Questions and Answers on Pharmacology
VerifiedAdded on 2023/06/12
|14
|3996
|326
AI Summary
This article provides answers to questions on pharmacology including similarities and differences between genotoxic and non-genotoxic carcinogens, gastrointestinal toxicant, blood toxicant, skin toxicant, and kidney toxicant.
Contribute Materials
Your contribution can guide someone’s learning journey. Share your
documents today.
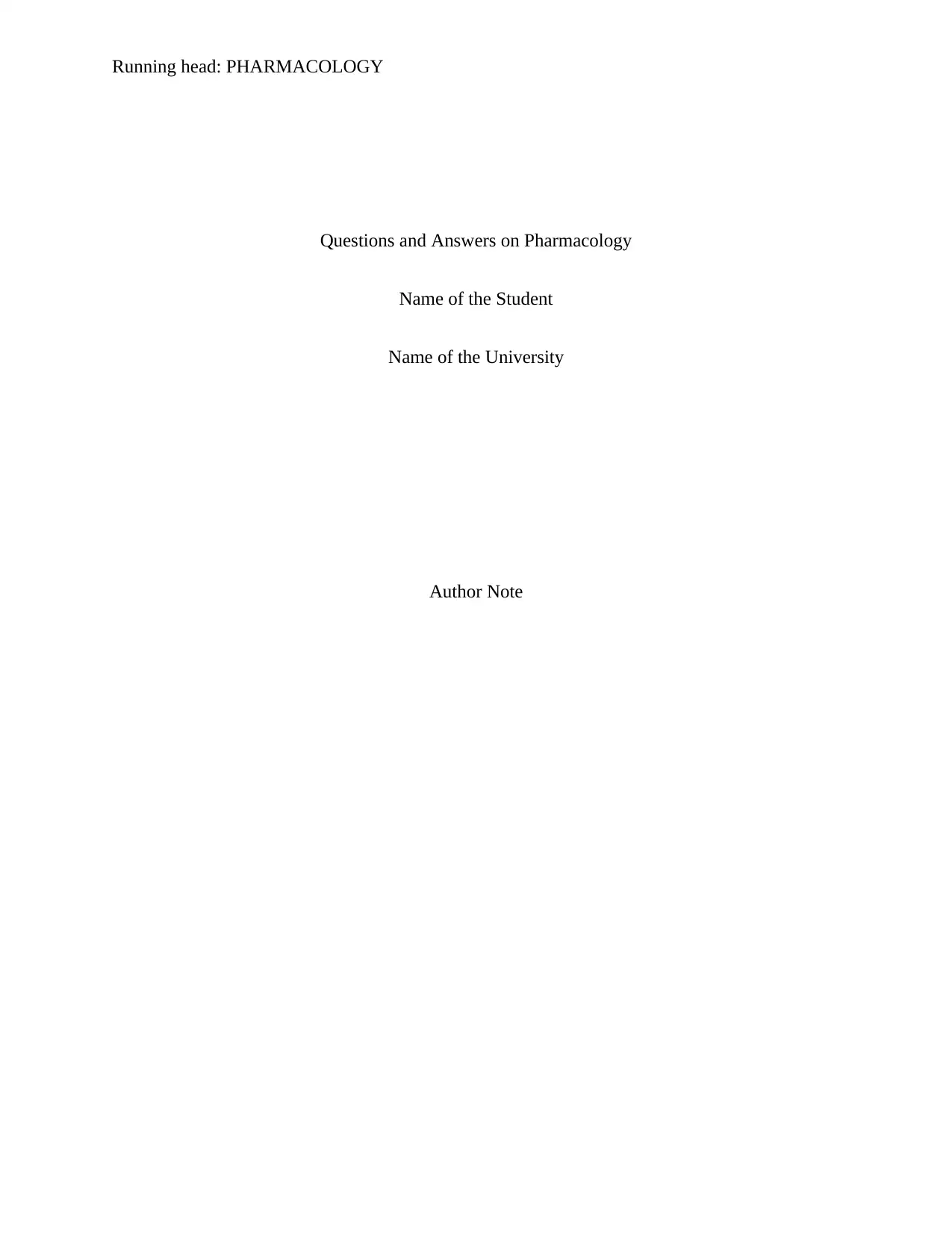
Running head: PHARMACOLOGY
Questions and Answers on Pharmacology
Name of the Student
Name of the University
Author Note
Questions and Answers on Pharmacology
Name of the Student
Name of the University
Author Note
Secure Best Marks with AI Grader
Need help grading? Try our AI Grader for instant feedback on your assignments.
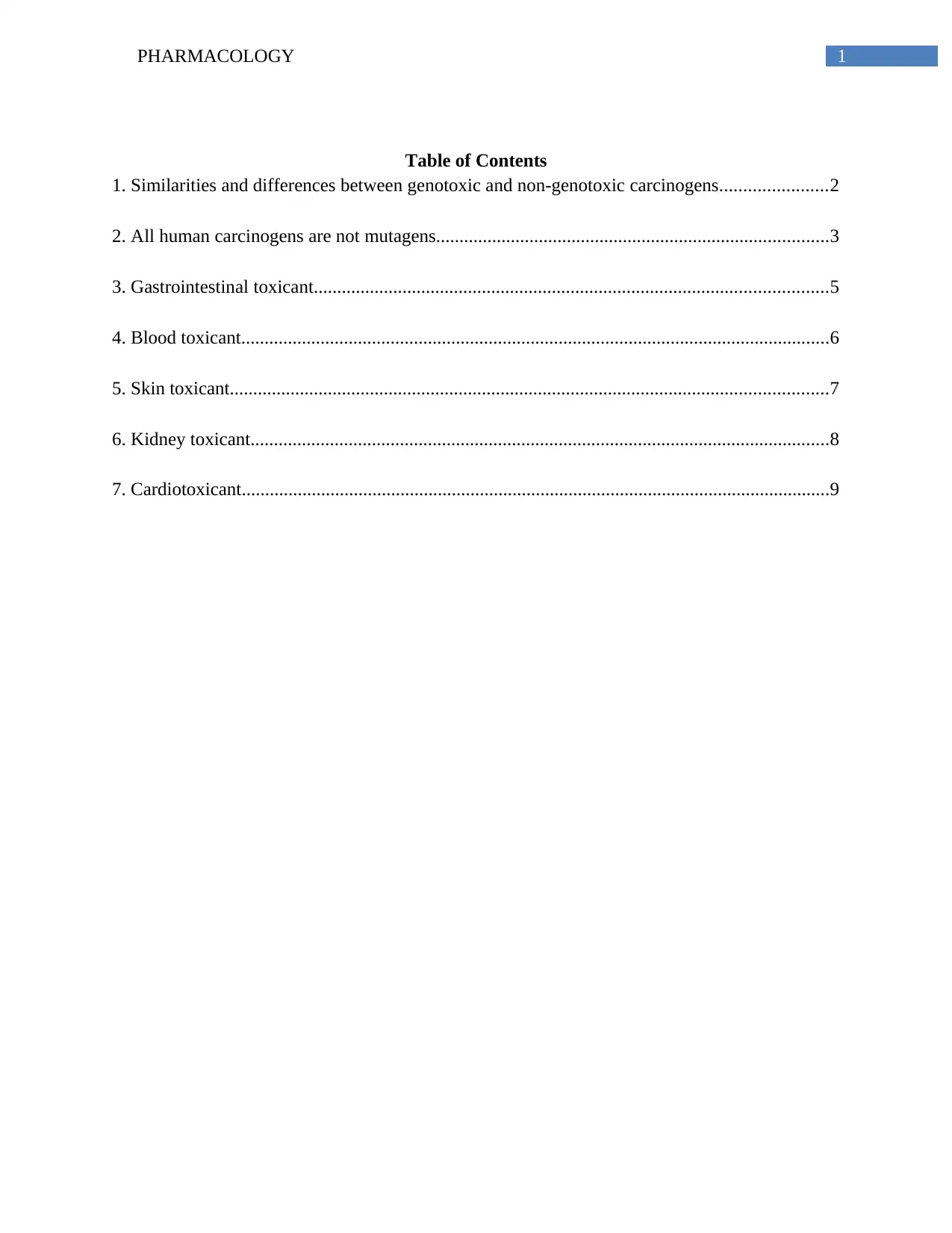
1PHARMACOLOGY
Table of Contents
1. Similarities and differences between genotoxic and non-genotoxic carcinogens.......................2
2. All human carcinogens are not mutagens....................................................................................3
3. Gastrointestinal toxicant..............................................................................................................5
4. Blood toxicant..............................................................................................................................6
5. Skin toxicant................................................................................................................................7
6. Kidney toxicant............................................................................................................................8
7. Cardiotoxicant..............................................................................................................................9
Table of Contents
1. Similarities and differences between genotoxic and non-genotoxic carcinogens.......................2
2. All human carcinogens are not mutagens....................................................................................3
3. Gastrointestinal toxicant..............................................................................................................5
4. Blood toxicant..............................................................................................................................6
5. Skin toxicant................................................................................................................................7
6. Kidney toxicant............................................................................................................................8
7. Cardiotoxicant..............................................................................................................................9
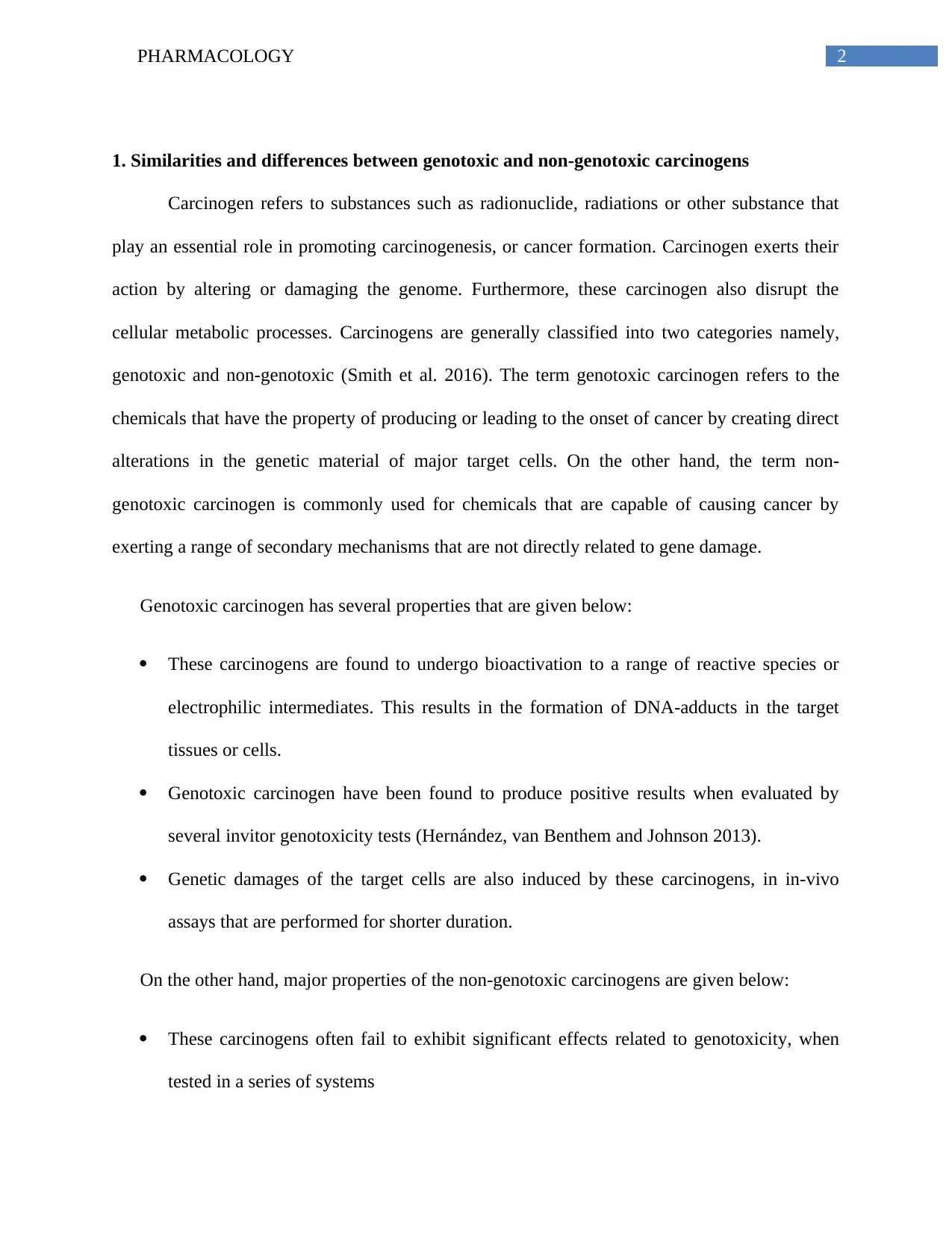
2PHARMACOLOGY
1. Similarities and differences between genotoxic and non-genotoxic carcinogens
Carcinogen refers to substances such as radionuclide, radiations or other substance that
play an essential role in promoting carcinogenesis, or cancer formation. Carcinogen exerts their
action by altering or damaging the genome. Furthermore, these carcinogen also disrupt the
cellular metabolic processes. Carcinogens are generally classified into two categories namely,
genotoxic and non-genotoxic (Smith et al. 2016). The term genotoxic carcinogen refers to the
chemicals that have the property of producing or leading to the onset of cancer by creating direct
alterations in the genetic material of major target cells. On the other hand, the term non-
genotoxic carcinogen is commonly used for chemicals that are capable of causing cancer by
exerting a range of secondary mechanisms that are not directly related to gene damage.
Genotoxic carcinogen has several properties that are given below:
These carcinogens are found to undergo bioactivation to a range of reactive species or
electrophilic intermediates. This results in the formation of DNA-adducts in the target
tissues or cells.
Genotoxic carcinogen have been found to produce positive results when evaluated by
several invitor genotoxicity tests (Hernández, van Benthem and Johnson 2013).
Genetic damages of the target cells are also induced by these carcinogens, in in-vivo
assays that are performed for shorter duration.
On the other hand, major properties of the non-genotoxic carcinogens are given below:
These carcinogens often fail to exhibit significant effects related to genotoxicity, when
tested in a series of systems
1. Similarities and differences between genotoxic and non-genotoxic carcinogens
Carcinogen refers to substances such as radionuclide, radiations or other substance that
play an essential role in promoting carcinogenesis, or cancer formation. Carcinogen exerts their
action by altering or damaging the genome. Furthermore, these carcinogen also disrupt the
cellular metabolic processes. Carcinogens are generally classified into two categories namely,
genotoxic and non-genotoxic (Smith et al. 2016). The term genotoxic carcinogen refers to the
chemicals that have the property of producing or leading to the onset of cancer by creating direct
alterations in the genetic material of major target cells. On the other hand, the term non-
genotoxic carcinogen is commonly used for chemicals that are capable of causing cancer by
exerting a range of secondary mechanisms that are not directly related to gene damage.
Genotoxic carcinogen has several properties that are given below:
These carcinogens are found to undergo bioactivation to a range of reactive species or
electrophilic intermediates. This results in the formation of DNA-adducts in the target
tissues or cells.
Genotoxic carcinogen have been found to produce positive results when evaluated by
several invitor genotoxicity tests (Hernández, van Benthem and Johnson 2013).
Genetic damages of the target cells are also induced by these carcinogens, in in-vivo
assays that are performed for shorter duration.
On the other hand, major properties of the non-genotoxic carcinogens are given below:
These carcinogens often fail to exhibit significant effects related to genotoxicity, when
tested in a series of systems
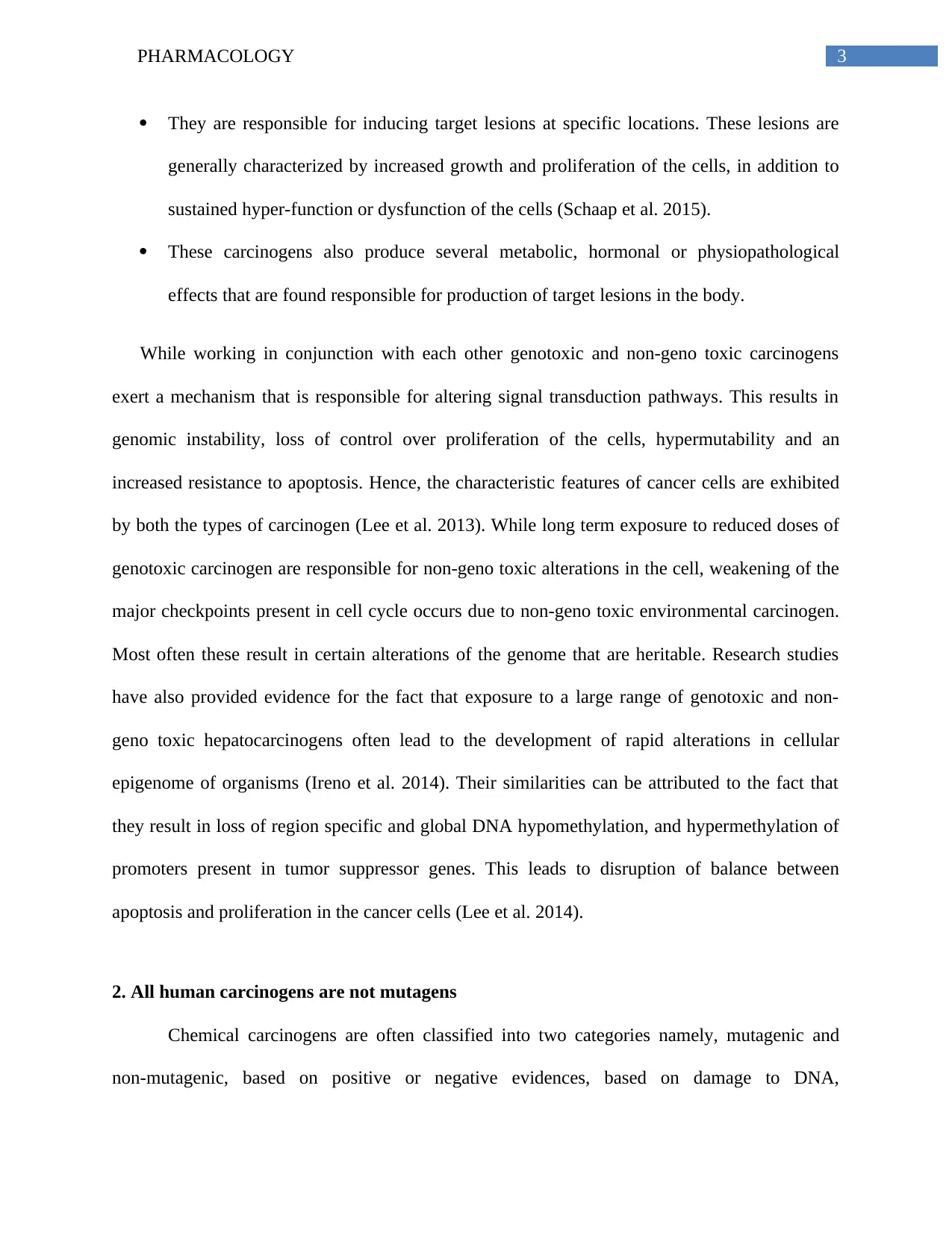
3PHARMACOLOGY
They are responsible for inducing target lesions at specific locations. These lesions are
generally characterized by increased growth and proliferation of the cells, in addition to
sustained hyper-function or dysfunction of the cells (Schaap et al. 2015).
These carcinogens also produce several metabolic, hormonal or physiopathological
effects that are found responsible for production of target lesions in the body.
While working in conjunction with each other genotoxic and non-geno toxic carcinogens
exert a mechanism that is responsible for altering signal transduction pathways. This results in
genomic instability, loss of control over proliferation of the cells, hypermutability and an
increased resistance to apoptosis. Hence, the characteristic features of cancer cells are exhibited
by both the types of carcinogen (Lee et al. 2013). While long term exposure to reduced doses of
genotoxic carcinogen are responsible for non-geno toxic alterations in the cell, weakening of the
major checkpoints present in cell cycle occurs due to non-geno toxic environmental carcinogen.
Most often these result in certain alterations of the genome that are heritable. Research studies
have also provided evidence for the fact that exposure to a large range of genotoxic and non-
geno toxic hepatocarcinogens often lead to the development of rapid alterations in cellular
epigenome of organisms (Ireno et al. 2014). Their similarities can be attributed to the fact that
they result in loss of region specific and global DNA hypomethylation, and hypermethylation of
promoters present in tumor suppressor genes. This leads to disruption of balance between
apoptosis and proliferation in the cancer cells (Lee et al. 2014).
2. All human carcinogens are not mutagens
Chemical carcinogens are often classified into two categories namely, mutagenic and
non-mutagenic, based on positive or negative evidences, based on damage to DNA,
They are responsible for inducing target lesions at specific locations. These lesions are
generally characterized by increased growth and proliferation of the cells, in addition to
sustained hyper-function or dysfunction of the cells (Schaap et al. 2015).
These carcinogens also produce several metabolic, hormonal or physiopathological
effects that are found responsible for production of target lesions in the body.
While working in conjunction with each other genotoxic and non-geno toxic carcinogens
exert a mechanism that is responsible for altering signal transduction pathways. This results in
genomic instability, loss of control over proliferation of the cells, hypermutability and an
increased resistance to apoptosis. Hence, the characteristic features of cancer cells are exhibited
by both the types of carcinogen (Lee et al. 2013). While long term exposure to reduced doses of
genotoxic carcinogen are responsible for non-geno toxic alterations in the cell, weakening of the
major checkpoints present in cell cycle occurs due to non-geno toxic environmental carcinogen.
Most often these result in certain alterations of the genome that are heritable. Research studies
have also provided evidence for the fact that exposure to a large range of genotoxic and non-
geno toxic hepatocarcinogens often lead to the development of rapid alterations in cellular
epigenome of organisms (Ireno et al. 2014). Their similarities can be attributed to the fact that
they result in loss of region specific and global DNA hypomethylation, and hypermethylation of
promoters present in tumor suppressor genes. This leads to disruption of balance between
apoptosis and proliferation in the cancer cells (Lee et al. 2014).
2. All human carcinogens are not mutagens
Chemical carcinogens are often classified into two categories namely, mutagenic and
non-mutagenic, based on positive or negative evidences, based on damage to DNA,
Secure Best Marks with AI Grader
Need help grading? Try our AI Grader for instant feedback on your assignments.
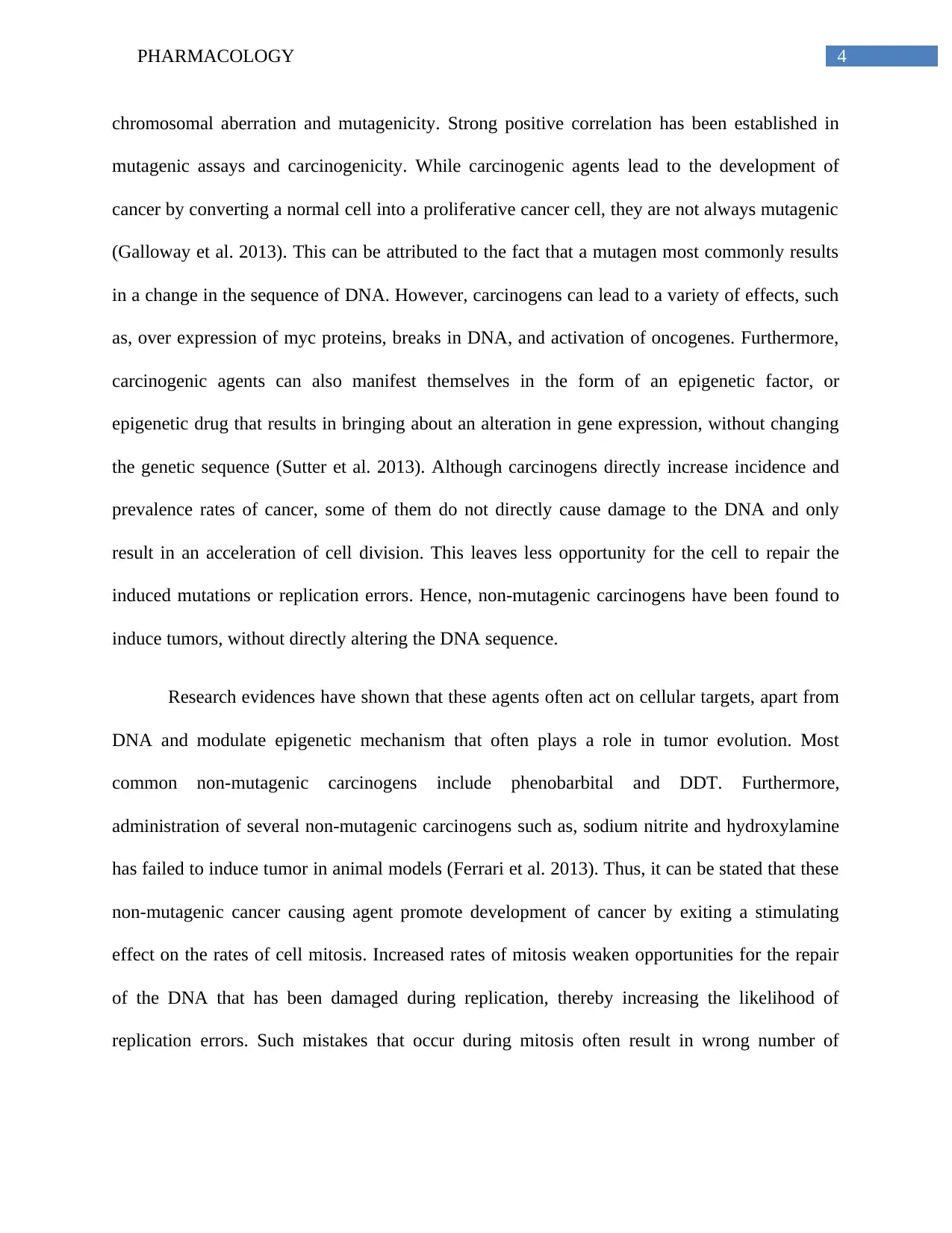
4PHARMACOLOGY
chromosomal aberration and mutagenicity. Strong positive correlation has been established in
mutagenic assays and carcinogenicity. While carcinogenic agents lead to the development of
cancer by converting a normal cell into a proliferative cancer cell, they are not always mutagenic
(Galloway et al. 2013). This can be attributed to the fact that a mutagen most commonly results
in a change in the sequence of DNA. However, carcinogens can lead to a variety of effects, such
as, over expression of myc proteins, breaks in DNA, and activation of oncogenes. Furthermore,
carcinogenic agents can also manifest themselves in the form of an epigenetic factor, or
epigenetic drug that results in bringing about an alteration in gene expression, without changing
the genetic sequence (Sutter et al. 2013). Although carcinogens directly increase incidence and
prevalence rates of cancer, some of them do not directly cause damage to the DNA and only
result in an acceleration of cell division. This leaves less opportunity for the cell to repair the
induced mutations or replication errors. Hence, non-mutagenic carcinogens have been found to
induce tumors, without directly altering the DNA sequence.
Research evidences have shown that these agents often act on cellular targets, apart from
DNA and modulate epigenetic mechanism that often plays a role in tumor evolution. Most
common non-mutagenic carcinogens include phenobarbital and DDT. Furthermore,
administration of several non-mutagenic carcinogens such as, sodium nitrite and hydroxylamine
has failed to induce tumor in animal models (Ferrari et al. 2013). Thus, it can be stated that these
non-mutagenic cancer causing agent promote development of cancer by exiting a stimulating
effect on the rates of cell mitosis. Increased rates of mitosis weaken opportunities for the repair
of the DNA that has been damaged during replication, thereby increasing the likelihood of
replication errors. Such mistakes that occur during mitosis often result in wrong number of
chromosomal aberration and mutagenicity. Strong positive correlation has been established in
mutagenic assays and carcinogenicity. While carcinogenic agents lead to the development of
cancer by converting a normal cell into a proliferative cancer cell, they are not always mutagenic
(Galloway et al. 2013). This can be attributed to the fact that a mutagen most commonly results
in a change in the sequence of DNA. However, carcinogens can lead to a variety of effects, such
as, over expression of myc proteins, breaks in DNA, and activation of oncogenes. Furthermore,
carcinogenic agents can also manifest themselves in the form of an epigenetic factor, or
epigenetic drug that results in bringing about an alteration in gene expression, without changing
the genetic sequence (Sutter et al. 2013). Although carcinogens directly increase incidence and
prevalence rates of cancer, some of them do not directly cause damage to the DNA and only
result in an acceleration of cell division. This leaves less opportunity for the cell to repair the
induced mutations or replication errors. Hence, non-mutagenic carcinogens have been found to
induce tumors, without directly altering the DNA sequence.
Research evidences have shown that these agents often act on cellular targets, apart from
DNA and modulate epigenetic mechanism that often plays a role in tumor evolution. Most
common non-mutagenic carcinogens include phenobarbital and DDT. Furthermore,
administration of several non-mutagenic carcinogens such as, sodium nitrite and hydroxylamine
has failed to induce tumor in animal models (Ferrari et al. 2013). Thus, it can be stated that these
non-mutagenic cancer causing agent promote development of cancer by exiting a stimulating
effect on the rates of cell mitosis. Increased rates of mitosis weaken opportunities for the repair
of the DNA that has been damaged during replication, thereby increasing the likelihood of
replication errors. Such mistakes that occur during mitosis often result in wrong number of
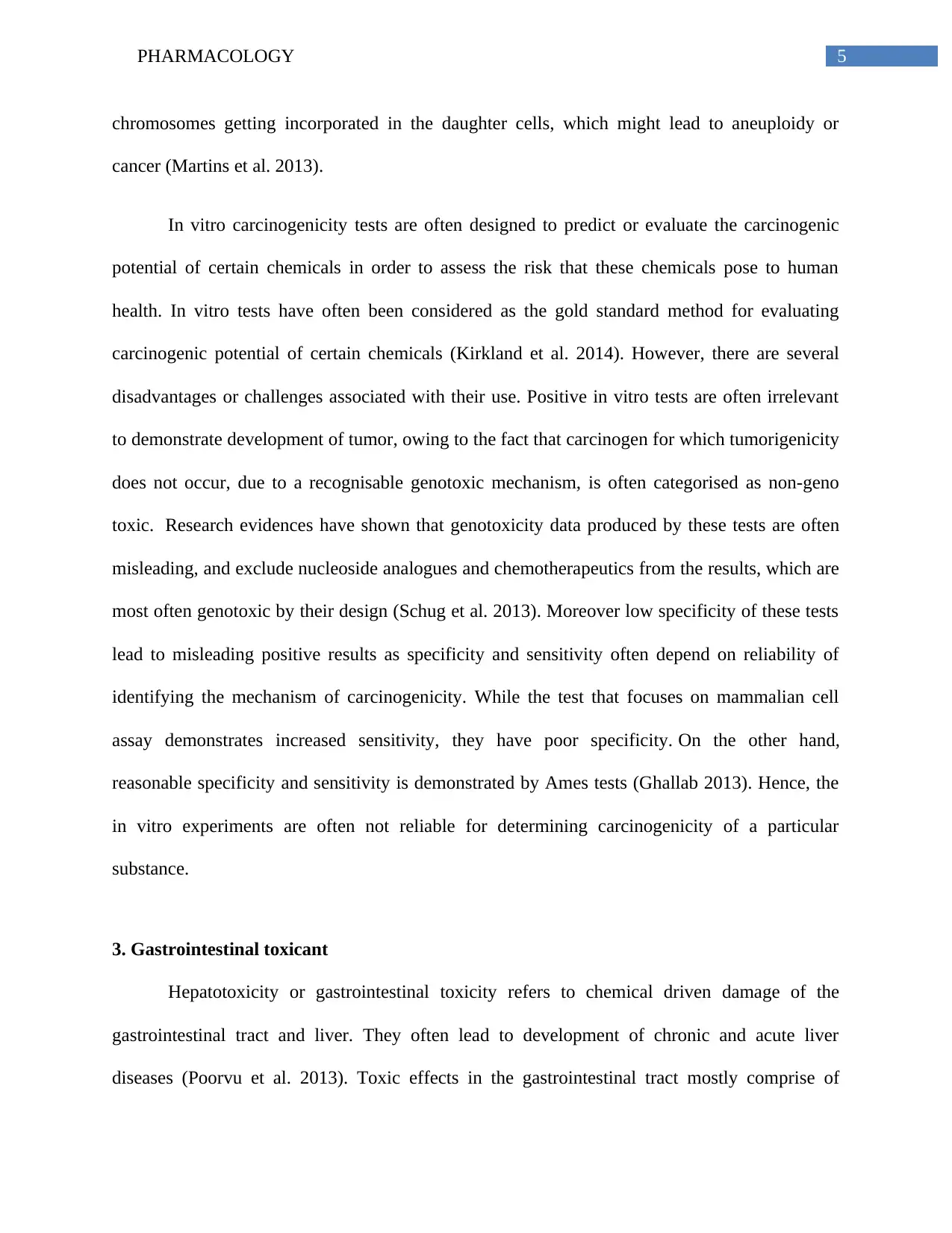
5PHARMACOLOGY
chromosomes getting incorporated in the daughter cells, which might lead to aneuploidy or
cancer (Martins et al. 2013).
In vitro carcinogenicity tests are often designed to predict or evaluate the carcinogenic
potential of certain chemicals in order to assess the risk that these chemicals pose to human
health. In vitro tests have often been considered as the gold standard method for evaluating
carcinogenic potential of certain chemicals (Kirkland et al. 2014). However, there are several
disadvantages or challenges associated with their use. Positive in vitro tests are often irrelevant
to demonstrate development of tumor, owing to the fact that carcinogen for which tumorigenicity
does not occur, due to a recognisable genotoxic mechanism, is often categorised as non-geno
toxic. Research evidences have shown that genotoxicity data produced by these tests are often
misleading, and exclude nucleoside analogues and chemotherapeutics from the results, which are
most often genotoxic by their design (Schug et al. 2013). Moreover low specificity of these tests
lead to misleading positive results as specificity and sensitivity often depend on reliability of
identifying the mechanism of carcinogenicity. While the test that focuses on mammalian cell
assay demonstrates increased sensitivity, they have poor specificity. On the other hand,
reasonable specificity and sensitivity is demonstrated by Ames tests (Ghallab 2013). Hence, the
in vitro experiments are often not reliable for determining carcinogenicity of a particular
substance.
3. Gastrointestinal toxicant
Hepatotoxicity or gastrointestinal toxicity refers to chemical driven damage of the
gastrointestinal tract and liver. They often lead to development of chronic and acute liver
diseases (Poorvu et al. 2013). Toxic effects in the gastrointestinal tract mostly comprise of
chromosomes getting incorporated in the daughter cells, which might lead to aneuploidy or
cancer (Martins et al. 2013).
In vitro carcinogenicity tests are often designed to predict or evaluate the carcinogenic
potential of certain chemicals in order to assess the risk that these chemicals pose to human
health. In vitro tests have often been considered as the gold standard method for evaluating
carcinogenic potential of certain chemicals (Kirkland et al. 2014). However, there are several
disadvantages or challenges associated with their use. Positive in vitro tests are often irrelevant
to demonstrate development of tumor, owing to the fact that carcinogen for which tumorigenicity
does not occur, due to a recognisable genotoxic mechanism, is often categorised as non-geno
toxic. Research evidences have shown that genotoxicity data produced by these tests are often
misleading, and exclude nucleoside analogues and chemotherapeutics from the results, which are
most often genotoxic by their design (Schug et al. 2013). Moreover low specificity of these tests
lead to misleading positive results as specificity and sensitivity often depend on reliability of
identifying the mechanism of carcinogenicity. While the test that focuses on mammalian cell
assay demonstrates increased sensitivity, they have poor specificity. On the other hand,
reasonable specificity and sensitivity is demonstrated by Ames tests (Ghallab 2013). Hence, the
in vitro experiments are often not reliable for determining carcinogenicity of a particular
substance.
3. Gastrointestinal toxicant
Hepatotoxicity or gastrointestinal toxicity refers to chemical driven damage of the
gastrointestinal tract and liver. They often lead to development of chronic and acute liver
diseases (Poorvu et al. 2013). Toxic effects in the gastrointestinal tract mostly comprise of
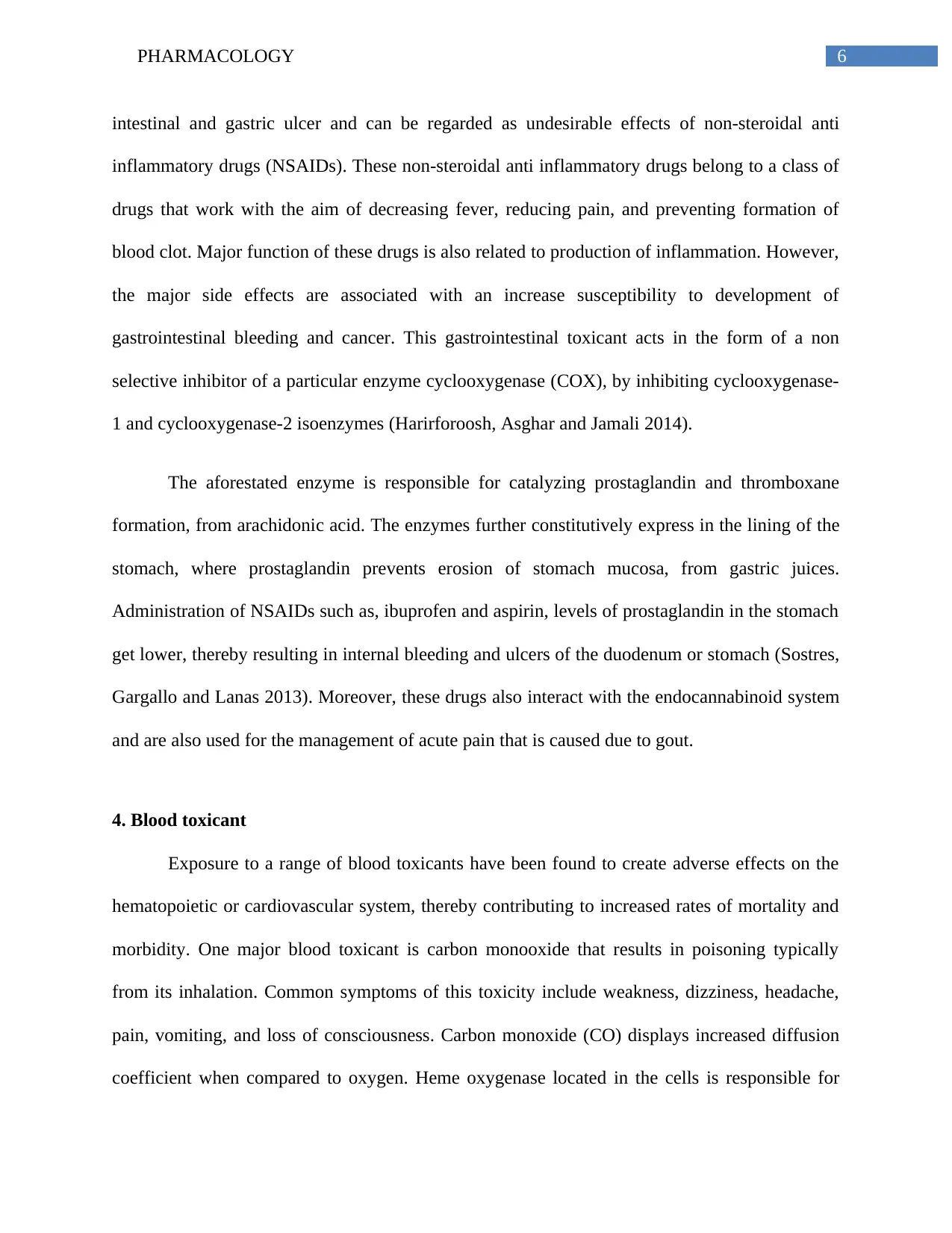
6PHARMACOLOGY
intestinal and gastric ulcer and can be regarded as undesirable effects of non-steroidal anti
inflammatory drugs (NSAIDs). These non-steroidal anti inflammatory drugs belong to a class of
drugs that work with the aim of decreasing fever, reducing pain, and preventing formation of
blood clot. Major function of these drugs is also related to production of inflammation. However,
the major side effects are associated with an increase susceptibility to development of
gastrointestinal bleeding and cancer. This gastrointestinal toxicant acts in the form of a non
selective inhibitor of a particular enzyme cyclooxygenase (COX), by inhibiting cyclooxygenase-
1 and cyclooxygenase-2 isoenzymes (Harirforoosh, Asghar and Jamali 2014).
The aforestated enzyme is responsible for catalyzing prostaglandin and thromboxane
formation, from arachidonic acid. The enzymes further constitutively express in the lining of the
stomach, where prostaglandin prevents erosion of stomach mucosa, from gastric juices.
Administration of NSAIDs such as, ibuprofen and aspirin, levels of prostaglandin in the stomach
get lower, thereby resulting in internal bleeding and ulcers of the duodenum or stomach (Sostres,
Gargallo and Lanas 2013). Moreover, these drugs also interact with the endocannabinoid system
and are also used for the management of acute pain that is caused due to gout.
4. Blood toxicant
Exposure to a range of blood toxicants have been found to create adverse effects on the
hematopoietic or cardiovascular system, thereby contributing to increased rates of mortality and
morbidity. One major blood toxicant is carbon monooxide that results in poisoning typically
from its inhalation. Common symptoms of this toxicity include weakness, dizziness, headache,
pain, vomiting, and loss of consciousness. Carbon monoxide (CO) displays increased diffusion
coefficient when compared to oxygen. Heme oxygenase located in the cells is responsible for
intestinal and gastric ulcer and can be regarded as undesirable effects of non-steroidal anti
inflammatory drugs (NSAIDs). These non-steroidal anti inflammatory drugs belong to a class of
drugs that work with the aim of decreasing fever, reducing pain, and preventing formation of
blood clot. Major function of these drugs is also related to production of inflammation. However,
the major side effects are associated with an increase susceptibility to development of
gastrointestinal bleeding and cancer. This gastrointestinal toxicant acts in the form of a non
selective inhibitor of a particular enzyme cyclooxygenase (COX), by inhibiting cyclooxygenase-
1 and cyclooxygenase-2 isoenzymes (Harirforoosh, Asghar and Jamali 2014).
The aforestated enzyme is responsible for catalyzing prostaglandin and thromboxane
formation, from arachidonic acid. The enzymes further constitutively express in the lining of the
stomach, where prostaglandin prevents erosion of stomach mucosa, from gastric juices.
Administration of NSAIDs such as, ibuprofen and aspirin, levels of prostaglandin in the stomach
get lower, thereby resulting in internal bleeding and ulcers of the duodenum or stomach (Sostres,
Gargallo and Lanas 2013). Moreover, these drugs also interact with the endocannabinoid system
and are also used for the management of acute pain that is caused due to gout.
4. Blood toxicant
Exposure to a range of blood toxicants have been found to create adverse effects on the
hematopoietic or cardiovascular system, thereby contributing to increased rates of mortality and
morbidity. One major blood toxicant is carbon monooxide that results in poisoning typically
from its inhalation. Common symptoms of this toxicity include weakness, dizziness, headache,
pain, vomiting, and loss of consciousness. Carbon monoxide (CO) displays increased diffusion
coefficient when compared to oxygen. Heme oxygenase located in the cells is responsible for
Paraphrase This Document
Need a fresh take? Get an instant paraphrase of this document with our AI Paraphraser
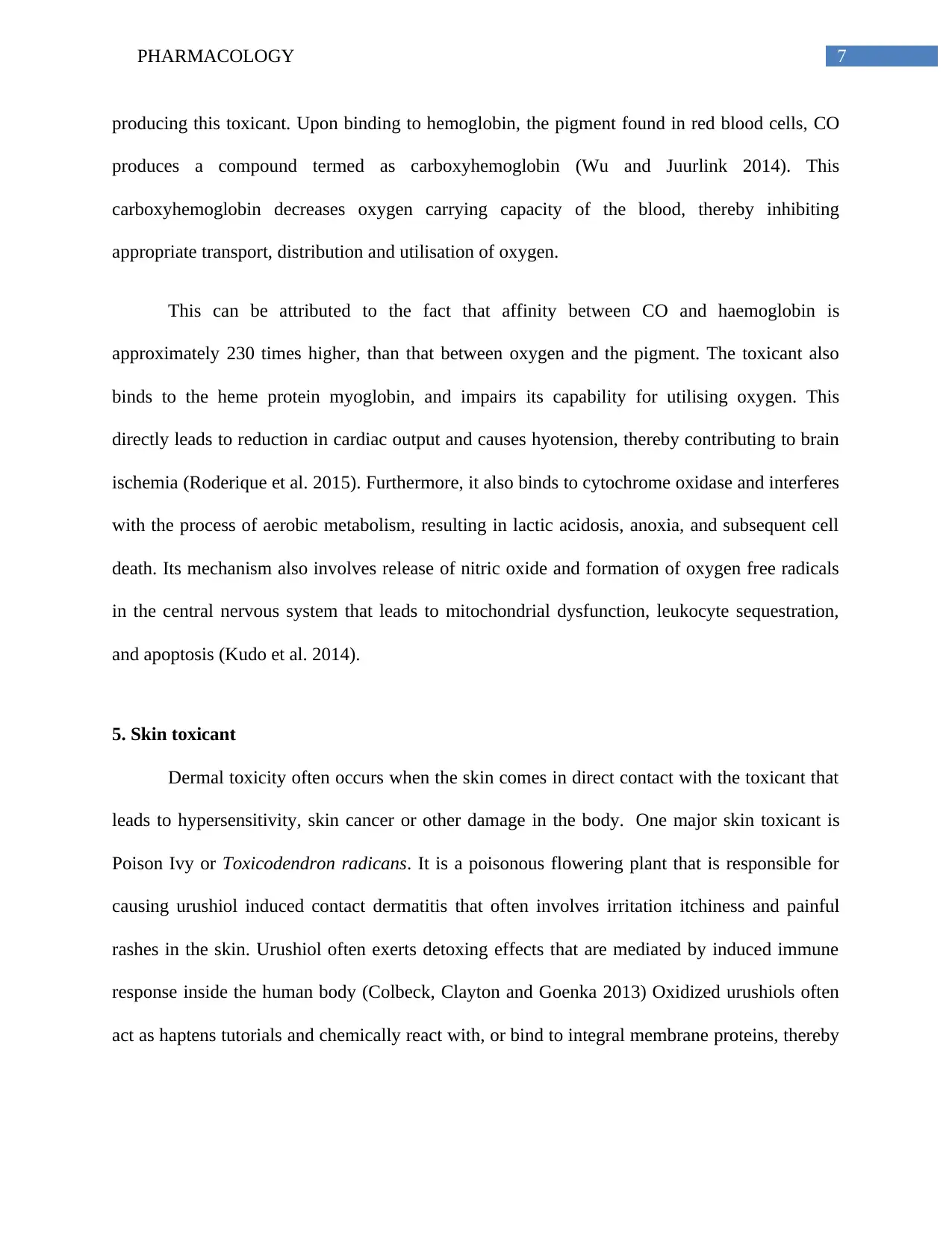
7PHARMACOLOGY
producing this toxicant. Upon binding to hemoglobin, the pigment found in red blood cells, CO
produces a compound termed as carboxyhemoglobin (Wu and Juurlink 2014). This
carboxyhemoglobin decreases oxygen carrying capacity of the blood, thereby inhibiting
appropriate transport, distribution and utilisation of oxygen.
This can be attributed to the fact that affinity between CO and haemoglobin is
approximately 230 times higher, than that between oxygen and the pigment. The toxicant also
binds to the heme protein myoglobin, and impairs its capability for utilising oxygen. This
directly leads to reduction in cardiac output and causes hyotension, thereby contributing to brain
ischemia (Roderique et al. 2015). Furthermore, it also binds to cytochrome oxidase and interferes
with the process of aerobic metabolism, resulting in lactic acidosis, anoxia, and subsequent cell
death. Its mechanism also involves release of nitric oxide and formation of oxygen free radicals
in the central nervous system that leads to mitochondrial dysfunction, leukocyte sequestration,
and apoptosis (Kudo et al. 2014).
5. Skin toxicant
Dermal toxicity often occurs when the skin comes in direct contact with the toxicant that
leads to hypersensitivity, skin cancer or other damage in the body. One major skin toxicant is
Poison Ivy or Toxicodendron radicans. It is a poisonous flowering plant that is responsible for
causing urushiol induced contact dermatitis that often involves irritation itchiness and painful
rashes in the skin. Urushiol often exerts detoxing effects that are mediated by induced immune
response inside the human body (Colbeck, Clayton and Goenka 2013) Oxidized urushiols often
act as haptens tutorials and chemically react with, or bind to integral membrane proteins, thereby
producing this toxicant. Upon binding to hemoglobin, the pigment found in red blood cells, CO
produces a compound termed as carboxyhemoglobin (Wu and Juurlink 2014). This
carboxyhemoglobin decreases oxygen carrying capacity of the blood, thereby inhibiting
appropriate transport, distribution and utilisation of oxygen.
This can be attributed to the fact that affinity between CO and haemoglobin is
approximately 230 times higher, than that between oxygen and the pigment. The toxicant also
binds to the heme protein myoglobin, and impairs its capability for utilising oxygen. This
directly leads to reduction in cardiac output and causes hyotension, thereby contributing to brain
ischemia (Roderique et al. 2015). Furthermore, it also binds to cytochrome oxidase and interferes
with the process of aerobic metabolism, resulting in lactic acidosis, anoxia, and subsequent cell
death. Its mechanism also involves release of nitric oxide and formation of oxygen free radicals
in the central nervous system that leads to mitochondrial dysfunction, leukocyte sequestration,
and apoptosis (Kudo et al. 2014).
5. Skin toxicant
Dermal toxicity often occurs when the skin comes in direct contact with the toxicant that
leads to hypersensitivity, skin cancer or other damage in the body. One major skin toxicant is
Poison Ivy or Toxicodendron radicans. It is a poisonous flowering plant that is responsible for
causing urushiol induced contact dermatitis that often involves irritation itchiness and painful
rashes in the skin. Urushiol often exerts detoxing effects that are mediated by induced immune
response inside the human body (Colbeck, Clayton and Goenka 2013) Oxidized urushiols often
act as haptens tutorials and chemically react with, or bind to integral membrane proteins, thereby
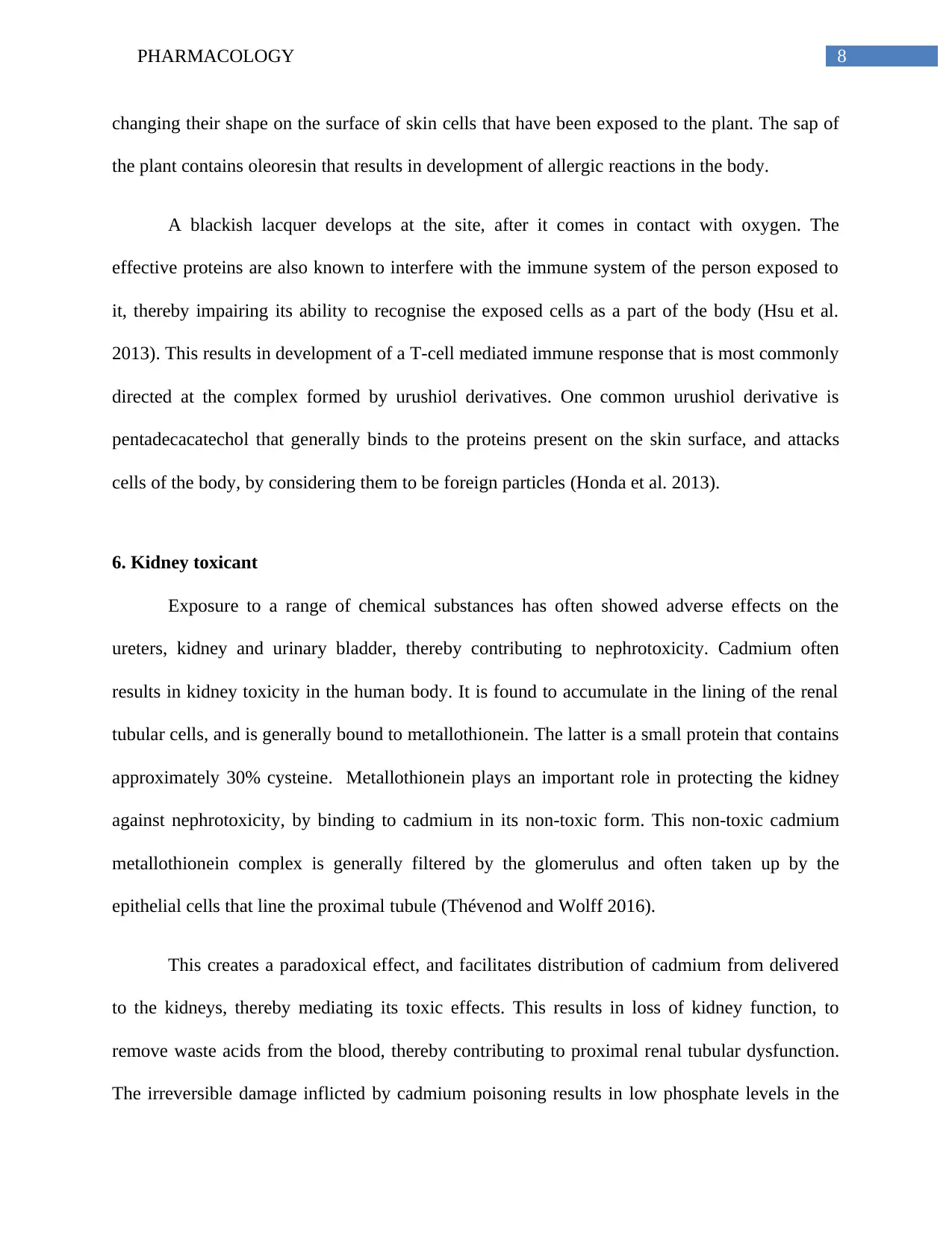
8PHARMACOLOGY
changing their shape on the surface of skin cells that have been exposed to the plant. The sap of
the plant contains oleoresin that results in development of allergic reactions in the body.
A blackish lacquer develops at the site, after it comes in contact with oxygen. The
effective proteins are also known to interfere with the immune system of the person exposed to
it, thereby impairing its ability to recognise the exposed cells as a part of the body (Hsu et al.
2013). This results in development of a T-cell mediated immune response that is most commonly
directed at the complex formed by urushiol derivatives. One common urushiol derivative is
pentadecacatechol that generally binds to the proteins present on the skin surface, and attacks
cells of the body, by considering them to be foreign particles (Honda et al. 2013).
6. Kidney toxicant
Exposure to a range of chemical substances has often showed adverse effects on the
ureters, kidney and urinary bladder, thereby contributing to nephrotoxicity. Cadmium often
results in kidney toxicity in the human body. It is found to accumulate in the lining of the renal
tubular cells, and is generally bound to metallothionein. The latter is a small protein that contains
approximately 30% cysteine. Metallothionein plays an important role in protecting the kidney
against nephrotoxicity, by binding to cadmium in its non-toxic form. This non-toxic cadmium
metallothionein complex is generally filtered by the glomerulus and often taken up by the
epithelial cells that line the proximal tubule (Thévenod and Wolff 2016).
This creates a paradoxical effect, and facilitates distribution of cadmium from delivered
to the kidneys, thereby mediating its toxic effects. This results in loss of kidney function, to
remove waste acids from the blood, thereby contributing to proximal renal tubular dysfunction.
The irreversible damage inflicted by cadmium poisoning results in low phosphate levels in the
changing their shape on the surface of skin cells that have been exposed to the plant. The sap of
the plant contains oleoresin that results in development of allergic reactions in the body.
A blackish lacquer develops at the site, after it comes in contact with oxygen. The
effective proteins are also known to interfere with the immune system of the person exposed to
it, thereby impairing its ability to recognise the exposed cells as a part of the body (Hsu et al.
2013). This results in development of a T-cell mediated immune response that is most commonly
directed at the complex formed by urushiol derivatives. One common urushiol derivative is
pentadecacatechol that generally binds to the proteins present on the skin surface, and attacks
cells of the body, by considering them to be foreign particles (Honda et al. 2013).
6. Kidney toxicant
Exposure to a range of chemical substances has often showed adverse effects on the
ureters, kidney and urinary bladder, thereby contributing to nephrotoxicity. Cadmium often
results in kidney toxicity in the human body. It is found to accumulate in the lining of the renal
tubular cells, and is generally bound to metallothionein. The latter is a small protein that contains
approximately 30% cysteine. Metallothionein plays an important role in protecting the kidney
against nephrotoxicity, by binding to cadmium in its non-toxic form. This non-toxic cadmium
metallothionein complex is generally filtered by the glomerulus and often taken up by the
epithelial cells that line the proximal tubule (Thévenod and Wolff 2016).
This creates a paradoxical effect, and facilitates distribution of cadmium from delivered
to the kidneys, thereby mediating its toxic effects. This results in loss of kidney function, to
remove waste acids from the blood, thereby contributing to proximal renal tubular dysfunction.
The irreversible damage inflicted by cadmium poisoning results in low phosphate levels in the
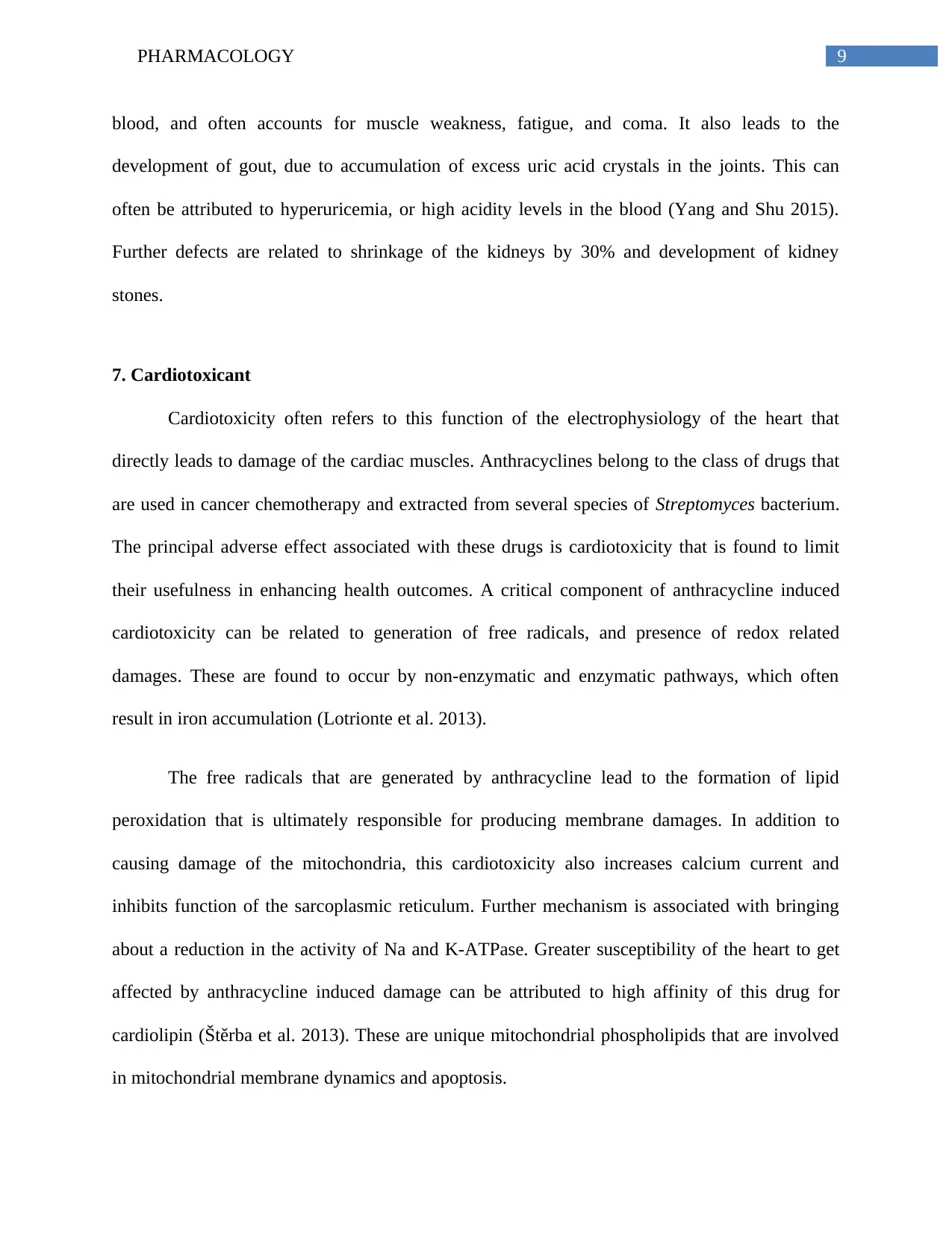
9PHARMACOLOGY
blood, and often accounts for muscle weakness, fatigue, and coma. It also leads to the
development of gout, due to accumulation of excess uric acid crystals in the joints. This can
often be attributed to hyperuricemia, or high acidity levels in the blood (Yang and Shu 2015).
Further defects are related to shrinkage of the kidneys by 30% and development of kidney
stones.
7. Cardiotoxicant
Cardiotoxicity often refers to this function of the electrophysiology of the heart that
directly leads to damage of the cardiac muscles. Anthracyclines belong to the class of drugs that
are used in cancer chemotherapy and extracted from several species of Streptomyces bacterium.
The principal adverse effect associated with these drugs is cardiotoxicity that is found to limit
their usefulness in enhancing health outcomes. A critical component of anthracycline induced
cardiotoxicity can be related to generation of free radicals, and presence of redox related
damages. These are found to occur by non-enzymatic and enzymatic pathways, which often
result in iron accumulation (Lotrionte et al. 2013).
The free radicals that are generated by anthracycline lead to the formation of lipid
peroxidation that is ultimately responsible for producing membrane damages. In addition to
causing damage of the mitochondria, this cardiotoxicity also increases calcium current and
inhibits function of the sarcoplasmic reticulum. Further mechanism is associated with bringing
about a reduction in the activity of Na and K-ATPase. Greater susceptibility of the heart to get
affected by anthracycline induced damage can be attributed to high affinity of this drug for
cardiolipin (Štěrba et al. 2013). These are unique mitochondrial phospholipids that are involved
in mitochondrial membrane dynamics and apoptosis.
blood, and often accounts for muscle weakness, fatigue, and coma. It also leads to the
development of gout, due to accumulation of excess uric acid crystals in the joints. This can
often be attributed to hyperuricemia, or high acidity levels in the blood (Yang and Shu 2015).
Further defects are related to shrinkage of the kidneys by 30% and development of kidney
stones.
7. Cardiotoxicant
Cardiotoxicity often refers to this function of the electrophysiology of the heart that
directly leads to damage of the cardiac muscles. Anthracyclines belong to the class of drugs that
are used in cancer chemotherapy and extracted from several species of Streptomyces bacterium.
The principal adverse effect associated with these drugs is cardiotoxicity that is found to limit
their usefulness in enhancing health outcomes. A critical component of anthracycline induced
cardiotoxicity can be related to generation of free radicals, and presence of redox related
damages. These are found to occur by non-enzymatic and enzymatic pathways, which often
result in iron accumulation (Lotrionte et al. 2013).
The free radicals that are generated by anthracycline lead to the formation of lipid
peroxidation that is ultimately responsible for producing membrane damages. In addition to
causing damage of the mitochondria, this cardiotoxicity also increases calcium current and
inhibits function of the sarcoplasmic reticulum. Further mechanism is associated with bringing
about a reduction in the activity of Na and K-ATPase. Greater susceptibility of the heart to get
affected by anthracycline induced damage can be attributed to high affinity of this drug for
cardiolipin (Štěrba et al. 2013). These are unique mitochondrial phospholipids that are involved
in mitochondrial membrane dynamics and apoptosis.
Secure Best Marks with AI Grader
Need help grading? Try our AI Grader for instant feedback on your assignments.
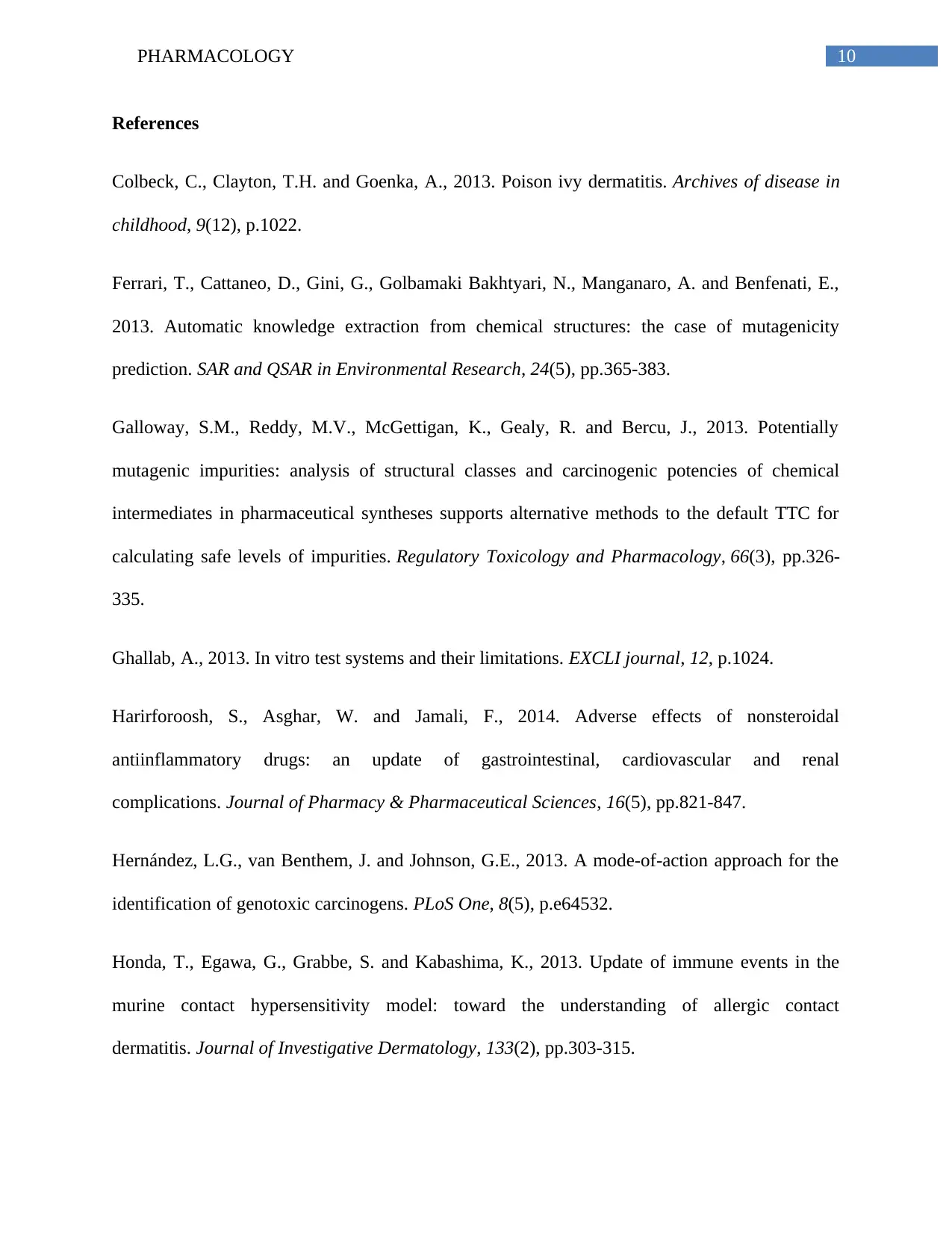
10PHARMACOLOGY
References
Colbeck, C., Clayton, T.H. and Goenka, A., 2013. Poison ivy dermatitis. Archives of disease in
childhood, 9(12), p.1022.
Ferrari, T., Cattaneo, D., Gini, G., Golbamaki Bakhtyari, N., Manganaro, A. and Benfenati, E.,
2013. Automatic knowledge extraction from chemical structures: the case of mutagenicity
prediction. SAR and QSAR in Environmental Research, 24(5), pp.365-383.
Galloway, S.M., Reddy, M.V., McGettigan, K., Gealy, R. and Bercu, J., 2013. Potentially
mutagenic impurities: analysis of structural classes and carcinogenic potencies of chemical
intermediates in pharmaceutical syntheses supports alternative methods to the default TTC for
calculating safe levels of impurities. Regulatory Toxicology and Pharmacology, 66(3), pp.326-
335.
Ghallab, A., 2013. In vitro test systems and their limitations. EXCLI journal, 12, p.1024.
Harirforoosh, S., Asghar, W. and Jamali, F., 2014. Adverse effects of nonsteroidal
antiinflammatory drugs: an update of gastrointestinal, cardiovascular and renal
complications. Journal of Pharmacy & Pharmaceutical Sciences, 16(5), pp.821-847.
Hernández, L.G., van Benthem, J. and Johnson, G.E., 2013. A mode-of-action approach for the
identification of genotoxic carcinogens. PLoS One, 8(5), p.e64532.
Honda, T., Egawa, G., Grabbe, S. and Kabashima, K., 2013. Update of immune events in the
murine contact hypersensitivity model: toward the understanding of allergic contact
dermatitis. Journal of Investigative Dermatology, 133(2), pp.303-315.
References
Colbeck, C., Clayton, T.H. and Goenka, A., 2013. Poison ivy dermatitis. Archives of disease in
childhood, 9(12), p.1022.
Ferrari, T., Cattaneo, D., Gini, G., Golbamaki Bakhtyari, N., Manganaro, A. and Benfenati, E.,
2013. Automatic knowledge extraction from chemical structures: the case of mutagenicity
prediction. SAR and QSAR in Environmental Research, 24(5), pp.365-383.
Galloway, S.M., Reddy, M.V., McGettigan, K., Gealy, R. and Bercu, J., 2013. Potentially
mutagenic impurities: analysis of structural classes and carcinogenic potencies of chemical
intermediates in pharmaceutical syntheses supports alternative methods to the default TTC for
calculating safe levels of impurities. Regulatory Toxicology and Pharmacology, 66(3), pp.326-
335.
Ghallab, A., 2013. In vitro test systems and their limitations. EXCLI journal, 12, p.1024.
Harirforoosh, S., Asghar, W. and Jamali, F., 2014. Adverse effects of nonsteroidal
antiinflammatory drugs: an update of gastrointestinal, cardiovascular and renal
complications. Journal of Pharmacy & Pharmaceutical Sciences, 16(5), pp.821-847.
Hernández, L.G., van Benthem, J. and Johnson, G.E., 2013. A mode-of-action approach for the
identification of genotoxic carcinogens. PLoS One, 8(5), p.e64532.
Honda, T., Egawa, G., Grabbe, S. and Kabashima, K., 2013. Update of immune events in the
murine contact hypersensitivity model: toward the understanding of allergic contact
dermatitis. Journal of Investigative Dermatology, 133(2), pp.303-315.
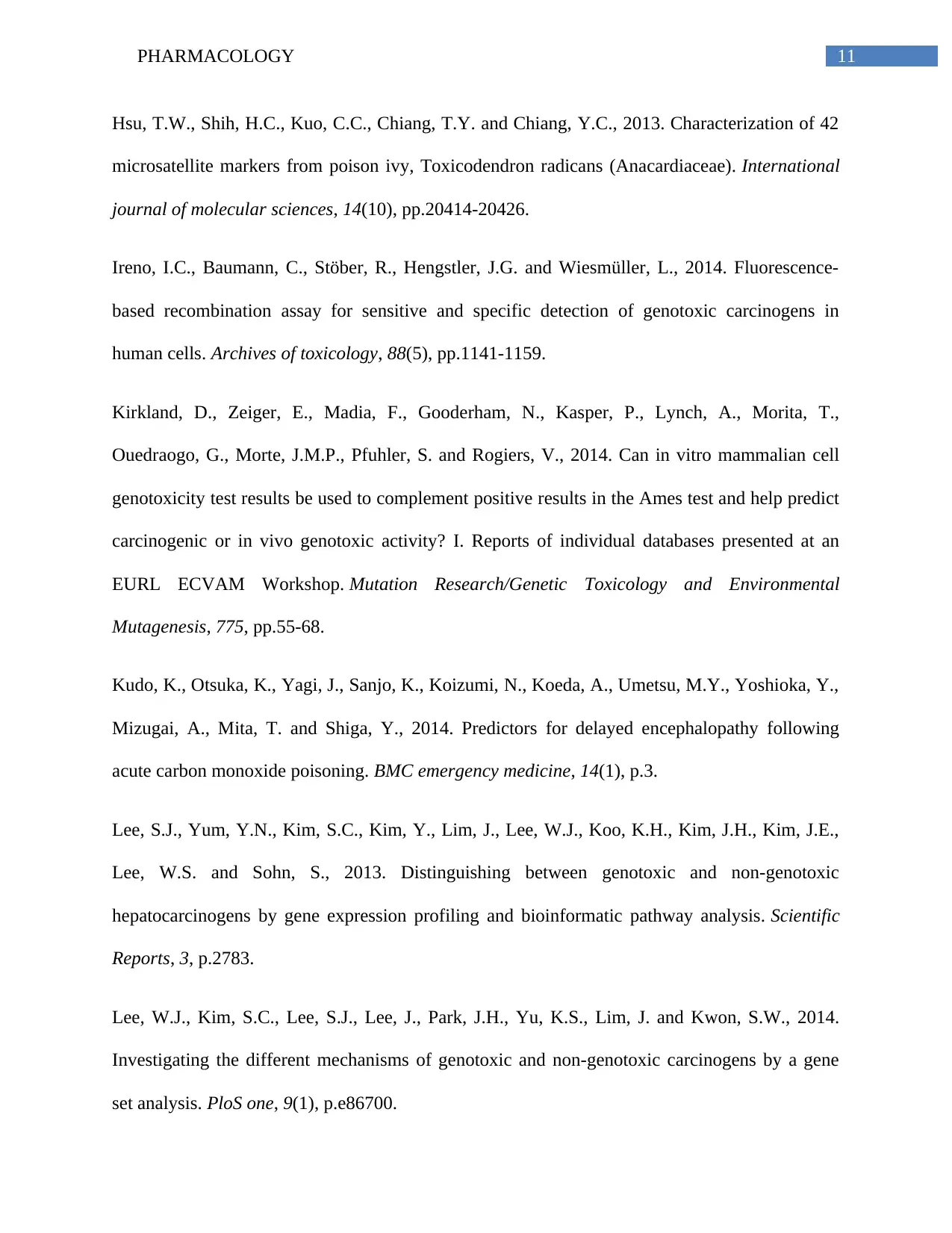
11PHARMACOLOGY
Hsu, T.W., Shih, H.C., Kuo, C.C., Chiang, T.Y. and Chiang, Y.C., 2013. Characterization of 42
microsatellite markers from poison ivy, Toxicodendron radicans (Anacardiaceae). International
journal of molecular sciences, 14(10), pp.20414-20426.
Ireno, I.C., Baumann, C., Stöber, R., Hengstler, J.G. and Wiesmüller, L., 2014. Fluorescence-
based recombination assay for sensitive and specific detection of genotoxic carcinogens in
human cells. Archives of toxicology, 88(5), pp.1141-1159.
Kirkland, D., Zeiger, E., Madia, F., Gooderham, N., Kasper, P., Lynch, A., Morita, T.,
Ouedraogo, G., Morte, J.M.P., Pfuhler, S. and Rogiers, V., 2014. Can in vitro mammalian cell
genotoxicity test results be used to complement positive results in the Ames test and help predict
carcinogenic or in vivo genotoxic activity? I. Reports of individual databases presented at an
EURL ECVAM Workshop. Mutation Research/Genetic Toxicology and Environmental
Mutagenesis, 775, pp.55-68.
Kudo, K., Otsuka, K., Yagi, J., Sanjo, K., Koizumi, N., Koeda, A., Umetsu, M.Y., Yoshioka, Y.,
Mizugai, A., Mita, T. and Shiga, Y., 2014. Predictors for delayed encephalopathy following
acute carbon monoxide poisoning. BMC emergency medicine, 14(1), p.3.
Lee, S.J., Yum, Y.N., Kim, S.C., Kim, Y., Lim, J., Lee, W.J., Koo, K.H., Kim, J.H., Kim, J.E.,
Lee, W.S. and Sohn, S., 2013. Distinguishing between genotoxic and non-genotoxic
hepatocarcinogens by gene expression profiling and bioinformatic pathway analysis. Scientific
Reports, 3, p.2783.
Lee, W.J., Kim, S.C., Lee, S.J., Lee, J., Park, J.H., Yu, K.S., Lim, J. and Kwon, S.W., 2014.
Investigating the different mechanisms of genotoxic and non-genotoxic carcinogens by a gene
set analysis. PloS one, 9(1), p.e86700.
Hsu, T.W., Shih, H.C., Kuo, C.C., Chiang, T.Y. and Chiang, Y.C., 2013. Characterization of 42
microsatellite markers from poison ivy, Toxicodendron radicans (Anacardiaceae). International
journal of molecular sciences, 14(10), pp.20414-20426.
Ireno, I.C., Baumann, C., Stöber, R., Hengstler, J.G. and Wiesmüller, L., 2014. Fluorescence-
based recombination assay for sensitive and specific detection of genotoxic carcinogens in
human cells. Archives of toxicology, 88(5), pp.1141-1159.
Kirkland, D., Zeiger, E., Madia, F., Gooderham, N., Kasper, P., Lynch, A., Morita, T.,
Ouedraogo, G., Morte, J.M.P., Pfuhler, S. and Rogiers, V., 2014. Can in vitro mammalian cell
genotoxicity test results be used to complement positive results in the Ames test and help predict
carcinogenic or in vivo genotoxic activity? I. Reports of individual databases presented at an
EURL ECVAM Workshop. Mutation Research/Genetic Toxicology and Environmental
Mutagenesis, 775, pp.55-68.
Kudo, K., Otsuka, K., Yagi, J., Sanjo, K., Koizumi, N., Koeda, A., Umetsu, M.Y., Yoshioka, Y.,
Mizugai, A., Mita, T. and Shiga, Y., 2014. Predictors for delayed encephalopathy following
acute carbon monoxide poisoning. BMC emergency medicine, 14(1), p.3.
Lee, S.J., Yum, Y.N., Kim, S.C., Kim, Y., Lim, J., Lee, W.J., Koo, K.H., Kim, J.H., Kim, J.E.,
Lee, W.S. and Sohn, S., 2013. Distinguishing between genotoxic and non-genotoxic
hepatocarcinogens by gene expression profiling and bioinformatic pathway analysis. Scientific
Reports, 3, p.2783.
Lee, W.J., Kim, S.C., Lee, S.J., Lee, J., Park, J.H., Yu, K.S., Lim, J. and Kwon, S.W., 2014.
Investigating the different mechanisms of genotoxic and non-genotoxic carcinogens by a gene
set analysis. PloS one, 9(1), p.e86700.
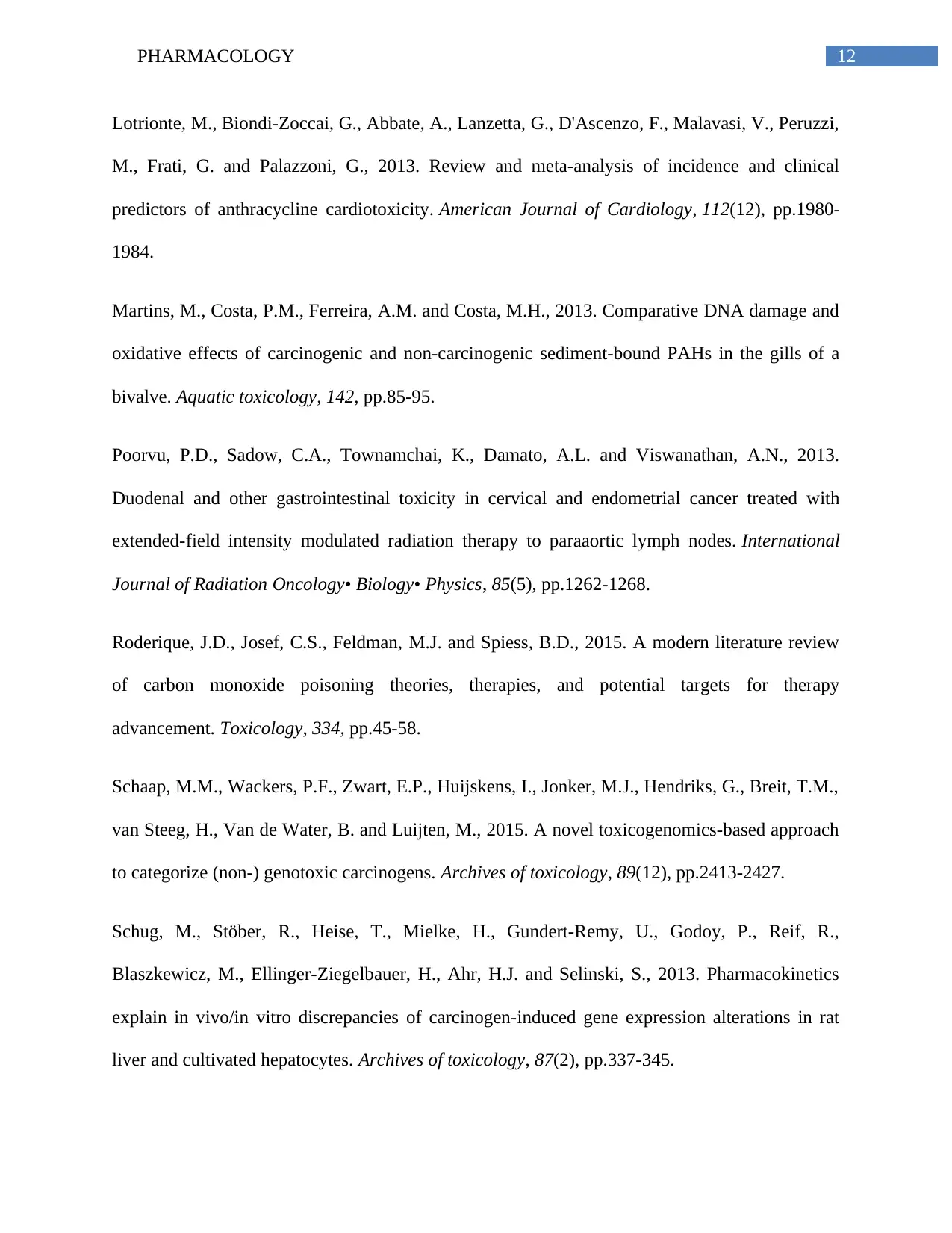
12PHARMACOLOGY
Lotrionte, M., Biondi-Zoccai, G., Abbate, A., Lanzetta, G., D'Ascenzo, F., Malavasi, V., Peruzzi,
M., Frati, G. and Palazzoni, G., 2013. Review and meta-analysis of incidence and clinical
predictors of anthracycline cardiotoxicity. American Journal of Cardiology, 112(12), pp.1980-
1984.
Martins, M., Costa, P.M., Ferreira, A.M. and Costa, M.H., 2013. Comparative DNA damage and
oxidative effects of carcinogenic and non-carcinogenic sediment-bound PAHs in the gills of a
bivalve. Aquatic toxicology, 142, pp.85-95.
Poorvu, P.D., Sadow, C.A., Townamchai, K., Damato, A.L. and Viswanathan, A.N., 2013.
Duodenal and other gastrointestinal toxicity in cervical and endometrial cancer treated with
extended-field intensity modulated radiation therapy to paraaortic lymph nodes. International
Journal of Radiation Oncology• Biology• Physics, 85(5), pp.1262-1268.
Roderique, J.D., Josef, C.S., Feldman, M.J. and Spiess, B.D., 2015. A modern literature review
of carbon monoxide poisoning theories, therapies, and potential targets for therapy
advancement. Toxicology, 334, pp.45-58.
Schaap, M.M., Wackers, P.F., Zwart, E.P., Huijskens, I., Jonker, M.J., Hendriks, G., Breit, T.M.,
van Steeg, H., Van de Water, B. and Luijten, M., 2015. A novel toxicogenomics-based approach
to categorize (non-) genotoxic carcinogens. Archives of toxicology, 89(12), pp.2413-2427.
Schug, M., Stöber, R., Heise, T., Mielke, H., Gundert-Remy, U., Godoy, P., Reif, R.,
Blaszkewicz, M., Ellinger-Ziegelbauer, H., Ahr, H.J. and Selinski, S., 2013. Pharmacokinetics
explain in vivo/in vitro discrepancies of carcinogen-induced gene expression alterations in rat
liver and cultivated hepatocytes. Archives of toxicology, 87(2), pp.337-345.
Lotrionte, M., Biondi-Zoccai, G., Abbate, A., Lanzetta, G., D'Ascenzo, F., Malavasi, V., Peruzzi,
M., Frati, G. and Palazzoni, G., 2013. Review and meta-analysis of incidence and clinical
predictors of anthracycline cardiotoxicity. American Journal of Cardiology, 112(12), pp.1980-
1984.
Martins, M., Costa, P.M., Ferreira, A.M. and Costa, M.H., 2013. Comparative DNA damage and
oxidative effects of carcinogenic and non-carcinogenic sediment-bound PAHs in the gills of a
bivalve. Aquatic toxicology, 142, pp.85-95.
Poorvu, P.D., Sadow, C.A., Townamchai, K., Damato, A.L. and Viswanathan, A.N., 2013.
Duodenal and other gastrointestinal toxicity in cervical and endometrial cancer treated with
extended-field intensity modulated radiation therapy to paraaortic lymph nodes. International
Journal of Radiation Oncology• Biology• Physics, 85(5), pp.1262-1268.
Roderique, J.D., Josef, C.S., Feldman, M.J. and Spiess, B.D., 2015. A modern literature review
of carbon monoxide poisoning theories, therapies, and potential targets for therapy
advancement. Toxicology, 334, pp.45-58.
Schaap, M.M., Wackers, P.F., Zwart, E.P., Huijskens, I., Jonker, M.J., Hendriks, G., Breit, T.M.,
van Steeg, H., Van de Water, B. and Luijten, M., 2015. A novel toxicogenomics-based approach
to categorize (non-) genotoxic carcinogens. Archives of toxicology, 89(12), pp.2413-2427.
Schug, M., Stöber, R., Heise, T., Mielke, H., Gundert-Remy, U., Godoy, P., Reif, R.,
Blaszkewicz, M., Ellinger-Ziegelbauer, H., Ahr, H.J. and Selinski, S., 2013. Pharmacokinetics
explain in vivo/in vitro discrepancies of carcinogen-induced gene expression alterations in rat
liver and cultivated hepatocytes. Archives of toxicology, 87(2), pp.337-345.
Paraphrase This Document
Need a fresh take? Get an instant paraphrase of this document with our AI Paraphraser
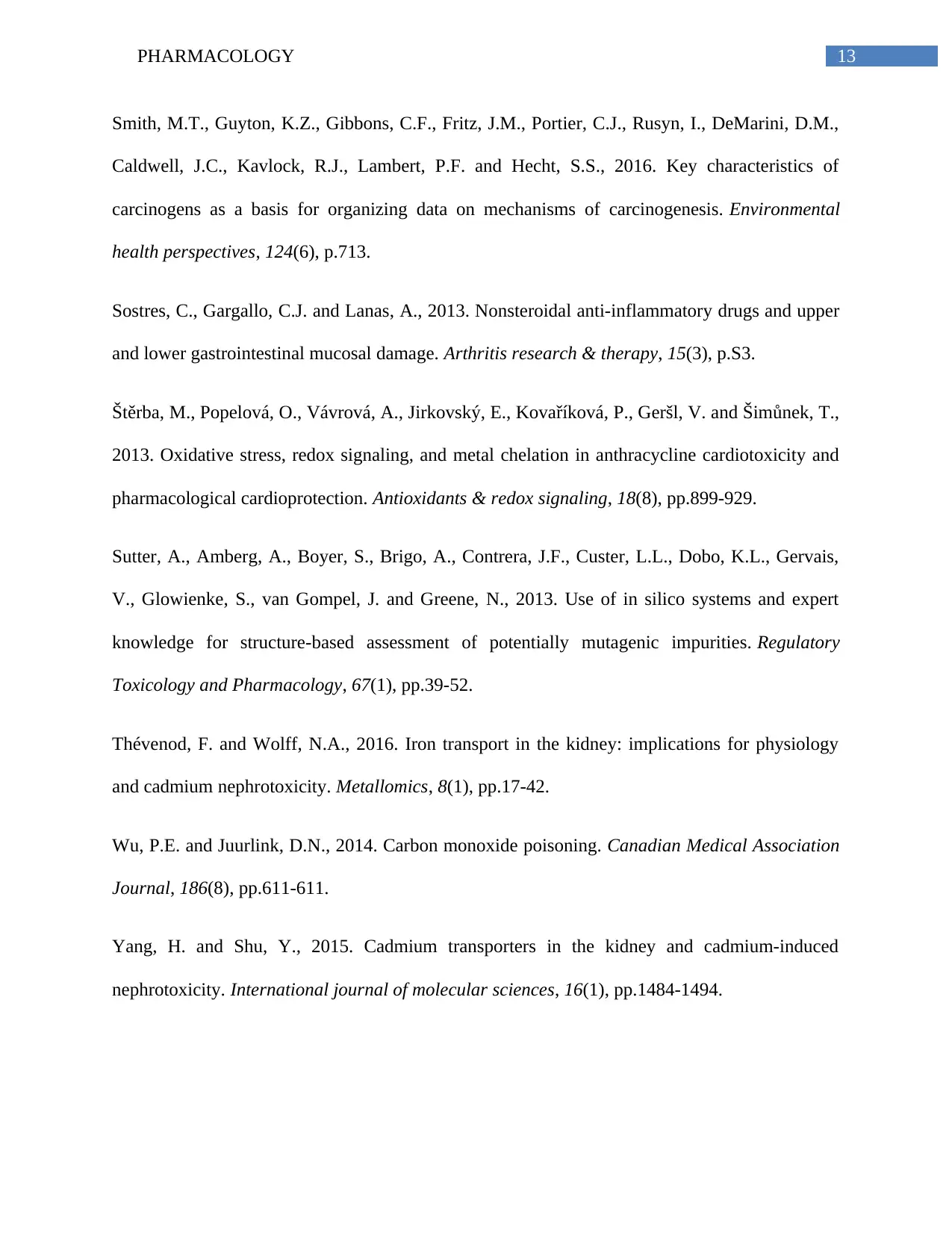
13PHARMACOLOGY
Smith, M.T., Guyton, K.Z., Gibbons, C.F., Fritz, J.M., Portier, C.J., Rusyn, I., DeMarini, D.M.,
Caldwell, J.C., Kavlock, R.J., Lambert, P.F. and Hecht, S.S., 2016. Key characteristics of
carcinogens as a basis for organizing data on mechanisms of carcinogenesis. Environmental
health perspectives, 124(6), p.713.
Sostres, C., Gargallo, C.J. and Lanas, A., 2013. Nonsteroidal anti-inflammatory drugs and upper
and lower gastrointestinal mucosal damage. Arthritis research & therapy, 15(3), p.S3.
Štěrba, M., Popelová, O., Vávrová, A., Jirkovský, E., Kovaříková, P., Geršl, V. and Šimůnek, T.,
2013. Oxidative stress, redox signaling, and metal chelation in anthracycline cardiotoxicity and
pharmacological cardioprotection. Antioxidants & redox signaling, 18(8), pp.899-929.
Sutter, A., Amberg, A., Boyer, S., Brigo, A., Contrera, J.F., Custer, L.L., Dobo, K.L., Gervais,
V., Glowienke, S., van Gompel, J. and Greene, N., 2013. Use of in silico systems and expert
knowledge for structure-based assessment of potentially mutagenic impurities. Regulatory
Toxicology and Pharmacology, 67(1), pp.39-52.
Thévenod, F. and Wolff, N.A., 2016. Iron transport in the kidney: implications for physiology
and cadmium nephrotoxicity. Metallomics, 8(1), pp.17-42.
Wu, P.E. and Juurlink, D.N., 2014. Carbon monoxide poisoning. Canadian Medical Association
Journal, 186(8), pp.611-611.
Yang, H. and Shu, Y., 2015. Cadmium transporters in the kidney and cadmium-induced
nephrotoxicity. International journal of molecular sciences, 16(1), pp.1484-1494.
Smith, M.T., Guyton, K.Z., Gibbons, C.F., Fritz, J.M., Portier, C.J., Rusyn, I., DeMarini, D.M.,
Caldwell, J.C., Kavlock, R.J., Lambert, P.F. and Hecht, S.S., 2016. Key characteristics of
carcinogens as a basis for organizing data on mechanisms of carcinogenesis. Environmental
health perspectives, 124(6), p.713.
Sostres, C., Gargallo, C.J. and Lanas, A., 2013. Nonsteroidal anti-inflammatory drugs and upper
and lower gastrointestinal mucosal damage. Arthritis research & therapy, 15(3), p.S3.
Štěrba, M., Popelová, O., Vávrová, A., Jirkovský, E., Kovaříková, P., Geršl, V. and Šimůnek, T.,
2013. Oxidative stress, redox signaling, and metal chelation in anthracycline cardiotoxicity and
pharmacological cardioprotection. Antioxidants & redox signaling, 18(8), pp.899-929.
Sutter, A., Amberg, A., Boyer, S., Brigo, A., Contrera, J.F., Custer, L.L., Dobo, K.L., Gervais,
V., Glowienke, S., van Gompel, J. and Greene, N., 2013. Use of in silico systems and expert
knowledge for structure-based assessment of potentially mutagenic impurities. Regulatory
Toxicology and Pharmacology, 67(1), pp.39-52.
Thévenod, F. and Wolff, N.A., 2016. Iron transport in the kidney: implications for physiology
and cadmium nephrotoxicity. Metallomics, 8(1), pp.17-42.
Wu, P.E. and Juurlink, D.N., 2014. Carbon monoxide poisoning. Canadian Medical Association
Journal, 186(8), pp.611-611.
Yang, H. and Shu, Y., 2015. Cadmium transporters in the kidney and cadmium-induced
nephrotoxicity. International journal of molecular sciences, 16(1), pp.1484-1494.
1 out of 14
Related Documents
![[object Object]](/_next/image/?url=%2F_next%2Fstatic%2Fmedia%2Flogo.6d15ce61.png&w=640&q=75)
Your All-in-One AI-Powered Toolkit for Academic Success.
+13062052269
info@desklib.com
Available 24*7 on WhatsApp / Email
Unlock your academic potential
© 2024 | Zucol Services PVT LTD | All rights reserved.