Pharmaceutical Preparations and their Testing
VerifiedAdded on 2023/04/05
|20
|5729
|407
AI Summary
This document provides information on pharmaceutical preparations and their testing. It covers topics such as quality aspects, physicochemical properties, manufacturing, specifications, stability, and finished medicinal products. The document also discusses the testing process and provides insights into the development of drug formulations.
Contribute Materials
Your contribution can guide someone’s learning journey. Share your
documents today.
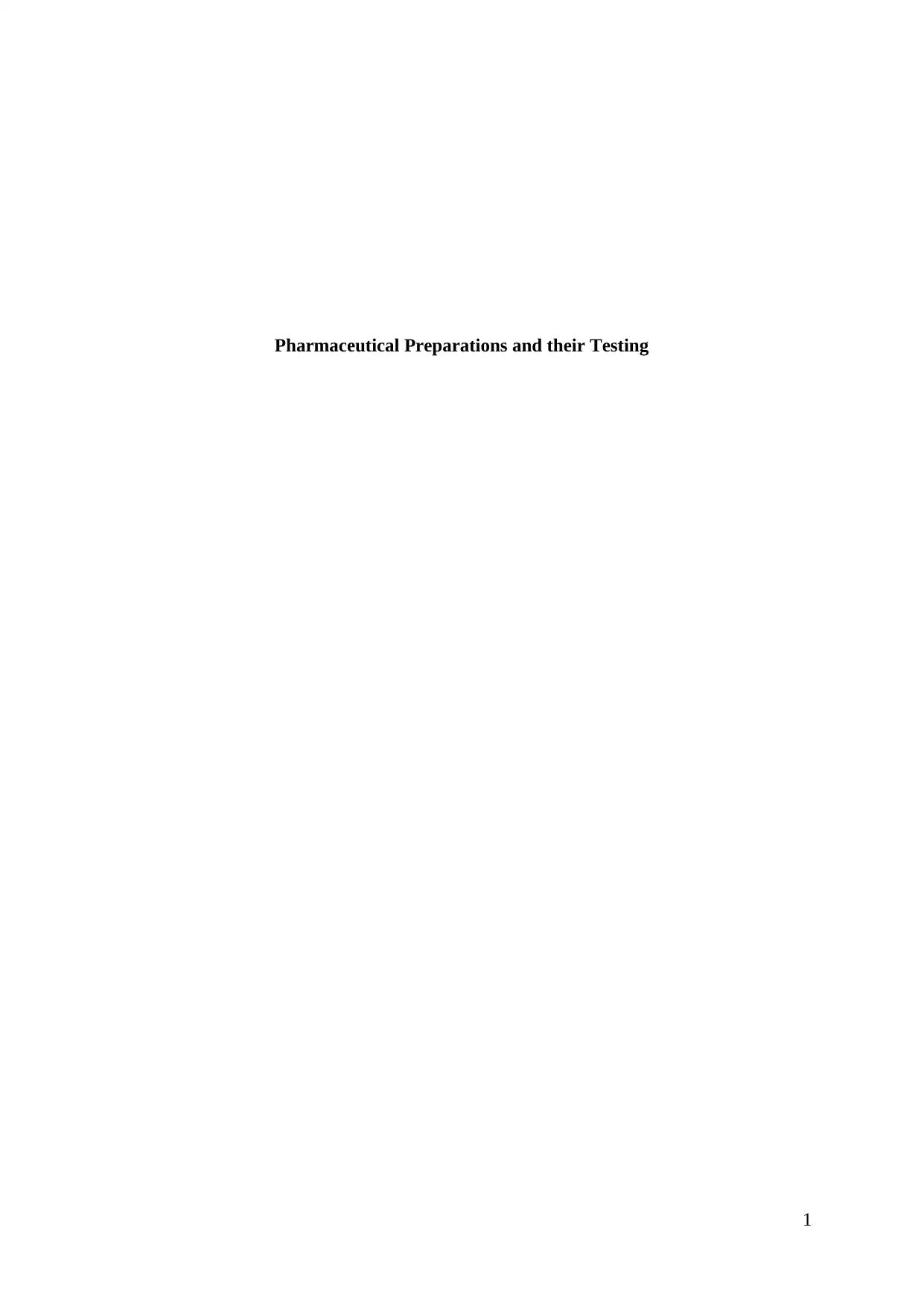
Pharmaceutical Preparations and their Testing
1
1
Secure Best Marks with AI Grader
Need help grading? Try our AI Grader for instant feedback on your assignments.
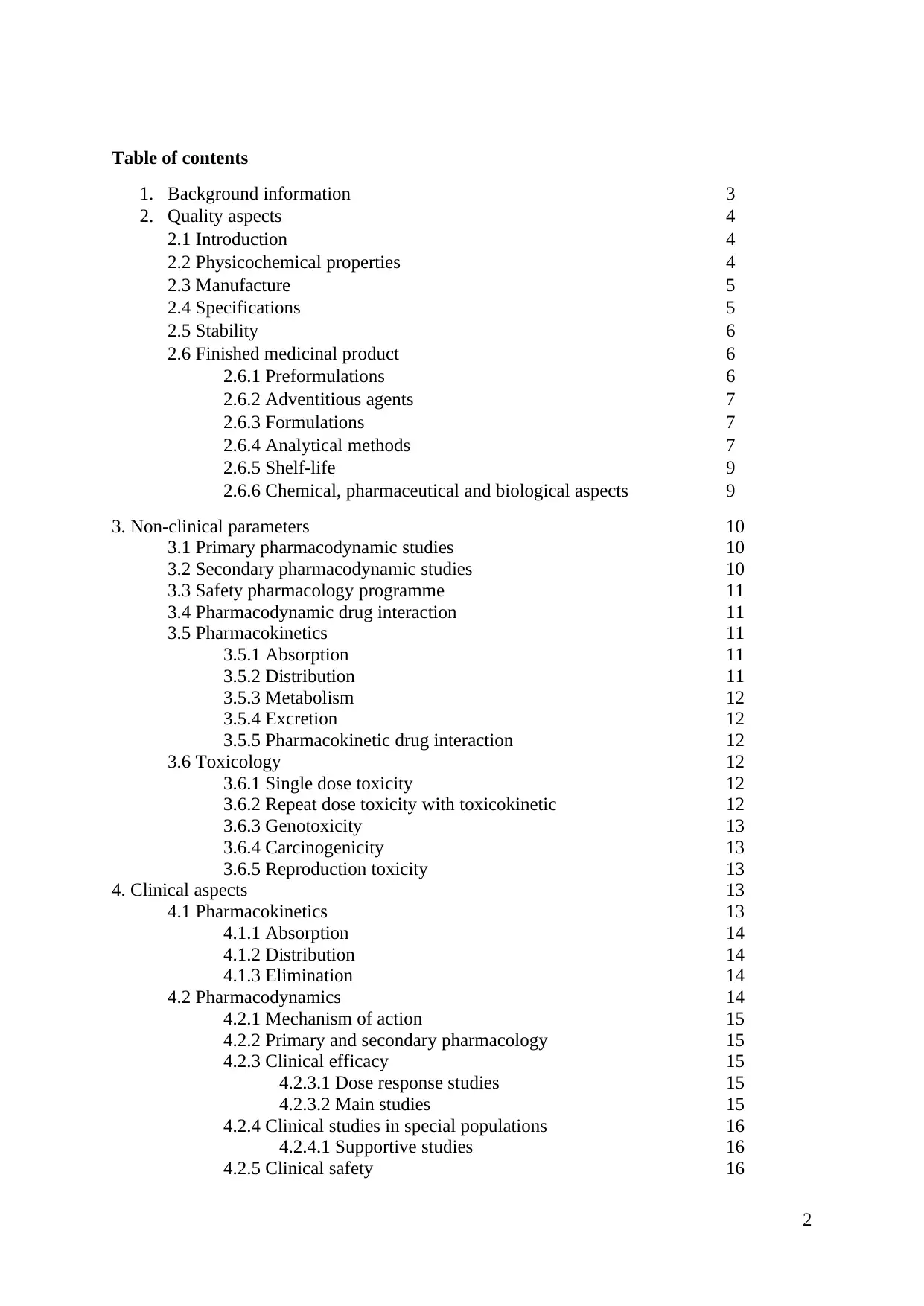
Table of contents
1. Background information 3
2. Quality aspects 4
2.1 Introduction 4
2.2 Physicochemical properties 4
2.3 Manufacture 5
2.4 Specifications 5
2.5 Stability 6
2.6 Finished medicinal product 6
2.6.1 Preformulations 6
2.6.2 Adventitious agents 7
2.6.3 Formulations 7
2.6.4 Analytical methods 7
2.6.5 Shelf-life 9
2.6.6 Chemical, pharmaceutical and biological aspects 9
3. Non-clinical parameters 10
3.1 Primary pharmacodynamic studies 10
3.2 Secondary pharmacodynamic studies 10
3.3 Safety pharmacology programme 11
3.4 Pharmacodynamic drug interaction 11
3.5 Pharmacokinetics 11
3.5.1 Absorption 11
3.5.2 Distribution 11
3.5.3 Metabolism 12
3.5.4 Excretion 12
3.5.5 Pharmacokinetic drug interaction 12
3.6 Toxicology 12
3.6.1 Single dose toxicity 12
3.6.2 Repeat dose toxicity with toxicokinetic 12
3.6.3 Genotoxicity 13
3.6.4 Carcinogenicity 13
3.6.5 Reproduction toxicity 13
4. Clinical aspects 13
4.1 Pharmacokinetics 13
4.1.1 Absorption 14
4.1.2 Distribution 14
4.1.3 Elimination 14
4.2 Pharmacodynamics 14
4.2.1 Mechanism of action 15
4.2.2 Primary and secondary pharmacology 15
4.2.3 Clinical efficacy 15
4.2.3.1 Dose response studies 15
4.2.3.2 Main studies 15
4.2.4 Clinical studies in special populations 16
4.2.4.1 Supportive studies 16
4.2.5 Clinical safety 16
2
1. Background information 3
2. Quality aspects 4
2.1 Introduction 4
2.2 Physicochemical properties 4
2.3 Manufacture 5
2.4 Specifications 5
2.5 Stability 6
2.6 Finished medicinal product 6
2.6.1 Preformulations 6
2.6.2 Adventitious agents 7
2.6.3 Formulations 7
2.6.4 Analytical methods 7
2.6.5 Shelf-life 9
2.6.6 Chemical, pharmaceutical and biological aspects 9
3. Non-clinical parameters 10
3.1 Primary pharmacodynamic studies 10
3.2 Secondary pharmacodynamic studies 10
3.3 Safety pharmacology programme 11
3.4 Pharmacodynamic drug interaction 11
3.5 Pharmacokinetics 11
3.5.1 Absorption 11
3.5.2 Distribution 11
3.5.3 Metabolism 12
3.5.4 Excretion 12
3.5.5 Pharmacokinetic drug interaction 12
3.6 Toxicology 12
3.6.1 Single dose toxicity 12
3.6.2 Repeat dose toxicity with toxicokinetic 12
3.6.3 Genotoxicity 13
3.6.4 Carcinogenicity 13
3.6.5 Reproduction toxicity 13
4. Clinical aspects 13
4.1 Pharmacokinetics 13
4.1.1 Absorption 14
4.1.2 Distribution 14
4.1.3 Elimination 14
4.2 Pharmacodynamics 14
4.2.1 Mechanism of action 15
4.2.2 Primary and secondary pharmacology 15
4.2.3 Clinical efficacy 15
4.2.3.1 Dose response studies 15
4.2.3.2 Main studies 15
4.2.4 Clinical studies in special populations 16
4.2.4.1 Supportive studies 16
4.2.5 Clinical safety 16
2
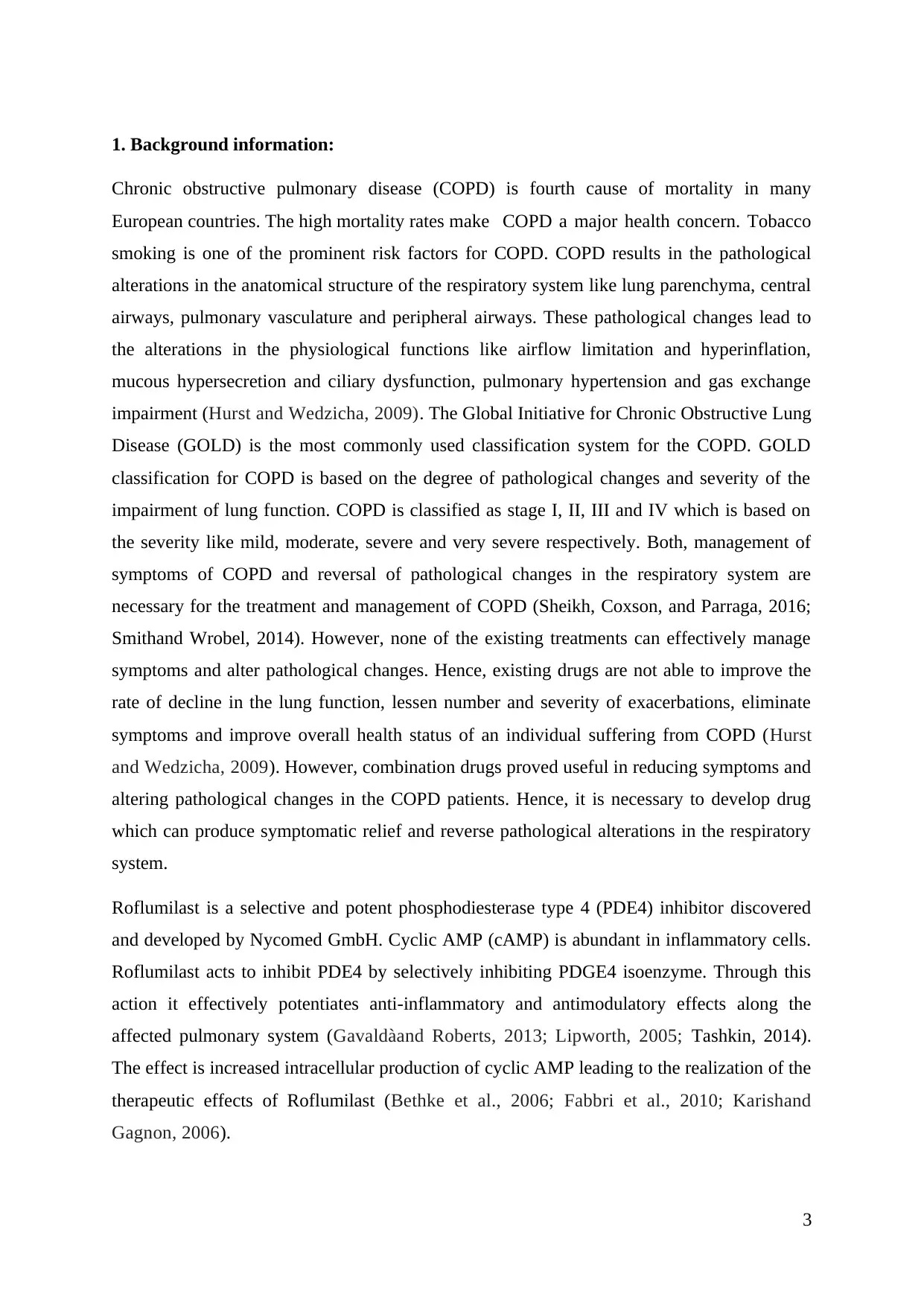
1. Background information:
Chronic obstructive pulmonary disease (COPD) is fourth cause of mortality in many
European countries. The high mortality rates make COPD a major health concern. Tobacco
smoking is one of the prominent risk factors for COPD. COPD results in the pathological
alterations in the anatomical structure of the respiratory system like lung parenchyma, central
airways, pulmonary vasculature and peripheral airways. These pathological changes lead to
the alterations in the physiological functions like airflow limitation and hyperinflation,
mucous hypersecretion and ciliary dysfunction, pulmonary hypertension and gas exchange
impairment (Hurst and Wedzicha, 2009). The Global Initiative for Chronic Obstructive Lung
Disease (GOLD) is the most commonly used classification system for the COPD. GOLD
classification for COPD is based on the degree of pathological changes and severity of the
impairment of lung function. COPD is classified as stage I, II, III and IV which is based on
the severity like mild, moderate, severe and very severe respectively. Both, management of
symptoms of COPD and reversal of pathological changes in the respiratory system are
necessary for the treatment and management of COPD (Sheikh, Coxson, and Parraga, 2016;
Smithand Wrobel, 2014). However, none of the existing treatments can effectively manage
symptoms and alter pathological changes. Hence, existing drugs are not able to improve the
rate of decline in the lung function, lessen number and severity of exacerbations, eliminate
symptoms and improve overall health status of an individual suffering from COPD (Hurst
and Wedzicha, 2009). However, combination drugs proved useful in reducing symptoms and
altering pathological changes in the COPD patients. Hence, it is necessary to develop drug
which can produce symptomatic relief and reverse pathological alterations in the respiratory
system.
Roflumilast is a selective and potent phosphodiesterase type 4 (PDE4) inhibitor discovered
and developed by Nycomed GmbH. Cyclic AMP (cAMP) is abundant in inflammatory cells.
Roflumilast acts to inhibit PDE4 by selectively inhibiting PDGE4 isoenzyme. Through this
action it effectively potentiates anti-inflammatory and antimodulatory effects along the
affected pulmonary system (Gavaldàand Roberts, 2013; Lipworth, 2005; Tashkin, 2014).
The effect is increased intracellular production of cyclic AMP leading to the realization of the
therapeutic effects of Roflumilast (Bethke et al., 2006; Fabbri et al., 2010; Karishand
Gagnon, 2006).
3
Chronic obstructive pulmonary disease (COPD) is fourth cause of mortality in many
European countries. The high mortality rates make COPD a major health concern. Tobacco
smoking is one of the prominent risk factors for COPD. COPD results in the pathological
alterations in the anatomical structure of the respiratory system like lung parenchyma, central
airways, pulmonary vasculature and peripheral airways. These pathological changes lead to
the alterations in the physiological functions like airflow limitation and hyperinflation,
mucous hypersecretion and ciliary dysfunction, pulmonary hypertension and gas exchange
impairment (Hurst and Wedzicha, 2009). The Global Initiative for Chronic Obstructive Lung
Disease (GOLD) is the most commonly used classification system for the COPD. GOLD
classification for COPD is based on the degree of pathological changes and severity of the
impairment of lung function. COPD is classified as stage I, II, III and IV which is based on
the severity like mild, moderate, severe and very severe respectively. Both, management of
symptoms of COPD and reversal of pathological changes in the respiratory system are
necessary for the treatment and management of COPD (Sheikh, Coxson, and Parraga, 2016;
Smithand Wrobel, 2014). However, none of the existing treatments can effectively manage
symptoms and alter pathological changes. Hence, existing drugs are not able to improve the
rate of decline in the lung function, lessen number and severity of exacerbations, eliminate
symptoms and improve overall health status of an individual suffering from COPD (Hurst
and Wedzicha, 2009). However, combination drugs proved useful in reducing symptoms and
altering pathological changes in the COPD patients. Hence, it is necessary to develop drug
which can produce symptomatic relief and reverse pathological alterations in the respiratory
system.
Roflumilast is a selective and potent phosphodiesterase type 4 (PDE4) inhibitor discovered
and developed by Nycomed GmbH. Cyclic AMP (cAMP) is abundant in inflammatory cells.
Roflumilast acts to inhibit PDE4 by selectively inhibiting PDGE4 isoenzyme. Through this
action it effectively potentiates anti-inflammatory and antimodulatory effects along the
affected pulmonary system (Gavaldàand Roberts, 2013; Lipworth, 2005; Tashkin, 2014).
The effect is increased intracellular production of cyclic AMP leading to the realization of the
therapeutic effects of Roflumilast (Bethke et al., 2006; Fabbri et al., 2010; Karishand
Gagnon, 2006).
3

2. Quality aspects:
2.1 Introduction:
The finished product would contain a 250 μg of active substance that is currently present in
the 500 μg Ruflumilast tablet. Roflumilast is formulated as a film-coated tablet (immediate
release) and was labelled as Daxas. Daxas contains 500 μg of active ingredient Roflumilast.
Daxas tablets are D-shaped and these are coated with a yellow non-active film coating. Daxas
tablets are embossed as “D” on the sides of the tablets. The ingredients of the Daxas tablet
include lactose monohydrate, maize starch, povidone (K90), and magnesium stearate. The
coating material of Daxas is composed of yellow Iron oxide (E72), Titanium dioxide (E71),
macrogol 4000, and hypromellose 2910. The packing material for Daxas tablets is either PVC
or PVDC aluminium blisters (Brück-Scheffler & Pontius, 2014).
2.2 Physicochemical properties:
Chemical name of Roflumilast is 3(cyclopropylmethoxy)-N-(3,5-dichloropyridin-4-yl)-4-
(difluoromethoxy) benzamide N-(3,5dichloropyridin-4-yl)-3-cyclopropylmethoxy-4-
difluoromethoxy-benzamide. The chemical structure of Roflumilast is as shown below.
Molecular formula of Roflumilast is C17H14Cl2F2N2O3
Molecular weight of Roflumilast is 403.207 g/mol.
It is a white powder.
Solubility:
Roflumilast is insoluble in water (0.52 – 0.56 mg/l at 22°C) and hexane. However, it is
sparingly soluble in ethanol. On the other hand, Roflumilast is freely soluble in acetone.
4
2.1 Introduction:
The finished product would contain a 250 μg of active substance that is currently present in
the 500 μg Ruflumilast tablet. Roflumilast is formulated as a film-coated tablet (immediate
release) and was labelled as Daxas. Daxas contains 500 μg of active ingredient Roflumilast.
Daxas tablets are D-shaped and these are coated with a yellow non-active film coating. Daxas
tablets are embossed as “D” on the sides of the tablets. The ingredients of the Daxas tablet
include lactose monohydrate, maize starch, povidone (K90), and magnesium stearate. The
coating material of Daxas is composed of yellow Iron oxide (E72), Titanium dioxide (E71),
macrogol 4000, and hypromellose 2910. The packing material for Daxas tablets is either PVC
or PVDC aluminium blisters (Brück-Scheffler & Pontius, 2014).
2.2 Physicochemical properties:
Chemical name of Roflumilast is 3(cyclopropylmethoxy)-N-(3,5-dichloropyridin-4-yl)-4-
(difluoromethoxy) benzamide N-(3,5dichloropyridin-4-yl)-3-cyclopropylmethoxy-4-
difluoromethoxy-benzamide. The chemical structure of Roflumilast is as shown below.
Molecular formula of Roflumilast is C17H14Cl2F2N2O3
Molecular weight of Roflumilast is 403.207 g/mol.
It is a white powder.
Solubility:
Roflumilast is insoluble in water (0.52 – 0.56 mg/l at 22°C) and hexane. However, it is
sparingly soluble in ethanol. On the other hand, Roflumilast is freely soluble in acetone.
4
Secure Best Marks with AI Grader
Need help grading? Try our AI Grader for instant feedback on your assignments.
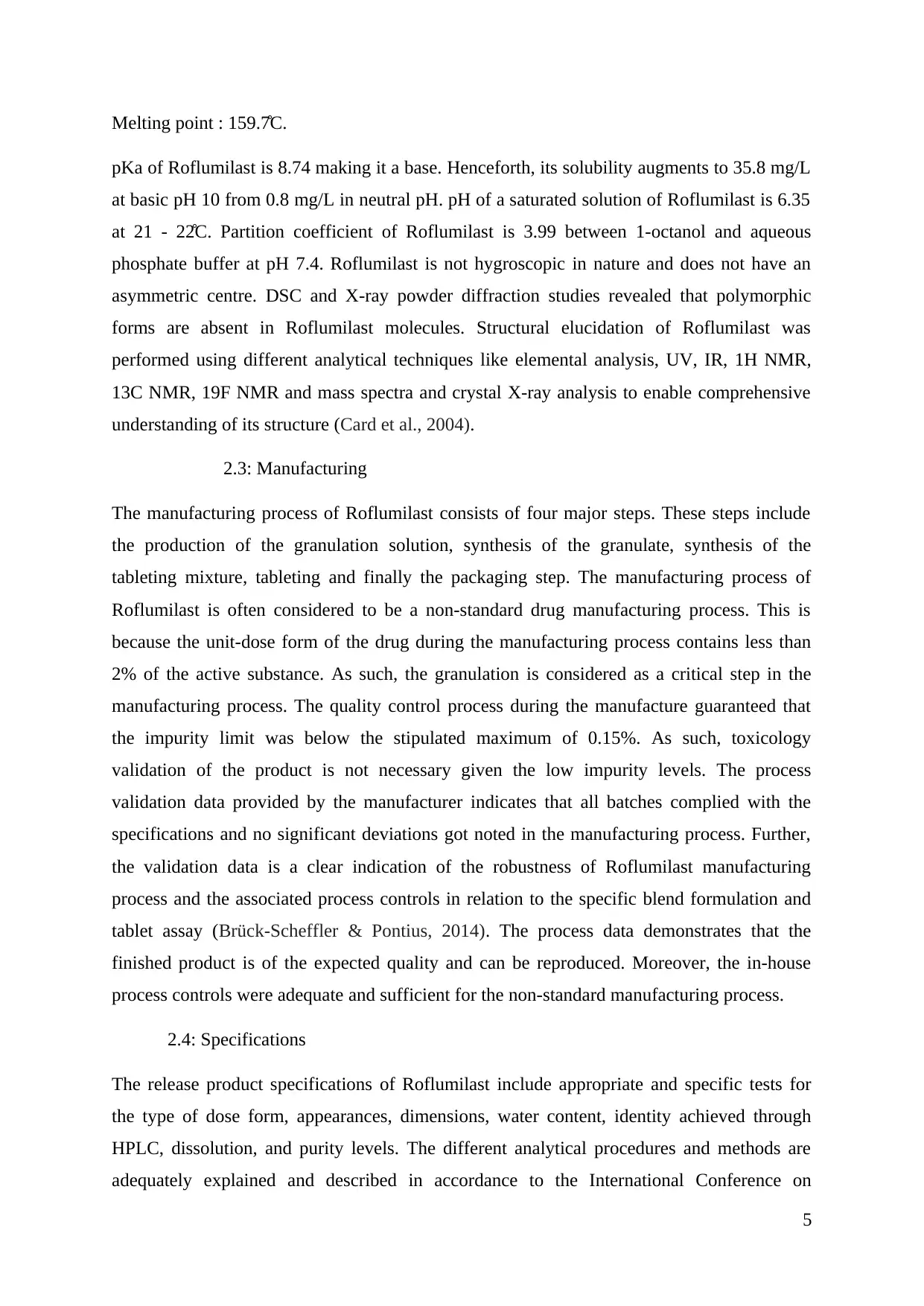
Melting point : 159.7̊C.
pKa of Roflumilast is 8.74 making it a base. Henceforth, its solubility augments to 35.8 mg/L
at basic pH 10 from 0.8 mg/L in neutral pH. pH of a saturated solution of Roflumilast is 6.35
at 21 - 22̊C. Partition coefficient of Roflumilast is 3.99 between 1-octanol and aqueous
phosphate buffer at pH 7.4. Roflumilast is not hygroscopic in nature and does not have an
asymmetric centre. DSC and X-ray powder diffraction studies revealed that polymorphic
forms are absent in Roflumilast molecules. Structural elucidation of Roflumilast was
performed using different analytical techniques like elemental analysis, UV, IR, 1H NMR,
13C NMR, 19F NMR and mass spectra and crystal X-ray analysis to enable comprehensive
understanding of its structure (Card et al., 2004).
2.3: Manufacturing
The manufacturing process of Roflumilast consists of four major steps. These steps include
the production of the granulation solution, synthesis of the granulate, synthesis of the
tableting mixture, tableting and finally the packaging step. The manufacturing process of
Roflumilast is often considered to be a non-standard drug manufacturing process. This is
because the unit-dose form of the drug during the manufacturing process contains less than
2% of the active substance. As such, the granulation is considered as a critical step in the
manufacturing process. The quality control process during the manufacture guaranteed that
the impurity limit was below the stipulated maximum of 0.15%. As such, toxicology
validation of the product is not necessary given the low impurity levels. The process
validation data provided by the manufacturer indicates that all batches complied with the
specifications and no significant deviations got noted in the manufacturing process. Further,
the validation data is a clear indication of the robustness of Roflumilast manufacturing
process and the associated process controls in relation to the specific blend formulation and
tablet assay (Brück-Scheffler & Pontius, 2014). The process data demonstrates that the
finished product is of the expected quality and can be reproduced. Moreover, the in-house
process controls were adequate and sufficient for the non-standard manufacturing process.
2.4: Specifications
The release product specifications of Roflumilast include appropriate and specific tests for
the type of dose form, appearances, dimensions, water content, identity achieved through
HPLC, dissolution, and purity levels. The different analytical procedures and methods are
adequately explained and described in accordance to the International Conference on
5
pKa of Roflumilast is 8.74 making it a base. Henceforth, its solubility augments to 35.8 mg/L
at basic pH 10 from 0.8 mg/L in neutral pH. pH of a saturated solution of Roflumilast is 6.35
at 21 - 22̊C. Partition coefficient of Roflumilast is 3.99 between 1-octanol and aqueous
phosphate buffer at pH 7.4. Roflumilast is not hygroscopic in nature and does not have an
asymmetric centre. DSC and X-ray powder diffraction studies revealed that polymorphic
forms are absent in Roflumilast molecules. Structural elucidation of Roflumilast was
performed using different analytical techniques like elemental analysis, UV, IR, 1H NMR,
13C NMR, 19F NMR and mass spectra and crystal X-ray analysis to enable comprehensive
understanding of its structure (Card et al., 2004).
2.3: Manufacturing
The manufacturing process of Roflumilast consists of four major steps. These steps include
the production of the granulation solution, synthesis of the granulate, synthesis of the
tableting mixture, tableting and finally the packaging step. The manufacturing process of
Roflumilast is often considered to be a non-standard drug manufacturing process. This is
because the unit-dose form of the drug during the manufacturing process contains less than
2% of the active substance. As such, the granulation is considered as a critical step in the
manufacturing process. The quality control process during the manufacture guaranteed that
the impurity limit was below the stipulated maximum of 0.15%. As such, toxicology
validation of the product is not necessary given the low impurity levels. The process
validation data provided by the manufacturer indicates that all batches complied with the
specifications and no significant deviations got noted in the manufacturing process. Further,
the validation data is a clear indication of the robustness of Roflumilast manufacturing
process and the associated process controls in relation to the specific blend formulation and
tablet assay (Brück-Scheffler & Pontius, 2014). The process data demonstrates that the
finished product is of the expected quality and can be reproduced. Moreover, the in-house
process controls were adequate and sufficient for the non-standard manufacturing process.
2.4: Specifications
The release product specifications of Roflumilast include appropriate and specific tests for
the type of dose form, appearances, dimensions, water content, identity achieved through
HPLC, dissolution, and purity levels. The different analytical procedures and methods are
adequately explained and described in accordance to the International Conference on
5
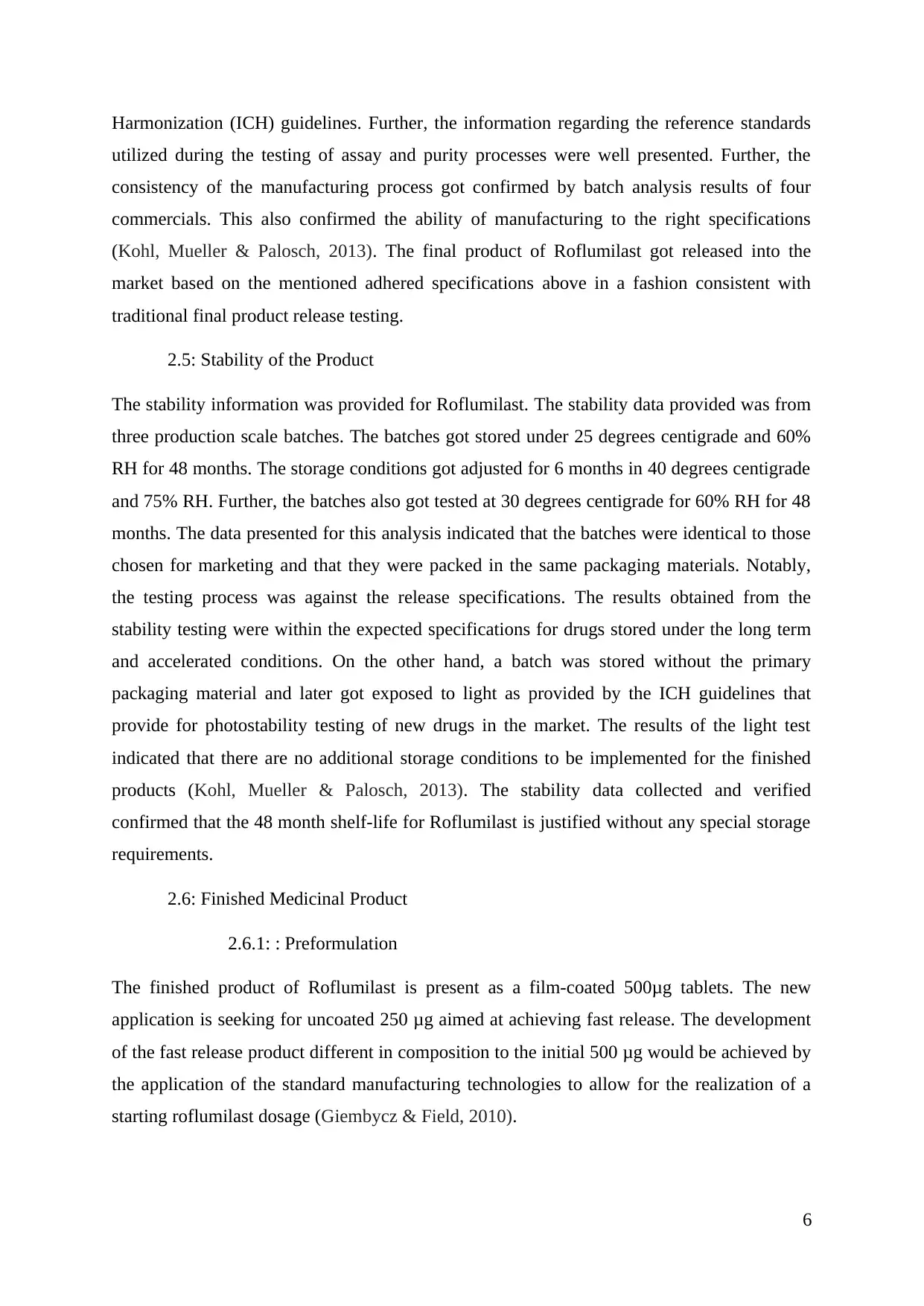
Harmonization (ICH) guidelines. Further, the information regarding the reference standards
utilized during the testing of assay and purity processes were well presented. Further, the
consistency of the manufacturing process got confirmed by batch analysis results of four
commercials. This also confirmed the ability of manufacturing to the right specifications
(Kohl, Mueller & Palosch, 2013). The final product of Roflumilast got released into the
market based on the mentioned adhered specifications above in a fashion consistent with
traditional final product release testing.
2.5: Stability of the Product
The stability information was provided for Roflumilast. The stability data provided was from
three production scale batches. The batches got stored under 25 degrees centigrade and 60%
RH for 48 months. The storage conditions got adjusted for 6 months in 40 degrees centigrade
and 75% RH. Further, the batches also got tested at 30 degrees centigrade for 60% RH for 48
months. The data presented for this analysis indicated that the batches were identical to those
chosen for marketing and that they were packed in the same packaging materials. Notably,
the testing process was against the release specifications. The results obtained from the
stability testing were within the expected specifications for drugs stored under the long term
and accelerated conditions. On the other hand, a batch was stored without the primary
packaging material and later got exposed to light as provided by the ICH guidelines that
provide for photostability testing of new drugs in the market. The results of the light test
indicated that there are no additional storage conditions to be implemented for the finished
products (Kohl, Mueller & Palosch, 2013). The stability data collected and verified
confirmed that the 48 month shelf-life for Roflumilast is justified without any special storage
requirements.
2.6: Finished Medicinal Product
2.6.1: : Preformulation
The finished product of Roflumilast is present as a film-coated 500μg tablets. The new
application is seeking for uncoated 250 μg aimed at achieving fast release. The development
of the fast release product different in composition to the initial 500 μg would be achieved by
the application of the standard manufacturing technologies to allow for the realization of a
starting roflumilast dosage (Giembycz & Field, 2010).
6
utilized during the testing of assay and purity processes were well presented. Further, the
consistency of the manufacturing process got confirmed by batch analysis results of four
commercials. This also confirmed the ability of manufacturing to the right specifications
(Kohl, Mueller & Palosch, 2013). The final product of Roflumilast got released into the
market based on the mentioned adhered specifications above in a fashion consistent with
traditional final product release testing.
2.5: Stability of the Product
The stability information was provided for Roflumilast. The stability data provided was from
three production scale batches. The batches got stored under 25 degrees centigrade and 60%
RH for 48 months. The storage conditions got adjusted for 6 months in 40 degrees centigrade
and 75% RH. Further, the batches also got tested at 30 degrees centigrade for 60% RH for 48
months. The data presented for this analysis indicated that the batches were identical to those
chosen for marketing and that they were packed in the same packaging materials. Notably,
the testing process was against the release specifications. The results obtained from the
stability testing were within the expected specifications for drugs stored under the long term
and accelerated conditions. On the other hand, a batch was stored without the primary
packaging material and later got exposed to light as provided by the ICH guidelines that
provide for photostability testing of new drugs in the market. The results of the light test
indicated that there are no additional storage conditions to be implemented for the finished
products (Kohl, Mueller & Palosch, 2013). The stability data collected and verified
confirmed that the 48 month shelf-life for Roflumilast is justified without any special storage
requirements.
2.6: Finished Medicinal Product
2.6.1: : Preformulation
The finished product of Roflumilast is present as a film-coated 500μg tablets. The new
application is seeking for uncoated 250 μg aimed at achieving fast release. The development
of the fast release product different in composition to the initial 500 μg would be achieved by
the application of the standard manufacturing technologies to allow for the realization of a
starting roflumilast dosage (Giembycz & Field, 2010).
6
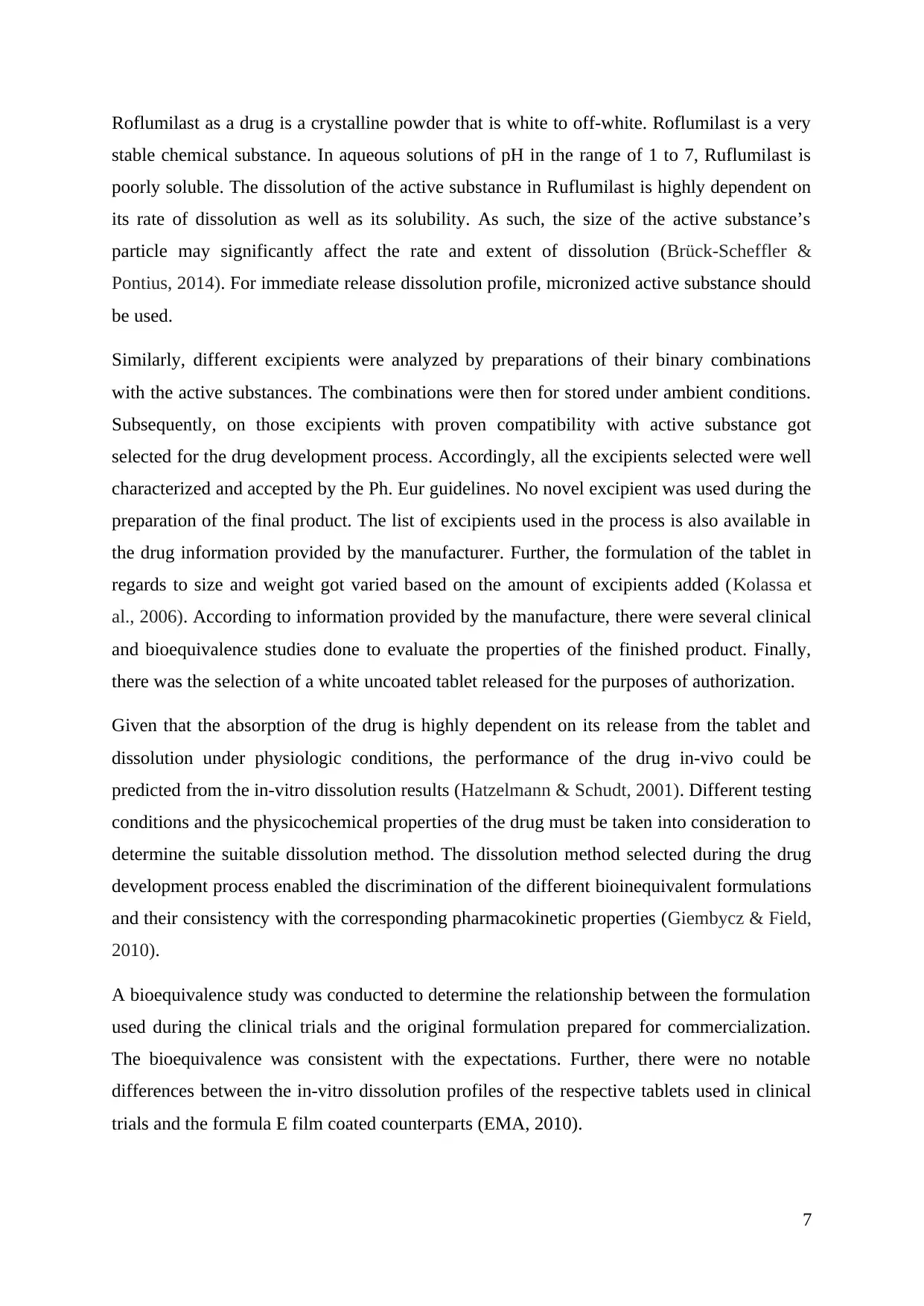
Roflumilast as a drug is a crystalline powder that is white to off-white. Roflumilast is a very
stable chemical substance. In aqueous solutions of pH in the range of 1 to 7, Ruflumilast is
poorly soluble. The dissolution of the active substance in Ruflumilast is highly dependent on
its rate of dissolution as well as its solubility. As such, the size of the active substance’s
particle may significantly affect the rate and extent of dissolution (Brück-Scheffler &
Pontius, 2014). For immediate release dissolution profile, micronized active substance should
be used.
Similarly, different excipients were analyzed by preparations of their binary combinations
with the active substances. The combinations were then for stored under ambient conditions.
Subsequently, on those excipients with proven compatibility with active substance got
selected for the drug development process. Accordingly, all the excipients selected were well
characterized and accepted by the Ph. Eur guidelines. No novel excipient was used during the
preparation of the final product. The list of excipients used in the process is also available in
the drug information provided by the manufacturer. Further, the formulation of the tablet in
regards to size and weight got varied based on the amount of excipients added (Kolassa et
al., 2006). According to information provided by the manufacture, there were several clinical
and bioequivalence studies done to evaluate the properties of the finished product. Finally,
there was the selection of a white uncoated tablet released for the purposes of authorization.
Given that the absorption of the drug is highly dependent on its release from the tablet and
dissolution under physiologic conditions, the performance of the drug in-vivo could be
predicted from the in-vitro dissolution results (Hatzelmann & Schudt, 2001). Different testing
conditions and the physicochemical properties of the drug must be taken into consideration to
determine the suitable dissolution method. The dissolution method selected during the drug
development process enabled the discrimination of the different bioinequivalent formulations
and their consistency with the corresponding pharmacokinetic properties (Giembycz & Field,
2010).
A bioequivalence study was conducted to determine the relationship between the formulation
used during the clinical trials and the original formulation prepared for commercialization.
The bioequivalence was consistent with the expectations. Further, there were no notable
differences between the in-vitro dissolution profiles of the respective tablets used in clinical
trials and the formula E film coated counterparts (EMA, 2010).
7
stable chemical substance. In aqueous solutions of pH in the range of 1 to 7, Ruflumilast is
poorly soluble. The dissolution of the active substance in Ruflumilast is highly dependent on
its rate of dissolution as well as its solubility. As such, the size of the active substance’s
particle may significantly affect the rate and extent of dissolution (Brück-Scheffler &
Pontius, 2014). For immediate release dissolution profile, micronized active substance should
be used.
Similarly, different excipients were analyzed by preparations of their binary combinations
with the active substances. The combinations were then for stored under ambient conditions.
Subsequently, on those excipients with proven compatibility with active substance got
selected for the drug development process. Accordingly, all the excipients selected were well
characterized and accepted by the Ph. Eur guidelines. No novel excipient was used during the
preparation of the final product. The list of excipients used in the process is also available in
the drug information provided by the manufacturer. Further, the formulation of the tablet in
regards to size and weight got varied based on the amount of excipients added (Kolassa et
al., 2006). According to information provided by the manufacture, there were several clinical
and bioequivalence studies done to evaluate the properties of the finished product. Finally,
there was the selection of a white uncoated tablet released for the purposes of authorization.
Given that the absorption of the drug is highly dependent on its release from the tablet and
dissolution under physiologic conditions, the performance of the drug in-vivo could be
predicted from the in-vitro dissolution results (Hatzelmann & Schudt, 2001). Different testing
conditions and the physicochemical properties of the drug must be taken into consideration to
determine the suitable dissolution method. The dissolution method selected during the drug
development process enabled the discrimination of the different bioinequivalent formulations
and their consistency with the corresponding pharmacokinetic properties (Giembycz & Field,
2010).
A bioequivalence study was conducted to determine the relationship between the formulation
used during the clinical trials and the original formulation prepared for commercialization.
The bioequivalence was consistent with the expectations. Further, there were no notable
differences between the in-vitro dissolution profiles of the respective tablets used in clinical
trials and the formula E film coated counterparts (EMA, 2010).
7
Paraphrase This Document
Need a fresh take? Get an instant paraphrase of this document with our AI Paraphraser
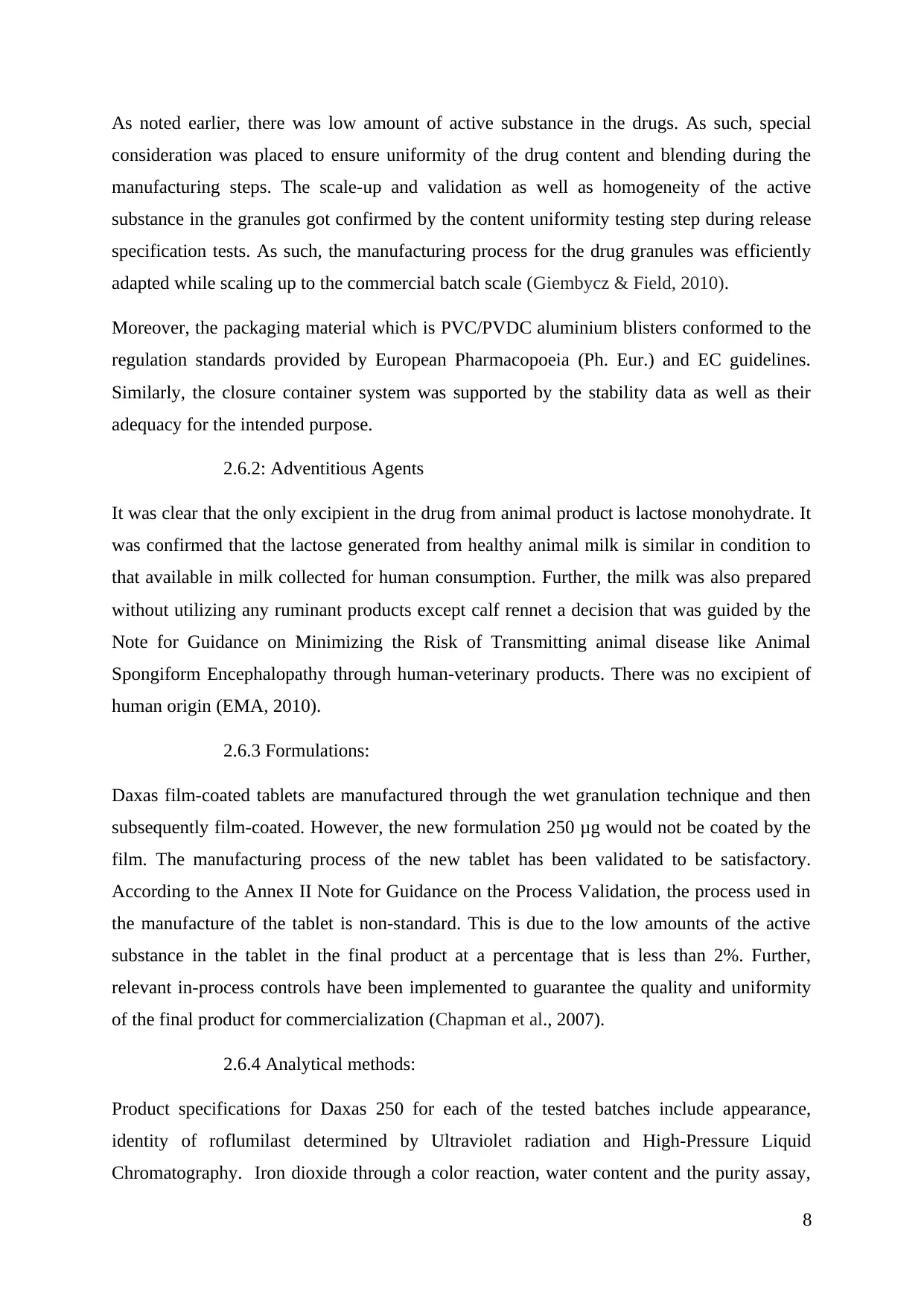
As noted earlier, there was low amount of active substance in the drugs. As such, special
consideration was placed to ensure uniformity of the drug content and blending during the
manufacturing steps. The scale-up and validation as well as homogeneity of the active
substance in the granules got confirmed by the content uniformity testing step during release
specification tests. As such, the manufacturing process for the drug granules was efficiently
adapted while scaling up to the commercial batch scale (Giembycz & Field, 2010).
Moreover, the packaging material which is PVC/PVDC aluminium blisters conformed to the
regulation standards provided by European Pharmacopoeia (Ph. Eur.) and EC guidelines.
Similarly, the closure container system was supported by the stability data as well as their
adequacy for the intended purpose.
2.6.2: Adventitious Agents
It was clear that the only excipient in the drug from animal product is lactose monohydrate. It
was confirmed that the lactose generated from healthy animal milk is similar in condition to
that available in milk collected for human consumption. Further, the milk was also prepared
without utilizing any ruminant products except calf rennet a decision that was guided by the
Note for Guidance on Minimizing the Risk of Transmitting animal disease like Animal
Spongiform Encephalopathy through human-veterinary products. There was no excipient of
human origin (EMA, 2010).
2.6.3 Formulations:
Daxas film-coated tablets are manufactured through the wet granulation technique and then
subsequently film-coated. However, the new formulation 250 μg would not be coated by the
film. The manufacturing process of the new tablet has been validated to be satisfactory.
According to the Annex II Note for Guidance on the Process Validation, the process used in
the manufacture of the tablet is non-standard. This is due to the low amounts of the active
substance in the tablet in the final product at a percentage that is less than 2%. Further,
relevant in-process controls have been implemented to guarantee the quality and uniformity
of the final product for commercialization (Chapman et al., 2007).
2.6.4 Analytical methods:
Product specifications for Daxas 250 for each of the tested batches include appearance,
identity of roflumilast determined by Ultraviolet radiation and High-Pressure Liquid
Chromatography. Iron dioxide through a color reaction, water content and the purity assay,
8
consideration was placed to ensure uniformity of the drug content and blending during the
manufacturing steps. The scale-up and validation as well as homogeneity of the active
substance in the granules got confirmed by the content uniformity testing step during release
specification tests. As such, the manufacturing process for the drug granules was efficiently
adapted while scaling up to the commercial batch scale (Giembycz & Field, 2010).
Moreover, the packaging material which is PVC/PVDC aluminium blisters conformed to the
regulation standards provided by European Pharmacopoeia (Ph. Eur.) and EC guidelines.
Similarly, the closure container system was supported by the stability data as well as their
adequacy for the intended purpose.
2.6.2: Adventitious Agents
It was clear that the only excipient in the drug from animal product is lactose monohydrate. It
was confirmed that the lactose generated from healthy animal milk is similar in condition to
that available in milk collected for human consumption. Further, the milk was also prepared
without utilizing any ruminant products except calf rennet a decision that was guided by the
Note for Guidance on Minimizing the Risk of Transmitting animal disease like Animal
Spongiform Encephalopathy through human-veterinary products. There was no excipient of
human origin (EMA, 2010).
2.6.3 Formulations:
Daxas film-coated tablets are manufactured through the wet granulation technique and then
subsequently film-coated. However, the new formulation 250 μg would not be coated by the
film. The manufacturing process of the new tablet has been validated to be satisfactory.
According to the Annex II Note for Guidance on the Process Validation, the process used in
the manufacture of the tablet is non-standard. This is due to the low amounts of the active
substance in the tablet in the final product at a percentage that is less than 2%. Further,
relevant in-process controls have been implemented to guarantee the quality and uniformity
of the final product for commercialization (Chapman et al., 2007).
2.6.4 Analytical methods:
Product specifications for Daxas 250 for each of the tested batches include appearance,
identity of roflumilast determined by Ultraviolet radiation and High-Pressure Liquid
Chromatography. Iron dioxide through a color reaction, water content and the purity assay,
8
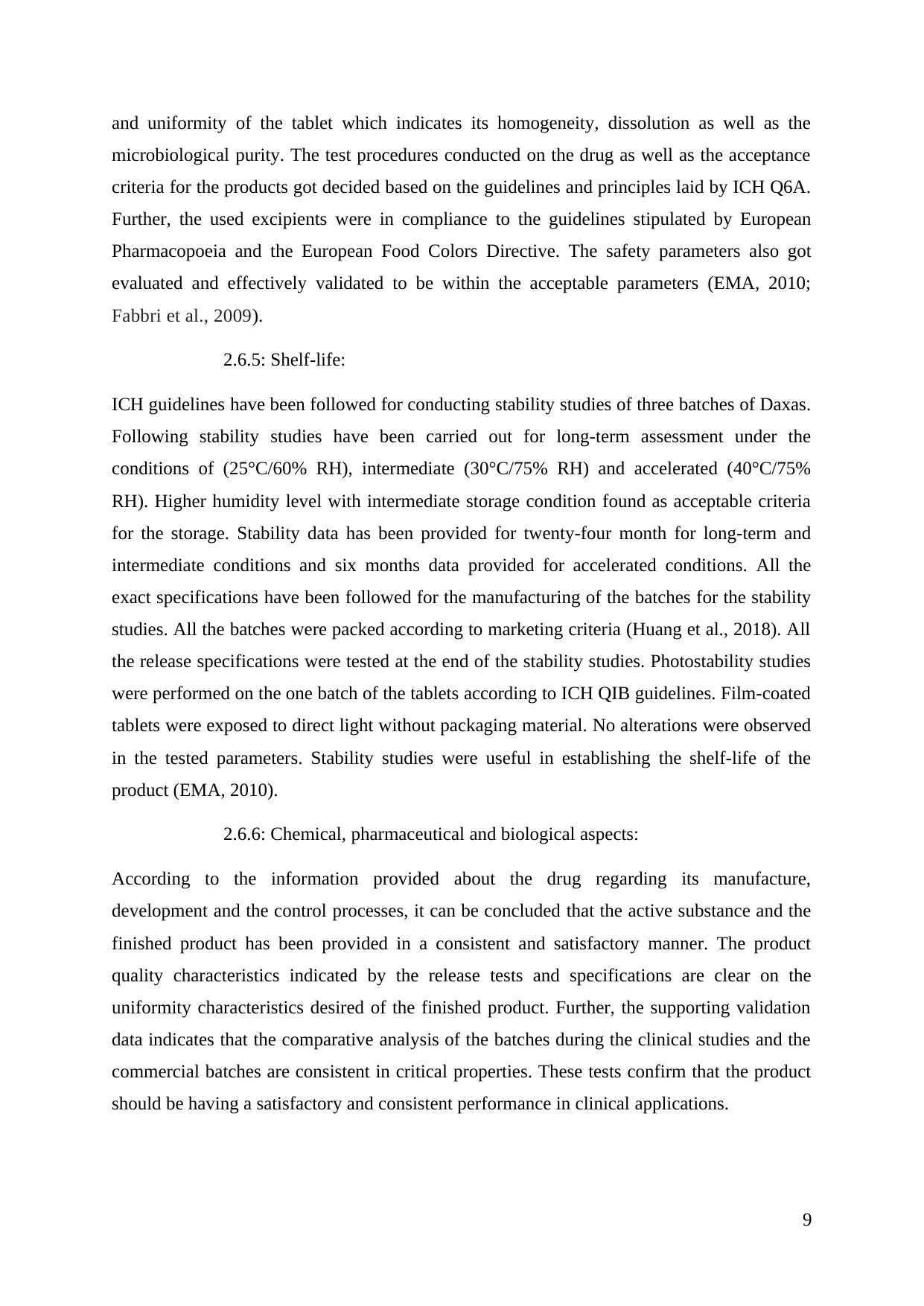
and uniformity of the tablet which indicates its homogeneity, dissolution as well as the
microbiological purity. The test procedures conducted on the drug as well as the acceptance
criteria for the products got decided based on the guidelines and principles laid by ICH Q6A.
Further, the used excipients were in compliance to the guidelines stipulated by European
Pharmacopoeia and the European Food Colors Directive. The safety parameters also got
evaluated and effectively validated to be within the acceptable parameters (EMA, 2010;
Fabbri et al., 2009).
2.6.5: Shelf-life:
ICH guidelines have been followed for conducting stability studies of three batches of Daxas.
Following stability studies have been carried out for long-term assessment under the
conditions of (25°C/60% RH), intermediate (30°C/75% RH) and accelerated (40°C/75%
RH). Higher humidity level with intermediate storage condition found as acceptable criteria
for the storage. Stability data has been provided for twenty-four month for long-term and
intermediate conditions and six months data provided for accelerated conditions. All the
exact specifications have been followed for the manufacturing of the batches for the stability
studies. All the batches were packed according to marketing criteria (Huang et al., 2018). All
the release specifications were tested at the end of the stability studies. Photostability studies
were performed on the one batch of the tablets according to ICH QIB guidelines. Film-coated
tablets were exposed to direct light without packaging material. No alterations were observed
in the tested parameters. Stability studies were useful in establishing the shelf-life of the
product (EMA, 2010).
2.6.6: Chemical, pharmaceutical and biological aspects:
According to the information provided about the drug regarding its manufacture,
development and the control processes, it can be concluded that the active substance and the
finished product has been provided in a consistent and satisfactory manner. The product
quality characteristics indicated by the release tests and specifications are clear on the
uniformity characteristics desired of the finished product. Further, the supporting validation
data indicates that the comparative analysis of the batches during the clinical studies and the
commercial batches are consistent in critical properties. These tests confirm that the product
should be having a satisfactory and consistent performance in clinical applications.
9
microbiological purity. The test procedures conducted on the drug as well as the acceptance
criteria for the products got decided based on the guidelines and principles laid by ICH Q6A.
Further, the used excipients were in compliance to the guidelines stipulated by European
Pharmacopoeia and the European Food Colors Directive. The safety parameters also got
evaluated and effectively validated to be within the acceptable parameters (EMA, 2010;
Fabbri et al., 2009).
2.6.5: Shelf-life:
ICH guidelines have been followed for conducting stability studies of three batches of Daxas.
Following stability studies have been carried out for long-term assessment under the
conditions of (25°C/60% RH), intermediate (30°C/75% RH) and accelerated (40°C/75%
RH). Higher humidity level with intermediate storage condition found as acceptable criteria
for the storage. Stability data has been provided for twenty-four month for long-term and
intermediate conditions and six months data provided for accelerated conditions. All the
exact specifications have been followed for the manufacturing of the batches for the stability
studies. All the batches were packed according to marketing criteria (Huang et al., 2018). All
the release specifications were tested at the end of the stability studies. Photostability studies
were performed on the one batch of the tablets according to ICH QIB guidelines. Film-coated
tablets were exposed to direct light without packaging material. No alterations were observed
in the tested parameters. Stability studies were useful in establishing the shelf-life of the
product (EMA, 2010).
2.6.6: Chemical, pharmaceutical and biological aspects:
According to the information provided about the drug regarding its manufacture,
development and the control processes, it can be concluded that the active substance and the
finished product has been provided in a consistent and satisfactory manner. The product
quality characteristics indicated by the release tests and specifications are clear on the
uniformity characteristics desired of the finished product. Further, the supporting validation
data indicates that the comparative analysis of the batches during the clinical studies and the
commercial batches are consistent in critical properties. These tests confirm that the product
should be having a satisfactory and consistent performance in clinical applications.
9
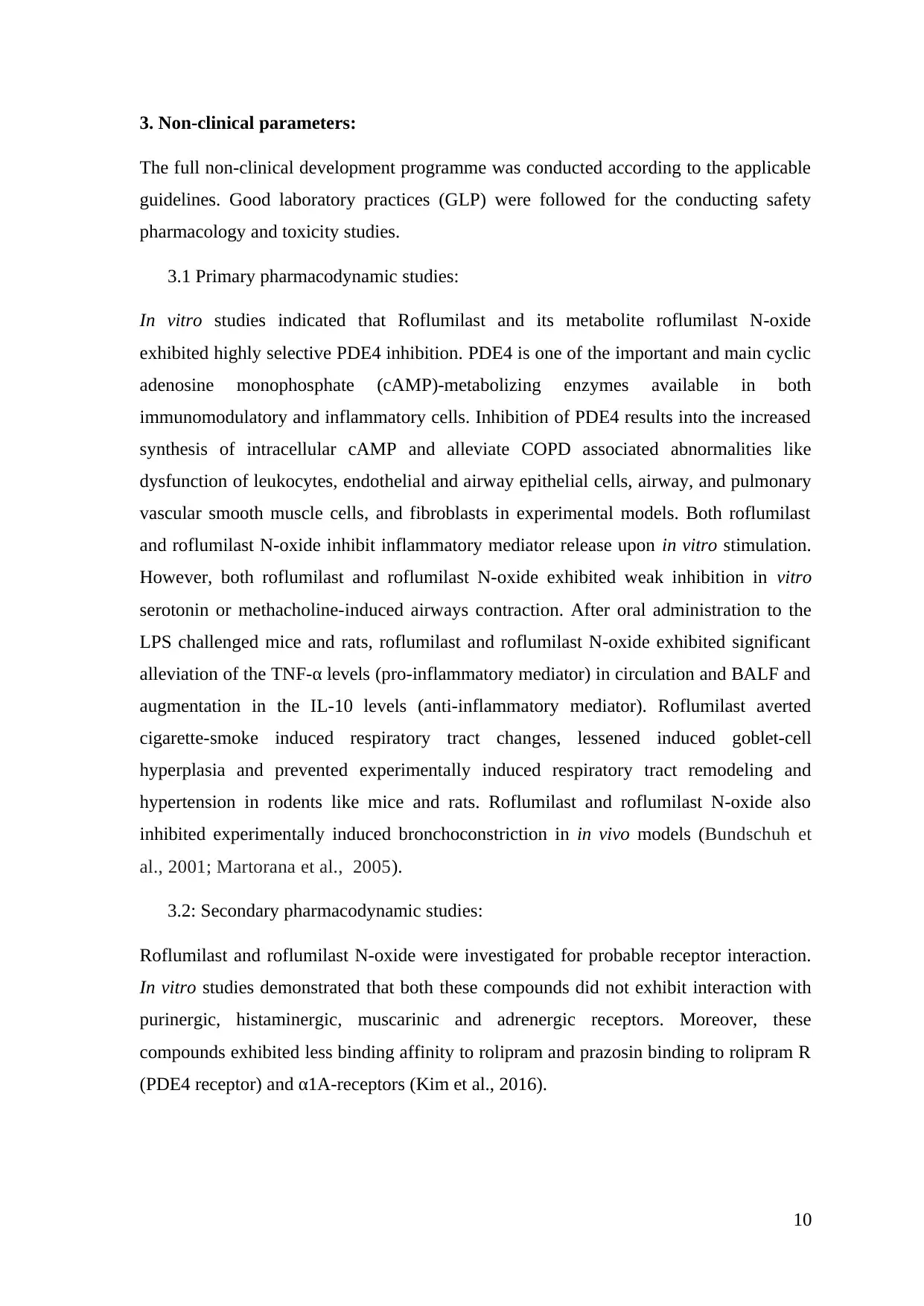
3. Non-clinical parameters:
The full non-clinical development programme was conducted according to the applicable
guidelines. Good laboratory practices (GLP) were followed for the conducting safety
pharmacology and toxicity studies.
3.1 Primary pharmacodynamic studies:
In vitro studies indicated that Roflumilast and its metabolite roflumilast N-oxide
exhibited highly selective PDE4 inhibition. PDE4 is one of the important and main cyclic
adenosine monophosphate (cAMP)-metabolizing enzymes available in both
immunomodulatory and inflammatory cells. Inhibition of PDE4 results into the increased
synthesis of intracellular cAMP and alleviate COPD associated abnormalities like
dysfunction of leukocytes, endothelial and airway epithelial cells, airway, and pulmonary
vascular smooth muscle cells, and fibroblasts in experimental models. Both roflumilast
and roflumilast N-oxide inhibit inflammatory mediator release upon in vitro stimulation.
However, both roflumilast and roflumilast N-oxide exhibited weak inhibition in vitro
serotonin or methacholine-induced airways contraction. After oral administration to the
LPS challenged mice and rats, roflumilast and roflumilast N-oxide exhibited significant
alleviation of the TNF-α levels (pro-inflammatory mediator) in circulation and BALF and
augmentation in the IL-10 levels (anti-inflammatory mediator). Roflumilast averted
cigarette-smoke induced respiratory tract changes, lessened induced goblet-cell
hyperplasia and prevented experimentally induced respiratory tract remodeling and
hypertension in rodents like mice and rats. Roflumilast and roflumilast N-oxide also
inhibited experimentally induced bronchoconstriction in in vivo models (Bundschuh et
al., 2001; Martorana et al., 2005).
3.2: Secondary pharmacodynamic studies:
Roflumilast and roflumilast N-oxide were investigated for probable receptor interaction.
In vitro studies demonstrated that both these compounds did not exhibit interaction with
purinergic, histaminergic, muscarinic and adrenergic receptors. Moreover, these
compounds exhibited less binding affinity to rolipram and prazosin binding to rolipram R
(PDE4 receptor) and α1A-receptors (Kim et al., 2016).
10
The full non-clinical development programme was conducted according to the applicable
guidelines. Good laboratory practices (GLP) were followed for the conducting safety
pharmacology and toxicity studies.
3.1 Primary pharmacodynamic studies:
In vitro studies indicated that Roflumilast and its metabolite roflumilast N-oxide
exhibited highly selective PDE4 inhibition. PDE4 is one of the important and main cyclic
adenosine monophosphate (cAMP)-metabolizing enzymes available in both
immunomodulatory and inflammatory cells. Inhibition of PDE4 results into the increased
synthesis of intracellular cAMP and alleviate COPD associated abnormalities like
dysfunction of leukocytes, endothelial and airway epithelial cells, airway, and pulmonary
vascular smooth muscle cells, and fibroblasts in experimental models. Both roflumilast
and roflumilast N-oxide inhibit inflammatory mediator release upon in vitro stimulation.
However, both roflumilast and roflumilast N-oxide exhibited weak inhibition in vitro
serotonin or methacholine-induced airways contraction. After oral administration to the
LPS challenged mice and rats, roflumilast and roflumilast N-oxide exhibited significant
alleviation of the TNF-α levels (pro-inflammatory mediator) in circulation and BALF and
augmentation in the IL-10 levels (anti-inflammatory mediator). Roflumilast averted
cigarette-smoke induced respiratory tract changes, lessened induced goblet-cell
hyperplasia and prevented experimentally induced respiratory tract remodeling and
hypertension in rodents like mice and rats. Roflumilast and roflumilast N-oxide also
inhibited experimentally induced bronchoconstriction in in vivo models (Bundschuh et
al., 2001; Martorana et al., 2005).
3.2: Secondary pharmacodynamic studies:
Roflumilast and roflumilast N-oxide were investigated for probable receptor interaction.
In vitro studies demonstrated that both these compounds did not exhibit interaction with
purinergic, histaminergic, muscarinic and adrenergic receptors. Moreover, these
compounds exhibited less binding affinity to rolipram and prazosin binding to rolipram R
(PDE4 receptor) and α1A-receptors (Kim et al., 2016).
10
Secure Best Marks with AI Grader
Need help grading? Try our AI Grader for instant feedback on your assignments.
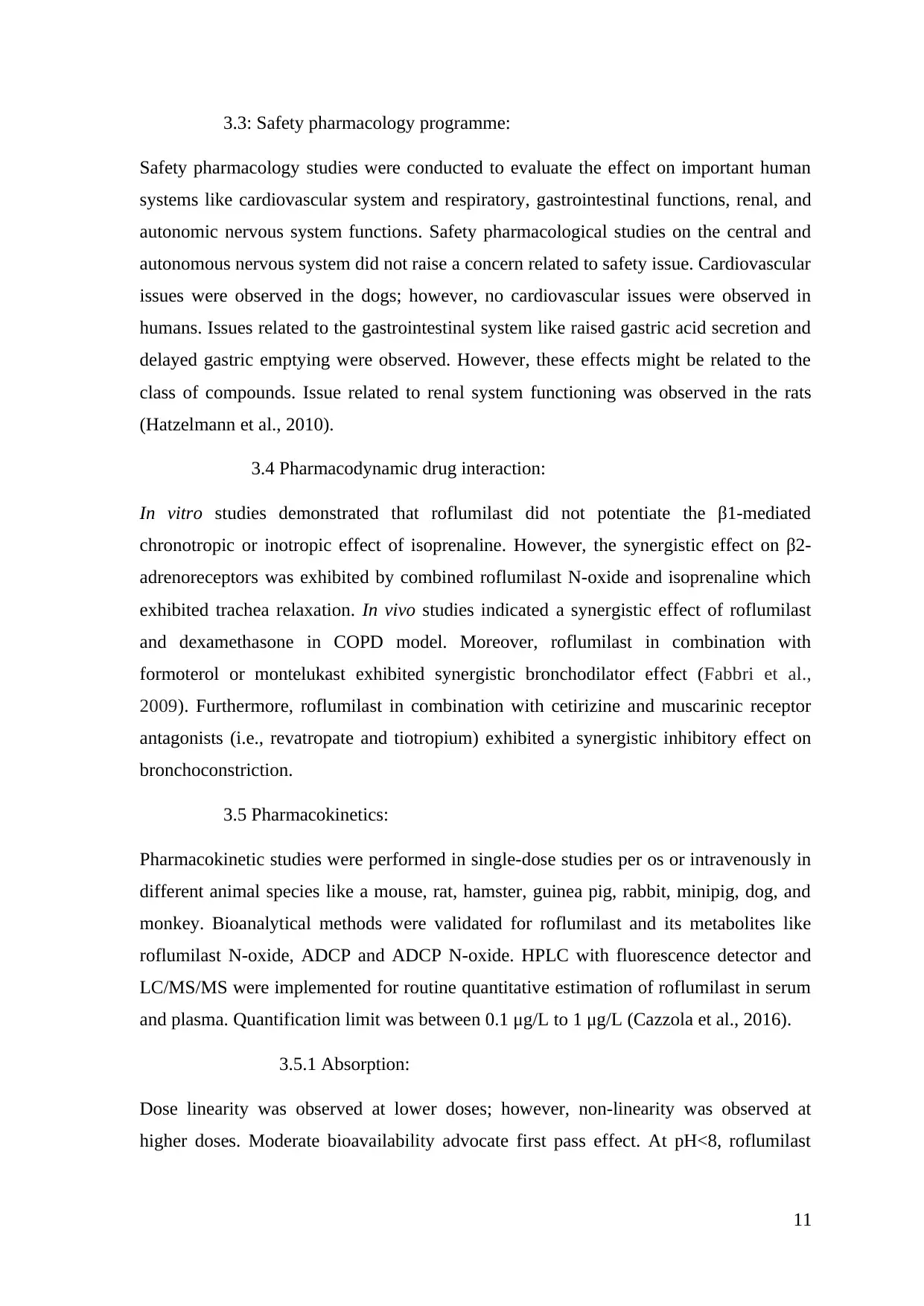
3.3: Safety pharmacology programme:
Safety pharmacology studies were conducted to evaluate the effect on important human
systems like cardiovascular system and respiratory, gastrointestinal functions, renal, and
autonomic nervous system functions. Safety pharmacological studies on the central and
autonomous nervous system did not raise a concern related to safety issue. Cardiovascular
issues were observed in the dogs; however, no cardiovascular issues were observed in
humans. Issues related to the gastrointestinal system like raised gastric acid secretion and
delayed gastric emptying were observed. However, these effects might be related to the
class of compounds. Issue related to renal system functioning was observed in the rats
(Hatzelmann et al., 2010).
3.4 Pharmacodynamic drug interaction:
In vitro studies demonstrated that roflumilast did not potentiate the β1-mediated
chronotropic or inotropic effect of isoprenaline. However, the synergistic effect on β2-
adrenoreceptors was exhibited by combined roflumilast N-oxide and isoprenaline which
exhibited trachea relaxation. In vivo studies indicated a synergistic effect of roflumilast
and dexamethasone in COPD model. Moreover, roflumilast in combination with
formoterol or montelukast exhibited synergistic bronchodilator effect (Fabbri et al.,
2009). Furthermore, roflumilast in combination with cetirizine and muscarinic receptor
antagonists (i.e., revatropate and tiotropium) exhibited a synergistic inhibitory effect on
bronchoconstriction.
3.5 Pharmacokinetics:
Pharmacokinetic studies were performed in single-dose studies per os or intravenously in
different animal species like a mouse, rat, hamster, guinea pig, rabbit, minipig, dog, and
monkey. Bioanalytical methods were validated for roflumilast and its metabolites like
roflumilast N-oxide, ADCP and ADCP N-oxide. HPLC with fluorescence detector and
LC/MS/MS were implemented for routine quantitative estimation of roflumilast in serum
and plasma. Quantification limit was between 0.1 μg/L to 1 μg/L (Cazzola et al., 2016).
3.5.1 Absorption:
Dose linearity was observed at lower doses; however, non-linearity was observed at
higher doses. Moderate bioavailability advocate first pass effect. At pH<8, roflumilast
11
Safety pharmacology studies were conducted to evaluate the effect on important human
systems like cardiovascular system and respiratory, gastrointestinal functions, renal, and
autonomic nervous system functions. Safety pharmacological studies on the central and
autonomous nervous system did not raise a concern related to safety issue. Cardiovascular
issues were observed in the dogs; however, no cardiovascular issues were observed in
humans. Issues related to the gastrointestinal system like raised gastric acid secretion and
delayed gastric emptying were observed. However, these effects might be related to the
class of compounds. Issue related to renal system functioning was observed in the rats
(Hatzelmann et al., 2010).
3.4 Pharmacodynamic drug interaction:
In vitro studies demonstrated that roflumilast did not potentiate the β1-mediated
chronotropic or inotropic effect of isoprenaline. However, the synergistic effect on β2-
adrenoreceptors was exhibited by combined roflumilast N-oxide and isoprenaline which
exhibited trachea relaxation. In vivo studies indicated a synergistic effect of roflumilast
and dexamethasone in COPD model. Moreover, roflumilast in combination with
formoterol or montelukast exhibited synergistic bronchodilator effect (Fabbri et al.,
2009). Furthermore, roflumilast in combination with cetirizine and muscarinic receptor
antagonists (i.e., revatropate and tiotropium) exhibited a synergistic inhibitory effect on
bronchoconstriction.
3.5 Pharmacokinetics:
Pharmacokinetic studies were performed in single-dose studies per os or intravenously in
different animal species like a mouse, rat, hamster, guinea pig, rabbit, minipig, dog, and
monkey. Bioanalytical methods were validated for roflumilast and its metabolites like
roflumilast N-oxide, ADCP and ADCP N-oxide. HPLC with fluorescence detector and
LC/MS/MS were implemented for routine quantitative estimation of roflumilast in serum
and plasma. Quantification limit was between 0.1 μg/L to 1 μg/L (Cazzola et al., 2016).
3.5.1 Absorption:
Dose linearity was observed at lower doses; however, non-linearity was observed at
higher doses. Moderate bioavailability advocate first pass effect. At pH<8, roflumilast
11
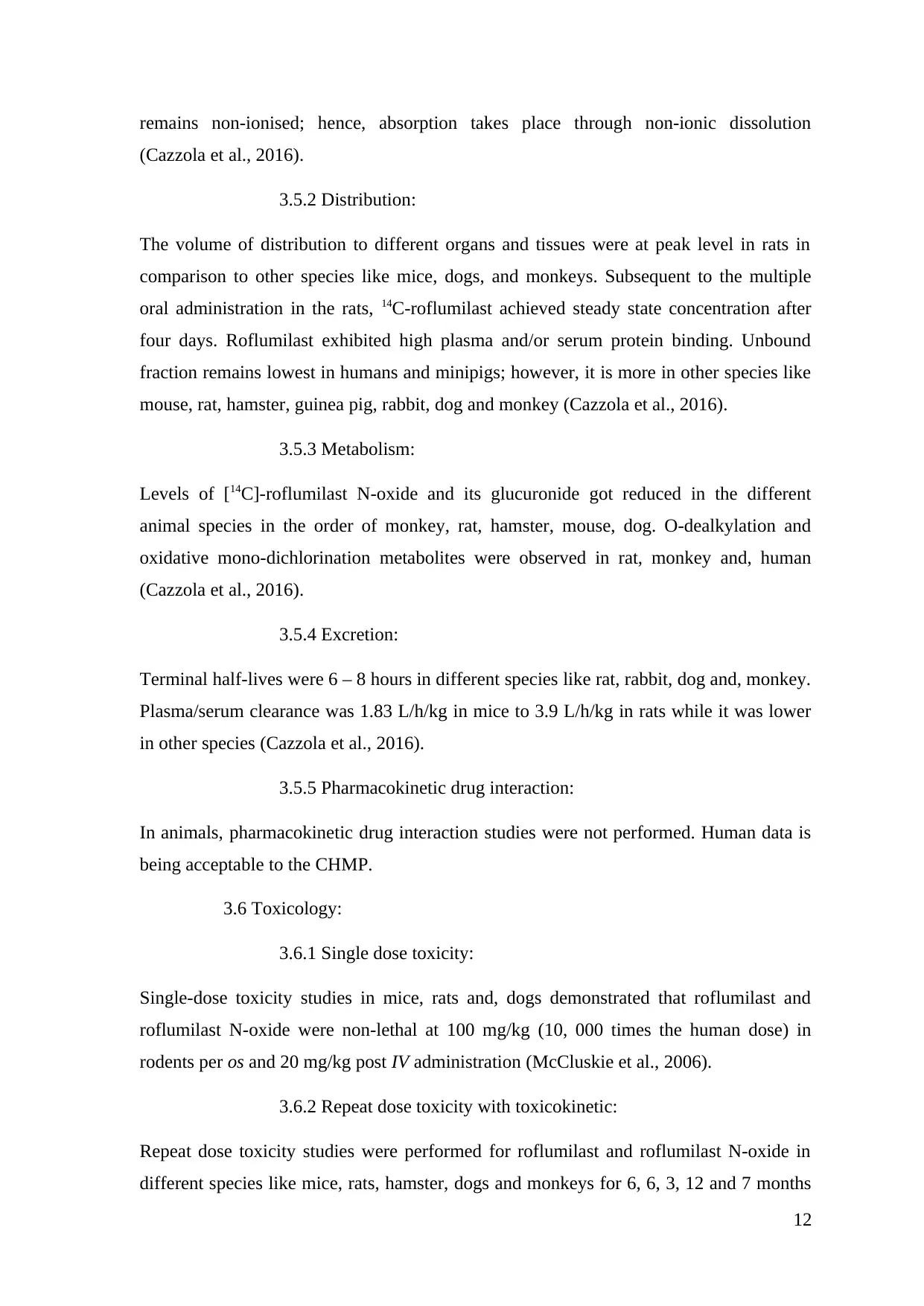
remains non-ionised; hence, absorption takes place through non-ionic dissolution
(Cazzola et al., 2016).
3.5.2 Distribution:
The volume of distribution to different organs and tissues were at peak level in rats in
comparison to other species like mice, dogs, and monkeys. Subsequent to the multiple
oral administration in the rats, 14C-roflumilast achieved steady state concentration after
four days. Roflumilast exhibited high plasma and/or serum protein binding. Unbound
fraction remains lowest in humans and minipigs; however, it is more in other species like
mouse, rat, hamster, guinea pig, rabbit, dog and monkey (Cazzola et al., 2016).
3.5.3 Metabolism:
Levels of [14C]-roflumilast N-oxide and its glucuronide got reduced in the different
animal species in the order of monkey, rat, hamster, mouse, dog. O-dealkylation and
oxidative mono-dichlorination metabolites were observed in rat, monkey and, human
(Cazzola et al., 2016).
3.5.4 Excretion:
Terminal half-lives were 6 – 8 hours in different species like rat, rabbit, dog and, monkey.
Plasma/serum clearance was 1.83 L/h/kg in mice to 3.9 L/h/kg in rats while it was lower
in other species (Cazzola et al., 2016).
3.5.5 Pharmacokinetic drug interaction:
In animals, pharmacokinetic drug interaction studies were not performed. Human data is
being acceptable to the CHMP.
3.6 Toxicology:
3.6.1 Single dose toxicity:
Single-dose toxicity studies in mice, rats and, dogs demonstrated that roflumilast and
roflumilast N-oxide were non-lethal at 100 mg/kg (10, 000 times the human dose) in
rodents per os and 20 mg/kg post IV administration (McCluskie et al., 2006).
3.6.2 Repeat dose toxicity with toxicokinetic:
Repeat dose toxicity studies were performed for roflumilast and roflumilast N-oxide in
different species like mice, rats, hamster, dogs and monkeys for 6, 6, 3, 12 and 7 months
12
(Cazzola et al., 2016).
3.5.2 Distribution:
The volume of distribution to different organs and tissues were at peak level in rats in
comparison to other species like mice, dogs, and monkeys. Subsequent to the multiple
oral administration in the rats, 14C-roflumilast achieved steady state concentration after
four days. Roflumilast exhibited high plasma and/or serum protein binding. Unbound
fraction remains lowest in humans and minipigs; however, it is more in other species like
mouse, rat, hamster, guinea pig, rabbit, dog and monkey (Cazzola et al., 2016).
3.5.3 Metabolism:
Levels of [14C]-roflumilast N-oxide and its glucuronide got reduced in the different
animal species in the order of monkey, rat, hamster, mouse, dog. O-dealkylation and
oxidative mono-dichlorination metabolites were observed in rat, monkey and, human
(Cazzola et al., 2016).
3.5.4 Excretion:
Terminal half-lives were 6 – 8 hours in different species like rat, rabbit, dog and, monkey.
Plasma/serum clearance was 1.83 L/h/kg in mice to 3.9 L/h/kg in rats while it was lower
in other species (Cazzola et al., 2016).
3.5.5 Pharmacokinetic drug interaction:
In animals, pharmacokinetic drug interaction studies were not performed. Human data is
being acceptable to the CHMP.
3.6 Toxicology:
3.6.1 Single dose toxicity:
Single-dose toxicity studies in mice, rats and, dogs demonstrated that roflumilast and
roflumilast N-oxide were non-lethal at 100 mg/kg (10, 000 times the human dose) in
rodents per os and 20 mg/kg post IV administration (McCluskie et al., 2006).
3.6.2 Repeat dose toxicity with toxicokinetic:
Repeat dose toxicity studies were performed for roflumilast and roflumilast N-oxide in
different species like mice, rats, hamster, dogs and monkeys for 6, 6, 3, 12 and 7 months
12

respectively. NOAEL (mg/kg/day) in mice were (4 - 6), rats (0.2 – 1.2), hamster (4), dogs
(0.2 – 1.2) and (0.25). Minor toxic effects were observed in olfactory mucosa,
gastrointestinal, cardiac toxicity, male reproductive organ and female reproductive organ
toxicity (McCluskie et al., 2006).
3.6.3 Genotoxicity:
Roflumilast and roflumilast N oxide exhibited positive micronuclei test at 300 mg/kg/day.
Moreover, it exhibited activation of erythrocyte parameters like erythrocyte count,
hemoglobin and haematocrit (McCluskie et al., 2006).
3.6.4 Carcinogenicity:
Roflumilast and its metabolites did not exhibit carcinogenic potential (McCluskie et al.,
2006).
3.6.5 Reproduction toxicity:
Roflumilast is not considered teratogenic; however, prominent tocolytic effect was
observed. Incomplete ossification in the rats reported contradiction for use in pregnancy
(McCluskie et al., 2006).
4. Clinical Aspects
Eighteen phases 2 and 3 studies were carried out in COPD patients to decide therapeutic
dose and to evaluate the safety and efficacy of roflumilast in comparison to placebo.
Fundamental studies like M2-124 and M2-125 are the basis for the safety and efficacy of
Roflumilast in COPD associated with chronic bronchitis. There are two supportive studies
like M2-111 and M2112 to establish safety and efficacy of Roflumilast (Huang et al.,
2018; Obaand Lone, 2013). Clinical studies performed according to GCP guidelines and
ethical standards of Directive 2001/20/EC. On request from the CHMP, routine GCP
inspection has been carried out at three sites like Germany, Poland and South Africa.
4.1 Pharmacokinetics:
Pharmacokinetic data for Roflumilast has been collected from healthy volunteers and also
from patients with COPD, asthma and hepatic or renal impairment. Roflumilast N-oxide
is the metabolite of roflumilast which exhibited potent PDE 4 inhibition activity with 10-
fold and 3-fold, higher AUC and free fraction respectively. 90 % PDE 4 inhibition
activity is mainly due to roflumilast N-oxide. Pharmacokinetic studies were performed in
13
(0.2 – 1.2) and (0.25). Minor toxic effects were observed in olfactory mucosa,
gastrointestinal, cardiac toxicity, male reproductive organ and female reproductive organ
toxicity (McCluskie et al., 2006).
3.6.3 Genotoxicity:
Roflumilast and roflumilast N oxide exhibited positive micronuclei test at 300 mg/kg/day.
Moreover, it exhibited activation of erythrocyte parameters like erythrocyte count,
hemoglobin and haematocrit (McCluskie et al., 2006).
3.6.4 Carcinogenicity:
Roflumilast and its metabolites did not exhibit carcinogenic potential (McCluskie et al.,
2006).
3.6.5 Reproduction toxicity:
Roflumilast is not considered teratogenic; however, prominent tocolytic effect was
observed. Incomplete ossification in the rats reported contradiction for use in pregnancy
(McCluskie et al., 2006).
4. Clinical Aspects
Eighteen phases 2 and 3 studies were carried out in COPD patients to decide therapeutic
dose and to evaluate the safety and efficacy of roflumilast in comparison to placebo.
Fundamental studies like M2-124 and M2-125 are the basis for the safety and efficacy of
Roflumilast in COPD associated with chronic bronchitis. There are two supportive studies
like M2-111 and M2112 to establish safety and efficacy of Roflumilast (Huang et al.,
2018; Obaand Lone, 2013). Clinical studies performed according to GCP guidelines and
ethical standards of Directive 2001/20/EC. On request from the CHMP, routine GCP
inspection has been carried out at three sites like Germany, Poland and South Africa.
4.1 Pharmacokinetics:
Pharmacokinetic data for Roflumilast has been collected from healthy volunteers and also
from patients with COPD, asthma and hepatic or renal impairment. Roflumilast N-oxide
is the metabolite of roflumilast which exhibited potent PDE 4 inhibition activity with 10-
fold and 3-fold, higher AUC and free fraction respectively. 90 % PDE 4 inhibition
activity is mainly due to roflumilast N-oxide. Pharmacokinetic studies were performed in
13
Paraphrase This Document
Need a fresh take? Get an instant paraphrase of this document with our AI Paraphraser
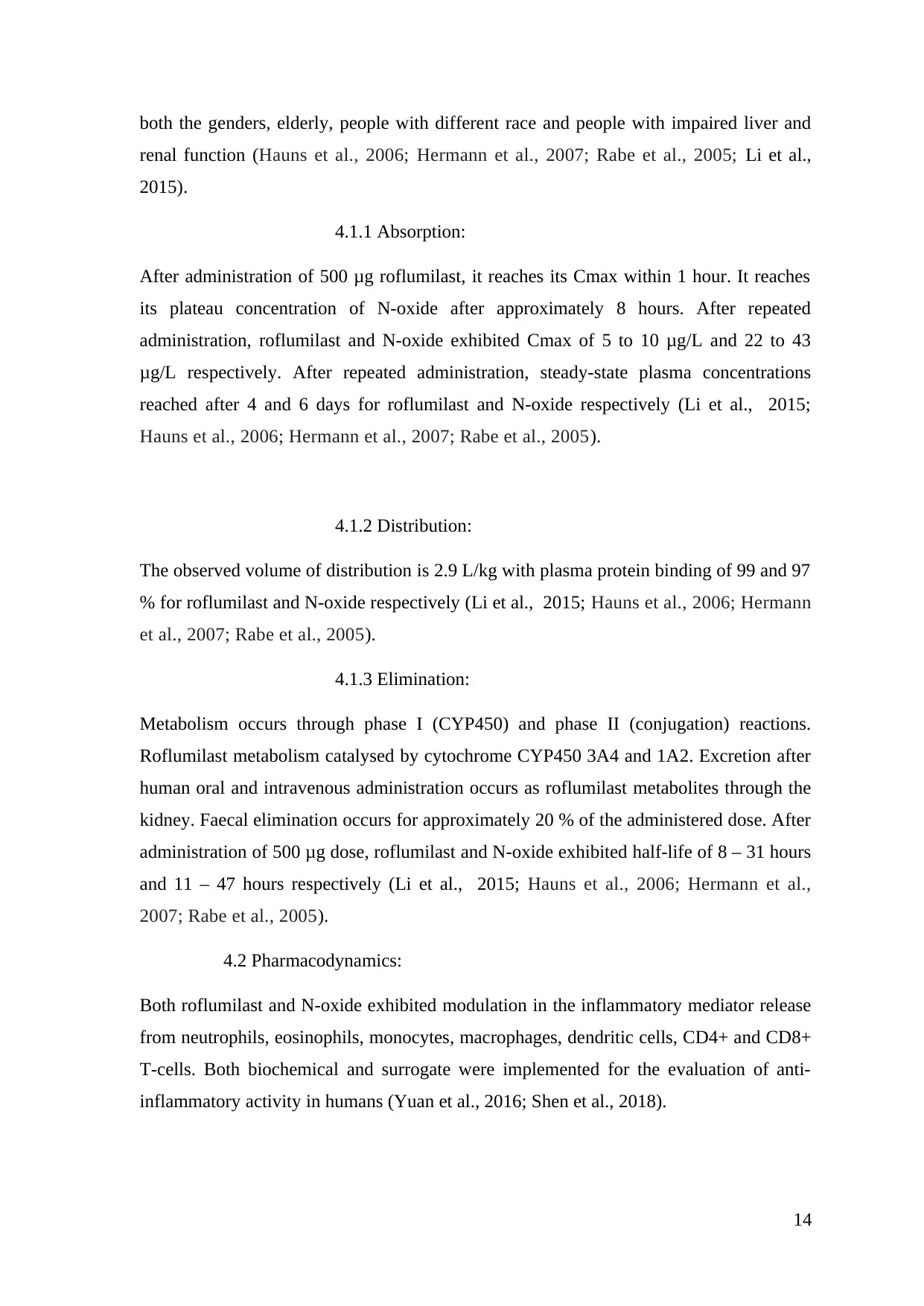
both the genders, elderly, people with different race and people with impaired liver and
renal function (Hauns et al., 2006; Hermann et al., 2007; Rabe et al., 2005; Li et al.,
2015).
4.1.1 Absorption:
After administration of 500 μg roflumilast, it reaches its Cmax within 1 hour. It reaches
its plateau concentration of N-oxide after approximately 8 hours. After repeated
administration, roflumilast and N-oxide exhibited Cmax of 5 to 10 μg/L and 22 to 43
μg/L respectively. After repeated administration, steady-state plasma concentrations
reached after 4 and 6 days for roflumilast and N-oxide respectively (Li et al., 2015;
Hauns et al., 2006; Hermann et al., 2007; Rabe et al., 2005).
4.1.2 Distribution:
The observed volume of distribution is 2.9 L/kg with plasma protein binding of 99 and 97
% for roflumilast and N-oxide respectively (Li et al., 2015; Hauns et al., 2006; Hermann
et al., 2007; Rabe et al., 2005).
4.1.3 Elimination:
Metabolism occurs through phase I (CYP450) and phase II (conjugation) reactions.
Roflumilast metabolism catalysed by cytochrome CYP450 3A4 and 1A2. Excretion after
human oral and intravenous administration occurs as roflumilast metabolites through the
kidney. Faecal elimination occurs for approximately 20 % of the administered dose. After
administration of 500 μg dose, roflumilast and N-oxide exhibited half-life of 8 – 31 hours
and 11 – 47 hours respectively (Li et al., 2015; Hauns et al., 2006; Hermann et al.,
2007; Rabe et al., 2005).
4.2 Pharmacodynamics:
Both roflumilast and N-oxide exhibited modulation in the inflammatory mediator release
from neutrophils, eosinophils, monocytes, macrophages, dendritic cells, CD4+ and CD8+
T-cells. Both biochemical and surrogate were implemented for the evaluation of anti-
inflammatory activity in humans (Yuan et al., 2016; Shen et al., 2018).
14
renal function (Hauns et al., 2006; Hermann et al., 2007; Rabe et al., 2005; Li et al.,
2015).
4.1.1 Absorption:
After administration of 500 μg roflumilast, it reaches its Cmax within 1 hour. It reaches
its plateau concentration of N-oxide after approximately 8 hours. After repeated
administration, roflumilast and N-oxide exhibited Cmax of 5 to 10 μg/L and 22 to 43
μg/L respectively. After repeated administration, steady-state plasma concentrations
reached after 4 and 6 days for roflumilast and N-oxide respectively (Li et al., 2015;
Hauns et al., 2006; Hermann et al., 2007; Rabe et al., 2005).
4.1.2 Distribution:
The observed volume of distribution is 2.9 L/kg with plasma protein binding of 99 and 97
% for roflumilast and N-oxide respectively (Li et al., 2015; Hauns et al., 2006; Hermann
et al., 2007; Rabe et al., 2005).
4.1.3 Elimination:
Metabolism occurs through phase I (CYP450) and phase II (conjugation) reactions.
Roflumilast metabolism catalysed by cytochrome CYP450 3A4 and 1A2. Excretion after
human oral and intravenous administration occurs as roflumilast metabolites through the
kidney. Faecal elimination occurs for approximately 20 % of the administered dose. After
administration of 500 μg dose, roflumilast and N-oxide exhibited half-life of 8 – 31 hours
and 11 – 47 hours respectively (Li et al., 2015; Hauns et al., 2006; Hermann et al.,
2007; Rabe et al., 2005).
4.2 Pharmacodynamics:
Both roflumilast and N-oxide exhibited modulation in the inflammatory mediator release
from neutrophils, eosinophils, monocytes, macrophages, dendritic cells, CD4+ and CD8+
T-cells. Both biochemical and surrogate were implemented for the evaluation of anti-
inflammatory activity in humans (Yuan et al., 2016; Shen et al., 2018).
14
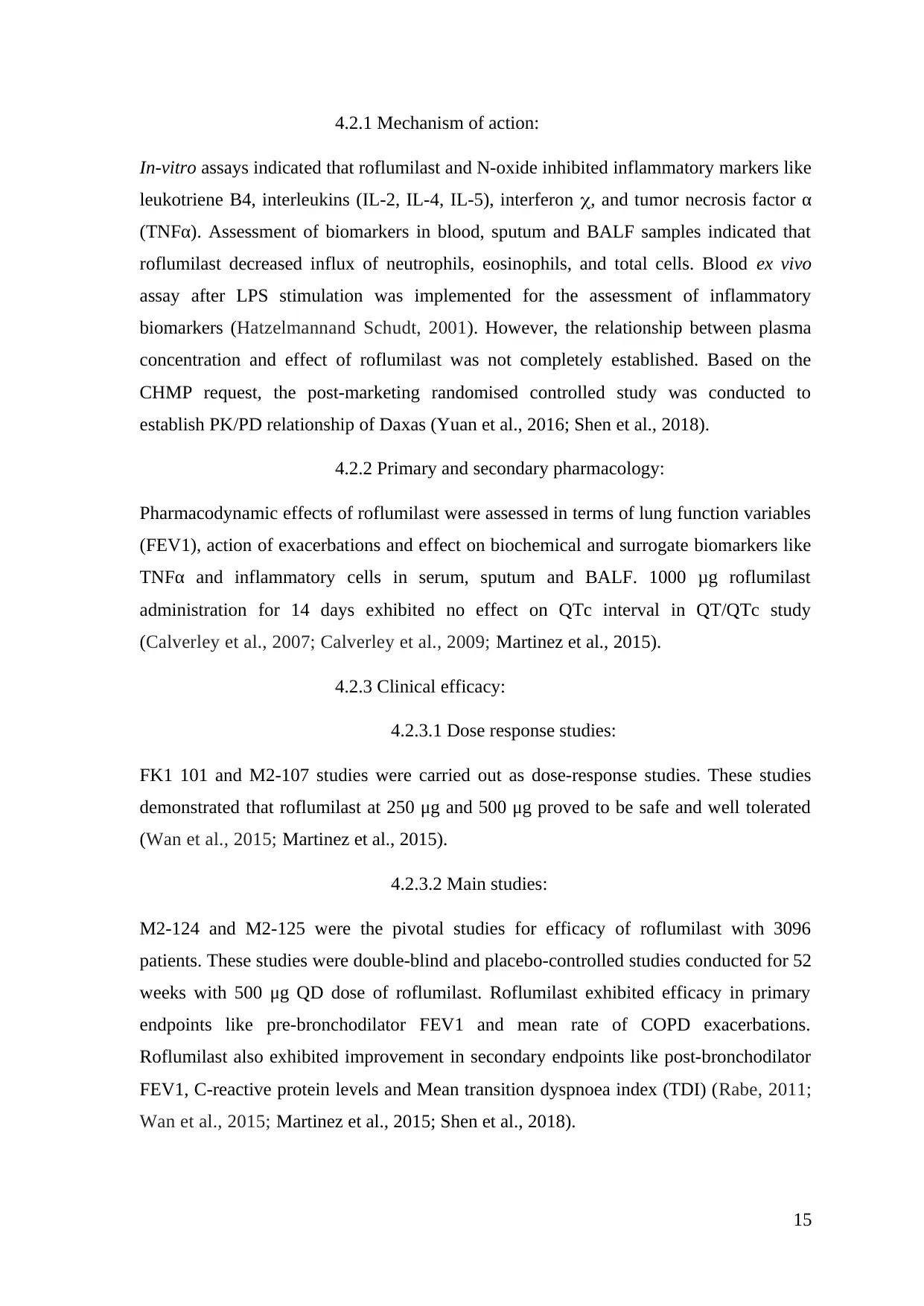
4.2.1 Mechanism of action:
In-vitro assays indicated that roflumilast and N-oxide inhibited inflammatory markers like
leukotriene B4, interleukins (IL-2, IL-4, IL-5), interferon , and tumor necrosis factor α
(TNFα). Assessment of biomarkers in blood, sputum and BALF samples indicated that
roflumilast decreased influx of neutrophils, eosinophils, and total cells. Blood ex vivo
assay after LPS stimulation was implemented for the assessment of inflammatory
biomarkers (Hatzelmannand Schudt, 2001). However, the relationship between plasma
concentration and effect of roflumilast was not completely established. Based on the
CHMP request, the post-marketing randomised controlled study was conducted to
establish PK/PD relationship of Daxas (Yuan et al., 2016; Shen et al., 2018).
4.2.2 Primary and secondary pharmacology:
Pharmacodynamic effects of roflumilast were assessed in terms of lung function variables
(FEV1), action of exacerbations and effect on biochemical and surrogate biomarkers like
TNFα and inflammatory cells in serum, sputum and BALF. 1000 μg roflumilast
administration for 14 days exhibited no effect on QTc interval in QT/QTc study
(Calverley et al., 2007; Calverley et al., 2009; Martinez et al., 2015).
4.2.3 Clinical efficacy:
4.2.3.1 Dose response studies:
FK1 101 and M2-107 studies were carried out as dose-response studies. These studies
demonstrated that roflumilast at 250 μg and 500 μg proved to be safe and well tolerated
(Wan et al., 2015; Martinez et al., 2015).
4.2.3.2 Main studies:
M2-124 and M2-125 were the pivotal studies for efficacy of roflumilast with 3096
patients. These studies were double-blind and placebo-controlled studies conducted for 52
weeks with 500 μg QD dose of roflumilast. Roflumilast exhibited efficacy in primary
endpoints like pre-bronchodilator FEV1 and mean rate of COPD exacerbations.
Roflumilast also exhibited improvement in secondary endpoints like post-bronchodilator
FEV1, C-reactive protein levels and Mean transition dyspnoea index (TDI) (Rabe, 2011;
Wan et al., 2015; Martinez et al., 2015; Shen et al., 2018).
15
In-vitro assays indicated that roflumilast and N-oxide inhibited inflammatory markers like
leukotriene B4, interleukins (IL-2, IL-4, IL-5), interferon , and tumor necrosis factor α
(TNFα). Assessment of biomarkers in blood, sputum and BALF samples indicated that
roflumilast decreased influx of neutrophils, eosinophils, and total cells. Blood ex vivo
assay after LPS stimulation was implemented for the assessment of inflammatory
biomarkers (Hatzelmannand Schudt, 2001). However, the relationship between plasma
concentration and effect of roflumilast was not completely established. Based on the
CHMP request, the post-marketing randomised controlled study was conducted to
establish PK/PD relationship of Daxas (Yuan et al., 2016; Shen et al., 2018).
4.2.2 Primary and secondary pharmacology:
Pharmacodynamic effects of roflumilast were assessed in terms of lung function variables
(FEV1), action of exacerbations and effect on biochemical and surrogate biomarkers like
TNFα and inflammatory cells in serum, sputum and BALF. 1000 μg roflumilast
administration for 14 days exhibited no effect on QTc interval in QT/QTc study
(Calverley et al., 2007; Calverley et al., 2009; Martinez et al., 2015).
4.2.3 Clinical efficacy:
4.2.3.1 Dose response studies:
FK1 101 and M2-107 studies were carried out as dose-response studies. These studies
demonstrated that roflumilast at 250 μg and 500 μg proved to be safe and well tolerated
(Wan et al., 2015; Martinez et al., 2015).
4.2.3.2 Main studies:
M2-124 and M2-125 were the pivotal studies for efficacy of roflumilast with 3096
patients. These studies were double-blind and placebo-controlled studies conducted for 52
weeks with 500 μg QD dose of roflumilast. Roflumilast exhibited efficacy in primary
endpoints like pre-bronchodilator FEV1 and mean rate of COPD exacerbations.
Roflumilast also exhibited improvement in secondary endpoints like post-bronchodilator
FEV1, C-reactive protein levels and Mean transition dyspnoea index (TDI) (Rabe, 2011;
Wan et al., 2015; Martinez et al., 2015; Shen et al., 2018).
15
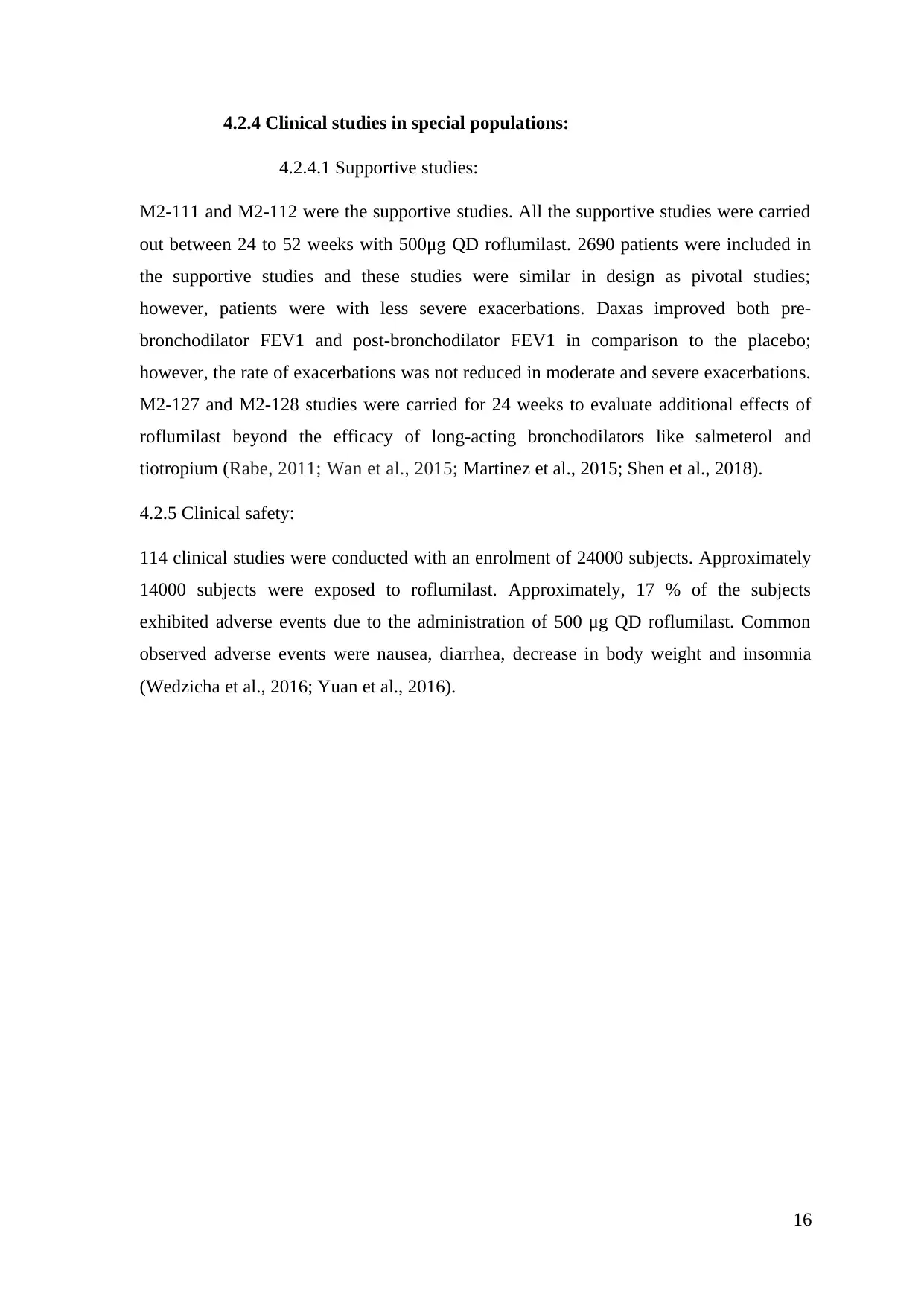
4.2.4 Clinical studies in special populations:
4.2.4.1 Supportive studies:
M2-111 and M2-112 were the supportive studies. All the supportive studies were carried
out between 24 to 52 weeks with 500μg QD roflumilast. 2690 patients were included in
the supportive studies and these studies were similar in design as pivotal studies;
however, patients were with less severe exacerbations. Daxas improved both pre-
bronchodilator FEV1 and post-bronchodilator FEV1 in comparison to the placebo;
however, the rate of exacerbations was not reduced in moderate and severe exacerbations.
M2-127 and M2-128 studies were carried for 24 weeks to evaluate additional effects of
roflumilast beyond the efficacy of long-acting bronchodilators like salmeterol and
tiotropium (Rabe, 2011; Wan et al., 2015; Martinez et al., 2015; Shen et al., 2018).
4.2.5 Clinical safety:
114 clinical studies were conducted with an enrolment of 24000 subjects. Approximately
14000 subjects were exposed to roflumilast. Approximately, 17 % of the subjects
exhibited adverse events due to the administration of 500 μg QD roflumilast. Common
observed adverse events were nausea, diarrhea, decrease in body weight and insomnia
(Wedzicha et al., 2016; Yuan et al., 2016).
16
4.2.4.1 Supportive studies:
M2-111 and M2-112 were the supportive studies. All the supportive studies were carried
out between 24 to 52 weeks with 500μg QD roflumilast. 2690 patients were included in
the supportive studies and these studies were similar in design as pivotal studies;
however, patients were with less severe exacerbations. Daxas improved both pre-
bronchodilator FEV1 and post-bronchodilator FEV1 in comparison to the placebo;
however, the rate of exacerbations was not reduced in moderate and severe exacerbations.
M2-127 and M2-128 studies were carried for 24 weeks to evaluate additional effects of
roflumilast beyond the efficacy of long-acting bronchodilators like salmeterol and
tiotropium (Rabe, 2011; Wan et al., 2015; Martinez et al., 2015; Shen et al., 2018).
4.2.5 Clinical safety:
114 clinical studies were conducted with an enrolment of 24000 subjects. Approximately
14000 subjects were exposed to roflumilast. Approximately, 17 % of the subjects
exhibited adverse events due to the administration of 500 μg QD roflumilast. Common
observed adverse events were nausea, diarrhea, decrease in body weight and insomnia
(Wedzicha et al., 2016; Yuan et al., 2016).
16
Secure Best Marks with AI Grader
Need help grading? Try our AI Grader for instant feedback on your assignments.
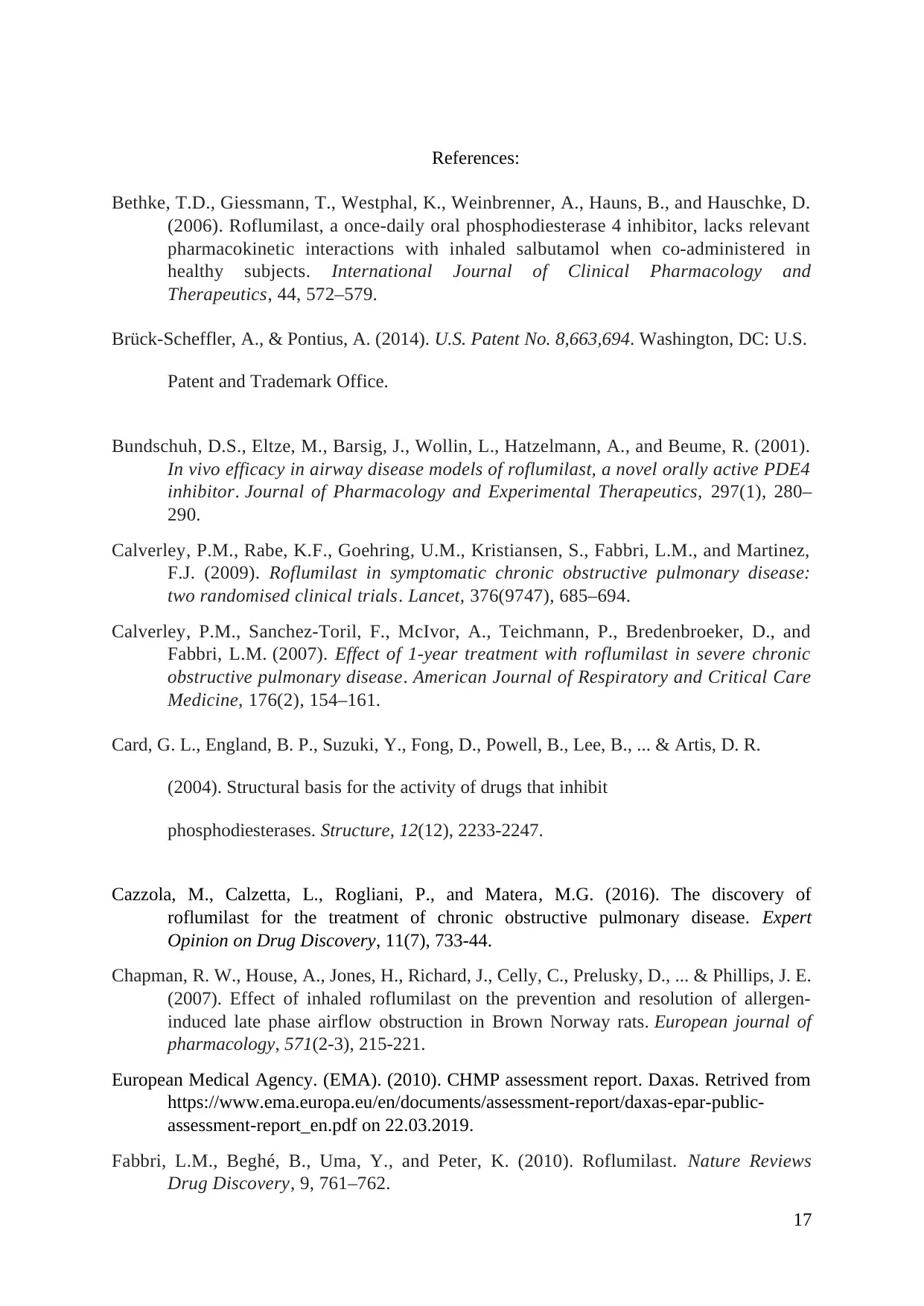
References:
Bethke, T.D., Giessmann, T., Westphal, K., Weinbrenner, A., Hauns, B., and Hauschke, D.
(2006). Roflumilast, a once-daily oral phosphodiesterase 4 inhibitor, lacks relevant
pharmacokinetic interactions with inhaled salbutamol when co-administered in
healthy subjects. International Journal of Clinical Pharmacology and
Therapeutics, 44, 572–579.
Brück-Scheffler, A., & Pontius, A. (2014). U.S. Patent No. 8,663,694. Washington, DC: U.S.
Patent and Trademark Office.
Bundschuh, D.S., Eltze, M., Barsig, J., Wollin, L., Hatzelmann, A., and Beume, R. (2001).
In vivo efficacy in airway disease models of roflumilast, a novel orally active PDE4
inhibitor. Journal of Pharmacology and Experimental Therapeutics, 297(1), 280–
290.
Calverley, P.M., Rabe, K.F., Goehring, U.M., Kristiansen, S., Fabbri, L.M., and Martinez,
F.J. (2009). Roflumilast in symptomatic chronic obstructive pulmonary disease:
two randomised clinical trials. Lancet, 376(9747), 685–694.
Calverley, P.M., Sanchez-Toril, F., McIvor, A., Teichmann, P., Bredenbroeker, D., and
Fabbri, L.M. (2007). Effect of 1-year treatment with roflumilast in severe chronic
obstructive pulmonary disease. American Journal of Respiratory and Critical Care
Medicine, 176(2), 154–161.
Card, G. L., England, B. P., Suzuki, Y., Fong, D., Powell, B., Lee, B., ... & Artis, D. R.
(2004). Structural basis for the activity of drugs that inhibit
phosphodiesterases. Structure, 12(12), 2233-2247.
Cazzola, M., Calzetta, L., Rogliani, P., and Matera, M.G. (2016). The discovery of
roflumilast for the treatment of chronic obstructive pulmonary disease. Expert
Opinion on Drug Discovery, 11(7), 733-44.
Chapman, R. W., House, A., Jones, H., Richard, J., Celly, C., Prelusky, D., ... & Phillips, J. E.
(2007). Effect of inhaled roflumilast on the prevention and resolution of allergen-
induced late phase airflow obstruction in Brown Norway rats. European journal of
pharmacology, 571(2-3), 215-221.
European Medical Agency. (EMA). (2010). CHMP assessment report. Daxas. Retrived from
https://www.ema.europa.eu/en/documents/assessment-report/daxas-epar-public-
assessment-report_en.pdf on 22.03.2019.
Fabbri, L.M., Beghé, B., Uma, Y., and Peter, K. (2010). Roflumilast. Nature Reviews
Drug Discovery, 9, 761–762.
17
Bethke, T.D., Giessmann, T., Westphal, K., Weinbrenner, A., Hauns, B., and Hauschke, D.
(2006). Roflumilast, a once-daily oral phosphodiesterase 4 inhibitor, lacks relevant
pharmacokinetic interactions with inhaled salbutamol when co-administered in
healthy subjects. International Journal of Clinical Pharmacology and
Therapeutics, 44, 572–579.
Brück-Scheffler, A., & Pontius, A. (2014). U.S. Patent No. 8,663,694. Washington, DC: U.S.
Patent and Trademark Office.
Bundschuh, D.S., Eltze, M., Barsig, J., Wollin, L., Hatzelmann, A., and Beume, R. (2001).
In vivo efficacy in airway disease models of roflumilast, a novel orally active PDE4
inhibitor. Journal of Pharmacology and Experimental Therapeutics, 297(1), 280–
290.
Calverley, P.M., Rabe, K.F., Goehring, U.M., Kristiansen, S., Fabbri, L.M., and Martinez,
F.J. (2009). Roflumilast in symptomatic chronic obstructive pulmonary disease:
two randomised clinical trials. Lancet, 376(9747), 685–694.
Calverley, P.M., Sanchez-Toril, F., McIvor, A., Teichmann, P., Bredenbroeker, D., and
Fabbri, L.M. (2007). Effect of 1-year treatment with roflumilast in severe chronic
obstructive pulmonary disease. American Journal of Respiratory and Critical Care
Medicine, 176(2), 154–161.
Card, G. L., England, B. P., Suzuki, Y., Fong, D., Powell, B., Lee, B., ... & Artis, D. R.
(2004). Structural basis for the activity of drugs that inhibit
phosphodiesterases. Structure, 12(12), 2233-2247.
Cazzola, M., Calzetta, L., Rogliani, P., and Matera, M.G. (2016). The discovery of
roflumilast for the treatment of chronic obstructive pulmonary disease. Expert
Opinion on Drug Discovery, 11(7), 733-44.
Chapman, R. W., House, A., Jones, H., Richard, J., Celly, C., Prelusky, D., ... & Phillips, J. E.
(2007). Effect of inhaled roflumilast on the prevention and resolution of allergen-
induced late phase airflow obstruction in Brown Norway rats. European journal of
pharmacology, 571(2-3), 215-221.
European Medical Agency. (EMA). (2010). CHMP assessment report. Daxas. Retrived from
https://www.ema.europa.eu/en/documents/assessment-report/daxas-epar-public-
assessment-report_en.pdf on 22.03.2019.
Fabbri, L.M., Beghé, B., Uma, Y., and Peter, K. (2010). Roflumilast. Nature Reviews
Drug Discovery, 9, 761–762.
17
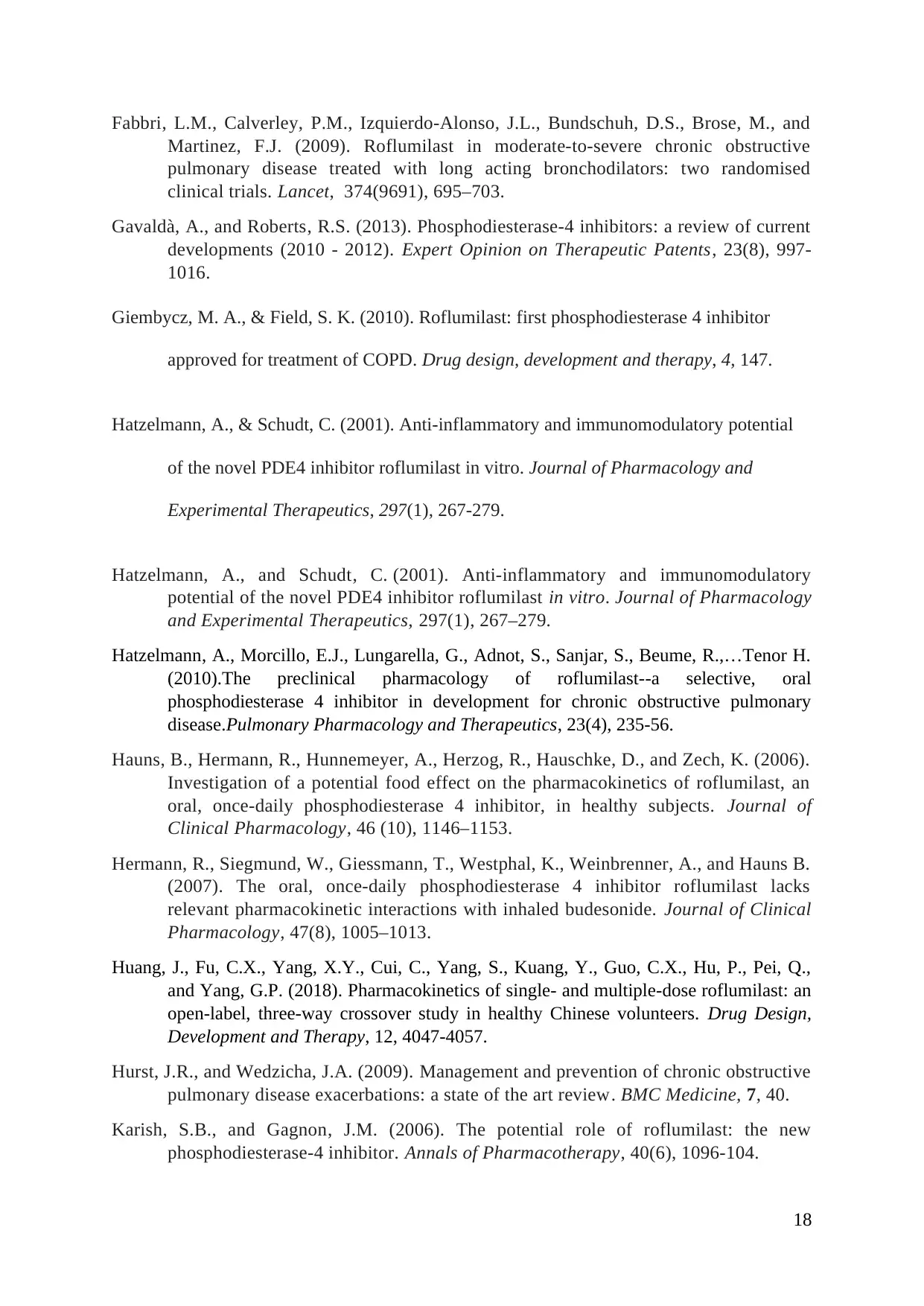
Fabbri, L.M., Calverley, P.M., Izquierdo-Alonso, J.L., Bundschuh, D.S., Brose, M., and
Martinez, F.J. (2009). Roflumilast in moderate-to-severe chronic obstructive
pulmonary disease treated with long acting bronchodilators: two randomised
clinical trials. Lancet, 374(9691), 695–703.
Gavaldà, A., and Roberts, R.S. (2013). Phosphodiesterase-4 inhibitors: a review of current
developments (2010 - 2012). Expert Opinion on Therapeutic Patents, 23(8), 997-
1016.
Giembycz, M. A., & Field, S. K. (2010). Roflumilast: first phosphodiesterase 4 inhibitor
approved for treatment of COPD. Drug design, development and therapy, 4, 147.
Hatzelmann, A., & Schudt, C. (2001). Anti-inflammatory and immunomodulatory potential
of the novel PDE4 inhibitor roflumilast in vitro. Journal of Pharmacology and
Experimental Therapeutics, 297(1), 267-279.
Hatzelmann, A., and Schudt, C. (2001). Anti-inflammatory and immunomodulatory
potential of the novel PDE4 inhibitor roflumilast in vitro. Journal of Pharmacology
and Experimental Therapeutics, 297(1), 267–279.
Hatzelmann, A., Morcillo, E.J., Lungarella, G., Adnot, S., Sanjar, S., Beume, R.,…Tenor H.
(2010).The preclinical pharmacology of roflumilast--a selective, oral
phosphodiesterase 4 inhibitor in development for chronic obstructive pulmonary
disease.Pulmonary Pharmacology and Therapeutics, 23(4), 235-56.
Hauns, B., Hermann, R., Hunnemeyer, A., Herzog, R., Hauschke, D., and Zech, K. (2006).
Investigation of a potential food effect on the pharmacokinetics of roflumilast, an
oral, once-daily phosphodiesterase 4 inhibitor, in healthy subjects. Journal of
Clinical Pharmacology, 46 (10), 1146–1153.
Hermann, R., Siegmund, W., Giessmann, T., Westphal, K., Weinbrenner, A., and Hauns B.
(2007). The oral, once-daily phosphodiesterase 4 inhibitor roflumilast lacks
relevant pharmacokinetic interactions with inhaled budesonide. Journal of Clinical
Pharmacology, 47(8), 1005–1013.
Huang, J., Fu, C.X., Yang, X.Y., Cui, C., Yang, S., Kuang, Y., Guo, C.X., Hu, P., Pei, Q.,
and Yang, G.P. (2018). Pharmacokinetics of single- and multiple-dose roflumilast: an
open-label, three-way crossover study in healthy Chinese volunteers. Drug Design,
Development and Therapy, 12, 4047-4057.
Hurst, J.R., and Wedzicha, J.A. (2009). Management and prevention of chronic obstructive
pulmonary disease exacerbations: a state of the art review. BMC Medicine, 7, 40.
Karish, S.B., and Gagnon, J.M. (2006). The potential role of roflumilast: the new
phosphodiesterase-4 inhibitor. Annals of Pharmacotherapy, 40(6), 1096-104.
18
Martinez, F.J. (2009). Roflumilast in moderate-to-severe chronic obstructive
pulmonary disease treated with long acting bronchodilators: two randomised
clinical trials. Lancet, 374(9691), 695–703.
Gavaldà, A., and Roberts, R.S. (2013). Phosphodiesterase-4 inhibitors: a review of current
developments (2010 - 2012). Expert Opinion on Therapeutic Patents, 23(8), 997-
1016.
Giembycz, M. A., & Field, S. K. (2010). Roflumilast: first phosphodiesterase 4 inhibitor
approved for treatment of COPD. Drug design, development and therapy, 4, 147.
Hatzelmann, A., & Schudt, C. (2001). Anti-inflammatory and immunomodulatory potential
of the novel PDE4 inhibitor roflumilast in vitro. Journal of Pharmacology and
Experimental Therapeutics, 297(1), 267-279.
Hatzelmann, A., and Schudt, C. (2001). Anti-inflammatory and immunomodulatory
potential of the novel PDE4 inhibitor roflumilast in vitro. Journal of Pharmacology
and Experimental Therapeutics, 297(1), 267–279.
Hatzelmann, A., Morcillo, E.J., Lungarella, G., Adnot, S., Sanjar, S., Beume, R.,…Tenor H.
(2010).The preclinical pharmacology of roflumilast--a selective, oral
phosphodiesterase 4 inhibitor in development for chronic obstructive pulmonary
disease.Pulmonary Pharmacology and Therapeutics, 23(4), 235-56.
Hauns, B., Hermann, R., Hunnemeyer, A., Herzog, R., Hauschke, D., and Zech, K. (2006).
Investigation of a potential food effect on the pharmacokinetics of roflumilast, an
oral, once-daily phosphodiesterase 4 inhibitor, in healthy subjects. Journal of
Clinical Pharmacology, 46 (10), 1146–1153.
Hermann, R., Siegmund, W., Giessmann, T., Westphal, K., Weinbrenner, A., and Hauns B.
(2007). The oral, once-daily phosphodiesterase 4 inhibitor roflumilast lacks
relevant pharmacokinetic interactions with inhaled budesonide. Journal of Clinical
Pharmacology, 47(8), 1005–1013.
Huang, J., Fu, C.X., Yang, X.Y., Cui, C., Yang, S., Kuang, Y., Guo, C.X., Hu, P., Pei, Q.,
and Yang, G.P. (2018). Pharmacokinetics of single- and multiple-dose roflumilast: an
open-label, three-way crossover study in healthy Chinese volunteers. Drug Design,
Development and Therapy, 12, 4047-4057.
Hurst, J.R., and Wedzicha, J.A. (2009). Management and prevention of chronic obstructive
pulmonary disease exacerbations: a state of the art review. BMC Medicine, 7, 40.
Karish, S.B., and Gagnon, J.M. (2006). The potential role of roflumilast: the new
phosphodiesterase-4 inhibitor. Annals of Pharmacotherapy, 40(6), 1096-104.
18
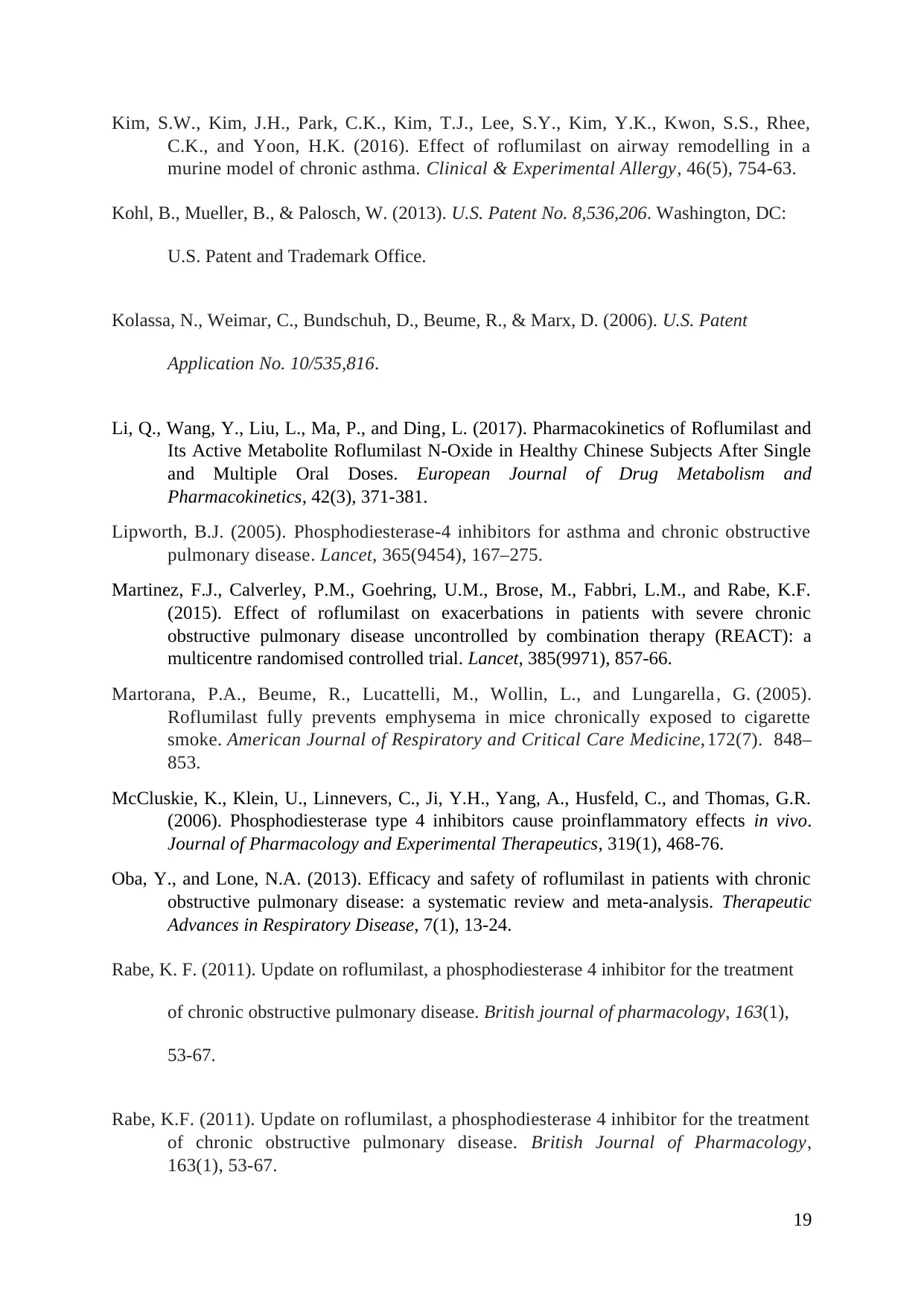
Kim, S.W., Kim, J.H., Park, C.K., Kim, T.J., Lee, S.Y., Kim, Y.K., Kwon, S.S., Rhee,
C.K., and Yoon, H.K. (2016). Effect of roflumilast on airway remodelling in a
murine model of chronic asthma. Clinical & Experimental Allergy, 46(5), 754-63.
Kohl, B., Mueller, B., & Palosch, W. (2013). U.S. Patent No. 8,536,206. Washington, DC:
U.S. Patent and Trademark Office.
Kolassa, N., Weimar, C., Bundschuh, D., Beume, R., & Marx, D. (2006). U.S. Patent
Application No. 10/535,816.
Li, Q., Wang, Y., Liu, L., Ma, P., and Ding, L. (2017). Pharmacokinetics of Roflumilast and
Its Active Metabolite Roflumilast N-Oxide in Healthy Chinese Subjects After Single
and Multiple Oral Doses. European Journal of Drug Metabolism and
Pharmacokinetics, 42(3), 371-381.
Lipworth, B.J. (2005). Phosphodiesterase-4 inhibitors for asthma and chronic obstructive
pulmonary disease. Lancet, 365(9454), 167–275.
Martinez, F.J., Calverley, P.M., Goehring, U.M., Brose, M., Fabbri, L.M., and Rabe, K.F.
(2015). Effect of roflumilast on exacerbations in patients with severe chronic
obstructive pulmonary disease uncontrolled by combination therapy (REACT): a
multicentre randomised controlled trial. Lancet, 385(9971), 857-66.
Martorana, P.A., Beume, R., Lucattelli, M., Wollin, L., and Lungarella , G. (2005).
Roflumilast fully prevents emphysema in mice chronically exposed to cigarette
smoke. American Journal of Respiratory and Critical Care Medicine, 172(7). 848–
853.
McCluskie, K., Klein, U., Linnevers, C., Ji, Y.H., Yang, A., Husfeld, C., and Thomas, G.R.
(2006). Phosphodiesterase type 4 inhibitors cause proinflammatory effects in vivo.
Journal of Pharmacology and Experimental Therapeutics, 319(1), 468-76.
Oba, Y., and Lone, N.A. (2013). Efficacy and safety of roflumilast in patients with chronic
obstructive pulmonary disease: a systematic review and meta-analysis. Therapeutic
Advances in Respiratory Disease, 7(1), 13-24.
Rabe, K. F. (2011). Update on roflumilast, a phosphodiesterase 4 inhibitor for the treatment
of chronic obstructive pulmonary disease. British journal of pharmacology, 163(1),
53-67.
Rabe, K.F. (2011). Update on roflumilast, a phosphodiesterase 4 inhibitor for the treatment
of chronic obstructive pulmonary disease. British Journal of Pharmacology,
163(1), 53-67.
19
C.K., and Yoon, H.K. (2016). Effect of roflumilast on airway remodelling in a
murine model of chronic asthma. Clinical & Experimental Allergy, 46(5), 754-63.
Kohl, B., Mueller, B., & Palosch, W. (2013). U.S. Patent No. 8,536,206. Washington, DC:
U.S. Patent and Trademark Office.
Kolassa, N., Weimar, C., Bundschuh, D., Beume, R., & Marx, D. (2006). U.S. Patent
Application No. 10/535,816.
Li, Q., Wang, Y., Liu, L., Ma, P., and Ding, L. (2017). Pharmacokinetics of Roflumilast and
Its Active Metabolite Roflumilast N-Oxide in Healthy Chinese Subjects After Single
and Multiple Oral Doses. European Journal of Drug Metabolism and
Pharmacokinetics, 42(3), 371-381.
Lipworth, B.J. (2005). Phosphodiesterase-4 inhibitors for asthma and chronic obstructive
pulmonary disease. Lancet, 365(9454), 167–275.
Martinez, F.J., Calverley, P.M., Goehring, U.M., Brose, M., Fabbri, L.M., and Rabe, K.F.
(2015). Effect of roflumilast on exacerbations in patients with severe chronic
obstructive pulmonary disease uncontrolled by combination therapy (REACT): a
multicentre randomised controlled trial. Lancet, 385(9971), 857-66.
Martorana, P.A., Beume, R., Lucattelli, M., Wollin, L., and Lungarella , G. (2005).
Roflumilast fully prevents emphysema in mice chronically exposed to cigarette
smoke. American Journal of Respiratory and Critical Care Medicine, 172(7). 848–
853.
McCluskie, K., Klein, U., Linnevers, C., Ji, Y.H., Yang, A., Husfeld, C., and Thomas, G.R.
(2006). Phosphodiesterase type 4 inhibitors cause proinflammatory effects in vivo.
Journal of Pharmacology and Experimental Therapeutics, 319(1), 468-76.
Oba, Y., and Lone, N.A. (2013). Efficacy and safety of roflumilast in patients with chronic
obstructive pulmonary disease: a systematic review and meta-analysis. Therapeutic
Advances in Respiratory Disease, 7(1), 13-24.
Rabe, K. F. (2011). Update on roflumilast, a phosphodiesterase 4 inhibitor for the treatment
of chronic obstructive pulmonary disease. British journal of pharmacology, 163(1),
53-67.
Rabe, K.F. (2011). Update on roflumilast, a phosphodiesterase 4 inhibitor for the treatment
of chronic obstructive pulmonary disease. British Journal of Pharmacology,
163(1), 53-67.
19
Paraphrase This Document
Need a fresh take? Get an instant paraphrase of this document with our AI Paraphraser
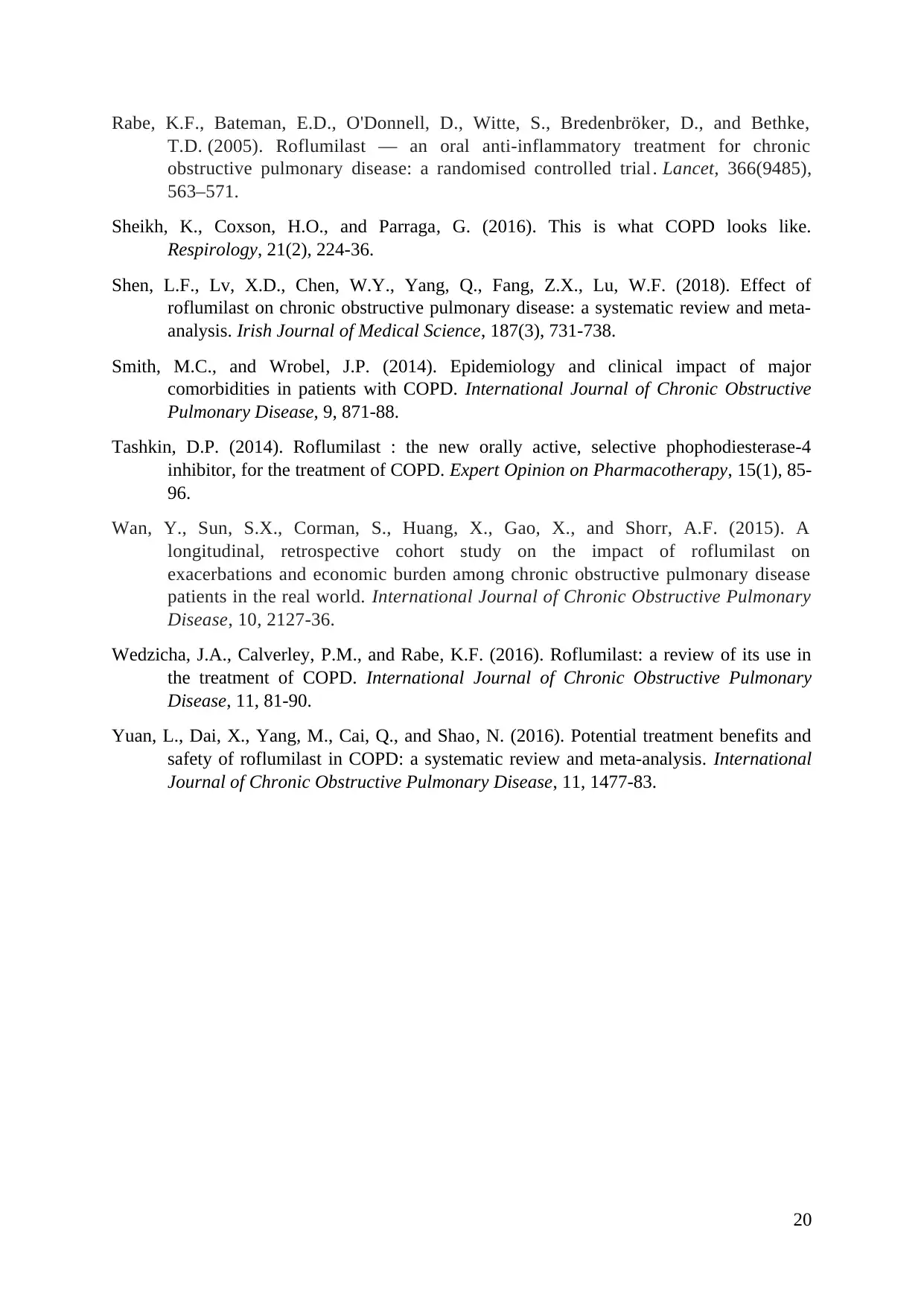
Rabe, K.F., Bateman, E.D., O'Donnell, D., Witte, S., Bredenbröker, D., and Bethke,
T.D. (2005). Roflumilast — an oral anti-inflammatory treatment for chronic
obstructive pulmonary disease: a randomised controlled trial . Lancet, 366(9485),
563–571.
Sheikh, K., Coxson, H.O., and Parraga, G. (2016). This is what COPD looks like.
Respirology, 21(2), 224-36.
Shen, L.F., Lv, X.D., Chen, W.Y., Yang, Q., Fang, Z.X., Lu, W.F. (2018). Effect of
roflumilast on chronic obstructive pulmonary disease: a systematic review and meta-
analysis. Irish Journal of Medical Science, 187(3), 731-738.
Smith, M.C., and Wrobel, J.P. (2014). Epidemiology and clinical impact of major
comorbidities in patients with COPD. International Journal of Chronic Obstructive
Pulmonary Disease, 9, 871-88.
Tashkin, D.P. (2014). Roflumilast : the new orally active, selective phophodiesterase-4
inhibitor, for the treatment of COPD. Expert Opinion on Pharmacotherapy, 15(1), 85-
96.
Wan, Y., Sun, S.X., Corman, S., Huang, X., Gao, X., and Shorr, A.F. (2015). A
longitudinal, retrospective cohort study on the impact of roflumilast on
exacerbations and economic burden among chronic obstructive pulmonary disease
patients in the real world. International Journal of Chronic Obstructive Pulmonary
Disease, 10, 2127-36.
Wedzicha, J.A., Calverley, P.M., and Rabe, K.F. (2016). Roflumilast: a review of its use in
the treatment of COPD. International Journal of Chronic Obstructive Pulmonary
Disease, 11, 81-90.
Yuan, L., Dai, X., Yang, M., Cai, Q., and Shao, N. (2016). Potential treatment benefits and
safety of roflumilast in COPD: a systematic review and meta-analysis. International
Journal of Chronic Obstructive Pulmonary Disease, 11, 1477-83.
20
T.D. (2005). Roflumilast — an oral anti-inflammatory treatment for chronic
obstructive pulmonary disease: a randomised controlled trial . Lancet, 366(9485),
563–571.
Sheikh, K., Coxson, H.O., and Parraga, G. (2016). This is what COPD looks like.
Respirology, 21(2), 224-36.
Shen, L.F., Lv, X.D., Chen, W.Y., Yang, Q., Fang, Z.X., Lu, W.F. (2018). Effect of
roflumilast on chronic obstructive pulmonary disease: a systematic review and meta-
analysis. Irish Journal of Medical Science, 187(3), 731-738.
Smith, M.C., and Wrobel, J.P. (2014). Epidemiology and clinical impact of major
comorbidities in patients with COPD. International Journal of Chronic Obstructive
Pulmonary Disease, 9, 871-88.
Tashkin, D.P. (2014). Roflumilast : the new orally active, selective phophodiesterase-4
inhibitor, for the treatment of COPD. Expert Opinion on Pharmacotherapy, 15(1), 85-
96.
Wan, Y., Sun, S.X., Corman, S., Huang, X., Gao, X., and Shorr, A.F. (2015). A
longitudinal, retrospective cohort study on the impact of roflumilast on
exacerbations and economic burden among chronic obstructive pulmonary disease
patients in the real world. International Journal of Chronic Obstructive Pulmonary
Disease, 10, 2127-36.
Wedzicha, J.A., Calverley, P.M., and Rabe, K.F. (2016). Roflumilast: a review of its use in
the treatment of COPD. International Journal of Chronic Obstructive Pulmonary
Disease, 11, 81-90.
Yuan, L., Dai, X., Yang, M., Cai, Q., and Shao, N. (2016). Potential treatment benefits and
safety of roflumilast in COPD: a systematic review and meta-analysis. International
Journal of Chronic Obstructive Pulmonary Disease, 11, 1477-83.
20
1 out of 20
Related Documents
![[object Object]](/_next/image/?url=%2F_next%2Fstatic%2Fmedia%2Flogo.6d15ce61.png&w=640&q=75)
Your All-in-One AI-Powered Toolkit for Academic Success.
+13062052269
info@desklib.com
Available 24*7 on WhatsApp / Email
Unlock your academic potential
© 2024 | Zucol Services PVT LTD | All rights reserved.