The PET Radiotracers
VerifiedAdded on 2023/06/04
|11
|2682
|409
AI Summary
This article discusses the applications of PET radiotracers in medical imaging for diagnosing diseases and monitoring treatment effects. It covers 18F-FDG, 11C-Choline, and 18F-Fluorodopa and their biochemical pathways in relation to lung cancer, brain tumor therapy, and Parkinson's disease.
Contribute Materials
Your contribution can guide someone’s learning journey. Share your
documents today.
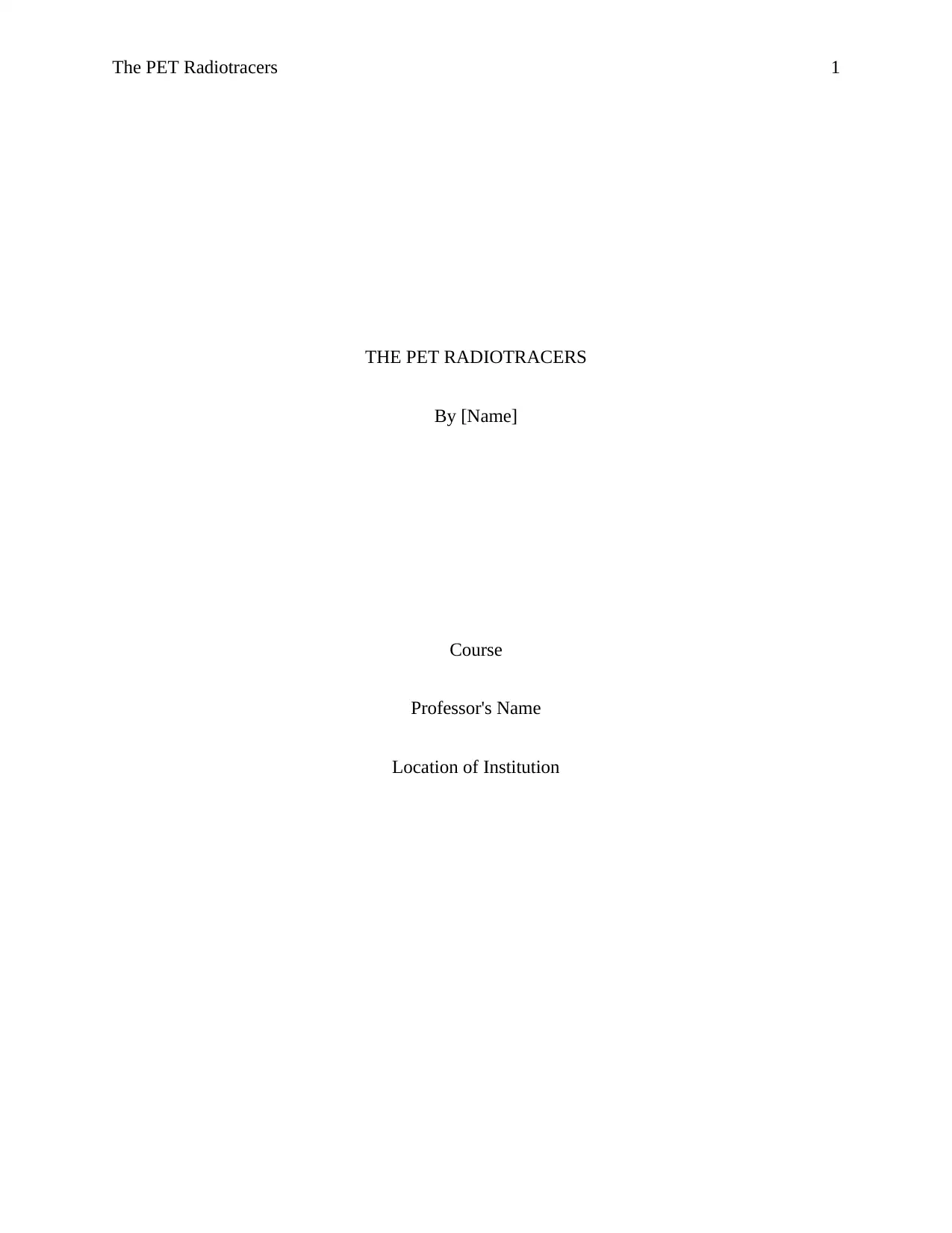
The PET Radiotracers 1
THE PET RADIOTRACERS
By [Name]
Course
Professor's Name
Location of Institution
THE PET RADIOTRACERS
By [Name]
Course
Professor's Name
Location of Institution
Secure Best Marks with AI Grader
Need help grading? Try our AI Grader for instant feedback on your assignments.
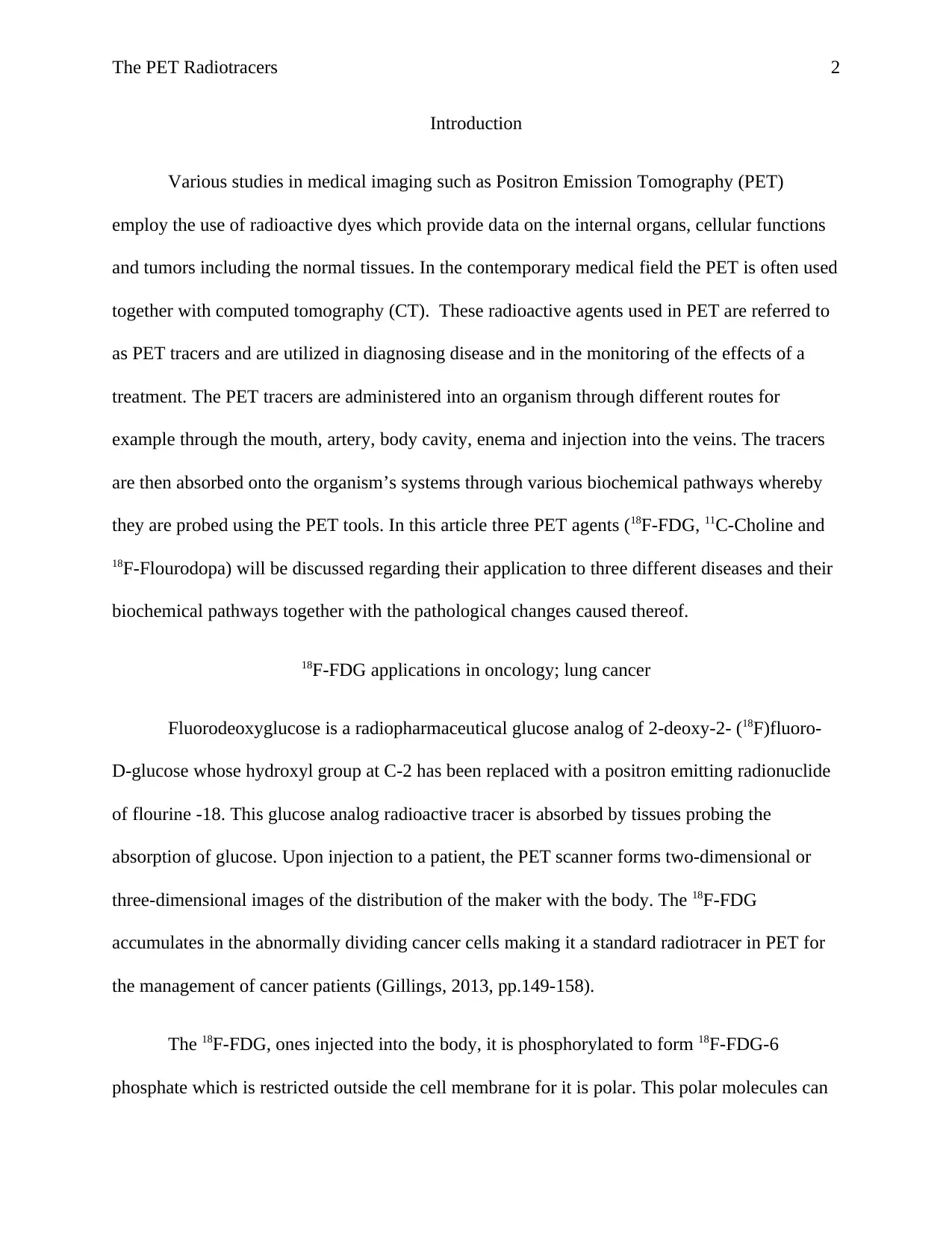
The PET Radiotracers 2
Introduction
Various studies in medical imaging such as Positron Emission Tomography (PET)
employ the use of radioactive dyes which provide data on the internal organs, cellular functions
and tumors including the normal tissues. In the contemporary medical field the PET is often used
together with computed tomography (CT). These radioactive agents used in PET are referred to
as PET tracers and are utilized in diagnosing disease and in the monitoring of the effects of a
treatment. The PET tracers are administered into an organism through different routes for
example through the mouth, artery, body cavity, enema and injection into the veins. The tracers
are then absorbed onto the organism’s systems through various biochemical pathways whereby
they are probed using the PET tools. In this article three PET agents (18F-FDG, 11C-Choline and
18F-Flourodopa) will be discussed regarding their application to three different diseases and their
biochemical pathways together with the pathological changes caused thereof.
18F-FDG applications in oncology; lung cancer
Fluorodeoxyglucose is a radiopharmaceutical glucose analog of 2-deoxy-2- (18F)fluoro-
D-glucose whose hydroxyl group at C-2 has been replaced with a positron emitting radionuclide
of flourine -18. This glucose analog radioactive tracer is absorbed by tissues probing the
absorption of glucose. Upon injection to a patient, the PET scanner forms two-dimensional or
three-dimensional images of the distribution of the maker with the body. The 18F-FDG
accumulates in the abnormally dividing cancer cells making it a standard radiotracer in PET for
the management of cancer patients (Gillings, 2013, pp.149-158).
The 18F-FDG, ones injected into the body, it is phosphorylated to form 18F-FDG-6
phosphate which is restricted outside the cell membrane for it is polar. This polar molecules can
Introduction
Various studies in medical imaging such as Positron Emission Tomography (PET)
employ the use of radioactive dyes which provide data on the internal organs, cellular functions
and tumors including the normal tissues. In the contemporary medical field the PET is often used
together with computed tomography (CT). These radioactive agents used in PET are referred to
as PET tracers and are utilized in diagnosing disease and in the monitoring of the effects of a
treatment. The PET tracers are administered into an organism through different routes for
example through the mouth, artery, body cavity, enema and injection into the veins. The tracers
are then absorbed onto the organism’s systems through various biochemical pathways whereby
they are probed using the PET tools. In this article three PET agents (18F-FDG, 11C-Choline and
18F-Flourodopa) will be discussed regarding their application to three different diseases and their
biochemical pathways together with the pathological changes caused thereof.
18F-FDG applications in oncology; lung cancer
Fluorodeoxyglucose is a radiopharmaceutical glucose analog of 2-deoxy-2- (18F)fluoro-
D-glucose whose hydroxyl group at C-2 has been replaced with a positron emitting radionuclide
of flourine -18. This glucose analog radioactive tracer is absorbed by tissues probing the
absorption of glucose. Upon injection to a patient, the PET scanner forms two-dimensional or
three-dimensional images of the distribution of the maker with the body. The 18F-FDG
accumulates in the abnormally dividing cancer cells making it a standard radiotracer in PET for
the management of cancer patients (Gillings, 2013, pp.149-158).
The 18F-FDG, ones injected into the body, it is phosphorylated to form 18F-FDG-6
phosphate which is restricted outside the cell membrane for it is polar. This polar molecules can
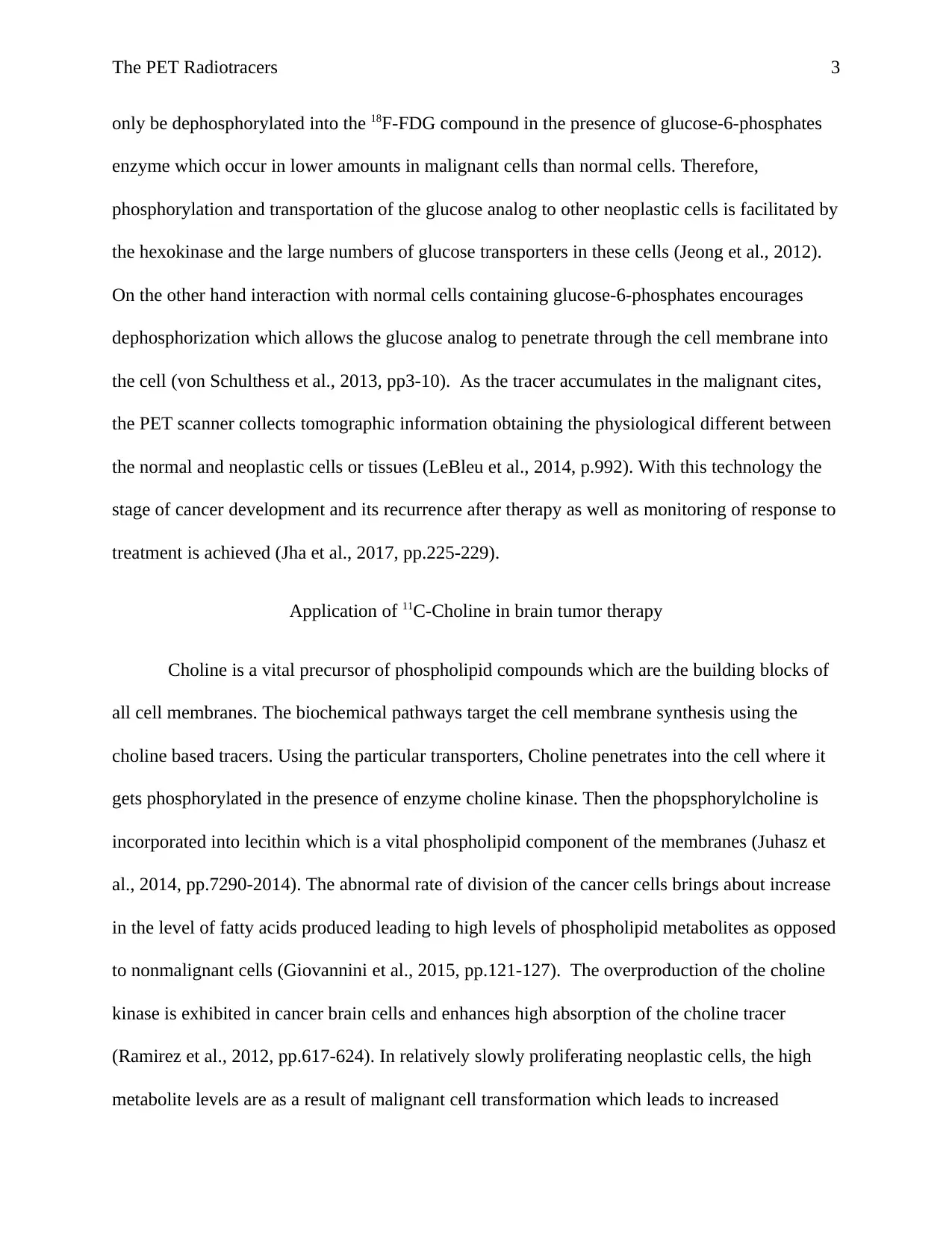
The PET Radiotracers 3
only be dephosphorylated into the 18F-FDG compound in the presence of glucose-6-phosphates
enzyme which occur in lower amounts in malignant cells than normal cells. Therefore,
phosphorylation and transportation of the glucose analog to other neoplastic cells is facilitated by
the hexokinase and the large numbers of glucose transporters in these cells (Jeong et al., 2012).
On the other hand interaction with normal cells containing glucose-6-phosphates encourages
dephosphorization which allows the glucose analog to penetrate through the cell membrane into
the cell (von Schulthess et al., 2013, pp3-10). As the tracer accumulates in the malignant cites,
the PET scanner collects tomographic information obtaining the physiological different between
the normal and neoplastic cells or tissues (LeBleu et al., 2014, p.992). With this technology the
stage of cancer development and its recurrence after therapy as well as monitoring of response to
treatment is achieved (Jha et al., 2017, pp.225-229).
Application of 11C-Choline in brain tumor therapy
Choline is a vital precursor of phospholipid compounds which are the building blocks of
all cell membranes. The biochemical pathways target the cell membrane synthesis using the
choline based tracers. Using the particular transporters, Choline penetrates into the cell where it
gets phosphorylated in the presence of enzyme choline kinase. Then the phopsphorylcholine is
incorporated into lecithin which is a vital phospholipid component of the membranes (Juhasz et
al., 2014, pp.7290-2014). The abnormal rate of division of the cancer cells brings about increase
in the level of fatty acids produced leading to high levels of phospholipid metabolites as opposed
to nonmalignant cells (Giovannini et al., 2015, pp.121-127). The overproduction of the choline
kinase is exhibited in cancer brain cells and enhances high absorption of the choline tracer
(Ramirez et al., 2012, pp.617-624). In relatively slowly proliferating neoplastic cells, the high
metabolite levels are as a result of malignant cell transformation which leads to increased
only be dephosphorylated into the 18F-FDG compound in the presence of glucose-6-phosphates
enzyme which occur in lower amounts in malignant cells than normal cells. Therefore,
phosphorylation and transportation of the glucose analog to other neoplastic cells is facilitated by
the hexokinase and the large numbers of glucose transporters in these cells (Jeong et al., 2012).
On the other hand interaction with normal cells containing glucose-6-phosphates encourages
dephosphorization which allows the glucose analog to penetrate through the cell membrane into
the cell (von Schulthess et al., 2013, pp3-10). As the tracer accumulates in the malignant cites,
the PET scanner collects tomographic information obtaining the physiological different between
the normal and neoplastic cells or tissues (LeBleu et al., 2014, p.992). With this technology the
stage of cancer development and its recurrence after therapy as well as monitoring of response to
treatment is achieved (Jha et al., 2017, pp.225-229).
Application of 11C-Choline in brain tumor therapy
Choline is a vital precursor of phospholipid compounds which are the building blocks of
all cell membranes. The biochemical pathways target the cell membrane synthesis using the
choline based tracers. Using the particular transporters, Choline penetrates into the cell where it
gets phosphorylated in the presence of enzyme choline kinase. Then the phopsphorylcholine is
incorporated into lecithin which is a vital phospholipid component of the membranes (Juhasz et
al., 2014, pp.7290-2014). The abnormal rate of division of the cancer cells brings about increase
in the level of fatty acids produced leading to high levels of phospholipid metabolites as opposed
to nonmalignant cells (Giovannini et al., 2015, pp.121-127). The overproduction of the choline
kinase is exhibited in cancer brain cells and enhances high absorption of the choline tracer
(Ramirez et al., 2012, pp.617-624). In relatively slowly proliferating neoplastic cells, the high
metabolite levels are as a result of malignant cell transformation which leads to increased
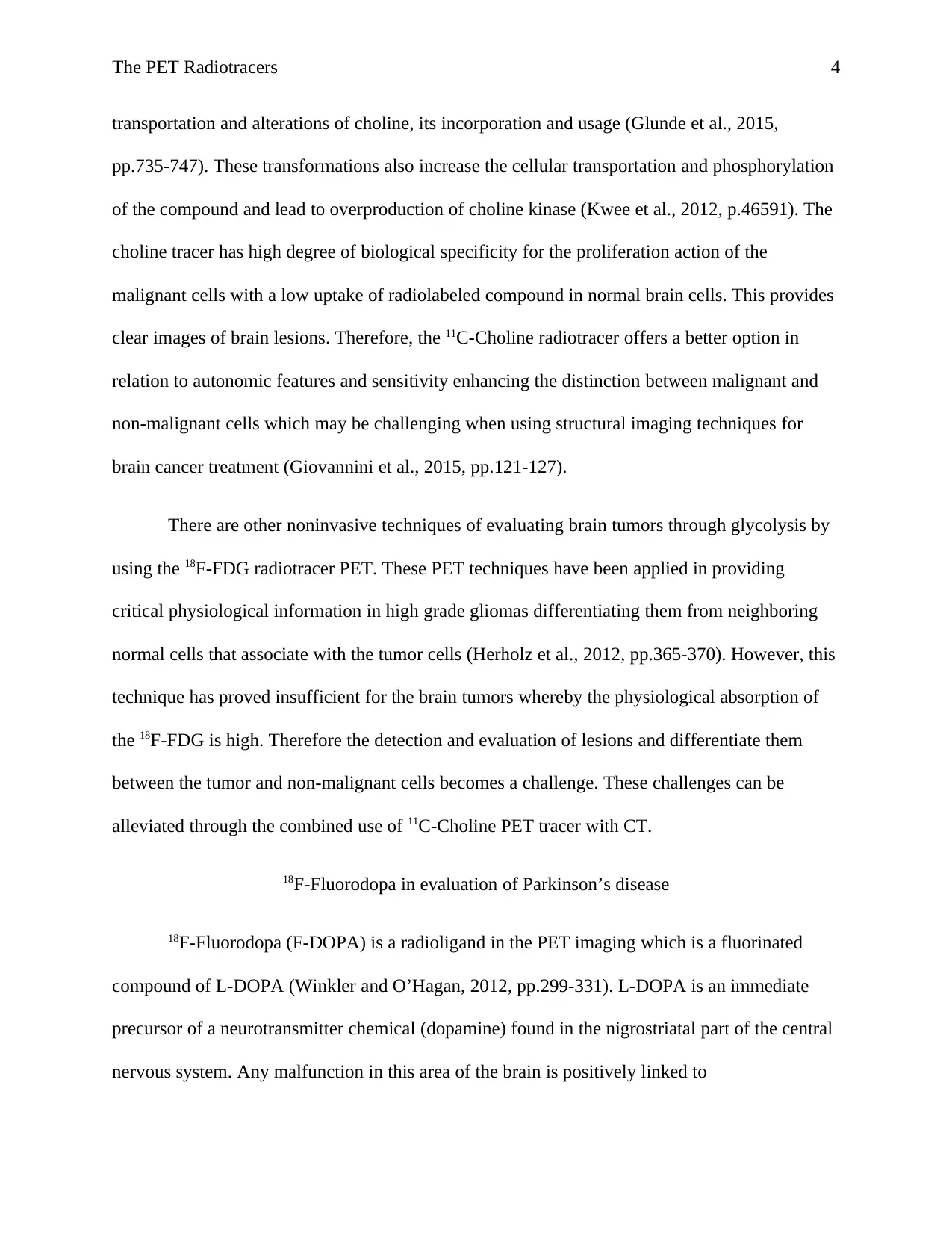
The PET Radiotracers 4
transportation and alterations of choline, its incorporation and usage (Glunde et al., 2015,
pp.735-747). These transformations also increase the cellular transportation and phosphorylation
of the compound and lead to overproduction of choline kinase (Kwee et al., 2012, p.46591). The
choline tracer has high degree of biological specificity for the proliferation action of the
malignant cells with a low uptake of radiolabeled compound in normal brain cells. This provides
clear images of brain lesions. Therefore, the 11C-Choline radiotracer offers a better option in
relation to autonomic features and sensitivity enhancing the distinction between malignant and
non-malignant cells which may be challenging when using structural imaging techniques for
brain cancer treatment (Giovannini et al., 2015, pp.121-127).
There are other noninvasive techniques of evaluating brain tumors through glycolysis by
using the 18F-FDG radiotracer PET. These PET techniques have been applied in providing
critical physiological information in high grade gliomas differentiating them from neighboring
normal cells that associate with the tumor cells (Herholz et al., 2012, pp.365-370). However, this
technique has proved insufficient for the brain tumors whereby the physiological absorption of
the 18F-FDG is high. Therefore the detection and evaluation of lesions and differentiate them
between the tumor and non-malignant cells becomes a challenge. These challenges can be
alleviated through the combined use of 11C-Choline PET tracer with CT.
18F-Fluorodopa in evaluation of Parkinson’s disease
18F-Fluorodopa (F-DOPA) is a radioligand in the PET imaging which is a fluorinated
compound of L-DOPA (Winkler and O’Hagan, 2012, pp.299-331). L-DOPA is an immediate
precursor of a neurotransmitter chemical (dopamine) found in the nigrostriatal part of the central
nervous system. Any malfunction in this area of the brain is positively linked to
transportation and alterations of choline, its incorporation and usage (Glunde et al., 2015,
pp.735-747). These transformations also increase the cellular transportation and phosphorylation
of the compound and lead to overproduction of choline kinase (Kwee et al., 2012, p.46591). The
choline tracer has high degree of biological specificity for the proliferation action of the
malignant cells with a low uptake of radiolabeled compound in normal brain cells. This provides
clear images of brain lesions. Therefore, the 11C-Choline radiotracer offers a better option in
relation to autonomic features and sensitivity enhancing the distinction between malignant and
non-malignant cells which may be challenging when using structural imaging techniques for
brain cancer treatment (Giovannini et al., 2015, pp.121-127).
There are other noninvasive techniques of evaluating brain tumors through glycolysis by
using the 18F-FDG radiotracer PET. These PET techniques have been applied in providing
critical physiological information in high grade gliomas differentiating them from neighboring
normal cells that associate with the tumor cells (Herholz et al., 2012, pp.365-370). However, this
technique has proved insufficient for the brain tumors whereby the physiological absorption of
the 18F-FDG is high. Therefore the detection and evaluation of lesions and differentiate them
between the tumor and non-malignant cells becomes a challenge. These challenges can be
alleviated through the combined use of 11C-Choline PET tracer with CT.
18F-Fluorodopa in evaluation of Parkinson’s disease
18F-Fluorodopa (F-DOPA) is a radioligand in the PET imaging which is a fluorinated
compound of L-DOPA (Winkler and O’Hagan, 2012, pp.299-331). L-DOPA is an immediate
precursor of a neurotransmitter chemical (dopamine) found in the nigrostriatal part of the central
nervous system. Any malfunction in this area of the brain is positively linked to
Secure Best Marks with AI Grader
Need help grading? Try our AI Grader for instant feedback on your assignments.
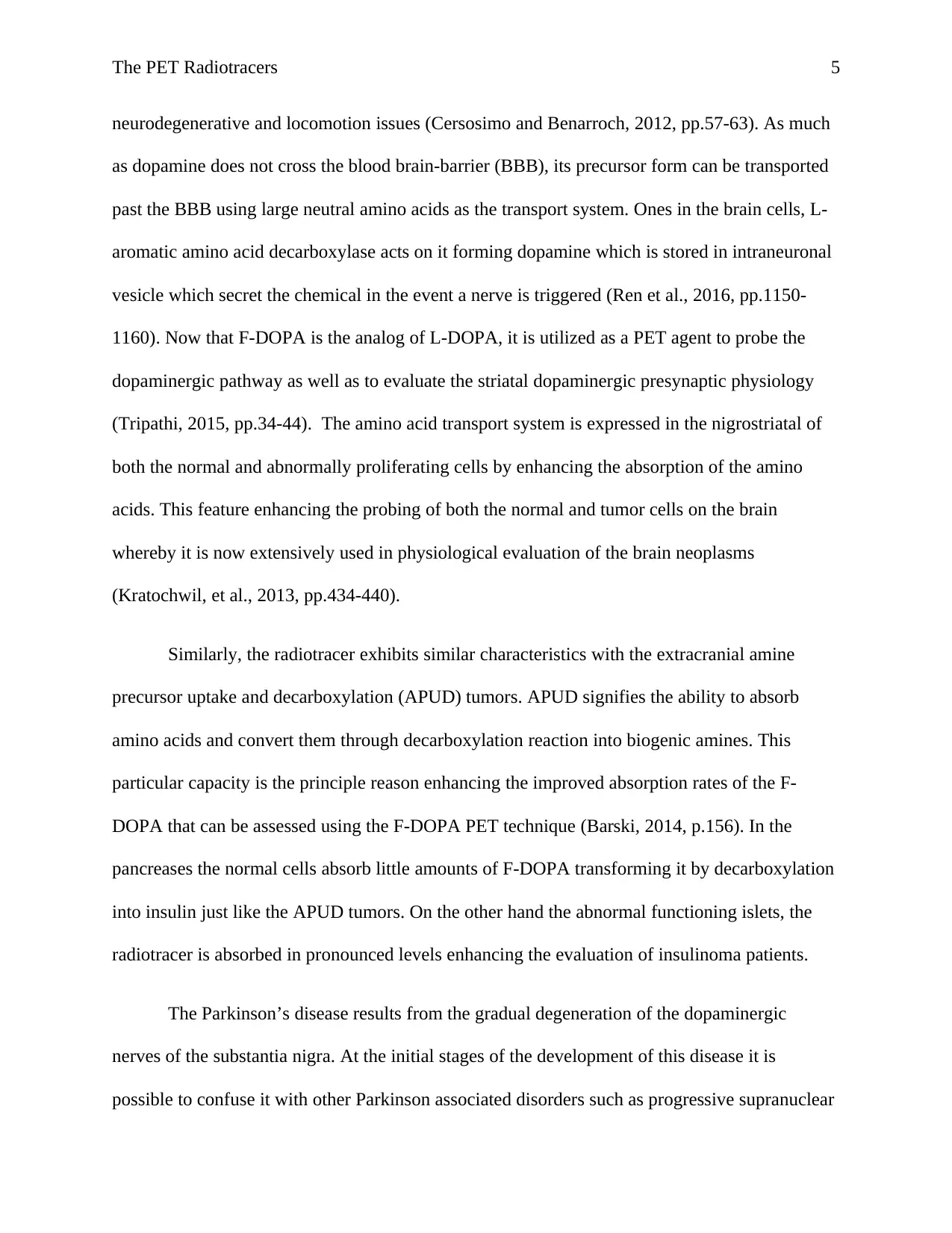
The PET Radiotracers 5
neurodegenerative and locomotion issues (Cersosimo and Benarroch, 2012, pp.57-63). As much
as dopamine does not cross the blood brain-barrier (BBB), its precursor form can be transported
past the BBB using large neutral amino acids as the transport system. Ones in the brain cells, L-
aromatic amino acid decarboxylase acts on it forming dopamine which is stored in intraneuronal
vesicle which secret the chemical in the event a nerve is triggered (Ren et al., 2016, pp.1150-
1160). Now that F-DOPA is the analog of L-DOPA, it is utilized as a PET agent to probe the
dopaminergic pathway as well as to evaluate the striatal dopaminergic presynaptic physiology
(Tripathi, 2015, pp.34-44). The amino acid transport system is expressed in the nigrostriatal of
both the normal and abnormally proliferating cells by enhancing the absorption of the amino
acids. This feature enhancing the probing of both the normal and tumor cells on the brain
whereby it is now extensively used in physiological evaluation of the brain neoplasms
(Kratochwil, et al., 2013, pp.434-440).
Similarly, the radiotracer exhibits similar characteristics with the extracranial amine
precursor uptake and decarboxylation (APUD) tumors. APUD signifies the ability to absorb
amino acids and convert them through decarboxylation reaction into biogenic amines. This
particular capacity is the principle reason enhancing the improved absorption rates of the F-
DOPA that can be assessed using the F-DOPA PET technique (Barski, 2014, p.156). In the
pancreases the normal cells absorb little amounts of F-DOPA transforming it by decarboxylation
into insulin just like the APUD tumors. On the other hand the abnormal functioning islets, the
radiotracer is absorbed in pronounced levels enhancing the evaluation of insulinoma patients.
The Parkinson’s disease results from the gradual degeneration of the dopaminergic
nerves of the substantia nigra. At the initial stages of the development of this disease it is
possible to confuse it with other Parkinson associated disorders such as progressive supranuclear
neurodegenerative and locomotion issues (Cersosimo and Benarroch, 2012, pp.57-63). As much
as dopamine does not cross the blood brain-barrier (BBB), its precursor form can be transported
past the BBB using large neutral amino acids as the transport system. Ones in the brain cells, L-
aromatic amino acid decarboxylase acts on it forming dopamine which is stored in intraneuronal
vesicle which secret the chemical in the event a nerve is triggered (Ren et al., 2016, pp.1150-
1160). Now that F-DOPA is the analog of L-DOPA, it is utilized as a PET agent to probe the
dopaminergic pathway as well as to evaluate the striatal dopaminergic presynaptic physiology
(Tripathi, 2015, pp.34-44). The amino acid transport system is expressed in the nigrostriatal of
both the normal and abnormally proliferating cells by enhancing the absorption of the amino
acids. This feature enhancing the probing of both the normal and tumor cells on the brain
whereby it is now extensively used in physiological evaluation of the brain neoplasms
(Kratochwil, et al., 2013, pp.434-440).
Similarly, the radiotracer exhibits similar characteristics with the extracranial amine
precursor uptake and decarboxylation (APUD) tumors. APUD signifies the ability to absorb
amino acids and convert them through decarboxylation reaction into biogenic amines. This
particular capacity is the principle reason enhancing the improved absorption rates of the F-
DOPA that can be assessed using the F-DOPA PET technique (Barski, 2014, p.156). In the
pancreases the normal cells absorb little amounts of F-DOPA transforming it by decarboxylation
into insulin just like the APUD tumors. On the other hand the abnormal functioning islets, the
radiotracer is absorbed in pronounced levels enhancing the evaluation of insulinoma patients.
The Parkinson’s disease results from the gradual degeneration of the dopaminergic
nerves of the substantia nigra. At the initial stages of the development of this disease it is
possible to confuse it with other Parkinson associated disorders such as progressive supranuclear
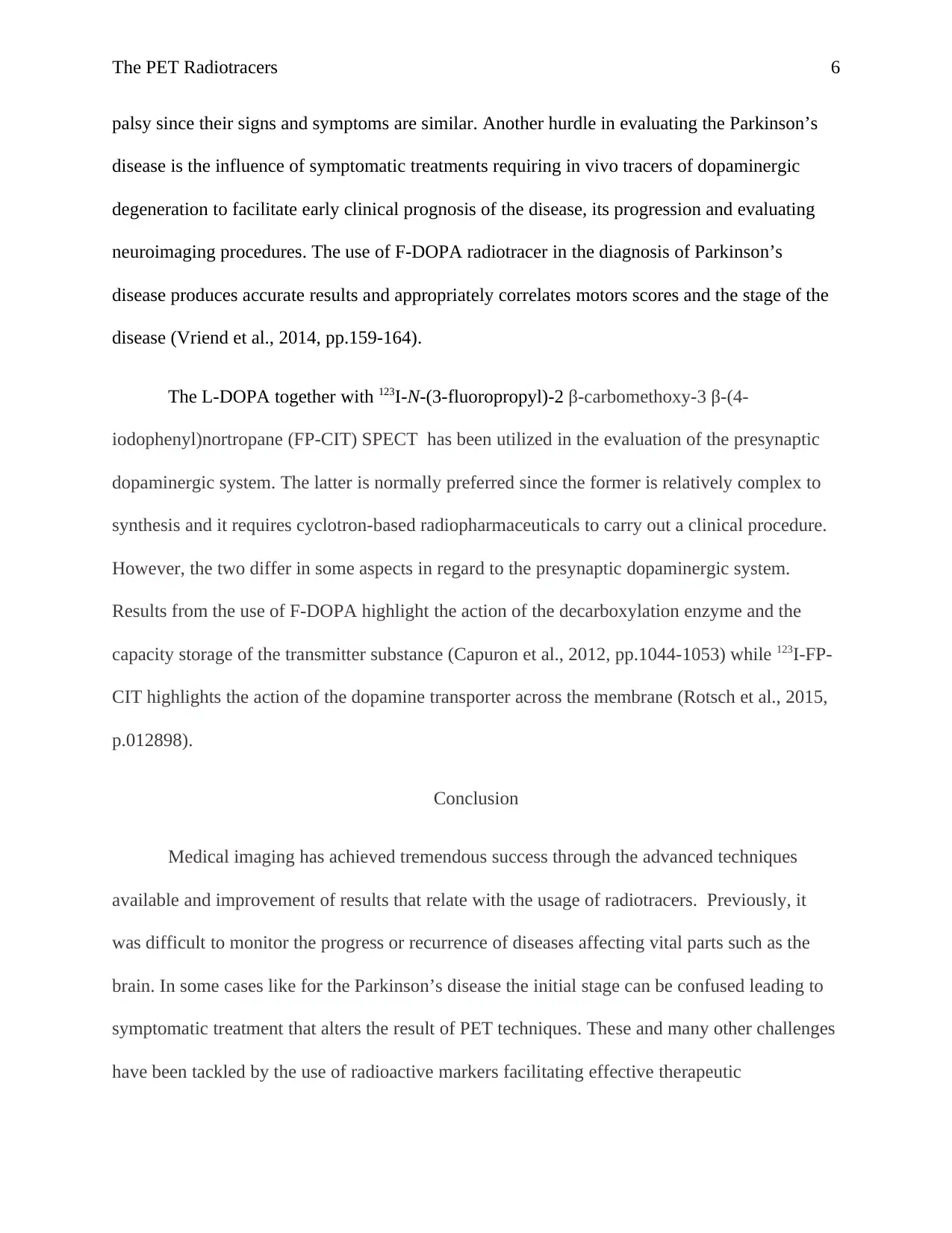
The PET Radiotracers 6
palsy since their signs and symptoms are similar. Another hurdle in evaluating the Parkinson’s
disease is the influence of symptomatic treatments requiring in vivo tracers of dopaminergic
degeneration to facilitate early clinical prognosis of the disease, its progression and evaluating
neuroimaging procedures. The use of F-DOPA radiotracer in the diagnosis of Parkinson’s
disease produces accurate results and appropriately correlates motors scores and the stage of the
disease (Vriend et al., 2014, pp.159-164).
The L-DOPA together with 123I-N-(3-fluoropropyl)-2 β-carbomethoxy-3 β-(4-
iodophenyl)nortropane (FP-CIT) SPECT has been utilized in the evaluation of the presynaptic
dopaminergic system. The latter is normally preferred since the former is relatively complex to
synthesis and it requires cyclotron-based radiopharmaceuticals to carry out a clinical procedure.
However, the two differ in some aspects in regard to the presynaptic dopaminergic system.
Results from the use of F-DOPA highlight the action of the decarboxylation enzyme and the
capacity storage of the transmitter substance (Capuron et al., 2012, pp.1044-1053) while 123I-FP-
CIT highlights the action of the dopamine transporter across the membrane (Rotsch et al., 2015,
p.012898).
Conclusion
Medical imaging has achieved tremendous success through the advanced techniques
available and improvement of results that relate with the usage of radiotracers. Previously, it
was difficult to monitor the progress or recurrence of diseases affecting vital parts such as the
brain. In some cases like for the Parkinson’s disease the initial stage can be confused leading to
symptomatic treatment that alters the result of PET techniques. These and many other challenges
have been tackled by the use of radioactive markers facilitating effective therapeutic
palsy since their signs and symptoms are similar. Another hurdle in evaluating the Parkinson’s
disease is the influence of symptomatic treatments requiring in vivo tracers of dopaminergic
degeneration to facilitate early clinical prognosis of the disease, its progression and evaluating
neuroimaging procedures. The use of F-DOPA radiotracer in the diagnosis of Parkinson’s
disease produces accurate results and appropriately correlates motors scores and the stage of the
disease (Vriend et al., 2014, pp.159-164).
The L-DOPA together with 123I-N-(3-fluoropropyl)-2 β-carbomethoxy-3 β-(4-
iodophenyl)nortropane (FP-CIT) SPECT has been utilized in the evaluation of the presynaptic
dopaminergic system. The latter is normally preferred since the former is relatively complex to
synthesis and it requires cyclotron-based radiopharmaceuticals to carry out a clinical procedure.
However, the two differ in some aspects in regard to the presynaptic dopaminergic system.
Results from the use of F-DOPA highlight the action of the decarboxylation enzyme and the
capacity storage of the transmitter substance (Capuron et al., 2012, pp.1044-1053) while 123I-FP-
CIT highlights the action of the dopamine transporter across the membrane (Rotsch et al., 2015,
p.012898).
Conclusion
Medical imaging has achieved tremendous success through the advanced techniques
available and improvement of results that relate with the usage of radiotracers. Previously, it
was difficult to monitor the progress or recurrence of diseases affecting vital parts such as the
brain. In some cases like for the Parkinson’s disease the initial stage can be confused leading to
symptomatic treatment that alters the result of PET techniques. These and many other challenges
have been tackled by the use of radioactive markers facilitating effective therapeutic
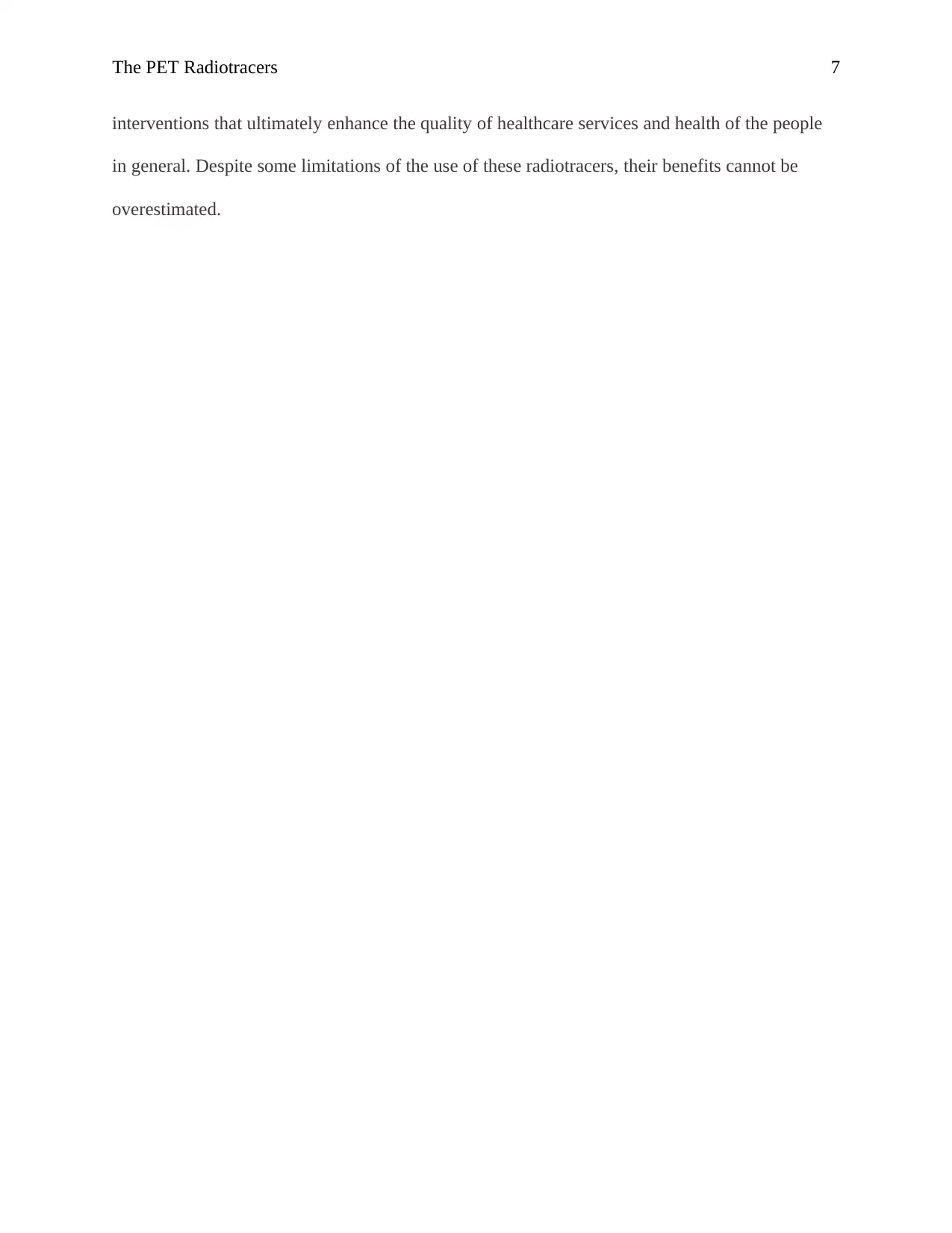
The PET Radiotracers 7
interventions that ultimately enhance the quality of healthcare services and health of the people
in general. Despite some limitations of the use of these radiotracers, their benefits cannot be
overestimated.
interventions that ultimately enhance the quality of healthcare services and health of the people
in general. Despite some limitations of the use of these radiotracers, their benefits cannot be
overestimated.
Paraphrase This Document
Need a fresh take? Get an instant paraphrase of this document with our AI Paraphraser
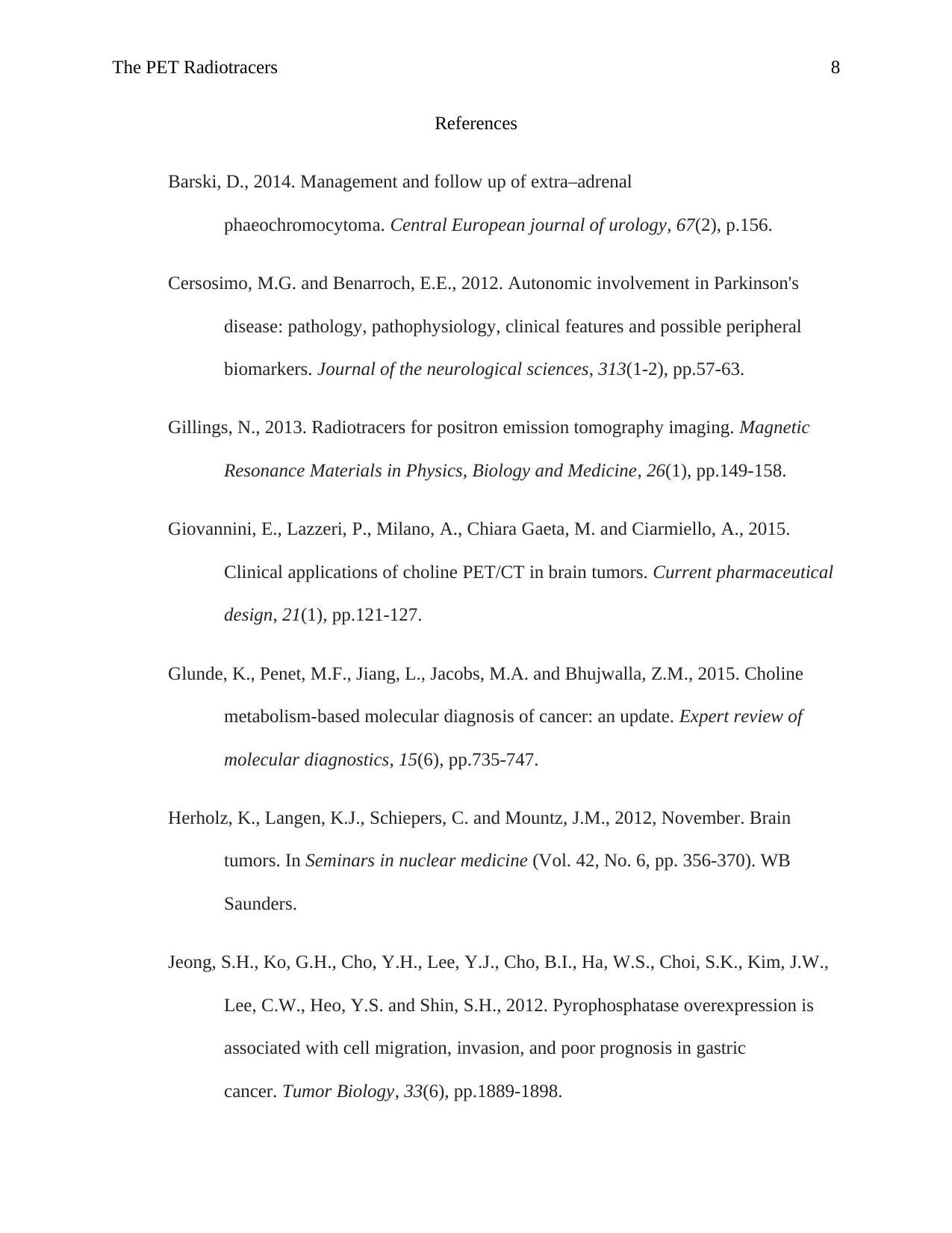
The PET Radiotracers 8
References
Barski, D., 2014. Management and follow up of extra–adrenal
phaeochromocytoma. Central European journal of urology, 67(2), p.156.
Cersosimo, M.G. and Benarroch, E.E., 2012. Autonomic involvement in Parkinson's
disease: pathology, pathophysiology, clinical features and possible peripheral
biomarkers. Journal of the neurological sciences, 313(1-2), pp.57-63.
Gillings, N., 2013. Radiotracers for positron emission tomography imaging. Magnetic
Resonance Materials in Physics, Biology and Medicine, 26(1), pp.149-158.
Giovannini, E., Lazzeri, P., Milano, A., Chiara Gaeta, M. and Ciarmiello, A., 2015.
Clinical applications of choline PET/CT in brain tumors. Current pharmaceutical
design, 21(1), pp.121-127.
Glunde, K., Penet, M.F., Jiang, L., Jacobs, M.A. and Bhujwalla, Z.M., 2015. Choline
metabolism-based molecular diagnosis of cancer: an update. Expert review of
molecular diagnostics, 15(6), pp.735-747.
Herholz, K., Langen, K.J., Schiepers, C. and Mountz, J.M., 2012, November. Brain
tumors. In Seminars in nuclear medicine (Vol. 42, No. 6, pp. 356-370). WB
Saunders.
Jeong, S.H., Ko, G.H., Cho, Y.H., Lee, Y.J., Cho, B.I., Ha, W.S., Choi, S.K., Kim, J.W.,
Lee, C.W., Heo, Y.S. and Shin, S.H., 2012. Pyrophosphatase overexpression is
associated with cell migration, invasion, and poor prognosis in gastric
cancer. Tumor Biology, 33(6), pp.1889-1898.
References
Barski, D., 2014. Management and follow up of extra–adrenal
phaeochromocytoma. Central European journal of urology, 67(2), p.156.
Cersosimo, M.G. and Benarroch, E.E., 2012. Autonomic involvement in Parkinson's
disease: pathology, pathophysiology, clinical features and possible peripheral
biomarkers. Journal of the neurological sciences, 313(1-2), pp.57-63.
Gillings, N., 2013. Radiotracers for positron emission tomography imaging. Magnetic
Resonance Materials in Physics, Biology and Medicine, 26(1), pp.149-158.
Giovannini, E., Lazzeri, P., Milano, A., Chiara Gaeta, M. and Ciarmiello, A., 2015.
Clinical applications of choline PET/CT in brain tumors. Current pharmaceutical
design, 21(1), pp.121-127.
Glunde, K., Penet, M.F., Jiang, L., Jacobs, M.A. and Bhujwalla, Z.M., 2015. Choline
metabolism-based molecular diagnosis of cancer: an update. Expert review of
molecular diagnostics, 15(6), pp.735-747.
Herholz, K., Langen, K.J., Schiepers, C. and Mountz, J.M., 2012, November. Brain
tumors. In Seminars in nuclear medicine (Vol. 42, No. 6, pp. 356-370). WB
Saunders.
Jeong, S.H., Ko, G.H., Cho, Y.H., Lee, Y.J., Cho, B.I., Ha, W.S., Choi, S.K., Kim, J.W.,
Lee, C.W., Heo, Y.S. and Shin, S.H., 2012. Pyrophosphatase overexpression is
associated with cell migration, invasion, and poor prognosis in gastric
cancer. Tumor Biology, 33(6), pp.1889-1898.
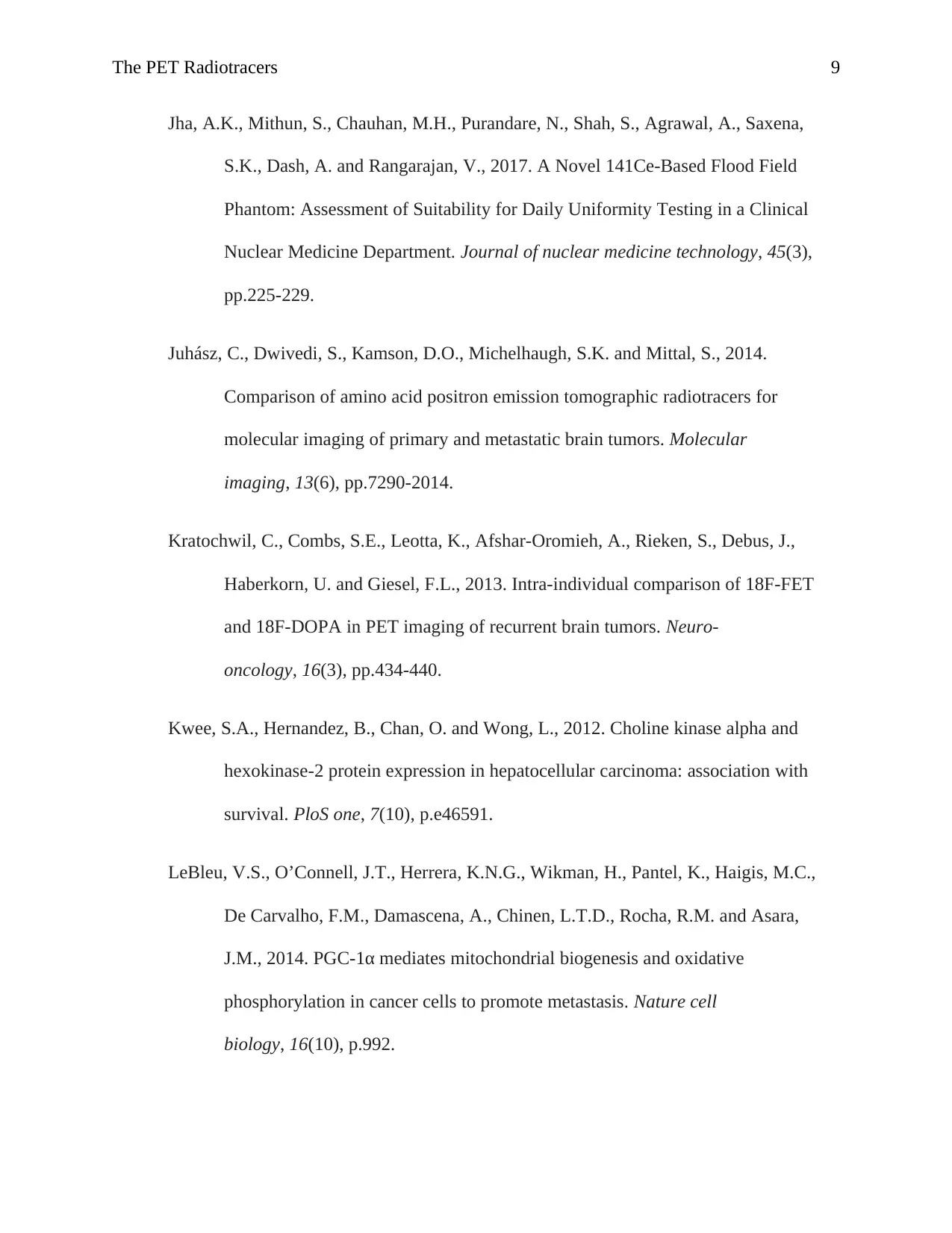
The PET Radiotracers 9
Jha, A.K., Mithun, S., Chauhan, M.H., Purandare, N., Shah, S., Agrawal, A., Saxena,
S.K., Dash, A. and Rangarajan, V., 2017. A Novel 141Ce-Based Flood Field
Phantom: Assessment of Suitability for Daily Uniformity Testing in a Clinical
Nuclear Medicine Department. Journal of nuclear medicine technology, 45(3),
pp.225-229.
Juhász, C., Dwivedi, S., Kamson, D.O., Michelhaugh, S.K. and Mittal, S., 2014.
Comparison of amino acid positron emission tomographic radiotracers for
molecular imaging of primary and metastatic brain tumors. Molecular
imaging, 13(6), pp.7290-2014.
Kratochwil, C., Combs, S.E., Leotta, K., Afshar-Oromieh, A., Rieken, S., Debus, J.,
Haberkorn, U. and Giesel, F.L., 2013. Intra-individual comparison of 18F-FET
and 18F-DOPA in PET imaging of recurrent brain tumors. Neuro-
oncology, 16(3), pp.434-440.
Kwee, S.A., Hernandez, B., Chan, O. and Wong, L., 2012. Choline kinase alpha and
hexokinase-2 protein expression in hepatocellular carcinoma: association with
survival. PloS one, 7(10), p.e46591.
LeBleu, V.S., O’Connell, J.T., Herrera, K.N.G., Wikman, H., Pantel, K., Haigis, M.C.,
De Carvalho, F.M., Damascena, A., Chinen, L.T.D., Rocha, R.M. and Asara,
J.M., 2014. PGC-1α mediates mitochondrial biogenesis and oxidative
phosphorylation in cancer cells to promote metastasis. Nature cell
biology, 16(10), p.992.
Jha, A.K., Mithun, S., Chauhan, M.H., Purandare, N., Shah, S., Agrawal, A., Saxena,
S.K., Dash, A. and Rangarajan, V., 2017. A Novel 141Ce-Based Flood Field
Phantom: Assessment of Suitability for Daily Uniformity Testing in a Clinical
Nuclear Medicine Department. Journal of nuclear medicine technology, 45(3),
pp.225-229.
Juhász, C., Dwivedi, S., Kamson, D.O., Michelhaugh, S.K. and Mittal, S., 2014.
Comparison of amino acid positron emission tomographic radiotracers for
molecular imaging of primary and metastatic brain tumors. Molecular
imaging, 13(6), pp.7290-2014.
Kratochwil, C., Combs, S.E., Leotta, K., Afshar-Oromieh, A., Rieken, S., Debus, J.,
Haberkorn, U. and Giesel, F.L., 2013. Intra-individual comparison of 18F-FET
and 18F-DOPA in PET imaging of recurrent brain tumors. Neuro-
oncology, 16(3), pp.434-440.
Kwee, S.A., Hernandez, B., Chan, O. and Wong, L., 2012. Choline kinase alpha and
hexokinase-2 protein expression in hepatocellular carcinoma: association with
survival. PloS one, 7(10), p.e46591.
LeBleu, V.S., O’Connell, J.T., Herrera, K.N.G., Wikman, H., Pantel, K., Haigis, M.C.,
De Carvalho, F.M., Damascena, A., Chinen, L.T.D., Rocha, R.M. and Asara,
J.M., 2014. PGC-1α mediates mitochondrial biogenesis and oxidative
phosphorylation in cancer cells to promote metastasis. Nature cell
biology, 16(10), p.992.
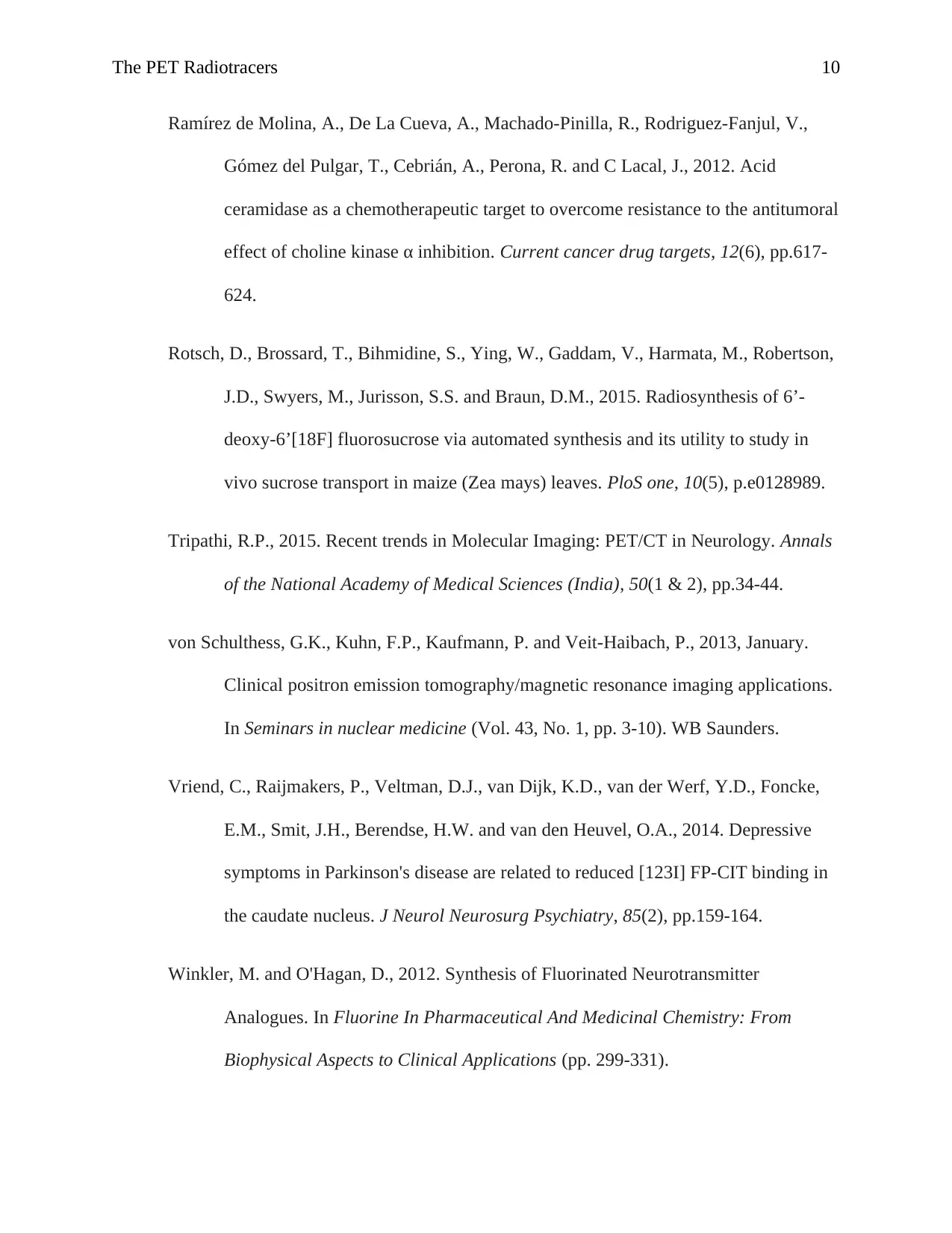
The PET Radiotracers 10
Ramírez de Molina, A., De La Cueva, A., Machado-Pinilla, R., Rodriguez-Fanjul, V.,
Gómez del Pulgar, T., Cebrián, A., Perona, R. and C Lacal, J., 2012. Acid
ceramidase as a chemotherapeutic target to overcome resistance to the antitumoral
effect of choline kinase α inhibition. Current cancer drug targets, 12(6), pp.617-
624.
Rotsch, D., Brossard, T., Bihmidine, S., Ying, W., Gaddam, V., Harmata, M., Robertson,
J.D., Swyers, M., Jurisson, S.S. and Braun, D.M., 2015. Radiosynthesis of 6’-
deoxy-6’[18F] fluorosucrose via automated synthesis and its utility to study in
vivo sucrose transport in maize (Zea mays) leaves. PloS one, 10(5), p.e0128989.
Tripathi, R.P., 2015. Recent trends in Molecular Imaging: PET/CT in Neurology. Annals
of the National Academy of Medical Sciences (India), 50(1 & 2), pp.34-44.
von Schulthess, G.K., Kuhn, F.P., Kaufmann, P. and Veit-Haibach, P., 2013, January.
Clinical positron emission tomography/magnetic resonance imaging applications.
In Seminars in nuclear medicine (Vol. 43, No. 1, pp. 3-10). WB Saunders.
Vriend, C., Raijmakers, P., Veltman, D.J., van Dijk, K.D., van der Werf, Y.D., Foncke,
E.M., Smit, J.H., Berendse, H.W. and van den Heuvel, O.A., 2014. Depressive
symptoms in Parkinson's disease are related to reduced [123I] FP-CIT binding in
the caudate nucleus. J Neurol Neurosurg Psychiatry, 85(2), pp.159-164.
Winkler, M. and O'Hagan, D., 2012. Synthesis of Fluorinated Neurotransmitter
Analogues. In Fluorine In Pharmaceutical And Medicinal Chemistry: From
Biophysical Aspects to Clinical Applications (pp. 299-331).
Ramírez de Molina, A., De La Cueva, A., Machado-Pinilla, R., Rodriguez-Fanjul, V.,
Gómez del Pulgar, T., Cebrián, A., Perona, R. and C Lacal, J., 2012. Acid
ceramidase as a chemotherapeutic target to overcome resistance to the antitumoral
effect of choline kinase α inhibition. Current cancer drug targets, 12(6), pp.617-
624.
Rotsch, D., Brossard, T., Bihmidine, S., Ying, W., Gaddam, V., Harmata, M., Robertson,
J.D., Swyers, M., Jurisson, S.S. and Braun, D.M., 2015. Radiosynthesis of 6’-
deoxy-6’[18F] fluorosucrose via automated synthesis and its utility to study in
vivo sucrose transport in maize (Zea mays) leaves. PloS one, 10(5), p.e0128989.
Tripathi, R.P., 2015. Recent trends in Molecular Imaging: PET/CT in Neurology. Annals
of the National Academy of Medical Sciences (India), 50(1 & 2), pp.34-44.
von Schulthess, G.K., Kuhn, F.P., Kaufmann, P. and Veit-Haibach, P., 2013, January.
Clinical positron emission tomography/magnetic resonance imaging applications.
In Seminars in nuclear medicine (Vol. 43, No. 1, pp. 3-10). WB Saunders.
Vriend, C., Raijmakers, P., Veltman, D.J., van Dijk, K.D., van der Werf, Y.D., Foncke,
E.M., Smit, J.H., Berendse, H.W. and van den Heuvel, O.A., 2014. Depressive
symptoms in Parkinson's disease are related to reduced [123I] FP-CIT binding in
the caudate nucleus. J Neurol Neurosurg Psychiatry, 85(2), pp.159-164.
Winkler, M. and O'Hagan, D., 2012. Synthesis of Fluorinated Neurotransmitter
Analogues. In Fluorine In Pharmaceutical And Medicinal Chemistry: From
Biophysical Aspects to Clinical Applications (pp. 299-331).
Secure Best Marks with AI Grader
Need help grading? Try our AI Grader for instant feedback on your assignments.
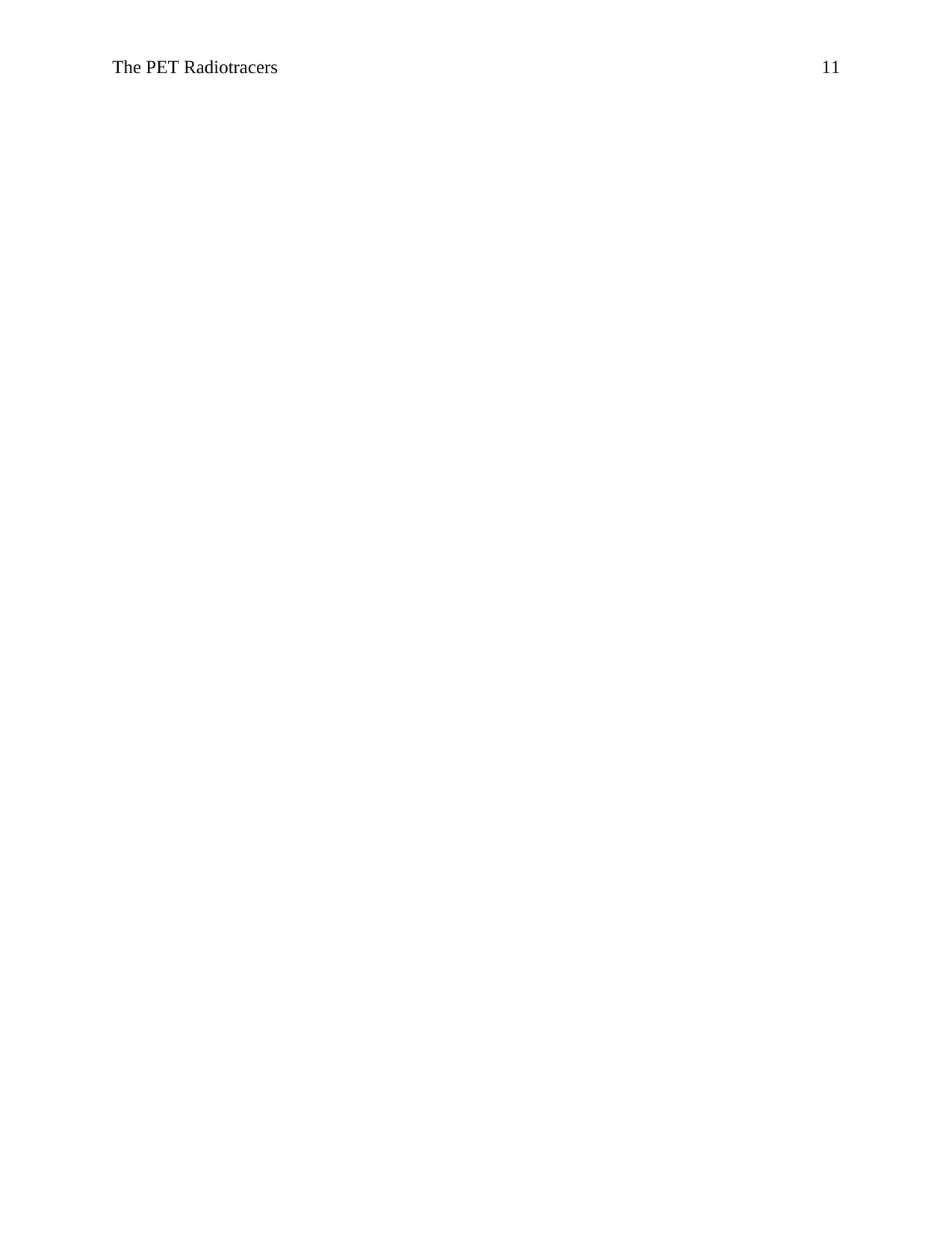
The PET Radiotracers 11
1 out of 11
![[object Object]](/_next/image/?url=%2F_next%2Fstatic%2Fmedia%2Flogo.6d15ce61.png&w=640&q=75)
Your All-in-One AI-Powered Toolkit for Academic Success.
+13062052269
info@desklib.com
Available 24*7 on WhatsApp / Email
Unlock your academic potential
© 2024 | Zucol Services PVT LTD | All rights reserved.