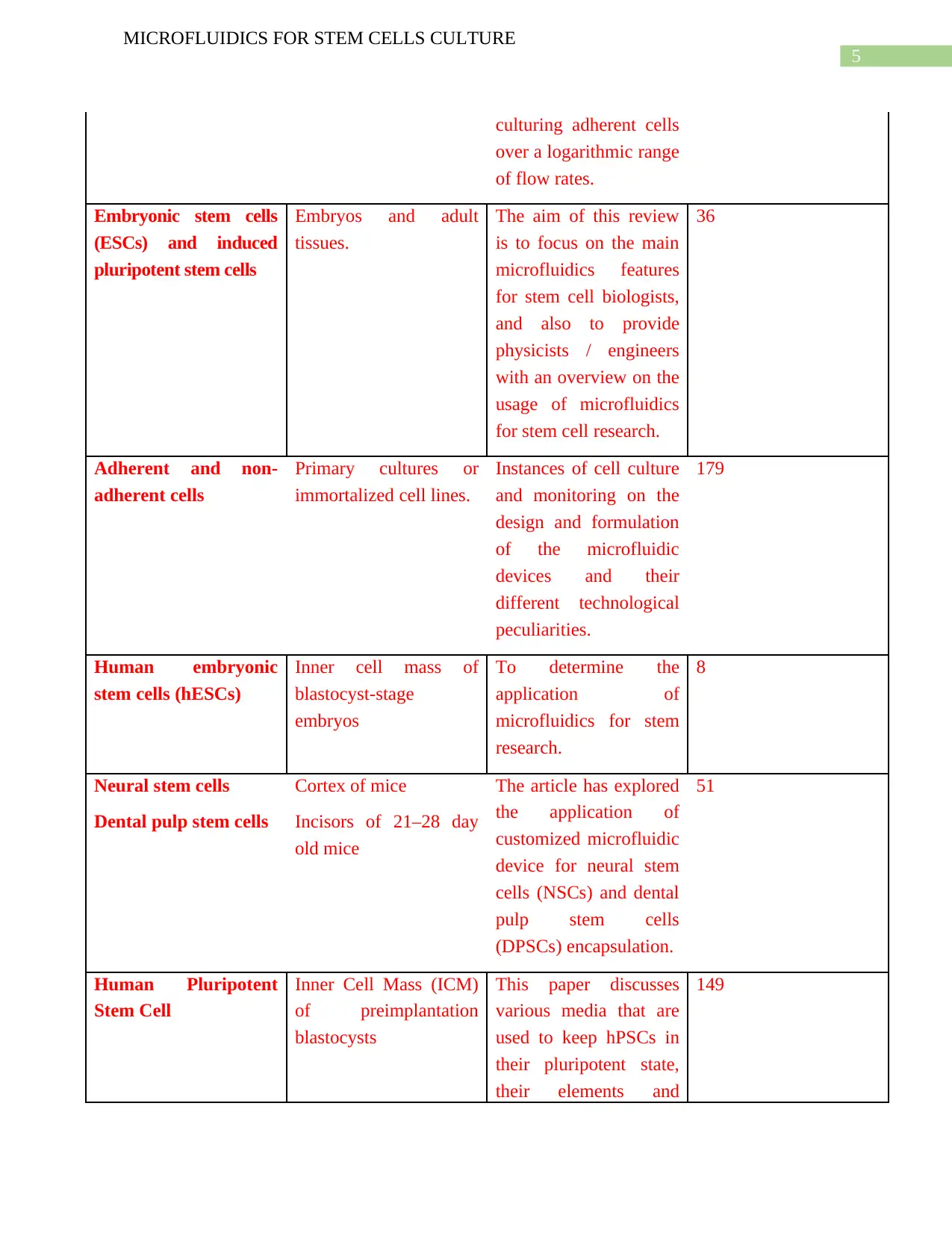
5
MICROFLUIDICS FOR STEM CELLS CULTURE
culturing adherent cells
over a logarithmic range
of flow rates.
Embryonic stem cells
(ESCs) and induced
pluripotent stem cells
Embryos and adult
tissues.
The aim of this review
is to focus on the main
microfluidics features
for stem cell biologists,
and also to provide
physicists / engineers
with an overview on the
usage of microfluidics
for stem cell research.
36
Adherent and non-
adherent cells
Primary cultures or
immortalized cell lines.
Instances of cell culture
and monitoring on the
design and formulation
of the microfluidic
devices and their
different technological
peculiarities.
179
Human embryonic
stem cells (hESCs)
Inner cell mass of
blastocyst-stage
embryos
To determine the
application of
microfluidics for stem
research.
8
Neural stem cells
Dental pulp stem cells
Cortex of mice
Incisors of 21–28 day
old mice
The article has explored
the application of
customized microfluidic
device for neural stem
cells (NSCs) and dental
pulp stem cells
(DPSCs) encapsulation.
51
Human Pluripotent
Stem Cell
Inner Cell Mass (ICM)
of preimplantation
blastocysts
This paper discusses
various media that are
used to keep hPSCs in
their pluripotent state,
their elements and
149
MICROFLUIDICS FOR STEM CELLS CULTURE
culturing adherent cells
over a logarithmic range
of flow rates.
Embryonic stem cells
(ESCs) and induced
pluripotent stem cells
Embryos and adult
tissues.
The aim of this review
is to focus on the main
microfluidics features
for stem cell biologists,
and also to provide
physicists / engineers
with an overview on the
usage of microfluidics
for stem cell research.
36
Adherent and non-
adherent cells
Primary cultures or
immortalized cell lines.
Instances of cell culture
and monitoring on the
design and formulation
of the microfluidic
devices and their
different technological
peculiarities.
179
Human embryonic
stem cells (hESCs)
Inner cell mass of
blastocyst-stage
embryos
To determine the
application of
microfluidics for stem
research.
8
Neural stem cells
Dental pulp stem cells
Cortex of mice
Incisors of 21–28 day
old mice
The article has explored
the application of
customized microfluidic
device for neural stem
cells (NSCs) and dental
pulp stem cells
(DPSCs) encapsulation.
51
Human Pluripotent
Stem Cell
Inner Cell Mass (ICM)
of preimplantation
blastocysts
This paper discusses
various media that are
used to keep hPSCs in
their pluripotent state,
their elements and
149
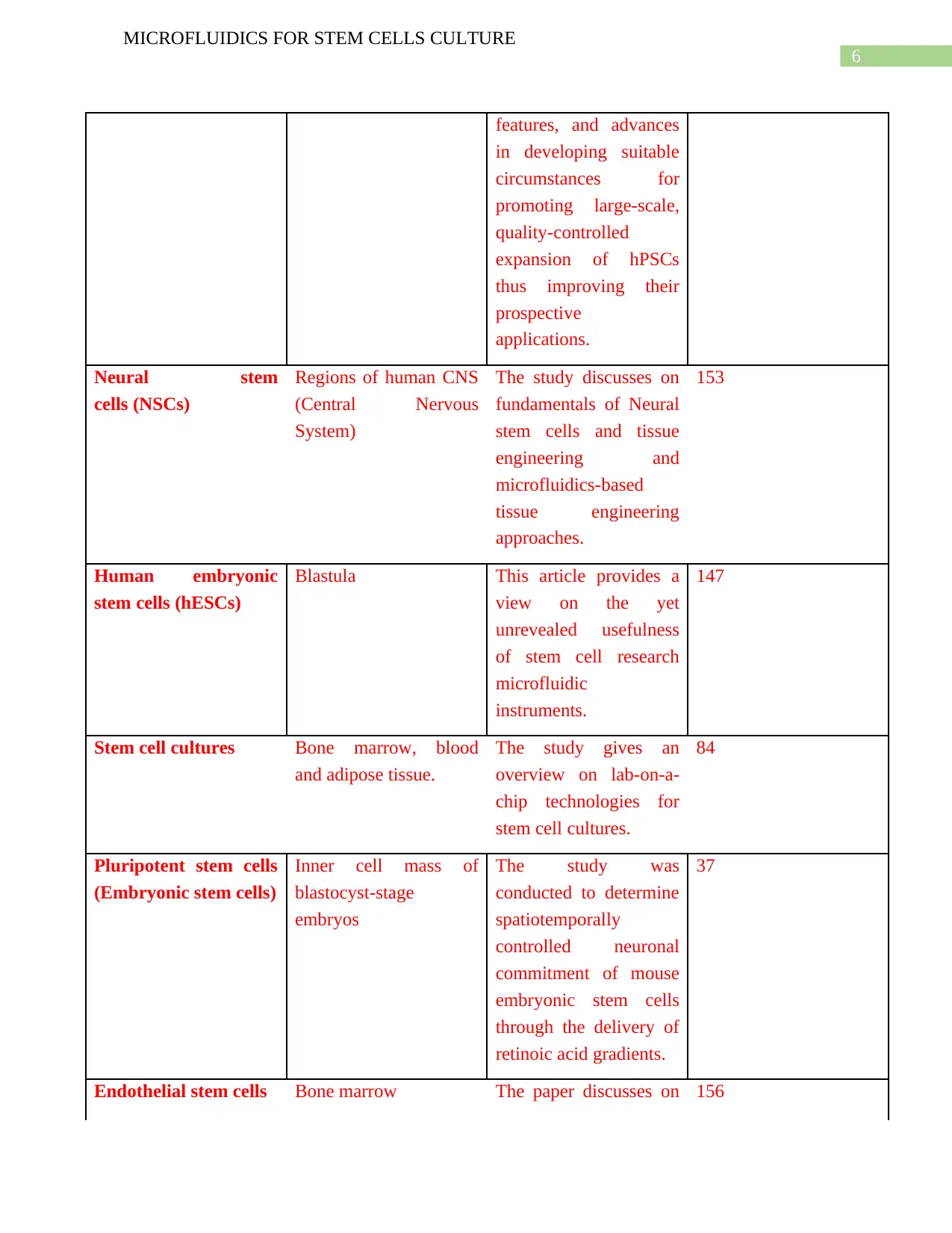
6
MICROFLUIDICS FOR STEM CELLS CULTURE
features, and advances
in developing suitable
circumstances for
promoting large-scale,
quality-controlled
expansion of hPSCs
thus improving their
prospective
applications.
Neural stem
cells (NSCs)
Regions of human CNS
(Central Nervous
System)
The study discusses on
fundamentals of Neural
stem cells and tissue
engineering and
microfluidics-based
tissue engineering
approaches.
153
Human embryonic
stem cells (hESCs)
Blastula This article provides a
view on the yet
unrevealed usefulness
of stem cell research
microfluidic
instruments.
147
Stem cell cultures Bone marrow, blood
and adipose tissue.
The study gives an
overview on lab-on-a-
chip technologies for
stem cell cultures.
84
Pluripotent stem cells
(Embryonic stem cells)
Inner cell mass of
blastocyst-stage
embryos
The study was
conducted to determine
spatiotemporally
controlled neuronal
commitment of mouse
embryonic stem cells
through the delivery of
retinoic acid gradients.
37
Endothelial stem cells Bone marrow The paper discusses on 156
MICROFLUIDICS FOR STEM CELLS CULTURE
features, and advances
in developing suitable
circumstances for
promoting large-scale,
quality-controlled
expansion of hPSCs
thus improving their
prospective
applications.
Neural stem
cells (NSCs)
Regions of human CNS
(Central Nervous
System)
The study discusses on
fundamentals of Neural
stem cells and tissue
engineering and
microfluidics-based
tissue engineering
approaches.
153
Human embryonic
stem cells (hESCs)
Blastula This article provides a
view on the yet
unrevealed usefulness
of stem cell research
microfluidic
instruments.
147
Stem cell cultures Bone marrow, blood
and adipose tissue.
The study gives an
overview on lab-on-a-
chip technologies for
stem cell cultures.
84
Pluripotent stem cells
(Embryonic stem cells)
Inner cell mass of
blastocyst-stage
embryos
The study was
conducted to determine
spatiotemporally
controlled neuronal
commitment of mouse
embryonic stem cells
through the delivery of
retinoic acid gradients.
37
Endothelial stem cells Bone marrow The paper discusses on 156
Paraphrase This Document
Need a fresh take? Get an instant paraphrase of this document with our AI Paraphraser
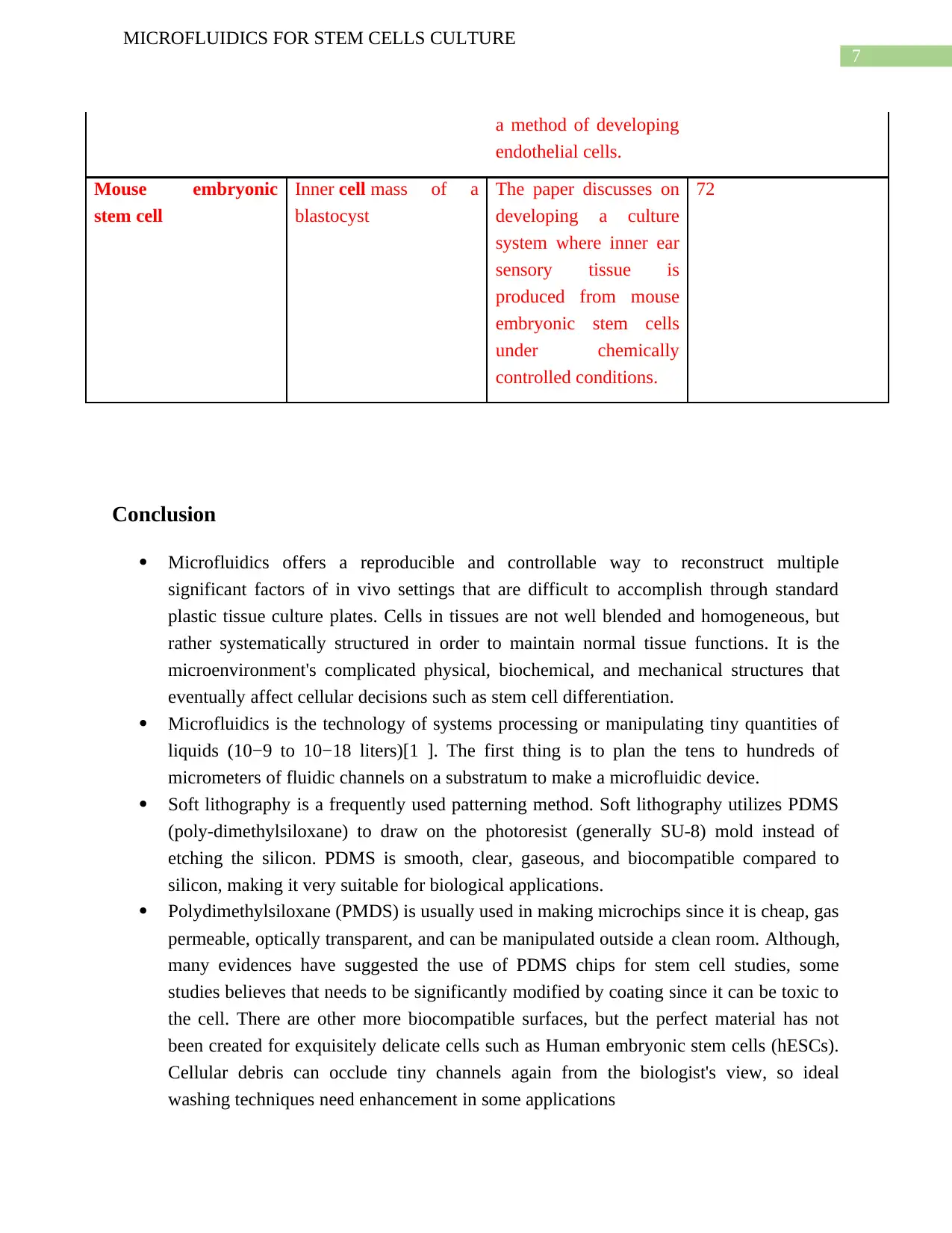
7
MICROFLUIDICS FOR STEM CELLS CULTURE
a method of developing
endothelial cells.
Mouse embryonic
stem cell
Inner cell mass of a
blastocyst
The paper discusses on
developing a culture
system where inner ear
sensory tissue is
produced from mouse
embryonic stem cells
under chemically
controlled conditions.
72
Conclusion
Microfluidics offers a reproducible and controllable way to reconstruct multiple
significant factors of in vivo settings that are difficult to accomplish through standard
plastic tissue culture plates. Cells in tissues are not well blended and homogeneous, but
rather systematically structured in order to maintain normal tissue functions. It is the
microenvironment's complicated physical, biochemical, and mechanical structures that
eventually affect cellular decisions such as stem cell differentiation.
Microfluidics is the technology of systems processing or manipulating tiny quantities of
liquids (10−9 to 10−18 liters)[1 ]. The first thing is to plan the tens to hundreds of
micrometers of fluidic channels on a substratum to make a microfluidic device.
Soft lithography is a frequently used patterning method. Soft lithography utilizes PDMS
(poly-dimethylsiloxane) to draw on the photoresist (generally SU-8) mold instead of
etching the silicon. PDMS is smooth, clear, gaseous, and biocompatible compared to
silicon, making it very suitable for biological applications.
Polydimethylsiloxane (PMDS) is usually used in making microchips since it is cheap, gas
permeable, optically transparent, and can be manipulated outside a clean room. Although,
many evidences have suggested the use of PDMS chips for stem cell studies, some
studies believes that needs to be significantly modified by coating since it can be toxic to
the cell. There are other more biocompatible surfaces, but the perfect material has not
been created for exquisitely delicate cells such as Human embryonic stem cells (hESCs).
Cellular debris can occlude tiny channels again from the biologist's view, so ideal
washing techniques need enhancement in some applications
MICROFLUIDICS FOR STEM CELLS CULTURE
a method of developing
endothelial cells.
Mouse embryonic
stem cell
Inner cell mass of a
blastocyst
The paper discusses on
developing a culture
system where inner ear
sensory tissue is
produced from mouse
embryonic stem cells
under chemically
controlled conditions.
72
Conclusion
Microfluidics offers a reproducible and controllable way to reconstruct multiple
significant factors of in vivo settings that are difficult to accomplish through standard
plastic tissue culture plates. Cells in tissues are not well blended and homogeneous, but
rather systematically structured in order to maintain normal tissue functions. It is the
microenvironment's complicated physical, biochemical, and mechanical structures that
eventually affect cellular decisions such as stem cell differentiation.
Microfluidics is the technology of systems processing or manipulating tiny quantities of
liquids (10−9 to 10−18 liters)[1 ]. The first thing is to plan the tens to hundreds of
micrometers of fluidic channels on a substratum to make a microfluidic device.
Soft lithography is a frequently used patterning method. Soft lithography utilizes PDMS
(poly-dimethylsiloxane) to draw on the photoresist (generally SU-8) mold instead of
etching the silicon. PDMS is smooth, clear, gaseous, and biocompatible compared to
silicon, making it very suitable for biological applications.
Polydimethylsiloxane (PMDS) is usually used in making microchips since it is cheap, gas
permeable, optically transparent, and can be manipulated outside a clean room. Although,
many evidences have suggested the use of PDMS chips for stem cell studies, some
studies believes that needs to be significantly modified by coating since it can be toxic to
the cell. There are other more biocompatible surfaces, but the perfect material has not
been created for exquisitely delicate cells such as Human embryonic stem cells (hESCs).
Cellular debris can occlude tiny channels again from the biologist's view, so ideal
washing techniques need enhancement in some applications
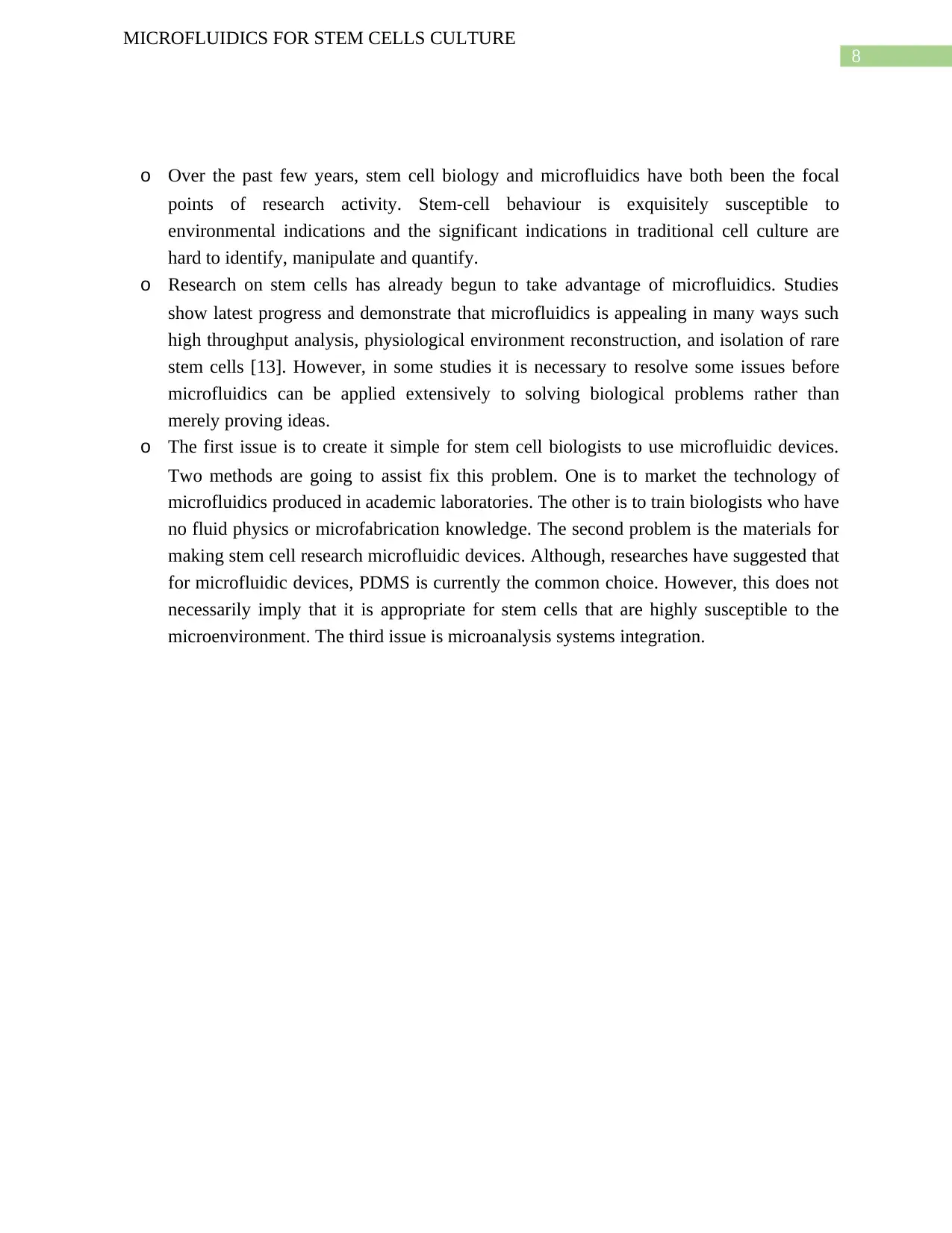
8
MICROFLUIDICS FOR STEM CELLS CULTURE
o Over the past few years, stem cell biology and microfluidics have both been the focal
points of research activity. Stem-cell behaviour is exquisitely susceptible to
environmental indications and the significant indications in traditional cell culture are
hard to identify, manipulate and quantify.
o Research on stem cells has already begun to take advantage of microfluidics. Studies
show latest progress and demonstrate that microfluidics is appealing in many ways such
high throughput analysis, physiological environment reconstruction, and isolation of rare
stem cells [13]. However, in some studies it is necessary to resolve some issues before
microfluidics can be applied extensively to solving biological problems rather than
merely proving ideas.
o The first issue is to create it simple for stem cell biologists to use microfluidic devices.
Two methods are going to assist fix this problem. One is to market the technology of
microfluidics produced in academic laboratories. The other is to train biologists who have
no fluid physics or microfabrication knowledge. The second problem is the materials for
making stem cell research microfluidic devices. Although, researches have suggested that
for microfluidic devices, PDMS is currently the common choice. However, this does not
necessarily imply that it is appropriate for stem cells that are highly susceptible to the
microenvironment. The third issue is microanalysis systems integration.
MICROFLUIDICS FOR STEM CELLS CULTURE
o Over the past few years, stem cell biology and microfluidics have both been the focal
points of research activity. Stem-cell behaviour is exquisitely susceptible to
environmental indications and the significant indications in traditional cell culture are
hard to identify, manipulate and quantify.
o Research on stem cells has already begun to take advantage of microfluidics. Studies
show latest progress and demonstrate that microfluidics is appealing in many ways such
high throughput analysis, physiological environment reconstruction, and isolation of rare
stem cells [13]. However, in some studies it is necessary to resolve some issues before
microfluidics can be applied extensively to solving biological problems rather than
merely proving ideas.
o The first issue is to create it simple for stem cell biologists to use microfluidic devices.
Two methods are going to assist fix this problem. One is to market the technology of
microfluidics produced in academic laboratories. The other is to train biologists who have
no fluid physics or microfabrication knowledge. The second problem is the materials for
making stem cell research microfluidic devices. Although, researches have suggested that
for microfluidic devices, PDMS is currently the common choice. However, this does not
necessarily imply that it is appropriate for stem cells that are highly susceptible to the
microenvironment. The third issue is microanalysis systems integration.
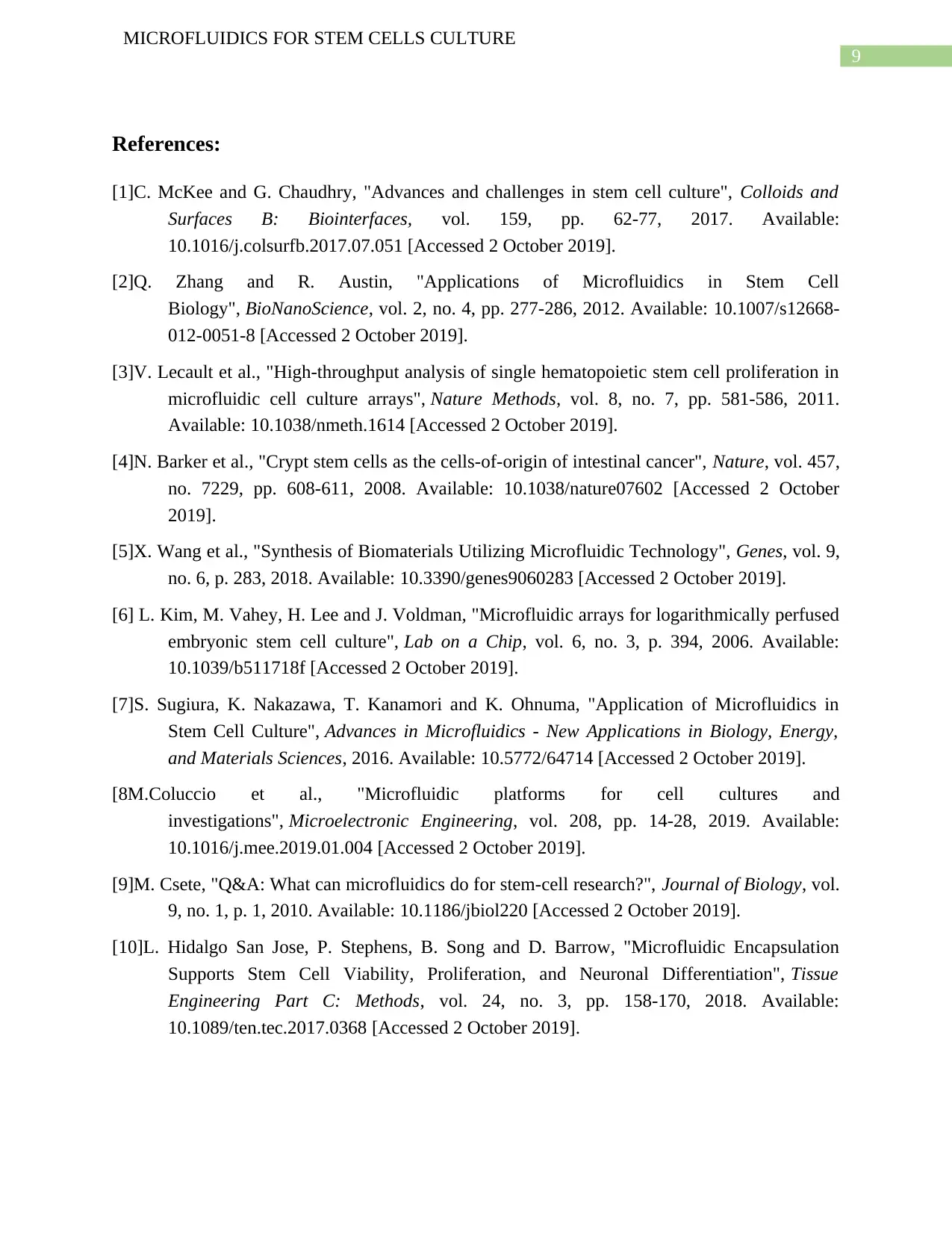
9
MICROFLUIDICS FOR STEM CELLS CULTURE
References:
[1]C. McKee and G. Chaudhry, "Advances and challenges in stem cell culture", Colloids and
Surfaces B: Biointerfaces, vol. 159, pp. 62-77, 2017. Available:
10.1016/j.colsurfb.2017.07.051 [Accessed 2 October 2019].
[2]Q. Zhang and R. Austin, "Applications of Microfluidics in Stem Cell
Biology", BioNanoScience, vol. 2, no. 4, pp. 277-286, 2012. Available: 10.1007/s12668-
012-0051-8 [Accessed 2 October 2019].
[3]V. Lecault et al., "High-throughput analysis of single hematopoietic stem cell proliferation in
microfluidic cell culture arrays", Nature Methods, vol. 8, no. 7, pp. 581-586, 2011.
Available: 10.1038/nmeth.1614 [Accessed 2 October 2019].
[4]N. Barker et al., "Crypt stem cells as the cells-of-origin of intestinal cancer", Nature, vol. 457,
no. 7229, pp. 608-611, 2008. Available: 10.1038/nature07602 [Accessed 2 October
2019].
[5]X. Wang et al., "Synthesis of Biomaterials Utilizing Microfluidic Technology", Genes, vol. 9,
no. 6, p. 283, 2018. Available: 10.3390/genes9060283 [Accessed 2 October 2019].
[6] L. Kim, M. Vahey, H. Lee and J. Voldman, "Microfluidic arrays for logarithmically perfused
embryonic stem cell culture", Lab on a Chip, vol. 6, no. 3, p. 394, 2006. Available:
10.1039/b511718f [Accessed 2 October 2019].
[7]S. Sugiura, K. Nakazawa, T. Kanamori and K. Ohnuma, "Application of Microfluidics in
Stem Cell Culture", Advances in Microfluidics - New Applications in Biology, Energy,
and Materials Sciences, 2016. Available: 10.5772/64714 [Accessed 2 October 2019].
[8M.Coluccio et al., "Microfluidic platforms for cell cultures and
investigations", Microelectronic Engineering, vol. 208, pp. 14-28, 2019. Available:
10.1016/j.mee.2019.01.004 [Accessed 2 October 2019].
[9]M. Csete, "Q&A: What can microfluidics do for stem-cell research?", Journal of Biology, vol.
9, no. 1, p. 1, 2010. Available: 10.1186/jbiol220 [Accessed 2 October 2019].
[10]L. Hidalgo San Jose, P. Stephens, B. Song and D. Barrow, "Microfluidic Encapsulation
Supports Stem Cell Viability, Proliferation, and Neuronal Differentiation", Tissue
Engineering Part C: Methods, vol. 24, no. 3, pp. 158-170, 2018. Available:
10.1089/ten.tec.2017.0368 [Accessed 2 October 2019].
MICROFLUIDICS FOR STEM CELLS CULTURE
References:
[1]C. McKee and G. Chaudhry, "Advances and challenges in stem cell culture", Colloids and
Surfaces B: Biointerfaces, vol. 159, pp. 62-77, 2017. Available:
10.1016/j.colsurfb.2017.07.051 [Accessed 2 October 2019].
[2]Q. Zhang and R. Austin, "Applications of Microfluidics in Stem Cell
Biology", BioNanoScience, vol. 2, no. 4, pp. 277-286, 2012. Available: 10.1007/s12668-
012-0051-8 [Accessed 2 October 2019].
[3]V. Lecault et al., "High-throughput analysis of single hematopoietic stem cell proliferation in
microfluidic cell culture arrays", Nature Methods, vol. 8, no. 7, pp. 581-586, 2011.
Available: 10.1038/nmeth.1614 [Accessed 2 October 2019].
[4]N. Barker et al., "Crypt stem cells as the cells-of-origin of intestinal cancer", Nature, vol. 457,
no. 7229, pp. 608-611, 2008. Available: 10.1038/nature07602 [Accessed 2 October
2019].
[5]X. Wang et al., "Synthesis of Biomaterials Utilizing Microfluidic Technology", Genes, vol. 9,
no. 6, p. 283, 2018. Available: 10.3390/genes9060283 [Accessed 2 October 2019].
[6] L. Kim, M. Vahey, H. Lee and J. Voldman, "Microfluidic arrays for logarithmically perfused
embryonic stem cell culture", Lab on a Chip, vol. 6, no. 3, p. 394, 2006. Available:
10.1039/b511718f [Accessed 2 October 2019].
[7]S. Sugiura, K. Nakazawa, T. Kanamori and K. Ohnuma, "Application of Microfluidics in
Stem Cell Culture", Advances in Microfluidics - New Applications in Biology, Energy,
and Materials Sciences, 2016. Available: 10.5772/64714 [Accessed 2 October 2019].
[8M.Coluccio et al., "Microfluidic platforms for cell cultures and
investigations", Microelectronic Engineering, vol. 208, pp. 14-28, 2019. Available:
10.1016/j.mee.2019.01.004 [Accessed 2 October 2019].
[9]M. Csete, "Q&A: What can microfluidics do for stem-cell research?", Journal of Biology, vol.
9, no. 1, p. 1, 2010. Available: 10.1186/jbiol220 [Accessed 2 October 2019].
[10]L. Hidalgo San Jose, P. Stephens, B. Song and D. Barrow, "Microfluidic Encapsulation
Supports Stem Cell Viability, Proliferation, and Neuronal Differentiation", Tissue
Engineering Part C: Methods, vol. 24, no. 3, pp. 158-170, 2018. Available:
10.1089/ten.tec.2017.0368 [Accessed 2 October 2019].
Secure Best Marks with AI Grader
Need help grading? Try our AI Grader for instant feedback on your assignments.
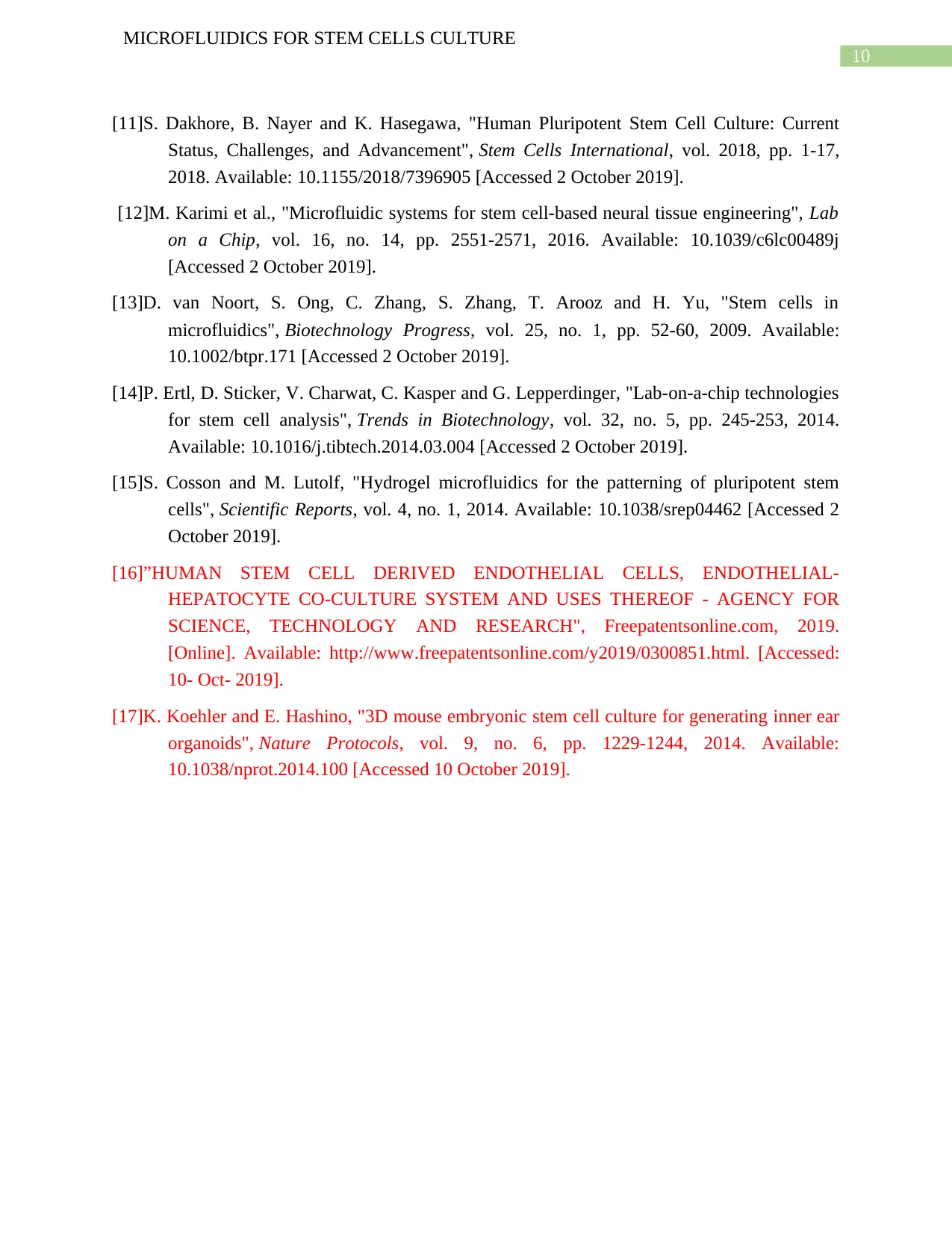
10
MICROFLUIDICS FOR STEM CELLS CULTURE
[11]S. Dakhore, B. Nayer and K. Hasegawa, "Human Pluripotent Stem Cell Culture: Current
Status, Challenges, and Advancement", Stem Cells International, vol. 2018, pp. 1-17,
2018. Available: 10.1155/2018/7396905 [Accessed 2 October 2019].
[12]M. Karimi et al., "Microfluidic systems for stem cell-based neural tissue engineering", Lab
on a Chip, vol. 16, no. 14, pp. 2551-2571, 2016. Available: 10.1039/c6lc00489j
[Accessed 2 October 2019].
[13]D. van Noort, S. Ong, C. Zhang, S. Zhang, T. Arooz and H. Yu, "Stem cells in
microfluidics", Biotechnology Progress, vol. 25, no. 1, pp. 52-60, 2009. Available:
10.1002/btpr.171 [Accessed 2 October 2019].
[14]P. Ertl, D. Sticker, V. Charwat, C. Kasper and G. Lepperdinger, "Lab-on-a-chip technologies
for stem cell analysis", Trends in Biotechnology, vol. 32, no. 5, pp. 245-253, 2014.
Available: 10.1016/j.tibtech.2014.03.004 [Accessed 2 October 2019].
[15]S. Cosson and M. Lutolf, "Hydrogel microfluidics for the patterning of pluripotent stem
cells", Scientific Reports, vol. 4, no. 1, 2014. Available: 10.1038/srep04462 [Accessed 2
October 2019].
[16]”HUMAN STEM CELL DERIVED ENDOTHELIAL CELLS, ENDOTHELIAL-
HEPATOCYTE CO-CULTURE SYSTEM AND USES THEREOF - AGENCY FOR
SCIENCE, TECHNOLOGY AND RESEARCH", Freepatentsonline.com, 2019.
[Online]. Available: http://www.freepatentsonline.com/y2019/0300851.html. [Accessed:
10- Oct- 2019].
[17]K. Koehler and E. Hashino, "3D mouse embryonic stem cell culture for generating inner ear
organoids", Nature Protocols, vol. 9, no. 6, pp. 1229-1244, 2014. Available:
10.1038/nprot.2014.100 [Accessed 10 October 2019].
MICROFLUIDICS FOR STEM CELLS CULTURE
[11]S. Dakhore, B. Nayer and K. Hasegawa, "Human Pluripotent Stem Cell Culture: Current
Status, Challenges, and Advancement", Stem Cells International, vol. 2018, pp. 1-17,
2018. Available: 10.1155/2018/7396905 [Accessed 2 October 2019].
[12]M. Karimi et al., "Microfluidic systems for stem cell-based neural tissue engineering", Lab
on a Chip, vol. 16, no. 14, pp. 2551-2571, 2016. Available: 10.1039/c6lc00489j
[Accessed 2 October 2019].
[13]D. van Noort, S. Ong, C. Zhang, S. Zhang, T. Arooz and H. Yu, "Stem cells in
microfluidics", Biotechnology Progress, vol. 25, no. 1, pp. 52-60, 2009. Available:
10.1002/btpr.171 [Accessed 2 October 2019].
[14]P. Ertl, D. Sticker, V. Charwat, C. Kasper and G. Lepperdinger, "Lab-on-a-chip technologies
for stem cell analysis", Trends in Biotechnology, vol. 32, no. 5, pp. 245-253, 2014.
Available: 10.1016/j.tibtech.2014.03.004 [Accessed 2 October 2019].
[15]S. Cosson and M. Lutolf, "Hydrogel microfluidics for the patterning of pluripotent stem
cells", Scientific Reports, vol. 4, no. 1, 2014. Available: 10.1038/srep04462 [Accessed 2
October 2019].
[16]”HUMAN STEM CELL DERIVED ENDOTHELIAL CELLS, ENDOTHELIAL-
HEPATOCYTE CO-CULTURE SYSTEM AND USES THEREOF - AGENCY FOR
SCIENCE, TECHNOLOGY AND RESEARCH", Freepatentsonline.com, 2019.
[Online]. Available: http://www.freepatentsonline.com/y2019/0300851.html. [Accessed:
10- Oct- 2019].
[17]K. Koehler and E. Hashino, "3D mouse embryonic stem cell culture for generating inner ear
organoids", Nature Protocols, vol. 9, no. 6, pp. 1229-1244, 2014. Available:
10.1038/nprot.2014.100 [Accessed 10 October 2019].
1 out of 11
![[object Object]](/_next/image/?url=%2F_next%2Fstatic%2Fmedia%2Flogo.6d15ce61.png&w=640&q=75)
Your All-in-One AI-Powered Toolkit for Academic Success.
+13062052269
info@desklib.com
Available 24*7 on WhatsApp / Email
Unlock your academic potential
© 2024 | Zucol Services PVT LTD | All rights reserved.