Nano Starch and their role in development of composite films/coatings: A review
VerifiedAdded on 2023/01/19
|42
|18791
|74
AI Summary
This review explores the role of nano starch in the development of composite films and coatings, specifically in enhancing the shelf life of fruits. It discusses the isolation and characterization of nano starch from different botanical sources and the methods used for its development. The properties of nano starch and its impact on the quality of composite films are also examined.
Contribute Materials
Your contribution can guide someone’s learning journey. Share your
documents today.
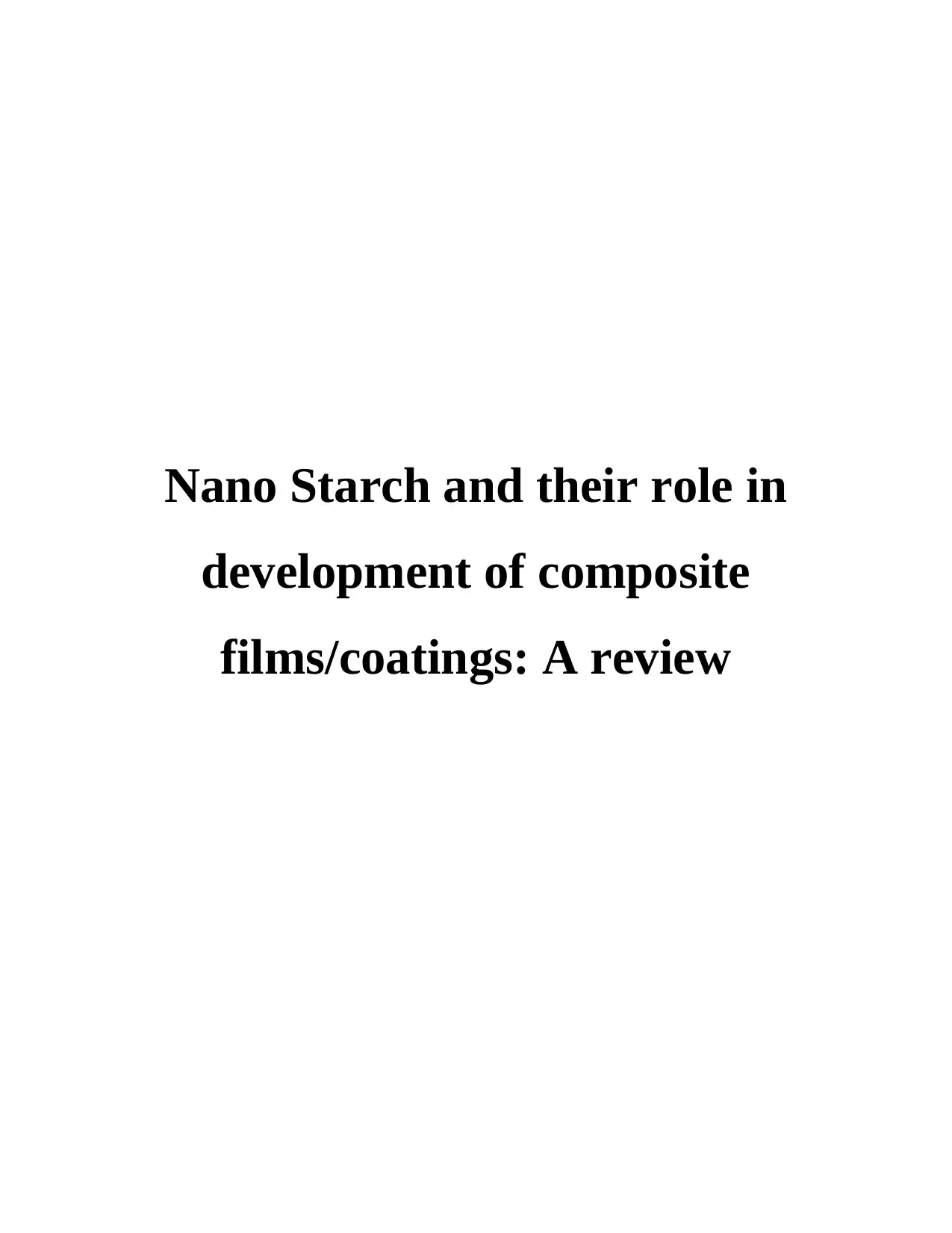
Nano Starch and their role in
development of composite
films/coatings: A review
development of composite
films/coatings: A review
Secure Best Marks with AI Grader
Need help grading? Try our AI Grader for instant feedback on your assignments.
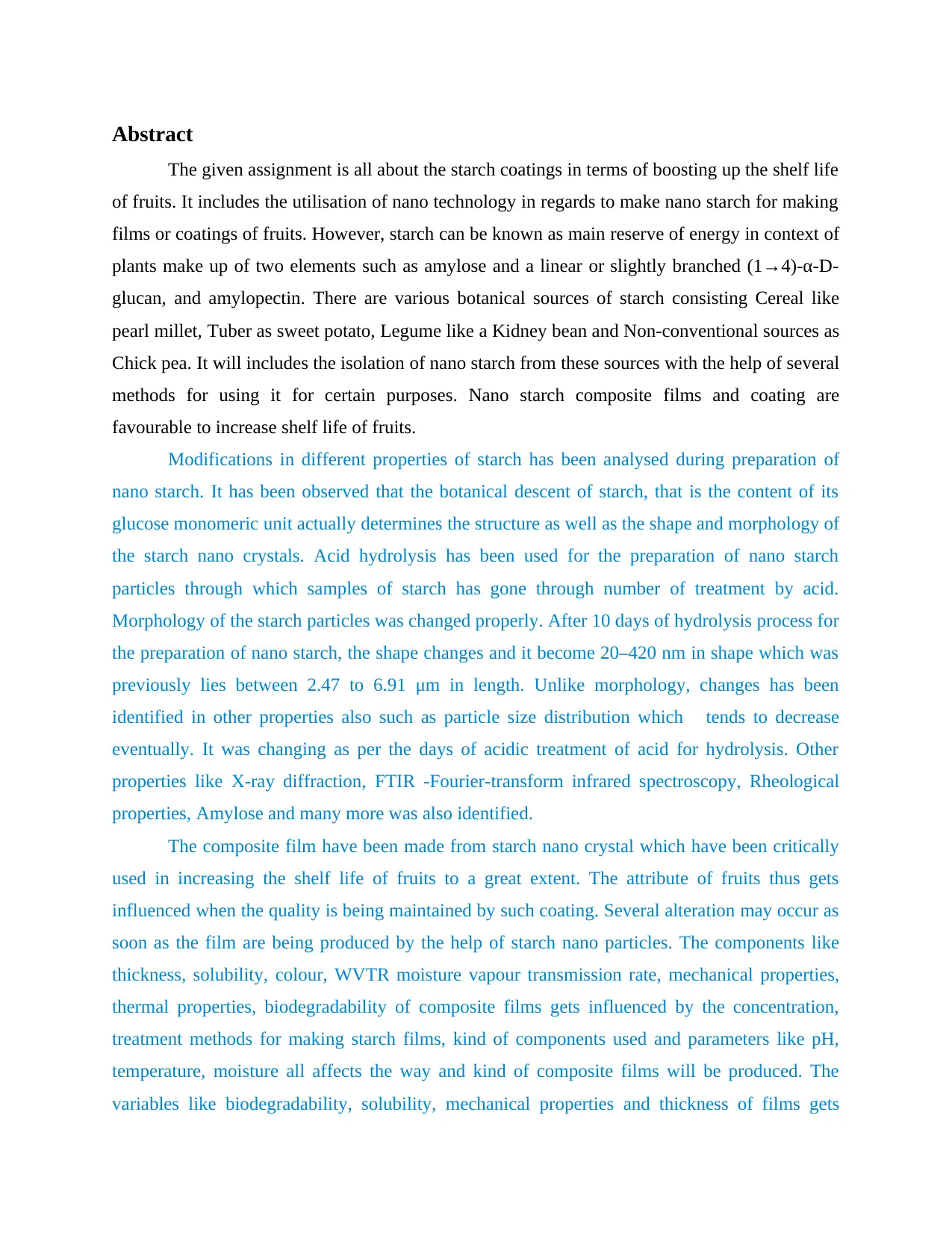
Abstract
The given assignment is all about the starch coatings in terms of boosting up the shelf life
of fruits. It includes the utilisation of nano technology in regards to make nano starch for making
films or coatings of fruits. However, starch can be known as main reserve of energy in context of
plants make up of two elements such as amylose and a linear or slightly branched (1→4)-α-D-
glucan, and amylopectin. There are various botanical sources of starch consisting Cereal like
pearl millet, Tuber as sweet potato, Legume like a Kidney bean and Non-conventional sources as
Chick pea. It will includes the isolation of nano starch from these sources with the help of several
methods for using it for certain purposes. Nano starch composite films and coating are
favourable to increase shelf life of fruits.
Modifications in different properties of starch has been analysed during preparation of
nano starch. It has been observed that the botanical descent of starch, that is the content of its
glucose monomeric unit actually determines the structure as well as the shape and morphology of
the starch nano crystals. Acid hydrolysis has been used for the preparation of nano starch
particles through which samples of starch has gone through number of treatment by acid.
Morphology of the starch particles was changed properly. After 10 days of hydrolysis process for
the preparation of nano starch, the shape changes and it become 20–420 nm in shape which was
previously lies between 2.47 to 6.91 μm in length. Unlike morphology, changes has been
identified in other properties also such as particle size distribution which tends to decrease
eventually. It was changing as per the days of acidic treatment of acid for hydrolysis. Other
properties like X-ray diffraction, FTIR -Fourier-transform infrared spectroscopy, Rheological
properties, Amylose and many more was also identified.
The composite film have been made from starch nano crystal which have been critically
used in increasing the shelf life of fruits to a great extent. The attribute of fruits thus gets
influenced when the quality is being maintained by such coating. Several alteration may occur as
soon as the film are being produced by the help of starch nano particles. The components like
thickness, solubility, colour, WVTR moisture vapour transmission rate, mechanical properties,
thermal properties, biodegradability of composite films gets influenced by the concentration,
treatment methods for making starch films, kind of components used and parameters like pH,
temperature, moisture all affects the way and kind of composite films will be produced. The
variables like biodegradability, solubility, mechanical properties and thickness of films gets
The given assignment is all about the starch coatings in terms of boosting up the shelf life
of fruits. It includes the utilisation of nano technology in regards to make nano starch for making
films or coatings of fruits. However, starch can be known as main reserve of energy in context of
plants make up of two elements such as amylose and a linear or slightly branched (1→4)-α-D-
glucan, and amylopectin. There are various botanical sources of starch consisting Cereal like
pearl millet, Tuber as sweet potato, Legume like a Kidney bean and Non-conventional sources as
Chick pea. It will includes the isolation of nano starch from these sources with the help of several
methods for using it for certain purposes. Nano starch composite films and coating are
favourable to increase shelf life of fruits.
Modifications in different properties of starch has been analysed during preparation of
nano starch. It has been observed that the botanical descent of starch, that is the content of its
glucose monomeric unit actually determines the structure as well as the shape and morphology of
the starch nano crystals. Acid hydrolysis has been used for the preparation of nano starch
particles through which samples of starch has gone through number of treatment by acid.
Morphology of the starch particles was changed properly. After 10 days of hydrolysis process for
the preparation of nano starch, the shape changes and it become 20–420 nm in shape which was
previously lies between 2.47 to 6.91 μm in length. Unlike morphology, changes has been
identified in other properties also such as particle size distribution which tends to decrease
eventually. It was changing as per the days of acidic treatment of acid for hydrolysis. Other
properties like X-ray diffraction, FTIR -Fourier-transform infrared spectroscopy, Rheological
properties, Amylose and many more was also identified.
The composite film have been made from starch nano crystal which have been critically
used in increasing the shelf life of fruits to a great extent. The attribute of fruits thus gets
influenced when the quality is being maintained by such coating. Several alteration may occur as
soon as the film are being produced by the help of starch nano particles. The components like
thickness, solubility, colour, WVTR moisture vapour transmission rate, mechanical properties,
thermal properties, biodegradability of composite films gets influenced by the concentration,
treatment methods for making starch films, kind of components used and parameters like pH,
temperature, moisture all affects the way and kind of composite films will be produced. The
variables like biodegradability, solubility, mechanical properties and thickness of films gets
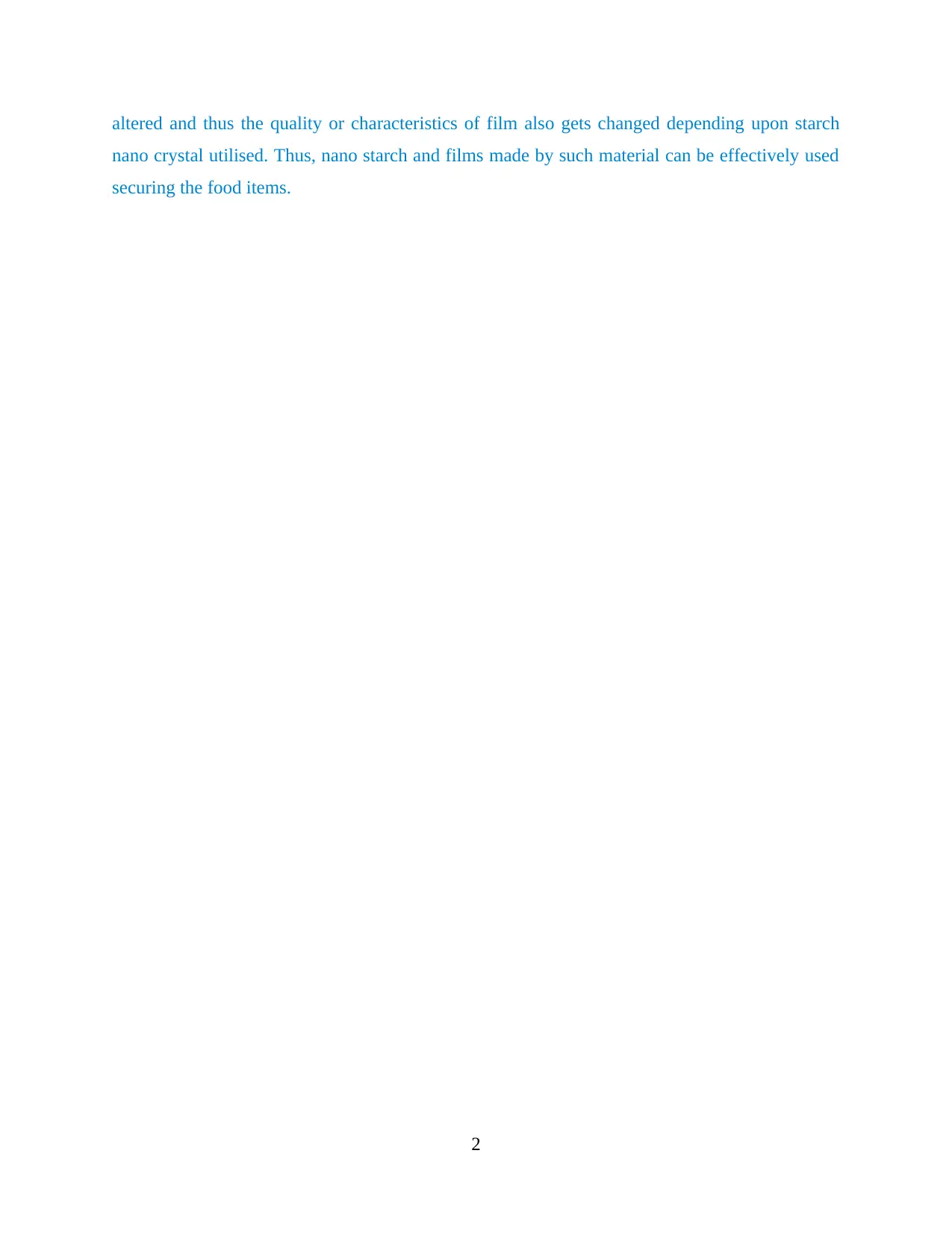
altered and thus the quality or characteristics of film also gets changed depending upon starch
nano crystal utilised. Thus, nano starch and films made by such material can be effectively used
securing the food items.
2
nano crystal utilised. Thus, nano starch and films made by such material can be effectively used
securing the food items.
2
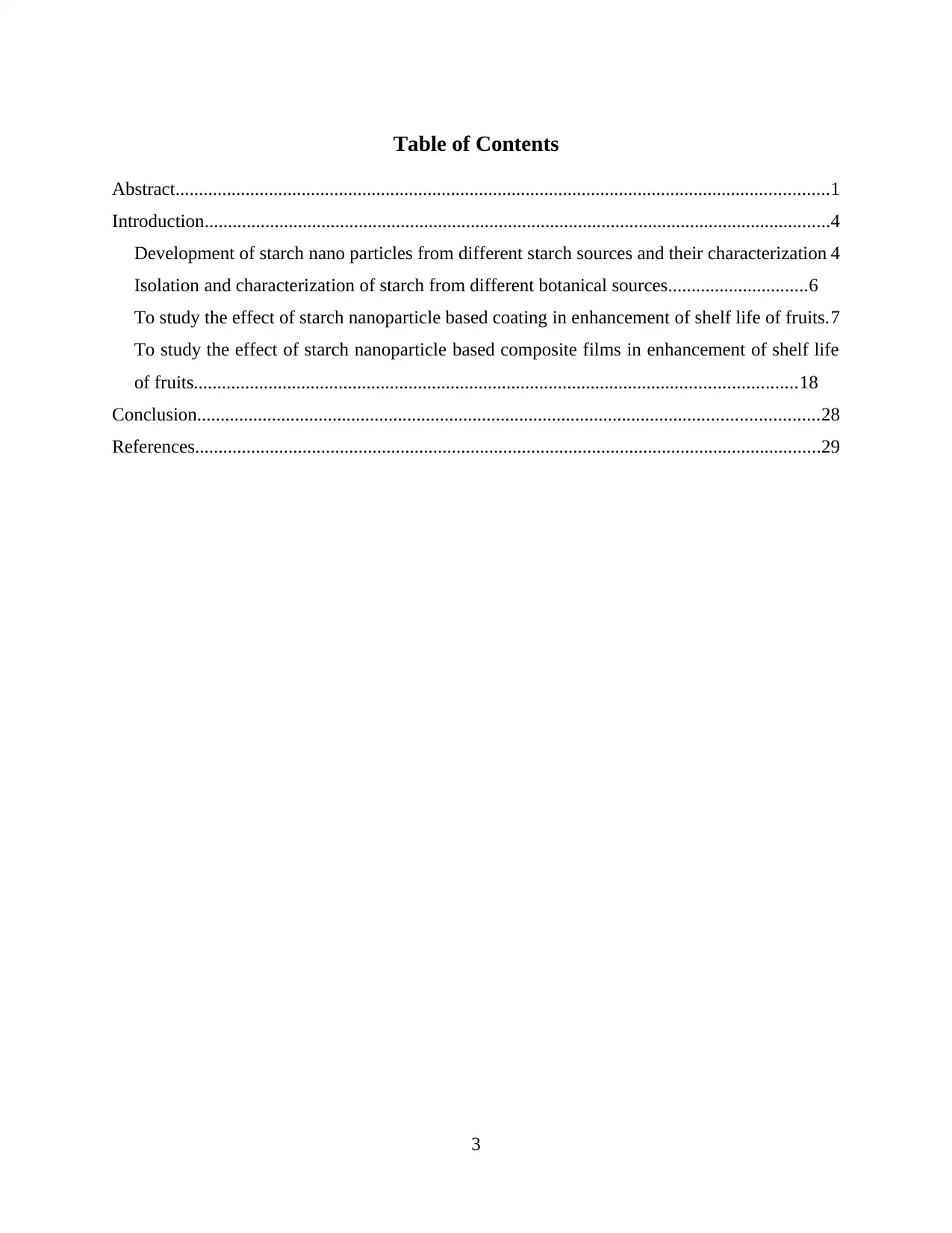
Table of Contents
Abstract............................................................................................................................................1
Introduction......................................................................................................................................4
Development of starch nano particles from different starch sources and their characterization 4
Isolation and characterization of starch from different botanical sources..............................6
To study the effect of starch nanoparticle based coating in enhancement of shelf life of fruits.7
To study the effect of starch nanoparticle based composite films in enhancement of shelf life
of fruits.................................................................................................................................18
Conclusion.....................................................................................................................................28
References......................................................................................................................................29
3
Abstract............................................................................................................................................1
Introduction......................................................................................................................................4
Development of starch nano particles from different starch sources and their characterization 4
Isolation and characterization of starch from different botanical sources..............................6
To study the effect of starch nanoparticle based coating in enhancement of shelf life of fruits.7
To study the effect of starch nanoparticle based composite films in enhancement of shelf life
of fruits.................................................................................................................................18
Conclusion.....................................................................................................................................28
References......................................................................................................................................29
3
Secure Best Marks with AI Grader
Need help grading? Try our AI Grader for instant feedback on your assignments.
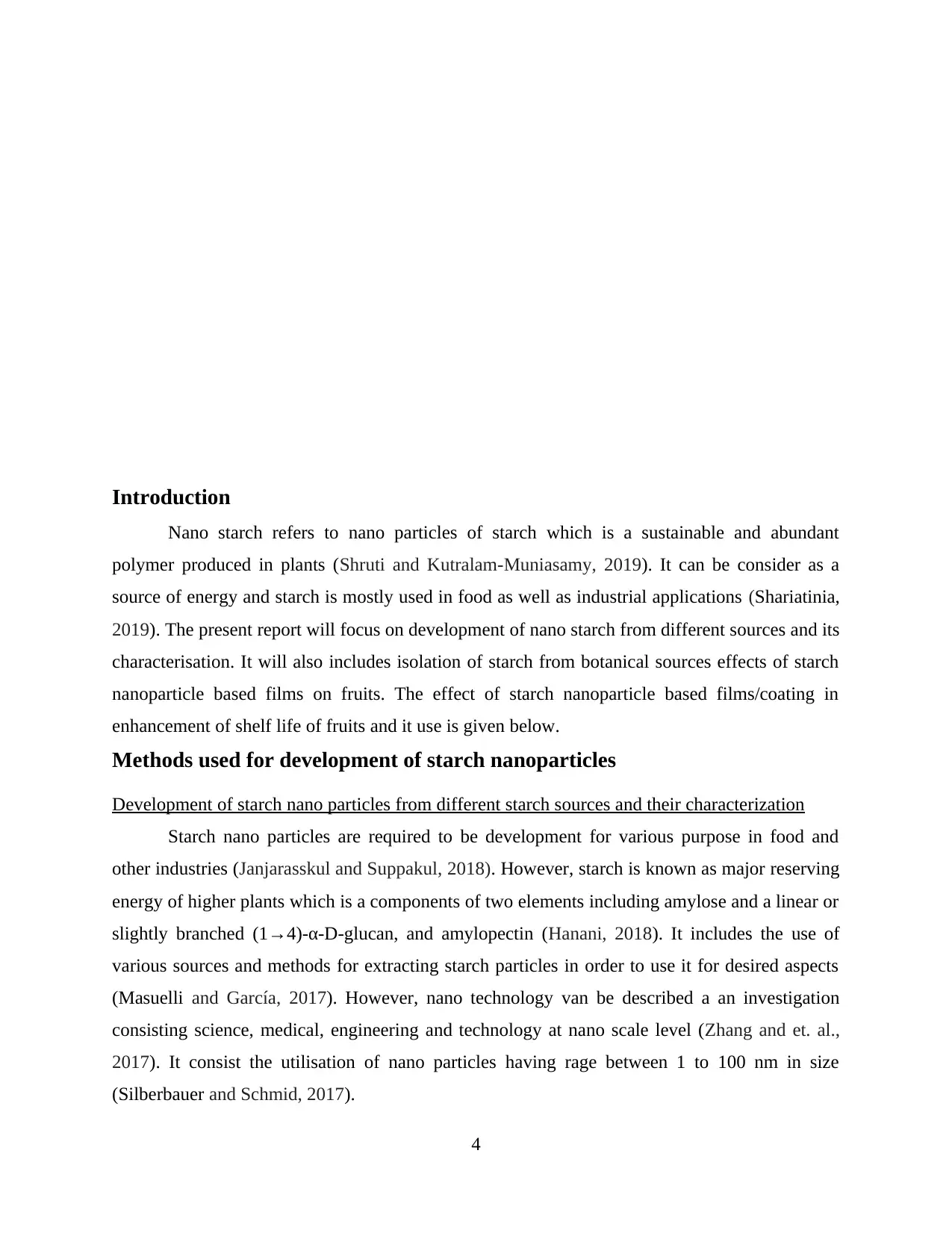
Introduction
Nano starch refers to nano particles of starch which is a sustainable and abundant
polymer produced in plants (Shruti and Kutralam-Muniasamy, 2019). It can be consider as a
source of energy and starch is mostly used in food as well as industrial applications (Shariatinia,
2019). The present report will focus on development of nano starch from different sources and its
characterisation. It will also includes isolation of starch from botanical sources effects of starch
nanoparticle based films on fruits. The effect of starch nanoparticle based films/coating in
enhancement of shelf life of fruits and it use is given below.
Methods used for development of starch nanoparticles
Development of starch nano particles from different starch sources and their characterization
Starch nano particles are required to be development for various purpose in food and
other industries (Janjarasskul and Suppakul, 2018). However, starch is known as major reserving
energy of higher plants which is a components of two elements including amylose and a linear or
slightly branched (1→4)-α-D-glucan, and amylopectin (Hanani, 2018). It includes the use of
various sources and methods for extracting starch particles in order to use it for desired aspects
(Masuelli and García, 2017). However, nano technology van be described a an investigation
consisting science, medical, engineering and technology at nano scale level (Zhang and et. al.,
2017). It consist the utilisation of nano particles having rage between 1 to 100 nm in size
(Silberbauer and Schmid, 2017).
4
Nano starch refers to nano particles of starch which is a sustainable and abundant
polymer produced in plants (Shruti and Kutralam-Muniasamy, 2019). It can be consider as a
source of energy and starch is mostly used in food as well as industrial applications (Shariatinia,
2019). The present report will focus on development of nano starch from different sources and its
characterisation. It will also includes isolation of starch from botanical sources effects of starch
nanoparticle based films on fruits. The effect of starch nanoparticle based films/coating in
enhancement of shelf life of fruits and it use is given below.
Methods used for development of starch nanoparticles
Development of starch nano particles from different starch sources and their characterization
Starch nano particles are required to be development for various purpose in food and
other industries (Janjarasskul and Suppakul, 2018). However, starch is known as major reserving
energy of higher plants which is a components of two elements including amylose and a linear or
slightly branched (1→4)-α-D-glucan, and amylopectin (Hanani, 2018). It includes the use of
various sources and methods for extracting starch particles in order to use it for desired aspects
(Masuelli and García, 2017). However, nano technology van be described a an investigation
consisting science, medical, engineering and technology at nano scale level (Zhang and et. al.,
2017). It consist the utilisation of nano particles having rage between 1 to 100 nm in size
(Silberbauer and Schmid, 2017).
4
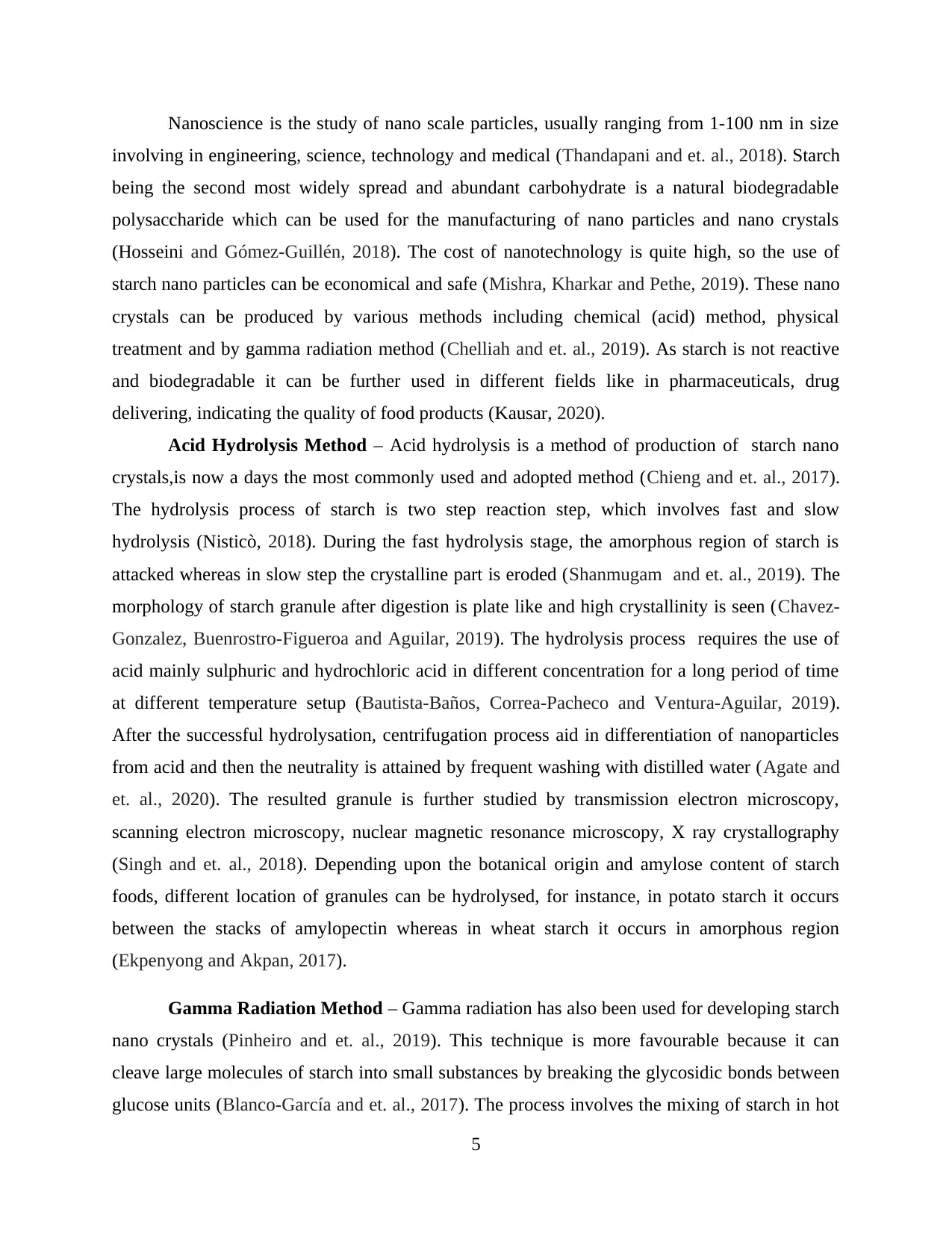
Nanoscience is the study of nano scale particles, usually ranging from 1-100 nm in size
involving in engineering, science, technology and medical (Thandapani and et. al., 2018). Starch
being the second most widely spread and abundant carbohydrate is a natural biodegradable
polysaccharide which can be used for the manufacturing of nano particles and nano crystals
(Hosseini and Gómez-Guillén, 2018). The cost of nanotechnology is quite high, so the use of
starch nano particles can be economical and safe (Mishra, Kharkar and Pethe, 2019). These nano
crystals can be produced by various methods including chemical (acid) method, physical
treatment and by gamma radiation method (Chelliah and et. al., 2019). As starch is not reactive
and biodegradable it can be further used in different fields like in pharmaceuticals, drug
delivering, indicating the quality of food products (Kausar, 2020).
Acid Hydrolysis Method – Acid hydrolysis is a method of production of starch nano
crystals,is now a days the most commonly used and adopted method (Chieng and et. al., 2017).
The hydrolysis process of starch is two step reaction step, which involves fast and slow
hydrolysis (Nisticò, 2018). During the fast hydrolysis stage, the amorphous region of starch is
attacked whereas in slow step the crystalline part is eroded (Shanmugam and et. al., 2019). The
morphology of starch granule after digestion is plate like and high crystallinity is seen (Chavez-
Gonzalez, Buenrostro-Figueroa and Aguilar, 2019). The hydrolysis process requires the use of
acid mainly sulphuric and hydrochloric acid in different concentration for a long period of time
at different temperature setup (Bautista‐Baños, Correa‐Pacheco and Ventura‐Aguilar, 2019).
After the successful hydrolysation, centrifugation process aid in differentiation of nanoparticles
from acid and then the neutrality is attained by frequent washing with distilled water (Agate and
et. al., 2020). The resulted granule is further studied by transmission electron microscopy,
scanning electron microscopy, nuclear magnetic resonance microscopy, X ray crystallography
(Singh and et. al., 2018). Depending upon the botanical origin and amylose content of starch
foods, different location of granules can be hydrolysed, for instance, in potato starch it occurs
between the stacks of amylopectin whereas in wheat starch it occurs in amorphous region
(Ekpenyong and Akpan, 2017).
Gamma Radiation Method – Gamma radiation has also been used for developing starch
nano crystals (Pinheiro and et. al., 2019). This technique is more favourable because it can
cleave large molecules of starch into small substances by breaking the glycosidic bonds between
glucose units (Blanco-García and et. al., 2017). The process involves the mixing of starch in hot
5
involving in engineering, science, technology and medical (Thandapani and et. al., 2018). Starch
being the second most widely spread and abundant carbohydrate is a natural biodegradable
polysaccharide which can be used for the manufacturing of nano particles and nano crystals
(Hosseini and Gómez-Guillén, 2018). The cost of nanotechnology is quite high, so the use of
starch nano particles can be economical and safe (Mishra, Kharkar and Pethe, 2019). These nano
crystals can be produced by various methods including chemical (acid) method, physical
treatment and by gamma radiation method (Chelliah and et. al., 2019). As starch is not reactive
and biodegradable it can be further used in different fields like in pharmaceuticals, drug
delivering, indicating the quality of food products (Kausar, 2020).
Acid Hydrolysis Method – Acid hydrolysis is a method of production of starch nano
crystals,is now a days the most commonly used and adopted method (Chieng and et. al., 2017).
The hydrolysis process of starch is two step reaction step, which involves fast and slow
hydrolysis (Nisticò, 2018). During the fast hydrolysis stage, the amorphous region of starch is
attacked whereas in slow step the crystalline part is eroded (Shanmugam and et. al., 2019). The
morphology of starch granule after digestion is plate like and high crystallinity is seen (Chavez-
Gonzalez, Buenrostro-Figueroa and Aguilar, 2019). The hydrolysis process requires the use of
acid mainly sulphuric and hydrochloric acid in different concentration for a long period of time
at different temperature setup (Bautista‐Baños, Correa‐Pacheco and Ventura‐Aguilar, 2019).
After the successful hydrolysation, centrifugation process aid in differentiation of nanoparticles
from acid and then the neutrality is attained by frequent washing with distilled water (Agate and
et. al., 2020). The resulted granule is further studied by transmission electron microscopy,
scanning electron microscopy, nuclear magnetic resonance microscopy, X ray crystallography
(Singh and et. al., 2018). Depending upon the botanical origin and amylose content of starch
foods, different location of granules can be hydrolysed, for instance, in potato starch it occurs
between the stacks of amylopectin whereas in wheat starch it occurs in amorphous region
(Ekpenyong and Akpan, 2017).
Gamma Radiation Method – Gamma radiation has also been used for developing starch
nano crystals (Pinheiro and et. al., 2019). This technique is more favourable because it can
cleave large molecules of starch into small substances by breaking the glycosidic bonds between
glucose units (Blanco-García and et. al., 2017). The process involves the mixing of starch in hot
5
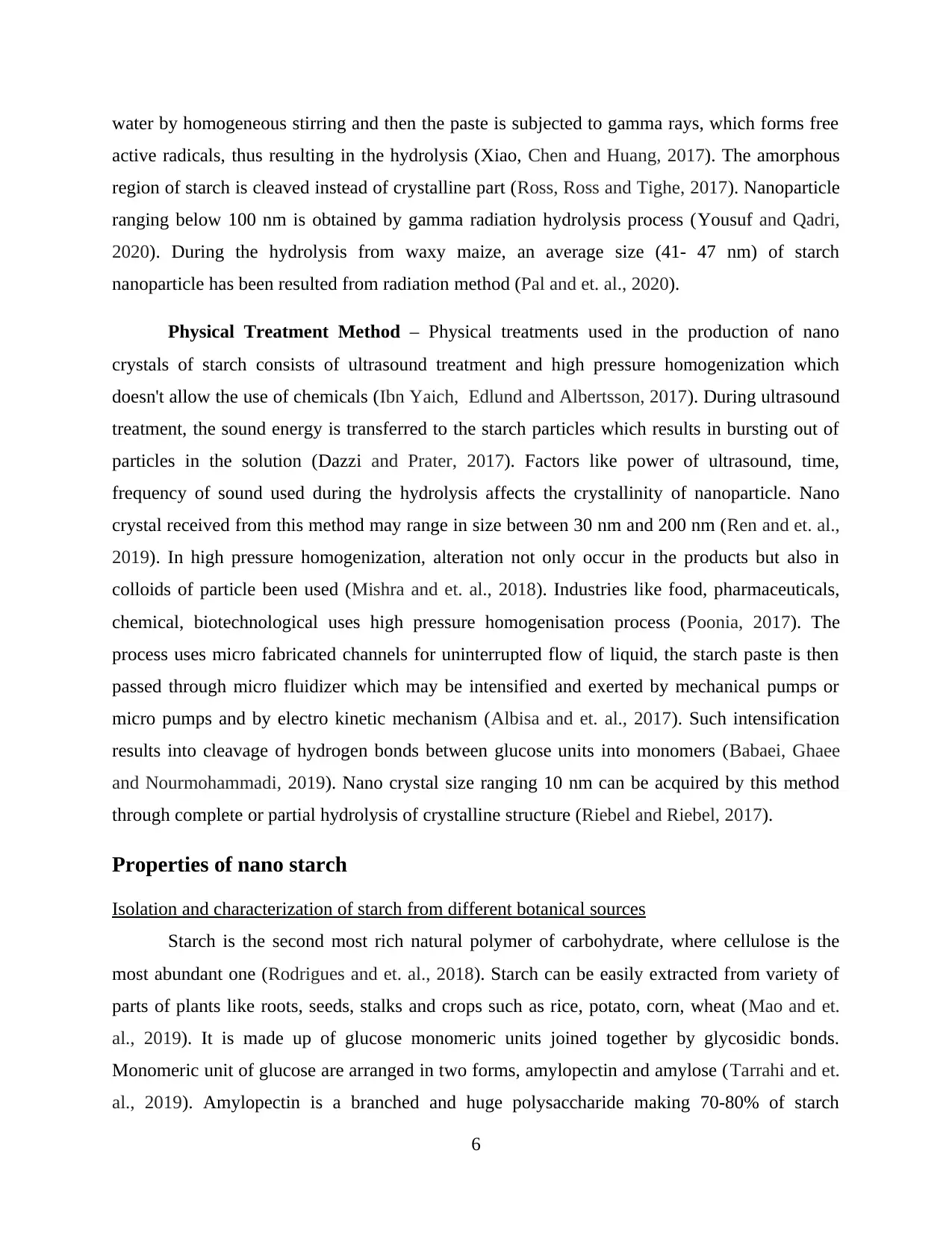
water by homogeneous stirring and then the paste is subjected to gamma rays, which forms free
active radicals, thus resulting in the hydrolysis (Xiao, Chen and Huang, 2017). The amorphous
region of starch is cleaved instead of crystalline part (Ross, Ross and Tighe, 2017). Nanoparticle
ranging below 100 nm is obtained by gamma radiation hydrolysis process (Yousuf and Qadri,
2020). During the hydrolysis from waxy maize, an average size (41- 47 nm) of starch
nanoparticle has been resulted from radiation method (Pal and et. al., 2020).
Physical Treatment Method – Physical treatments used in the production of nano
crystals of starch consists of ultrasound treatment and high pressure homogenization which
doesn't allow the use of chemicals (Ibn Yaich, Edlund and Albertsson, 2017). During ultrasound
treatment, the sound energy is transferred to the starch particles which results in bursting out of
particles in the solution (Dazzi and Prater, 2017). Factors like power of ultrasound, time,
frequency of sound used during the hydrolysis affects the crystallinity of nanoparticle. Nano
crystal received from this method may range in size between 30 nm and 200 nm (Ren and et. al.,
2019). In high pressure homogenization, alteration not only occur in the products but also in
colloids of particle been used (Mishra and et. al., 2018). Industries like food, pharmaceuticals,
chemical, biotechnological uses high pressure homogenisation process (Poonia, 2017). The
process uses micro fabricated channels for uninterrupted flow of liquid, the starch paste is then
passed through micro fluidizer which may be intensified and exerted by mechanical pumps or
micro pumps and by electro kinetic mechanism (Albisa and et. al., 2017). Such intensification
results into cleavage of hydrogen bonds between glucose units into monomers (Babaei, Ghaee
and Nourmohammadi, 2019). Nano crystal size ranging 10 nm can be acquired by this method
through complete or partial hydrolysis of crystalline structure (Riebel and Riebel, 2017).
Properties of nano starch
Isolation and characterization of starch from different botanical sources
Starch is the second most rich natural polymer of carbohydrate, where cellulose is the
most abundant one (Rodrigues and et. al., 2018). Starch can be easily extracted from variety of
parts of plants like roots, seeds, stalks and crops such as rice, potato, corn, wheat (Mao and et.
al., 2019). It is made up of glucose monomeric units joined together by glycosidic bonds.
Monomeric unit of glucose are arranged in two forms, amylopectin and amylose (Tarrahi and et.
al., 2019). Amylopectin is a branched and huge polysaccharide making 70-80% of starch
6
active radicals, thus resulting in the hydrolysis (Xiao, Chen and Huang, 2017). The amorphous
region of starch is cleaved instead of crystalline part (Ross, Ross and Tighe, 2017). Nanoparticle
ranging below 100 nm is obtained by gamma radiation hydrolysis process (Yousuf and Qadri,
2020). During the hydrolysis from waxy maize, an average size (41- 47 nm) of starch
nanoparticle has been resulted from radiation method (Pal and et. al., 2020).
Physical Treatment Method – Physical treatments used in the production of nano
crystals of starch consists of ultrasound treatment and high pressure homogenization which
doesn't allow the use of chemicals (Ibn Yaich, Edlund and Albertsson, 2017). During ultrasound
treatment, the sound energy is transferred to the starch particles which results in bursting out of
particles in the solution (Dazzi and Prater, 2017). Factors like power of ultrasound, time,
frequency of sound used during the hydrolysis affects the crystallinity of nanoparticle. Nano
crystal received from this method may range in size between 30 nm and 200 nm (Ren and et. al.,
2019). In high pressure homogenization, alteration not only occur in the products but also in
colloids of particle been used (Mishra and et. al., 2018). Industries like food, pharmaceuticals,
chemical, biotechnological uses high pressure homogenisation process (Poonia, 2017). The
process uses micro fabricated channels for uninterrupted flow of liquid, the starch paste is then
passed through micro fluidizer which may be intensified and exerted by mechanical pumps or
micro pumps and by electro kinetic mechanism (Albisa and et. al., 2017). Such intensification
results into cleavage of hydrogen bonds between glucose units into monomers (Babaei, Ghaee
and Nourmohammadi, 2019). Nano crystal size ranging 10 nm can be acquired by this method
through complete or partial hydrolysis of crystalline structure (Riebel and Riebel, 2017).
Properties of nano starch
Isolation and characterization of starch from different botanical sources
Starch is the second most rich natural polymer of carbohydrate, where cellulose is the
most abundant one (Rodrigues and et. al., 2018). Starch can be easily extracted from variety of
parts of plants like roots, seeds, stalks and crops such as rice, potato, corn, wheat (Mao and et.
al., 2019). It is made up of glucose monomeric units joined together by glycosidic bonds.
Monomeric unit of glucose are arranged in two forms, amylopectin and amylose (Tarrahi and et.
al., 2019). Amylopectin is a branched and huge polysaccharide making 70-80% of starch
6
Paraphrase This Document
Need a fresh take? Get an instant paraphrase of this document with our AI Paraphraser
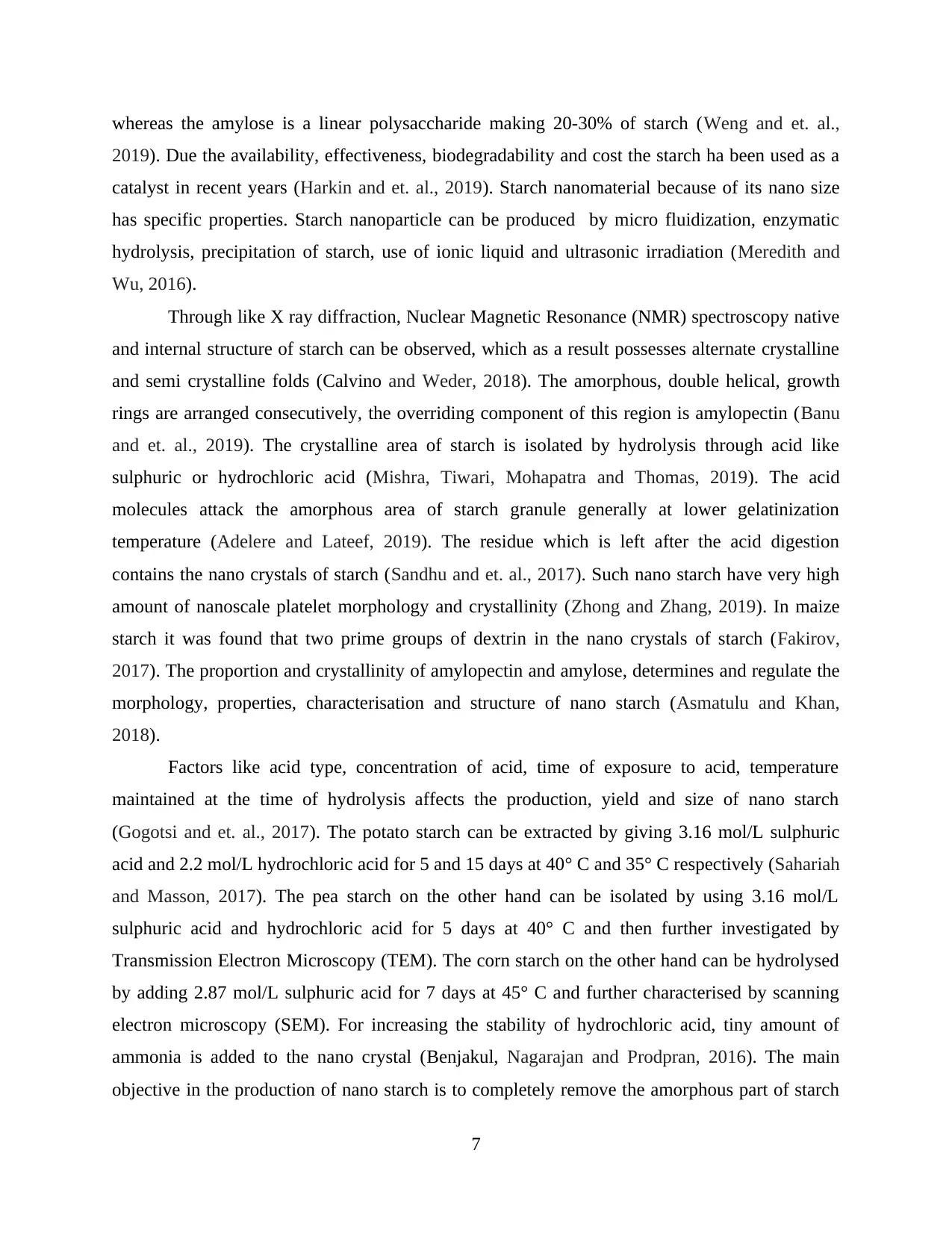
whereas the amylose is a linear polysaccharide making 20-30% of starch (Weng and et. al.,
2019). Due the availability, effectiveness, biodegradability and cost the starch ha been used as a
catalyst in recent years (Harkin and et. al., 2019). Starch nanomaterial because of its nano size
has specific properties. Starch nanoparticle can be produced by micro fluidization, enzymatic
hydrolysis, precipitation of starch, use of ionic liquid and ultrasonic irradiation (Meredith and
Wu, 2016).
Through like X ray diffraction, Nuclear Magnetic Resonance (NMR) spectroscopy native
and internal structure of starch can be observed, which as a result possesses alternate crystalline
and semi crystalline folds (Calvino and Weder, 2018). The amorphous, double helical, growth
rings are arranged consecutively, the overriding component of this region is amylopectin (Banu
and et. al., 2019). The crystalline area of starch is isolated by hydrolysis through acid like
sulphuric or hydrochloric acid (Mishra, Tiwari, Mohapatra and Thomas, 2019). The acid
molecules attack the amorphous area of starch granule generally at lower gelatinization
temperature (Adelere and Lateef, 2019). The residue which is left after the acid digestion
contains the nano crystals of starch (Sandhu and et. al., 2017). Such nano starch have very high
amount of nanoscale platelet morphology and crystallinity (Zhong and Zhang, 2019). In maize
starch it was found that two prime groups of dextrin in the nano crystals of starch (Fakirov,
2017). The proportion and crystallinity of amylopectin and amylose, determines and regulate the
morphology, properties, characterisation and structure of nano starch (Asmatulu and Khan,
2018).
Factors like acid type, concentration of acid, time of exposure to acid, temperature
maintained at the time of hydrolysis affects the production, yield and size of nano starch
(Gogotsi and et. al., 2017). The potato starch can be extracted by giving 3.16 mol/L sulphuric
acid and 2.2 mol/L hydrochloric acid for 5 and 15 days at 40° C and 35° C respectively (Sahariah
and Masson, 2017). The pea starch on the other hand can be isolated by using 3.16 mol/L
sulphuric acid and hydrochloric acid for 5 days at 40° C and then further investigated by
Transmission Electron Microscopy (TEM). The corn starch on the other hand can be hydrolysed
by adding 2.87 mol/L sulphuric acid for 7 days at 45° C and further characterised by scanning
electron microscopy (SEM). For increasing the stability of hydrochloric acid, tiny amount of
ammonia is added to the nano crystal (Benjakul, Nagarajan and Prodpran, 2016). The main
objective in the production of nano starch is to completely remove the amorphous part of starch
7
2019). Due the availability, effectiveness, biodegradability and cost the starch ha been used as a
catalyst in recent years (Harkin and et. al., 2019). Starch nanomaterial because of its nano size
has specific properties. Starch nanoparticle can be produced by micro fluidization, enzymatic
hydrolysis, precipitation of starch, use of ionic liquid and ultrasonic irradiation (Meredith and
Wu, 2016).
Through like X ray diffraction, Nuclear Magnetic Resonance (NMR) spectroscopy native
and internal structure of starch can be observed, which as a result possesses alternate crystalline
and semi crystalline folds (Calvino and Weder, 2018). The amorphous, double helical, growth
rings are arranged consecutively, the overriding component of this region is amylopectin (Banu
and et. al., 2019). The crystalline area of starch is isolated by hydrolysis through acid like
sulphuric or hydrochloric acid (Mishra, Tiwari, Mohapatra and Thomas, 2019). The acid
molecules attack the amorphous area of starch granule generally at lower gelatinization
temperature (Adelere and Lateef, 2019). The residue which is left after the acid digestion
contains the nano crystals of starch (Sandhu and et. al., 2017). Such nano starch have very high
amount of nanoscale platelet morphology and crystallinity (Zhong and Zhang, 2019). In maize
starch it was found that two prime groups of dextrin in the nano crystals of starch (Fakirov,
2017). The proportion and crystallinity of amylopectin and amylose, determines and regulate the
morphology, properties, characterisation and structure of nano starch (Asmatulu and Khan,
2018).
Factors like acid type, concentration of acid, time of exposure to acid, temperature
maintained at the time of hydrolysis affects the production, yield and size of nano starch
(Gogotsi and et. al., 2017). The potato starch can be extracted by giving 3.16 mol/L sulphuric
acid and 2.2 mol/L hydrochloric acid for 5 and 15 days at 40° C and 35° C respectively (Sahariah
and Masson, 2017). The pea starch on the other hand can be isolated by using 3.16 mol/L
sulphuric acid and hydrochloric acid for 5 days at 40° C and then further investigated by
Transmission Electron Microscopy (TEM). The corn starch on the other hand can be hydrolysed
by adding 2.87 mol/L sulphuric acid for 7 days at 45° C and further characterised by scanning
electron microscopy (SEM). For increasing the stability of hydrochloric acid, tiny amount of
ammonia is added to the nano crystal (Benjakul, Nagarajan and Prodpran, 2016). The main
objective in the production of nano starch is to completely remove the amorphous part of starch
7
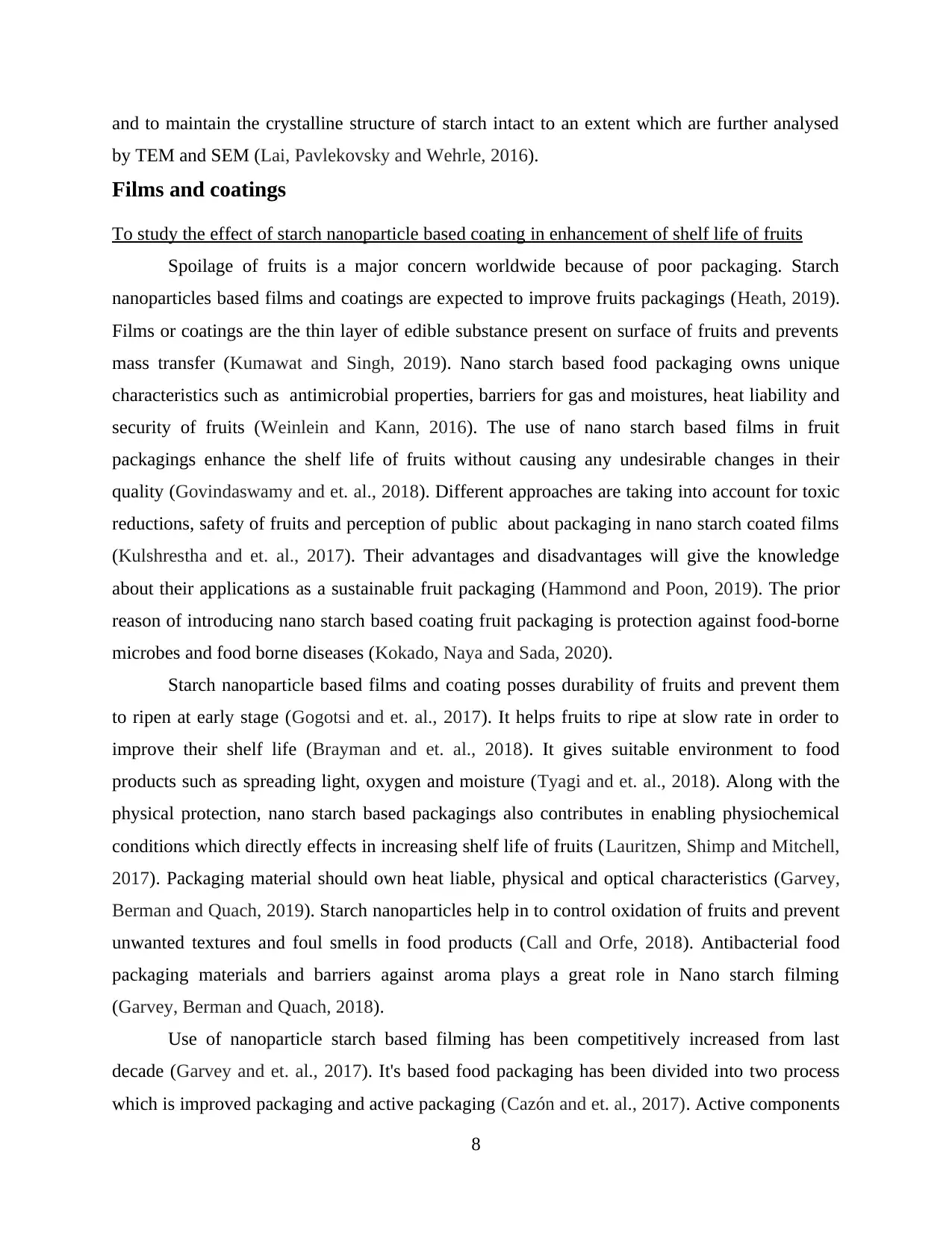
and to maintain the crystalline structure of starch intact to an extent which are further analysed
by TEM and SEM (Lai, Pavlekovsky and Wehrle, 2016).
Films and coatings
To study the effect of starch nanoparticle based coating in enhancement of shelf life of fruits
Spoilage of fruits is a major concern worldwide because of poor packaging. Starch
nanoparticles based films and coatings are expected to improve fruits packagings (Heath, 2019).
Films or coatings are the thin layer of edible substance present on surface of fruits and prevents
mass transfer (Kumawat and Singh, 2019). Nano starch based food packaging owns unique
characteristics such as antimicrobial properties, barriers for gas and moistures, heat liability and
security of fruits (Weinlein and Kann, 2016). The use of nano starch based films in fruit
packagings enhance the shelf life of fruits without causing any undesirable changes in their
quality (Govindaswamy and et. al., 2018). Different approaches are taking into account for toxic
reductions, safety of fruits and perception of public about packaging in nano starch coated films
(Kulshrestha and et. al., 2017). Their advantages and disadvantages will give the knowledge
about their applications as a sustainable fruit packaging (Hammond and Poon, 2019). The prior
reason of introducing nano starch based coating fruit packaging is protection against food-borne
microbes and food borne diseases (Kokado, Naya and Sada, 2020).
Starch nanoparticle based films and coating posses durability of fruits and prevent them
to ripen at early stage (Gogotsi and et. al., 2017). It helps fruits to ripe at slow rate in order to
improve their shelf life (Brayman and et. al., 2018). It gives suitable environment to food
products such as spreading light, oxygen and moisture (Tyagi and et. al., 2018). Along with the
physical protection, nano starch based packagings also contributes in enabling physiochemical
conditions which directly effects in increasing shelf life of fruits (Lauritzen, Shimp and Mitchell,
2017). Packaging material should own heat liable, physical and optical characteristics (Garvey,
Berman and Quach, 2019). Starch nanoparticles help in to control oxidation of fruits and prevent
unwanted textures and foul smells in food products (Call and Orfe, 2018). Antibacterial food
packaging materials and barriers against aroma plays a great role in Nano starch filming
(Garvey, Berman and Quach, 2018).
Use of nanoparticle starch based filming has been competitively increased from last
decade (Garvey and et. al., 2017). It's based food packaging has been divided into two process
which is improved packaging and active packaging (Cazón and et. al., 2017). Active components
8
by TEM and SEM (Lai, Pavlekovsky and Wehrle, 2016).
Films and coatings
To study the effect of starch nanoparticle based coating in enhancement of shelf life of fruits
Spoilage of fruits is a major concern worldwide because of poor packaging. Starch
nanoparticles based films and coatings are expected to improve fruits packagings (Heath, 2019).
Films or coatings are the thin layer of edible substance present on surface of fruits and prevents
mass transfer (Kumawat and Singh, 2019). Nano starch based food packaging owns unique
characteristics such as antimicrobial properties, barriers for gas and moistures, heat liability and
security of fruits (Weinlein and Kann, 2016). The use of nano starch based films in fruit
packagings enhance the shelf life of fruits without causing any undesirable changes in their
quality (Govindaswamy and et. al., 2018). Different approaches are taking into account for toxic
reductions, safety of fruits and perception of public about packaging in nano starch coated films
(Kulshrestha and et. al., 2017). Their advantages and disadvantages will give the knowledge
about their applications as a sustainable fruit packaging (Hammond and Poon, 2019). The prior
reason of introducing nano starch based coating fruit packaging is protection against food-borne
microbes and food borne diseases (Kokado, Naya and Sada, 2020).
Starch nanoparticle based films and coating posses durability of fruits and prevent them
to ripen at early stage (Gogotsi and et. al., 2017). It helps fruits to ripe at slow rate in order to
improve their shelf life (Brayman and et. al., 2018). It gives suitable environment to food
products such as spreading light, oxygen and moisture (Tyagi and et. al., 2018). Along with the
physical protection, nano starch based packagings also contributes in enabling physiochemical
conditions which directly effects in increasing shelf life of fruits (Lauritzen, Shimp and Mitchell,
2017). Packaging material should own heat liable, physical and optical characteristics (Garvey,
Berman and Quach, 2019). Starch nanoparticles help in to control oxidation of fruits and prevent
unwanted textures and foul smells in food products (Call and Orfe, 2018). Antibacterial food
packaging materials and barriers against aroma plays a great role in Nano starch filming
(Garvey, Berman and Quach, 2018).
Use of nanoparticle starch based filming has been competitively increased from last
decade (Garvey and et. al., 2017). It's based food packaging has been divided into two process
which is improved packaging and active packaging (Cazón and et. al., 2017). Active components
8
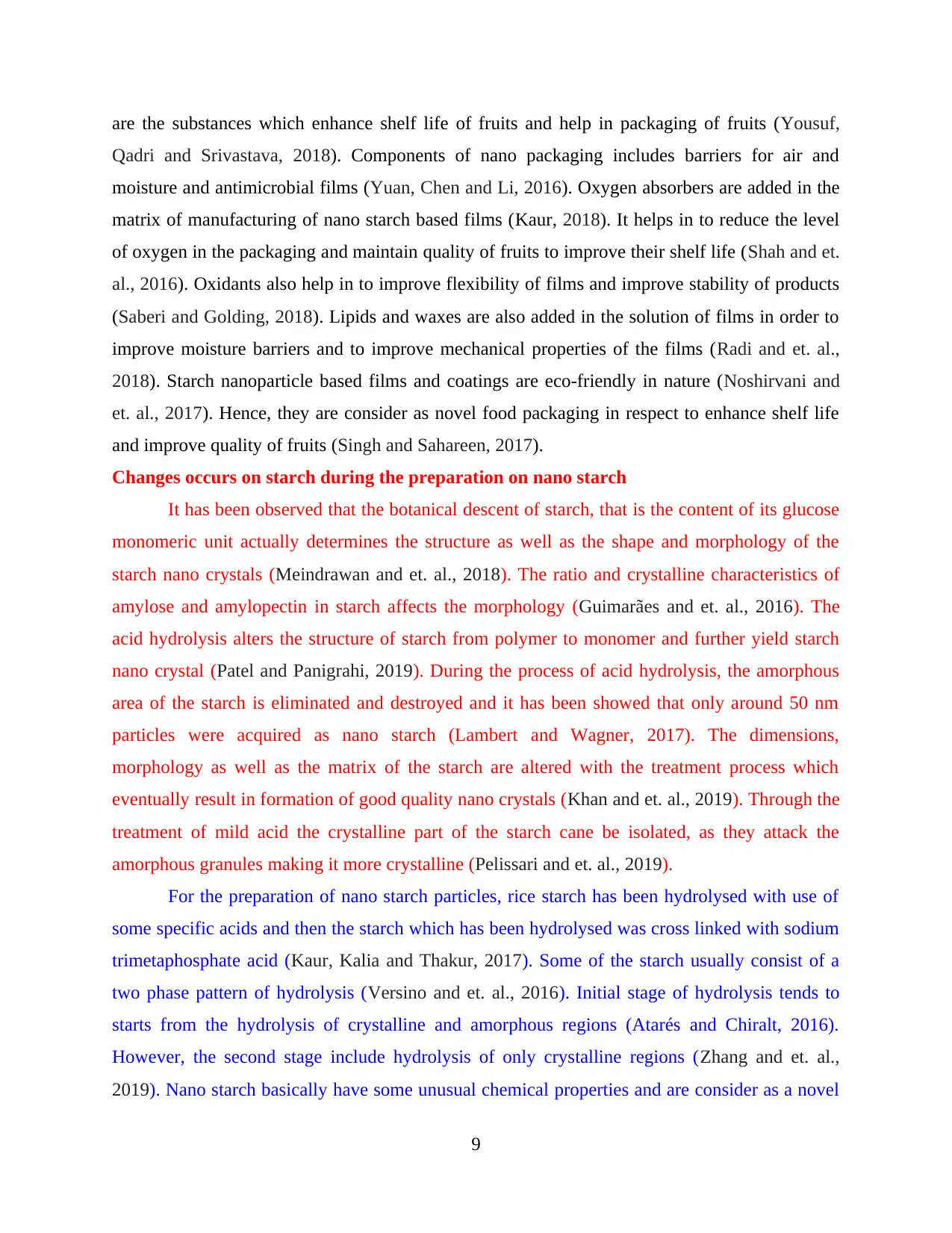
are the substances which enhance shelf life of fruits and help in packaging of fruits (Yousuf,
Qadri and Srivastava, 2018). Components of nano packaging includes barriers for air and
moisture and antimicrobial films (Yuan, Chen and Li, 2016). Oxygen absorbers are added in the
matrix of manufacturing of nano starch based films (Kaur, 2018). It helps in to reduce the level
of oxygen in the packaging and maintain quality of fruits to improve their shelf life (Shah and et.
al., 2016). Oxidants also help in to improve flexibility of films and improve stability of products
(Saberi and Golding, 2018). Lipids and waxes are also added in the solution of films in order to
improve moisture barriers and to improve mechanical properties of the films (Radi and et. al.,
2018). Starch nanoparticle based films and coatings are eco-friendly in nature (Noshirvani and
et. al., 2017). Hence, they are consider as novel food packaging in respect to enhance shelf life
and improve quality of fruits (Singh and Sahareen, 2017).
Changes occurs on starch during the preparation on nano starch
It has been observed that the botanical descent of starch, that is the content of its glucose
monomeric unit actually determines the structure as well as the shape and morphology of the
starch nano crystals (Meindrawan and et. al., 2018). The ratio and crystalline characteristics of
amylose and amylopectin in starch affects the morphology (Guimarães and et. al., 2016). The
acid hydrolysis alters the structure of starch from polymer to monomer and further yield starch
nano crystal (Patel and Panigrahi, 2019). During the process of acid hydrolysis, the amorphous
area of the starch is eliminated and destroyed and it has been showed that only around 50 nm
particles were acquired as nano starch (Lambert and Wagner, 2017). The dimensions,
morphology as well as the matrix of the starch are altered with the treatment process which
eventually result in formation of good quality nano crystals (Khan and et. al., 2019). Through the
treatment of mild acid the crystalline part of the starch cane be isolated, as they attack the
amorphous granules making it more crystalline (Pelissari and et. al., 2019).
For the preparation of nano starch particles, rice starch has been hydrolysed with use of
some specific acids and then the starch which has been hydrolysed was cross linked with sodium
trimetaphosphate acid (Kaur, Kalia and Thakur, 2017). Some of the starch usually consist of a
two phase pattern of hydrolysis (Versino and et. al., 2016). Initial stage of hydrolysis tends to
starts from the hydrolysis of crystalline and amorphous regions (Atarés and Chiralt, 2016).
However, the second stage include hydrolysis of only crystalline regions (Zhang and et. al.,
2019). Nano starch basically have some unusual chemical properties and are consider as a novel
9
Qadri and Srivastava, 2018). Components of nano packaging includes barriers for air and
moisture and antimicrobial films (Yuan, Chen and Li, 2016). Oxygen absorbers are added in the
matrix of manufacturing of nano starch based films (Kaur, 2018). It helps in to reduce the level
of oxygen in the packaging and maintain quality of fruits to improve their shelf life (Shah and et.
al., 2016). Oxidants also help in to improve flexibility of films and improve stability of products
(Saberi and Golding, 2018). Lipids and waxes are also added in the solution of films in order to
improve moisture barriers and to improve mechanical properties of the films (Radi and et. al.,
2018). Starch nanoparticle based films and coatings are eco-friendly in nature (Noshirvani and
et. al., 2017). Hence, they are consider as novel food packaging in respect to enhance shelf life
and improve quality of fruits (Singh and Sahareen, 2017).
Changes occurs on starch during the preparation on nano starch
It has been observed that the botanical descent of starch, that is the content of its glucose
monomeric unit actually determines the structure as well as the shape and morphology of the
starch nano crystals (Meindrawan and et. al., 2018). The ratio and crystalline characteristics of
amylose and amylopectin in starch affects the morphology (Guimarães and et. al., 2016). The
acid hydrolysis alters the structure of starch from polymer to monomer and further yield starch
nano crystal (Patel and Panigrahi, 2019). During the process of acid hydrolysis, the amorphous
area of the starch is eliminated and destroyed and it has been showed that only around 50 nm
particles were acquired as nano starch (Lambert and Wagner, 2017). The dimensions,
morphology as well as the matrix of the starch are altered with the treatment process which
eventually result in formation of good quality nano crystals (Khan and et. al., 2019). Through the
treatment of mild acid the crystalline part of the starch cane be isolated, as they attack the
amorphous granules making it more crystalline (Pelissari and et. al., 2019).
For the preparation of nano starch particles, rice starch has been hydrolysed with use of
some specific acids and then the starch which has been hydrolysed was cross linked with sodium
trimetaphosphate acid (Kaur, Kalia and Thakur, 2017). Some of the starch usually consist of a
two phase pattern of hydrolysis (Versino and et. al., 2016). Initial stage of hydrolysis tends to
starts from the hydrolysis of crystalline and amorphous regions (Atarés and Chiralt, 2016).
However, the second stage include hydrolysis of only crystalline regions (Zhang and et. al.,
2019). Nano starch basically have some unusual chemical properties and are consider as a novel
9
Secure Best Marks with AI Grader
Need help grading? Try our AI Grader for instant feedback on your assignments.
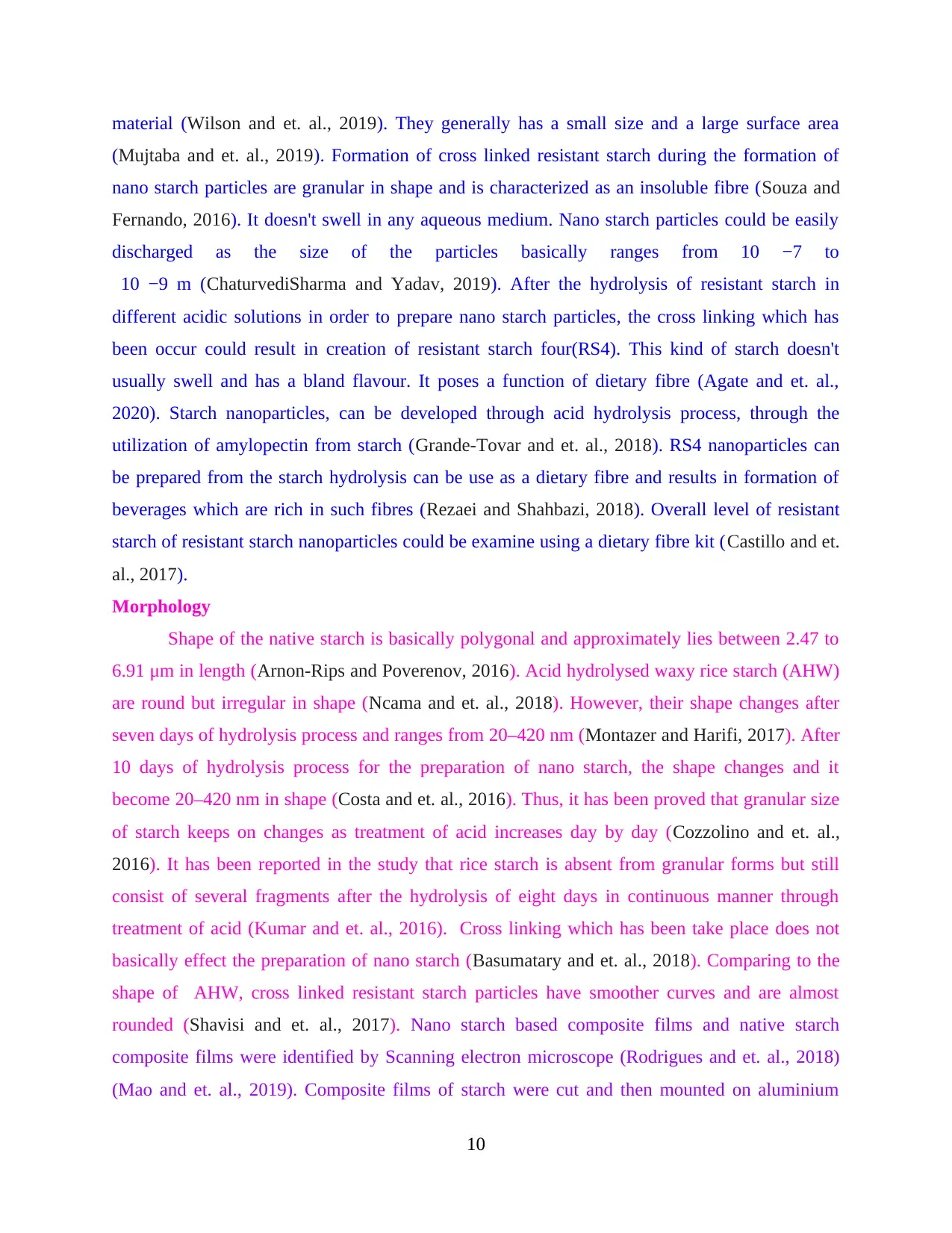
material (Wilson and et. al., 2019). They generally has a small size and a large surface area
(Mujtaba and et. al., 2019). Formation of cross linked resistant starch during the formation of
nano starch particles are granular in shape and is characterized as an insoluble fibre (Souza and
Fernando, 2016). It doesn't swell in any aqueous medium. Nano starch particles could be easily
discharged as the size of the particles basically ranges from 10 −7 to
10 −9 m (ChaturvediSharma and Yadav, 2019). After the hydrolysis of resistant starch in
different acidic solutions in order to prepare nano starch particles, the cross linking which has
been occur could result in creation of resistant starch four(RS4). This kind of starch doesn't
usually swell and has a bland flavour. It poses a function of dietary fibre (Agate and et. al.,
2020). Starch nanoparticles, can be developed through acid hydrolysis process, through the
utilization of amylopectin from starch (Grande-Tovar and et. al., 2018). RS4 nanoparticles can
be prepared from the starch hydrolysis can be use as a dietary fibre and results in formation of
beverages which are rich in such fibres (Rezaei and Shahbazi, 2018). Overall level of resistant
starch of resistant starch nanoparticles could be examine using a dietary fibre kit (Castillo and et.
al., 2017).
Morphology
Shape of the native starch is basically polygonal and approximately lies between 2.47 to
6.91 μm in length (Arnon-Rips and Poverenov, 2016). Acid hydrolysed waxy rice starch (AHW)
are round but irregular in shape (Ncama and et. al., 2018). However, their shape changes after
seven days of hydrolysis process and ranges from 20–420 nm (Montazer and Harifi, 2017). After
10 days of hydrolysis process for the preparation of nano starch, the shape changes and it
become 20–420 nm in shape (Costa and et. al., 2016). Thus, it has been proved that granular size
of starch keeps on changes as treatment of acid increases day by day (Cozzolino and et. al.,
2016). It has been reported in the study that rice starch is absent from granular forms but still
consist of several fragments after the hydrolysis of eight days in continuous manner through
treatment of acid (Kumar and et. al., 2016). Cross linking which has been take place does not
basically effect the preparation of nano starch (Basumatary and et. al., 2018). Comparing to the
shape of AHW, cross linked resistant starch particles have smoother curves and are almost
rounded (Shavisi and et. al., 2017). Nano starch based composite films and native starch
composite films were identified by Scanning electron microscope (Rodrigues and et. al., 2018)
(Mao and et. al., 2019). Composite films of starch were cut and then mounted on aluminium
10
(Mujtaba and et. al., 2019). Formation of cross linked resistant starch during the formation of
nano starch particles are granular in shape and is characterized as an insoluble fibre (Souza and
Fernando, 2016). It doesn't swell in any aqueous medium. Nano starch particles could be easily
discharged as the size of the particles basically ranges from 10 −7 to
10 −9 m (ChaturvediSharma and Yadav, 2019). After the hydrolysis of resistant starch in
different acidic solutions in order to prepare nano starch particles, the cross linking which has
been occur could result in creation of resistant starch four(RS4). This kind of starch doesn't
usually swell and has a bland flavour. It poses a function of dietary fibre (Agate and et. al.,
2020). Starch nanoparticles, can be developed through acid hydrolysis process, through the
utilization of amylopectin from starch (Grande-Tovar and et. al., 2018). RS4 nanoparticles can
be prepared from the starch hydrolysis can be use as a dietary fibre and results in formation of
beverages which are rich in such fibres (Rezaei and Shahbazi, 2018). Overall level of resistant
starch of resistant starch nanoparticles could be examine using a dietary fibre kit (Castillo and et.
al., 2017).
Morphology
Shape of the native starch is basically polygonal and approximately lies between 2.47 to
6.91 μm in length (Arnon-Rips and Poverenov, 2016). Acid hydrolysed waxy rice starch (AHW)
are round but irregular in shape (Ncama and et. al., 2018). However, their shape changes after
seven days of hydrolysis process and ranges from 20–420 nm (Montazer and Harifi, 2017). After
10 days of hydrolysis process for the preparation of nano starch, the shape changes and it
become 20–420 nm in shape (Costa and et. al., 2016). Thus, it has been proved that granular size
of starch keeps on changes as treatment of acid increases day by day (Cozzolino and et. al.,
2016). It has been reported in the study that rice starch is absent from granular forms but still
consist of several fragments after the hydrolysis of eight days in continuous manner through
treatment of acid (Kumar and et. al., 2016). Cross linking which has been take place does not
basically effect the preparation of nano starch (Basumatary and et. al., 2018). Comparing to the
shape of AHW, cross linked resistant starch particles have smoother curves and are almost
rounded (Shavisi and et. al., 2017). Nano starch based composite films and native starch
composite films were identified by Scanning electron microscope (Rodrigues and et. al., 2018)
(Mao and et. al., 2019). Composite films of starch were cut and then mounted on aluminium
10
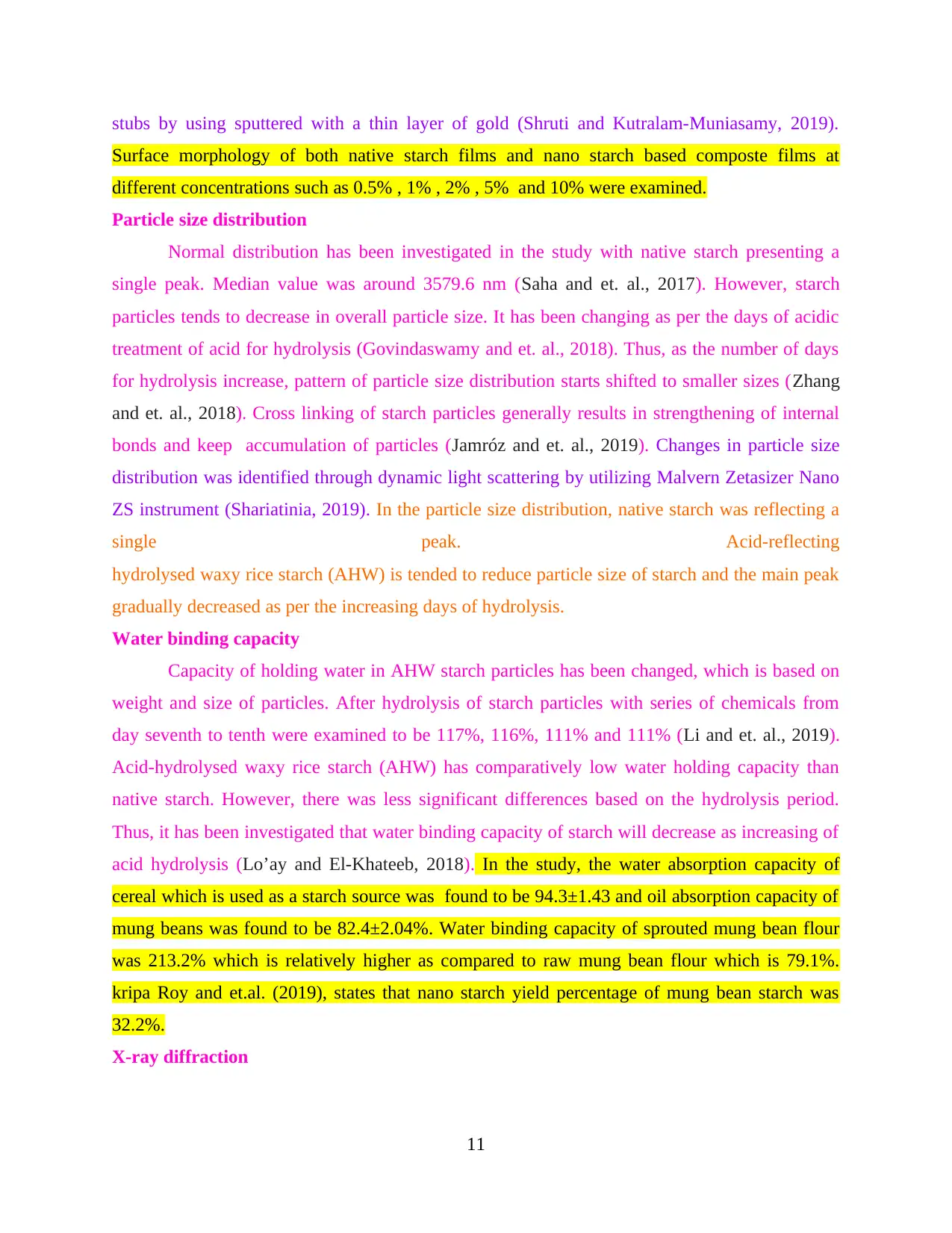
stubs by using sputtered with a thin layer of gold (Shruti and Kutralam-Muniasamy, 2019).
Surface morphology of both native starch films and nano starch based composte films at
different concentrations such as 0.5% , 1% , 2% , 5% and 10% were examined.
Particle size distribution
Normal distribution has been investigated in the study with native starch presenting a
single peak. Median value was around 3579.6 nm (Saha and et. al., 2017). However, starch
particles tends to decrease in overall particle size. It has been changing as per the days of acidic
treatment of acid for hydrolysis (Govindaswamy and et. al., 2018). Thus, as the number of days
for hydrolysis increase, pattern of particle size distribution starts shifted to smaller sizes (Zhang
and et. al., 2018). Cross linking of starch particles generally results in strengthening of internal
bonds and keep accumulation of particles (Jamróz and et. al., 2019). Changes in particle size
distribution was identified through dynamic light scattering by utilizing Malvern Zetasizer Nano
ZS instrument (Shariatinia, 2019). In the particle size distribution, native starch was reflecting a
single peak. Acid-reflecting
hydrolysed waxy rice starch (AHW) is tended to reduce particle size of starch and the main peak
gradually decreased as per the increasing days of hydrolysis.
Water binding capacity
Capacity of holding water in AHW starch particles has been changed, which is based on
weight and size of particles. After hydrolysis of starch particles with series of chemicals from
day seventh to tenth were examined to be 117%, 116%, 111% and 111% (Li and et. al., 2019).
Acid-hydrolysed waxy rice starch (AHW) has comparatively low water holding capacity than
native starch. However, there was less significant differences based on the hydrolysis period.
Thus, it has been investigated that water binding capacity of starch will decrease as increasing of
acid hydrolysis (Lo’ay and El-Khateeb, 2018). In the study, the water absorption capacity of
cereal which is used as a starch source was found to be 94.3±1.43 and oil absorption capacity of
mung beans was found to be 82.4±2.04%. Water binding capacity of sprouted mung bean flour
was 213.2% which is relatively higher as compared to raw mung bean flour which is 79.1%.
kripa Roy and et.al. (2019), states that nano starch yield percentage of mung bean starch was
32.2%.
X-ray diffraction
11
Surface morphology of both native starch films and nano starch based composte films at
different concentrations such as 0.5% , 1% , 2% , 5% and 10% were examined.
Particle size distribution
Normal distribution has been investigated in the study with native starch presenting a
single peak. Median value was around 3579.6 nm (Saha and et. al., 2017). However, starch
particles tends to decrease in overall particle size. It has been changing as per the days of acidic
treatment of acid for hydrolysis (Govindaswamy and et. al., 2018). Thus, as the number of days
for hydrolysis increase, pattern of particle size distribution starts shifted to smaller sizes (Zhang
and et. al., 2018). Cross linking of starch particles generally results in strengthening of internal
bonds and keep accumulation of particles (Jamróz and et. al., 2019). Changes in particle size
distribution was identified through dynamic light scattering by utilizing Malvern Zetasizer Nano
ZS instrument (Shariatinia, 2019). In the particle size distribution, native starch was reflecting a
single peak. Acid-reflecting
hydrolysed waxy rice starch (AHW) is tended to reduce particle size of starch and the main peak
gradually decreased as per the increasing days of hydrolysis.
Water binding capacity
Capacity of holding water in AHW starch particles has been changed, which is based on
weight and size of particles. After hydrolysis of starch particles with series of chemicals from
day seventh to tenth were examined to be 117%, 116%, 111% and 111% (Li and et. al., 2019).
Acid-hydrolysed waxy rice starch (AHW) has comparatively low water holding capacity than
native starch. However, there was less significant differences based on the hydrolysis period.
Thus, it has been investigated that water binding capacity of starch will decrease as increasing of
acid hydrolysis (Lo’ay and El-Khateeb, 2018). In the study, the water absorption capacity of
cereal which is used as a starch source was found to be 94.3±1.43 and oil absorption capacity of
mung beans was found to be 82.4±2.04%. Water binding capacity of sprouted mung bean flour
was 213.2% which is relatively higher as compared to raw mung bean flour which is 79.1%.
kripa Roy and et.al. (2019), states that nano starch yield percentage of mung bean starch was
32.2%.
X-ray diffraction
11
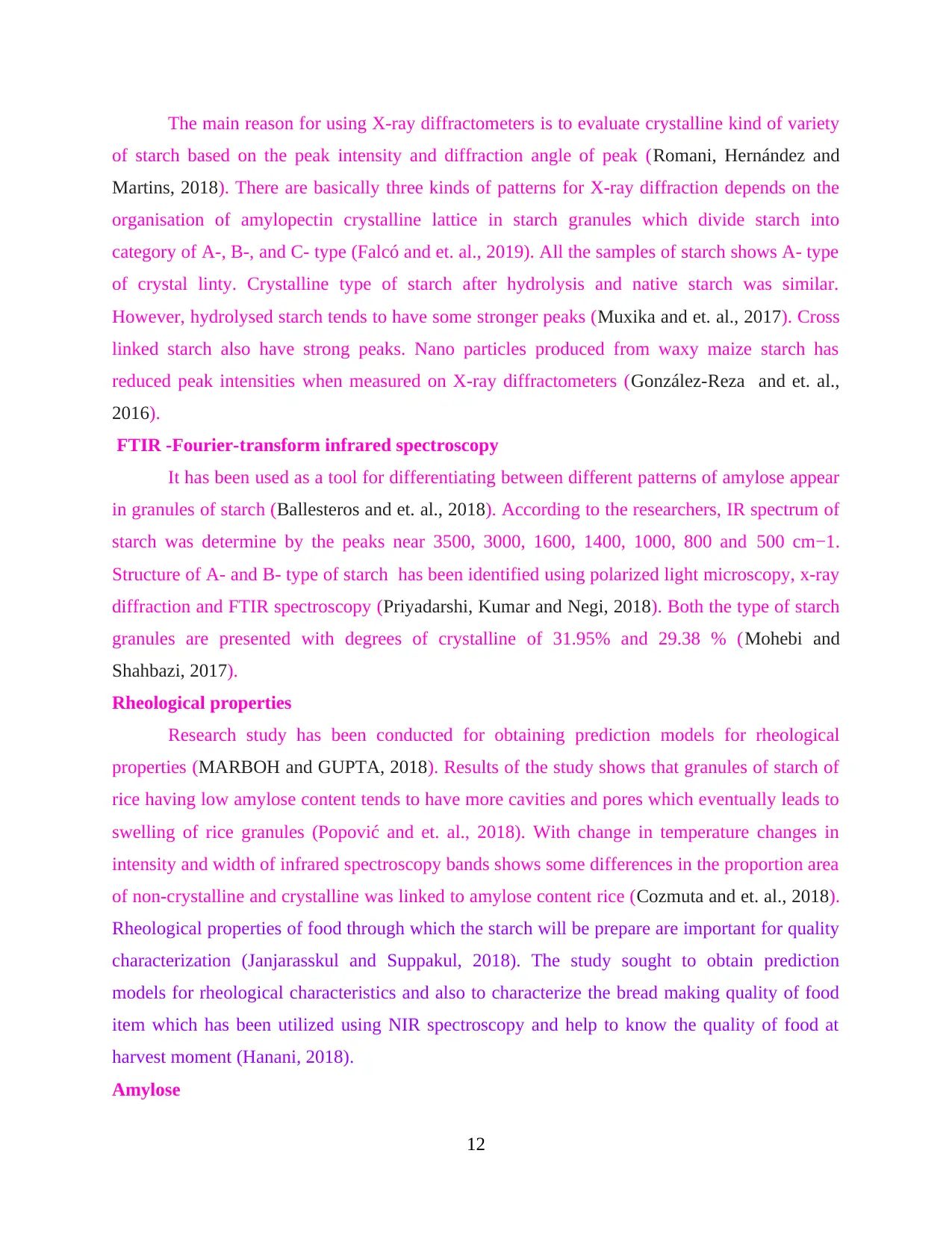
The main reason for using X-ray diffractometers is to evaluate crystalline kind of variety
of starch based on the peak intensity and diffraction angle of peak (Romani, Hernández and
Martins, 2018). There are basically three kinds of patterns for X-ray diffraction depends on the
organisation of amylopectin crystalline lattice in starch granules which divide starch into
category of A-, B-, and C- type (Falcó and et. al., 2019). All the samples of starch shows A- type
of crystal linty. Crystalline type of starch after hydrolysis and native starch was similar.
However, hydrolysed starch tends to have some stronger peaks (Muxika and et. al., 2017). Cross
linked starch also have strong peaks. Nano particles produced from waxy maize starch has
reduced peak intensities when measured on X-ray diffractometers (González-Reza and et. al.,
2016).
FTIR -Fourier-transform infrared spectroscopy
It has been used as a tool for differentiating between different patterns of amylose appear
in granules of starch (Ballesteros and et. al., 2018). According to the researchers, IR spectrum of
starch was determine by the peaks near 3500, 3000, 1600, 1400, 1000, 800 and 500 cm−1.
Structure of A- and B- type of starch has been identified using polarized light microscopy, x-ray
diffraction and FTIR spectroscopy (Priyadarshi, Kumar and Negi, 2018). Both the type of starch
granules are presented with degrees of crystalline of 31.95% and 29.38 % (Mohebi and
Shahbazi, 2017).
Rheological properties
Research study has been conducted for obtaining prediction models for rheological
properties (MARBOH and GUPTA, 2018). Results of the study shows that granules of starch of
rice having low amylose content tends to have more cavities and pores which eventually leads to
swelling of rice granules (Popović and et. al., 2018). With change in temperature changes in
intensity and width of infrared spectroscopy bands shows some differences in the proportion area
of non-crystalline and crystalline was linked to amylose content rice (Cozmuta and et. al., 2018).
Rheological properties of food through which the starch will be prepare are important for quality
characterization (Janjarasskul and Suppakul, 2018). The study sought to obtain prediction
models for rheological characteristics and also to characterize the bread making quality of food
item which has been utilized using NIR spectroscopy and help to know the quality of food at
harvest moment (Hanani, 2018).
Amylose
12
of starch based on the peak intensity and diffraction angle of peak (Romani, Hernández and
Martins, 2018). There are basically three kinds of patterns for X-ray diffraction depends on the
organisation of amylopectin crystalline lattice in starch granules which divide starch into
category of A-, B-, and C- type (Falcó and et. al., 2019). All the samples of starch shows A- type
of crystal linty. Crystalline type of starch after hydrolysis and native starch was similar.
However, hydrolysed starch tends to have some stronger peaks (Muxika and et. al., 2017). Cross
linked starch also have strong peaks. Nano particles produced from waxy maize starch has
reduced peak intensities when measured on X-ray diffractometers (González-Reza and et. al.,
2016).
FTIR -Fourier-transform infrared spectroscopy
It has been used as a tool for differentiating between different patterns of amylose appear
in granules of starch (Ballesteros and et. al., 2018). According to the researchers, IR spectrum of
starch was determine by the peaks near 3500, 3000, 1600, 1400, 1000, 800 and 500 cm−1.
Structure of A- and B- type of starch has been identified using polarized light microscopy, x-ray
diffraction and FTIR spectroscopy (Priyadarshi, Kumar and Negi, 2018). Both the type of starch
granules are presented with degrees of crystalline of 31.95% and 29.38 % (Mohebi and
Shahbazi, 2017).
Rheological properties
Research study has been conducted for obtaining prediction models for rheological
properties (MARBOH and GUPTA, 2018). Results of the study shows that granules of starch of
rice having low amylose content tends to have more cavities and pores which eventually leads to
swelling of rice granules (Popović and et. al., 2018). With change in temperature changes in
intensity and width of infrared spectroscopy bands shows some differences in the proportion area
of non-crystalline and crystalline was linked to amylose content rice (Cozmuta and et. al., 2018).
Rheological properties of food through which the starch will be prepare are important for quality
characterization (Janjarasskul and Suppakul, 2018). The study sought to obtain prediction
models for rheological characteristics and also to characterize the bread making quality of food
item which has been utilized using NIR spectroscopy and help to know the quality of food at
harvest moment (Hanani, 2018).
Amylose
12
Paraphrase This Document
Need a fresh take? Get an instant paraphrase of this document with our AI Paraphraser
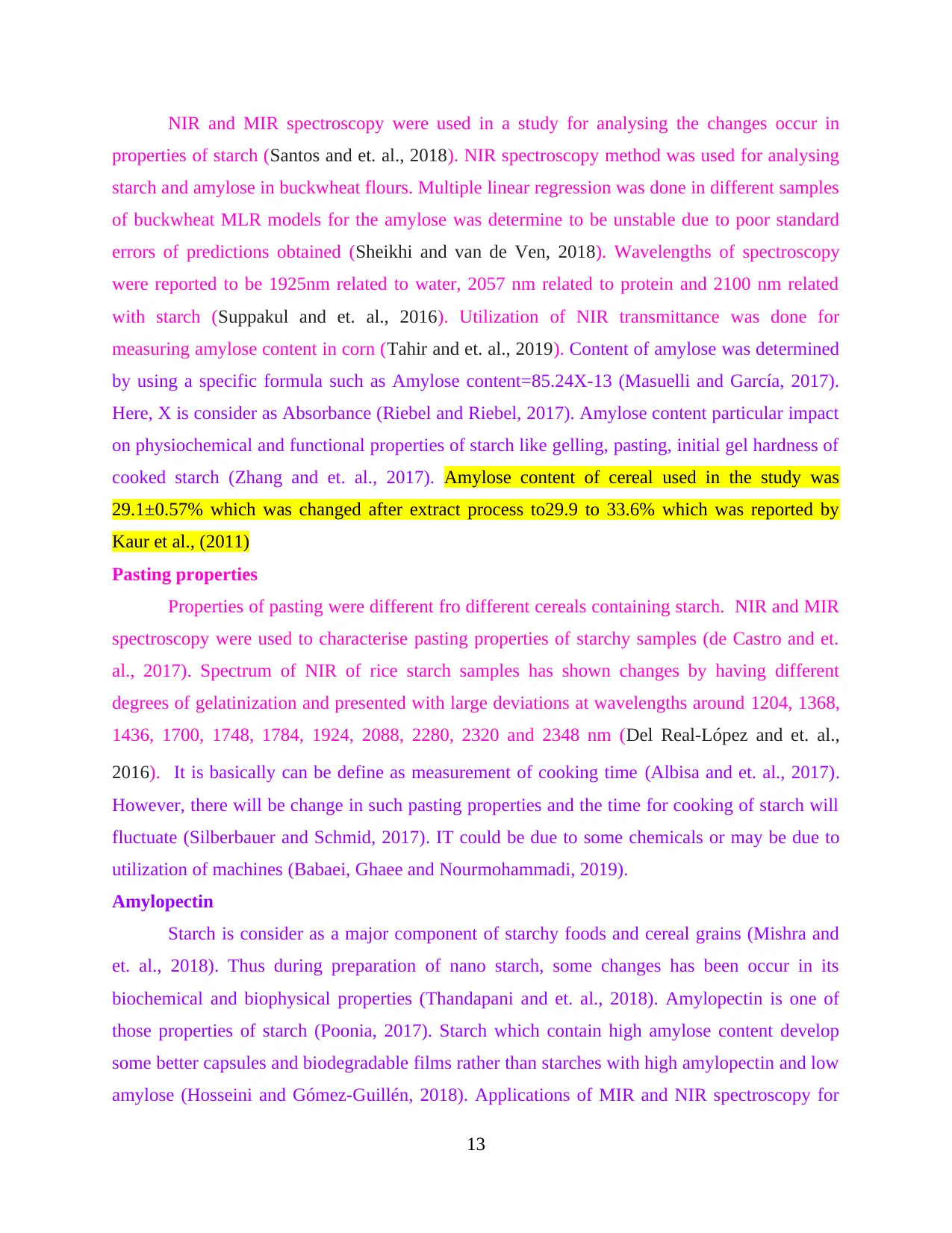
NIR and MIR spectroscopy were used in a study for analysing the changes occur in
properties of starch (Santos and et. al., 2018). NIR spectroscopy method was used for analysing
starch and amylose in buckwheat flours. Multiple linear regression was done in different samples
of buckwheat MLR models for the amylose was determine to be unstable due to poor standard
errors of predictions obtained (Sheikhi and van de Ven, 2018). Wavelengths of spectroscopy
were reported to be 1925nm related to water, 2057 nm related to protein and 2100 nm related
with starch (Suppakul and et. al., 2016). Utilization of NIR transmittance was done for
measuring amylose content in corn (Tahir and et. al., 2019). Content of amylose was determined
by using a specific formula such as Amylose content=85.24X-13 (Masuelli and García, 2017).
Here, X is consider as Absorbance (Riebel and Riebel, 2017). Amylose content particular impact
on physiochemical and functional properties of starch like gelling, pasting, initial gel hardness of
cooked starch (Zhang and et. al., 2017). Amylose content of cereal used in the study was
29.1±0.57% which was changed after extract process to29.9 to 33.6% which was reported by
Kaur et al., (2011)
Pasting properties
Properties of pasting were different fro different cereals containing starch. NIR and MIR
spectroscopy were used to characterise pasting properties of starchy samples (de Castro and et.
al., 2017). Spectrum of NIR of rice starch samples has shown changes by having different
degrees of gelatinization and presented with large deviations at wavelengths around 1204, 1368,
1436, 1700, 1748, 1784, 1924, 2088, 2280, 2320 and 2348 nm (Del Real-López and et. al.,
2016). It is basically can be define as measurement of cooking time (Albisa and et. al., 2017).
However, there will be change in such pasting properties and the time for cooking of starch will
fluctuate (Silberbauer and Schmid, 2017). IT could be due to some chemicals or may be due to
utilization of machines (Babaei, Ghaee and Nourmohammadi, 2019).
Amylopectin
Starch is consider as a major component of starchy foods and cereal grains (Mishra and
et. al., 2018). Thus during preparation of nano starch, some changes has been occur in its
biochemical and biophysical properties (Thandapani and et. al., 2018). Amylopectin is one of
those properties of starch (Poonia, 2017). Starch which contain high amylose content develop
some better capsules and biodegradable films rather than starches with high amylopectin and low
amylose (Hosseini and Gómez-Guillén, 2018). Applications of MIR and NIR spectroscopy for
13
properties of starch (Santos and et. al., 2018). NIR spectroscopy method was used for analysing
starch and amylose in buckwheat flours. Multiple linear regression was done in different samples
of buckwheat MLR models for the amylose was determine to be unstable due to poor standard
errors of predictions obtained (Sheikhi and van de Ven, 2018). Wavelengths of spectroscopy
were reported to be 1925nm related to water, 2057 nm related to protein and 2100 nm related
with starch (Suppakul and et. al., 2016). Utilization of NIR transmittance was done for
measuring amylose content in corn (Tahir and et. al., 2019). Content of amylose was determined
by using a specific formula such as Amylose content=85.24X-13 (Masuelli and García, 2017).
Here, X is consider as Absorbance (Riebel and Riebel, 2017). Amylose content particular impact
on physiochemical and functional properties of starch like gelling, pasting, initial gel hardness of
cooked starch (Zhang and et. al., 2017). Amylose content of cereal used in the study was
29.1±0.57% which was changed after extract process to29.9 to 33.6% which was reported by
Kaur et al., (2011)
Pasting properties
Properties of pasting were different fro different cereals containing starch. NIR and MIR
spectroscopy were used to characterise pasting properties of starchy samples (de Castro and et.
al., 2017). Spectrum of NIR of rice starch samples has shown changes by having different
degrees of gelatinization and presented with large deviations at wavelengths around 1204, 1368,
1436, 1700, 1748, 1784, 1924, 2088, 2280, 2320 and 2348 nm (Del Real-López and et. al.,
2016). It is basically can be define as measurement of cooking time (Albisa and et. al., 2017).
However, there will be change in such pasting properties and the time for cooking of starch will
fluctuate (Silberbauer and Schmid, 2017). IT could be due to some chemicals or may be due to
utilization of machines (Babaei, Ghaee and Nourmohammadi, 2019).
Amylopectin
Starch is consider as a major component of starchy foods and cereal grains (Mishra and
et. al., 2018). Thus during preparation of nano starch, some changes has been occur in its
biochemical and biophysical properties (Thandapani and et. al., 2018). Amylopectin is one of
those properties of starch (Poonia, 2017). Starch which contain high amylose content develop
some better capsules and biodegradable films rather than starches with high amylopectin and low
amylose (Hosseini and Gómez-Guillén, 2018). Applications of MIR and NIR spectroscopy for
13
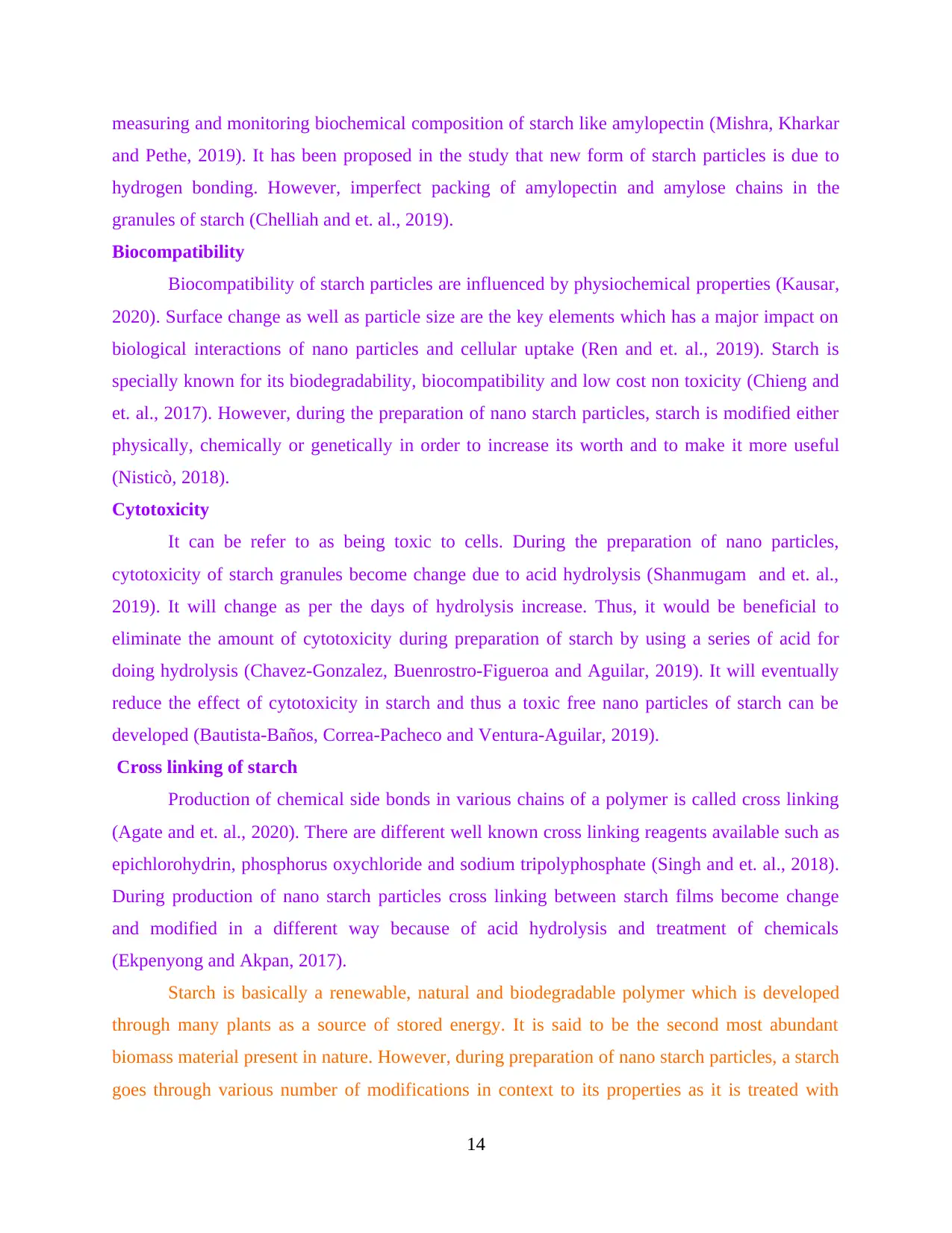
measuring and monitoring biochemical composition of starch like amylopectin (Mishra, Kharkar
and Pethe, 2019). It has been proposed in the study that new form of starch particles is due to
hydrogen bonding. However, imperfect packing of amylopectin and amylose chains in the
granules of starch (Chelliah and et. al., 2019).
Biocompatibility
Biocompatibility of starch particles are influenced by physiochemical properties (Kausar,
2020). Surface change as well as particle size are the key elements which has a major impact on
biological interactions of nano particles and cellular uptake (Ren and et. al., 2019). Starch is
specially known for its biodegradability, biocompatibility and low cost non toxicity (Chieng and
et. al., 2017). However, during the preparation of nano starch particles, starch is modified either
physically, chemically or genetically in order to increase its worth and to make it more useful
(Nisticò, 2018).
Cytotoxicity
It can be refer to as being toxic to cells. During the preparation of nano particles,
cytotoxicity of starch granules become change due to acid hydrolysis (Shanmugam and et. al.,
2019). It will change as per the days of hydrolysis increase. Thus, it would be beneficial to
eliminate the amount of cytotoxicity during preparation of starch by using a series of acid for
doing hydrolysis (Chavez-Gonzalez, Buenrostro-Figueroa and Aguilar, 2019). It will eventually
reduce the effect of cytotoxicity in starch and thus a toxic free nano particles of starch can be
developed (Bautista‐Baños, Correa‐Pacheco and Ventura‐Aguilar, 2019).
Cross linking of starch
Production of chemical side bonds in various chains of a polymer is called cross linking
(Agate and et. al., 2020). There are different well known cross linking reagents available such as
epichlorohydrin, phosphorus oxychloride and sodium tripolyphosphate (Singh and et. al., 2018).
During production of nano starch particles cross linking between starch films become change
and modified in a different way because of acid hydrolysis and treatment of chemicals
(Ekpenyong and Akpan, 2017).
Starch is basically a renewable, natural and biodegradable polymer which is developed
through many plants as a source of stored energy. It is said to be the second most abundant
biomass material present in nature. However, during preparation of nano starch particles, a starch
goes through various number of modifications in context to its properties as it is treated with
14
and Pethe, 2019). It has been proposed in the study that new form of starch particles is due to
hydrogen bonding. However, imperfect packing of amylopectin and amylose chains in the
granules of starch (Chelliah and et. al., 2019).
Biocompatibility
Biocompatibility of starch particles are influenced by physiochemical properties (Kausar,
2020). Surface change as well as particle size are the key elements which has a major impact on
biological interactions of nano particles and cellular uptake (Ren and et. al., 2019). Starch is
specially known for its biodegradability, biocompatibility and low cost non toxicity (Chieng and
et. al., 2017). However, during the preparation of nano starch particles, starch is modified either
physically, chemically or genetically in order to increase its worth and to make it more useful
(Nisticò, 2018).
Cytotoxicity
It can be refer to as being toxic to cells. During the preparation of nano particles,
cytotoxicity of starch granules become change due to acid hydrolysis (Shanmugam and et. al.,
2019). It will change as per the days of hydrolysis increase. Thus, it would be beneficial to
eliminate the amount of cytotoxicity during preparation of starch by using a series of acid for
doing hydrolysis (Chavez-Gonzalez, Buenrostro-Figueroa and Aguilar, 2019). It will eventually
reduce the effect of cytotoxicity in starch and thus a toxic free nano particles of starch can be
developed (Bautista‐Baños, Correa‐Pacheco and Ventura‐Aguilar, 2019).
Cross linking of starch
Production of chemical side bonds in various chains of a polymer is called cross linking
(Agate and et. al., 2020). There are different well known cross linking reagents available such as
epichlorohydrin, phosphorus oxychloride and sodium tripolyphosphate (Singh and et. al., 2018).
During production of nano starch particles cross linking between starch films become change
and modified in a different way because of acid hydrolysis and treatment of chemicals
(Ekpenyong and Akpan, 2017).
Starch is basically a renewable, natural and biodegradable polymer which is developed
through many plants as a source of stored energy. It is said to be the second most abundant
biomass material present in nature. However, during preparation of nano starch particles, a starch
goes through various number of modifications in context to its properties as it is treated with
14
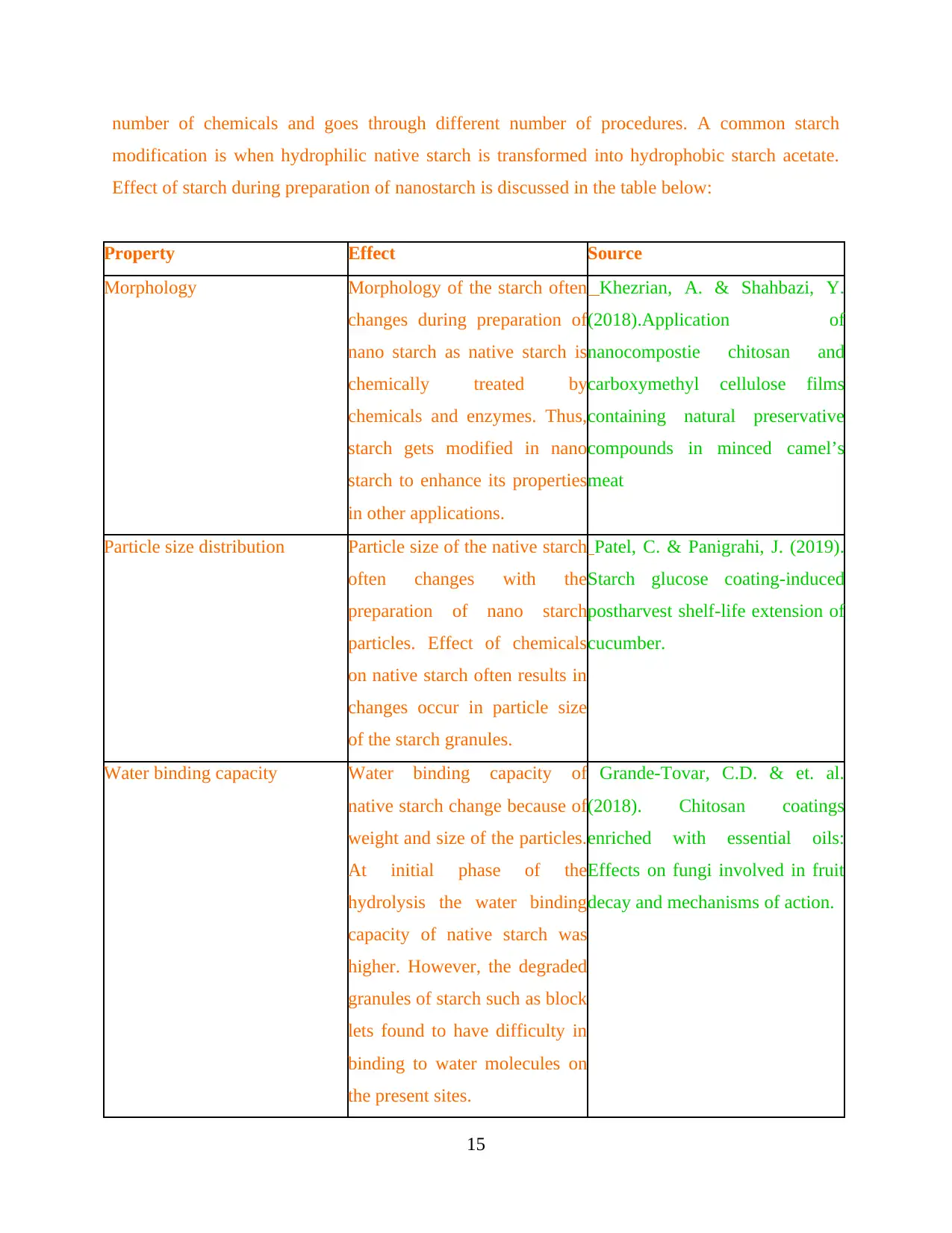
number of chemicals and goes through different number of procedures. A common starch
modification is when hydrophilic native starch is transformed into hydrophobic starch acetate.
Effect of starch during preparation of nanostarch is discussed in the table below:
Property Effect Source
Morphology Morphology of the starch often
changes during preparation of
nano starch as native starch is
chemically treated by
chemicals and enzymes. Thus,
starch gets modified in nano
starch to enhance its properties
in other applications.
Khezrian, A. & Shahbazi, Y.
(2018).Application of
nanocompostie chitosan and
carboxymethyl cellulose films
containing natural preservative
compounds in minced camel’s
meat
Particle size distribution Particle size of the native starch
often changes with the
preparation of nano starch
particles. Effect of chemicals
on native starch often results in
changes occur in particle size
of the starch granules.
Patel, C. & Panigrahi, J. (2019).
Starch glucose coating-induced
postharvest shelf-life extension of
cucumber.
Water binding capacity Water binding capacity of
native starch change because of
weight and size of the particles.
At initial phase of the
hydrolysis the water binding
capacity of native starch was
higher. However, the degraded
granules of starch such as block
lets found to have difficulty in
binding to water molecules on
the present sites.
Grande-Tovar, C.D. & et. al.
(2018). Chitosan coatings
enriched with essential oils:
Effects on fungi involved in fruit
decay and mechanisms of action.
15
modification is when hydrophilic native starch is transformed into hydrophobic starch acetate.
Effect of starch during preparation of nanostarch is discussed in the table below:
Property Effect Source
Morphology Morphology of the starch often
changes during preparation of
nano starch as native starch is
chemically treated by
chemicals and enzymes. Thus,
starch gets modified in nano
starch to enhance its properties
in other applications.
Khezrian, A. & Shahbazi, Y.
(2018).Application of
nanocompostie chitosan and
carboxymethyl cellulose films
containing natural preservative
compounds in minced camel’s
meat
Particle size distribution Particle size of the native starch
often changes with the
preparation of nano starch
particles. Effect of chemicals
on native starch often results in
changes occur in particle size
of the starch granules.
Patel, C. & Panigrahi, J. (2019).
Starch glucose coating-induced
postharvest shelf-life extension of
cucumber.
Water binding capacity Water binding capacity of
native starch change because of
weight and size of the particles.
At initial phase of the
hydrolysis the water binding
capacity of native starch was
higher. However, the degraded
granules of starch such as block
lets found to have difficulty in
binding to water molecules on
the present sites.
Grande-Tovar, C.D. & et. al.
(2018). Chitosan coatings
enriched with essential oils:
Effects on fungi involved in fruit
decay and mechanisms of action.
15
Secure Best Marks with AI Grader
Need help grading? Try our AI Grader for instant feedback on your assignments.
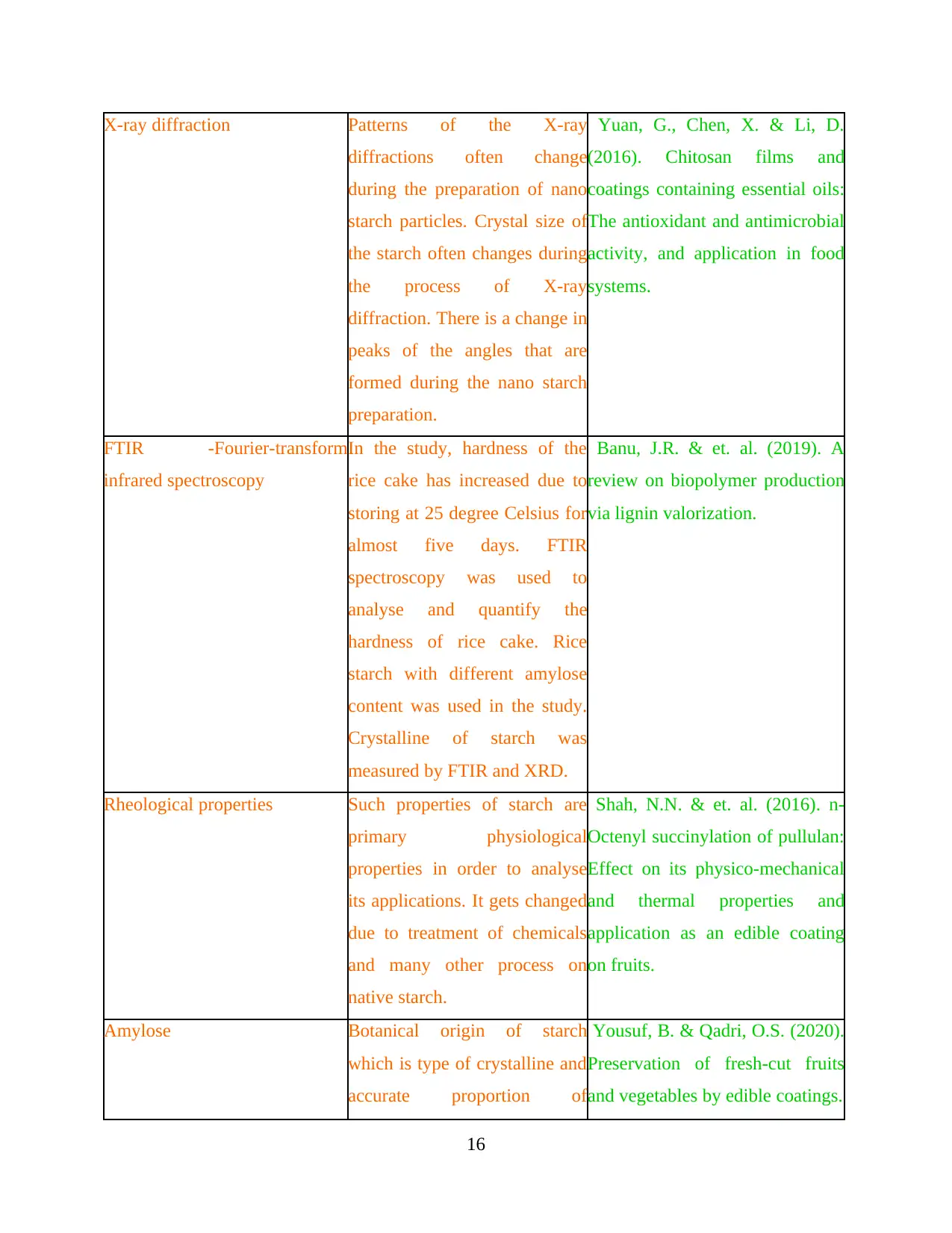
X-ray diffraction Patterns of the X-ray
diffractions often change
during the preparation of nano
starch particles. Crystal size of
the starch often changes during
the process of X-ray
diffraction. There is a change in
peaks of the angles that are
formed during the nano starch
preparation.
Yuan, G., Chen, X. & Li, D.
(2016). Chitosan films and
coatings containing essential oils:
The antioxidant and antimicrobial
activity, and application in food
systems.
FTIR -Fourier-transform
infrared spectroscopy
In the study, hardness of the
rice cake has increased due to
storing at 25 degree Celsius for
almost five days. FTIR
spectroscopy was used to
analyse and quantify the
hardness of rice cake. Rice
starch with different amylose
content was used in the study.
Crystalline of starch was
measured by FTIR and XRD.
Banu, J.R. & et. al. (2019). A
review on biopolymer production
via lignin valorization.
Rheological properties Such properties of starch are
primary physiological
properties in order to analyse
its applications. It gets changed
due to treatment of chemicals
and many other process on
native starch.
Shah, N.N. & et. al. (2016). n-
Octenyl succinylation of pullulan:
Effect on its physico-mechanical
and thermal properties and
application as an edible coating
on fruits.
Amylose Botanical origin of starch
which is type of crystalline and
accurate proportion of
Yousuf, B. & Qadri, O.S. (2020).
Preservation of fresh-cut fruits
and vegetables by edible coatings.
16
diffractions often change
during the preparation of nano
starch particles. Crystal size of
the starch often changes during
the process of X-ray
diffraction. There is a change in
peaks of the angles that are
formed during the nano starch
preparation.
Yuan, G., Chen, X. & Li, D.
(2016). Chitosan films and
coatings containing essential oils:
The antioxidant and antimicrobial
activity, and application in food
systems.
FTIR -Fourier-transform
infrared spectroscopy
In the study, hardness of the
rice cake has increased due to
storing at 25 degree Celsius for
almost five days. FTIR
spectroscopy was used to
analyse and quantify the
hardness of rice cake. Rice
starch with different amylose
content was used in the study.
Crystalline of starch was
measured by FTIR and XRD.
Banu, J.R. & et. al. (2019). A
review on biopolymer production
via lignin valorization.
Rheological properties Such properties of starch are
primary physiological
properties in order to analyse
its applications. It gets changed
due to treatment of chemicals
and many other process on
native starch.
Shah, N.N. & et. al. (2016). n-
Octenyl succinylation of pullulan:
Effect on its physico-mechanical
and thermal properties and
application as an edible coating
on fruits.
Amylose Botanical origin of starch
which is type of crystalline and
accurate proportion of
Yousuf, B. & Qadri, O.S. (2020).
Preservation of fresh-cut fruits
and vegetables by edible coatings.
16
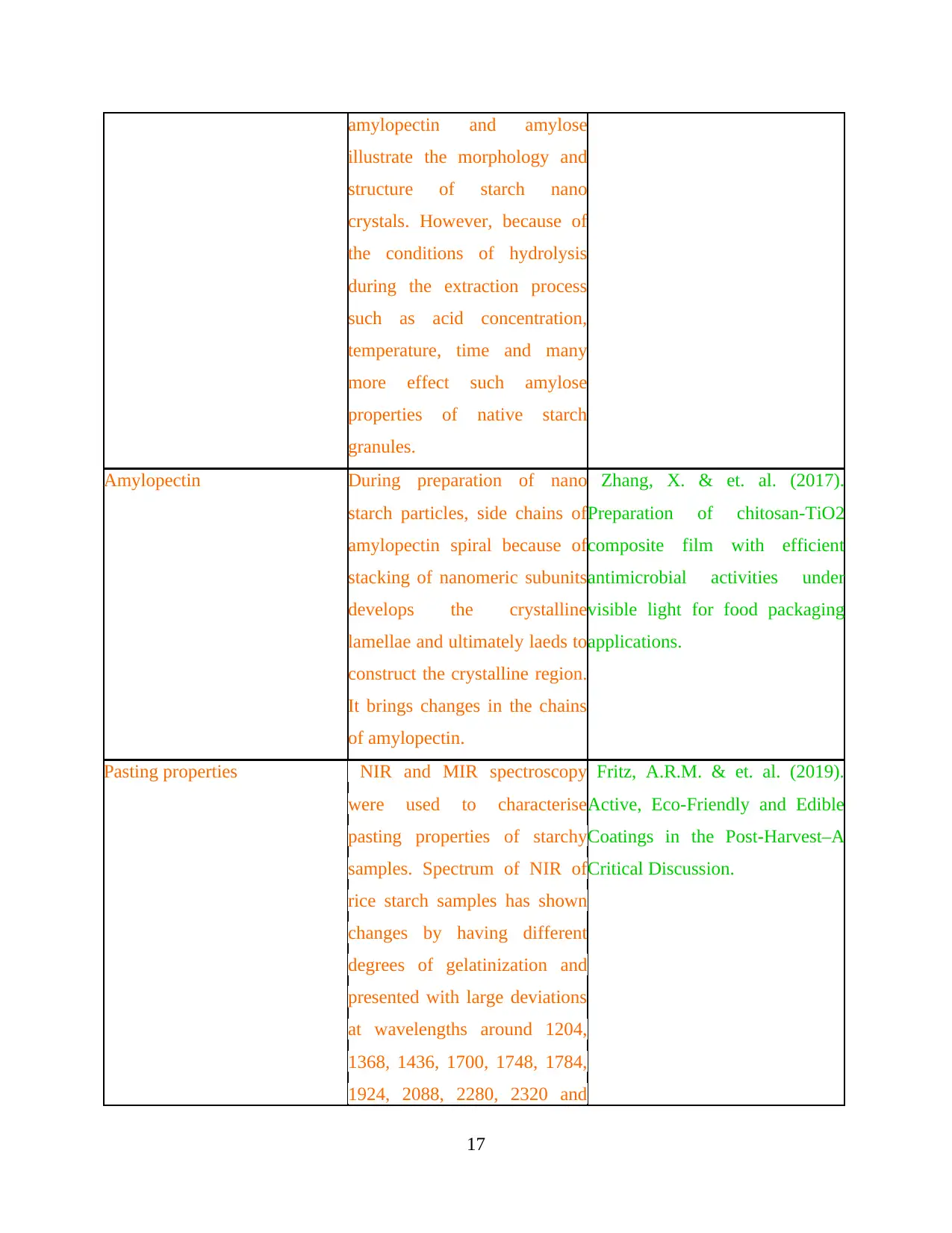
amylopectin and amylose
illustrate the morphology and
structure of starch nano
crystals. However, because of
the conditions of hydrolysis
during the extraction process
such as acid concentration,
temperature, time and many
more effect such amylose
properties of native starch
granules.
Amylopectin During preparation of nano
starch particles, side chains of
amylopectin spiral because of
stacking of nanomeric subunits
develops the crystalline
lamellae and ultimately laeds to
construct the crystalline region.
It brings changes in the chains
of amylopectin.
Zhang, X. & et. al. (2017).
Preparation of chitosan-TiO2
composite film with efficient
antimicrobial activities under
visible light for food packaging
applications.
Pasting properties NIR and MIR spectroscopy
were used to characterise
pasting properties of starchy
samples. Spectrum of NIR of
rice starch samples has shown
changes by having different
degrees of gelatinization and
presented with large deviations
at wavelengths around 1204,
1368, 1436, 1700, 1748, 1784,
1924, 2088, 2280, 2320 and
Fritz, A.R.M. & et. al. (2019).
Active, Eco-Friendly and Edible
Coatings in the Post-Harvest–A
Critical Discussion.
17
illustrate the morphology and
structure of starch nano
crystals. However, because of
the conditions of hydrolysis
during the extraction process
such as acid concentration,
temperature, time and many
more effect such amylose
properties of native starch
granules.
Amylopectin During preparation of nano
starch particles, side chains of
amylopectin spiral because of
stacking of nanomeric subunits
develops the crystalline
lamellae and ultimately laeds to
construct the crystalline region.
It brings changes in the chains
of amylopectin.
Zhang, X. & et. al. (2017).
Preparation of chitosan-TiO2
composite film with efficient
antimicrobial activities under
visible light for food packaging
applications.
Pasting properties NIR and MIR spectroscopy
were used to characterise
pasting properties of starchy
samples. Spectrum of NIR of
rice starch samples has shown
changes by having different
degrees of gelatinization and
presented with large deviations
at wavelengths around 1204,
1368, 1436, 1700, 1748, 1784,
1924, 2088, 2280, 2320 and
Fritz, A.R.M. & et. al. (2019).
Active, Eco-Friendly and Edible
Coatings in the Post-Harvest–A
Critical Discussion.
17
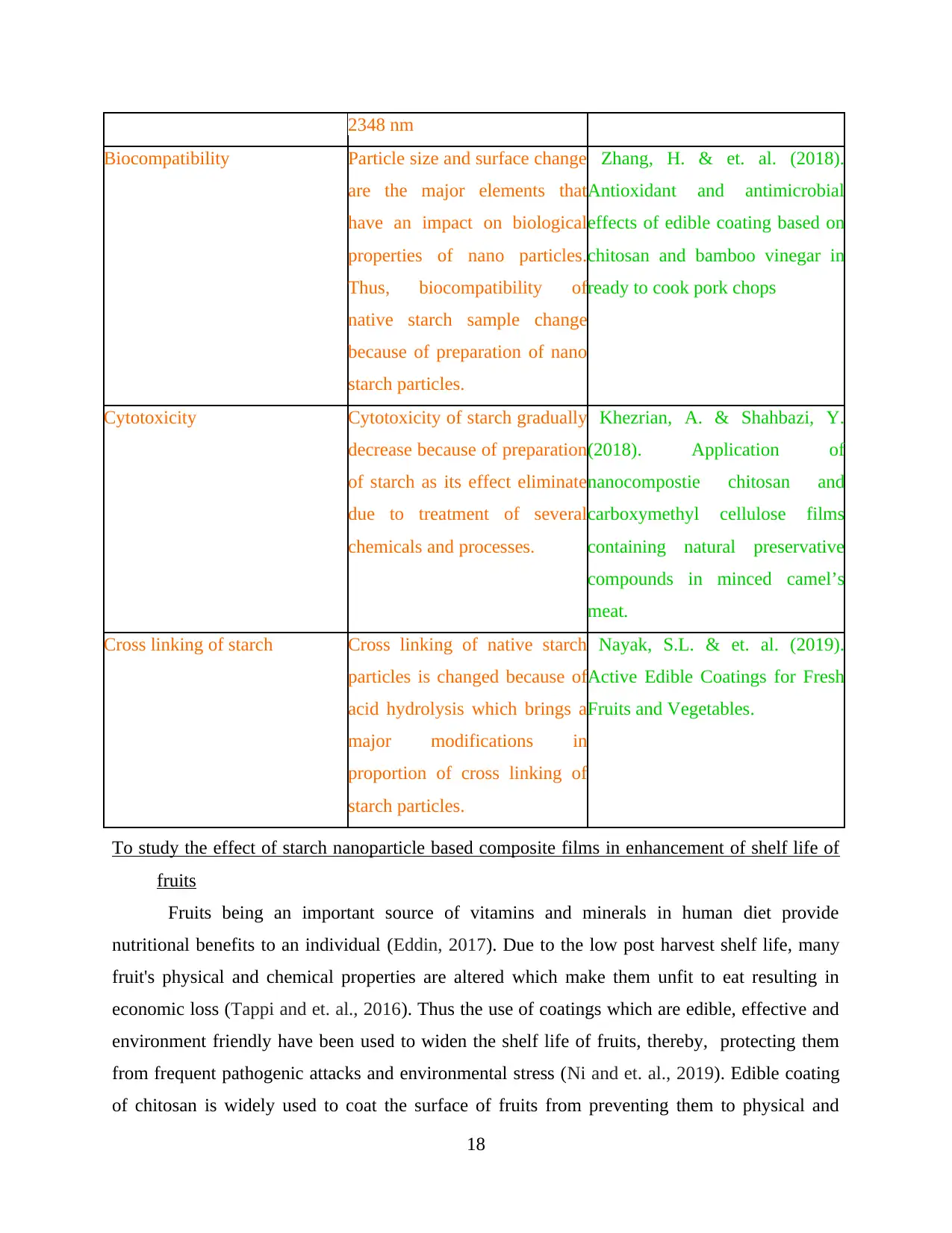
2348 nm
Biocompatibility Particle size and surface change
are the major elements that
have an impact on biological
properties of nano particles.
Thus, biocompatibility of
native starch sample change
because of preparation of nano
starch particles.
Zhang, H. & et. al. (2018).
Antioxidant and antimicrobial
effects of edible coating based on
chitosan and bamboo vinegar in
ready to cook pork chops
Cytotoxicity Cytotoxicity of starch gradually
decrease because of preparation
of starch as its effect eliminate
due to treatment of several
chemicals and processes.
Khezrian, A. & Shahbazi, Y.
(2018). Application of
nanocompostie chitosan and
carboxymethyl cellulose films
containing natural preservative
compounds in minced camel’s
meat.
Cross linking of starch Cross linking of native starch
particles is changed because of
acid hydrolysis which brings a
major modifications in
proportion of cross linking of
starch particles.
Nayak, S.L. & et. al. (2019).
Active Edible Coatings for Fresh
Fruits and Vegetables.
To study the effect of starch nanoparticle based composite films in enhancement of shelf life of
fruits
Fruits being an important source of vitamins and minerals in human diet provide
nutritional benefits to an individual (Eddin, 2017). Due to the low post harvest shelf life, many
fruit's physical and chemical properties are altered which make them unfit to eat resulting in
economic loss (Tappi and et. al., 2016). Thus the use of coatings which are edible, effective and
environment friendly have been used to widen the shelf life of fruits, thereby, protecting them
from frequent pathogenic attacks and environmental stress (Ni and et. al., 2019). Edible coating
of chitosan is widely used to coat the surface of fruits from preventing them to physical and
18
Biocompatibility Particle size and surface change
are the major elements that
have an impact on biological
properties of nano particles.
Thus, biocompatibility of
native starch sample change
because of preparation of nano
starch particles.
Zhang, H. & et. al. (2018).
Antioxidant and antimicrobial
effects of edible coating based on
chitosan and bamboo vinegar in
ready to cook pork chops
Cytotoxicity Cytotoxicity of starch gradually
decrease because of preparation
of starch as its effect eliminate
due to treatment of several
chemicals and processes.
Khezrian, A. & Shahbazi, Y.
(2018). Application of
nanocompostie chitosan and
carboxymethyl cellulose films
containing natural preservative
compounds in minced camel’s
meat.
Cross linking of starch Cross linking of native starch
particles is changed because of
acid hydrolysis which brings a
major modifications in
proportion of cross linking of
starch particles.
Nayak, S.L. & et. al. (2019).
Active Edible Coatings for Fresh
Fruits and Vegetables.
To study the effect of starch nanoparticle based composite films in enhancement of shelf life of
fruits
Fruits being an important source of vitamins and minerals in human diet provide
nutritional benefits to an individual (Eddin, 2017). Due to the low post harvest shelf life, many
fruit's physical and chemical properties are altered which make them unfit to eat resulting in
economic loss (Tappi and et. al., 2016). Thus the use of coatings which are edible, effective and
environment friendly have been used to widen the shelf life of fruits, thereby, protecting them
from frequent pathogenic attacks and environmental stress (Ni and et. al., 2019). Edible coating
of chitosan is widely used to coat the surface of fruits from preventing them to physical and
18
Paraphrase This Document
Need a fresh take? Get an instant paraphrase of this document with our AI Paraphraser
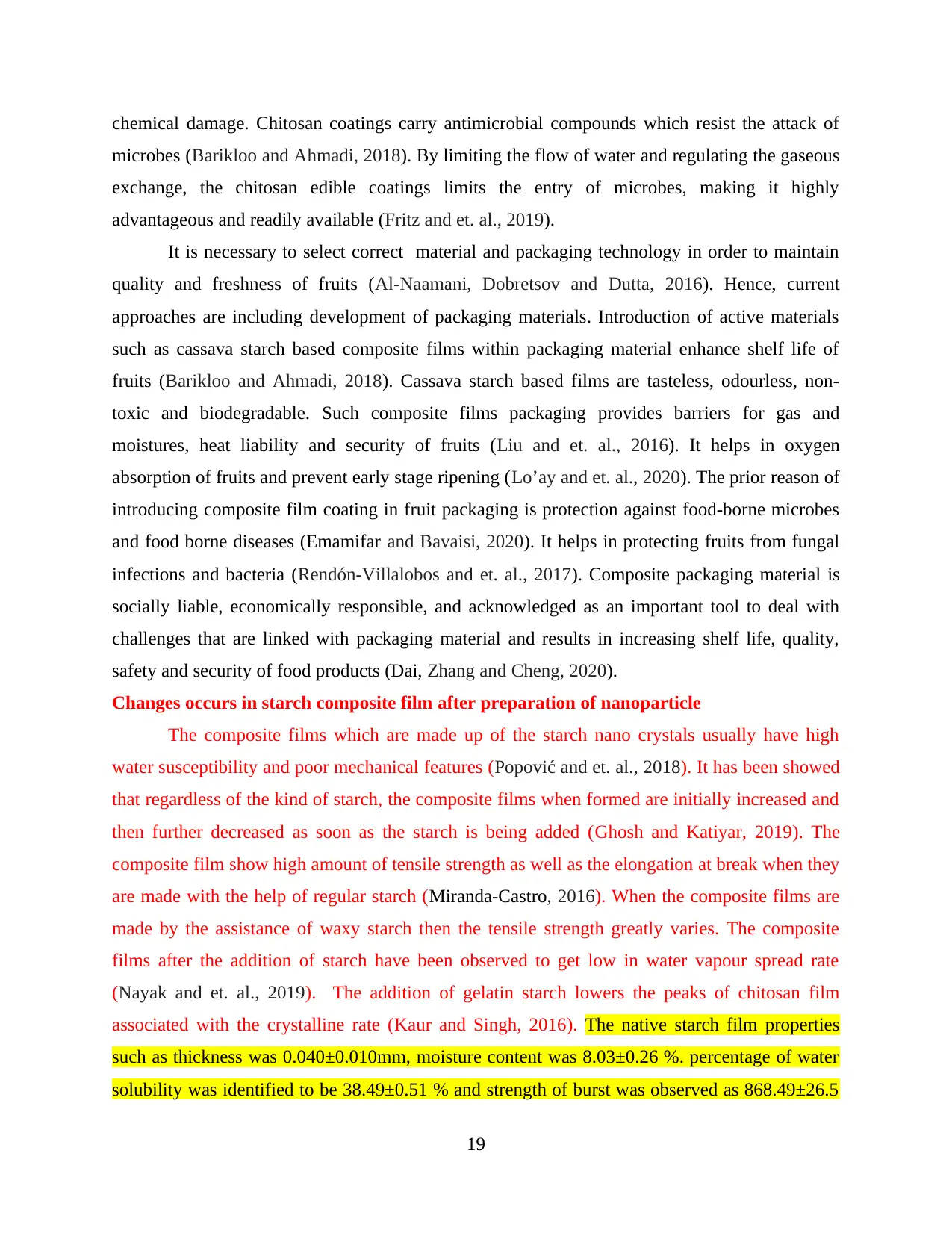
chemical damage. Chitosan coatings carry antimicrobial compounds which resist the attack of
microbes (Barikloo and Ahmadi, 2018). By limiting the flow of water and regulating the gaseous
exchange, the chitosan edible coatings limits the entry of microbes, making it highly
advantageous and readily available (Fritz and et. al., 2019).
It is necessary to select correct material and packaging technology in order to maintain
quality and freshness of fruits (Al-Naamani, Dobretsov and Dutta, 2016). Hence, current
approaches are including development of packaging materials. Introduction of active materials
such as cassava starch based composite films within packaging material enhance shelf life of
fruits (Barikloo and Ahmadi, 2018). Cassava starch based films are tasteless, odourless, non-
toxic and biodegradable. Such composite films packaging provides barriers for gas and
moistures, heat liability and security of fruits (Liu and et. al., 2016). It helps in oxygen
absorption of fruits and prevent early stage ripening (Lo’ay and et. al., 2020). The prior reason of
introducing composite film coating in fruit packaging is protection against food-borne microbes
and food borne diseases (Emamifar and Bavaisi, 2020). It helps in protecting fruits from fungal
infections and bacteria (Rendón-Villalobos and et. al., 2017). Composite packaging material is
socially liable, economically responsible, and acknowledged as an important tool to deal with
challenges that are linked with packaging material and results in increasing shelf life, quality,
safety and security of food products (Dai, Zhang and Cheng, 2020).
Changes occurs in starch composite film after preparation of nanoparticle
The composite films which are made up of the starch nano crystals usually have high
water susceptibility and poor mechanical features (Popović and et. al., 2018). It has been showed
that regardless of the kind of starch, the composite films when formed are initially increased and
then further decreased as soon as the starch is being added (Ghosh and Katiyar, 2019). The
composite film show high amount of tensile strength as well as the elongation at break when they
are made with the help of regular starch (Miranda-Castro, 2016). When the composite films are
made by the assistance of waxy starch then the tensile strength greatly varies. The composite
films after the addition of starch have been observed to get low in water vapour spread rate
(Nayak and et. al., 2019). The addition of gelatin starch lowers the peaks of chitosan film
associated with the crystalline rate (Kaur and Singh, 2016). The native starch film properties
such as thickness was 0.040±0.010mm, moisture content was 8.03±0.26 %. percentage of water
solubility was identified to be 38.49±0.51 % and strength of burst was observed as 868.49±26.5
19
microbes (Barikloo and Ahmadi, 2018). By limiting the flow of water and regulating the gaseous
exchange, the chitosan edible coatings limits the entry of microbes, making it highly
advantageous and readily available (Fritz and et. al., 2019).
It is necessary to select correct material and packaging technology in order to maintain
quality and freshness of fruits (Al-Naamani, Dobretsov and Dutta, 2016). Hence, current
approaches are including development of packaging materials. Introduction of active materials
such as cassava starch based composite films within packaging material enhance shelf life of
fruits (Barikloo and Ahmadi, 2018). Cassava starch based films are tasteless, odourless, non-
toxic and biodegradable. Such composite films packaging provides barriers for gas and
moistures, heat liability and security of fruits (Liu and et. al., 2016). It helps in oxygen
absorption of fruits and prevent early stage ripening (Lo’ay and et. al., 2020). The prior reason of
introducing composite film coating in fruit packaging is protection against food-borne microbes
and food borne diseases (Emamifar and Bavaisi, 2020). It helps in protecting fruits from fungal
infections and bacteria (Rendón-Villalobos and et. al., 2017). Composite packaging material is
socially liable, economically responsible, and acknowledged as an important tool to deal with
challenges that are linked with packaging material and results in increasing shelf life, quality,
safety and security of food products (Dai, Zhang and Cheng, 2020).
Changes occurs in starch composite film after preparation of nanoparticle
The composite films which are made up of the starch nano crystals usually have high
water susceptibility and poor mechanical features (Popović and et. al., 2018). It has been showed
that regardless of the kind of starch, the composite films when formed are initially increased and
then further decreased as soon as the starch is being added (Ghosh and Katiyar, 2019). The
composite film show high amount of tensile strength as well as the elongation at break when they
are made with the help of regular starch (Miranda-Castro, 2016). When the composite films are
made by the assistance of waxy starch then the tensile strength greatly varies. The composite
films after the addition of starch have been observed to get low in water vapour spread rate
(Nayak and et. al., 2019). The addition of gelatin starch lowers the peaks of chitosan film
associated with the crystalline rate (Kaur and Singh, 2016). The native starch film properties
such as thickness was 0.040±0.010mm, moisture content was 8.03±0.26 %. percentage of water
solubility was identified to be 38.49±0.51 % and strength of burst was observed as 868.49±26.5
19
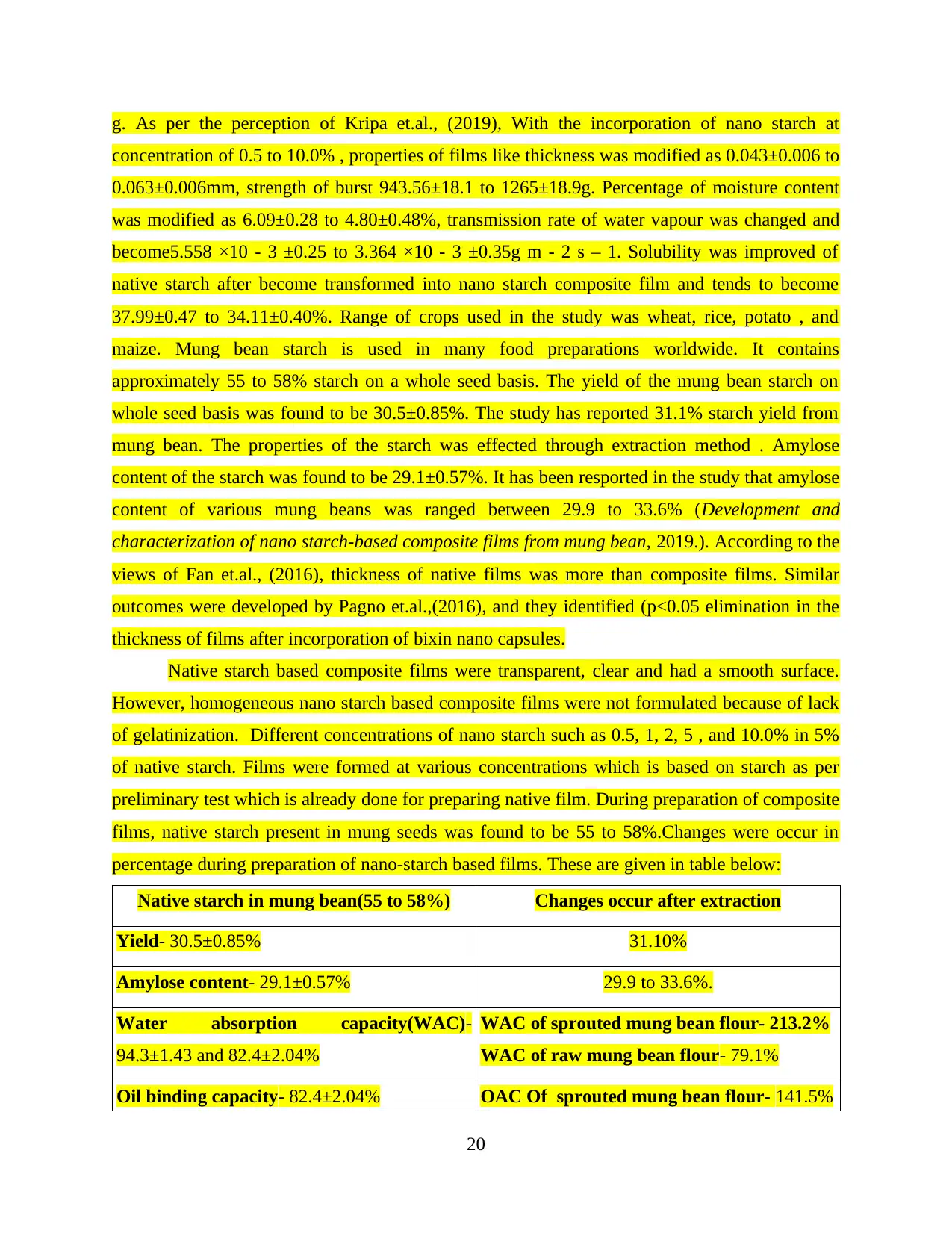
g. As per the perception of Kripa et.al., (2019), With the incorporation of nano starch at
concentration of 0.5 to 10.0% , properties of films like thickness was modified as 0.043±0.006 to
0.063±0.006mm, strength of burst 943.56±18.1 to 1265±18.9g. Percentage of moisture content
was modified as 6.09±0.28 to 4.80±0.48%, transmission rate of water vapour was changed and
become5.558 ×10 - 3 ±0.25 to 3.364 ×10 - 3 ±0.35g m - 2 s – 1. Solubility was improved of
native starch after become transformed into nano starch composite film and tends to become
37.99±0.47 to 34.11±0.40%. Range of crops used in the study was wheat, rice, potato , and
maize. Mung bean starch is used in many food preparations worldwide. It contains
approximately 55 to 58% starch on a whole seed basis. The yield of the mung bean starch on
whole seed basis was found to be 30.5±0.85%. The study has reported 31.1% starch yield from
mung bean. The properties of the starch was effected through extraction method . Amylose
content of the starch was found to be 29.1±0.57%. It has been resported in the study that amylose
content of various mung beans was ranged between 29.9 to 33.6% (Development and
characterization of nano starch-based composite films from mung bean, 2019.). According to the
views of Fan et.al., (2016), thickness of native films was more than composite films. Similar
outcomes were developed by Pagno et.al.,(2016), and they identified (p<0.05 elimination in the
thickness of films after incorporation of bixin nano capsules.
Native starch based composite films were transparent, clear and had a smooth surface.
However, homogeneous nano starch based composite films were not formulated because of lack
of gelatinization. Different concentrations of nano starch such as 0.5, 1, 2, 5 , and 10.0% in 5%
of native starch. Films were formed at various concentrations which is based on starch as per
preliminary test which is already done for preparing native film. During preparation of composite
films, native starch present in mung seeds was found to be 55 to 58%.Changes were occur in
percentage during preparation of nano-starch based films. These are given in table below:
Native starch in mung bean(55 to 58%) Changes occur after extraction
Yield- 30.5±0.85% 31.10%
Amylose content- 29.1±0.57% 29.9 to 33.6%.
Water absorption capacity(WAC)-
94.3±1.43 and 82.4±2.04%
WAC of sprouted mung bean flour- 213.2%
WAC of raw mung bean flour- 79.1%
Oil binding capacity- 82.4±2.04% OAC Of sprouted mung bean flour- 141.5%
20
concentration of 0.5 to 10.0% , properties of films like thickness was modified as 0.043±0.006 to
0.063±0.006mm, strength of burst 943.56±18.1 to 1265±18.9g. Percentage of moisture content
was modified as 6.09±0.28 to 4.80±0.48%, transmission rate of water vapour was changed and
become5.558 ×10 - 3 ±0.25 to 3.364 ×10 - 3 ±0.35g m - 2 s – 1. Solubility was improved of
native starch after become transformed into nano starch composite film and tends to become
37.99±0.47 to 34.11±0.40%. Range of crops used in the study was wheat, rice, potato , and
maize. Mung bean starch is used in many food preparations worldwide. It contains
approximately 55 to 58% starch on a whole seed basis. The yield of the mung bean starch on
whole seed basis was found to be 30.5±0.85%. The study has reported 31.1% starch yield from
mung bean. The properties of the starch was effected through extraction method . Amylose
content of the starch was found to be 29.1±0.57%. It has been resported in the study that amylose
content of various mung beans was ranged between 29.9 to 33.6% (Development and
characterization of nano starch-based composite films from mung bean, 2019.). According to the
views of Fan et.al., (2016), thickness of native films was more than composite films. Similar
outcomes were developed by Pagno et.al.,(2016), and they identified (p<0.05 elimination in the
thickness of films after incorporation of bixin nano capsules.
Native starch based composite films were transparent, clear and had a smooth surface.
However, homogeneous nano starch based composite films were not formulated because of lack
of gelatinization. Different concentrations of nano starch such as 0.5, 1, 2, 5 , and 10.0% in 5%
of native starch. Films were formed at various concentrations which is based on starch as per
preliminary test which is already done for preparing native film. During preparation of composite
films, native starch present in mung seeds was found to be 55 to 58%.Changes were occur in
percentage during preparation of nano-starch based films. These are given in table below:
Native starch in mung bean(55 to 58%) Changes occur after extraction
Yield- 30.5±0.85% 31.10%
Amylose content- 29.1±0.57% 29.9 to 33.6%.
Water absorption capacity(WAC)-
94.3±1.43 and 82.4±2.04%
WAC of sprouted mung bean flour- 213.2%
WAC of raw mung bean flour- 79.1%
Oil binding capacity- 82.4±2.04% OAC Of sprouted mung bean flour- 141.5%
20
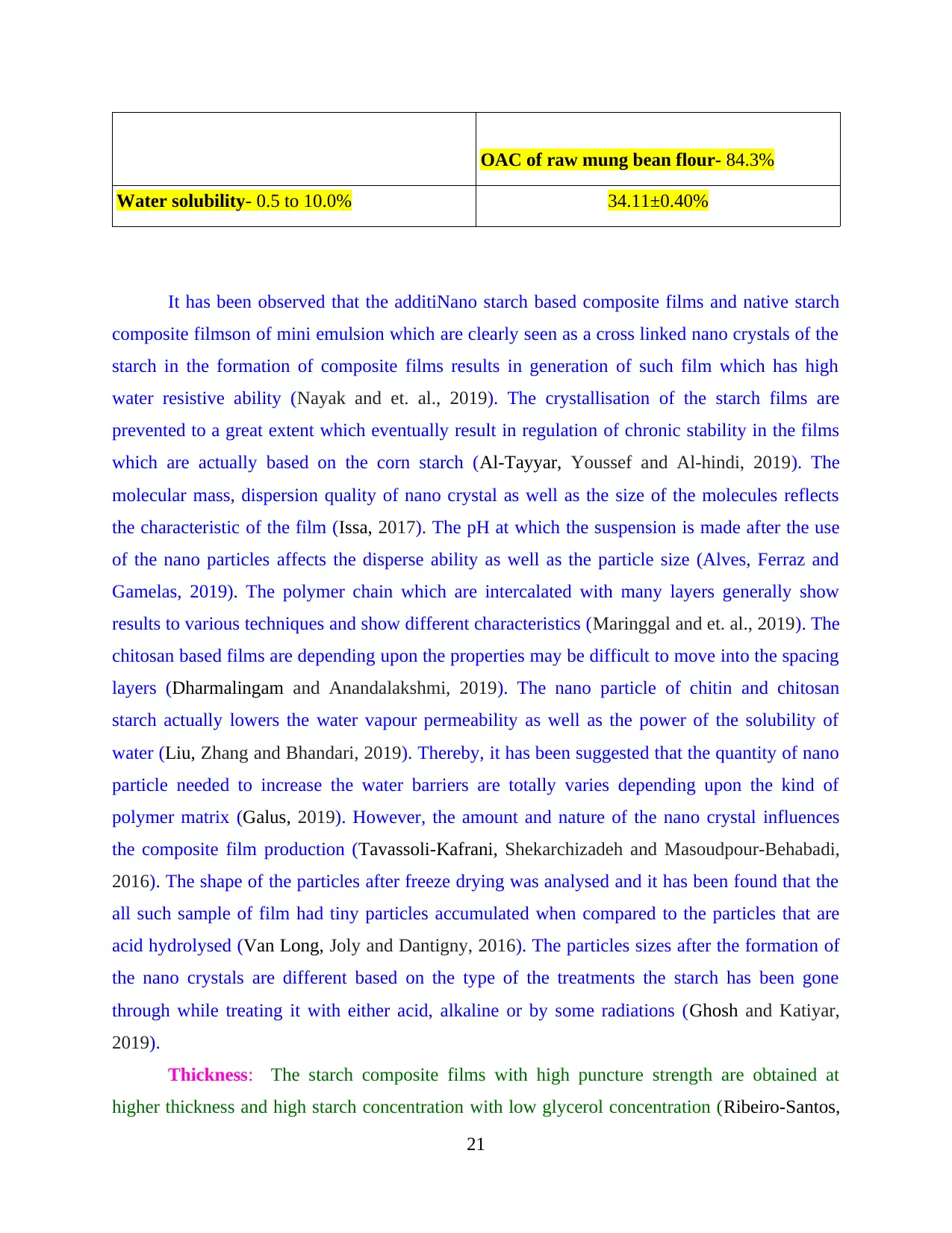
OAC of raw mung bean flour- 84.3%
Water solubility- 0.5 to 10.0% 34.11±0.40%
It has been observed that the additiNano starch based composite films and native starch
composite filmson of mini emulsion which are clearly seen as a cross linked nano crystals of the
starch in the formation of composite films results in generation of such film which has high
water resistive ability (Nayak and et. al., 2019). The crystallisation of the starch films are
prevented to a great extent which eventually result in regulation of chronic stability in the films
which are actually based on the corn starch (Al-Tayyar, Youssef and Al-hindi, 2019). The
molecular mass, dispersion quality of nano crystal as well as the size of the molecules reflects
the characteristic of the film (Issa, 2017). The pH at which the suspension is made after the use
of the nano particles affects the disperse ability as well as the particle size (Alves, Ferraz and
Gamelas, 2019). The polymer chain which are intercalated with many layers generally show
results to various techniques and show different characteristics (Maringgal and et. al., 2019). The
chitosan based films are depending upon the properties may be difficult to move into the spacing
layers (Dharmalingam and Anandalakshmi, 2019). The nano particle of chitin and chitosan
starch actually lowers the water vapour permeability as well as the power of the solubility of
water (Liu, Zhang and Bhandari, 2019). Thereby, it has been suggested that the quantity of nano
particle needed to increase the water barriers are totally varies depending upon the kind of
polymer matrix (Galus, 2019). However, the amount and nature of the nano crystal influences
the composite film production (Tavassoli-Kafrani, Shekarchizadeh and Masoudpour-Behabadi,
2016). The shape of the particles after freeze drying was analysed and it has been found that the
all such sample of film had tiny particles accumulated when compared to the particles that are
acid hydrolysed (Van Long, Joly and Dantigny, 2016). The particles sizes after the formation of
the nano crystals are different based on the type of the treatments the starch has been gone
through while treating it with either acid, alkaline or by some radiations (Ghosh and Katiyar,
2019).
Thickness: The starch composite films with high puncture strength are obtained at
higher thickness and high starch concentration with low glycerol concentration (Ribeiro-Santos,
21
Water solubility- 0.5 to 10.0% 34.11±0.40%
It has been observed that the additiNano starch based composite films and native starch
composite filmson of mini emulsion which are clearly seen as a cross linked nano crystals of the
starch in the formation of composite films results in generation of such film which has high
water resistive ability (Nayak and et. al., 2019). The crystallisation of the starch films are
prevented to a great extent which eventually result in regulation of chronic stability in the films
which are actually based on the corn starch (Al-Tayyar, Youssef and Al-hindi, 2019). The
molecular mass, dispersion quality of nano crystal as well as the size of the molecules reflects
the characteristic of the film (Issa, 2017). The pH at which the suspension is made after the use
of the nano particles affects the disperse ability as well as the particle size (Alves, Ferraz and
Gamelas, 2019). The polymer chain which are intercalated with many layers generally show
results to various techniques and show different characteristics (Maringgal and et. al., 2019). The
chitosan based films are depending upon the properties may be difficult to move into the spacing
layers (Dharmalingam and Anandalakshmi, 2019). The nano particle of chitin and chitosan
starch actually lowers the water vapour permeability as well as the power of the solubility of
water (Liu, Zhang and Bhandari, 2019). Thereby, it has been suggested that the quantity of nano
particle needed to increase the water barriers are totally varies depending upon the kind of
polymer matrix (Galus, 2019). However, the amount and nature of the nano crystal influences
the composite film production (Tavassoli-Kafrani, Shekarchizadeh and Masoudpour-Behabadi,
2016). The shape of the particles after freeze drying was analysed and it has been found that the
all such sample of film had tiny particles accumulated when compared to the particles that are
acid hydrolysed (Van Long, Joly and Dantigny, 2016). The particles sizes after the formation of
the nano crystals are different based on the type of the treatments the starch has been gone
through while treating it with either acid, alkaline or by some radiations (Ghosh and Katiyar,
2019).
Thickness: The starch composite films with high puncture strength are obtained at
higher thickness and high starch concentration with low glycerol concentration (Ribeiro-Santos,
21
Secure Best Marks with AI Grader
Need help grading? Try our AI Grader for instant feedback on your assignments.
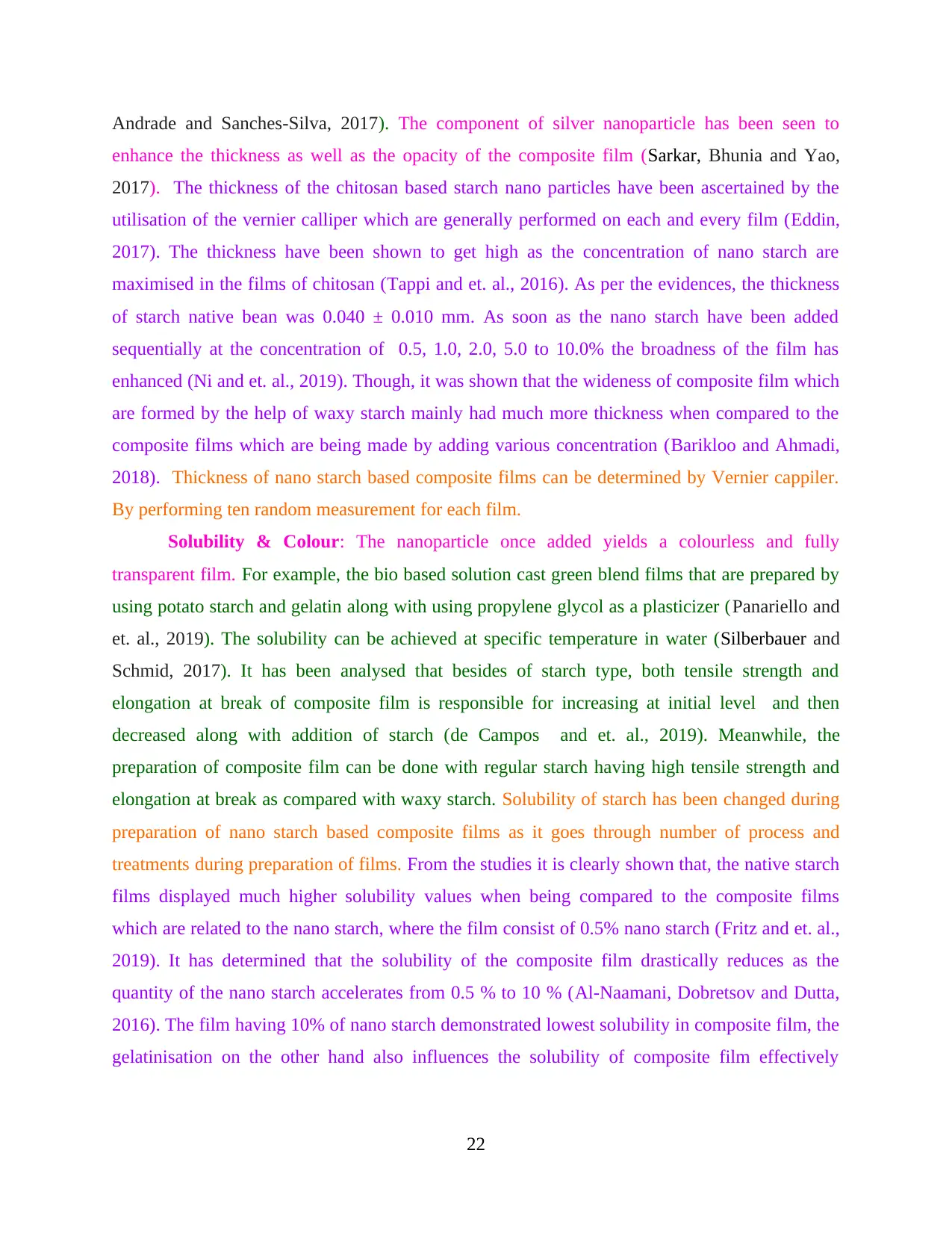
Andrade and Sanches-Silva, 2017). The component of silver nanoparticle has been seen to
enhance the thickness as well as the opacity of the composite film (Sarkar, Bhunia and Yao,
2017). The thickness of the chitosan based starch nano particles have been ascertained by the
utilisation of the vernier calliper which are generally performed on each and every film (Eddin,
2017). The thickness have been shown to get high as the concentration of nano starch are
maximised in the films of chitosan (Tappi and et. al., 2016). As per the evidences, the thickness
of starch native bean was 0.040 ± 0.010 mm. As soon as the nano starch have been added
sequentially at the concentration of 0.5, 1.0, 2.0, 5.0 to 10.0% the broadness of the film has
enhanced (Ni and et. al., 2019). Though, it was shown that the wideness of composite film which
are formed by the help of waxy starch mainly had much more thickness when compared to the
composite films which are being made by adding various concentration (Barikloo and Ahmadi,
2018). Thickness of nano starch based composite films can be determined by Vernier cappiler.
By performing ten random measurement for each film.
Solubility & Colour: The nanoparticle once added yields a colourless and fully
transparent film. For example, the bio based solution cast green blend films that are prepared by
using potato starch and gelatin along with using propylene glycol as a plasticizer (Panariello and
et. al., 2019). The solubility can be achieved at specific temperature in water (Silberbauer and
Schmid, 2017). It has been analysed that besides of starch type, both tensile strength and
elongation at break of composite film is responsible for increasing at initial level and then
decreased along with addition of starch (de Campos and et. al., 2019). Meanwhile, the
preparation of composite film can be done with regular starch having high tensile strength and
elongation at break as compared with waxy starch. Solubility of starch has been changed during
preparation of nano starch based composite films as it goes through number of process and
treatments during preparation of films. From the studies it is clearly shown that, the native starch
films displayed much higher solubility values when being compared to the composite films
which are related to the nano starch, where the film consist of 0.5% nano starch (Fritz and et. al.,
2019). It has determined that the solubility of the composite film drastically reduces as the
quantity of the nano starch accelerates from 0.5 % to 10 % (Al-Naamani, Dobretsov and Dutta,
2016). The film having 10% of nano starch demonstrated lowest solubility in composite film, the
gelatinisation on the other hand also influences the solubility of composite film effectively
22
enhance the thickness as well as the opacity of the composite film (Sarkar, Bhunia and Yao,
2017). The thickness of the chitosan based starch nano particles have been ascertained by the
utilisation of the vernier calliper which are generally performed on each and every film (Eddin,
2017). The thickness have been shown to get high as the concentration of nano starch are
maximised in the films of chitosan (Tappi and et. al., 2016). As per the evidences, the thickness
of starch native bean was 0.040 ± 0.010 mm. As soon as the nano starch have been added
sequentially at the concentration of 0.5, 1.0, 2.0, 5.0 to 10.0% the broadness of the film has
enhanced (Ni and et. al., 2019). Though, it was shown that the wideness of composite film which
are formed by the help of waxy starch mainly had much more thickness when compared to the
composite films which are being made by adding various concentration (Barikloo and Ahmadi,
2018). Thickness of nano starch based composite films can be determined by Vernier cappiler.
By performing ten random measurement for each film.
Solubility & Colour: The nanoparticle once added yields a colourless and fully
transparent film. For example, the bio based solution cast green blend films that are prepared by
using potato starch and gelatin along with using propylene glycol as a plasticizer (Panariello and
et. al., 2019). The solubility can be achieved at specific temperature in water (Silberbauer and
Schmid, 2017). It has been analysed that besides of starch type, both tensile strength and
elongation at break of composite film is responsible for increasing at initial level and then
decreased along with addition of starch (de Campos and et. al., 2019). Meanwhile, the
preparation of composite film can be done with regular starch having high tensile strength and
elongation at break as compared with waxy starch. Solubility of starch has been changed during
preparation of nano starch based composite films as it goes through number of process and
treatments during preparation of films. From the studies it is clearly shown that, the native starch
films displayed much higher solubility values when being compared to the composite films
which are related to the nano starch, where the film consist of 0.5% nano starch (Fritz and et. al.,
2019). It has determined that the solubility of the composite film drastically reduces as the
quantity of the nano starch accelerates from 0.5 % to 10 % (Al-Naamani, Dobretsov and Dutta,
2016). The film having 10% of nano starch demonstrated lowest solubility in composite film, the
gelatinisation on the other hand also influences the solubility of composite film effectively
22
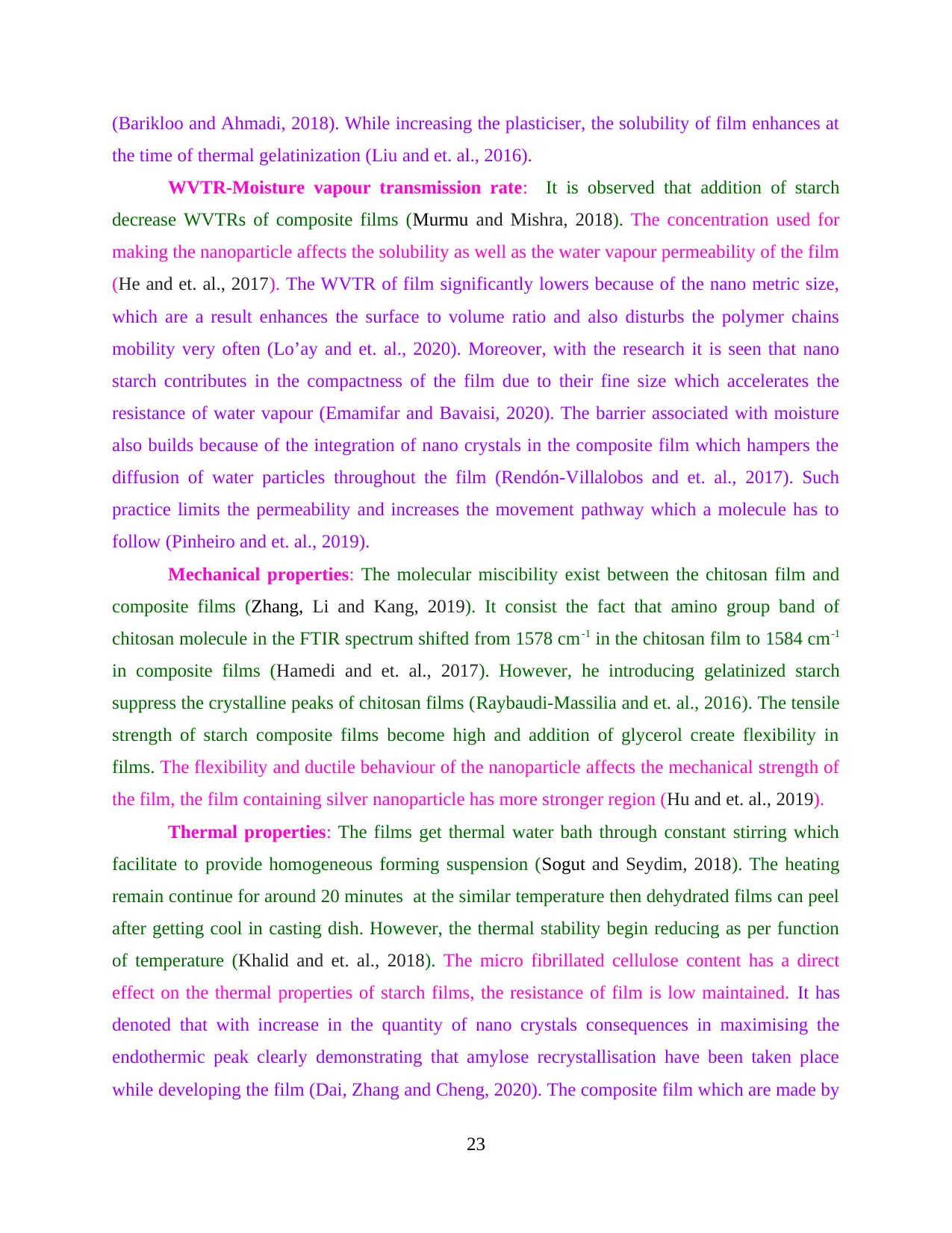
(Barikloo and Ahmadi, 2018). While increasing the plasticiser, the solubility of film enhances at
the time of thermal gelatinization (Liu and et. al., 2016).
WVTR-Moisture vapour transmission rate: It is observed that addition of starch
decrease WVTRs of composite films (Murmu and Mishra, 2018). The concentration used for
making the nanoparticle affects the solubility as well as the water vapour permeability of the film
(He and et. al., 2017). The WVTR of film significantly lowers because of the nano metric size,
which are a result enhances the surface to volume ratio and also disturbs the polymer chains
mobility very often (Lo’ay and et. al., 2020). Moreover, with the research it is seen that nano
starch contributes in the compactness of the film due to their fine size which accelerates the
resistance of water vapour (Emamifar and Bavaisi, 2020). The barrier associated with moisture
also builds because of the integration of nano crystals in the composite film which hampers the
diffusion of water particles throughout the film (Rendón-Villalobos and et. al., 2017). Such
practice limits the permeability and increases the movement pathway which a molecule has to
follow (Pinheiro and et. al., 2019).
Mechanical properties: The molecular miscibility exist between the chitosan film and
composite films (Zhang, Li and Kang, 2019). It consist the fact that amino group band of
chitosan molecule in the FTIR spectrum shifted from 1578 cm-1 in the chitosan film to 1584 cm-1
in composite films (Hamedi and et. al., 2017). However, he introducing gelatinized starch
suppress the crystalline peaks of chitosan films (Raybaudi-Massilia and et. al., 2016). The tensile
strength of starch composite films become high and addition of glycerol create flexibility in
films. The flexibility and ductile behaviour of the nanoparticle affects the mechanical strength of
the film, the film containing silver nanoparticle has more stronger region (Hu and et. al., 2019).
Thermal properties: The films get thermal water bath through constant stirring which
facilitate to provide homogeneous forming suspension (Sogut and Seydim, 2018). The heating
remain continue for around 20 minutes at the similar temperature then dehydrated films can peel
after getting cool in casting dish. However, the thermal stability begin reducing as per function
of temperature (Khalid and et. al., 2018). The micro fibrillated cellulose content has a direct
effect on the thermal properties of starch films, the resistance of film is low maintained. It has
denoted that with increase in the quantity of nano crystals consequences in maximising the
endothermic peak clearly demonstrating that amylose recrystallisation have been taken place
while developing the film (Dai, Zhang and Cheng, 2020). The composite film which are made by
23
the time of thermal gelatinization (Liu and et. al., 2016).
WVTR-Moisture vapour transmission rate: It is observed that addition of starch
decrease WVTRs of composite films (Murmu and Mishra, 2018). The concentration used for
making the nanoparticle affects the solubility as well as the water vapour permeability of the film
(He and et. al., 2017). The WVTR of film significantly lowers because of the nano metric size,
which are a result enhances the surface to volume ratio and also disturbs the polymer chains
mobility very often (Lo’ay and et. al., 2020). Moreover, with the research it is seen that nano
starch contributes in the compactness of the film due to their fine size which accelerates the
resistance of water vapour (Emamifar and Bavaisi, 2020). The barrier associated with moisture
also builds because of the integration of nano crystals in the composite film which hampers the
diffusion of water particles throughout the film (Rendón-Villalobos and et. al., 2017). Such
practice limits the permeability and increases the movement pathway which a molecule has to
follow (Pinheiro and et. al., 2019).
Mechanical properties: The molecular miscibility exist between the chitosan film and
composite films (Zhang, Li and Kang, 2019). It consist the fact that amino group band of
chitosan molecule in the FTIR spectrum shifted from 1578 cm-1 in the chitosan film to 1584 cm-1
in composite films (Hamedi and et. al., 2017). However, he introducing gelatinized starch
suppress the crystalline peaks of chitosan films (Raybaudi-Massilia and et. al., 2016). The tensile
strength of starch composite films become high and addition of glycerol create flexibility in
films. The flexibility and ductile behaviour of the nanoparticle affects the mechanical strength of
the film, the film containing silver nanoparticle has more stronger region (Hu and et. al., 2019).
Thermal properties: The films get thermal water bath through constant stirring which
facilitate to provide homogeneous forming suspension (Sogut and Seydim, 2018). The heating
remain continue for around 20 minutes at the similar temperature then dehydrated films can peel
after getting cool in casting dish. However, the thermal stability begin reducing as per function
of temperature (Khalid and et. al., 2018). The micro fibrillated cellulose content has a direct
effect on the thermal properties of starch films, the resistance of film is low maintained. It has
denoted that with increase in the quantity of nano crystals consequences in maximising the
endothermic peak clearly demonstrating that amylose recrystallisation have been taken place
while developing the film (Dai, Zhang and Cheng, 2020). The composite film which are made by
23
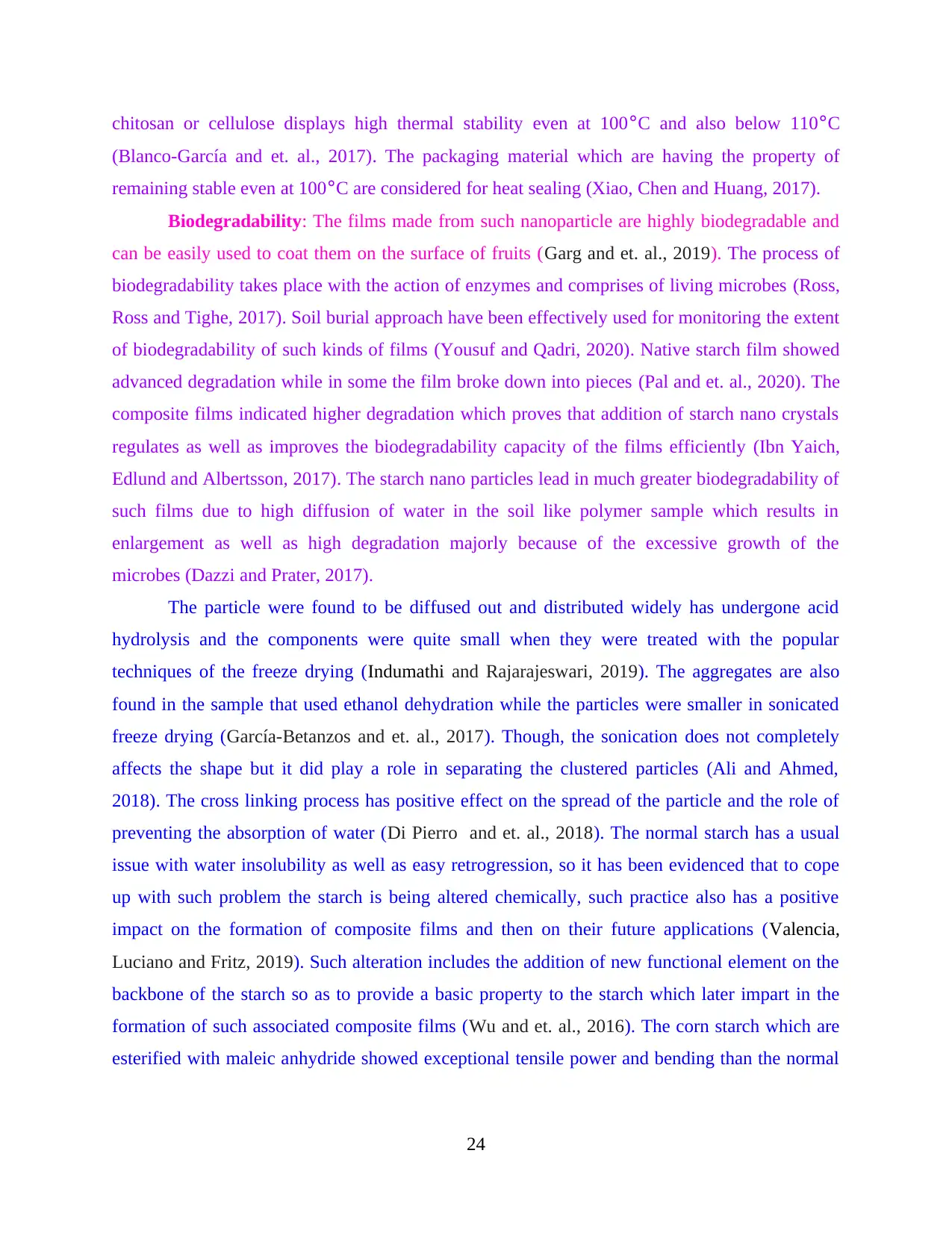
chitosan or cellulose displays high thermal stability even at 100°C and also below 110°C
(Blanco-García and et. al., 2017). The packaging material which are having the property of
remaining stable even at 100°C are considered for heat sealing (Xiao, Chen and Huang, 2017).
Biodegradability: The films made from such nanoparticle are highly biodegradable and
can be easily used to coat them on the surface of fruits (Garg and et. al., 2019). The process of
biodegradability takes place with the action of enzymes and comprises of living microbes (Ross,
Ross and Tighe, 2017). Soil burial approach have been effectively used for monitoring the extent
of biodegradability of such kinds of films (Yousuf and Qadri, 2020). Native starch film showed
advanced degradation while in some the film broke down into pieces (Pal and et. al., 2020). The
composite films indicated higher degradation which proves that addition of starch nano crystals
regulates as well as improves the biodegradability capacity of the films efficiently (Ibn Yaich,
Edlund and Albertsson, 2017). The starch nano particles lead in much greater biodegradability of
such films due to high diffusion of water in the soil like polymer sample which results in
enlargement as well as high degradation majorly because of the excessive growth of the
microbes (Dazzi and Prater, 2017).
The particle were found to be diffused out and distributed widely has undergone acid
hydrolysis and the components were quite small when they were treated with the popular
techniques of the freeze drying (Indumathi and Rajarajeswari, 2019). The aggregates are also
found in the sample that used ethanol dehydration while the particles were smaller in sonicated
freeze drying (García-Betanzos and et. al., 2017). Though, the sonication does not completely
affects the shape but it did play a role in separating the clustered particles (Ali and Ahmed,
2018). The cross linking process has positive effect on the spread of the particle and the role of
preventing the absorption of water (Di Pierro and et. al., 2018). The normal starch has a usual
issue with water insolubility as well as easy retrogression, so it has been evidenced that to cope
up with such problem the starch is being altered chemically, such practice also has a positive
impact on the formation of composite films and then on their future applications (Valencia,
Luciano and Fritz, 2019). Such alteration includes the addition of new functional element on the
backbone of the starch so as to provide a basic property to the starch which later impart in the
formation of such associated composite films (Wu and et. al., 2016). The corn starch which are
esterified with maleic anhydride showed exceptional tensile power and bending than the normal
24
(Blanco-García and et. al., 2017). The packaging material which are having the property of
remaining stable even at 100°C are considered for heat sealing (Xiao, Chen and Huang, 2017).
Biodegradability: The films made from such nanoparticle are highly biodegradable and
can be easily used to coat them on the surface of fruits (Garg and et. al., 2019). The process of
biodegradability takes place with the action of enzymes and comprises of living microbes (Ross,
Ross and Tighe, 2017). Soil burial approach have been effectively used for monitoring the extent
of biodegradability of such kinds of films (Yousuf and Qadri, 2020). Native starch film showed
advanced degradation while in some the film broke down into pieces (Pal and et. al., 2020). The
composite films indicated higher degradation which proves that addition of starch nano crystals
regulates as well as improves the biodegradability capacity of the films efficiently (Ibn Yaich,
Edlund and Albertsson, 2017). The starch nano particles lead in much greater biodegradability of
such films due to high diffusion of water in the soil like polymer sample which results in
enlargement as well as high degradation majorly because of the excessive growth of the
microbes (Dazzi and Prater, 2017).
The particle were found to be diffused out and distributed widely has undergone acid
hydrolysis and the components were quite small when they were treated with the popular
techniques of the freeze drying (Indumathi and Rajarajeswari, 2019). The aggregates are also
found in the sample that used ethanol dehydration while the particles were smaller in sonicated
freeze drying (García-Betanzos and et. al., 2017). Though, the sonication does not completely
affects the shape but it did play a role in separating the clustered particles (Ali and Ahmed,
2018). The cross linking process has positive effect on the spread of the particle and the role of
preventing the absorption of water (Di Pierro and et. al., 2018). The normal starch has a usual
issue with water insolubility as well as easy retrogression, so it has been evidenced that to cope
up with such problem the starch is being altered chemically, such practice also has a positive
impact on the formation of composite films and then on their future applications (Valencia,
Luciano and Fritz, 2019). Such alteration includes the addition of new functional element on the
backbone of the starch so as to provide a basic property to the starch which later impart in the
formation of such associated composite films (Wu and et. al., 2016). The corn starch which are
esterified with maleic anhydride showed exceptional tensile power and bending than the normal
24
Paraphrase This Document
Need a fresh take? Get an instant paraphrase of this document with our AI Paraphraser
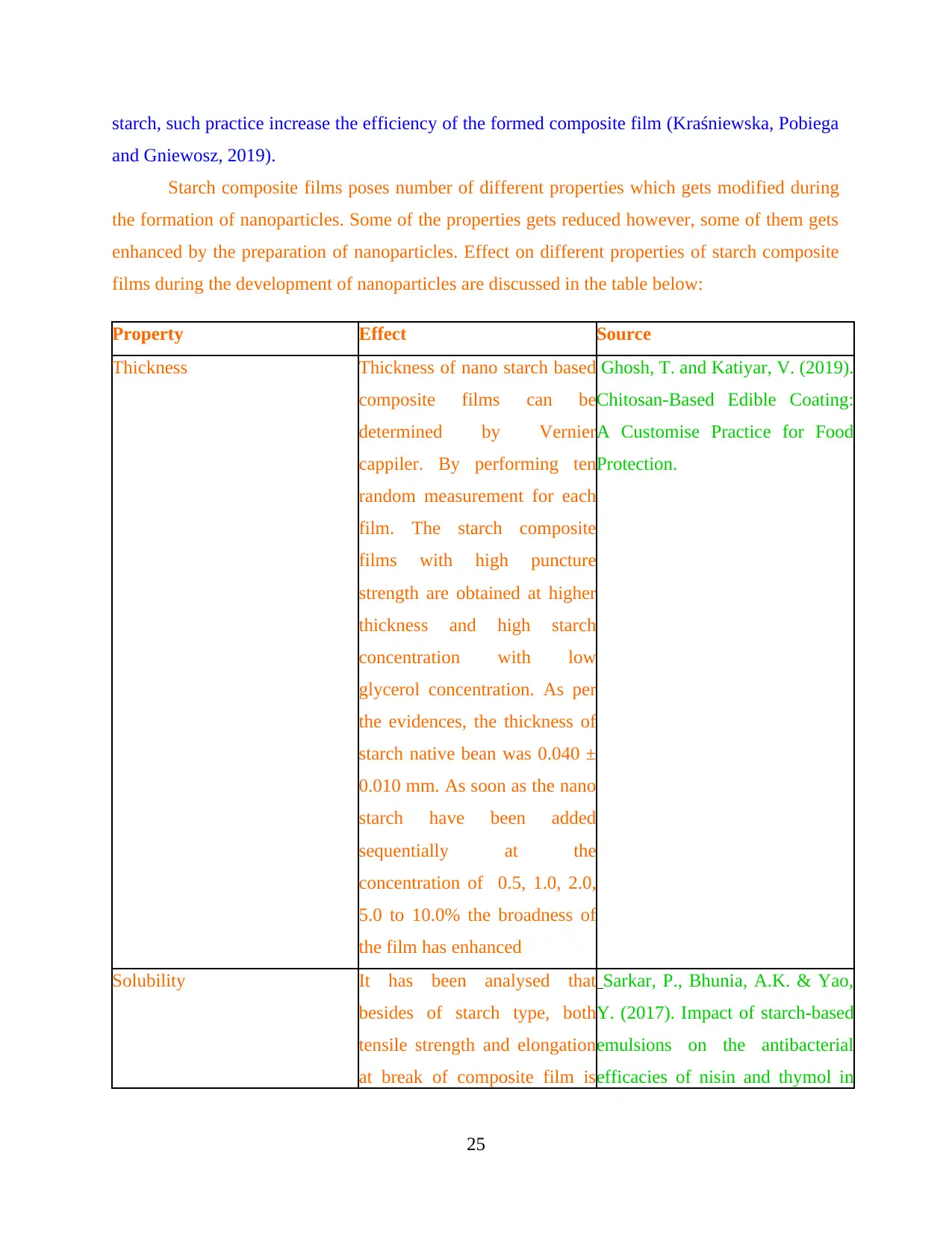
starch, such practice increase the efficiency of the formed composite film (Kraśniewska, Pobiega
and Gniewosz, 2019).
Starch composite films poses number of different properties which gets modified during
the formation of nanoparticles. Some of the properties gets reduced however, some of them gets
enhanced by the preparation of nanoparticles. Effect on different properties of starch composite
films during the development of nanoparticles are discussed in the table below:
Property Effect Source
Thickness Thickness of nano starch based
composite films can be
determined by Vernier
cappiler. By performing ten
random measurement for each
film. The starch composite
films with high puncture
strength are obtained at higher
thickness and high starch
concentration with low
glycerol concentration. As per
the evidences, the thickness of
starch native bean was 0.040 ±
0.010 mm. As soon as the nano
starch have been added
sequentially at the
concentration of 0.5, 1.0, 2.0,
5.0 to 10.0% the broadness of
the film has enhanced
Ghosh, T. and Katiyar, V. (2019).
Chitosan-Based Edible Coating:
A Customise Practice for Food
Protection.
Solubility It has been analysed that
besides of starch type, both
tensile strength and elongation
at break of composite film is
Sarkar, P., Bhunia, A.K. & Yao,
Y. (2017). Impact of starch-based
emulsions on the antibacterial
efficacies of nisin and thymol in
25
and Gniewosz, 2019).
Starch composite films poses number of different properties which gets modified during
the formation of nanoparticles. Some of the properties gets reduced however, some of them gets
enhanced by the preparation of nanoparticles. Effect on different properties of starch composite
films during the development of nanoparticles are discussed in the table below:
Property Effect Source
Thickness Thickness of nano starch based
composite films can be
determined by Vernier
cappiler. By performing ten
random measurement for each
film. The starch composite
films with high puncture
strength are obtained at higher
thickness and high starch
concentration with low
glycerol concentration. As per
the evidences, the thickness of
starch native bean was 0.040 ±
0.010 mm. As soon as the nano
starch have been added
sequentially at the
concentration of 0.5, 1.0, 2.0,
5.0 to 10.0% the broadness of
the film has enhanced
Ghosh, T. and Katiyar, V. (2019).
Chitosan-Based Edible Coating:
A Customise Practice for Food
Protection.
Solubility It has been analysed that
besides of starch type, both
tensile strength and elongation
at break of composite film is
Sarkar, P., Bhunia, A.K. & Yao,
Y. (2017). Impact of starch-based
emulsions on the antibacterial
efficacies of nisin and thymol in
25
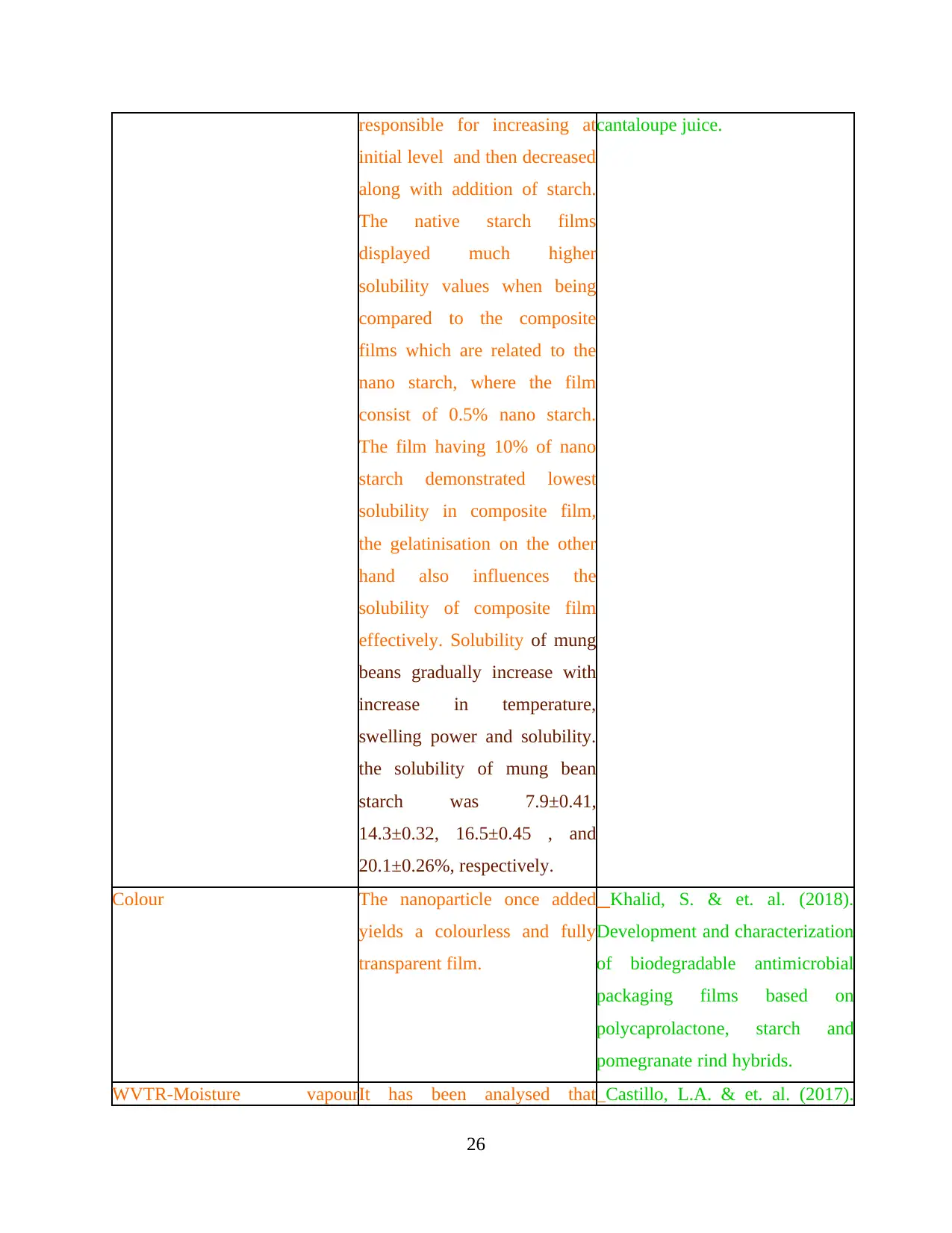
responsible for increasing at
initial level and then decreased
along with addition of starch.
The native starch films
displayed much higher
solubility values when being
compared to the composite
films which are related to the
nano starch, where the film
consist of 0.5% nano starch.
The film having 10% of nano
starch demonstrated lowest
solubility in composite film,
the gelatinisation on the other
hand also influences the
solubility of composite film
effectively. Solubility of mung
beans gradually increase with
increase in temperature,
swelling power and solubility.
the solubility of mung bean
starch was 7.9±0.41,
14.3±0.32, 16.5±0.45 , and
20.1±0.26%, respectively.
cantaloupe juice.
Colour The nanoparticle once added
yields a colourless and fully
transparent film.
Khalid, S. & et. al. (2018).
Development and characterization
of biodegradable antimicrobial
packaging films based on
polycaprolactone, starch and
pomegranate rind hybrids.
WVTR-Moisture vapourIt has been analysed that Castillo, L.A. & et. al. (2017).
26
initial level and then decreased
along with addition of starch.
The native starch films
displayed much higher
solubility values when being
compared to the composite
films which are related to the
nano starch, where the film
consist of 0.5% nano starch.
The film having 10% of nano
starch demonstrated lowest
solubility in composite film,
the gelatinisation on the other
hand also influences the
solubility of composite film
effectively. Solubility of mung
beans gradually increase with
increase in temperature,
swelling power and solubility.
the solubility of mung bean
starch was 7.9±0.41,
14.3±0.32, 16.5±0.45 , and
20.1±0.26%, respectively.
cantaloupe juice.
Colour The nanoparticle once added
yields a colourless and fully
transparent film.
Khalid, S. & et. al. (2018).
Development and characterization
of biodegradable antimicrobial
packaging films based on
polycaprolactone, starch and
pomegranate rind hybrids.
WVTR-Moisture vapourIt has been analysed that Castillo, L.A. & et. al. (2017).
26
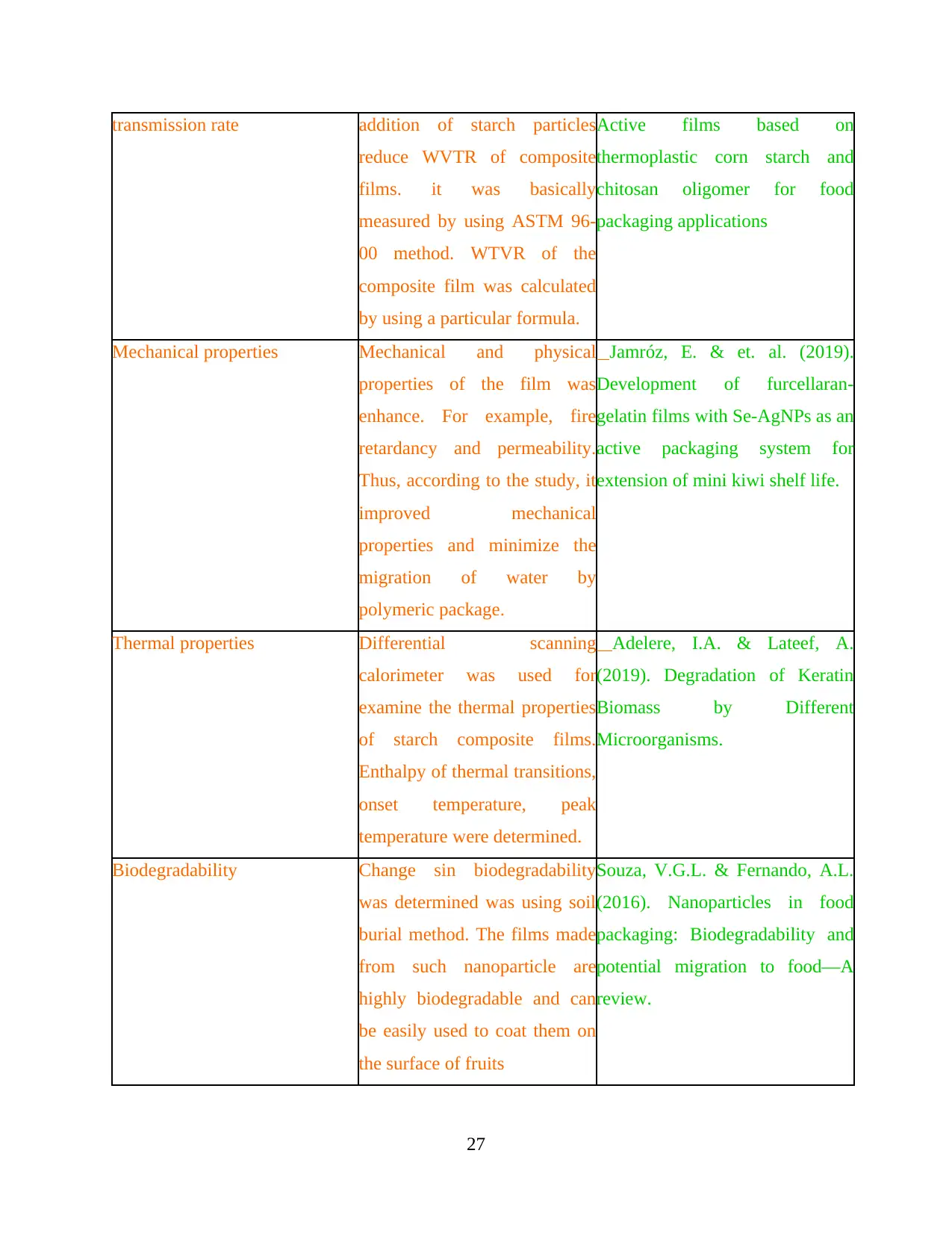
transmission rate addition of starch particles
reduce WVTR of composite
films. it was basically
measured by using ASTM 96-
00 method. WTVR of the
composite film was calculated
by using a particular formula.
Active films based on
thermoplastic corn starch and
chitosan oligomer for food
packaging applications
Mechanical properties Mechanical and physical
properties of the film was
enhance. For example, fire
retardancy and permeability.
Thus, according to the study, it
improved mechanical
properties and minimize the
migration of water by
polymeric package.
Jamróz, E. & et. al. (2019).
Development of furcellaran-
gelatin films with Se-AgNPs as an
active packaging system for
extension of mini kiwi shelf life.
Thermal properties Differential scanning
calorimeter was used for
examine the thermal properties
of starch composite films.
Enthalpy of thermal transitions,
onset temperature, peak
temperature were determined.
Adelere, I.A. & Lateef, A.
(2019). Degradation of Keratin
Biomass by Different
Microorganisms.
Biodegradability Change sin biodegradability
was determined was using soil
burial method. The films made
from such nanoparticle are
highly biodegradable and can
be easily used to coat them on
the surface of fruits
Souza, V.G.L. & Fernando, A.L.
(2016). Nanoparticles in food
packaging: Biodegradability and
potential migration to food—A
review.
27
reduce WVTR of composite
films. it was basically
measured by using ASTM 96-
00 method. WTVR of the
composite film was calculated
by using a particular formula.
Active films based on
thermoplastic corn starch and
chitosan oligomer for food
packaging applications
Mechanical properties Mechanical and physical
properties of the film was
enhance. For example, fire
retardancy and permeability.
Thus, according to the study, it
improved mechanical
properties and minimize the
migration of water by
polymeric package.
Jamróz, E. & et. al. (2019).
Development of furcellaran-
gelatin films with Se-AgNPs as an
active packaging system for
extension of mini kiwi shelf life.
Thermal properties Differential scanning
calorimeter was used for
examine the thermal properties
of starch composite films.
Enthalpy of thermal transitions,
onset temperature, peak
temperature were determined.
Adelere, I.A. & Lateef, A.
(2019). Degradation of Keratin
Biomass by Different
Microorganisms.
Biodegradability Change sin biodegradability
was determined was using soil
burial method. The films made
from such nanoparticle are
highly biodegradable and can
be easily used to coat them on
the surface of fruits
Souza, V.G.L. & Fernando, A.L.
(2016). Nanoparticles in food
packaging: Biodegradability and
potential migration to food—A
review.
27
Secure Best Marks with AI Grader
Need help grading? Try our AI Grader for instant feedback on your assignments.
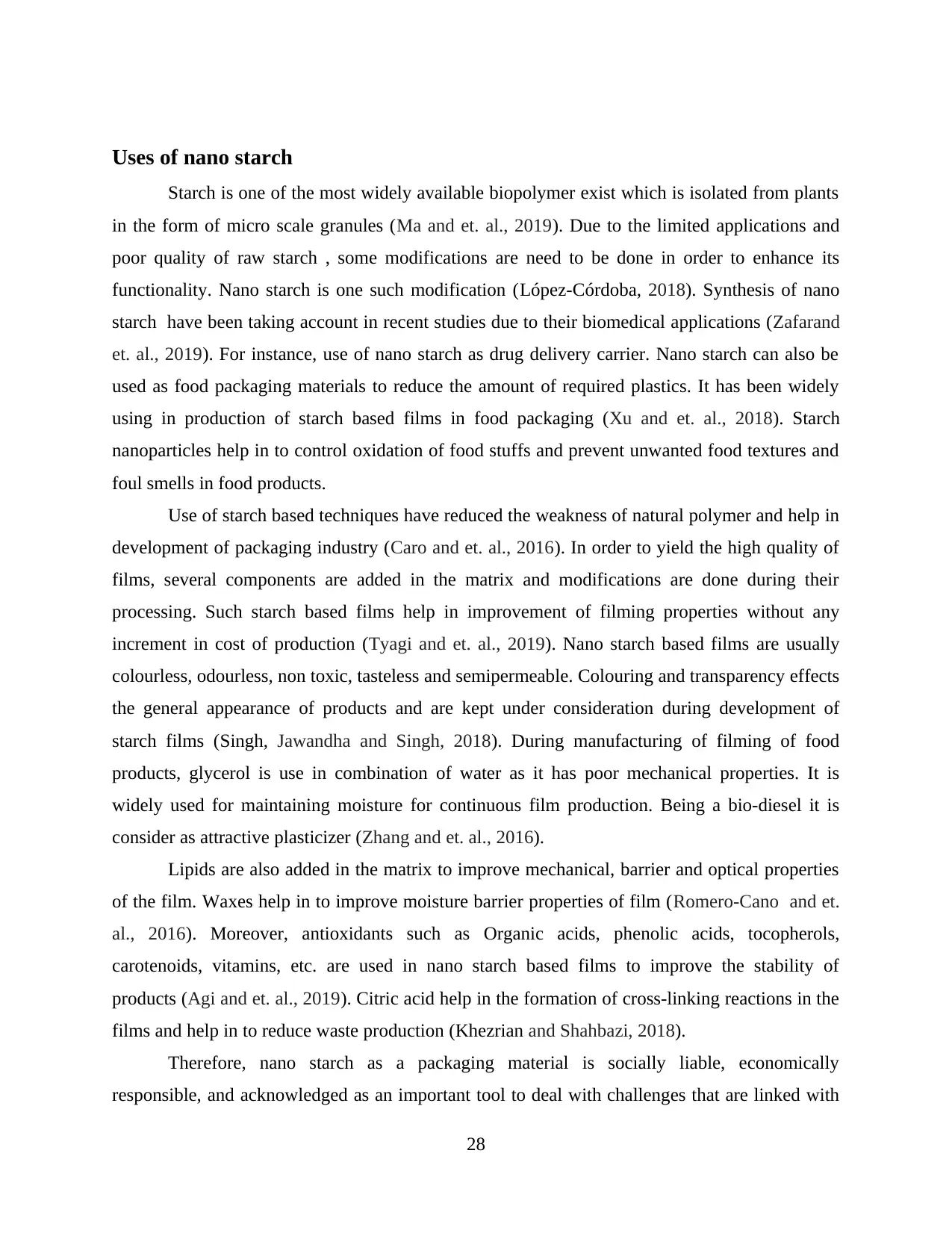
Uses of nano starch
Starch is one of the most widely available biopolymer exist which is isolated from plants
in the form of micro scale granules (Ma and et. al., 2019). Due to the limited applications and
poor quality of raw starch , some modifications are need to be done in order to enhance its
functionality. Nano starch is one such modification (López-Córdoba, 2018). Synthesis of nano
starch have been taking account in recent studies due to their biomedical applications (Zafarand
et. al., 2019). For instance, use of nano starch as drug delivery carrier. Nano starch can also be
used as food packaging materials to reduce the amount of required plastics. It has been widely
using in production of starch based films in food packaging (Xu and et. al., 2018). Starch
nanoparticles help in to control oxidation of food stuffs and prevent unwanted food textures and
foul smells in food products.
Use of starch based techniques have reduced the weakness of natural polymer and help in
development of packaging industry (Caro and et. al., 2016). In order to yield the high quality of
films, several components are added in the matrix and modifications are done during their
processing. Such starch based films help in improvement of filming properties without any
increment in cost of production (Tyagi and et. al., 2019). Nano starch based films are usually
colourless, odourless, non toxic, tasteless and semipermeable. Colouring and transparency effects
the general appearance of products and are kept under consideration during development of
starch films (Singh, Jawandha and Singh, 2018). During manufacturing of filming of food
products, glycerol is use in combination of water as it has poor mechanical properties. It is
widely used for maintaining moisture for continuous film production. Being a bio-diesel it is
consider as attractive plasticizer (Zhang and et. al., 2016).
Lipids are also added in the matrix to improve mechanical, barrier and optical properties
of the film. Waxes help in to improve moisture barrier properties of film (Romero-Cano and et.
al., 2016). Moreover, antioxidants such as Organic acids, phenolic acids, tocopherols,
carotenoids, vitamins, etc. are used in nano starch based films to improve the stability of
products (Agi and et. al., 2019). Citric acid help in the formation of cross-linking reactions in the
films and help in to reduce waste production (Khezrian and Shahbazi, 2018).
Therefore, nano starch as a packaging material is socially liable, economically
responsible, and acknowledged as an important tool to deal with challenges that are linked with
28
Starch is one of the most widely available biopolymer exist which is isolated from plants
in the form of micro scale granules (Ma and et. al., 2019). Due to the limited applications and
poor quality of raw starch , some modifications are need to be done in order to enhance its
functionality. Nano starch is one such modification (López-Córdoba, 2018). Synthesis of nano
starch have been taking account in recent studies due to their biomedical applications (Zafarand
et. al., 2019). For instance, use of nano starch as drug delivery carrier. Nano starch can also be
used as food packaging materials to reduce the amount of required plastics. It has been widely
using in production of starch based films in food packaging (Xu and et. al., 2018). Starch
nanoparticles help in to control oxidation of food stuffs and prevent unwanted food textures and
foul smells in food products.
Use of starch based techniques have reduced the weakness of natural polymer and help in
development of packaging industry (Caro and et. al., 2016). In order to yield the high quality of
films, several components are added in the matrix and modifications are done during their
processing. Such starch based films help in improvement of filming properties without any
increment in cost of production (Tyagi and et. al., 2019). Nano starch based films are usually
colourless, odourless, non toxic, tasteless and semipermeable. Colouring and transparency effects
the general appearance of products and are kept under consideration during development of
starch films (Singh, Jawandha and Singh, 2018). During manufacturing of filming of food
products, glycerol is use in combination of water as it has poor mechanical properties. It is
widely used for maintaining moisture for continuous film production. Being a bio-diesel it is
consider as attractive plasticizer (Zhang and et. al., 2016).
Lipids are also added in the matrix to improve mechanical, barrier and optical properties
of the film. Waxes help in to improve moisture barrier properties of film (Romero-Cano and et.
al., 2016). Moreover, antioxidants such as Organic acids, phenolic acids, tocopherols,
carotenoids, vitamins, etc. are used in nano starch based films to improve the stability of
products (Agi and et. al., 2019). Citric acid help in the formation of cross-linking reactions in the
films and help in to reduce waste production (Khezrian and Shahbazi, 2018).
Therefore, nano starch as a packaging material is socially liable, economically
responsible, and acknowledged as an important tool to deal with challenges that are linked with
28
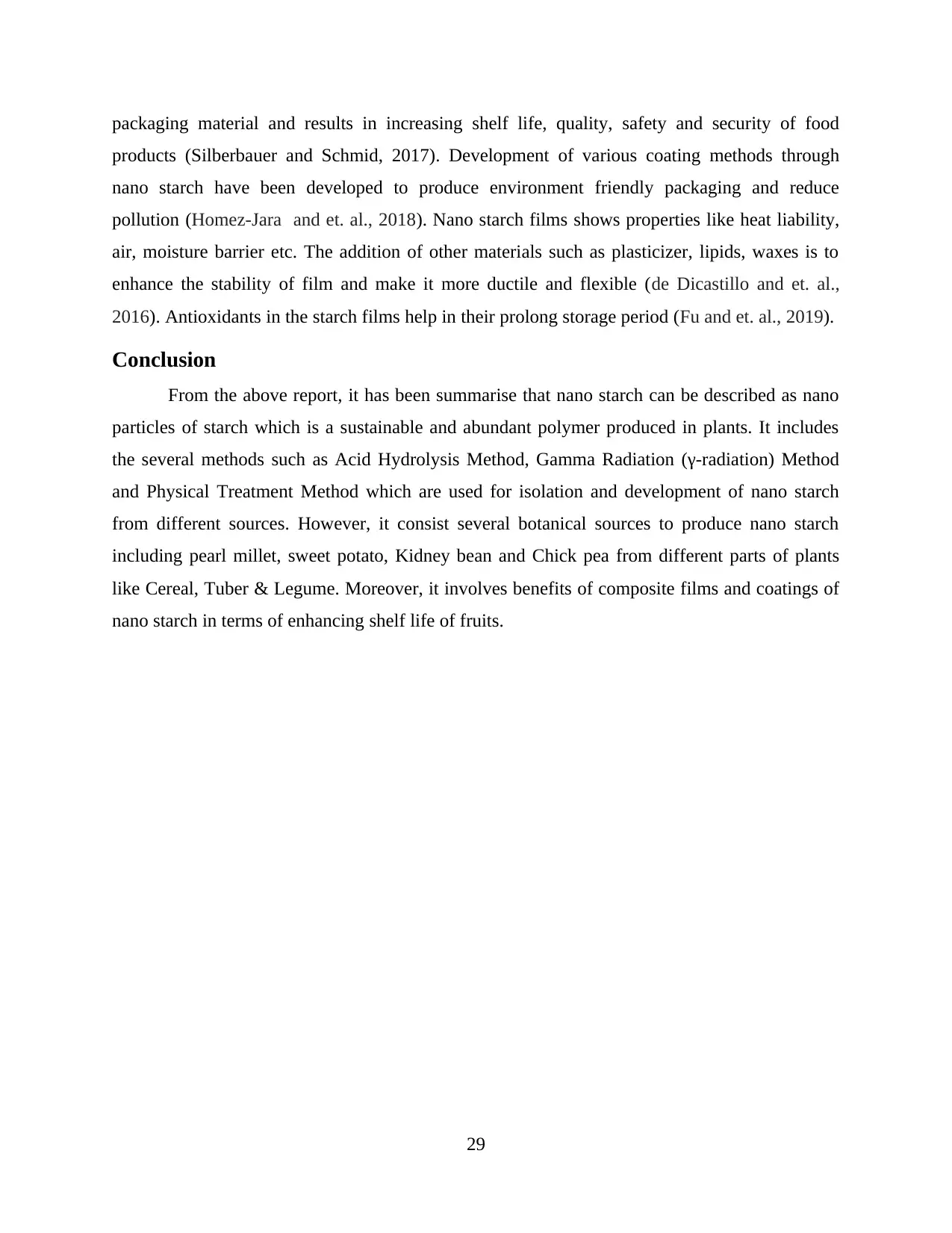
packaging material and results in increasing shelf life, quality, safety and security of food
products (Silberbauer and Schmid, 2017). Development of various coating methods through
nano starch have been developed to produce environment friendly packaging and reduce
pollution (Homez-Jara and et. al., 2018). Nano starch films shows properties like heat liability,
air, moisture barrier etc. The addition of other materials such as plasticizer, lipids, waxes is to
enhance the stability of film and make it more ductile and flexible (de Dicastillo and et. al.,
2016). Antioxidants in the starch films help in their prolong storage period (Fu and et. al., 2019).
Conclusion
From the above report, it has been summarise that nano starch can be described as nano
particles of starch which is a sustainable and abundant polymer produced in plants. It includes
the several methods such as Acid Hydrolysis Method, Gamma Radiation (γ-radiation) Method
and Physical Treatment Method which are used for isolation and development of nano starch
from different sources. However, it consist several botanical sources to produce nano starch
including pearl millet, sweet potato, Kidney bean and Chick pea from different parts of plants
like Cereal, Tuber & Legume. Moreover, it involves benefits of composite films and coatings of
nano starch in terms of enhancing shelf life of fruits.
29
products (Silberbauer and Schmid, 2017). Development of various coating methods through
nano starch have been developed to produce environment friendly packaging and reduce
pollution (Homez-Jara and et. al., 2018). Nano starch films shows properties like heat liability,
air, moisture barrier etc. The addition of other materials such as plasticizer, lipids, waxes is to
enhance the stability of film and make it more ductile and flexible (de Dicastillo and et. al.,
2016). Antioxidants in the starch films help in their prolong storage period (Fu and et. al., 2019).
Conclusion
From the above report, it has been summarise that nano starch can be described as nano
particles of starch which is a sustainable and abundant polymer produced in plants. It includes
the several methods such as Acid Hydrolysis Method, Gamma Radiation (γ-radiation) Method
and Physical Treatment Method which are used for isolation and development of nano starch
from different sources. However, it consist several botanical sources to produce nano starch
including pearl millet, sweet potato, Kidney bean and Chick pea from different parts of plants
like Cereal, Tuber & Legume. Moreover, it involves benefits of composite films and coatings of
nano starch in terms of enhancing shelf life of fruits.
29
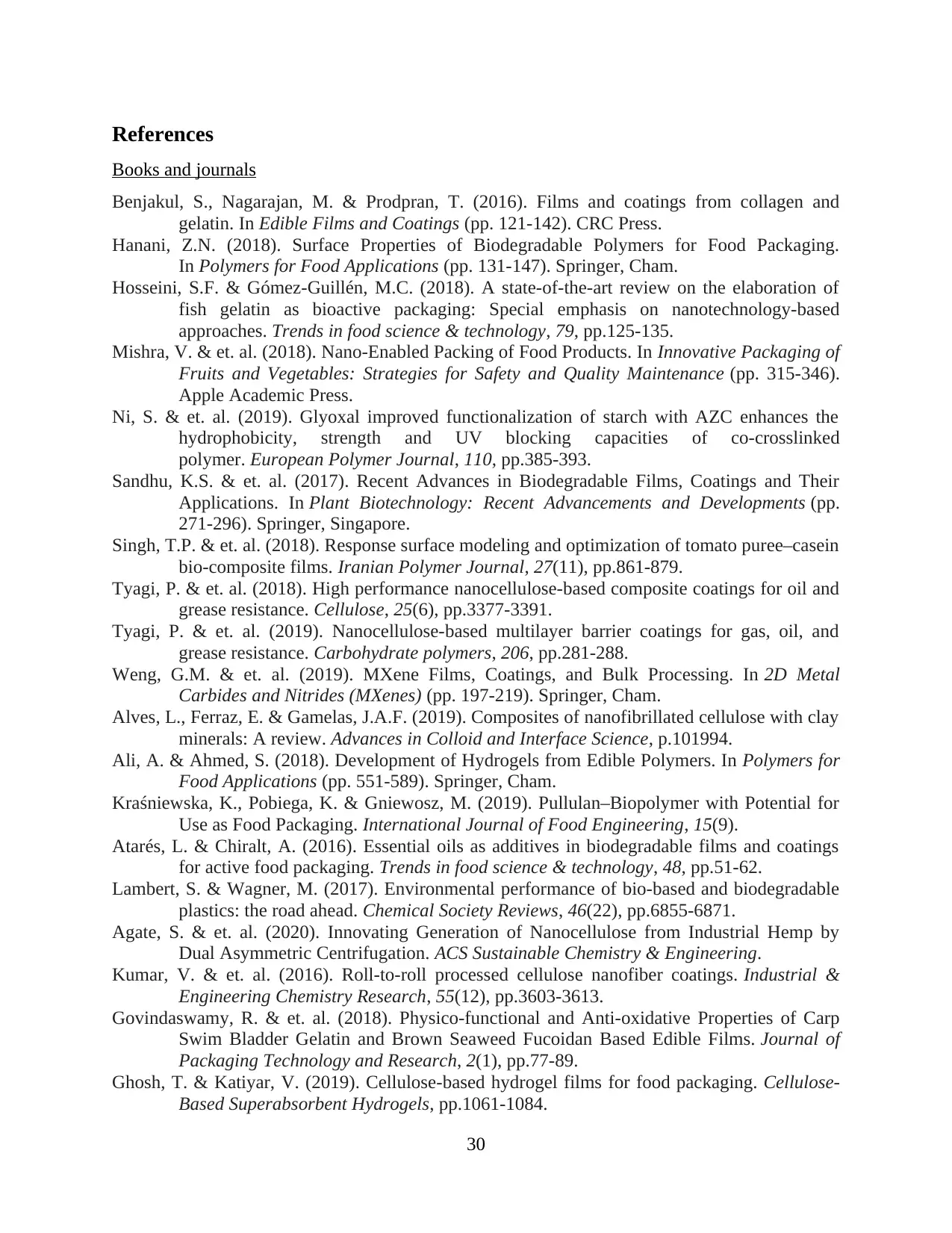
References
Books and journals
Benjakul, S., Nagarajan, M. & Prodpran, T. (2016). Films and coatings from collagen and
gelatin. In Edible Films and Coatings (pp. 121-142). CRC Press.
Hanani, Z.N. (2018). Surface Properties of Biodegradable Polymers for Food Packaging.
In Polymers for Food Applications (pp. 131-147). Springer, Cham.
Hosseini, S.F. & Gómez-Guillén, M.C. (2018). A state-of-the-art review on the elaboration of
fish gelatin as bioactive packaging: Special emphasis on nanotechnology-based
approaches. Trends in food science & technology, 79, pp.125-135.
Mishra, V. & et. al. (2018). Nano-Enabled Packing of Food Products. In Innovative Packaging of
Fruits and Vegetables: Strategies for Safety and Quality Maintenance (pp. 315-346).
Apple Academic Press.
Ni, S. & et. al. (2019). Glyoxal improved functionalization of starch with AZC enhances the
hydrophobicity, strength and UV blocking capacities of co-crosslinked
polymer. European Polymer Journal, 110, pp.385-393.
Sandhu, K.S. & et. al. (2017). Recent Advances in Biodegradable Films, Coatings and Their
Applications. In Plant Biotechnology: Recent Advancements and Developments (pp.
271-296). Springer, Singapore.
Singh, T.P. & et. al. (2018). Response surface modeling and optimization of tomato puree–casein
bio-composite films. Iranian Polymer Journal, 27(11), pp.861-879.
Tyagi, P. & et. al. (2018). High performance nanocellulose-based composite coatings for oil and
grease resistance. Cellulose, 25(6), pp.3377-3391.
Tyagi, P. & et. al. (2019). Nanocellulose-based multilayer barrier coatings for gas, oil, and
grease resistance. Carbohydrate polymers, 206, pp.281-288.
Weng, G.M. & et. al. (2019). MXene Films, Coatings, and Bulk Processing. In 2D Metal
Carbides and Nitrides (MXenes) (pp. 197-219). Springer, Cham.
Alves, L., Ferraz, E. & Gamelas, J.A.F. (2019). Composites of nanofibrillated cellulose with clay
minerals: A review. Advances in Colloid and Interface Science, p.101994.
Ali, A. & Ahmed, S. (2018). Development of Hydrogels from Edible Polymers. In Polymers for
Food Applications (pp. 551-589). Springer, Cham.
Kraśniewska, K., Pobiega, K. & Gniewosz, M. (2019). Pullulan–Biopolymer with Potential for
Use as Food Packaging. International Journal of Food Engineering, 15(9).
Atarés, L. & Chiralt, A. (2016). Essential oils as additives in biodegradable films and coatings
for active food packaging. Trends in food science & technology, 48, pp.51-62.
Lambert, S. & Wagner, M. (2017). Environmental performance of bio-based and biodegradable
plastics: the road ahead. Chemical Society Reviews, 46(22), pp.6855-6871.
Agate, S. & et. al. (2020). Innovating Generation of Nanocellulose from Industrial Hemp by
Dual Asymmetric Centrifugation. ACS Sustainable Chemistry & Engineering.
Kumar, V. & et. al. (2016). Roll-to-roll processed cellulose nanofiber coatings. Industrial &
Engineering Chemistry Research, 55(12), pp.3603-3613.
Govindaswamy, R. & et. al. (2018). Physico-functional and Anti-oxidative Properties of Carp
Swim Bladder Gelatin and Brown Seaweed Fucoidan Based Edible Films. Journal of
Packaging Technology and Research, 2(1), pp.77-89.
Ghosh, T. & Katiyar, V. (2019). Cellulose-based hydrogel films for food packaging. Cellulose-
Based Superabsorbent Hydrogels, pp.1061-1084.
30
Books and journals
Benjakul, S., Nagarajan, M. & Prodpran, T. (2016). Films and coatings from collagen and
gelatin. In Edible Films and Coatings (pp. 121-142). CRC Press.
Hanani, Z.N. (2018). Surface Properties of Biodegradable Polymers for Food Packaging.
In Polymers for Food Applications (pp. 131-147). Springer, Cham.
Hosseini, S.F. & Gómez-Guillén, M.C. (2018). A state-of-the-art review on the elaboration of
fish gelatin as bioactive packaging: Special emphasis on nanotechnology-based
approaches. Trends in food science & technology, 79, pp.125-135.
Mishra, V. & et. al. (2018). Nano-Enabled Packing of Food Products. In Innovative Packaging of
Fruits and Vegetables: Strategies for Safety and Quality Maintenance (pp. 315-346).
Apple Academic Press.
Ni, S. & et. al. (2019). Glyoxal improved functionalization of starch with AZC enhances the
hydrophobicity, strength and UV blocking capacities of co-crosslinked
polymer. European Polymer Journal, 110, pp.385-393.
Sandhu, K.S. & et. al. (2017). Recent Advances in Biodegradable Films, Coatings and Their
Applications. In Plant Biotechnology: Recent Advancements and Developments (pp.
271-296). Springer, Singapore.
Singh, T.P. & et. al. (2018). Response surface modeling and optimization of tomato puree–casein
bio-composite films. Iranian Polymer Journal, 27(11), pp.861-879.
Tyagi, P. & et. al. (2018). High performance nanocellulose-based composite coatings for oil and
grease resistance. Cellulose, 25(6), pp.3377-3391.
Tyagi, P. & et. al. (2019). Nanocellulose-based multilayer barrier coatings for gas, oil, and
grease resistance. Carbohydrate polymers, 206, pp.281-288.
Weng, G.M. & et. al. (2019). MXene Films, Coatings, and Bulk Processing. In 2D Metal
Carbides and Nitrides (MXenes) (pp. 197-219). Springer, Cham.
Alves, L., Ferraz, E. & Gamelas, J.A.F. (2019). Composites of nanofibrillated cellulose with clay
minerals: A review. Advances in Colloid and Interface Science, p.101994.
Ali, A. & Ahmed, S. (2018). Development of Hydrogels from Edible Polymers. In Polymers for
Food Applications (pp. 551-589). Springer, Cham.
Kraśniewska, K., Pobiega, K. & Gniewosz, M. (2019). Pullulan–Biopolymer with Potential for
Use as Food Packaging. International Journal of Food Engineering, 15(9).
Atarés, L. & Chiralt, A. (2016). Essential oils as additives in biodegradable films and coatings
for active food packaging. Trends in food science & technology, 48, pp.51-62.
Lambert, S. & Wagner, M. (2017). Environmental performance of bio-based and biodegradable
plastics: the road ahead. Chemical Society Reviews, 46(22), pp.6855-6871.
Agate, S. & et. al. (2020). Innovating Generation of Nanocellulose from Industrial Hemp by
Dual Asymmetric Centrifugation. ACS Sustainable Chemistry & Engineering.
Kumar, V. & et. al. (2016). Roll-to-roll processed cellulose nanofiber coatings. Industrial &
Engineering Chemistry Research, 55(12), pp.3603-3613.
Govindaswamy, R. & et. al. (2018). Physico-functional and Anti-oxidative Properties of Carp
Swim Bladder Gelatin and Brown Seaweed Fucoidan Based Edible Films. Journal of
Packaging Technology and Research, 2(1), pp.77-89.
Ghosh, T. & Katiyar, V. (2019). Cellulose-based hydrogel films for food packaging. Cellulose-
Based Superabsorbent Hydrogels, pp.1061-1084.
30
Paraphrase This Document
Need a fresh take? Get an instant paraphrase of this document with our AI Paraphraser
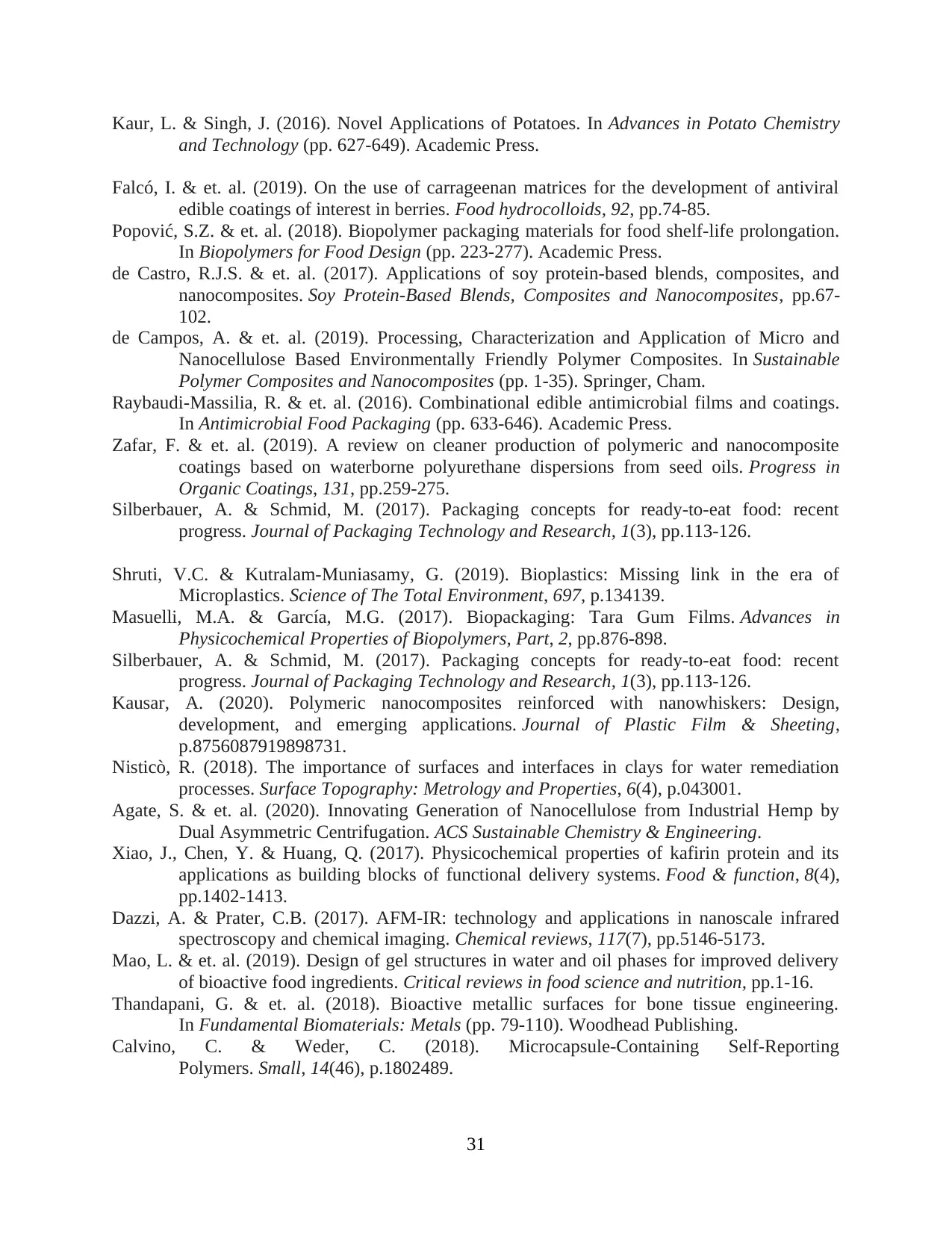
Kaur, L. & Singh, J. (2016). Novel Applications of Potatoes. In Advances in Potato Chemistry
and Technology (pp. 627-649). Academic Press.
Falcó, I. & et. al. (2019). On the use of carrageenan matrices for the development of antiviral
edible coatings of interest in berries. Food hydrocolloids, 92, pp.74-85.
Popović, S.Z. & et. al. (2018). Biopolymer packaging materials for food shelf-life prolongation.
In Biopolymers for Food Design (pp. 223-277). Academic Press.
de Castro, R.J.S. & et. al. (2017). Applications of soy protein-based blends, composites, and
nanocomposites. Soy Protein-Based Blends, Composites and Nanocomposites, pp.67-
102.
de Campos, A. & et. al. (2019). Processing, Characterization and Application of Micro and
Nanocellulose Based Environmentally Friendly Polymer Composites. In Sustainable
Polymer Composites and Nanocomposites (pp. 1-35). Springer, Cham.
Raybaudi-Massilia, R. & et. al. (2016). Combinational edible antimicrobial films and coatings.
In Antimicrobial Food Packaging (pp. 633-646). Academic Press.
Zafar, F. & et. al. (2019). A review on cleaner production of polymeric and nanocomposite
coatings based on waterborne polyurethane dispersions from seed oils. Progress in
Organic Coatings, 131, pp.259-275.
Silberbauer, A. & Schmid, M. (2017). Packaging concepts for ready-to-eat food: recent
progress. Journal of Packaging Technology and Research, 1(3), pp.113-126.
Shruti, V.C. & Kutralam-Muniasamy, G. (2019). Bioplastics: Missing link in the era of
Microplastics. Science of The Total Environment, 697, p.134139.
Masuelli, M.A. & García, M.G. (2017). Biopackaging: Tara Gum Films. Advances in
Physicochemical Properties of Biopolymers, Part, 2, pp.876-898.
Silberbauer, A. & Schmid, M. (2017). Packaging concepts for ready-to-eat food: recent
progress. Journal of Packaging Technology and Research, 1(3), pp.113-126.
Kausar, A. (2020). Polymeric nanocomposites reinforced with nanowhiskers: Design,
development, and emerging applications. Journal of Plastic Film & Sheeting,
p.8756087919898731.
Nisticò, R. (2018). The importance of surfaces and interfaces in clays for water remediation
processes. Surface Topography: Metrology and Properties, 6(4), p.043001.
Agate, S. & et. al. (2020). Innovating Generation of Nanocellulose from Industrial Hemp by
Dual Asymmetric Centrifugation. ACS Sustainable Chemistry & Engineering.
Xiao, J., Chen, Y. & Huang, Q. (2017). Physicochemical properties of kafirin protein and its
applications as building blocks of functional delivery systems. Food & function, 8(4),
pp.1402-1413.
Dazzi, A. & Prater, C.B. (2017). AFM-IR: technology and applications in nanoscale infrared
spectroscopy and chemical imaging. Chemical reviews, 117(7), pp.5146-5173.
Mao, L. & et. al. (2019). Design of gel structures in water and oil phases for improved delivery
of bioactive food ingredients. Critical reviews in food science and nutrition, pp.1-16.
Thandapani, G. & et. al. (2018). Bioactive metallic surfaces for bone tissue engineering.
In Fundamental Biomaterials: Metals (pp. 79-110). Woodhead Publishing.
Calvino, C. & Weder, C. (2018). Microcapsule‐Containing Self‐Reporting
Polymers. Small, 14(46), p.1802489.
31
and Technology (pp. 627-649). Academic Press.
Falcó, I. & et. al. (2019). On the use of carrageenan matrices for the development of antiviral
edible coatings of interest in berries. Food hydrocolloids, 92, pp.74-85.
Popović, S.Z. & et. al. (2018). Biopolymer packaging materials for food shelf-life prolongation.
In Biopolymers for Food Design (pp. 223-277). Academic Press.
de Castro, R.J.S. & et. al. (2017). Applications of soy protein-based blends, composites, and
nanocomposites. Soy Protein-Based Blends, Composites and Nanocomposites, pp.67-
102.
de Campos, A. & et. al. (2019). Processing, Characterization and Application of Micro and
Nanocellulose Based Environmentally Friendly Polymer Composites. In Sustainable
Polymer Composites and Nanocomposites (pp. 1-35). Springer, Cham.
Raybaudi-Massilia, R. & et. al. (2016). Combinational edible antimicrobial films and coatings.
In Antimicrobial Food Packaging (pp. 633-646). Academic Press.
Zafar, F. & et. al. (2019). A review on cleaner production of polymeric and nanocomposite
coatings based on waterborne polyurethane dispersions from seed oils. Progress in
Organic Coatings, 131, pp.259-275.
Silberbauer, A. & Schmid, M. (2017). Packaging concepts for ready-to-eat food: recent
progress. Journal of Packaging Technology and Research, 1(3), pp.113-126.
Shruti, V.C. & Kutralam-Muniasamy, G. (2019). Bioplastics: Missing link in the era of
Microplastics. Science of The Total Environment, 697, p.134139.
Masuelli, M.A. & García, M.G. (2017). Biopackaging: Tara Gum Films. Advances in
Physicochemical Properties of Biopolymers, Part, 2, pp.876-898.
Silberbauer, A. & Schmid, M. (2017). Packaging concepts for ready-to-eat food: recent
progress. Journal of Packaging Technology and Research, 1(3), pp.113-126.
Kausar, A. (2020). Polymeric nanocomposites reinforced with nanowhiskers: Design,
development, and emerging applications. Journal of Plastic Film & Sheeting,
p.8756087919898731.
Nisticò, R. (2018). The importance of surfaces and interfaces in clays for water remediation
processes. Surface Topography: Metrology and Properties, 6(4), p.043001.
Agate, S. & et. al. (2020). Innovating Generation of Nanocellulose from Industrial Hemp by
Dual Asymmetric Centrifugation. ACS Sustainable Chemistry & Engineering.
Xiao, J., Chen, Y. & Huang, Q. (2017). Physicochemical properties of kafirin protein and its
applications as building blocks of functional delivery systems. Food & function, 8(4),
pp.1402-1413.
Dazzi, A. & Prater, C.B. (2017). AFM-IR: technology and applications in nanoscale infrared
spectroscopy and chemical imaging. Chemical reviews, 117(7), pp.5146-5173.
Mao, L. & et. al. (2019). Design of gel structures in water and oil phases for improved delivery
of bioactive food ingredients. Critical reviews in food science and nutrition, pp.1-16.
Thandapani, G. & et. al. (2018). Bioactive metallic surfaces for bone tissue engineering.
In Fundamental Biomaterials: Metals (pp. 79-110). Woodhead Publishing.
Calvino, C. & Weder, C. (2018). Microcapsule‐Containing Self‐Reporting
Polymers. Small, 14(46), p.1802489.
31
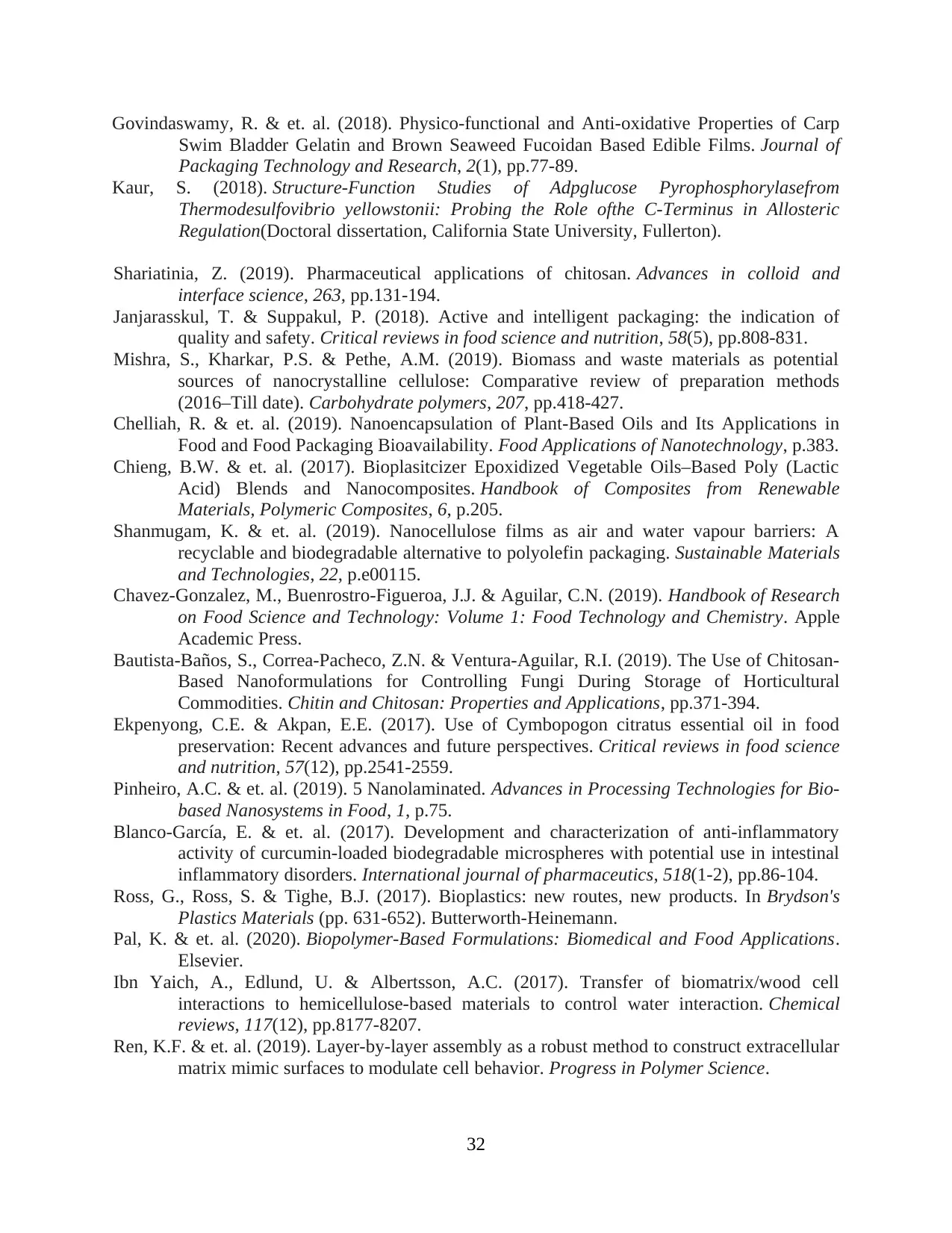
Govindaswamy, R. & et. al. (2018). Physico-functional and Anti-oxidative Properties of Carp
Swim Bladder Gelatin and Brown Seaweed Fucoidan Based Edible Films. Journal of
Packaging Technology and Research, 2(1), pp.77-89.
Kaur, S. (2018). Structure-Function Studies of Adpglucose Pyrophosphorylasefrom
Thermodesulfovibrio yellowstonii: Probing the Role ofthe C-Terminus in Allosteric
Regulation(Doctoral dissertation, California State University, Fullerton).
Shariatinia, Z. (2019). Pharmaceutical applications of chitosan. Advances in colloid and
interface science, 263, pp.131-194.
Janjarasskul, T. & Suppakul, P. (2018). Active and intelligent packaging: the indication of
quality and safety. Critical reviews in food science and nutrition, 58(5), pp.808-831.
Mishra, S., Kharkar, P.S. & Pethe, A.M. (2019). Biomass and waste materials as potential
sources of nanocrystalline cellulose: Comparative review of preparation methods
(2016–Till date). Carbohydrate polymers, 207, pp.418-427.
Chelliah, R. & et. al. (2019). Nanoencapsulation of Plant-Based Oils and Its Applications in
Food and Food Packaging Bioavailability. Food Applications of Nanotechnology, p.383.
Chieng, B.W. & et. al. (2017). Bioplasitcizer Epoxidized Vegetable Oils–Based Poly (Lactic
Acid) Blends and Nanocomposites. Handbook of Composites from Renewable
Materials, Polymeric Composites, 6, p.205.
Shanmugam, K. & et. al. (2019). Nanocellulose films as air and water vapour barriers: A
recyclable and biodegradable alternative to polyolefin packaging. Sustainable Materials
and Technologies, 22, p.e00115.
Chavez-Gonzalez, M., Buenrostro-Figueroa, J.J. & Aguilar, C.N. (2019). Handbook of Research
on Food Science and Technology: Volume 1: Food Technology and Chemistry. Apple
Academic Press.
Bautista‐Baños, S., Correa‐Pacheco, Z.N. & Ventura‐Aguilar, R.I. (2019). The Use of Chitosan‐
Based Nanoformulations for Controlling Fungi During Storage of Horticultural
Commodities. Chitin and Chitosan: Properties and Applications, pp.371-394.
Ekpenyong, C.E. & Akpan, E.E. (2017). Use of Cymbopogon citratus essential oil in food
preservation: Recent advances and future perspectives. Critical reviews in food science
and nutrition, 57(12), pp.2541-2559.
Pinheiro, A.C. & et. al. (2019). 5 Nanolaminated. Advances in Processing Technologies for Bio-
based Nanosystems in Food, 1, p.75.
Blanco-García, E. & et. al. (2017). Development and characterization of anti-inflammatory
activity of curcumin-loaded biodegradable microspheres with potential use in intestinal
inflammatory disorders. International journal of pharmaceutics, 518(1-2), pp.86-104.
Ross, G., Ross, S. & Tighe, B.J. (2017). Bioplastics: new routes, new products. In Brydson's
Plastics Materials (pp. 631-652). Butterworth-Heinemann.
Pal, K. & et. al. (2020). Biopolymer-Based Formulations: Biomedical and Food Applications.
Elsevier.
Ibn Yaich, A., Edlund, U. & Albertsson, A.C. (2017). Transfer of biomatrix/wood cell
interactions to hemicellulose-based materials to control water interaction. Chemical
reviews, 117(12), pp.8177-8207.
Ren, K.F. & et. al. (2019). Layer-by-layer assembly as a robust method to construct extracellular
matrix mimic surfaces to modulate cell behavior. Progress in Polymer Science.
32
Swim Bladder Gelatin and Brown Seaweed Fucoidan Based Edible Films. Journal of
Packaging Technology and Research, 2(1), pp.77-89.
Kaur, S. (2018). Structure-Function Studies of Adpglucose Pyrophosphorylasefrom
Thermodesulfovibrio yellowstonii: Probing the Role ofthe C-Terminus in Allosteric
Regulation(Doctoral dissertation, California State University, Fullerton).
Shariatinia, Z. (2019). Pharmaceutical applications of chitosan. Advances in colloid and
interface science, 263, pp.131-194.
Janjarasskul, T. & Suppakul, P. (2018). Active and intelligent packaging: the indication of
quality and safety. Critical reviews in food science and nutrition, 58(5), pp.808-831.
Mishra, S., Kharkar, P.S. & Pethe, A.M. (2019). Biomass and waste materials as potential
sources of nanocrystalline cellulose: Comparative review of preparation methods
(2016–Till date). Carbohydrate polymers, 207, pp.418-427.
Chelliah, R. & et. al. (2019). Nanoencapsulation of Plant-Based Oils and Its Applications in
Food and Food Packaging Bioavailability. Food Applications of Nanotechnology, p.383.
Chieng, B.W. & et. al. (2017). Bioplasitcizer Epoxidized Vegetable Oils–Based Poly (Lactic
Acid) Blends and Nanocomposites. Handbook of Composites from Renewable
Materials, Polymeric Composites, 6, p.205.
Shanmugam, K. & et. al. (2019). Nanocellulose films as air and water vapour barriers: A
recyclable and biodegradable alternative to polyolefin packaging. Sustainable Materials
and Technologies, 22, p.e00115.
Chavez-Gonzalez, M., Buenrostro-Figueroa, J.J. & Aguilar, C.N. (2019). Handbook of Research
on Food Science and Technology: Volume 1: Food Technology and Chemistry. Apple
Academic Press.
Bautista‐Baños, S., Correa‐Pacheco, Z.N. & Ventura‐Aguilar, R.I. (2019). The Use of Chitosan‐
Based Nanoformulations for Controlling Fungi During Storage of Horticultural
Commodities. Chitin and Chitosan: Properties and Applications, pp.371-394.
Ekpenyong, C.E. & Akpan, E.E. (2017). Use of Cymbopogon citratus essential oil in food
preservation: Recent advances and future perspectives. Critical reviews in food science
and nutrition, 57(12), pp.2541-2559.
Pinheiro, A.C. & et. al. (2019). 5 Nanolaminated. Advances in Processing Technologies for Bio-
based Nanosystems in Food, 1, p.75.
Blanco-García, E. & et. al. (2017). Development and characterization of anti-inflammatory
activity of curcumin-loaded biodegradable microspheres with potential use in intestinal
inflammatory disorders. International journal of pharmaceutics, 518(1-2), pp.86-104.
Ross, G., Ross, S. & Tighe, B.J. (2017). Bioplastics: new routes, new products. In Brydson's
Plastics Materials (pp. 631-652). Butterworth-Heinemann.
Pal, K. & et. al. (2020). Biopolymer-Based Formulations: Biomedical and Food Applications.
Elsevier.
Ibn Yaich, A., Edlund, U. & Albertsson, A.C. (2017). Transfer of biomatrix/wood cell
interactions to hemicellulose-based materials to control water interaction. Chemical
reviews, 117(12), pp.8177-8207.
Ren, K.F. & et. al. (2019). Layer-by-layer assembly as a robust method to construct extracellular
matrix mimic surfaces to modulate cell behavior. Progress in Polymer Science.
32
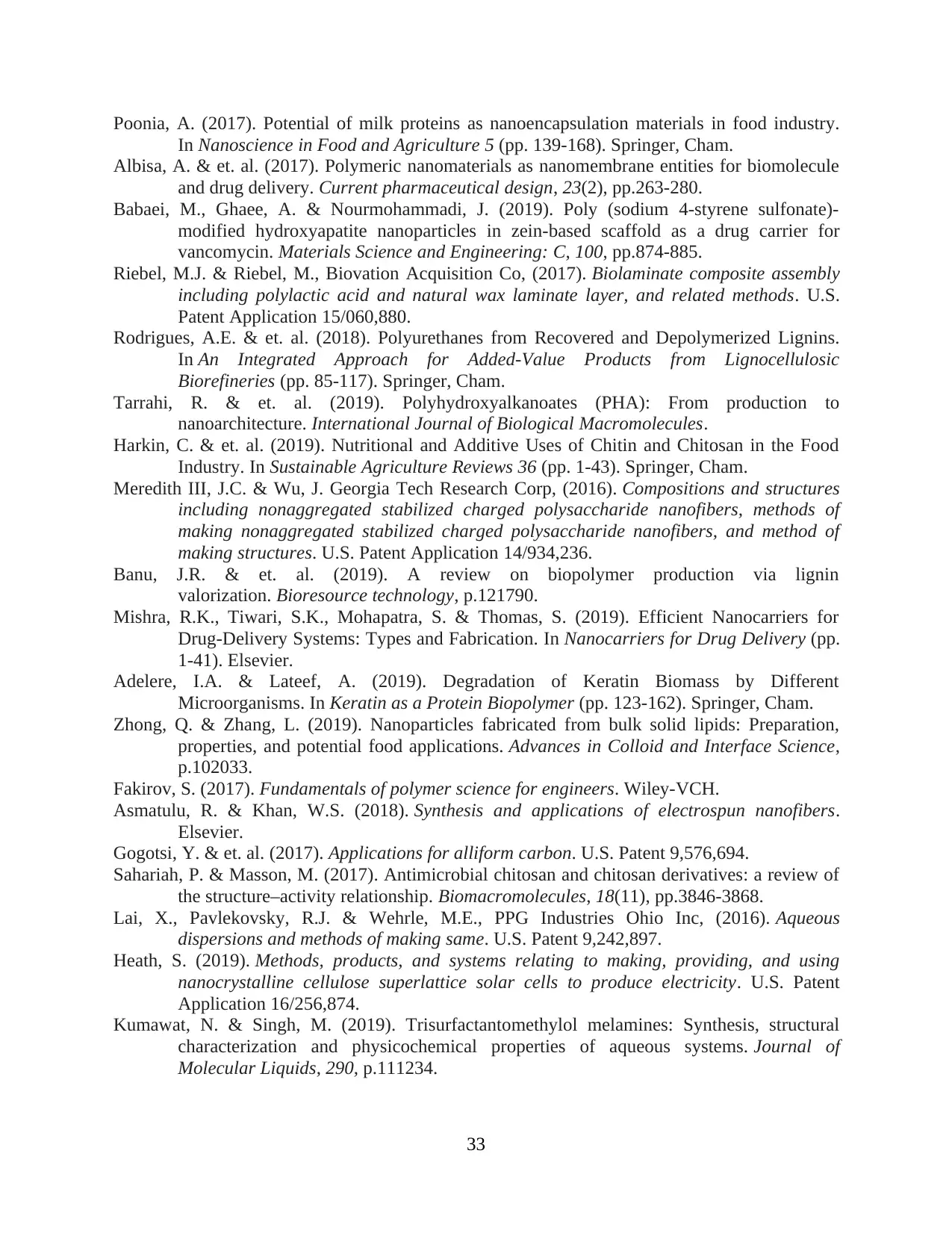
Poonia, A. (2017). Potential of milk proteins as nanoencapsulation materials in food industry.
In Nanoscience in Food and Agriculture 5 (pp. 139-168). Springer, Cham.
Albisa, A. & et. al. (2017). Polymeric nanomaterials as nanomembrane entities for biomolecule
and drug delivery. Current pharmaceutical design, 23(2), pp.263-280.
Babaei, M., Ghaee, A. & Nourmohammadi, J. (2019). Poly (sodium 4-styrene sulfonate)-
modified hydroxyapatite nanoparticles in zein-based scaffold as a drug carrier for
vancomycin. Materials Science and Engineering: C, 100, pp.874-885.
Riebel, M.J. & Riebel, M., Biovation Acquisition Co, (2017). Biolaminate composite assembly
including polylactic acid and natural wax laminate layer, and related methods. U.S.
Patent Application 15/060,880.
Rodrigues, A.E. & et. al. (2018). Polyurethanes from Recovered and Depolymerized Lignins.
In An Integrated Approach for Added-Value Products from Lignocellulosic
Biorefineries (pp. 85-117). Springer, Cham.
Tarrahi, R. & et. al. (2019). Polyhydroxyalkanoates (PHA): From production to
nanoarchitecture. International Journal of Biological Macromolecules.
Harkin, C. & et. al. (2019). Nutritional and Additive Uses of Chitin and Chitosan in the Food
Industry. In Sustainable Agriculture Reviews 36 (pp. 1-43). Springer, Cham.
Meredith III, J.C. & Wu, J. Georgia Tech Research Corp, (2016). Compositions and structures
including nonaggregated stabilized charged polysaccharide nanofibers, methods of
making nonaggregated stabilized charged polysaccharide nanofibers, and method of
making structures. U.S. Patent Application 14/934,236.
Banu, J.R. & et. al. (2019). A review on biopolymer production via lignin
valorization. Bioresource technology, p.121790.
Mishra, R.K., Tiwari, S.K., Mohapatra, S. & Thomas, S. (2019). Efficient Nanocarriers for
Drug-Delivery Systems: Types and Fabrication. In Nanocarriers for Drug Delivery (pp.
1-41). Elsevier.
Adelere, I.A. & Lateef, A. (2019). Degradation of Keratin Biomass by Different
Microorganisms. In Keratin as a Protein Biopolymer (pp. 123-162). Springer, Cham.
Zhong, Q. & Zhang, L. (2019). Nanoparticles fabricated from bulk solid lipids: Preparation,
properties, and potential food applications. Advances in Colloid and Interface Science,
p.102033.
Fakirov, S. (2017). Fundamentals of polymer science for engineers. Wiley-VCH.
Asmatulu, R. & Khan, W.S. (2018). Synthesis and applications of electrospun nanofibers.
Elsevier.
Gogotsi, Y. & et. al. (2017). Applications for alliform carbon. U.S. Patent 9,576,694.
Sahariah, P. & Masson, M. (2017). Antimicrobial chitosan and chitosan derivatives: a review of
the structure–activity relationship. Biomacromolecules, 18(11), pp.3846-3868.
Lai, X., Pavlekovsky, R.J. & Wehrle, M.E., PPG Industries Ohio Inc, (2016). Aqueous
dispersions and methods of making same. U.S. Patent 9,242,897.
Heath, S. (2019). Methods, products, and systems relating to making, providing, and using
nanocrystalline cellulose superlattice solar cells to produce electricity. U.S. Patent
Application 16/256,874.
Kumawat, N. & Singh, M. (2019). Trisurfactantomethylol melamines: Synthesis, structural
characterization and physicochemical properties of aqueous systems. Journal of
Molecular Liquids, 290, p.111234.
33
In Nanoscience in Food and Agriculture 5 (pp. 139-168). Springer, Cham.
Albisa, A. & et. al. (2017). Polymeric nanomaterials as nanomembrane entities for biomolecule
and drug delivery. Current pharmaceutical design, 23(2), pp.263-280.
Babaei, M., Ghaee, A. & Nourmohammadi, J. (2019). Poly (sodium 4-styrene sulfonate)-
modified hydroxyapatite nanoparticles in zein-based scaffold as a drug carrier for
vancomycin. Materials Science and Engineering: C, 100, pp.874-885.
Riebel, M.J. & Riebel, M., Biovation Acquisition Co, (2017). Biolaminate composite assembly
including polylactic acid and natural wax laminate layer, and related methods. U.S.
Patent Application 15/060,880.
Rodrigues, A.E. & et. al. (2018). Polyurethanes from Recovered and Depolymerized Lignins.
In An Integrated Approach for Added-Value Products from Lignocellulosic
Biorefineries (pp. 85-117). Springer, Cham.
Tarrahi, R. & et. al. (2019). Polyhydroxyalkanoates (PHA): From production to
nanoarchitecture. International Journal of Biological Macromolecules.
Harkin, C. & et. al. (2019). Nutritional and Additive Uses of Chitin and Chitosan in the Food
Industry. In Sustainable Agriculture Reviews 36 (pp. 1-43). Springer, Cham.
Meredith III, J.C. & Wu, J. Georgia Tech Research Corp, (2016). Compositions and structures
including nonaggregated stabilized charged polysaccharide nanofibers, methods of
making nonaggregated stabilized charged polysaccharide nanofibers, and method of
making structures. U.S. Patent Application 14/934,236.
Banu, J.R. & et. al. (2019). A review on biopolymer production via lignin
valorization. Bioresource technology, p.121790.
Mishra, R.K., Tiwari, S.K., Mohapatra, S. & Thomas, S. (2019). Efficient Nanocarriers for
Drug-Delivery Systems: Types and Fabrication. In Nanocarriers for Drug Delivery (pp.
1-41). Elsevier.
Adelere, I.A. & Lateef, A. (2019). Degradation of Keratin Biomass by Different
Microorganisms. In Keratin as a Protein Biopolymer (pp. 123-162). Springer, Cham.
Zhong, Q. & Zhang, L. (2019). Nanoparticles fabricated from bulk solid lipids: Preparation,
properties, and potential food applications. Advances in Colloid and Interface Science,
p.102033.
Fakirov, S. (2017). Fundamentals of polymer science for engineers. Wiley-VCH.
Asmatulu, R. & Khan, W.S. (2018). Synthesis and applications of electrospun nanofibers.
Elsevier.
Gogotsi, Y. & et. al. (2017). Applications for alliform carbon. U.S. Patent 9,576,694.
Sahariah, P. & Masson, M. (2017). Antimicrobial chitosan and chitosan derivatives: a review of
the structure–activity relationship. Biomacromolecules, 18(11), pp.3846-3868.
Lai, X., Pavlekovsky, R.J. & Wehrle, M.E., PPG Industries Ohio Inc, (2016). Aqueous
dispersions and methods of making same. U.S. Patent 9,242,897.
Heath, S. (2019). Methods, products, and systems relating to making, providing, and using
nanocrystalline cellulose superlattice solar cells to produce electricity. U.S. Patent
Application 16/256,874.
Kumawat, N. & Singh, M. (2019). Trisurfactantomethylol melamines: Synthesis, structural
characterization and physicochemical properties of aqueous systems. Journal of
Molecular Liquids, 290, p.111234.
33
Secure Best Marks with AI Grader
Need help grading? Try our AI Grader for instant feedback on your assignments.
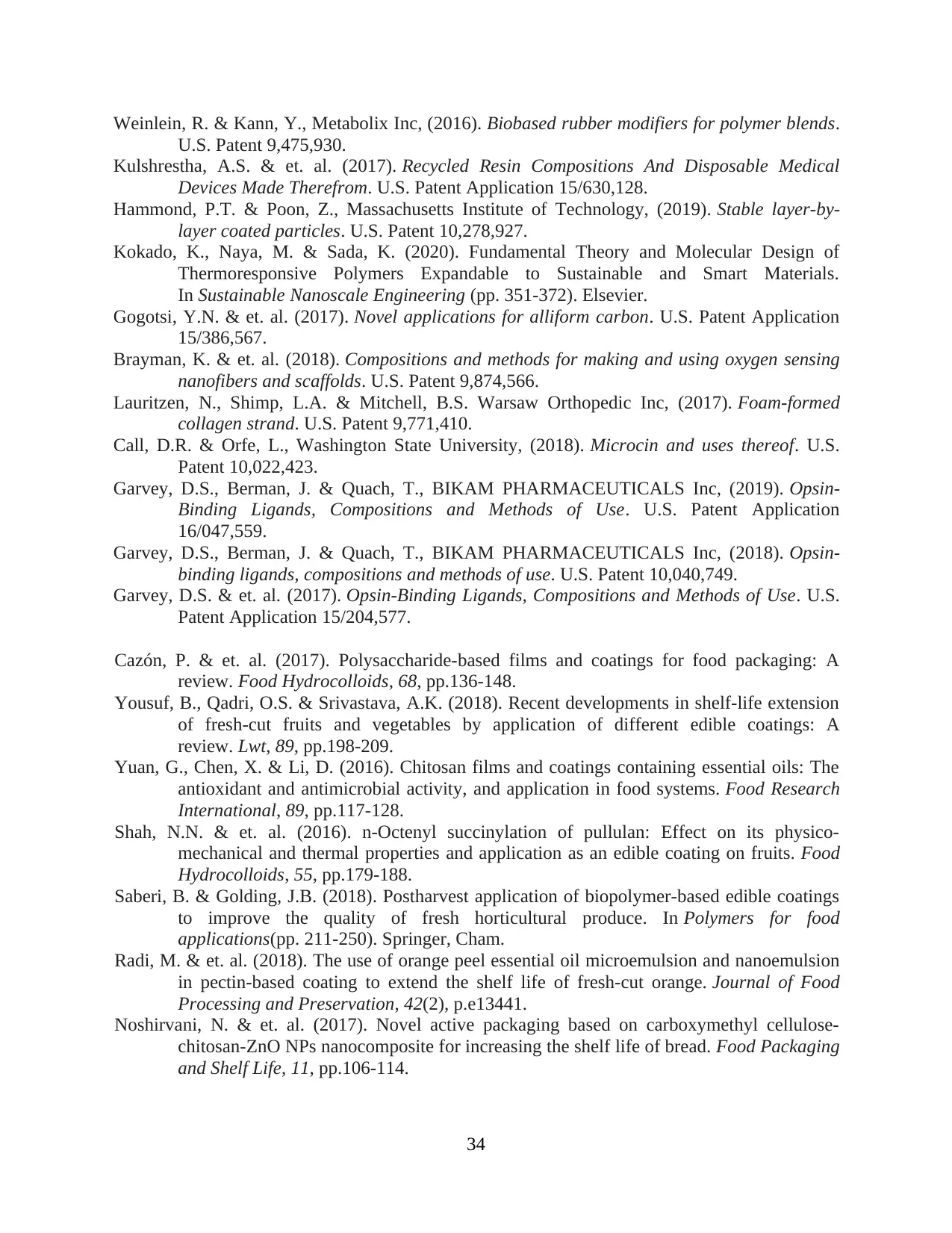
Weinlein, R. & Kann, Y., Metabolix Inc, (2016). Biobased rubber modifiers for polymer blends.
U.S. Patent 9,475,930.
Kulshrestha, A.S. & et. al. (2017). Recycled Resin Compositions And Disposable Medical
Devices Made Therefrom. U.S. Patent Application 15/630,128.
Hammond, P.T. & Poon, Z., Massachusetts Institute of Technology, (2019). Stable layer-by-
layer coated particles. U.S. Patent 10,278,927.
Kokado, K., Naya, M. & Sada, K. (2020). Fundamental Theory and Molecular Design of
Thermoresponsive Polymers Expandable to Sustainable and Smart Materials.
In Sustainable Nanoscale Engineering (pp. 351-372). Elsevier.
Gogotsi, Y.N. & et. al. (2017). Novel applications for alliform carbon. U.S. Patent Application
15/386,567.
Brayman, K. & et. al. (2018). Compositions and methods for making and using oxygen sensing
nanofibers and scaffolds. U.S. Patent 9,874,566.
Lauritzen, N., Shimp, L.A. & Mitchell, B.S. Warsaw Orthopedic Inc, (2017). Foam-formed
collagen strand. U.S. Patent 9,771,410.
Call, D.R. & Orfe, L., Washington State University, (2018). Microcin and uses thereof. U.S.
Patent 10,022,423.
Garvey, D.S., Berman, J. & Quach, T., BIKAM PHARMACEUTICALS Inc, (2019). Opsin-
Binding Ligands, Compositions and Methods of Use. U.S. Patent Application
16/047,559.
Garvey, D.S., Berman, J. & Quach, T., BIKAM PHARMACEUTICALS Inc, (2018). Opsin-
binding ligands, compositions and methods of use. U.S. Patent 10,040,749.
Garvey, D.S. & et. al. (2017). Opsin-Binding Ligands, Compositions and Methods of Use. U.S.
Patent Application 15/204,577.
Cazón, P. & et. al. (2017). Polysaccharide-based films and coatings for food packaging: A
review. Food Hydrocolloids, 68, pp.136-148.
Yousuf, B., Qadri, O.S. & Srivastava, A.K. (2018). Recent developments in shelf-life extension
of fresh-cut fruits and vegetables by application of different edible coatings: A
review. Lwt, 89, pp.198-209.
Yuan, G., Chen, X. & Li, D. (2016). Chitosan films and coatings containing essential oils: The
antioxidant and antimicrobial activity, and application in food systems. Food Research
International, 89, pp.117-128.
Shah, N.N. & et. al. (2016). n-Octenyl succinylation of pullulan: Effect on its physico-
mechanical and thermal properties and application as an edible coating on fruits. Food
Hydrocolloids, 55, pp.179-188.
Saberi, B. & Golding, J.B. (2018). Postharvest application of biopolymer-based edible coatings
to improve the quality of fresh horticultural produce. In Polymers for food
applications(pp. 211-250). Springer, Cham.
Radi, M. & et. al. (2018). The use of orange peel essential oil microemulsion and nanoemulsion
in pectin‐based coating to extend the shelf life of fresh‐cut orange. Journal of Food
Processing and Preservation, 42(2), p.e13441.
Noshirvani, N. & et. al. (2017). Novel active packaging based on carboxymethyl cellulose-
chitosan-ZnO NPs nanocomposite for increasing the shelf life of bread. Food Packaging
and Shelf Life, 11, pp.106-114.
34
U.S. Patent 9,475,930.
Kulshrestha, A.S. & et. al. (2017). Recycled Resin Compositions And Disposable Medical
Devices Made Therefrom. U.S. Patent Application 15/630,128.
Hammond, P.T. & Poon, Z., Massachusetts Institute of Technology, (2019). Stable layer-by-
layer coated particles. U.S. Patent 10,278,927.
Kokado, K., Naya, M. & Sada, K. (2020). Fundamental Theory and Molecular Design of
Thermoresponsive Polymers Expandable to Sustainable and Smart Materials.
In Sustainable Nanoscale Engineering (pp. 351-372). Elsevier.
Gogotsi, Y.N. & et. al. (2017). Novel applications for alliform carbon. U.S. Patent Application
15/386,567.
Brayman, K. & et. al. (2018). Compositions and methods for making and using oxygen sensing
nanofibers and scaffolds. U.S. Patent 9,874,566.
Lauritzen, N., Shimp, L.A. & Mitchell, B.S. Warsaw Orthopedic Inc, (2017). Foam-formed
collagen strand. U.S. Patent 9,771,410.
Call, D.R. & Orfe, L., Washington State University, (2018). Microcin and uses thereof. U.S.
Patent 10,022,423.
Garvey, D.S., Berman, J. & Quach, T., BIKAM PHARMACEUTICALS Inc, (2019). Opsin-
Binding Ligands, Compositions and Methods of Use. U.S. Patent Application
16/047,559.
Garvey, D.S., Berman, J. & Quach, T., BIKAM PHARMACEUTICALS Inc, (2018). Opsin-
binding ligands, compositions and methods of use. U.S. Patent 10,040,749.
Garvey, D.S. & et. al. (2017). Opsin-Binding Ligands, Compositions and Methods of Use. U.S.
Patent Application 15/204,577.
Cazón, P. & et. al. (2017). Polysaccharide-based films and coatings for food packaging: A
review. Food Hydrocolloids, 68, pp.136-148.
Yousuf, B., Qadri, O.S. & Srivastava, A.K. (2018). Recent developments in shelf-life extension
of fresh-cut fruits and vegetables by application of different edible coatings: A
review. Lwt, 89, pp.198-209.
Yuan, G., Chen, X. & Li, D. (2016). Chitosan films and coatings containing essential oils: The
antioxidant and antimicrobial activity, and application in food systems. Food Research
International, 89, pp.117-128.
Shah, N.N. & et. al. (2016). n-Octenyl succinylation of pullulan: Effect on its physico-
mechanical and thermal properties and application as an edible coating on fruits. Food
Hydrocolloids, 55, pp.179-188.
Saberi, B. & Golding, J.B. (2018). Postharvest application of biopolymer-based edible coatings
to improve the quality of fresh horticultural produce. In Polymers for food
applications(pp. 211-250). Springer, Cham.
Radi, M. & et. al. (2018). The use of orange peel essential oil microemulsion and nanoemulsion
in pectin‐based coating to extend the shelf life of fresh‐cut orange. Journal of Food
Processing and Preservation, 42(2), p.e13441.
Noshirvani, N. & et. al. (2017). Novel active packaging based on carboxymethyl cellulose-
chitosan-ZnO NPs nanocomposite for increasing the shelf life of bread. Food Packaging
and Shelf Life, 11, pp.106-114.
34
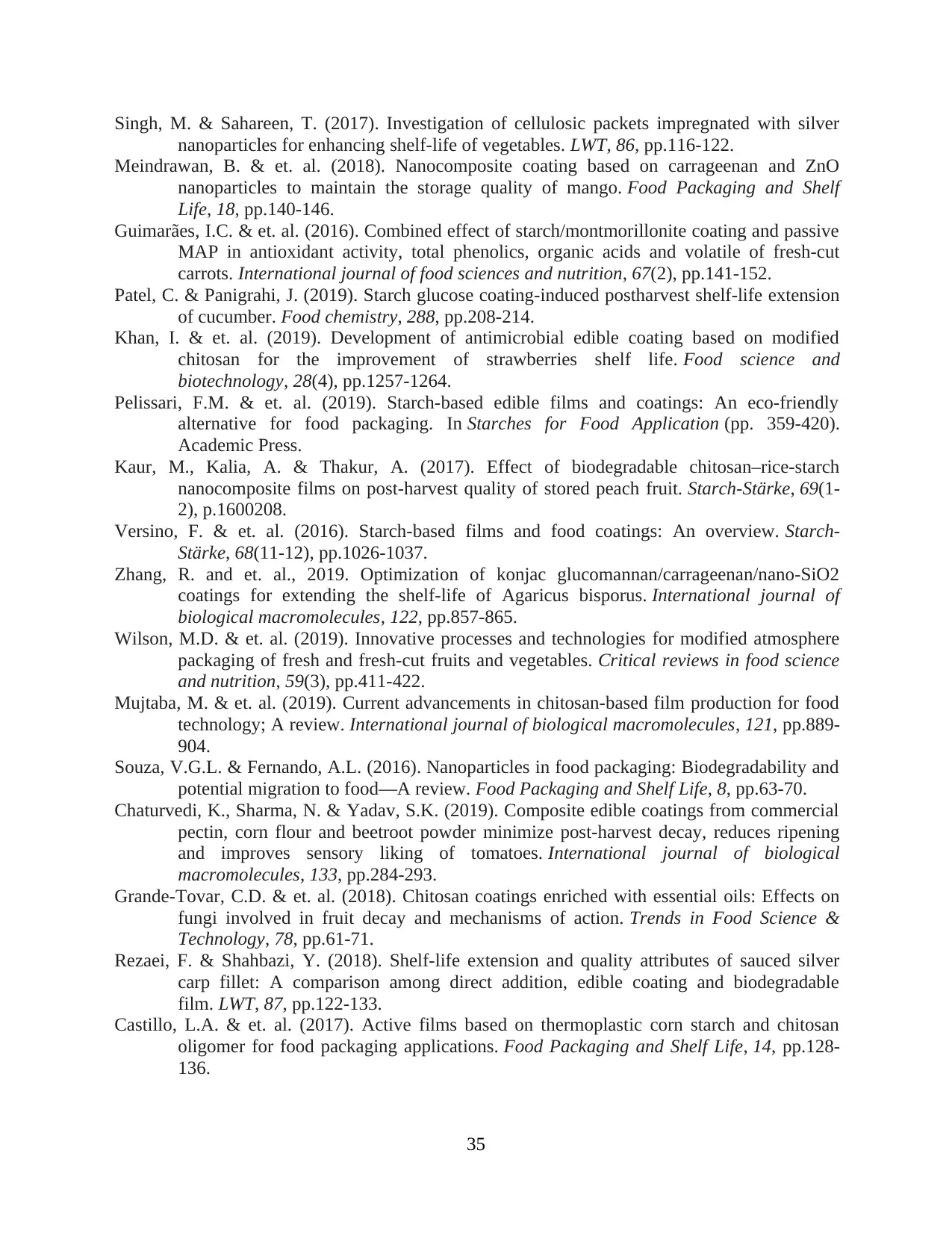
Singh, M. & Sahareen, T. (2017). Investigation of cellulosic packets impregnated with silver
nanoparticles for enhancing shelf-life of vegetables. LWT, 86, pp.116-122.
Meindrawan, B. & et. al. (2018). Nanocomposite coating based on carrageenan and ZnO
nanoparticles to maintain the storage quality of mango. Food Packaging and Shelf
Life, 18, pp.140-146.
Guimarães, I.C. & et. al. (2016). Combined effect of starch/montmorillonite coating and passive
MAP in antioxidant activity, total phenolics, organic acids and volatile of fresh-cut
carrots. International journal of food sciences and nutrition, 67(2), pp.141-152.
Patel, C. & Panigrahi, J. (2019). Starch glucose coating-induced postharvest shelf-life extension
of cucumber. Food chemistry, 288, pp.208-214.
Khan, I. & et. al. (2019). Development of antimicrobial edible coating based on modified
chitosan for the improvement of strawberries shelf life. Food science and
biotechnology, 28(4), pp.1257-1264.
Pelissari, F.M. & et. al. (2019). Starch-based edible films and coatings: An eco-friendly
alternative for food packaging. In Starches for Food Application (pp. 359-420).
Academic Press.
Kaur, M., Kalia, A. & Thakur, A. (2017). Effect of biodegradable chitosan–rice‐starch
nanocomposite films on post‐harvest quality of stored peach fruit. Starch‐Stärke, 69(1-
2), p.1600208.
Versino, F. & et. al. (2016). Starch‐based films and food coatings: An overview. Starch‐
Stärke, 68(11-12), pp.1026-1037.
Zhang, R. and et. al., 2019. Optimization of konjac glucomannan/carrageenan/nano-SiO2
coatings for extending the shelf-life of Agaricus bisporus. International journal of
biological macromolecules, 122, pp.857-865.
Wilson, M.D. & et. al. (2019). Innovative processes and technologies for modified atmosphere
packaging of fresh and fresh-cut fruits and vegetables. Critical reviews in food science
and nutrition, 59(3), pp.411-422.
Mujtaba, M. & et. al. (2019). Current advancements in chitosan-based film production for food
technology; A review. International journal of biological macromolecules, 121, pp.889-
904.
Souza, V.G.L. & Fernando, A.L. (2016). Nanoparticles in food packaging: Biodegradability and
potential migration to food—A review. Food Packaging and Shelf Life, 8, pp.63-70.
Chaturvedi, K., Sharma, N. & Yadav, S.K. (2019). Composite edible coatings from commercial
pectin, corn flour and beetroot powder minimize post-harvest decay, reduces ripening
and improves sensory liking of tomatoes. International journal of biological
macromolecules, 133, pp.284-293.
Grande-Tovar, C.D. & et. al. (2018). Chitosan coatings enriched with essential oils: Effects on
fungi involved in fruit decay and mechanisms of action. Trends in Food Science &
Technology, 78, pp.61-71.
Rezaei, F. & Shahbazi, Y. (2018). Shelf-life extension and quality attributes of sauced silver
carp fillet: A comparison among direct addition, edible coating and biodegradable
film. LWT, 87, pp.122-133.
Castillo, L.A. & et. al. (2017). Active films based on thermoplastic corn starch and chitosan
oligomer for food packaging applications. Food Packaging and Shelf Life, 14, pp.128-
136.
35
nanoparticles for enhancing shelf-life of vegetables. LWT, 86, pp.116-122.
Meindrawan, B. & et. al. (2018). Nanocomposite coating based on carrageenan and ZnO
nanoparticles to maintain the storage quality of mango. Food Packaging and Shelf
Life, 18, pp.140-146.
Guimarães, I.C. & et. al. (2016). Combined effect of starch/montmorillonite coating and passive
MAP in antioxidant activity, total phenolics, organic acids and volatile of fresh-cut
carrots. International journal of food sciences and nutrition, 67(2), pp.141-152.
Patel, C. & Panigrahi, J. (2019). Starch glucose coating-induced postharvest shelf-life extension
of cucumber. Food chemistry, 288, pp.208-214.
Khan, I. & et. al. (2019). Development of antimicrobial edible coating based on modified
chitosan for the improvement of strawberries shelf life. Food science and
biotechnology, 28(4), pp.1257-1264.
Pelissari, F.M. & et. al. (2019). Starch-based edible films and coatings: An eco-friendly
alternative for food packaging. In Starches for Food Application (pp. 359-420).
Academic Press.
Kaur, M., Kalia, A. & Thakur, A. (2017). Effect of biodegradable chitosan–rice‐starch
nanocomposite films on post‐harvest quality of stored peach fruit. Starch‐Stärke, 69(1-
2), p.1600208.
Versino, F. & et. al. (2016). Starch‐based films and food coatings: An overview. Starch‐
Stärke, 68(11-12), pp.1026-1037.
Zhang, R. and et. al., 2019. Optimization of konjac glucomannan/carrageenan/nano-SiO2
coatings for extending the shelf-life of Agaricus bisporus. International journal of
biological macromolecules, 122, pp.857-865.
Wilson, M.D. & et. al. (2019). Innovative processes and technologies for modified atmosphere
packaging of fresh and fresh-cut fruits and vegetables. Critical reviews in food science
and nutrition, 59(3), pp.411-422.
Mujtaba, M. & et. al. (2019). Current advancements in chitosan-based film production for food
technology; A review. International journal of biological macromolecules, 121, pp.889-
904.
Souza, V.G.L. & Fernando, A.L. (2016). Nanoparticles in food packaging: Biodegradability and
potential migration to food—A review. Food Packaging and Shelf Life, 8, pp.63-70.
Chaturvedi, K., Sharma, N. & Yadav, S.K. (2019). Composite edible coatings from commercial
pectin, corn flour and beetroot powder minimize post-harvest decay, reduces ripening
and improves sensory liking of tomatoes. International journal of biological
macromolecules, 133, pp.284-293.
Grande-Tovar, C.D. & et. al. (2018). Chitosan coatings enriched with essential oils: Effects on
fungi involved in fruit decay and mechanisms of action. Trends in Food Science &
Technology, 78, pp.61-71.
Rezaei, F. & Shahbazi, Y. (2018). Shelf-life extension and quality attributes of sauced silver
carp fillet: A comparison among direct addition, edible coating and biodegradable
film. LWT, 87, pp.122-133.
Castillo, L.A. & et. al. (2017). Active films based on thermoplastic corn starch and chitosan
oligomer for food packaging applications. Food Packaging and Shelf Life, 14, pp.128-
136.
35
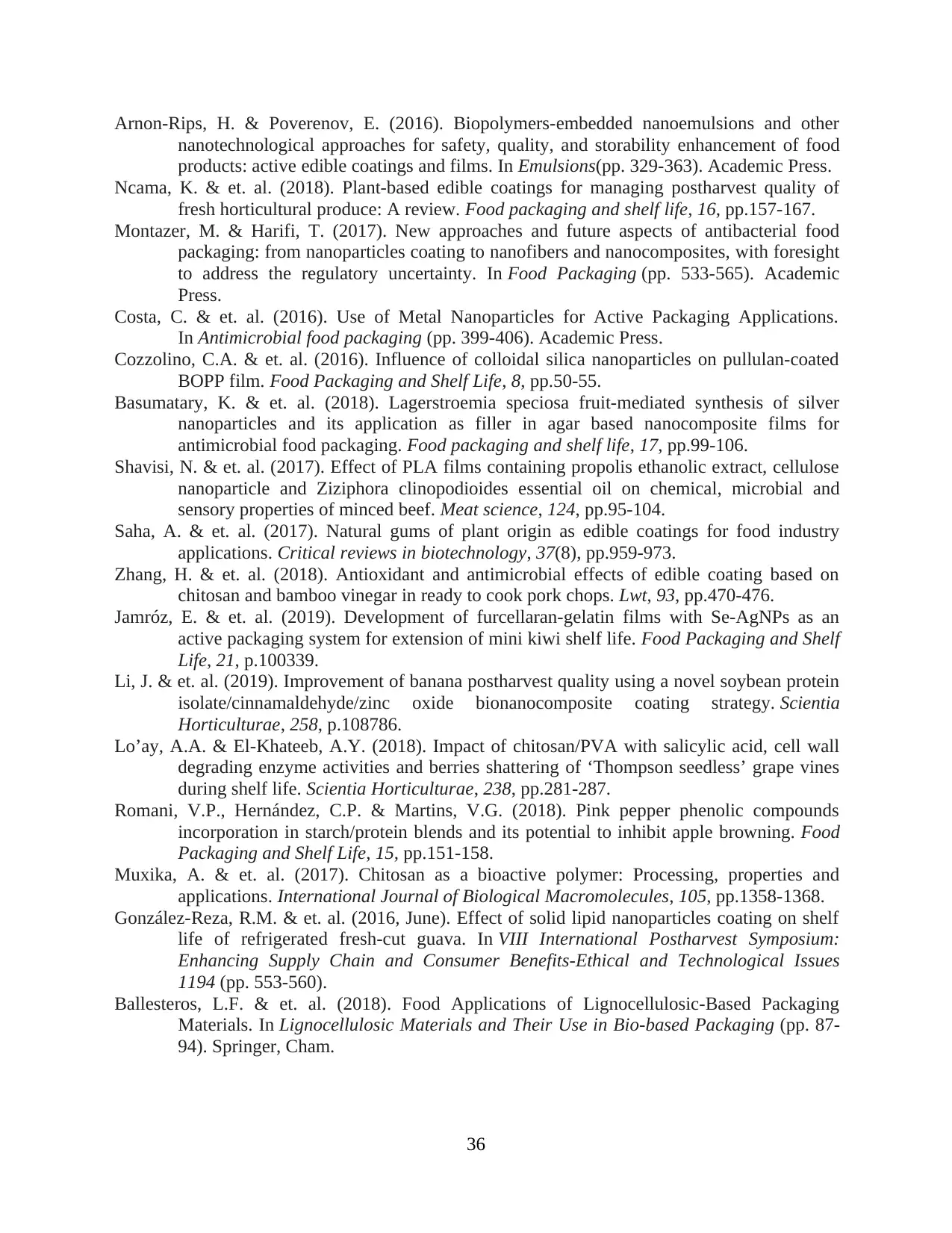
Arnon-Rips, H. & Poverenov, E. (2016). Biopolymers-embedded nanoemulsions and other
nanotechnological approaches for safety, quality, and storability enhancement of food
products: active edible coatings and films. In Emulsions(pp. 329-363). Academic Press.
Ncama, K. & et. al. (2018). Plant-based edible coatings for managing postharvest quality of
fresh horticultural produce: A review. Food packaging and shelf life, 16, pp.157-167.
Montazer, M. & Harifi, T. (2017). New approaches and future aspects of antibacterial food
packaging: from nanoparticles coating to nanofibers and nanocomposites, with foresight
to address the regulatory uncertainty. In Food Packaging (pp. 533-565). Academic
Press.
Costa, C. & et. al. (2016). Use of Metal Nanoparticles for Active Packaging Applications.
In Antimicrobial food packaging (pp. 399-406). Academic Press.
Cozzolino, C.A. & et. al. (2016). Influence of colloidal silica nanoparticles on pullulan-coated
BOPP film. Food Packaging and Shelf Life, 8, pp.50-55.
Basumatary, K. & et. al. (2018). Lagerstroemia speciosa fruit-mediated synthesis of silver
nanoparticles and its application as filler in agar based nanocomposite films for
antimicrobial food packaging. Food packaging and shelf life, 17, pp.99-106.
Shavisi, N. & et. al. (2017). Effect of PLA films containing propolis ethanolic extract, cellulose
nanoparticle and Ziziphora clinopodioides essential oil on chemical, microbial and
sensory properties of minced beef. Meat science, 124, pp.95-104.
Saha, A. & et. al. (2017). Natural gums of plant origin as edible coatings for food industry
applications. Critical reviews in biotechnology, 37(8), pp.959-973.
Zhang, H. & et. al. (2018). Antioxidant and antimicrobial effects of edible coating based on
chitosan and bamboo vinegar in ready to cook pork chops. Lwt, 93, pp.470-476.
Jamróz, E. & et. al. (2019). Development of furcellaran-gelatin films with Se-AgNPs as an
active packaging system for extension of mini kiwi shelf life. Food Packaging and Shelf
Life, 21, p.100339.
Li, J. & et. al. (2019). Improvement of banana postharvest quality using a novel soybean protein
isolate/cinnamaldehyde/zinc oxide bionanocomposite coating strategy. Scientia
Horticulturae, 258, p.108786.
Lo’ay, A.A. & El-Khateeb, A.Y. (2018). Impact of chitosan/PVA with salicylic acid, cell wall
degrading enzyme activities and berries shattering of ‘Thompson seedless’ grape vines
during shelf life. Scientia Horticulturae, 238, pp.281-287.
Romani, V.P., Hernández, C.P. & Martins, V.G. (2018). Pink pepper phenolic compounds
incorporation in starch/protein blends and its potential to inhibit apple browning. Food
Packaging and Shelf Life, 15, pp.151-158.
Muxika, A. & et. al. (2017). Chitosan as a bioactive polymer: Processing, properties and
applications. International Journal of Biological Macromolecules, 105, pp.1358-1368.
González-Reza, R.M. & et. al. (2016, June). Effect of solid lipid nanoparticles coating on shelf
life of refrigerated fresh-cut guava. In VIII International Postharvest Symposium:
Enhancing Supply Chain and Consumer Benefits-Ethical and Technological Issues
1194 (pp. 553-560).
Ballesteros, L.F. & et. al. (2018). Food Applications of Lignocellulosic-Based Packaging
Materials. In Lignocellulosic Materials and Their Use in Bio-based Packaging (pp. 87-
94). Springer, Cham.
36
nanotechnological approaches for safety, quality, and storability enhancement of food
products: active edible coatings and films. In Emulsions(pp. 329-363). Academic Press.
Ncama, K. & et. al. (2018). Plant-based edible coatings for managing postharvest quality of
fresh horticultural produce: A review. Food packaging and shelf life, 16, pp.157-167.
Montazer, M. & Harifi, T. (2017). New approaches and future aspects of antibacterial food
packaging: from nanoparticles coating to nanofibers and nanocomposites, with foresight
to address the regulatory uncertainty. In Food Packaging (pp. 533-565). Academic
Press.
Costa, C. & et. al. (2016). Use of Metal Nanoparticles for Active Packaging Applications.
In Antimicrobial food packaging (pp. 399-406). Academic Press.
Cozzolino, C.A. & et. al. (2016). Influence of colloidal silica nanoparticles on pullulan-coated
BOPP film. Food Packaging and Shelf Life, 8, pp.50-55.
Basumatary, K. & et. al. (2018). Lagerstroemia speciosa fruit-mediated synthesis of silver
nanoparticles and its application as filler in agar based nanocomposite films for
antimicrobial food packaging. Food packaging and shelf life, 17, pp.99-106.
Shavisi, N. & et. al. (2017). Effect of PLA films containing propolis ethanolic extract, cellulose
nanoparticle and Ziziphora clinopodioides essential oil on chemical, microbial and
sensory properties of minced beef. Meat science, 124, pp.95-104.
Saha, A. & et. al. (2017). Natural gums of plant origin as edible coatings for food industry
applications. Critical reviews in biotechnology, 37(8), pp.959-973.
Zhang, H. & et. al. (2018). Antioxidant and antimicrobial effects of edible coating based on
chitosan and bamboo vinegar in ready to cook pork chops. Lwt, 93, pp.470-476.
Jamróz, E. & et. al. (2019). Development of furcellaran-gelatin films with Se-AgNPs as an
active packaging system for extension of mini kiwi shelf life. Food Packaging and Shelf
Life, 21, p.100339.
Li, J. & et. al. (2019). Improvement of banana postharvest quality using a novel soybean protein
isolate/cinnamaldehyde/zinc oxide bionanocomposite coating strategy. Scientia
Horticulturae, 258, p.108786.
Lo’ay, A.A. & El-Khateeb, A.Y. (2018). Impact of chitosan/PVA with salicylic acid, cell wall
degrading enzyme activities and berries shattering of ‘Thompson seedless’ grape vines
during shelf life. Scientia Horticulturae, 238, pp.281-287.
Romani, V.P., Hernández, C.P. & Martins, V.G. (2018). Pink pepper phenolic compounds
incorporation in starch/protein blends and its potential to inhibit apple browning. Food
Packaging and Shelf Life, 15, pp.151-158.
Muxika, A. & et. al. (2017). Chitosan as a bioactive polymer: Processing, properties and
applications. International Journal of Biological Macromolecules, 105, pp.1358-1368.
González-Reza, R.M. & et. al. (2016, June). Effect of solid lipid nanoparticles coating on shelf
life of refrigerated fresh-cut guava. In VIII International Postharvest Symposium:
Enhancing Supply Chain and Consumer Benefits-Ethical and Technological Issues
1194 (pp. 553-560).
Ballesteros, L.F. & et. al. (2018). Food Applications of Lignocellulosic-Based Packaging
Materials. In Lignocellulosic Materials and Their Use in Bio-based Packaging (pp. 87-
94). Springer, Cham.
36
Paraphrase This Document
Need a fresh take? Get an instant paraphrase of this document with our AI Paraphraser
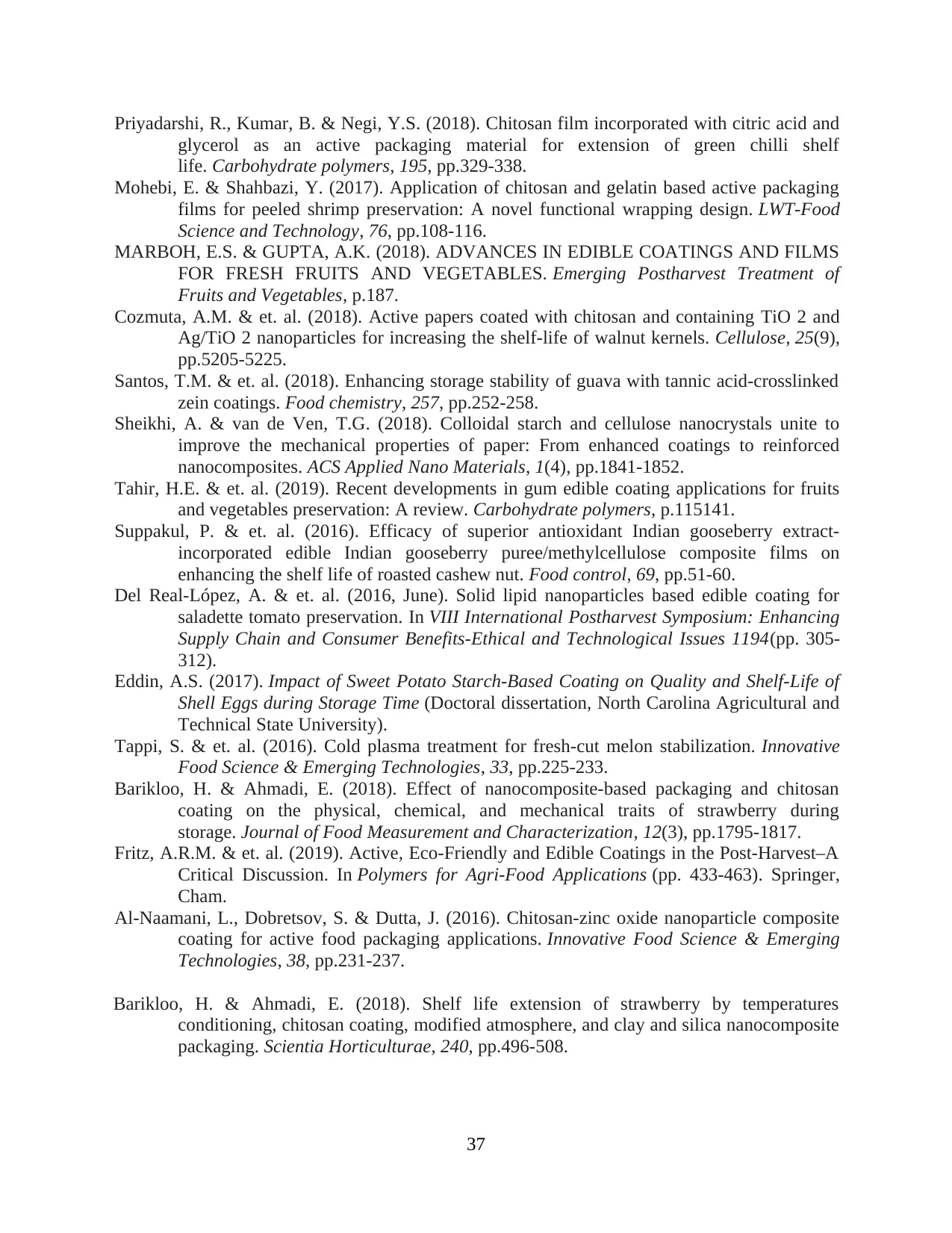
Priyadarshi, R., Kumar, B. & Negi, Y.S. (2018). Chitosan film incorporated with citric acid and
glycerol as an active packaging material for extension of green chilli shelf
life. Carbohydrate polymers, 195, pp.329-338.
Mohebi, E. & Shahbazi, Y. (2017). Application of chitosan and gelatin based active packaging
films for peeled shrimp preservation: A novel functional wrapping design. LWT-Food
Science and Technology, 76, pp.108-116.
MARBOH, E.S. & GUPTA, A.K. (2018). ADVANCES IN EDIBLE COATINGS AND FILMS
FOR FRESH FRUITS AND VEGETABLES. Emerging Postharvest Treatment of
Fruits and Vegetables, p.187.
Cozmuta, A.M. & et. al. (2018). Active papers coated with chitosan and containing TiO 2 and
Ag/TiO 2 nanoparticles for increasing the shelf-life of walnut kernels. Cellulose, 25(9),
pp.5205-5225.
Santos, T.M. & et. al. (2018). Enhancing storage stability of guava with tannic acid-crosslinked
zein coatings. Food chemistry, 257, pp.252-258.
Sheikhi, A. & van de Ven, T.G. (2018). Colloidal starch and cellulose nanocrystals unite to
improve the mechanical properties of paper: From enhanced coatings to reinforced
nanocomposites. ACS Applied Nano Materials, 1(4), pp.1841-1852.
Tahir, H.E. & et. al. (2019). Recent developments in gum edible coating applications for fruits
and vegetables preservation: A review. Carbohydrate polymers, p.115141.
Suppakul, P. & et. al. (2016). Efficacy of superior antioxidant Indian gooseberry extract-
incorporated edible Indian gooseberry puree/methylcellulose composite films on
enhancing the shelf life of roasted cashew nut. Food control, 69, pp.51-60.
Del Real-López, A. & et. al. (2016, June). Solid lipid nanoparticles based edible coating for
saladette tomato preservation. In VIII International Postharvest Symposium: Enhancing
Supply Chain and Consumer Benefits-Ethical and Technological Issues 1194(pp. 305-
312).
Eddin, A.S. (2017). Impact of Sweet Potato Starch-Based Coating on Quality and Shelf-Life of
Shell Eggs during Storage Time (Doctoral dissertation, North Carolina Agricultural and
Technical State University).
Tappi, S. & et. al. (2016). Cold plasma treatment for fresh-cut melon stabilization. Innovative
Food Science & Emerging Technologies, 33, pp.225-233.
Barikloo, H. & Ahmadi, E. (2018). Effect of nanocomposite-based packaging and chitosan
coating on the physical, chemical, and mechanical traits of strawberry during
storage. Journal of Food Measurement and Characterization, 12(3), pp.1795-1817.
Fritz, A.R.M. & et. al. (2019). Active, Eco-Friendly and Edible Coatings in the Post-Harvest–A
Critical Discussion. In Polymers for Agri-Food Applications (pp. 433-463). Springer,
Cham.
Al-Naamani, L., Dobretsov, S. & Dutta, J. (2016). Chitosan-zinc oxide nanoparticle composite
coating for active food packaging applications. Innovative Food Science & Emerging
Technologies, 38, pp.231-237.
Barikloo, H. & Ahmadi, E. (2018). Shelf life extension of strawberry by temperatures
conditioning, chitosan coating, modified atmosphere, and clay and silica nanocomposite
packaging. Scientia Horticulturae, 240, pp.496-508.
37
glycerol as an active packaging material for extension of green chilli shelf
life. Carbohydrate polymers, 195, pp.329-338.
Mohebi, E. & Shahbazi, Y. (2017). Application of chitosan and gelatin based active packaging
films for peeled shrimp preservation: A novel functional wrapping design. LWT-Food
Science and Technology, 76, pp.108-116.
MARBOH, E.S. & GUPTA, A.K. (2018). ADVANCES IN EDIBLE COATINGS AND FILMS
FOR FRESH FRUITS AND VEGETABLES. Emerging Postharvest Treatment of
Fruits and Vegetables, p.187.
Cozmuta, A.M. & et. al. (2018). Active papers coated with chitosan and containing TiO 2 and
Ag/TiO 2 nanoparticles for increasing the shelf-life of walnut kernels. Cellulose, 25(9),
pp.5205-5225.
Santos, T.M. & et. al. (2018). Enhancing storage stability of guava with tannic acid-crosslinked
zein coatings. Food chemistry, 257, pp.252-258.
Sheikhi, A. & van de Ven, T.G. (2018). Colloidal starch and cellulose nanocrystals unite to
improve the mechanical properties of paper: From enhanced coatings to reinforced
nanocomposites. ACS Applied Nano Materials, 1(4), pp.1841-1852.
Tahir, H.E. & et. al. (2019). Recent developments in gum edible coating applications for fruits
and vegetables preservation: A review. Carbohydrate polymers, p.115141.
Suppakul, P. & et. al. (2016). Efficacy of superior antioxidant Indian gooseberry extract-
incorporated edible Indian gooseberry puree/methylcellulose composite films on
enhancing the shelf life of roasted cashew nut. Food control, 69, pp.51-60.
Del Real-López, A. & et. al. (2016, June). Solid lipid nanoparticles based edible coating for
saladette tomato preservation. In VIII International Postharvest Symposium: Enhancing
Supply Chain and Consumer Benefits-Ethical and Technological Issues 1194(pp. 305-
312).
Eddin, A.S. (2017). Impact of Sweet Potato Starch-Based Coating on Quality and Shelf-Life of
Shell Eggs during Storage Time (Doctoral dissertation, North Carolina Agricultural and
Technical State University).
Tappi, S. & et. al. (2016). Cold plasma treatment for fresh-cut melon stabilization. Innovative
Food Science & Emerging Technologies, 33, pp.225-233.
Barikloo, H. & Ahmadi, E. (2018). Effect of nanocomposite-based packaging and chitosan
coating on the physical, chemical, and mechanical traits of strawberry during
storage. Journal of Food Measurement and Characterization, 12(3), pp.1795-1817.
Fritz, A.R.M. & et. al. (2019). Active, Eco-Friendly and Edible Coatings in the Post-Harvest–A
Critical Discussion. In Polymers for Agri-Food Applications (pp. 433-463). Springer,
Cham.
Al-Naamani, L., Dobretsov, S. & Dutta, J. (2016). Chitosan-zinc oxide nanoparticle composite
coating for active food packaging applications. Innovative Food Science & Emerging
Technologies, 38, pp.231-237.
Barikloo, H. & Ahmadi, E. (2018). Shelf life extension of strawberry by temperatures
conditioning, chitosan coating, modified atmosphere, and clay and silica nanocomposite
packaging. Scientia Horticulturae, 240, pp.496-508.
37
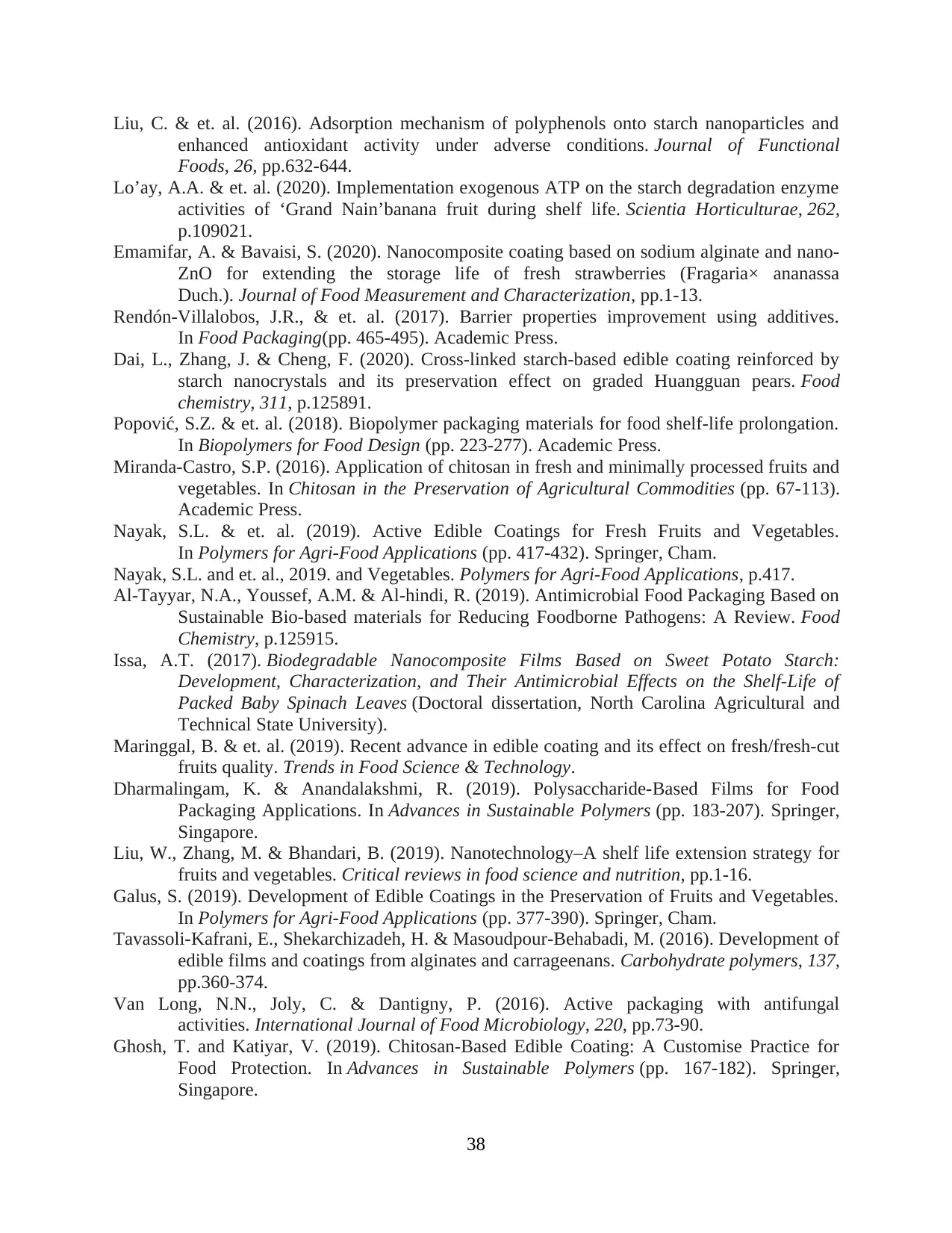
Liu, C. & et. al. (2016). Adsorption mechanism of polyphenols onto starch nanoparticles and
enhanced antioxidant activity under adverse conditions. Journal of Functional
Foods, 26, pp.632-644.
Lo’ay, A.A. & et. al. (2020). Implementation exogenous ATP on the starch degradation enzyme
activities of ‘Grand Nain’banana fruit during shelf life. Scientia Horticulturae, 262,
p.109021.
Emamifar, A. & Bavaisi, S. (2020). Nanocomposite coating based on sodium alginate and nano-
ZnO for extending the storage life of fresh strawberries (Fragaria× ananassa
Duch.). Journal of Food Measurement and Characterization, pp.1-13.
Rendón-Villalobos, J.R., & et. al. (2017). Barrier properties improvement using additives.
In Food Packaging(pp. 465-495). Academic Press.
Dai, L., Zhang, J. & Cheng, F. (2020). Cross-linked starch-based edible coating reinforced by
starch nanocrystals and its preservation effect on graded Huangguan pears. Food
chemistry, 311, p.125891.
Popović, S.Z. & et. al. (2018). Biopolymer packaging materials for food shelf-life prolongation.
In Biopolymers for Food Design (pp. 223-277). Academic Press.
Miranda-Castro, S.P. (2016). Application of chitosan in fresh and minimally processed fruits and
vegetables. In Chitosan in the Preservation of Agricultural Commodities (pp. 67-113).
Academic Press.
Nayak, S.L. & et. al. (2019). Active Edible Coatings for Fresh Fruits and Vegetables.
In Polymers for Agri-Food Applications (pp. 417-432). Springer, Cham.
Nayak, S.L. and et. al., 2019. and Vegetables. Polymers for Agri-Food Applications, p.417.
Al-Tayyar, N.A., Youssef, A.M. & Al-hindi, R. (2019). Antimicrobial Food Packaging Based on
Sustainable Bio-based materials for Reducing Foodborne Pathogens: A Review. Food
Chemistry, p.125915.
Issa, A.T. (2017). Biodegradable Nanocomposite Films Based on Sweet Potato Starch:
Development, Characterization, and Their Antimicrobial Effects on the Shelf-Life of
Packed Baby Spinach Leaves (Doctoral dissertation, North Carolina Agricultural and
Technical State University).
Maringgal, B. & et. al. (2019). Recent advance in edible coating and its effect on fresh/fresh-cut
fruits quality. Trends in Food Science & Technology.
Dharmalingam, K. & Anandalakshmi, R. (2019). Polysaccharide-Based Films for Food
Packaging Applications. In Advances in Sustainable Polymers (pp. 183-207). Springer,
Singapore.
Liu, W., Zhang, M. & Bhandari, B. (2019). Nanotechnology–A shelf life extension strategy for
fruits and vegetables. Critical reviews in food science and nutrition, pp.1-16.
Galus, S. (2019). Development of Edible Coatings in the Preservation of Fruits and Vegetables.
In Polymers for Agri-Food Applications (pp. 377-390). Springer, Cham.
Tavassoli-Kafrani, E., Shekarchizadeh, H. & Masoudpour-Behabadi, M. (2016). Development of
edible films and coatings from alginates and carrageenans. Carbohydrate polymers, 137,
pp.360-374.
Van Long, N.N., Joly, C. & Dantigny, P. (2016). Active packaging with antifungal
activities. International Journal of Food Microbiology, 220, pp.73-90.
Ghosh, T. and Katiyar, V. (2019). Chitosan-Based Edible Coating: A Customise Practice for
Food Protection. In Advances in Sustainable Polymers (pp. 167-182). Springer,
Singapore.
38
enhanced antioxidant activity under adverse conditions. Journal of Functional
Foods, 26, pp.632-644.
Lo’ay, A.A. & et. al. (2020). Implementation exogenous ATP on the starch degradation enzyme
activities of ‘Grand Nain’banana fruit during shelf life. Scientia Horticulturae, 262,
p.109021.
Emamifar, A. & Bavaisi, S. (2020). Nanocomposite coating based on sodium alginate and nano-
ZnO for extending the storage life of fresh strawberries (Fragaria× ananassa
Duch.). Journal of Food Measurement and Characterization, pp.1-13.
Rendón-Villalobos, J.R., & et. al. (2017). Barrier properties improvement using additives.
In Food Packaging(pp. 465-495). Academic Press.
Dai, L., Zhang, J. & Cheng, F. (2020). Cross-linked starch-based edible coating reinforced by
starch nanocrystals and its preservation effect on graded Huangguan pears. Food
chemistry, 311, p.125891.
Popović, S.Z. & et. al. (2018). Biopolymer packaging materials for food shelf-life prolongation.
In Biopolymers for Food Design (pp. 223-277). Academic Press.
Miranda-Castro, S.P. (2016). Application of chitosan in fresh and minimally processed fruits and
vegetables. In Chitosan in the Preservation of Agricultural Commodities (pp. 67-113).
Academic Press.
Nayak, S.L. & et. al. (2019). Active Edible Coatings for Fresh Fruits and Vegetables.
In Polymers for Agri-Food Applications (pp. 417-432). Springer, Cham.
Nayak, S.L. and et. al., 2019. and Vegetables. Polymers for Agri-Food Applications, p.417.
Al-Tayyar, N.A., Youssef, A.M. & Al-hindi, R. (2019). Antimicrobial Food Packaging Based on
Sustainable Bio-based materials for Reducing Foodborne Pathogens: A Review. Food
Chemistry, p.125915.
Issa, A.T. (2017). Biodegradable Nanocomposite Films Based on Sweet Potato Starch:
Development, Characterization, and Their Antimicrobial Effects on the Shelf-Life of
Packed Baby Spinach Leaves (Doctoral dissertation, North Carolina Agricultural and
Technical State University).
Maringgal, B. & et. al. (2019). Recent advance in edible coating and its effect on fresh/fresh-cut
fruits quality. Trends in Food Science & Technology.
Dharmalingam, K. & Anandalakshmi, R. (2019). Polysaccharide-Based Films for Food
Packaging Applications. In Advances in Sustainable Polymers (pp. 183-207). Springer,
Singapore.
Liu, W., Zhang, M. & Bhandari, B. (2019). Nanotechnology–A shelf life extension strategy for
fruits and vegetables. Critical reviews in food science and nutrition, pp.1-16.
Galus, S. (2019). Development of Edible Coatings in the Preservation of Fruits and Vegetables.
In Polymers for Agri-Food Applications (pp. 377-390). Springer, Cham.
Tavassoli-Kafrani, E., Shekarchizadeh, H. & Masoudpour-Behabadi, M. (2016). Development of
edible films and coatings from alginates and carrageenans. Carbohydrate polymers, 137,
pp.360-374.
Van Long, N.N., Joly, C. & Dantigny, P. (2016). Active packaging with antifungal
activities. International Journal of Food Microbiology, 220, pp.73-90.
Ghosh, T. and Katiyar, V. (2019). Chitosan-Based Edible Coating: A Customise Practice for
Food Protection. In Advances in Sustainable Polymers (pp. 167-182). Springer,
Singapore.
38
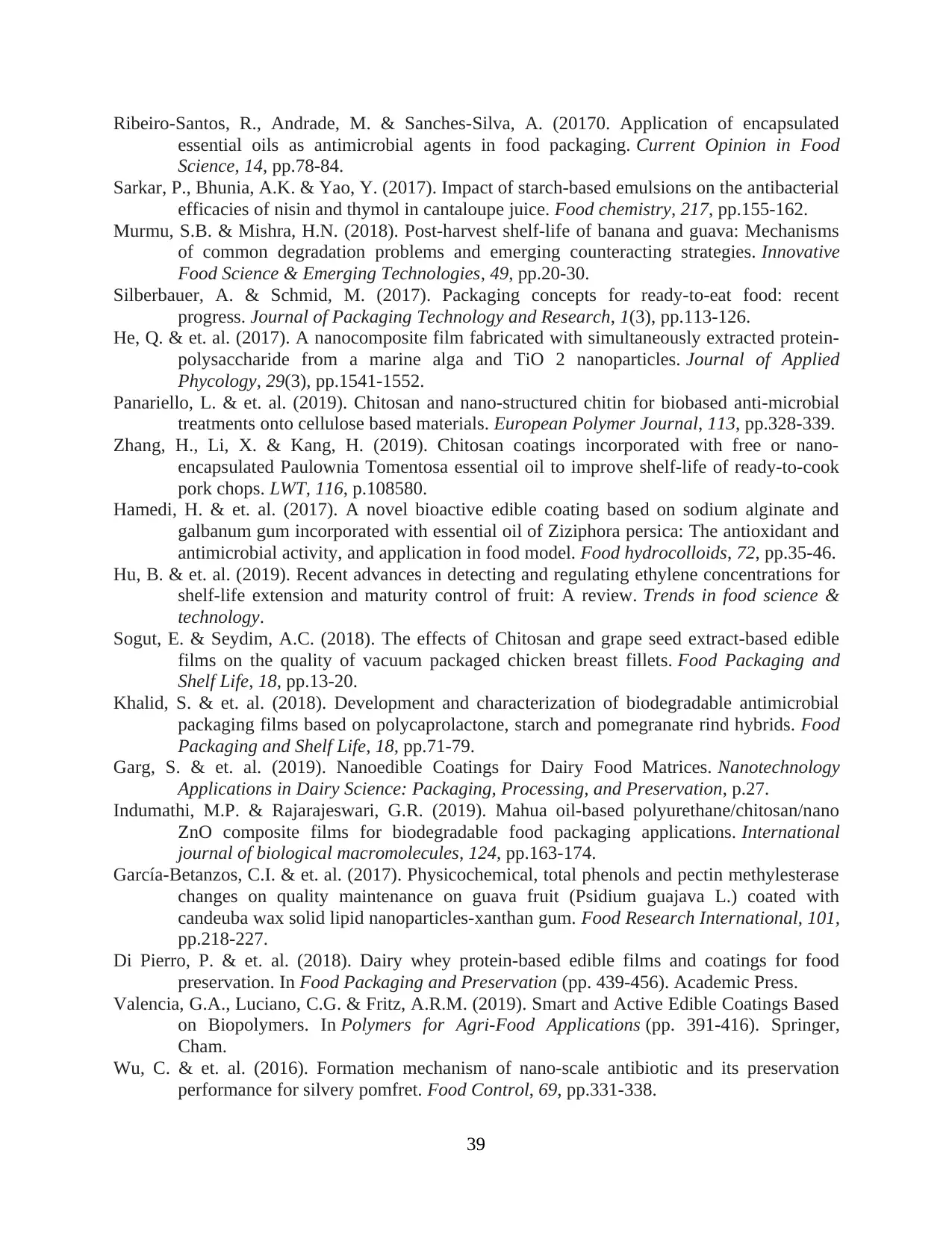
Ribeiro-Santos, R., Andrade, M. & Sanches-Silva, A. (20170. Application of encapsulated
essential oils as antimicrobial agents in food packaging. Current Opinion in Food
Science, 14, pp.78-84.
Sarkar, P., Bhunia, A.K. & Yao, Y. (2017). Impact of starch-based emulsions on the antibacterial
efficacies of nisin and thymol in cantaloupe juice. Food chemistry, 217, pp.155-162.
Murmu, S.B. & Mishra, H.N. (2018). Post-harvest shelf-life of banana and guava: Mechanisms
of common degradation problems and emerging counteracting strategies. Innovative
Food Science & Emerging Technologies, 49, pp.20-30.
Silberbauer, A. & Schmid, M. (2017). Packaging concepts for ready-to-eat food: recent
progress. Journal of Packaging Technology and Research, 1(3), pp.113-126.
He, Q. & et. al. (2017). A nanocomposite film fabricated with simultaneously extracted protein-
polysaccharide from a marine alga and TiO 2 nanoparticles. Journal of Applied
Phycology, 29(3), pp.1541-1552.
Panariello, L. & et. al. (2019). Chitosan and nano-structured chitin for biobased anti-microbial
treatments onto cellulose based materials. European Polymer Journal, 113, pp.328-339.
Zhang, H., Li, X. & Kang, H. (2019). Chitosan coatings incorporated with free or nano-
encapsulated Paulownia Tomentosa essential oil to improve shelf-life of ready-to-cook
pork chops. LWT, 116, p.108580.
Hamedi, H. & et. al. (2017). A novel bioactive edible coating based on sodium alginate and
galbanum gum incorporated with essential oil of Ziziphora persica: The antioxidant and
antimicrobial activity, and application in food model. Food hydrocolloids, 72, pp.35-46.
Hu, B. & et. al. (2019). Recent advances in detecting and regulating ethylene concentrations for
shelf-life extension and maturity control of fruit: A review. Trends in food science &
technology.
Sogut, E. & Seydim, A.C. (2018). The effects of Chitosan and grape seed extract-based edible
films on the quality of vacuum packaged chicken breast fillets. Food Packaging and
Shelf Life, 18, pp.13-20.
Khalid, S. & et. al. (2018). Development and characterization of biodegradable antimicrobial
packaging films based on polycaprolactone, starch and pomegranate rind hybrids. Food
Packaging and Shelf Life, 18, pp.71-79.
Garg, S. & et. al. (2019). Nanoedible Coatings for Dairy Food Matrices. Nanotechnology
Applications in Dairy Science: Packaging, Processing, and Preservation, p.27.
Indumathi, M.P. & Rajarajeswari, G.R. (2019). Mahua oil-based polyurethane/chitosan/nano
ZnO composite films for biodegradable food packaging applications. International
journal of biological macromolecules, 124, pp.163-174.
García-Betanzos, C.I. & et. al. (2017). Physicochemical, total phenols and pectin methylesterase
changes on quality maintenance on guava fruit (Psidium guajava L.) coated with
candeuba wax solid lipid nanoparticles-xanthan gum. Food Research International, 101,
pp.218-227.
Di Pierro, P. & et. al. (2018). Dairy whey protein-based edible films and coatings for food
preservation. In Food Packaging and Preservation (pp. 439-456). Academic Press.
Valencia, G.A., Luciano, C.G. & Fritz, A.R.M. (2019). Smart and Active Edible Coatings Based
on Biopolymers. In Polymers for Agri-Food Applications (pp. 391-416). Springer,
Cham.
Wu, C. & et. al. (2016). Formation mechanism of nano-scale antibiotic and its preservation
performance for silvery pomfret. Food Control, 69, pp.331-338.
39
essential oils as antimicrobial agents in food packaging. Current Opinion in Food
Science, 14, pp.78-84.
Sarkar, P., Bhunia, A.K. & Yao, Y. (2017). Impact of starch-based emulsions on the antibacterial
efficacies of nisin and thymol in cantaloupe juice. Food chemistry, 217, pp.155-162.
Murmu, S.B. & Mishra, H.N. (2018). Post-harvest shelf-life of banana and guava: Mechanisms
of common degradation problems and emerging counteracting strategies. Innovative
Food Science & Emerging Technologies, 49, pp.20-30.
Silberbauer, A. & Schmid, M. (2017). Packaging concepts for ready-to-eat food: recent
progress. Journal of Packaging Technology and Research, 1(3), pp.113-126.
He, Q. & et. al. (2017). A nanocomposite film fabricated with simultaneously extracted protein-
polysaccharide from a marine alga and TiO 2 nanoparticles. Journal of Applied
Phycology, 29(3), pp.1541-1552.
Panariello, L. & et. al. (2019). Chitosan and nano-structured chitin for biobased anti-microbial
treatments onto cellulose based materials. European Polymer Journal, 113, pp.328-339.
Zhang, H., Li, X. & Kang, H. (2019). Chitosan coatings incorporated with free or nano-
encapsulated Paulownia Tomentosa essential oil to improve shelf-life of ready-to-cook
pork chops. LWT, 116, p.108580.
Hamedi, H. & et. al. (2017). A novel bioactive edible coating based on sodium alginate and
galbanum gum incorporated with essential oil of Ziziphora persica: The antioxidant and
antimicrobial activity, and application in food model. Food hydrocolloids, 72, pp.35-46.
Hu, B. & et. al. (2019). Recent advances in detecting and regulating ethylene concentrations for
shelf-life extension and maturity control of fruit: A review. Trends in food science &
technology.
Sogut, E. & Seydim, A.C. (2018). The effects of Chitosan and grape seed extract-based edible
films on the quality of vacuum packaged chicken breast fillets. Food Packaging and
Shelf Life, 18, pp.13-20.
Khalid, S. & et. al. (2018). Development and characterization of biodegradable antimicrobial
packaging films based on polycaprolactone, starch and pomegranate rind hybrids. Food
Packaging and Shelf Life, 18, pp.71-79.
Garg, S. & et. al. (2019). Nanoedible Coatings for Dairy Food Matrices. Nanotechnology
Applications in Dairy Science: Packaging, Processing, and Preservation, p.27.
Indumathi, M.P. & Rajarajeswari, G.R. (2019). Mahua oil-based polyurethane/chitosan/nano
ZnO composite films for biodegradable food packaging applications. International
journal of biological macromolecules, 124, pp.163-174.
García-Betanzos, C.I. & et. al. (2017). Physicochemical, total phenols and pectin methylesterase
changes on quality maintenance on guava fruit (Psidium guajava L.) coated with
candeuba wax solid lipid nanoparticles-xanthan gum. Food Research International, 101,
pp.218-227.
Di Pierro, P. & et. al. (2018). Dairy whey protein-based edible films and coatings for food
preservation. In Food Packaging and Preservation (pp. 439-456). Academic Press.
Valencia, G.A., Luciano, C.G. & Fritz, A.R.M. (2019). Smart and Active Edible Coatings Based
on Biopolymers. In Polymers for Agri-Food Applications (pp. 391-416). Springer,
Cham.
Wu, C. & et. al. (2016). Formation mechanism of nano-scale antibiotic and its preservation
performance for silvery pomfret. Food Control, 69, pp.331-338.
39
Secure Best Marks with AI Grader
Need help grading? Try our AI Grader for instant feedback on your assignments.
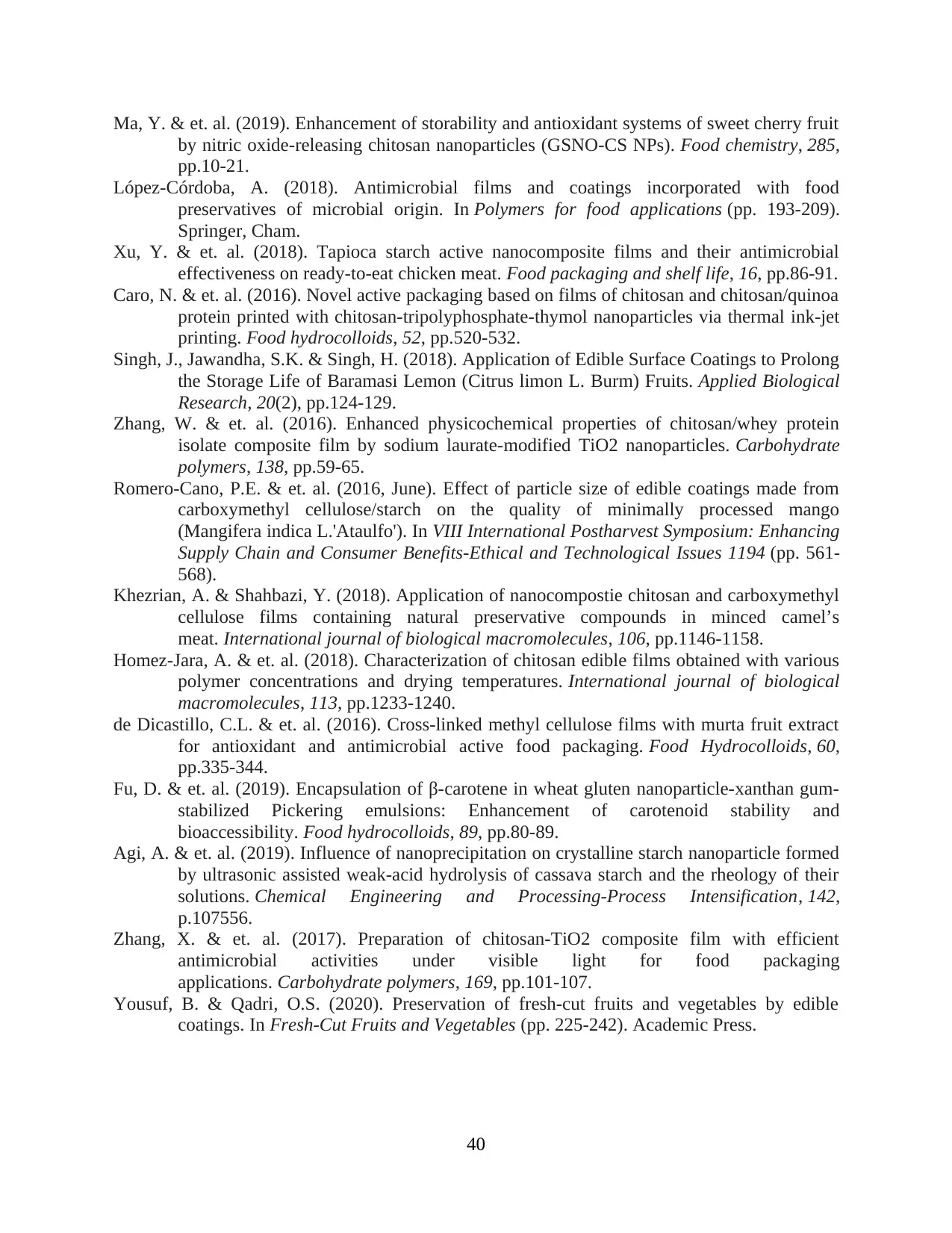
Ma, Y. & et. al. (2019). Enhancement of storability and antioxidant systems of sweet cherry fruit
by nitric oxide-releasing chitosan nanoparticles (GSNO-CS NPs). Food chemistry, 285,
pp.10-21.
López-Córdoba, A. (2018). Antimicrobial films and coatings incorporated with food
preservatives of microbial origin. In Polymers for food applications (pp. 193-209).
Springer, Cham.
Xu, Y. & et. al. (2018). Tapioca starch active nanocomposite films and their antimicrobial
effectiveness on ready-to-eat chicken meat. Food packaging and shelf life, 16, pp.86-91.
Caro, N. & et. al. (2016). Novel active packaging based on films of chitosan and chitosan/quinoa
protein printed with chitosan-tripolyphosphate-thymol nanoparticles via thermal ink-jet
printing. Food hydrocolloids, 52, pp.520-532.
Singh, J., Jawandha, S.K. & Singh, H. (2018). Application of Edible Surface Coatings to Prolong
the Storage Life of Baramasi Lemon (Citrus limon L. Burm) Fruits. Applied Biological
Research, 20(2), pp.124-129.
Zhang, W. & et. al. (2016). Enhanced physicochemical properties of chitosan/whey protein
isolate composite film by sodium laurate-modified TiO2 nanoparticles. Carbohydrate
polymers, 138, pp.59-65.
Romero-Cano, P.E. & et. al. (2016, June). Effect of particle size of edible coatings made from
carboxymethyl cellulose/starch on the quality of minimally processed mango
(Mangifera indica L.'Ataulfo'). In VIII International Postharvest Symposium: Enhancing
Supply Chain and Consumer Benefits-Ethical and Technological Issues 1194 (pp. 561-
568).
Khezrian, A. & Shahbazi, Y. (2018). Application of nanocompostie chitosan and carboxymethyl
cellulose films containing natural preservative compounds in minced camel’s
meat. International journal of biological macromolecules, 106, pp.1146-1158.
Homez-Jara, A. & et. al. (2018). Characterization of chitosan edible films obtained with various
polymer concentrations and drying temperatures. International journal of biological
macromolecules, 113, pp.1233-1240.
de Dicastillo, C.L. & et. al. (2016). Cross-linked methyl cellulose films with murta fruit extract
for antioxidant and antimicrobial active food packaging. Food Hydrocolloids, 60,
pp.335-344.
Fu, D. & et. al. (2019). Encapsulation of β-carotene in wheat gluten nanoparticle-xanthan gum-
stabilized Pickering emulsions: Enhancement of carotenoid stability and
bioaccessibility. Food hydrocolloids, 89, pp.80-89.
Agi, A. & et. al. (2019). Influence of nanoprecipitation on crystalline starch nanoparticle formed
by ultrasonic assisted weak-acid hydrolysis of cassava starch and the rheology of their
solutions. Chemical Engineering and Processing-Process Intensification, 142,
p.107556.
Zhang, X. & et. al. (2017). Preparation of chitosan-TiO2 composite film with efficient
antimicrobial activities under visible light for food packaging
applications. Carbohydrate polymers, 169, pp.101-107.
Yousuf, B. & Qadri, O.S. (2020). Preservation of fresh-cut fruits and vegetables by edible
coatings. In Fresh-Cut Fruits and Vegetables (pp. 225-242). Academic Press.
40
by nitric oxide-releasing chitosan nanoparticles (GSNO-CS NPs). Food chemistry, 285,
pp.10-21.
López-Córdoba, A. (2018). Antimicrobial films and coatings incorporated with food
preservatives of microbial origin. In Polymers for food applications (pp. 193-209).
Springer, Cham.
Xu, Y. & et. al. (2018). Tapioca starch active nanocomposite films and their antimicrobial
effectiveness on ready-to-eat chicken meat. Food packaging and shelf life, 16, pp.86-91.
Caro, N. & et. al. (2016). Novel active packaging based on films of chitosan and chitosan/quinoa
protein printed with chitosan-tripolyphosphate-thymol nanoparticles via thermal ink-jet
printing. Food hydrocolloids, 52, pp.520-532.
Singh, J., Jawandha, S.K. & Singh, H. (2018). Application of Edible Surface Coatings to Prolong
the Storage Life of Baramasi Lemon (Citrus limon L. Burm) Fruits. Applied Biological
Research, 20(2), pp.124-129.
Zhang, W. & et. al. (2016). Enhanced physicochemical properties of chitosan/whey protein
isolate composite film by sodium laurate-modified TiO2 nanoparticles. Carbohydrate
polymers, 138, pp.59-65.
Romero-Cano, P.E. & et. al. (2016, June). Effect of particle size of edible coatings made from
carboxymethyl cellulose/starch on the quality of minimally processed mango
(Mangifera indica L.'Ataulfo'). In VIII International Postharvest Symposium: Enhancing
Supply Chain and Consumer Benefits-Ethical and Technological Issues 1194 (pp. 561-
568).
Khezrian, A. & Shahbazi, Y. (2018). Application of nanocompostie chitosan and carboxymethyl
cellulose films containing natural preservative compounds in minced camel’s
meat. International journal of biological macromolecules, 106, pp.1146-1158.
Homez-Jara, A. & et. al. (2018). Characterization of chitosan edible films obtained with various
polymer concentrations and drying temperatures. International journal of biological
macromolecules, 113, pp.1233-1240.
de Dicastillo, C.L. & et. al. (2016). Cross-linked methyl cellulose films with murta fruit extract
for antioxidant and antimicrobial active food packaging. Food Hydrocolloids, 60,
pp.335-344.
Fu, D. & et. al. (2019). Encapsulation of β-carotene in wheat gluten nanoparticle-xanthan gum-
stabilized Pickering emulsions: Enhancement of carotenoid stability and
bioaccessibility. Food hydrocolloids, 89, pp.80-89.
Agi, A. & et. al. (2019). Influence of nanoprecipitation on crystalline starch nanoparticle formed
by ultrasonic assisted weak-acid hydrolysis of cassava starch and the rheology of their
solutions. Chemical Engineering and Processing-Process Intensification, 142,
p.107556.
Zhang, X. & et. al. (2017). Preparation of chitosan-TiO2 composite film with efficient
antimicrobial activities under visible light for food packaging
applications. Carbohydrate polymers, 169, pp.101-107.
Yousuf, B. & Qadri, O.S. (2020). Preservation of fresh-cut fruits and vegetables by edible
coatings. In Fresh-Cut Fruits and Vegetables (pp. 225-242). Academic Press.
40
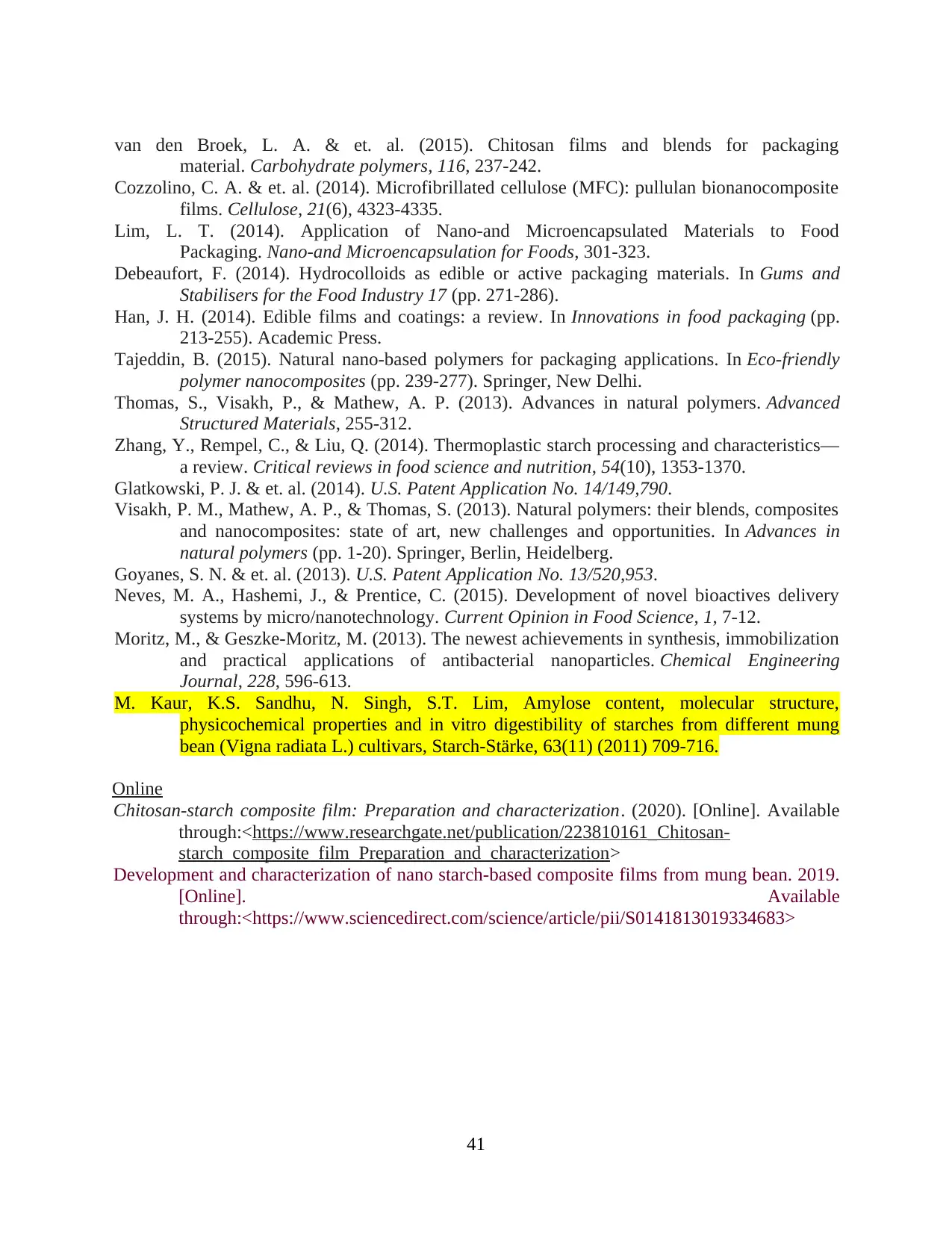
van den Broek, L. A. & et. al. (2015). Chitosan films and blends for packaging
material. Carbohydrate polymers, 116, 237-242.
Cozzolino, C. A. & et. al. (2014). Microfibrillated cellulose (MFC): pullulan bionanocomposite
films. Cellulose, 21(6), 4323-4335.
Lim, L. T. (2014). Application of Nano‐and Microencapsulated Materials to Food
Packaging. Nano‐and Microencapsulation for Foods, 301-323.
Debeaufort, F. (2014). Hydrocolloids as edible or active packaging materials. In Gums and
Stabilisers for the Food Industry 17 (pp. 271-286).
Han, J. H. (2014). Edible films and coatings: a review. In Innovations in food packaging (pp.
213-255). Academic Press.
Tajeddin, B. (2015). Natural nano-based polymers for packaging applications. In Eco-friendly
polymer nanocomposites (pp. 239-277). Springer, New Delhi.
Thomas, S., Visakh, P., & Mathew, A. P. (2013). Advances in natural polymers. Advanced
Structured Materials, 255-312.
Zhang, Y., Rempel, C., & Liu, Q. (2014). Thermoplastic starch processing and characteristics—
a review. Critical reviews in food science and nutrition, 54(10), 1353-1370.
Glatkowski, P. J. & et. al. (2014). U.S. Patent Application No. 14/149,790.
Visakh, P. M., Mathew, A. P., & Thomas, S. (2013). Natural polymers: their blends, composites
and nanocomposites: state of art, new challenges and opportunities. In Advances in
natural polymers (pp. 1-20). Springer, Berlin, Heidelberg.
Goyanes, S. N. & et. al. (2013). U.S. Patent Application No. 13/520,953.
Neves, M. A., Hashemi, J., & Prentice, C. (2015). Development of novel bioactives delivery
systems by micro/nanotechnology. Current Opinion in Food Science, 1, 7-12.
Moritz, M., & Geszke-Moritz, M. (2013). The newest achievements in synthesis, immobilization
and practical applications of antibacterial nanoparticles. Chemical Engineering
Journal, 228, 596-613.
M. Kaur, K.S. Sandhu, N. Singh, S.T. Lim, Amylose content, molecular structure,
physicochemical properties and in vitro digestibility of starches from different mung
bean (Vigna radiata L.) cultivars, Starch‐Stärke, 63(11) (2011) 709-716.
Online
Chitosan-starch composite film: Preparation and characterization. (2020). [Online]. Available
through:<https://www.researchgate.net/publication/223810161_Chitosan-
starch_composite_film_Preparation_and_characterization>
Development and characterization of nano starch-based composite films from mung bean. 2019.
[Online]. Available
through:<https://www.sciencedirect.com/science/article/pii/S0141813019334683>
41
material. Carbohydrate polymers, 116, 237-242.
Cozzolino, C. A. & et. al. (2014). Microfibrillated cellulose (MFC): pullulan bionanocomposite
films. Cellulose, 21(6), 4323-4335.
Lim, L. T. (2014). Application of Nano‐and Microencapsulated Materials to Food
Packaging. Nano‐and Microencapsulation for Foods, 301-323.
Debeaufort, F. (2014). Hydrocolloids as edible or active packaging materials. In Gums and
Stabilisers for the Food Industry 17 (pp. 271-286).
Han, J. H. (2014). Edible films and coatings: a review. In Innovations in food packaging (pp.
213-255). Academic Press.
Tajeddin, B. (2015). Natural nano-based polymers for packaging applications. In Eco-friendly
polymer nanocomposites (pp. 239-277). Springer, New Delhi.
Thomas, S., Visakh, P., & Mathew, A. P. (2013). Advances in natural polymers. Advanced
Structured Materials, 255-312.
Zhang, Y., Rempel, C., & Liu, Q. (2014). Thermoplastic starch processing and characteristics—
a review. Critical reviews in food science and nutrition, 54(10), 1353-1370.
Glatkowski, P. J. & et. al. (2014). U.S. Patent Application No. 14/149,790.
Visakh, P. M., Mathew, A. P., & Thomas, S. (2013). Natural polymers: their blends, composites
and nanocomposites: state of art, new challenges and opportunities. In Advances in
natural polymers (pp. 1-20). Springer, Berlin, Heidelberg.
Goyanes, S. N. & et. al. (2013). U.S. Patent Application No. 13/520,953.
Neves, M. A., Hashemi, J., & Prentice, C. (2015). Development of novel bioactives delivery
systems by micro/nanotechnology. Current Opinion in Food Science, 1, 7-12.
Moritz, M., & Geszke-Moritz, M. (2013). The newest achievements in synthesis, immobilization
and practical applications of antibacterial nanoparticles. Chemical Engineering
Journal, 228, 596-613.
M. Kaur, K.S. Sandhu, N. Singh, S.T. Lim, Amylose content, molecular structure,
physicochemical properties and in vitro digestibility of starches from different mung
bean (Vigna radiata L.) cultivars, Starch‐Stärke, 63(11) (2011) 709-716.
Online
Chitosan-starch composite film: Preparation and characterization. (2020). [Online]. Available
through:<https://www.researchgate.net/publication/223810161_Chitosan-
starch_composite_film_Preparation_and_characterization>
Development and characterization of nano starch-based composite films from mung bean. 2019.
[Online]. Available
through:<https://www.sciencedirect.com/science/article/pii/S0141813019334683>
41
1 out of 42
![[object Object]](/_next/image/?url=%2F_next%2Fstatic%2Fmedia%2Flogo.6d15ce61.png&w=640&q=75)
Your All-in-One AI-Powered Toolkit for Academic Success.
+13062052269
info@desklib.com
Available 24*7 on WhatsApp / Email
Unlock your academic potential
© 2024 | Zucol Services PVT LTD | All rights reserved.