5G mobile technology Paper 2022
VerifiedAdded on 2022/10/18
|6
|5536
|16
AI Summary
Contribute Materials
Your contribution can guide someone’s learning journey. Share your
documents today.
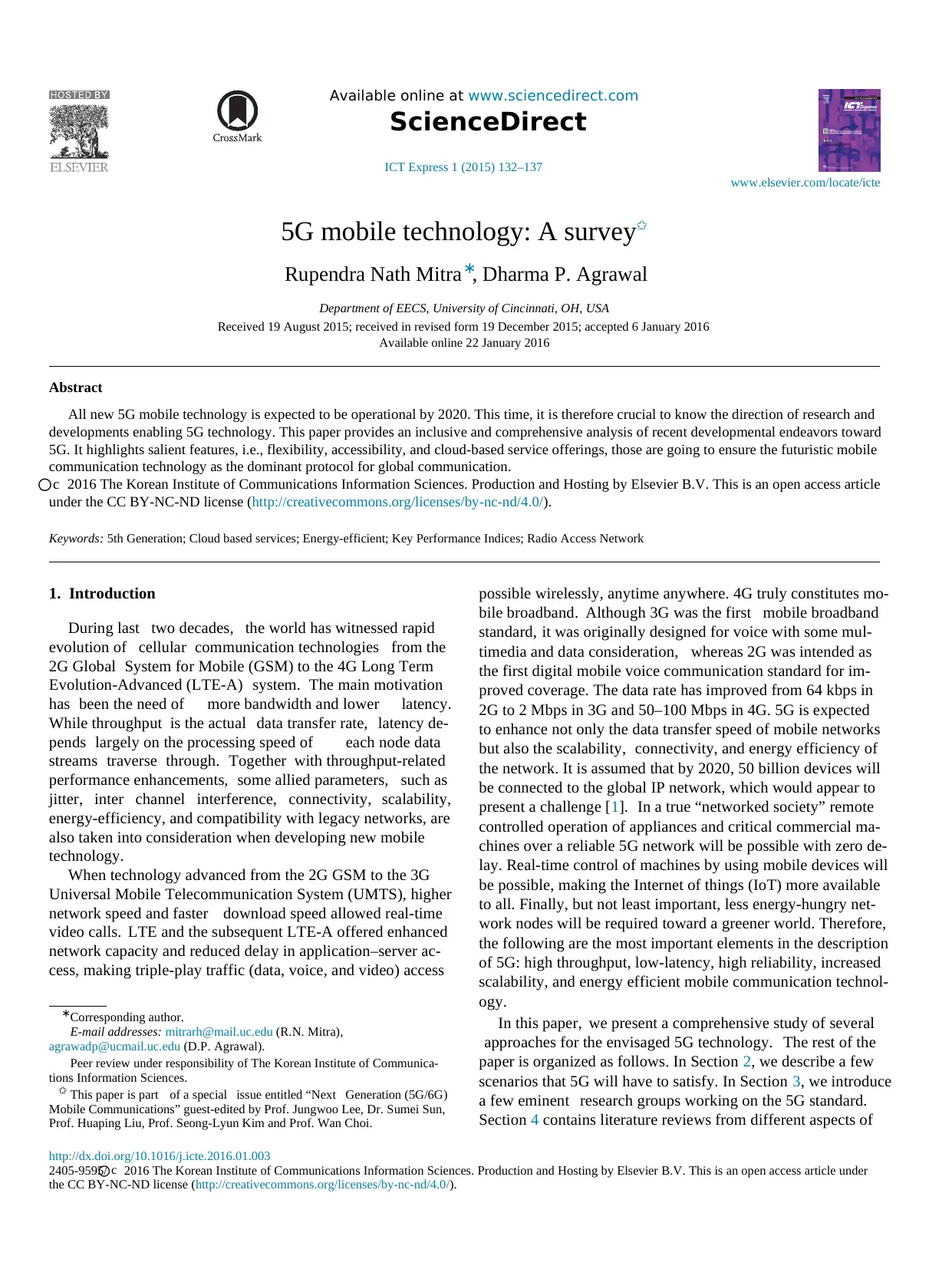
Available online at www.sciencedirect.com
ScienceDirect
ICT Express 1 (2015) 132–137
www.elsevier.com/locate/icte
5G mobile technology: A survey✩
Rupendra Nath Mitra∗, Dharma P. Agrawal
Department of EECS, University of Cincinnati, OH, USA
Received 19 August 2015; received in revised form 19 December 2015; accepted 6 January 2016
Available online 22 January 2016
Abstract
All new 5G mobile technology is expected to be operational by 2020. This time, it is therefore crucial to know the direction of research and
developments enabling 5G technology. This paper provides an inclusive and comprehensive analysis of recent developmental endeavors toward
5G. It highlights salient features, i.e., flexibility, accessibility, and cloud-based service offerings, those are going to ensure the futuristic mobile
communication technology as the dominant protocol for global communication.
c⃝ 2016 The Korean Institute of Communications Information Sciences. Production and Hosting by Elsevier B.V. This is an open access article
under the CC BY-NC-ND license (http://creativecommons.org/licenses/by-nc-nd/4.0/).
Keywords: 5th Generation; Cloud based services; Energy-efficient; Key Performance Indices; Radio Access Network
1. Introduction
During last two decades, the world has witnessed rapid
evolution of cellular communication technologies from the
2G Global System for Mobile (GSM) to the 4G Long Term
Evolution-Advanced (LTE-A) system. The main motivation
has been the need of more bandwidth and lower latency.
While throughput is the actual data transfer rate, latency de-
pends largely on the processing speed of each node data
streams traverse through. Together with throughput-related
performance enhancements, some allied parameters, such as
jitter, inter channel interference, connectivity, scalability,
energy-efficiency, and compatibility with legacy networks, are
also taken into consideration when developing new mobile
technology.
When technology advanced from the 2G GSM to the 3G
Universal Mobile Telecommunication System (UMTS), higher
network speed and faster download speed allowed real-time
video calls. LTE and the subsequent LTE-A offered enhanced
network capacity and reduced delay in application–server ac-
cess, making triple-play traffic (data, voice, and video) access
∗Corresponding author.
E-mail addresses: mitrarh@mail.uc.edu (R.N. Mitra),
agrawadp@ucmail.uc.edu (D.P. Agrawal).
Peer review under responsibility of The Korean Institute of Communica-
tions Information Sciences.
✩ This paper is part of a special issue entitled “Next Generation (5G/6G)
Mobile Communications” guest-edited by Prof. Jungwoo Lee, Dr. Sumei Sun,
Prof. Huaping Liu, Prof. Seong-Lyun Kim and Prof. Wan Choi.
possible wirelessly, anytime anywhere. 4G truly constitutes mo-
bile broadband. Although 3G was the first mobile broadband
standard, it was originally designed for voice with some mul-
timedia and data consideration, whereas 2G was intended as
the first digital mobile voice communication standard for im-
proved coverage. The data rate has improved from 64 kbps in
2G to 2 Mbps in 3G and 50–100 Mbps in 4G. 5G is expected
to enhance not only the data transfer speed of mobile networks
but also the scalability, connectivity, and energy efficiency of
the network. It is assumed that by 2020, 50 billion devices will
be connected to the global IP network, which would appear to
present a challenge [1]. In a true “networked society” remote
controlled operation of appliances and critical commercial ma-
chines over a reliable 5G network will be possible with zero de-
lay. Real-time control of machines by using mobile devices will
be possible, making the Internet of things (IoT) more available
to all. Finally, but not least important, less energy-hungry net-
work nodes will be required toward a greener world. Therefore,
the following are the most important elements in the description
of 5G: high throughput, low-latency, high reliability, increased
scalability, and energy efficient mobile communication technol-
ogy.
In this paper, we present a comprehensive study of several
approaches for the envisaged 5G technology. The rest of the
paper is organized as follows. In Section 2, we describe a few
scenarios that 5G will have to satisfy. In Section 3, we introduce
a few eminent research groups working on the 5G standard.
Section 4 contains literature reviews from different aspects of
http://dx.doi.org/10.1016/j.icte.2016.01.003
2405-9595/ c⃝ 2016 The Korean Institute of Communications Information Sciences. Production and Hosting by Elsevier B.V. This is an open access article under
the CC BY-NC-ND license (http://creativecommons.org/licenses/by-nc-nd/4.0/).
ScienceDirect
ICT Express 1 (2015) 132–137
www.elsevier.com/locate/icte
5G mobile technology: A survey✩
Rupendra Nath Mitra∗, Dharma P. Agrawal
Department of EECS, University of Cincinnati, OH, USA
Received 19 August 2015; received in revised form 19 December 2015; accepted 6 January 2016
Available online 22 January 2016
Abstract
All new 5G mobile technology is expected to be operational by 2020. This time, it is therefore crucial to know the direction of research and
developments enabling 5G technology. This paper provides an inclusive and comprehensive analysis of recent developmental endeavors toward
5G. It highlights salient features, i.e., flexibility, accessibility, and cloud-based service offerings, those are going to ensure the futuristic mobile
communication technology as the dominant protocol for global communication.
c⃝ 2016 The Korean Institute of Communications Information Sciences. Production and Hosting by Elsevier B.V. This is an open access article
under the CC BY-NC-ND license (http://creativecommons.org/licenses/by-nc-nd/4.0/).
Keywords: 5th Generation; Cloud based services; Energy-efficient; Key Performance Indices; Radio Access Network
1. Introduction
During last two decades, the world has witnessed rapid
evolution of cellular communication technologies from the
2G Global System for Mobile (GSM) to the 4G Long Term
Evolution-Advanced (LTE-A) system. The main motivation
has been the need of more bandwidth and lower latency.
While throughput is the actual data transfer rate, latency de-
pends largely on the processing speed of each node data
streams traverse through. Together with throughput-related
performance enhancements, some allied parameters, such as
jitter, inter channel interference, connectivity, scalability,
energy-efficiency, and compatibility with legacy networks, are
also taken into consideration when developing new mobile
technology.
When technology advanced from the 2G GSM to the 3G
Universal Mobile Telecommunication System (UMTS), higher
network speed and faster download speed allowed real-time
video calls. LTE and the subsequent LTE-A offered enhanced
network capacity and reduced delay in application–server ac-
cess, making triple-play traffic (data, voice, and video) access
∗Corresponding author.
E-mail addresses: mitrarh@mail.uc.edu (R.N. Mitra),
agrawadp@ucmail.uc.edu (D.P. Agrawal).
Peer review under responsibility of The Korean Institute of Communica-
tions Information Sciences.
✩ This paper is part of a special issue entitled “Next Generation (5G/6G)
Mobile Communications” guest-edited by Prof. Jungwoo Lee, Dr. Sumei Sun,
Prof. Huaping Liu, Prof. Seong-Lyun Kim and Prof. Wan Choi.
possible wirelessly, anytime anywhere. 4G truly constitutes mo-
bile broadband. Although 3G was the first mobile broadband
standard, it was originally designed for voice with some mul-
timedia and data consideration, whereas 2G was intended as
the first digital mobile voice communication standard for im-
proved coverage. The data rate has improved from 64 kbps in
2G to 2 Mbps in 3G and 50–100 Mbps in 4G. 5G is expected
to enhance not only the data transfer speed of mobile networks
but also the scalability, connectivity, and energy efficiency of
the network. It is assumed that by 2020, 50 billion devices will
be connected to the global IP network, which would appear to
present a challenge [1]. In a true “networked society” remote
controlled operation of appliances and critical commercial ma-
chines over a reliable 5G network will be possible with zero de-
lay. Real-time control of machines by using mobile devices will
be possible, making the Internet of things (IoT) more available
to all. Finally, but not least important, less energy-hungry net-
work nodes will be required toward a greener world. Therefore,
the following are the most important elements in the description
of 5G: high throughput, low-latency, high reliability, increased
scalability, and energy efficient mobile communication technol-
ogy.
In this paper, we present a comprehensive study of several
approaches for the envisaged 5G technology. The rest of the
paper is organized as follows. In Section 2, we describe a few
scenarios that 5G will have to satisfy. In Section 3, we introduce
a few eminent research groups working on the 5G standard.
Section 4 contains literature reviews from different aspects of
http://dx.doi.org/10.1016/j.icte.2016.01.003
2405-9595/ c⃝ 2016 The Korean Institute of Communications Information Sciences. Production and Hosting by Elsevier B.V. This is an open access article under
the CC BY-NC-ND license (http://creativecommons.org/licenses/by-nc-nd/4.0/).
Secure Best Marks with AI Grader
Need help grading? Try our AI Grader for instant feedback on your assignments.
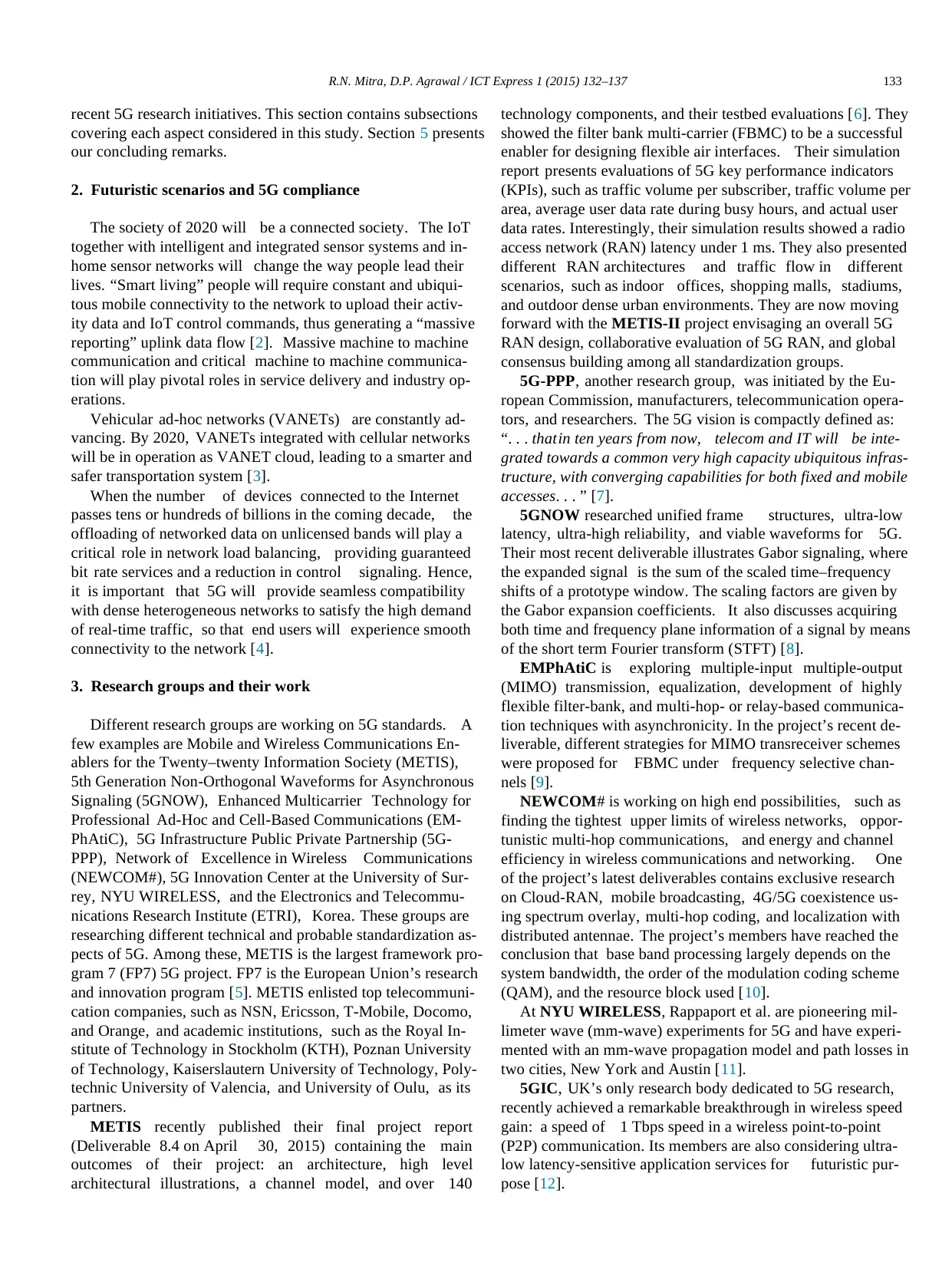
R.N. Mitra, D.P. Agrawal / ICT Express 1 (2015) 132–137 133
recent 5G research initiatives. This section contains subsections
covering each aspect considered in this study. Section 5 presents
our concluding remarks.
2. Futuristic scenarios and 5G compliance
The society of 2020 will be a connected society. The IoT
together with intelligent and integrated sensor systems and in-
home sensor networks will change the way people lead their
lives. “Smart living” people will require constant and ubiqui-
tous mobile connectivity to the network to upload their activ-
ity data and IoT control commands, thus generating a “massive
reporting” uplink data flow [2]. Massive machine to machine
communication and critical machine to machine communica-
tion will play pivotal roles in service delivery and industry op-
erations.
Vehicular ad-hoc networks (VANETs) are constantly ad-
vancing. By 2020, VANETs integrated with cellular networks
will be in operation as VANET cloud, leading to a smarter and
safer transportation system [3].
When the number of devices connected to the Internet
passes tens or hundreds of billions in the coming decade, the
offloading of networked data on unlicensed bands will play a
critical role in network load balancing, providing guaranteed
bit rate services and a reduction in control signaling. Hence,
it is important that 5G will provide seamless compatibility
with dense heterogeneous networks to satisfy the high demand
of real-time traffic, so that end users will experience smooth
connectivity to the network [4].
3. Research groups and their work
Different research groups are working on 5G standards. A
few examples are Mobile and Wireless Communications En-
ablers for the Twenty–twenty Information Society (METIS),
5th Generation Non-Orthogonal Waveforms for Asynchronous
Signaling (5GNOW), Enhanced Multicarrier Technology for
Professional Ad-Hoc and Cell-Based Communications (EM-
PhAtiC), 5G Infrastructure Public Private Partnership (5G-
PPP), Network of Excellence in Wireless Communications
(NEWCOM#), 5G Innovation Center at the University of Sur-
rey, NYU WIRELESS, and the Electronics and Telecommu-
nications Research Institute (ETRI), Korea. These groups are
researching different technical and probable standardization as-
pects of 5G. Among these, METIS is the largest framework pro-
gram 7 (FP7) 5G project. FP7 is the European Union’s research
and innovation program [5]. METIS enlisted top telecommuni-
cation companies, such as NSN, Ericsson, T-Mobile, Docomo,
and Orange, and academic institutions, such as the Royal In-
stitute of Technology in Stockholm (KTH), Poznan University
of Technology, Kaiserslautern University of Technology, Poly-
technic University of Valencia, and University of Oulu, as its
partners.
METIS recently published their final project report
(Deliverable 8.4 on April 30, 2015) containing the main
outcomes of their project: an architecture, high level
architectural illustrations, a channel model, and over 140
technology components, and their testbed evaluations [6]. They
showed the filter bank multi-carrier (FBMC) to be a successful
enabler for designing flexible air interfaces. Their simulation
report presents evaluations of 5G key performance indicators
(KPIs), such as traffic volume per subscriber, traffic volume per
area, average user data rate during busy hours, and actual user
data rates. Interestingly, their simulation results showed a radio
access network (RAN) latency under 1 ms. They also presented
different RAN architectures and traffic flow in different
scenarios, such as indoor offices, shopping malls, stadiums,
and outdoor dense urban environments. They are now moving
forward with the METIS-II project envisaging an overall 5G
RAN design, collaborative evaluation of 5G RAN, and global
consensus building among all standardization groups.
5G-PPP, another research group, was initiated by the Eu-
ropean Commission, manufacturers, telecommunication opera-
tors, and researchers. The 5G vision is compactly defined as:
“. . . thatin ten years from now, telecom and IT will be inte-
grated towards a common very high capacity ubiquitous infras-
tructure, with converging capabilities for both fixed and mobile
accesses. . . ” [7].
5GNOW researched unified frame structures, ultra-low
latency, ultra-high reliability, and viable waveforms for 5G.
Their most recent deliverable illustrates Gabor signaling, where
the expanded signal is the sum of the scaled time–frequency
shifts of a prototype window. The scaling factors are given by
the Gabor expansion coefficients. It also discusses acquiring
both time and frequency plane information of a signal by means
of the short term Fourier transform (STFT) [8].
EMPhAtiC is exploring multiple-input multiple-output
(MIMO) transmission, equalization, development of highly
flexible filter-bank, and multi-hop- or relay-based communica-
tion techniques with asynchronicity. In the project’s recent de-
liverable, different strategies for MIMO transreceiver schemes
were proposed for FBMC under frequency selective chan-
nels [9].
NEWCOM# is working on high end possibilities, such as
finding the tightest upper limits of wireless networks, oppor-
tunistic multi-hop communications, and energy and channel
efficiency in wireless communications and networking. One
of the project’s latest deliverables contains exclusive research
on Cloud-RAN, mobile broadcasting, 4G/5G coexistence us-
ing spectrum overlay, multi-hop coding, and localization with
distributed antennae. The project’s members have reached the
conclusion that base band processing largely depends on the
system bandwidth, the order of the modulation coding scheme
(QAM), and the resource block used [10].
At NYU WIRELESS, Rappaport et al. are pioneering mil-
limeter wave (mm-wave) experiments for 5G and have experi-
mented with an mm-wave propagation model and path losses in
two cities, New York and Austin [11].
5GIC, UK’s only research body dedicated to 5G research,
recently achieved a remarkable breakthrough in wireless speed
gain: a speed of 1 Tbps speed in a wireless point-to-point
(P2P) communication. Its members are also considering ultra-
low latency-sensitive application services for futuristic pur-
pose [12].
recent 5G research initiatives. This section contains subsections
covering each aspect considered in this study. Section 5 presents
our concluding remarks.
2. Futuristic scenarios and 5G compliance
The society of 2020 will be a connected society. The IoT
together with intelligent and integrated sensor systems and in-
home sensor networks will change the way people lead their
lives. “Smart living” people will require constant and ubiqui-
tous mobile connectivity to the network to upload their activ-
ity data and IoT control commands, thus generating a “massive
reporting” uplink data flow [2]. Massive machine to machine
communication and critical machine to machine communica-
tion will play pivotal roles in service delivery and industry op-
erations.
Vehicular ad-hoc networks (VANETs) are constantly ad-
vancing. By 2020, VANETs integrated with cellular networks
will be in operation as VANET cloud, leading to a smarter and
safer transportation system [3].
When the number of devices connected to the Internet
passes tens or hundreds of billions in the coming decade, the
offloading of networked data on unlicensed bands will play a
critical role in network load balancing, providing guaranteed
bit rate services and a reduction in control signaling. Hence,
it is important that 5G will provide seamless compatibility
with dense heterogeneous networks to satisfy the high demand
of real-time traffic, so that end users will experience smooth
connectivity to the network [4].
3. Research groups and their work
Different research groups are working on 5G standards. A
few examples are Mobile and Wireless Communications En-
ablers for the Twenty–twenty Information Society (METIS),
5th Generation Non-Orthogonal Waveforms for Asynchronous
Signaling (5GNOW), Enhanced Multicarrier Technology for
Professional Ad-Hoc and Cell-Based Communications (EM-
PhAtiC), 5G Infrastructure Public Private Partnership (5G-
PPP), Network of Excellence in Wireless Communications
(NEWCOM#), 5G Innovation Center at the University of Sur-
rey, NYU WIRELESS, and the Electronics and Telecommu-
nications Research Institute (ETRI), Korea. These groups are
researching different technical and probable standardization as-
pects of 5G. Among these, METIS is the largest framework pro-
gram 7 (FP7) 5G project. FP7 is the European Union’s research
and innovation program [5]. METIS enlisted top telecommuni-
cation companies, such as NSN, Ericsson, T-Mobile, Docomo,
and Orange, and academic institutions, such as the Royal In-
stitute of Technology in Stockholm (KTH), Poznan University
of Technology, Kaiserslautern University of Technology, Poly-
technic University of Valencia, and University of Oulu, as its
partners.
METIS recently published their final project report
(Deliverable 8.4 on April 30, 2015) containing the main
outcomes of their project: an architecture, high level
architectural illustrations, a channel model, and over 140
technology components, and their testbed evaluations [6]. They
showed the filter bank multi-carrier (FBMC) to be a successful
enabler for designing flexible air interfaces. Their simulation
report presents evaluations of 5G key performance indicators
(KPIs), such as traffic volume per subscriber, traffic volume per
area, average user data rate during busy hours, and actual user
data rates. Interestingly, their simulation results showed a radio
access network (RAN) latency under 1 ms. They also presented
different RAN architectures and traffic flow in different
scenarios, such as indoor offices, shopping malls, stadiums,
and outdoor dense urban environments. They are now moving
forward with the METIS-II project envisaging an overall 5G
RAN design, collaborative evaluation of 5G RAN, and global
consensus building among all standardization groups.
5G-PPP, another research group, was initiated by the Eu-
ropean Commission, manufacturers, telecommunication opera-
tors, and researchers. The 5G vision is compactly defined as:
“. . . thatin ten years from now, telecom and IT will be inte-
grated towards a common very high capacity ubiquitous infras-
tructure, with converging capabilities for both fixed and mobile
accesses. . . ” [7].
5GNOW researched unified frame structures, ultra-low
latency, ultra-high reliability, and viable waveforms for 5G.
Their most recent deliverable illustrates Gabor signaling, where
the expanded signal is the sum of the scaled time–frequency
shifts of a prototype window. The scaling factors are given by
the Gabor expansion coefficients. It also discusses acquiring
both time and frequency plane information of a signal by means
of the short term Fourier transform (STFT) [8].
EMPhAtiC is exploring multiple-input multiple-output
(MIMO) transmission, equalization, development of highly
flexible filter-bank, and multi-hop- or relay-based communica-
tion techniques with asynchronicity. In the project’s recent de-
liverable, different strategies for MIMO transreceiver schemes
were proposed for FBMC under frequency selective chan-
nels [9].
NEWCOM# is working on high end possibilities, such as
finding the tightest upper limits of wireless networks, oppor-
tunistic multi-hop communications, and energy and channel
efficiency in wireless communications and networking. One
of the project’s latest deliverables contains exclusive research
on Cloud-RAN, mobile broadcasting, 4G/5G coexistence us-
ing spectrum overlay, multi-hop coding, and localization with
distributed antennae. The project’s members have reached the
conclusion that base band processing largely depends on the
system bandwidth, the order of the modulation coding scheme
(QAM), and the resource block used [10].
At NYU WIRELESS, Rappaport et al. are pioneering mil-
limeter wave (mm-wave) experiments for 5G and have experi-
mented with an mm-wave propagation model and path losses in
two cities, New York and Austin [11].
5GIC, UK’s only research body dedicated to 5G research,
recently achieved a remarkable breakthrough in wireless speed
gain: a speed of 1 Tbps speed in a wireless point-to-point
(P2P) communication. Its members are also considering ultra-
low latency-sensitive application services for futuristic pur-
pose [12].
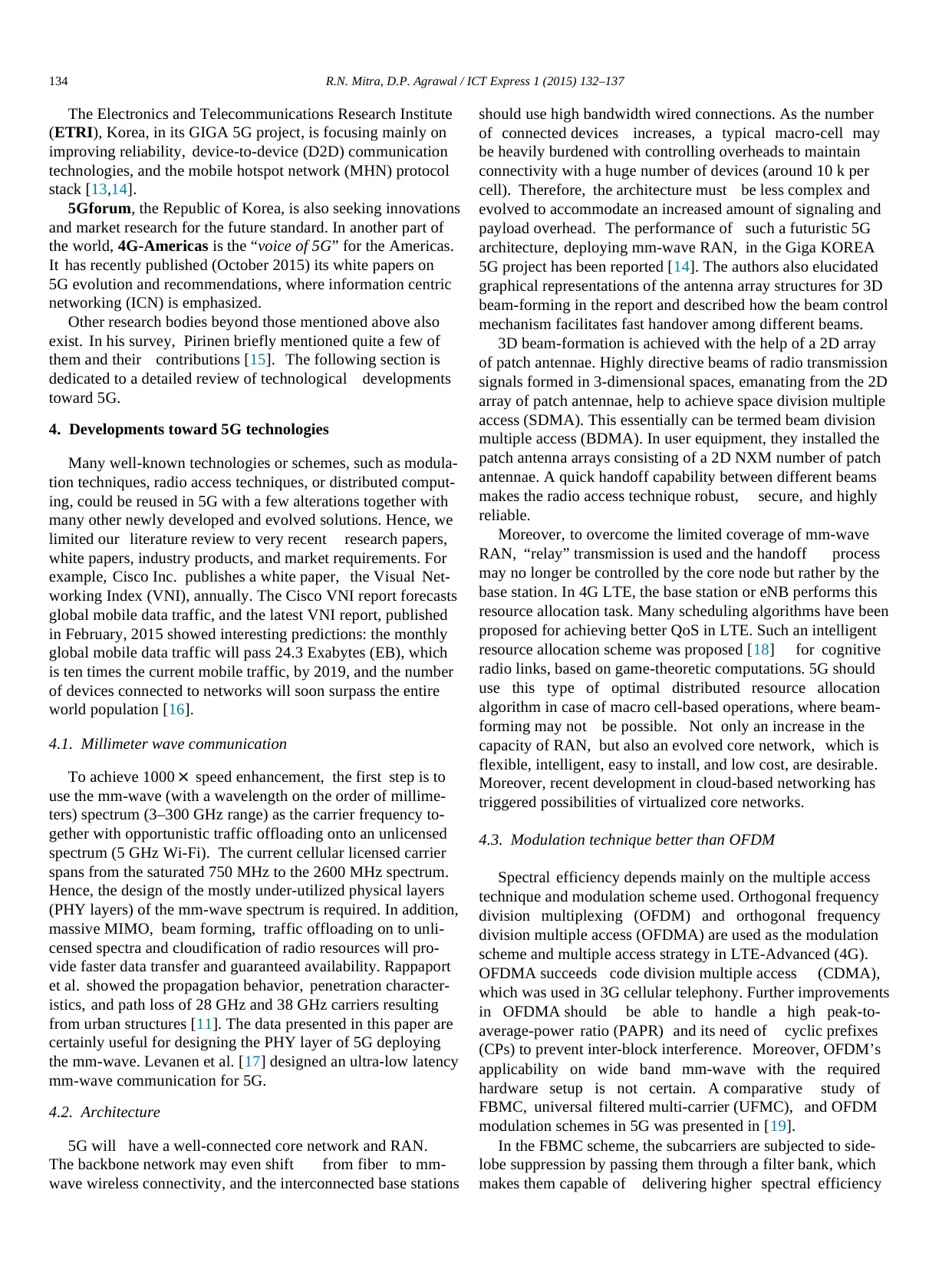
134 R.N. Mitra, D.P. Agrawal / ICT Express 1 (2015) 132–137
The Electronics and Telecommunications Research Institute
(ETRI), Korea, in its GIGA 5G project, is focusing mainly on
improving reliability, device-to-device (D2D) communication
technologies, and the mobile hotspot network (MHN) protocol
stack [13,14].
5Gforum, the Republic of Korea, is also seeking innovations
and market research for the future standard. In another part of
the world, 4G-Americas is the “voice of 5G” for the Americas.
It has recently published (October 2015) its white papers on
5G evolution and recommendations, where information centric
networking (ICN) is emphasized.
Other research bodies beyond those mentioned above also
exist. In his survey, Pirinen briefly mentioned quite a few of
them and their contributions [15]. The following section is
dedicated to a detailed review of technological developments
toward 5G.
4. Developments toward 5G technologies
Many well-known technologies or schemes, such as modula-
tion techniques, radio access techniques, or distributed comput-
ing, could be reused in 5G with a few alterations together with
many other newly developed and evolved solutions. Hence, we
limited our literature review to very recent research papers,
white papers, industry products, and market requirements. For
example, Cisco Inc. publishes a white paper, the Visual Net-
working Index (VNI), annually. The Cisco VNI report forecasts
global mobile data traffic, and the latest VNI report, published
in February, 2015 showed interesting predictions: the monthly
global mobile data traffic will pass 24.3 Exabytes (EB), which
is ten times the current mobile traffic, by 2019, and the number
of devices connected to networks will soon surpass the entire
world population [16].
4.1. Millimeter wave communication
To achieve 1000 × speed enhancement, the first step is to
use the mm-wave (with a wavelength on the order of millime-
ters) spectrum (3–300 GHz range) as the carrier frequency to-
gether with opportunistic traffic offloading onto an unlicensed
spectrum (5 GHz Wi-Fi). The current cellular licensed carrier
spans from the saturated 750 MHz to the 2600 MHz spectrum.
Hence, the design of the mostly under-utilized physical layers
(PHY layers) of the mm-wave spectrum is required. In addition,
massive MIMO, beam forming, traffic offloading on to unli-
censed spectra and cloudification of radio resources will pro-
vide faster data transfer and guaranteed availability. Rappaport
et al. showed the propagation behavior, penetration character-
istics, and path loss of 28 GHz and 38 GHz carriers resulting
from urban structures [11]. The data presented in this paper are
certainly useful for designing the PHY layer of 5G deploying
the mm-wave. Levanen et al. [17] designed an ultra-low latency
mm-wave communication for 5G.
4.2. Architecture
5G will have a well-connected core network and RAN.
The backbone network may even shift from fiber to mm-
wave wireless connectivity, and the interconnected base stations
should use high bandwidth wired connections. As the number
of connected devices increases, a typical macro-cell may
be heavily burdened with controlling overheads to maintain
connectivity with a huge number of devices (around 10 k per
cell). Therefore, the architecture must be less complex and
evolved to accommodate an increased amount of signaling and
payload overhead. The performance of such a futuristic 5G
architecture, deploying mm-wave RAN, in the Giga KOREA
5G project has been reported [14]. The authors also elucidated
graphical representations of the antenna array structures for 3D
beam-forming in the report and described how the beam control
mechanism facilitates fast handover among different beams.
3D beam-formation is achieved with the help of a 2D array
of patch antennae. Highly directive beams of radio transmission
signals formed in 3-dimensional spaces, emanating from the 2D
array of patch antennae, help to achieve space division multiple
access (SDMA). This essentially can be termed beam division
multiple access (BDMA). In user equipment, they installed the
patch antenna arrays consisting of a 2D NXM number of patch
antennae. A quick handoff capability between different beams
makes the radio access technique robust, secure, and highly
reliable.
Moreover, to overcome the limited coverage of mm-wave
RAN, “relay” transmission is used and the handoff process
may no longer be controlled by the core node but rather by the
base station. In 4G LTE, the base station or eNB performs this
resource allocation task. Many scheduling algorithms have been
proposed for achieving better QoS in LTE. Such an intelligent
resource allocation scheme was proposed [18] for cognitive
radio links, based on game-theoretic computations. 5G should
use this type of optimal distributed resource allocation
algorithm in case of macro cell-based operations, where beam-
forming may not be possible. Not only an increase in the
capacity of RAN, but also an evolved core network, which is
flexible, intelligent, easy to install, and low cost, are desirable.
Moreover, recent development in cloud-based networking has
triggered possibilities of virtualized core networks.
4.3. Modulation technique better than OFDM
Spectral efficiency depends mainly on the multiple access
technique and modulation scheme used. Orthogonal frequency
division multiplexing (OFDM) and orthogonal frequency
division multiple access (OFDMA) are used as the modulation
scheme and multiple access strategy in LTE-Advanced (4G).
OFDMA succeeds code division multiple access (CDMA),
which was used in 3G cellular telephony. Further improvements
in OFDMA should be able to handle a high peak-to-
average-power ratio (PAPR) and its need of cyclic prefixes
(CPs) to prevent inter-block interference. Moreover, OFDM’s
applicability on wide band mm-wave with the required
hardware setup is not certain. A comparative study of
FBMC, universal filtered multi-carrier (UFMC), and OFDM
modulation schemes in 5G was presented in [19].
In the FBMC scheme, the subcarriers are subjected to side-
lobe suppression by passing them through a filter bank, which
makes them capable of delivering higher spectral efficiency
The Electronics and Telecommunications Research Institute
(ETRI), Korea, in its GIGA 5G project, is focusing mainly on
improving reliability, device-to-device (D2D) communication
technologies, and the mobile hotspot network (MHN) protocol
stack [13,14].
5Gforum, the Republic of Korea, is also seeking innovations
and market research for the future standard. In another part of
the world, 4G-Americas is the “voice of 5G” for the Americas.
It has recently published (October 2015) its white papers on
5G evolution and recommendations, where information centric
networking (ICN) is emphasized.
Other research bodies beyond those mentioned above also
exist. In his survey, Pirinen briefly mentioned quite a few of
them and their contributions [15]. The following section is
dedicated to a detailed review of technological developments
toward 5G.
4. Developments toward 5G technologies
Many well-known technologies or schemes, such as modula-
tion techniques, radio access techniques, or distributed comput-
ing, could be reused in 5G with a few alterations together with
many other newly developed and evolved solutions. Hence, we
limited our literature review to very recent research papers,
white papers, industry products, and market requirements. For
example, Cisco Inc. publishes a white paper, the Visual Net-
working Index (VNI), annually. The Cisco VNI report forecasts
global mobile data traffic, and the latest VNI report, published
in February, 2015 showed interesting predictions: the monthly
global mobile data traffic will pass 24.3 Exabytes (EB), which
is ten times the current mobile traffic, by 2019, and the number
of devices connected to networks will soon surpass the entire
world population [16].
4.1. Millimeter wave communication
To achieve 1000 × speed enhancement, the first step is to
use the mm-wave (with a wavelength on the order of millime-
ters) spectrum (3–300 GHz range) as the carrier frequency to-
gether with opportunistic traffic offloading onto an unlicensed
spectrum (5 GHz Wi-Fi). The current cellular licensed carrier
spans from the saturated 750 MHz to the 2600 MHz spectrum.
Hence, the design of the mostly under-utilized physical layers
(PHY layers) of the mm-wave spectrum is required. In addition,
massive MIMO, beam forming, traffic offloading on to unli-
censed spectra and cloudification of radio resources will pro-
vide faster data transfer and guaranteed availability. Rappaport
et al. showed the propagation behavior, penetration character-
istics, and path loss of 28 GHz and 38 GHz carriers resulting
from urban structures [11]. The data presented in this paper are
certainly useful for designing the PHY layer of 5G deploying
the mm-wave. Levanen et al. [17] designed an ultra-low latency
mm-wave communication for 5G.
4.2. Architecture
5G will have a well-connected core network and RAN.
The backbone network may even shift from fiber to mm-
wave wireless connectivity, and the interconnected base stations
should use high bandwidth wired connections. As the number
of connected devices increases, a typical macro-cell may
be heavily burdened with controlling overheads to maintain
connectivity with a huge number of devices (around 10 k per
cell). Therefore, the architecture must be less complex and
evolved to accommodate an increased amount of signaling and
payload overhead. The performance of such a futuristic 5G
architecture, deploying mm-wave RAN, in the Giga KOREA
5G project has been reported [14]. The authors also elucidated
graphical representations of the antenna array structures for 3D
beam-forming in the report and described how the beam control
mechanism facilitates fast handover among different beams.
3D beam-formation is achieved with the help of a 2D array
of patch antennae. Highly directive beams of radio transmission
signals formed in 3-dimensional spaces, emanating from the 2D
array of patch antennae, help to achieve space division multiple
access (SDMA). This essentially can be termed beam division
multiple access (BDMA). In user equipment, they installed the
patch antenna arrays consisting of a 2D NXM number of patch
antennae. A quick handoff capability between different beams
makes the radio access technique robust, secure, and highly
reliable.
Moreover, to overcome the limited coverage of mm-wave
RAN, “relay” transmission is used and the handoff process
may no longer be controlled by the core node but rather by the
base station. In 4G LTE, the base station or eNB performs this
resource allocation task. Many scheduling algorithms have been
proposed for achieving better QoS in LTE. Such an intelligent
resource allocation scheme was proposed [18] for cognitive
radio links, based on game-theoretic computations. 5G should
use this type of optimal distributed resource allocation
algorithm in case of macro cell-based operations, where beam-
forming may not be possible. Not only an increase in the
capacity of RAN, but also an evolved core network, which is
flexible, intelligent, easy to install, and low cost, are desirable.
Moreover, recent development in cloud-based networking has
triggered possibilities of virtualized core networks.
4.3. Modulation technique better than OFDM
Spectral efficiency depends mainly on the multiple access
technique and modulation scheme used. Orthogonal frequency
division multiplexing (OFDM) and orthogonal frequency
division multiple access (OFDMA) are used as the modulation
scheme and multiple access strategy in LTE-Advanced (4G).
OFDMA succeeds code division multiple access (CDMA),
which was used in 3G cellular telephony. Further improvements
in OFDMA should be able to handle a high peak-to-
average-power ratio (PAPR) and its need of cyclic prefixes
(CPs) to prevent inter-block interference. Moreover, OFDM’s
applicability on wide band mm-wave with the required
hardware setup is not certain. A comparative study of
FBMC, universal filtered multi-carrier (UFMC), and OFDM
modulation schemes in 5G was presented in [19].
In the FBMC scheme, the subcarriers are subjected to side-
lobe suppression by passing them through a filter bank, which
makes them capable of delivering higher spectral efficiency
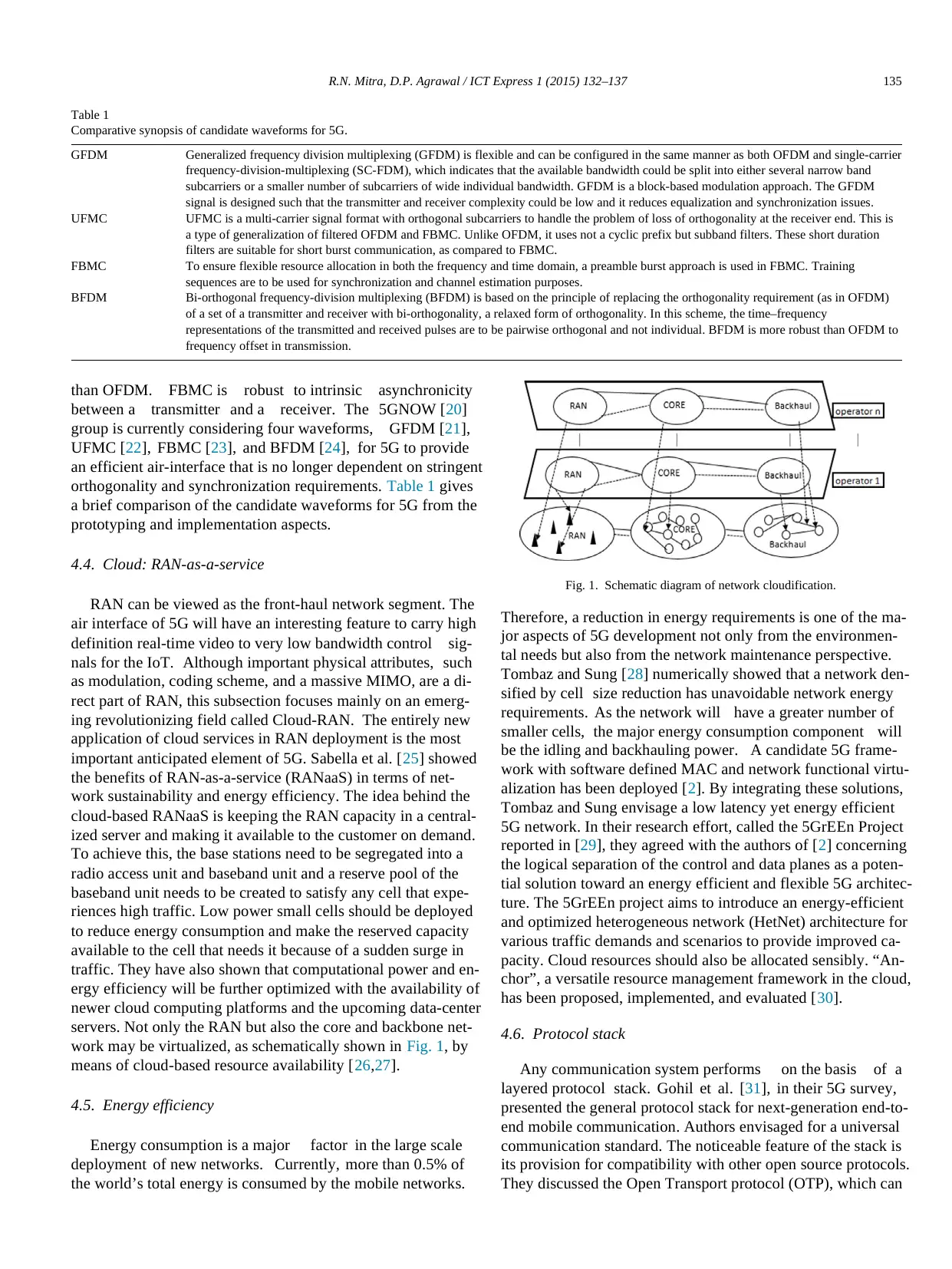
R.N. Mitra, D.P. Agrawal / ICT Express 1 (2015) 132–137 135
Table 1
Comparative synopsis of candidate waveforms for 5G.
GFDM Generalized frequency division multiplexing (GFDM) is flexible and can be configured in the same manner as both OFDM and single-carrier
frequency-division-multiplexing (SC-FDM), which indicates that the available bandwidth could be split into either several narrow band
subcarriers or a smaller number of subcarriers of wide individual bandwidth. GFDM is a block-based modulation approach. The GFDM
signal is designed such that the transmitter and receiver complexity could be low and it reduces equalization and synchronization issues.
UFMC UFMC is a multi-carrier signal format with orthogonal subcarriers to handle the problem of loss of orthogonality at the receiver end. This is
a type of generalization of filtered OFDM and FBMC. Unlike OFDM, it uses not a cyclic prefix but subband filters. These short duration
filters are suitable for short burst communication, as compared to FBMC.
FBMC To ensure flexible resource allocation in both the frequency and time domain, a preamble burst approach is used in FBMC. Training
sequences are to be used for synchronization and channel estimation purposes.
BFDM Bi-orthogonal frequency-division multiplexing (BFDM) is based on the principle of replacing the orthogonality requirement (as in OFDM)
of a set of a transmitter and receiver with bi-orthogonality, a relaxed form of orthogonality. In this scheme, the time–frequency
representations of the transmitted and received pulses are to be pairwise orthogonal and not individual. BFDM is more robust than OFDM to
frequency offset in transmission.
than OFDM. FBMC is robust to intrinsic asynchronicity
between a transmitter and a receiver. The 5GNOW [20]
group is currently considering four waveforms, GFDM [21],
UFMC [22], FBMC [23], and BFDM [24], for 5G to provide
an efficient air-interface that is no longer dependent on stringent
orthogonality and synchronization requirements. Table 1 gives
a brief comparison of the candidate waveforms for 5G from the
prototyping and implementation aspects.
4.4. Cloud: RAN-as-a-service
RAN can be viewed as the front-haul network segment. The
air interface of 5G will have an interesting feature to carry high
definition real-time video to very low bandwidth control sig-
nals for the IoT. Although important physical attributes, such
as modulation, coding scheme, and a massive MIMO, are a di-
rect part of RAN, this subsection focuses mainly on an emerg-
ing revolutionizing field called Cloud-RAN. The entirely new
application of cloud services in RAN deployment is the most
important anticipated element of 5G. Sabella et al. [25] showed
the benefits of RAN-as-a-service (RANaaS) in terms of net-
work sustainability and energy efficiency. The idea behind the
cloud-based RANaaS is keeping the RAN capacity in a central-
ized server and making it available to the customer on demand.
To achieve this, the base stations need to be segregated into a
radio access unit and baseband unit and a reserve pool of the
baseband unit needs to be created to satisfy any cell that expe-
riences high traffic. Low power small cells should be deployed
to reduce energy consumption and make the reserved capacity
available to the cell that needs it because of a sudden surge in
traffic. They have also shown that computational power and en-
ergy efficiency will be further optimized with the availability of
newer cloud computing platforms and the upcoming data-center
servers. Not only the RAN but also the core and backbone net-
work may be virtualized, as schematically shown in Fig. 1, by
means of cloud-based resource availability [26,27].
4.5. Energy efficiency
Energy consumption is a major factor in the large scale
deployment of new networks. Currently, more than 0.5% of
the world’s total energy is consumed by the mobile networks.
Fig. 1. Schematic diagram of network cloudification.
Therefore, a reduction in energy requirements is one of the ma-
jor aspects of 5G development not only from the environmen-
tal needs but also from the network maintenance perspective.
Tombaz and Sung [28] numerically showed that a network den-
sified by cell size reduction has unavoidable network energy
requirements. As the network will have a greater number of
smaller cells, the major energy consumption component will
be the idling and backhauling power. A candidate 5G frame-
work with software defined MAC and network functional virtu-
alization has been deployed [2]. By integrating these solutions,
Tombaz and Sung envisage a low latency yet energy efficient
5G network. In their research effort, called the 5GrEEn Project
reported in [29], they agreed with the authors of [2] concerning
the logical separation of the control and data planes as a poten-
tial solution toward an energy efficient and flexible 5G architec-
ture. The 5GrEEn project aims to introduce an energy-efficient
and optimized heterogeneous network (HetNet) architecture for
various traffic demands and scenarios to provide improved ca-
pacity. Cloud resources should also be allocated sensibly. “An-
chor”, a versatile resource management framework in the cloud,
has been proposed, implemented, and evaluated [30].
4.6. Protocol stack
Any communication system performs on the basis of a
layered protocol stack. Gohil et al. [31], in their 5G survey,
presented the general protocol stack for next-generation end-to-
end mobile communication. Authors envisaged for a universal
communication standard. The noticeable feature of the stack is
its provision for compatibility with other open source protocols.
They discussed the Open Transport protocol (OTP), which can
Table 1
Comparative synopsis of candidate waveforms for 5G.
GFDM Generalized frequency division multiplexing (GFDM) is flexible and can be configured in the same manner as both OFDM and single-carrier
frequency-division-multiplexing (SC-FDM), which indicates that the available bandwidth could be split into either several narrow band
subcarriers or a smaller number of subcarriers of wide individual bandwidth. GFDM is a block-based modulation approach. The GFDM
signal is designed such that the transmitter and receiver complexity could be low and it reduces equalization and synchronization issues.
UFMC UFMC is a multi-carrier signal format with orthogonal subcarriers to handle the problem of loss of orthogonality at the receiver end. This is
a type of generalization of filtered OFDM and FBMC. Unlike OFDM, it uses not a cyclic prefix but subband filters. These short duration
filters are suitable for short burst communication, as compared to FBMC.
FBMC To ensure flexible resource allocation in both the frequency and time domain, a preamble burst approach is used in FBMC. Training
sequences are to be used for synchronization and channel estimation purposes.
BFDM Bi-orthogonal frequency-division multiplexing (BFDM) is based on the principle of replacing the orthogonality requirement (as in OFDM)
of a set of a transmitter and receiver with bi-orthogonality, a relaxed form of orthogonality. In this scheme, the time–frequency
representations of the transmitted and received pulses are to be pairwise orthogonal and not individual. BFDM is more robust than OFDM to
frequency offset in transmission.
than OFDM. FBMC is robust to intrinsic asynchronicity
between a transmitter and a receiver. The 5GNOW [20]
group is currently considering four waveforms, GFDM [21],
UFMC [22], FBMC [23], and BFDM [24], for 5G to provide
an efficient air-interface that is no longer dependent on stringent
orthogonality and synchronization requirements. Table 1 gives
a brief comparison of the candidate waveforms for 5G from the
prototyping and implementation aspects.
4.4. Cloud: RAN-as-a-service
RAN can be viewed as the front-haul network segment. The
air interface of 5G will have an interesting feature to carry high
definition real-time video to very low bandwidth control sig-
nals for the IoT. Although important physical attributes, such
as modulation, coding scheme, and a massive MIMO, are a di-
rect part of RAN, this subsection focuses mainly on an emerg-
ing revolutionizing field called Cloud-RAN. The entirely new
application of cloud services in RAN deployment is the most
important anticipated element of 5G. Sabella et al. [25] showed
the benefits of RAN-as-a-service (RANaaS) in terms of net-
work sustainability and energy efficiency. The idea behind the
cloud-based RANaaS is keeping the RAN capacity in a central-
ized server and making it available to the customer on demand.
To achieve this, the base stations need to be segregated into a
radio access unit and baseband unit and a reserve pool of the
baseband unit needs to be created to satisfy any cell that expe-
riences high traffic. Low power small cells should be deployed
to reduce energy consumption and make the reserved capacity
available to the cell that needs it because of a sudden surge in
traffic. They have also shown that computational power and en-
ergy efficiency will be further optimized with the availability of
newer cloud computing platforms and the upcoming data-center
servers. Not only the RAN but also the core and backbone net-
work may be virtualized, as schematically shown in Fig. 1, by
means of cloud-based resource availability [26,27].
4.5. Energy efficiency
Energy consumption is a major factor in the large scale
deployment of new networks. Currently, more than 0.5% of
the world’s total energy is consumed by the mobile networks.
Fig. 1. Schematic diagram of network cloudification.
Therefore, a reduction in energy requirements is one of the ma-
jor aspects of 5G development not only from the environmen-
tal needs but also from the network maintenance perspective.
Tombaz and Sung [28] numerically showed that a network den-
sified by cell size reduction has unavoidable network energy
requirements. As the network will have a greater number of
smaller cells, the major energy consumption component will
be the idling and backhauling power. A candidate 5G frame-
work with software defined MAC and network functional virtu-
alization has been deployed [2]. By integrating these solutions,
Tombaz and Sung envisage a low latency yet energy efficient
5G network. In their research effort, called the 5GrEEn Project
reported in [29], they agreed with the authors of [2] concerning
the logical separation of the control and data planes as a poten-
tial solution toward an energy efficient and flexible 5G architec-
ture. The 5GrEEn project aims to introduce an energy-efficient
and optimized heterogeneous network (HetNet) architecture for
various traffic demands and scenarios to provide improved ca-
pacity. Cloud resources should also be allocated sensibly. “An-
chor”, a versatile resource management framework in the cloud,
has been proposed, implemented, and evaluated [30].
4.6. Protocol stack
Any communication system performs on the basis of a
layered protocol stack. Gohil et al. [31], in their 5G survey,
presented the general protocol stack for next-generation end-to-
end mobile communication. Authors envisaged for a universal
communication standard. The noticeable feature of the stack is
its provision for compatibility with other open source protocols.
They discussed the Open Transport protocol (OTP), which can
Paraphrase This Document
Need a fresh take? Get an instant paraphrase of this document with our AI Paraphraser
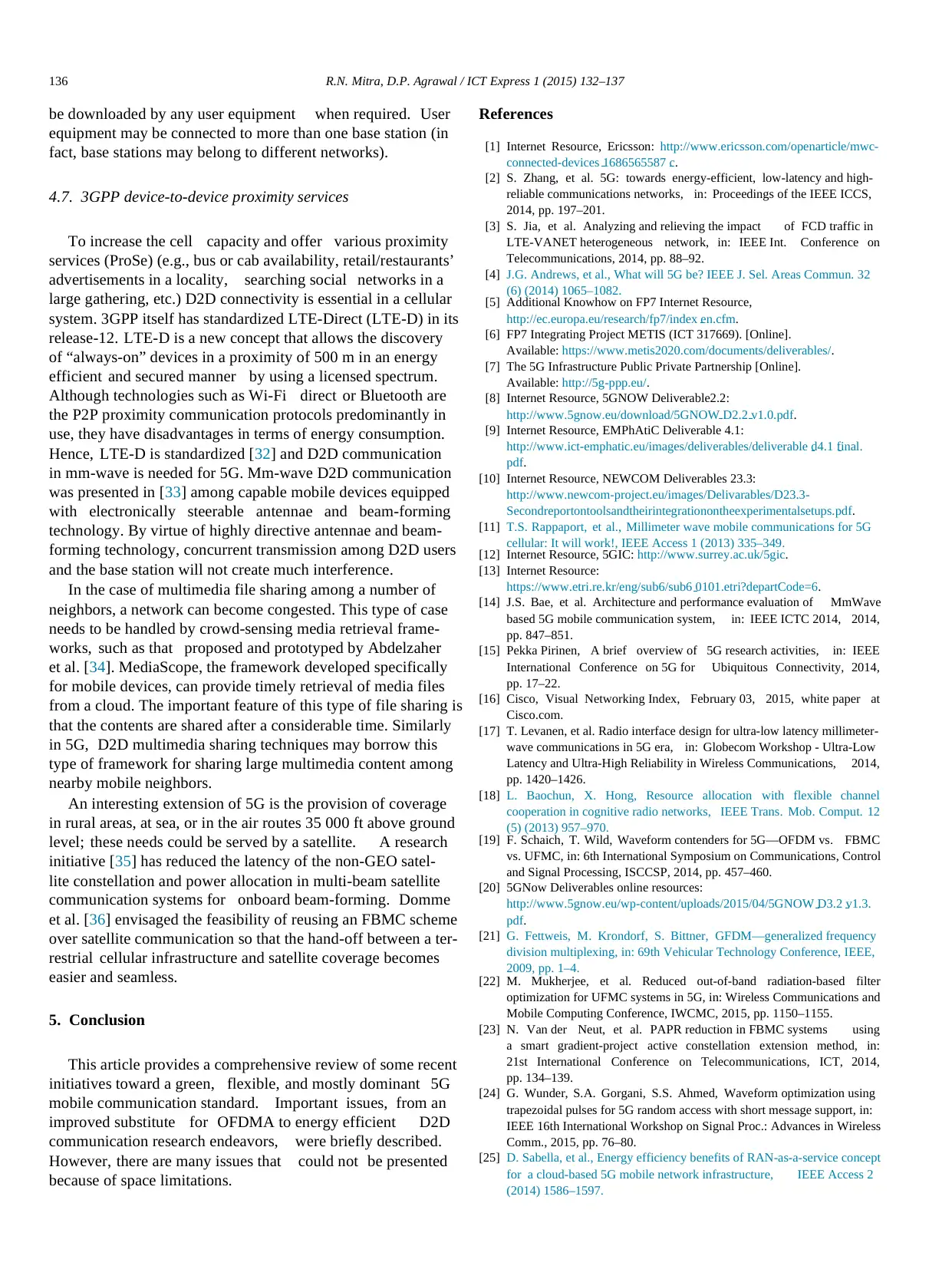
136 R.N. Mitra, D.P. Agrawal / ICT Express 1 (2015) 132–137
be downloaded by any user equipment when required. User
equipment may be connected to more than one base station (in
fact, base stations may belong to different networks).
4.7. 3GPP device-to-device proximity services
To increase the cell capacity and offer various proximity
services (ProSe) (e.g., bus or cab availability, retail/restaurants’
advertisements in a locality, searching social networks in a
large gathering, etc.) D2D connectivity is essential in a cellular
system. 3GPP itself has standardized LTE-Direct (LTE-D) in its
release-12. LTE-D is a new concept that allows the discovery
of “always-on” devices in a proximity of 500 m in an energy
efficient and secured manner by using a licensed spectrum.
Although technologies such as Wi-Fi direct or Bluetooth are
the P2P proximity communication protocols predominantly in
use, they have disadvantages in terms of energy consumption.
Hence, LTE-D is standardized [32] and D2D communication
in mm-wave is needed for 5G. Mm-wave D2D communication
was presented in [33] among capable mobile devices equipped
with electronically steerable antennae and beam-forming
technology. By virtue of highly directive antennae and beam-
forming technology, concurrent transmission among D2D users
and the base station will not create much interference.
In the case of multimedia file sharing among a number of
neighbors, a network can become congested. This type of case
needs to be handled by crowd-sensing media retrieval frame-
works, such as that proposed and prototyped by Abdelzaher
et al. [34]. MediaScope, the framework developed specifically
for mobile devices, can provide timely retrieval of media files
from a cloud. The important feature of this type of file sharing is
that the contents are shared after a considerable time. Similarly
in 5G, D2D multimedia sharing techniques may borrow this
type of framework for sharing large multimedia content among
nearby mobile neighbors.
An interesting extension of 5G is the provision of coverage
in rural areas, at sea, or in the air routes 35 000 ft above ground
level; these needs could be served by a satellite. A research
initiative [35] has reduced the latency of the non-GEO satel-
lite constellation and power allocation in multi-beam satellite
communication systems for onboard beam-forming. Domme
et al. [36] envisaged the feasibility of reusing an FBMC scheme
over satellite communication so that the hand-off between a ter-
restrial cellular infrastructure and satellite coverage becomes
easier and seamless.
5. Conclusion
This article provides a comprehensive review of some recent
initiatives toward a green, flexible, and mostly dominant 5G
mobile communication standard. Important issues, from an
improved substitute for OFDMA to energy efficient D2D
communication research endeavors, were briefly described.
However, there are many issues that could not be presented
because of space limitations.
References
[1] Internet Resource, Ericsson: http://www.ericsson.com/openarticle/mwc-
connected-devices 1686565587 c.
[2] S. Zhang, et al. 5G: towards energy-efficient, low-latency and high-
reliable communications networks, in: Proceedings of the IEEE ICCS,
2014, pp. 197–201.
[3] S. Jia, et al. Analyzing and relieving the impact of FCD traffic in
LTE-VANET heterogeneous network, in: IEEE Int. Conference on
Telecommunications, 2014, pp. 88–92.
[4] J.G. Andrews, et al., What will 5G be? IEEE J. Sel. Areas Commun. 32
(6) (2014) 1065–1082.
[5] Additional Knowhow on FP7 Internet Resource,
http://ec.europa.eu/research/fp7/index en.cfm.
[6] FP7 Integrating Project METIS (ICT 317669). [Online].
Available: https://www.metis2020.com/documents/deliverables/.
[7] The 5G Infrastructure Public Private Partnership [Online].
Available: http://5g-ppp.eu/.
[8] Internet Resource, 5GNOW Deliverable2.2:
http://www.5gnow.eu/download/5GNOW D2.2 v1.0.pdf.
[9] Internet Resource, EMPhAtiC Deliverable 4.1:
http://www.ict-emphatic.eu/images/deliverables/deliverable d4.1 final.
pdf.
[10] Internet Resource, NEWCOM Deliverables 23.3:
http://www.newcom-project.eu/images/Delivarables/D23.3-
Secondreportontoolsandtheirintegrationontheexperimentalsetups.pdf.
[11] T.S. Rappaport, et al., Millimeter wave mobile communications for 5G
cellular: It will work!, IEEE Access 1 (2013) 335–349.
[12] Internet Resource, 5GIC: http://www.surrey.ac.uk/5gic.
[13] Internet Resource:
https://www.etri.re.kr/eng/sub6/sub6 0101.etri?departCode=6.
[14] J.S. Bae, et al. Architecture and performance evaluation of MmWave
based 5G mobile communication system, in: IEEE ICTC 2014, 2014,
pp. 847–851.
[15] Pekka Pirinen, A brief overview of 5G research activities, in: IEEE
International Conference on 5G for Ubiquitous Connectivity, 2014,
pp. 17–22.
[16] Cisco, Visual Networking Index, February 03, 2015, white paper at
Cisco.com.
[17] T. Levanen, et al. Radio interface design for ultra-low latency millimeter-
wave communications in 5G era, in: Globecom Workshop - Ultra-Low
Latency and Ultra-High Reliability in Wireless Communications, 2014,
pp. 1420–1426.
[18] L. Baochun, X. Hong, Resource allocation with flexible channel
cooperation in cognitive radio networks, IEEE Trans. Mob. Comput. 12
(5) (2013) 957–970.
[19] F. Schaich, T. Wild, Waveform contenders for 5G—OFDM vs. FBMC
vs. UFMC, in: 6th International Symposium on Communications, Control
and Signal Processing, ISCCSP, 2014, pp. 457–460.
[20] 5GNow Deliverables online resources:
http://www.5gnow.eu/wp-content/uploads/2015/04/5GNOW D3.2 v1.3.
pdf.
[21] G. Fettweis, M. Krondorf, S. Bittner, GFDM—generalized frequency
division multiplexing, in: 69th Vehicular Technology Conference, IEEE,
2009, pp. 1–4.
[22] M. Mukherjee, et al. Reduced out-of-band radiation-based filter
optimization for UFMC systems in 5G, in: Wireless Communications and
Mobile Computing Conference, IWCMC, 2015, pp. 1150–1155.
[23] N. Van der Neut, et al. PAPR reduction in FBMC systems using
a smart gradient-project active constellation extension method, in:
21st International Conference on Telecommunications, ICT, 2014,
pp. 134–139.
[24] G. Wunder, S.A. Gorgani, S.S. Ahmed, Waveform optimization using
trapezoidal pulses for 5G random access with short message support, in:
IEEE 16th International Workshop on Signal Proc.: Advances in Wireless
Comm., 2015, pp. 76–80.
[25] D. Sabella, et al., Energy efficiency benefits of RAN-as-a-service concept
for a cloud-based 5G mobile network infrastructure, IEEE Access 2
(2014) 1586–1597.
be downloaded by any user equipment when required. User
equipment may be connected to more than one base station (in
fact, base stations may belong to different networks).
4.7. 3GPP device-to-device proximity services
To increase the cell capacity and offer various proximity
services (ProSe) (e.g., bus or cab availability, retail/restaurants’
advertisements in a locality, searching social networks in a
large gathering, etc.) D2D connectivity is essential in a cellular
system. 3GPP itself has standardized LTE-Direct (LTE-D) in its
release-12. LTE-D is a new concept that allows the discovery
of “always-on” devices in a proximity of 500 m in an energy
efficient and secured manner by using a licensed spectrum.
Although technologies such as Wi-Fi direct or Bluetooth are
the P2P proximity communication protocols predominantly in
use, they have disadvantages in terms of energy consumption.
Hence, LTE-D is standardized [32] and D2D communication
in mm-wave is needed for 5G. Mm-wave D2D communication
was presented in [33] among capable mobile devices equipped
with electronically steerable antennae and beam-forming
technology. By virtue of highly directive antennae and beam-
forming technology, concurrent transmission among D2D users
and the base station will not create much interference.
In the case of multimedia file sharing among a number of
neighbors, a network can become congested. This type of case
needs to be handled by crowd-sensing media retrieval frame-
works, such as that proposed and prototyped by Abdelzaher
et al. [34]. MediaScope, the framework developed specifically
for mobile devices, can provide timely retrieval of media files
from a cloud. The important feature of this type of file sharing is
that the contents are shared after a considerable time. Similarly
in 5G, D2D multimedia sharing techniques may borrow this
type of framework for sharing large multimedia content among
nearby mobile neighbors.
An interesting extension of 5G is the provision of coverage
in rural areas, at sea, or in the air routes 35 000 ft above ground
level; these needs could be served by a satellite. A research
initiative [35] has reduced the latency of the non-GEO satel-
lite constellation and power allocation in multi-beam satellite
communication systems for onboard beam-forming. Domme
et al. [36] envisaged the feasibility of reusing an FBMC scheme
over satellite communication so that the hand-off between a ter-
restrial cellular infrastructure and satellite coverage becomes
easier and seamless.
5. Conclusion
This article provides a comprehensive review of some recent
initiatives toward a green, flexible, and mostly dominant 5G
mobile communication standard. Important issues, from an
improved substitute for OFDMA to energy efficient D2D
communication research endeavors, were briefly described.
However, there are many issues that could not be presented
because of space limitations.
References
[1] Internet Resource, Ericsson: http://www.ericsson.com/openarticle/mwc-
connected-devices 1686565587 c.
[2] S. Zhang, et al. 5G: towards energy-efficient, low-latency and high-
reliable communications networks, in: Proceedings of the IEEE ICCS,
2014, pp. 197–201.
[3] S. Jia, et al. Analyzing and relieving the impact of FCD traffic in
LTE-VANET heterogeneous network, in: IEEE Int. Conference on
Telecommunications, 2014, pp. 88–92.
[4] J.G. Andrews, et al., What will 5G be? IEEE J. Sel. Areas Commun. 32
(6) (2014) 1065–1082.
[5] Additional Knowhow on FP7 Internet Resource,
http://ec.europa.eu/research/fp7/index en.cfm.
[6] FP7 Integrating Project METIS (ICT 317669). [Online].
Available: https://www.metis2020.com/documents/deliverables/.
[7] The 5G Infrastructure Public Private Partnership [Online].
Available: http://5g-ppp.eu/.
[8] Internet Resource, 5GNOW Deliverable2.2:
http://www.5gnow.eu/download/5GNOW D2.2 v1.0.pdf.
[9] Internet Resource, EMPhAtiC Deliverable 4.1:
http://www.ict-emphatic.eu/images/deliverables/deliverable d4.1 final.
pdf.
[10] Internet Resource, NEWCOM Deliverables 23.3:
http://www.newcom-project.eu/images/Delivarables/D23.3-
Secondreportontoolsandtheirintegrationontheexperimentalsetups.pdf.
[11] T.S. Rappaport, et al., Millimeter wave mobile communications for 5G
cellular: It will work!, IEEE Access 1 (2013) 335–349.
[12] Internet Resource, 5GIC: http://www.surrey.ac.uk/5gic.
[13] Internet Resource:
https://www.etri.re.kr/eng/sub6/sub6 0101.etri?departCode=6.
[14] J.S. Bae, et al. Architecture and performance evaluation of MmWave
based 5G mobile communication system, in: IEEE ICTC 2014, 2014,
pp. 847–851.
[15] Pekka Pirinen, A brief overview of 5G research activities, in: IEEE
International Conference on 5G for Ubiquitous Connectivity, 2014,
pp. 17–22.
[16] Cisco, Visual Networking Index, February 03, 2015, white paper at
Cisco.com.
[17] T. Levanen, et al. Radio interface design for ultra-low latency millimeter-
wave communications in 5G era, in: Globecom Workshop - Ultra-Low
Latency and Ultra-High Reliability in Wireless Communications, 2014,
pp. 1420–1426.
[18] L. Baochun, X. Hong, Resource allocation with flexible channel
cooperation in cognitive radio networks, IEEE Trans. Mob. Comput. 12
(5) (2013) 957–970.
[19] F. Schaich, T. Wild, Waveform contenders for 5G—OFDM vs. FBMC
vs. UFMC, in: 6th International Symposium on Communications, Control
and Signal Processing, ISCCSP, 2014, pp. 457–460.
[20] 5GNow Deliverables online resources:
http://www.5gnow.eu/wp-content/uploads/2015/04/5GNOW D3.2 v1.3.
pdf.
[21] G. Fettweis, M. Krondorf, S. Bittner, GFDM—generalized frequency
division multiplexing, in: 69th Vehicular Technology Conference, IEEE,
2009, pp. 1–4.
[22] M. Mukherjee, et al. Reduced out-of-band radiation-based filter
optimization for UFMC systems in 5G, in: Wireless Communications and
Mobile Computing Conference, IWCMC, 2015, pp. 1150–1155.
[23] N. Van der Neut, et al. PAPR reduction in FBMC systems using
a smart gradient-project active constellation extension method, in:
21st International Conference on Telecommunications, ICT, 2014,
pp. 134–139.
[24] G. Wunder, S.A. Gorgani, S.S. Ahmed, Waveform optimization using
trapezoidal pulses for 5G random access with short message support, in:
IEEE 16th International Workshop on Signal Proc.: Advances in Wireless
Comm., 2015, pp. 76–80.
[25] D. Sabella, et al., Energy efficiency benefits of RAN-as-a-service concept
for a cloud-based 5G mobile network infrastructure, IEEE Access 2
(2014) 1586–1597.
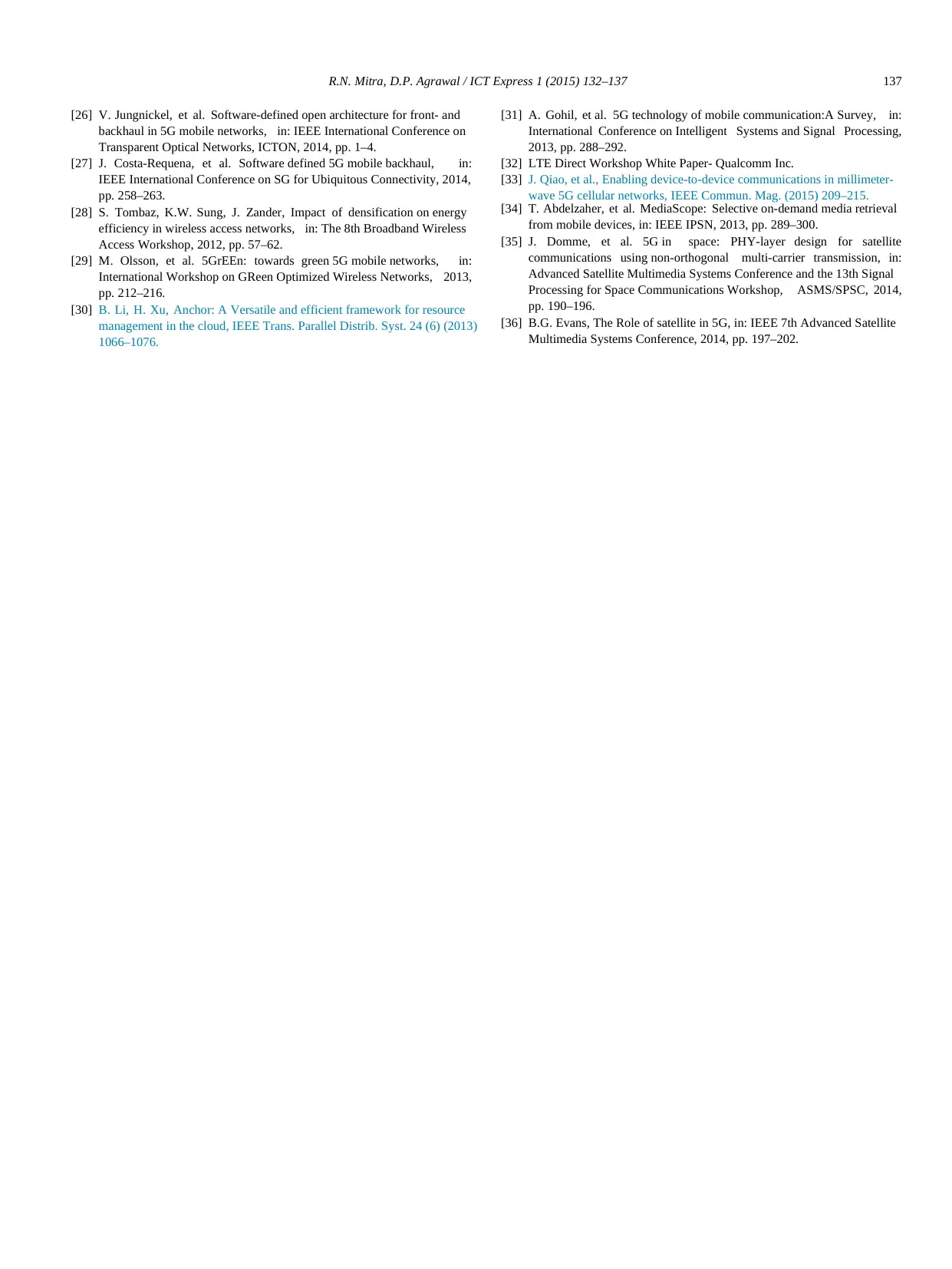
R.N. Mitra, D.P. Agrawal / ICT Express 1 (2015) 132–137 137
[26] V. Jungnickel, et al. Software-defined open architecture for front- and
backhaul in 5G mobile networks, in: IEEE International Conference on
Transparent Optical Networks, ICTON, 2014, pp. 1–4.
[27] J. Costa-Requena, et al. Software defined 5G mobile backhaul, in:
IEEE International Conference on SG for Ubiquitous Connectivity, 2014,
pp. 258–263.
[28] S. Tombaz, K.W. Sung, J. Zander, Impact of densification on energy
efficiency in wireless access networks, in: The 8th Broadband Wireless
Access Workshop, 2012, pp. 57–62.
[29] M. Olsson, et al. 5GrEEn: towards green 5G mobile networks, in:
International Workshop on GReen Optimized Wireless Networks, 2013,
pp. 212–216.
[30] B. Li, H. Xu, Anchor: A Versatile and efficient framework for resource
management in the cloud, IEEE Trans. Parallel Distrib. Syst. 24 (6) (2013)
1066–1076.
[31] A. Gohil, et al. 5G technology of mobile communication:A Survey, in:
International Conference on Intelligent Systems and Signal Processing,
2013, pp. 288–292.
[32] LTE Direct Workshop White Paper- Qualcomm Inc.
[33] J. Qiao, et al., Enabling device-to-device communications in millimeter-
wave 5G cellular networks, IEEE Commun. Mag. (2015) 209–215.
[34] T. Abdelzaher, et al. MediaScope: Selective on-demand media retrieval
from mobile devices, in: IEEE IPSN, 2013, pp. 289–300.
[35] J. Domme, et al. 5G in space: PHY-layer design for satellite
communications using non-orthogonal multi-carrier transmission, in:
Advanced Satellite Multimedia Systems Conference and the 13th Signal
Processing for Space Communications Workshop, ASMS/SPSC, 2014,
pp. 190–196.
[36] B.G. Evans, The Role of satellite in 5G, in: IEEE 7th Advanced Satellite
Multimedia Systems Conference, 2014, pp. 197–202.
[26] V. Jungnickel, et al. Software-defined open architecture for front- and
backhaul in 5G mobile networks, in: IEEE International Conference on
Transparent Optical Networks, ICTON, 2014, pp. 1–4.
[27] J. Costa-Requena, et al. Software defined 5G mobile backhaul, in:
IEEE International Conference on SG for Ubiquitous Connectivity, 2014,
pp. 258–263.
[28] S. Tombaz, K.W. Sung, J. Zander, Impact of densification on energy
efficiency in wireless access networks, in: The 8th Broadband Wireless
Access Workshop, 2012, pp. 57–62.
[29] M. Olsson, et al. 5GrEEn: towards green 5G mobile networks, in:
International Workshop on GReen Optimized Wireless Networks, 2013,
pp. 212–216.
[30] B. Li, H. Xu, Anchor: A Versatile and efficient framework for resource
management in the cloud, IEEE Trans. Parallel Distrib. Syst. 24 (6) (2013)
1066–1076.
[31] A. Gohil, et al. 5G technology of mobile communication:A Survey, in:
International Conference on Intelligent Systems and Signal Processing,
2013, pp. 288–292.
[32] LTE Direct Workshop White Paper- Qualcomm Inc.
[33] J. Qiao, et al., Enabling device-to-device communications in millimeter-
wave 5G cellular networks, IEEE Commun. Mag. (2015) 209–215.
[34] T. Abdelzaher, et al. MediaScope: Selective on-demand media retrieval
from mobile devices, in: IEEE IPSN, 2013, pp. 289–300.
[35] J. Domme, et al. 5G in space: PHY-layer design for satellite
communications using non-orthogonal multi-carrier transmission, in:
Advanced Satellite Multimedia Systems Conference and the 13th Signal
Processing for Space Communications Workshop, ASMS/SPSC, 2014,
pp. 190–196.
[36] B.G. Evans, The Role of satellite in 5G, in: IEEE 7th Advanced Satellite
Multimedia Systems Conference, 2014, pp. 197–202.
1 out of 6
Related Documents
![[object Object]](/_next/image/?url=%2F_next%2Fstatic%2Fmedia%2Flogo.6d15ce61.png&w=640&q=75)
Your All-in-One AI-Powered Toolkit for Academic Success.
+13062052269
info@desklib.com
Available 24*7 on WhatsApp / Email
Unlock your academic potential
© 2024 | Zucol Services PVT LTD | All rights reserved.