Phenol Compounds: Overview, Properties, and Sources
VerifiedAdded on 2023/04/06
|46
|9000
|103
AI Summary
This document provides an overview of phenol compounds, including their properties, classification, and sources. It discusses the impact of phenol compounds on the environment and human health. The document also explores the different types of phenol compounds based on carbon chain, number of phenol units, nature of distribution, and location in plants. It further examines the sources of phenolic compounds in water, including natural and anthropogenic sources.
Contribute Materials
Your contribution can guide someone’s learning journey. Share your
documents today.
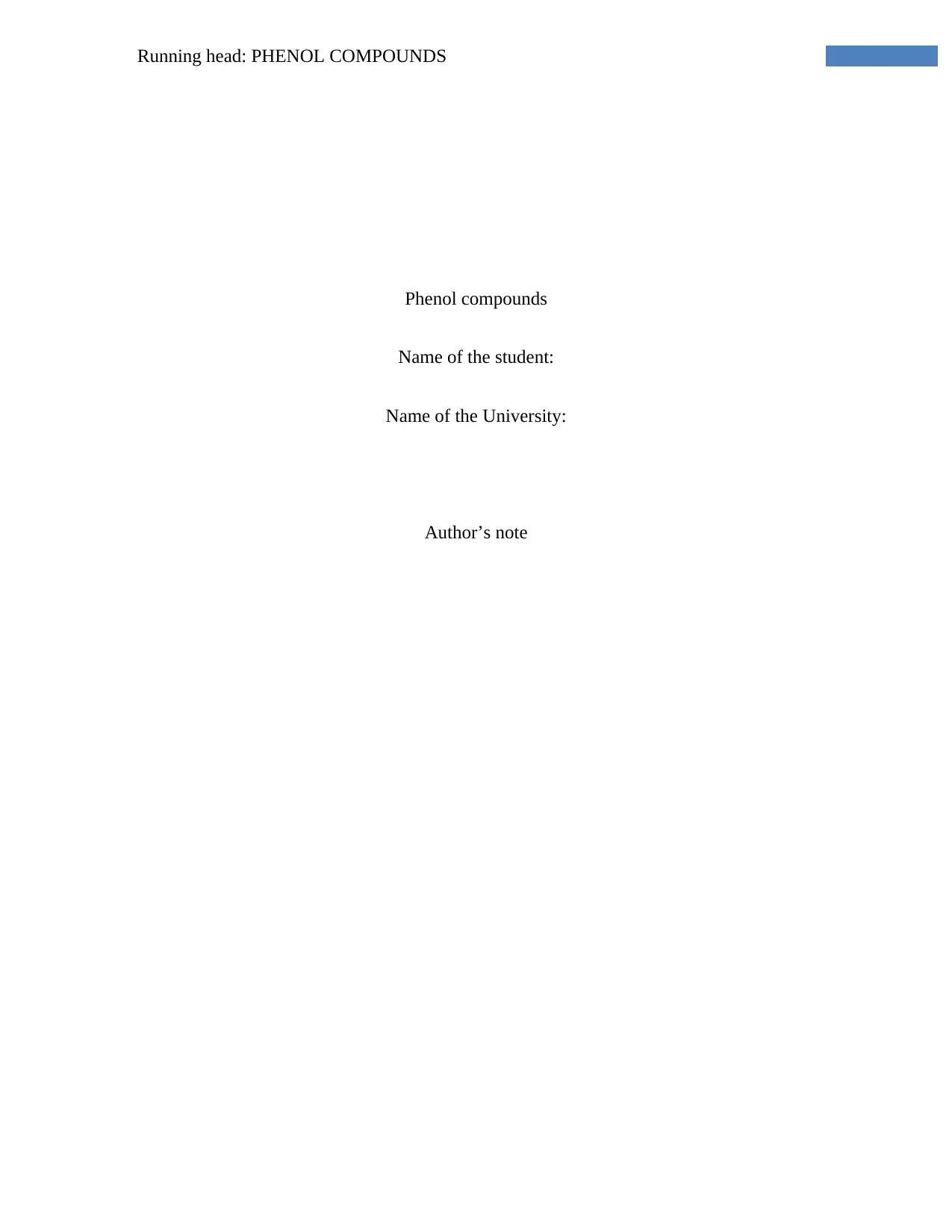
Running head: PHENOL COMPOUNDS
Phenol compounds
Name of the student:
Name of the University:
Author’s note
Phenol compounds
Name of the student:
Name of the University:
Author’s note
Secure Best Marks with AI Grader
Need help grading? Try our AI Grader for instant feedback on your assignments.
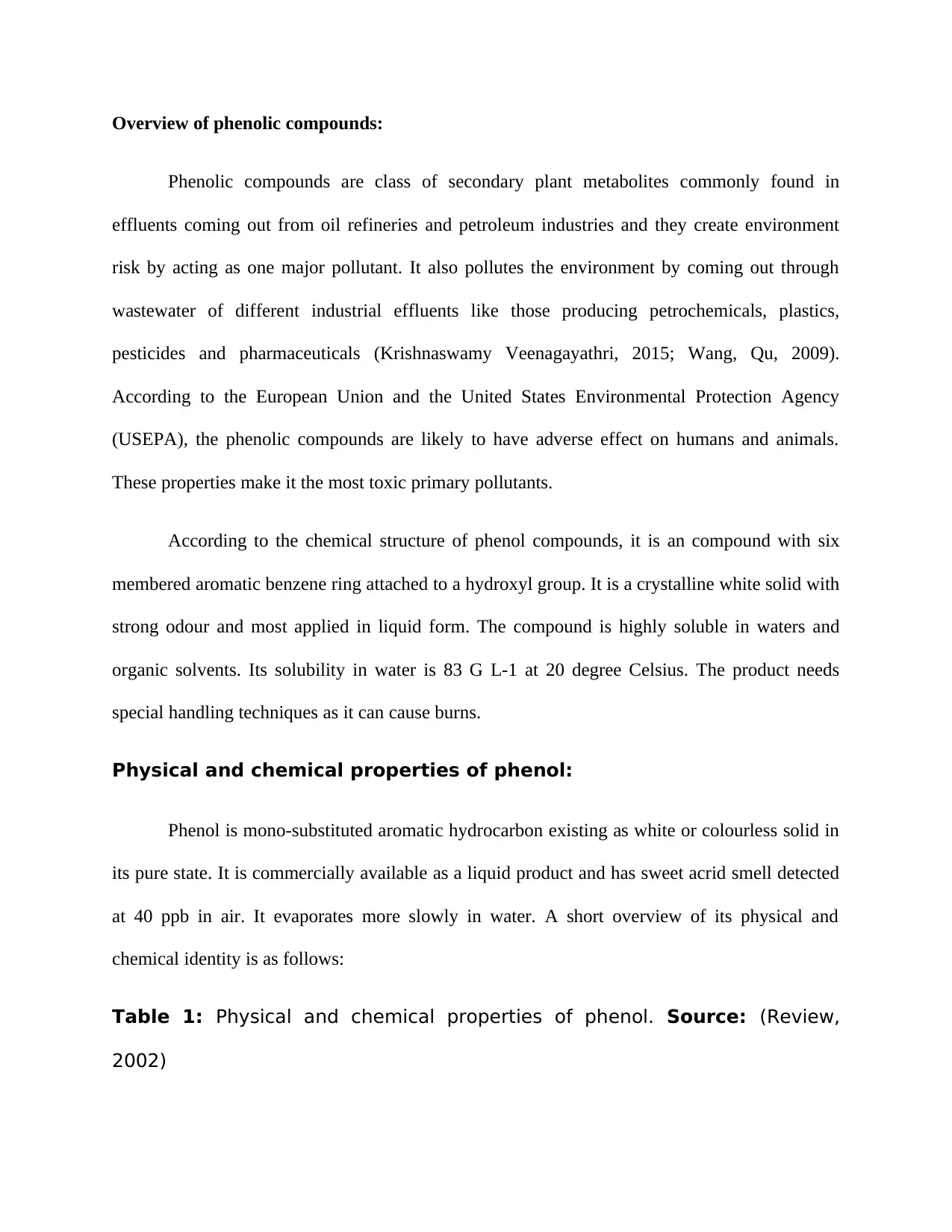
Overview of phenolic compounds:
Phenolic compounds are class of secondary plant metabolites commonly found in
effluents coming out from oil refineries and petroleum industries and they create environment
risk by acting as one major pollutant. It also pollutes the environment by coming out through
wastewater of different industrial effluents like those producing petrochemicals, plastics,
pesticides and pharmaceuticals (Krishnaswamy Veenagayathri, 2015; Wang, Qu, 2009).
According to the European Union and the United States Environmental Protection Agency
(USEPA), the phenolic compounds are likely to have adverse effect on humans and animals.
These properties make it the most toxic primary pollutants.
According to the chemical structure of phenol compounds, it is an compound with six
membered aromatic benzene ring attached to a hydroxyl group. It is a crystalline white solid with
strong odour and most applied in liquid form. The compound is highly soluble in waters and
organic solvents. Its solubility in water is 83 G L-1 at 20 degree Celsius. The product needs
special handling techniques as it can cause burns.
Physical and chemical properties of phenol:
Phenol is mono-substituted aromatic hydrocarbon existing as white or colourless solid in
its pure state. It is commercially available as a liquid product and has sweet acrid smell detected
at 40 ppb in air. It evaporates more slowly in water. A short overview of its physical and
chemical identity is as follows:
Table 1: Physical and chemical properties of phenol. Source: (Review,
2002)
Phenolic compounds are class of secondary plant metabolites commonly found in
effluents coming out from oil refineries and petroleum industries and they create environment
risk by acting as one major pollutant. It also pollutes the environment by coming out through
wastewater of different industrial effluents like those producing petrochemicals, plastics,
pesticides and pharmaceuticals (Krishnaswamy Veenagayathri, 2015; Wang, Qu, 2009).
According to the European Union and the United States Environmental Protection Agency
(USEPA), the phenolic compounds are likely to have adverse effect on humans and animals.
These properties make it the most toxic primary pollutants.
According to the chemical structure of phenol compounds, it is an compound with six
membered aromatic benzene ring attached to a hydroxyl group. It is a crystalline white solid with
strong odour and most applied in liquid form. The compound is highly soluble in waters and
organic solvents. Its solubility in water is 83 G L-1 at 20 degree Celsius. The product needs
special handling techniques as it can cause burns.
Physical and chemical properties of phenol:
Phenol is mono-substituted aromatic hydrocarbon existing as white or colourless solid in
its pure state. It is commercially available as a liquid product and has sweet acrid smell detected
at 40 ppb in air. It evaporates more slowly in water. A short overview of its physical and
chemical identity is as follows:
Table 1: Physical and chemical properties of phenol. Source: (Review,
2002)
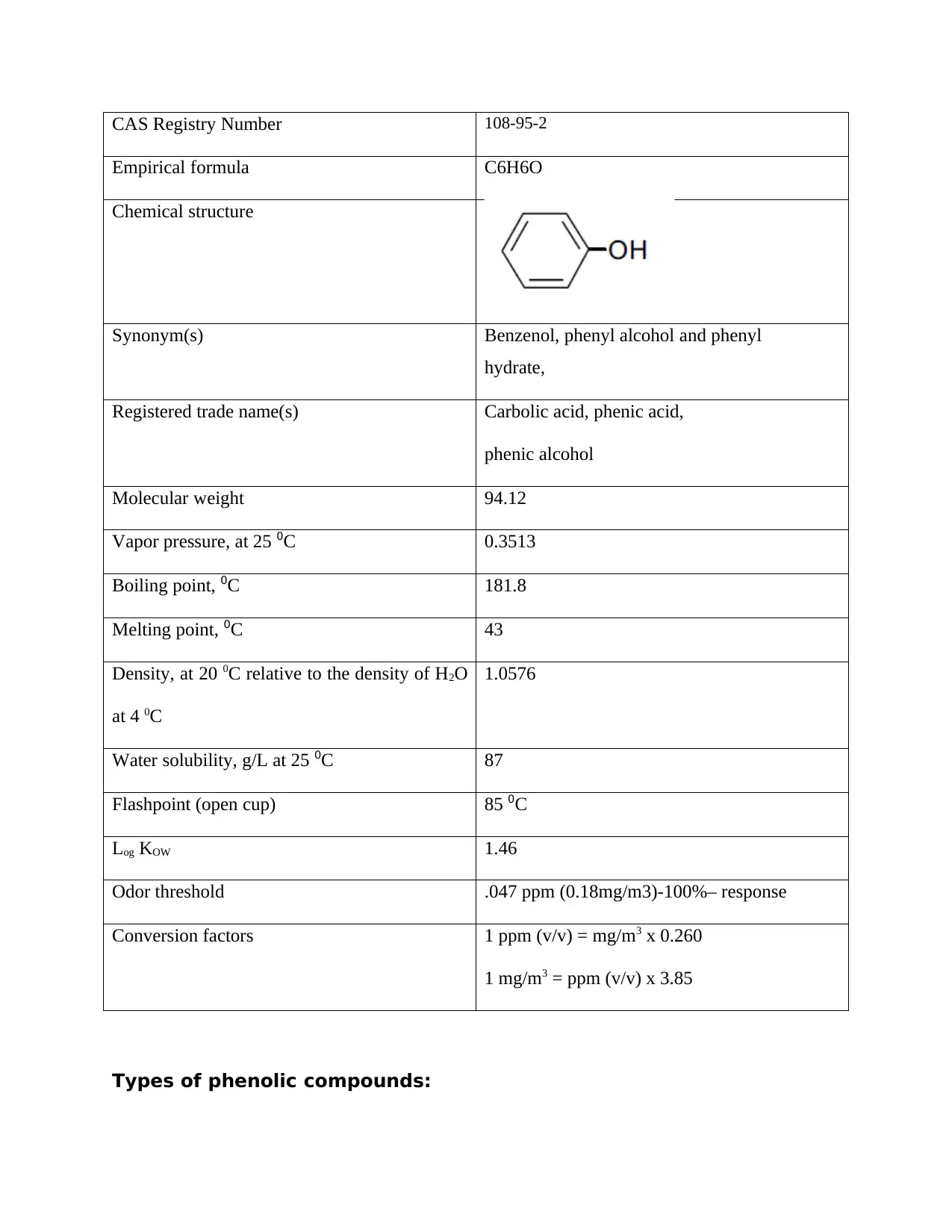
CAS Registry Number 108-95-2
Empirical formula C6H6O
Chemical structure
Synonym(s) Benzenol, phenyl alcohol and phenyl
hydrate,
Registered trade name(s) Carbolic acid, phenic acid,
phenic alcohol
Molecular weight 94.12
Vapor pressure, at 25 0C 0.3513
Boiling point, 0C 181.8
Melting point, 0C 43
Density, at 20 0C relative to the density of H2O
at 4 0C
1.0576
Water solubility, g/L at 25 0C 87
Flashpoint (open cup) 85 0C
Log KOW 1.46
Odor threshold .047 ppm (0.18mg/m3)-100%– response
Conversion factors 1 ppm (v/v) = mg/m3 x 0.260
1 mg/m3 = ppm (v/v) x 3.85
Types of phenolic compounds:
Empirical formula C6H6O
Chemical structure
Synonym(s) Benzenol, phenyl alcohol and phenyl
hydrate,
Registered trade name(s) Carbolic acid, phenic acid,
phenic alcohol
Molecular weight 94.12
Vapor pressure, at 25 0C 0.3513
Boiling point, 0C 181.8
Melting point, 0C 43
Density, at 20 0C relative to the density of H2O
at 4 0C
1.0576
Water solubility, g/L at 25 0C 87
Flashpoint (open cup) 85 0C
Log KOW 1.46
Odor threshold .047 ppm (0.18mg/m3)-100%– response
Conversion factors 1 ppm (v/v) = mg/m3 x 0.260
1 mg/m3 = ppm (v/v) x 3.85
Types of phenolic compounds:
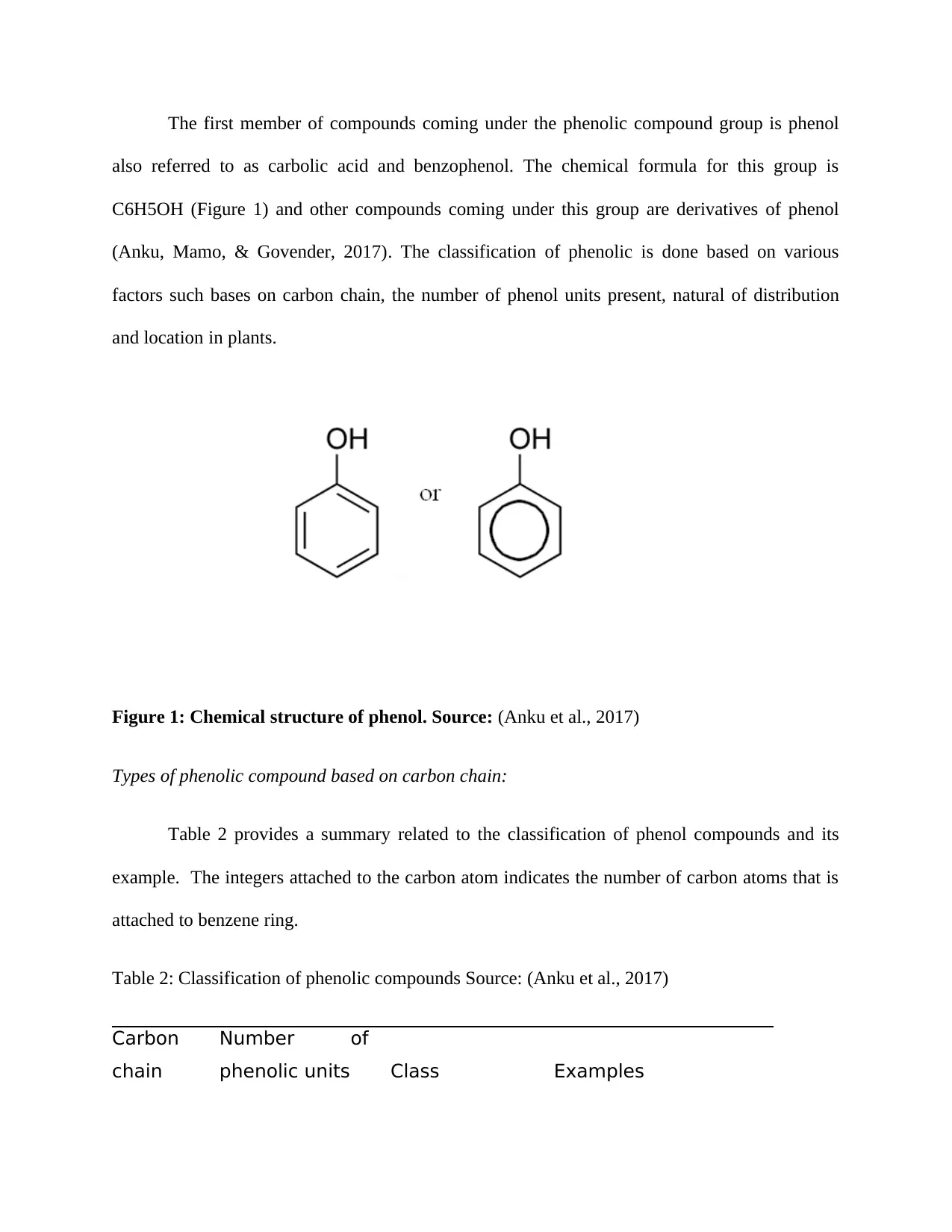
The first member of compounds coming under the phenolic compound group is phenol
also referred to as carbolic acid and benzophenol. The chemical formula for this group is
C6H5OH (Figure 1) and other compounds coming under this group are derivatives of phenol
(Anku, Mamo, & Govender, 2017). The classification of phenolic is done based on various
factors such bases on carbon chain, the number of phenol units present, natural of distribution
and location in plants.
Figure 1: Chemical structure of phenol. Source: (Anku et al., 2017)
Types of phenolic compound based on carbon chain:
Table 2 provides a summary related to the classification of phenol compounds and its
example. The integers attached to the carbon atom indicates the number of carbon atoms that is
attached to benzene ring.
Table 2: Classification of phenolic compounds Source: (Anku et al., 2017)
Carbon
chain
Number of
phenolic units Class Examples
also referred to as carbolic acid and benzophenol. The chemical formula for this group is
C6H5OH (Figure 1) and other compounds coming under this group are derivatives of phenol
(Anku, Mamo, & Govender, 2017). The classification of phenolic is done based on various
factors such bases on carbon chain, the number of phenol units present, natural of distribution
and location in plants.
Figure 1: Chemical structure of phenol. Source: (Anku et al., 2017)
Types of phenolic compound based on carbon chain:
Table 2 provides a summary related to the classification of phenol compounds and its
example. The integers attached to the carbon atom indicates the number of carbon atoms that is
attached to benzene ring.
Table 2: Classification of phenolic compounds Source: (Anku et al., 2017)
Carbon
chain
Number of
phenolic units Class Examples
Secure Best Marks with AI Grader
Need help grading? Try our AI Grader for instant feedback on your assignments.
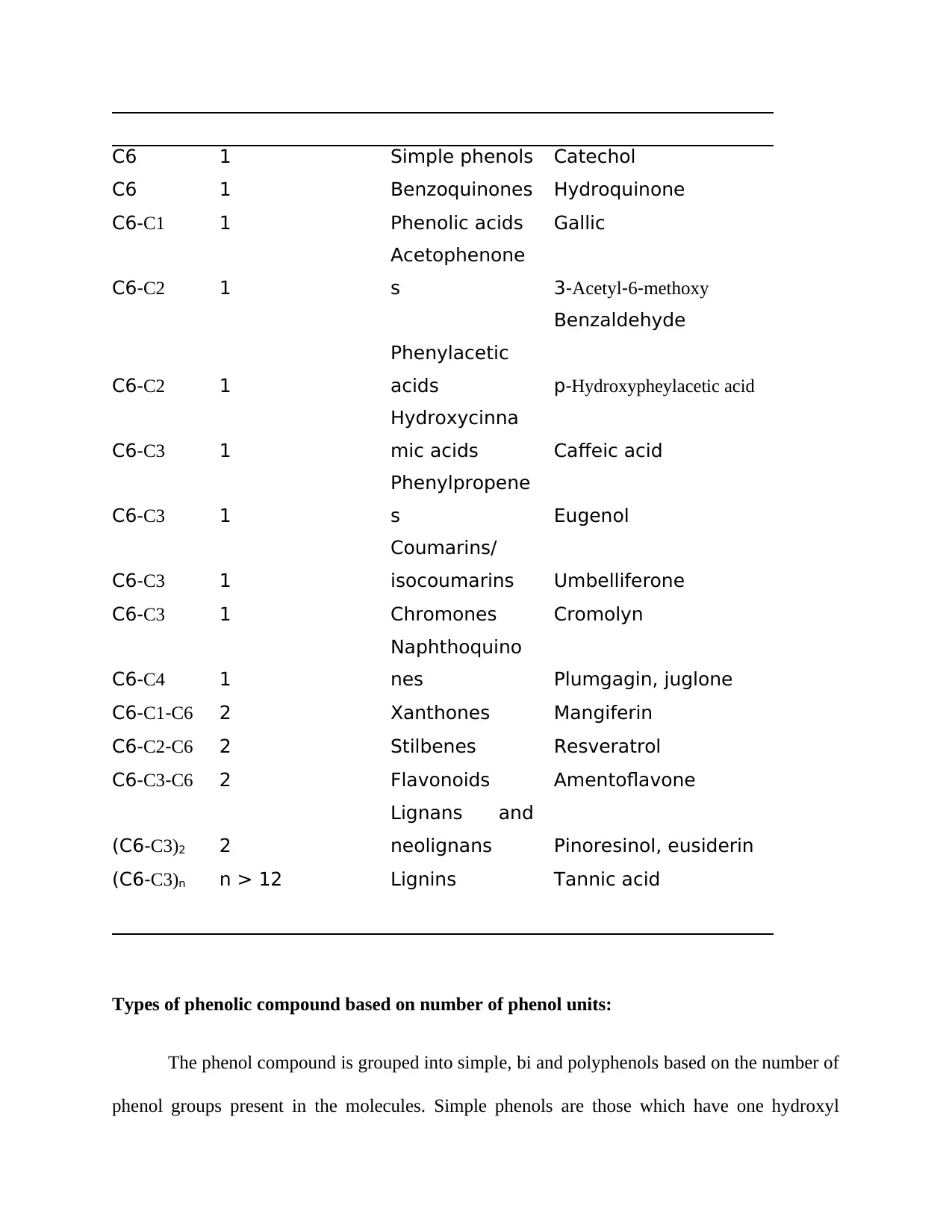
C6 1 Simple phenols Catechol
C6 1 Benzoquinones Hydroquinone
C6‐C1 1 Phenolic acids Gallic
C6‐C2 1
Acetophenone
s 3‐Acetyl‐6‐methoxy
Benzaldehyde
C6‐C2 1
Phenylacetic
acids p‐Hydroxypheylacetic acid
C6‐C3 1
Hydroxycinna
mic acids Caffeic acid
C6‐C3 1
Phenylpropene
s Eugenol
C6‐C3 1
Coumarins/
isocoumarins Umbelliferone
C6‐C3 1 Chromones Cromolyn
C6‐C4 1
Naphthoquino
nes Plumgagin, juglone
C6‐C1‐C6 2 Xanthones Mangiferin
C6‐C2‐C6 2 Stilbenes Resveratrol
C6‐C3‐C6 2 Flavonoids Amentoflavone
(C6‐C3)2 2
Lignans and
neolignans Pinoresinol, eusiderin
(C6‐C3)n n > 12 Lignins Tannic acid
Types of phenolic compound based on number of phenol units:
The phenol compound is grouped into simple, bi and polyphenols based on the number of
phenol groups present in the molecules. Simple phenols are those which have one hydroxyl
C6 1 Benzoquinones Hydroquinone
C6‐C1 1 Phenolic acids Gallic
C6‐C2 1
Acetophenone
s 3‐Acetyl‐6‐methoxy
Benzaldehyde
C6‐C2 1
Phenylacetic
acids p‐Hydroxypheylacetic acid
C6‐C3 1
Hydroxycinna
mic acids Caffeic acid
C6‐C3 1
Phenylpropene
s Eugenol
C6‐C3 1
Coumarins/
isocoumarins Umbelliferone
C6‐C3 1 Chromones Cromolyn
C6‐C4 1
Naphthoquino
nes Plumgagin, juglone
C6‐C1‐C6 2 Xanthones Mangiferin
C6‐C2‐C6 2 Stilbenes Resveratrol
C6‐C3‐C6 2 Flavonoids Amentoflavone
(C6‐C3)2 2
Lignans and
neolignans Pinoresinol, eusiderin
(C6‐C3)n n > 12 Lignins Tannic acid
Types of phenolic compound based on number of phenol units:
The phenol compound is grouped into simple, bi and polyphenols based on the number of
phenol groups present in the molecules. Simple phenols are those which have one hydroxyl
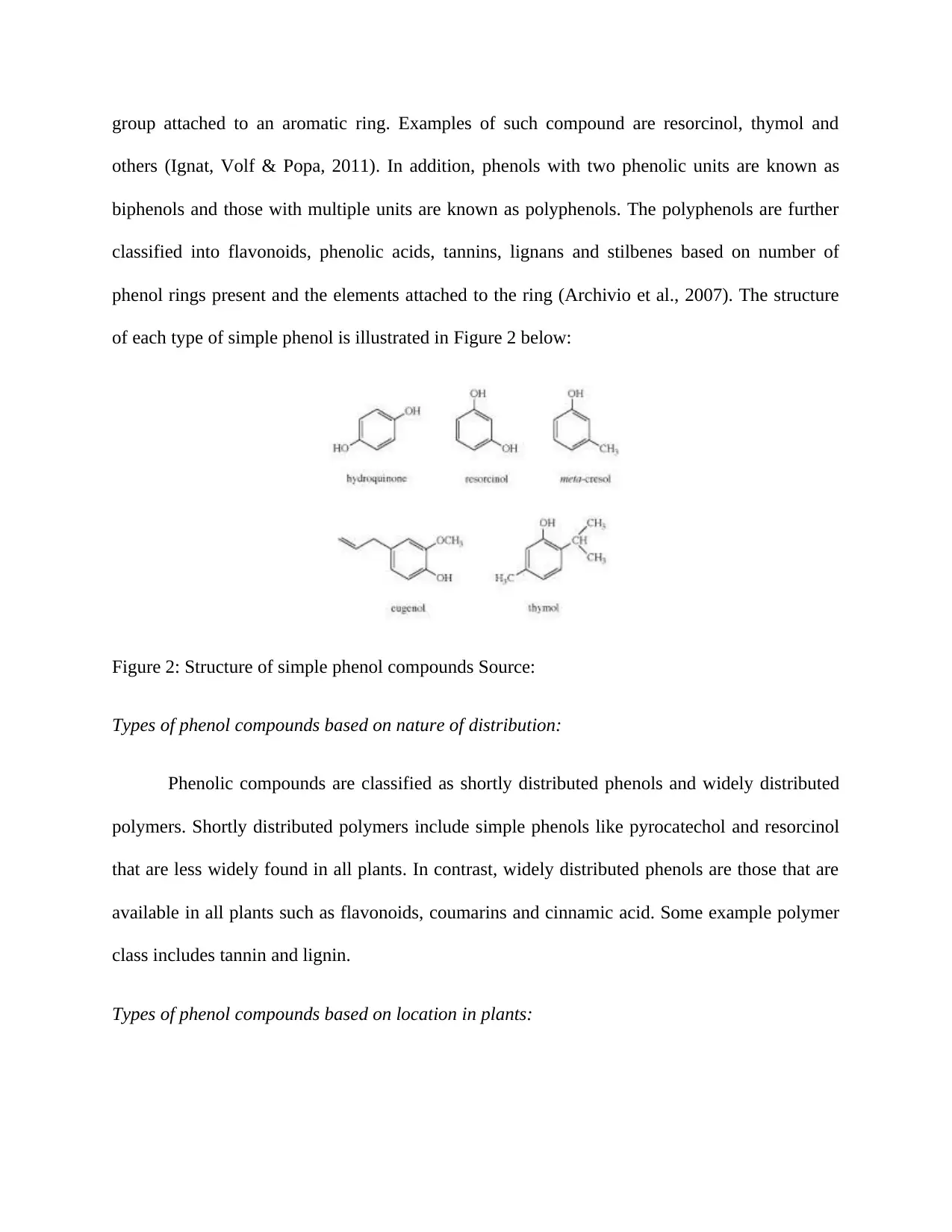
group attached to an aromatic ring. Examples of such compound are resorcinol, thymol and
others (Ignat, Volf & Popa, 2011). In addition, phenols with two phenolic units are known as
biphenols and those with multiple units are known as polyphenols. The polyphenols are further
classified into flavonoids, phenolic acids, tannins, lignans and stilbenes based on number of
phenol rings present and the elements attached to the ring (Archivio et al., 2007). The structure
of each type of simple phenol is illustrated in Figure 2 below:
Figure 2: Structure of simple phenol compounds Source:
Types of phenol compounds based on nature of distribution:
Phenolic compounds are classified as shortly distributed phenols and widely distributed
polymers. Shortly distributed polymers include simple phenols like pyrocatechol and resorcinol
that are less widely found in all plants. In contrast, widely distributed phenols are those that are
available in all plants such as flavonoids, coumarins and cinnamic acid. Some example polymer
class includes tannin and lignin.
Types of phenol compounds based on location in plants:
others (Ignat, Volf & Popa, 2011). In addition, phenols with two phenolic units are known as
biphenols and those with multiple units are known as polyphenols. The polyphenols are further
classified into flavonoids, phenolic acids, tannins, lignans and stilbenes based on number of
phenol rings present and the elements attached to the ring (Archivio et al., 2007). The structure
of each type of simple phenol is illustrated in Figure 2 below:
Figure 2: Structure of simple phenol compounds Source:
Types of phenol compounds based on nature of distribution:
Phenolic compounds are classified as shortly distributed phenols and widely distributed
polymers. Shortly distributed polymers include simple phenols like pyrocatechol and resorcinol
that are less widely found in all plants. In contrast, widely distributed phenols are those that are
available in all plants such as flavonoids, coumarins and cinnamic acid. Some example polymer
class includes tannin and lignin.
Types of phenol compounds based on location in plants:
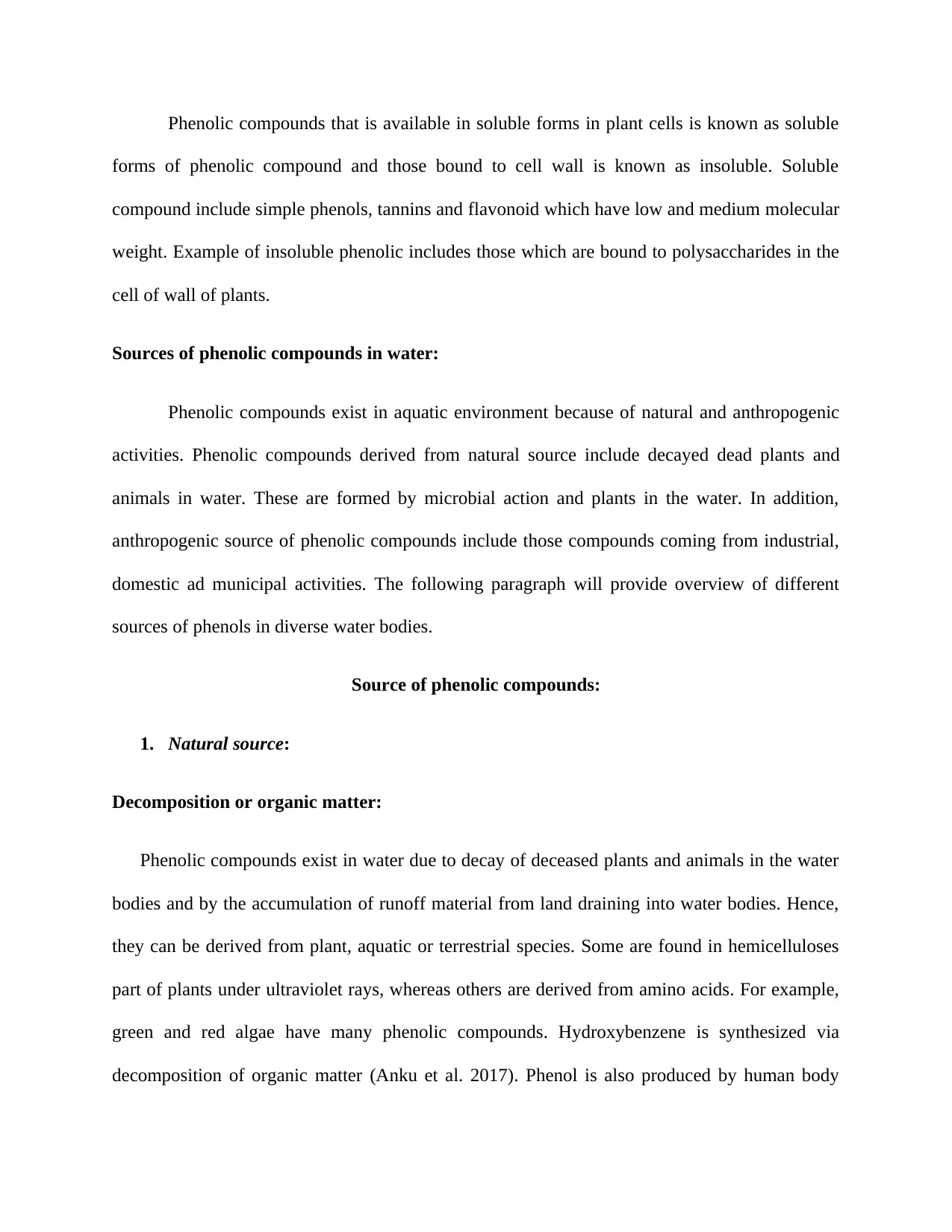
Phenolic compounds that is available in soluble forms in plant cells is known as soluble
forms of phenolic compound and those bound to cell wall is known as insoluble. Soluble
compound include simple phenols, tannins and flavonoid which have low and medium molecular
weight. Example of insoluble phenolic includes those which are bound to polysaccharides in the
cell of wall of plants.
Sources of phenolic compounds in water:
Phenolic compounds exist in aquatic environment because of natural and anthropogenic
activities. Phenolic compounds derived from natural source include decayed dead plants and
animals in water. These are formed by microbial action and plants in the water. In addition,
anthropogenic source of phenolic compounds include those compounds coming from industrial,
domestic ad municipal activities. The following paragraph will provide overview of different
sources of phenols in diverse water bodies.
Source of phenolic compounds:
1. Natural source:
Decomposition or organic matter:
Phenolic compounds exist in water due to decay of deceased plants and animals in the water
bodies and by the accumulation of runoff material from land draining into water bodies. Hence,
they can be derived from plant, aquatic or terrestrial species. Some are found in hemicelluloses
part of plants under ultraviolet rays, whereas others are derived from amino acids. For example,
green and red algae have many phenolic compounds. Hydroxybenzene is synthesized via
decomposition of organic matter (Anku et al. 2017). Phenol is also produced by human body
forms of phenolic compound and those bound to cell wall is known as insoluble. Soluble
compound include simple phenols, tannins and flavonoid which have low and medium molecular
weight. Example of insoluble phenolic includes those which are bound to polysaccharides in the
cell of wall of plants.
Sources of phenolic compounds in water:
Phenolic compounds exist in aquatic environment because of natural and anthropogenic
activities. Phenolic compounds derived from natural source include decayed dead plants and
animals in water. These are formed by microbial action and plants in the water. In addition,
anthropogenic source of phenolic compounds include those compounds coming from industrial,
domestic ad municipal activities. The following paragraph will provide overview of different
sources of phenols in diverse water bodies.
Source of phenolic compounds:
1. Natural source:
Decomposition or organic matter:
Phenolic compounds exist in water due to decay of deceased plants and animals in the water
bodies and by the accumulation of runoff material from land draining into water bodies. Hence,
they can be derived from plant, aquatic or terrestrial species. Some are found in hemicelluloses
part of plants under ultraviolet rays, whereas others are derived from amino acids. For example,
green and red algae have many phenolic compounds. Hydroxybenzene is synthesized via
decomposition of organic matter (Anku et al. 2017). Phenol is also produced by human body
Paraphrase This Document
Need a fresh take? Get an instant paraphrase of this document with our AI Paraphraser
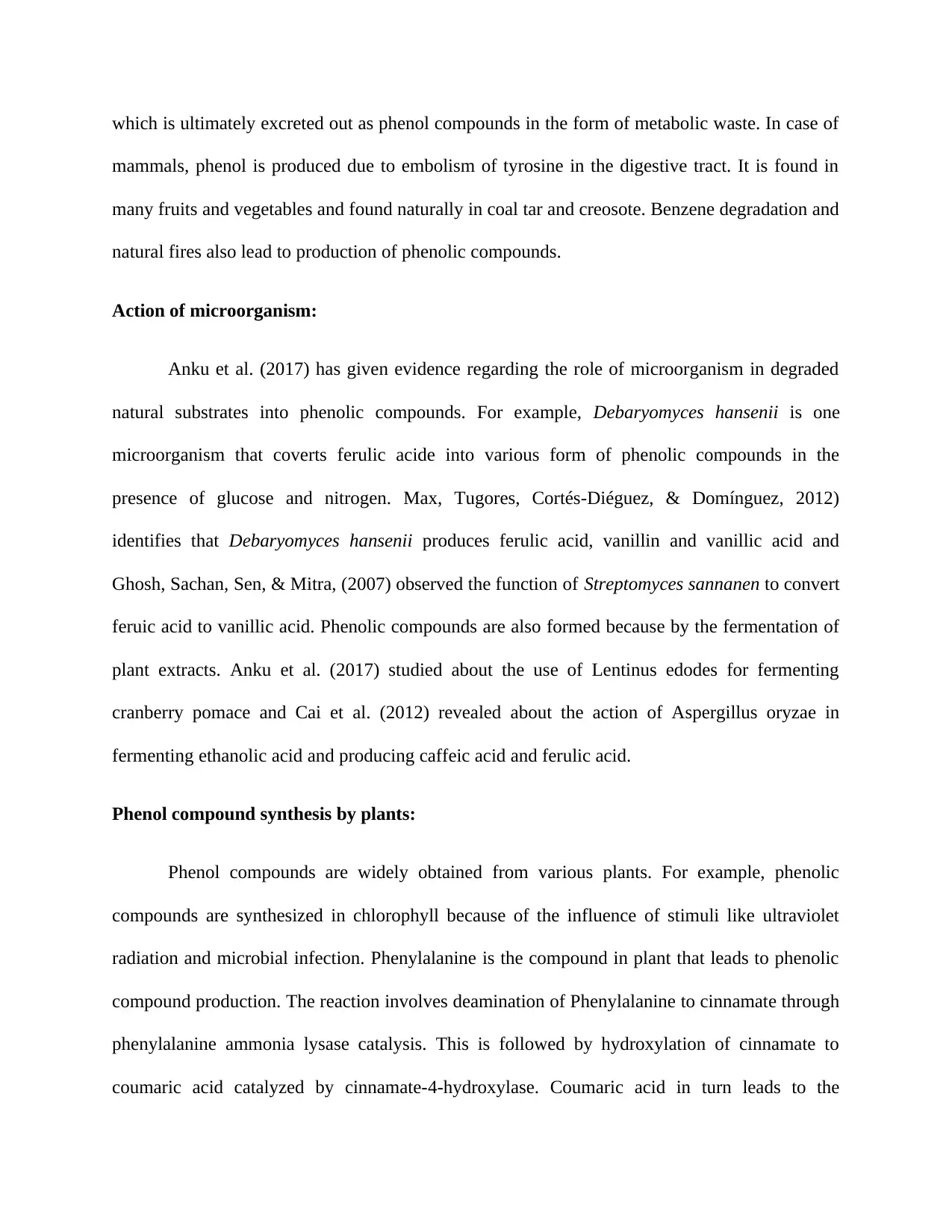
which is ultimately excreted out as phenol compounds in the form of metabolic waste. In case of
mammals, phenol is produced due to embolism of tyrosine in the digestive tract. It is found in
many fruits and vegetables and found naturally in coal tar and creosote. Benzene degradation and
natural fires also lead to production of phenolic compounds.
Action of microorganism:
Anku et al. (2017) has given evidence regarding the role of microorganism in degraded
natural substrates into phenolic compounds. For example, Debaryomyces hansenii is one
microorganism that coverts ferulic acide into various form of phenolic compounds in the
presence of glucose and nitrogen. Max, Tugores, Cortés-Diéguez, & Domínguez, 2012)
identifies that Debaryomyces hansenii produces ferulic acid, vanillin and vanillic acid and
Ghosh, Sachan, Sen, & Mitra, (2007) observed the function of Streptomyces sannanen to convert
feruic acid to vanillic acid. Phenolic compounds are also formed because by the fermentation of
plant extracts. Anku et al. (2017) studied about the use of Lentinus edodes for fermenting
cranberry pomace and Cai et al. (2012) revealed about the action of Aspergillus oryzae in
fermenting ethanolic acid and producing caffeic acid and ferulic acid.
Phenol compound synthesis by plants:
Phenol compounds are widely obtained from various plants. For example, phenolic
compounds are synthesized in chlorophyll because of the influence of stimuli like ultraviolet
radiation and microbial infection. Phenylalanine is the compound in plant that leads to phenolic
compound production. The reaction involves deamination of Phenylalanine to cinnamate through
phenylalanine ammonia lysase catalysis. This is followed by hydroxylation of cinnamate to
coumaric acid catalyzed by cinnamate-4-hydroxylase. Coumaric acid in turn leads to the
mammals, phenol is produced due to embolism of tyrosine in the digestive tract. It is found in
many fruits and vegetables and found naturally in coal tar and creosote. Benzene degradation and
natural fires also lead to production of phenolic compounds.
Action of microorganism:
Anku et al. (2017) has given evidence regarding the role of microorganism in degraded
natural substrates into phenolic compounds. For example, Debaryomyces hansenii is one
microorganism that coverts ferulic acide into various form of phenolic compounds in the
presence of glucose and nitrogen. Max, Tugores, Cortés-Diéguez, & Domínguez, 2012)
identifies that Debaryomyces hansenii produces ferulic acid, vanillin and vanillic acid and
Ghosh, Sachan, Sen, & Mitra, (2007) observed the function of Streptomyces sannanen to convert
feruic acid to vanillic acid. Phenolic compounds are also formed because by the fermentation of
plant extracts. Anku et al. (2017) studied about the use of Lentinus edodes for fermenting
cranberry pomace and Cai et al. (2012) revealed about the action of Aspergillus oryzae in
fermenting ethanolic acid and producing caffeic acid and ferulic acid.
Phenol compound synthesis by plants:
Phenol compounds are widely obtained from various plants. For example, phenolic
compounds are synthesized in chlorophyll because of the influence of stimuli like ultraviolet
radiation and microbial infection. Phenylalanine is the compound in plant that leads to phenolic
compound production. The reaction involves deamination of Phenylalanine to cinnamate through
phenylalanine ammonia lysase catalysis. This is followed by hydroxylation of cinnamate to
coumaric acid catalyzed by cinnamate-4-hydroxylase. Coumaric acid in turn leads to the
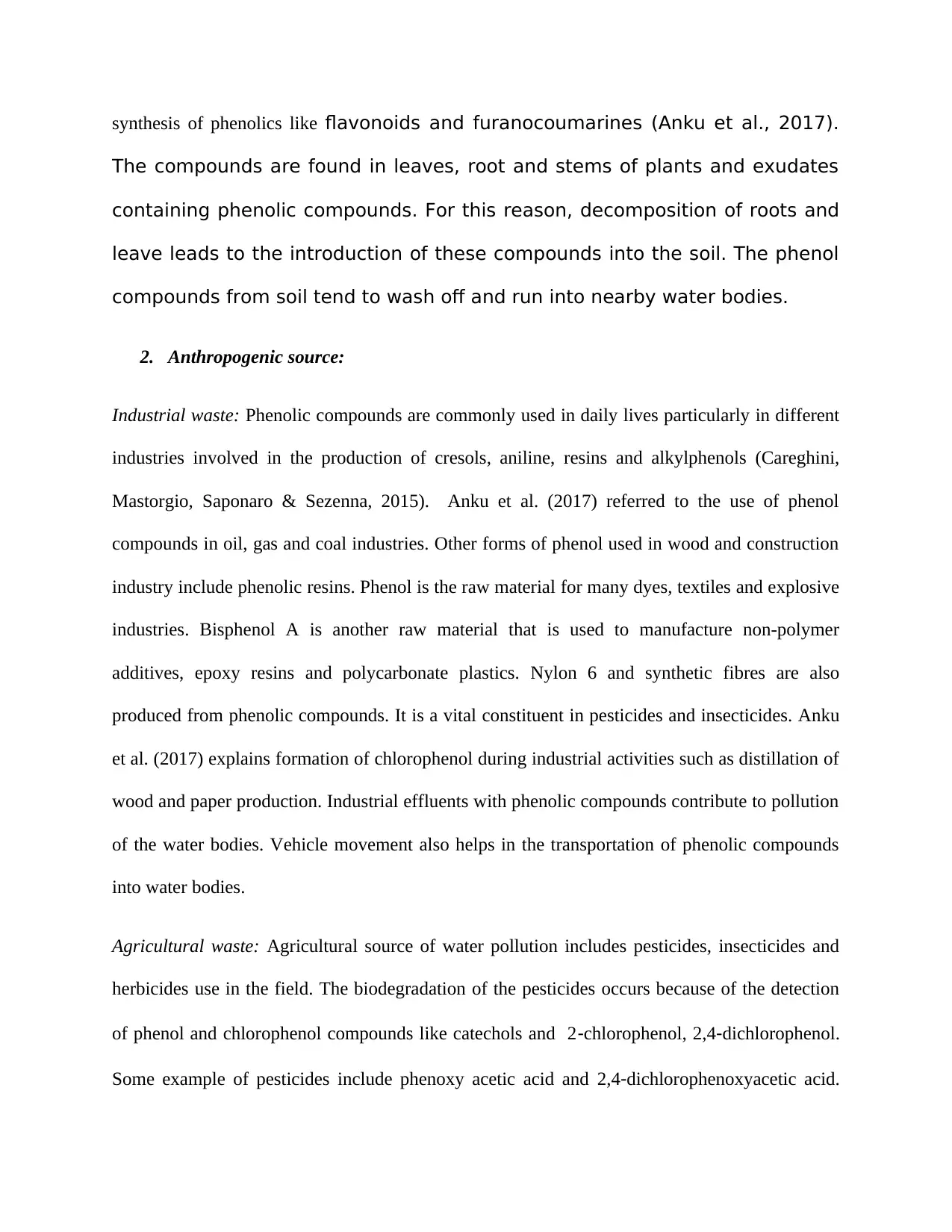
synthesis of phenolics like flavonoids and furanocoumarines (Anku et al., 2017).
The compounds are found in leaves, root and stems of plants and exudates
containing phenolic compounds. For this reason, decomposition of roots and
leave leads to the introduction of these compounds into the soil. The phenol
compounds from soil tend to wash off and run into nearby water bodies.
2. Anthropogenic source:
Industrial waste: Phenolic compounds are commonly used in daily lives particularly in different
industries involved in the production of cresols, aniline, resins and alkylphenols (Careghini,
Mastorgio, Saponaro & Sezenna, 2015). Anku et al. (2017) referred to the use of phenol
compounds in oil, gas and coal industries. Other forms of phenol used in wood and construction
industry include phenolic resins. Phenol is the raw material for many dyes, textiles and explosive
industries. Bisphenol A is another raw material that is used to manufacture non-polymer
additives, epoxy resins and polycarbonate plastics. Nylon 6 and synthetic fibres are also
produced from phenolic compounds. It is a vital constituent in pesticides and insecticides. Anku
et al. (2017) explains formation of chlorophenol during industrial activities such as distillation of
wood and paper production. Industrial effluents with phenolic compounds contribute to pollution
of the water bodies. Vehicle movement also helps in the transportation of phenolic compounds
into water bodies.
Agricultural waste: Agricultural source of water pollution includes pesticides, insecticides and
herbicides use in the field. The biodegradation of the pesticides occurs because of the detection
of phenol and chlorophenol compounds like catechols and 2‐chlorophenol, 2,4‐dichlorophenol.
Some example of pesticides include phenoxy acetic acid and 2,4‐dichlorophenoxyacetic acid.
The compounds are found in leaves, root and stems of plants and exudates
containing phenolic compounds. For this reason, decomposition of roots and
leave leads to the introduction of these compounds into the soil. The phenol
compounds from soil tend to wash off and run into nearby water bodies.
2. Anthropogenic source:
Industrial waste: Phenolic compounds are commonly used in daily lives particularly in different
industries involved in the production of cresols, aniline, resins and alkylphenols (Careghini,
Mastorgio, Saponaro & Sezenna, 2015). Anku et al. (2017) referred to the use of phenol
compounds in oil, gas and coal industries. Other forms of phenol used in wood and construction
industry include phenolic resins. Phenol is the raw material for many dyes, textiles and explosive
industries. Bisphenol A is another raw material that is used to manufacture non-polymer
additives, epoxy resins and polycarbonate plastics. Nylon 6 and synthetic fibres are also
produced from phenolic compounds. It is a vital constituent in pesticides and insecticides. Anku
et al. (2017) explains formation of chlorophenol during industrial activities such as distillation of
wood and paper production. Industrial effluents with phenolic compounds contribute to pollution
of the water bodies. Vehicle movement also helps in the transportation of phenolic compounds
into water bodies.
Agricultural waste: Agricultural source of water pollution includes pesticides, insecticides and
herbicides use in the field. The biodegradation of the pesticides occurs because of the detection
of phenol and chlorophenol compounds like catechols and 2‐chlorophenol, 2,4‐dichlorophenol.
Some example of pesticides include phenoxy acetic acid and 2,4‐dichlorophenoxyacetic acid.
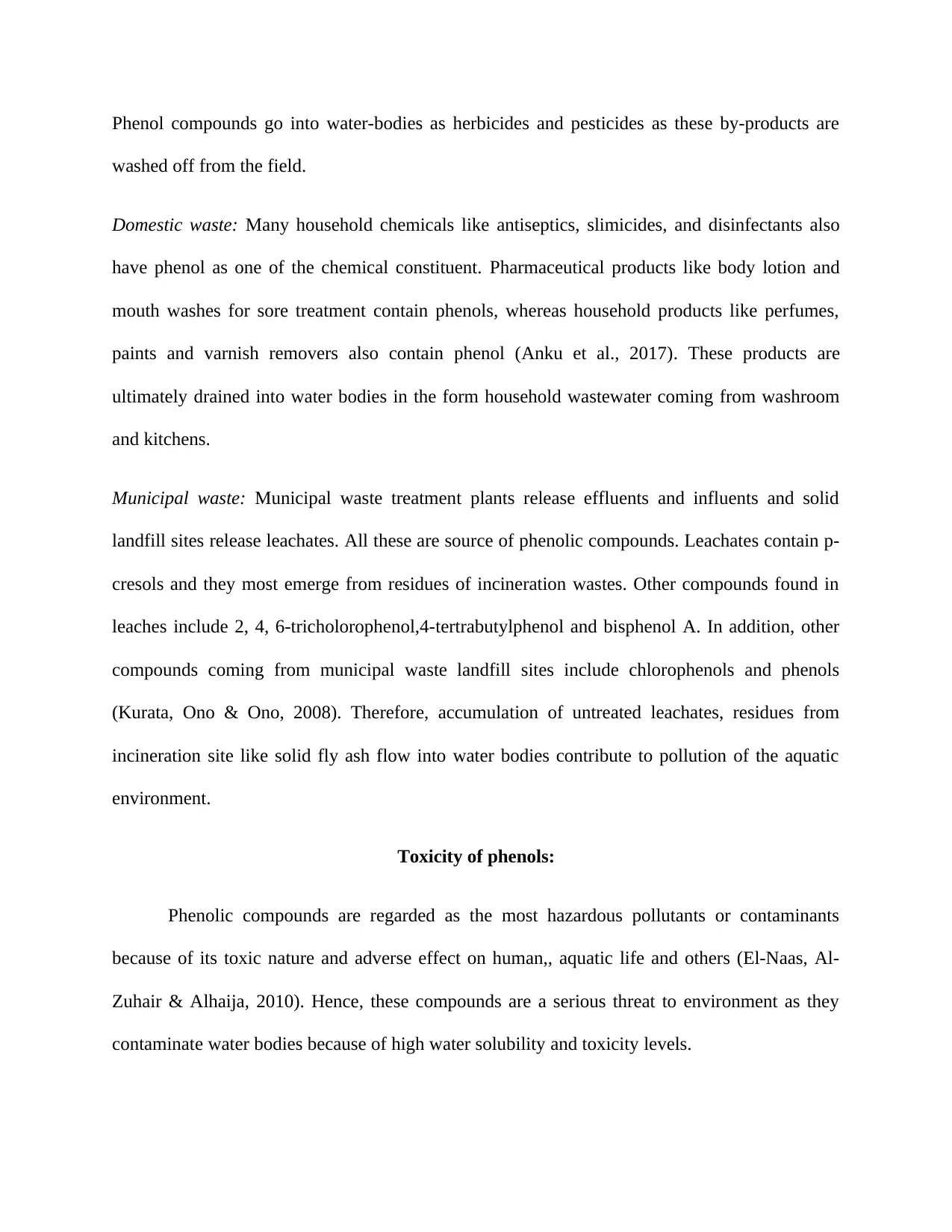
Phenol compounds go into water-bodies as herbicides and pesticides as these by-products are
washed off from the field.
Domestic waste: Many household chemicals like antiseptics, slimicides, and disinfectants also
have phenol as one of the chemical constituent. Pharmaceutical products like body lotion and
mouth washes for sore treatment contain phenols, whereas household products like perfumes,
paints and varnish removers also contain phenol (Anku et al., 2017). These products are
ultimately drained into water bodies in the form household wastewater coming from washroom
and kitchens.
Municipal waste: Municipal waste treatment plants release effluents and influents and solid
landfill sites release leachates. All these are source of phenolic compounds. Leachates contain p-
cresols and they most emerge from residues of incineration wastes. Other compounds found in
leaches include 2, 4, 6-tricholorophenol,4-tertrabutylphenol and bisphenol A. In addition, other
compounds coming from municipal waste landfill sites include chlorophenols and phenols
(Kurata, Ono & Ono, 2008). Therefore, accumulation of untreated leachates, residues from
incineration site like solid fly ash flow into water bodies contribute to pollution of the aquatic
environment.
Toxicity of phenols:
Phenolic compounds are regarded as the most hazardous pollutants or contaminants
because of its toxic nature and adverse effect on human,, aquatic life and others (El-Naas, Al-
Zuhair & Alhaija, 2010). Hence, these compounds are a serious threat to environment as they
contaminate water bodies because of high water solubility and toxicity levels.
washed off from the field.
Domestic waste: Many household chemicals like antiseptics, slimicides, and disinfectants also
have phenol as one of the chemical constituent. Pharmaceutical products like body lotion and
mouth washes for sore treatment contain phenols, whereas household products like perfumes,
paints and varnish removers also contain phenol (Anku et al., 2017). These products are
ultimately drained into water bodies in the form household wastewater coming from washroom
and kitchens.
Municipal waste: Municipal waste treatment plants release effluents and influents and solid
landfill sites release leachates. All these are source of phenolic compounds. Leachates contain p-
cresols and they most emerge from residues of incineration wastes. Other compounds found in
leaches include 2, 4, 6-tricholorophenol,4-tertrabutylphenol and bisphenol A. In addition, other
compounds coming from municipal waste landfill sites include chlorophenols and phenols
(Kurata, Ono & Ono, 2008). Therefore, accumulation of untreated leachates, residues from
incineration site like solid fly ash flow into water bodies contribute to pollution of the aquatic
environment.
Toxicity of phenols:
Phenolic compounds are regarded as the most hazardous pollutants or contaminants
because of its toxic nature and adverse effect on human,, aquatic life and others (El-Naas, Al-
Zuhair & Alhaija, 2010). Hence, these compounds are a serious threat to environment as they
contaminate water bodies because of high water solubility and toxicity levels.
Secure Best Marks with AI Grader
Need help grading? Try our AI Grader for instant feedback on your assignments.
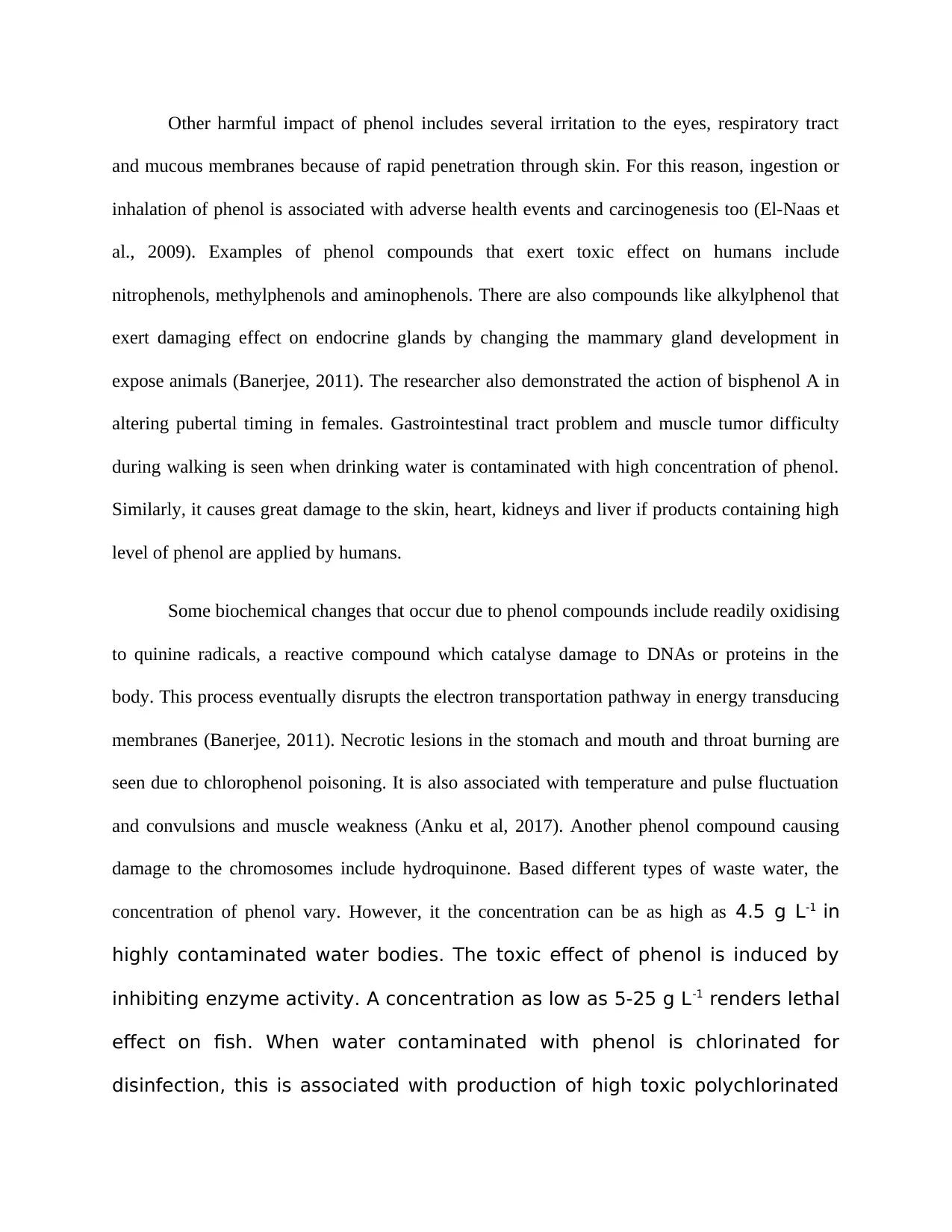
Other harmful impact of phenol includes several irritation to the eyes, respiratory tract
and mucous membranes because of rapid penetration through skin. For this reason, ingestion or
inhalation of phenol is associated with adverse health events and carcinogenesis too (El-Naas et
al., 2009). Examples of phenol compounds that exert toxic effect on humans include
nitrophenols, methylphenols and aminophenols. There are also compounds like alkylphenol that
exert damaging effect on endocrine glands by changing the mammary gland development in
expose animals (Banerjee, 2011). The researcher also demonstrated the action of bisphenol A in
altering pubertal timing in females. Gastrointestinal tract problem and muscle tumor difficulty
during walking is seen when drinking water is contaminated with high concentration of phenol.
Similarly, it causes great damage to the skin, heart, kidneys and liver if products containing high
level of phenol are applied by humans.
Some biochemical changes that occur due to phenol compounds include readily oxidising
to quinine radicals, a reactive compound which catalyse damage to DNAs or proteins in the
body. This process eventually disrupts the electron transportation pathway in energy transducing
membranes (Banerjee, 2011). Necrotic lesions in the stomach and mouth and throat burning are
seen due to chlorophenol poisoning. It is also associated with temperature and pulse fluctuation
and convulsions and muscle weakness (Anku et al, 2017). Another phenol compound causing
damage to the chromosomes include hydroquinone. Based different types of waste water, the
concentration of phenol vary. However, it the concentration can be as high as 4.5 g L-1 in
highly contaminated water bodies. The toxic effect of phenol is induced by
inhibiting enzyme activity. A concentration as low as 5-25 g L-1 renders lethal
effect on fish. When water contaminated with phenol is chlorinated for
disinfection, this is associated with production of high toxic polychlorinated
and mucous membranes because of rapid penetration through skin. For this reason, ingestion or
inhalation of phenol is associated with adverse health events and carcinogenesis too (El-Naas et
al., 2009). Examples of phenol compounds that exert toxic effect on humans include
nitrophenols, methylphenols and aminophenols. There are also compounds like alkylphenol that
exert damaging effect on endocrine glands by changing the mammary gland development in
expose animals (Banerjee, 2011). The researcher also demonstrated the action of bisphenol A in
altering pubertal timing in females. Gastrointestinal tract problem and muscle tumor difficulty
during walking is seen when drinking water is contaminated with high concentration of phenol.
Similarly, it causes great damage to the skin, heart, kidneys and liver if products containing high
level of phenol are applied by humans.
Some biochemical changes that occur due to phenol compounds include readily oxidising
to quinine radicals, a reactive compound which catalyse damage to DNAs or proteins in the
body. This process eventually disrupts the electron transportation pathway in energy transducing
membranes (Banerjee, 2011). Necrotic lesions in the stomach and mouth and throat burning are
seen due to chlorophenol poisoning. It is also associated with temperature and pulse fluctuation
and convulsions and muscle weakness (Anku et al, 2017). Another phenol compound causing
damage to the chromosomes include hydroquinone. Based different types of waste water, the
concentration of phenol vary. However, it the concentration can be as high as 4.5 g L-1 in
highly contaminated water bodies. The toxic effect of phenol is induced by
inhibiting enzyme activity. A concentration as low as 5-25 g L-1 renders lethal
effect on fish. When water contaminated with phenol is chlorinated for
disinfection, this is associated with production of high toxic polychlorinated
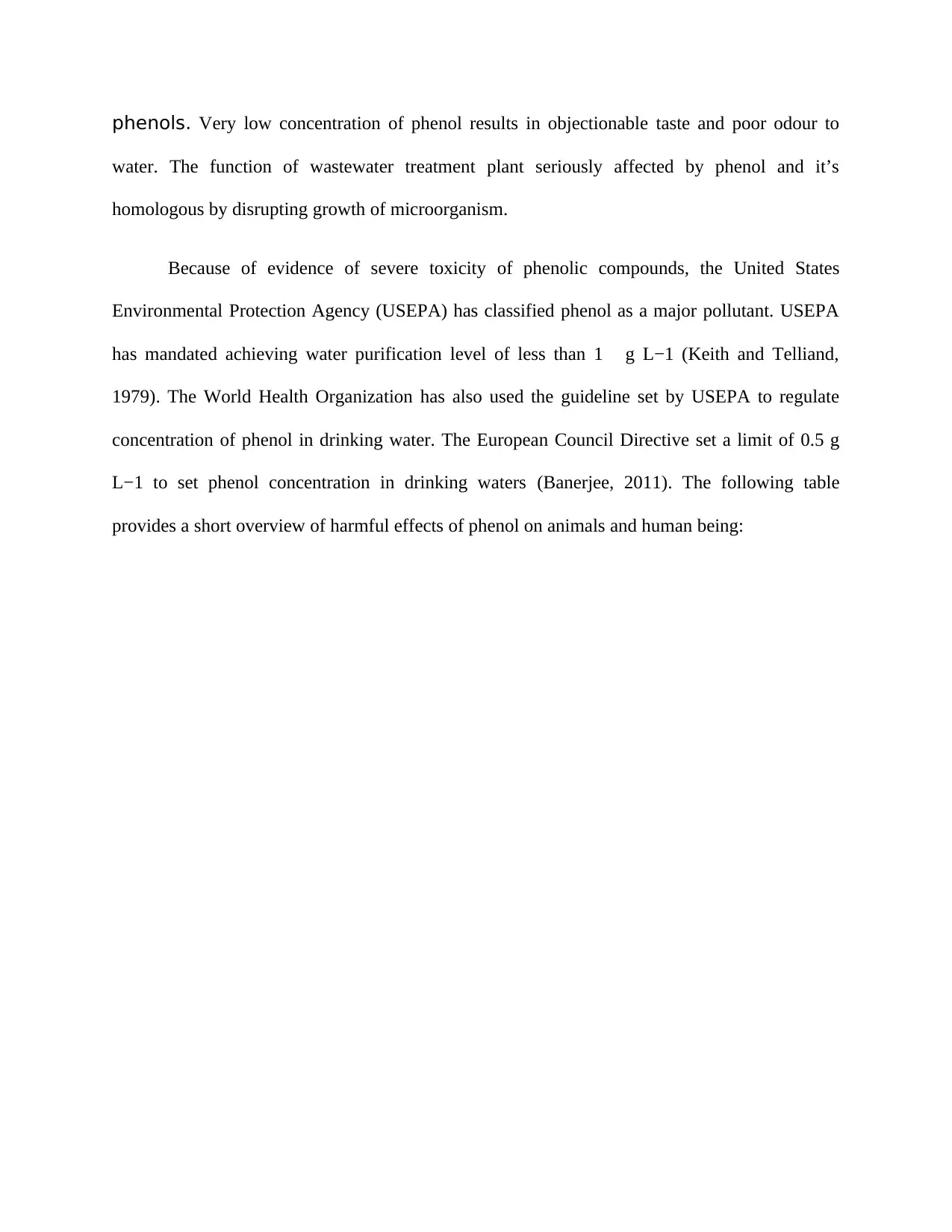
phenols. Very low concentration of phenol results in objectionable taste and poor odour to
water. The function of wastewater treatment plant seriously affected by phenol and it’s
homologous by disrupting growth of microorganism.
Because of evidence of severe toxicity of phenolic compounds, the United States
Environmental Protection Agency (USEPA) has classified phenol as a major pollutant. USEPA
has mandated achieving water purification level of less than 1 g L−1 (Keith and Telliand,
1979). The World Health Organization has also used the guideline set by USEPA to regulate
concentration of phenol in drinking water. The European Council Directive set a limit of 0.5 g
L−1 to set phenol concentration in drinking waters (Banerjee, 2011). The following table
provides a short overview of harmful effects of phenol on animals and human being:
water. The function of wastewater treatment plant seriously affected by phenol and it’s
homologous by disrupting growth of microorganism.
Because of evidence of severe toxicity of phenolic compounds, the United States
Environmental Protection Agency (USEPA) has classified phenol as a major pollutant. USEPA
has mandated achieving water purification level of less than 1 g L−1 (Keith and Telliand,
1979). The World Health Organization has also used the guideline set by USEPA to regulate
concentration of phenol in drinking water. The European Council Directive set a limit of 0.5 g
L−1 to set phenol concentration in drinking waters (Banerjee, 2011). The following table
provides a short overview of harmful effects of phenol on animals and human being:
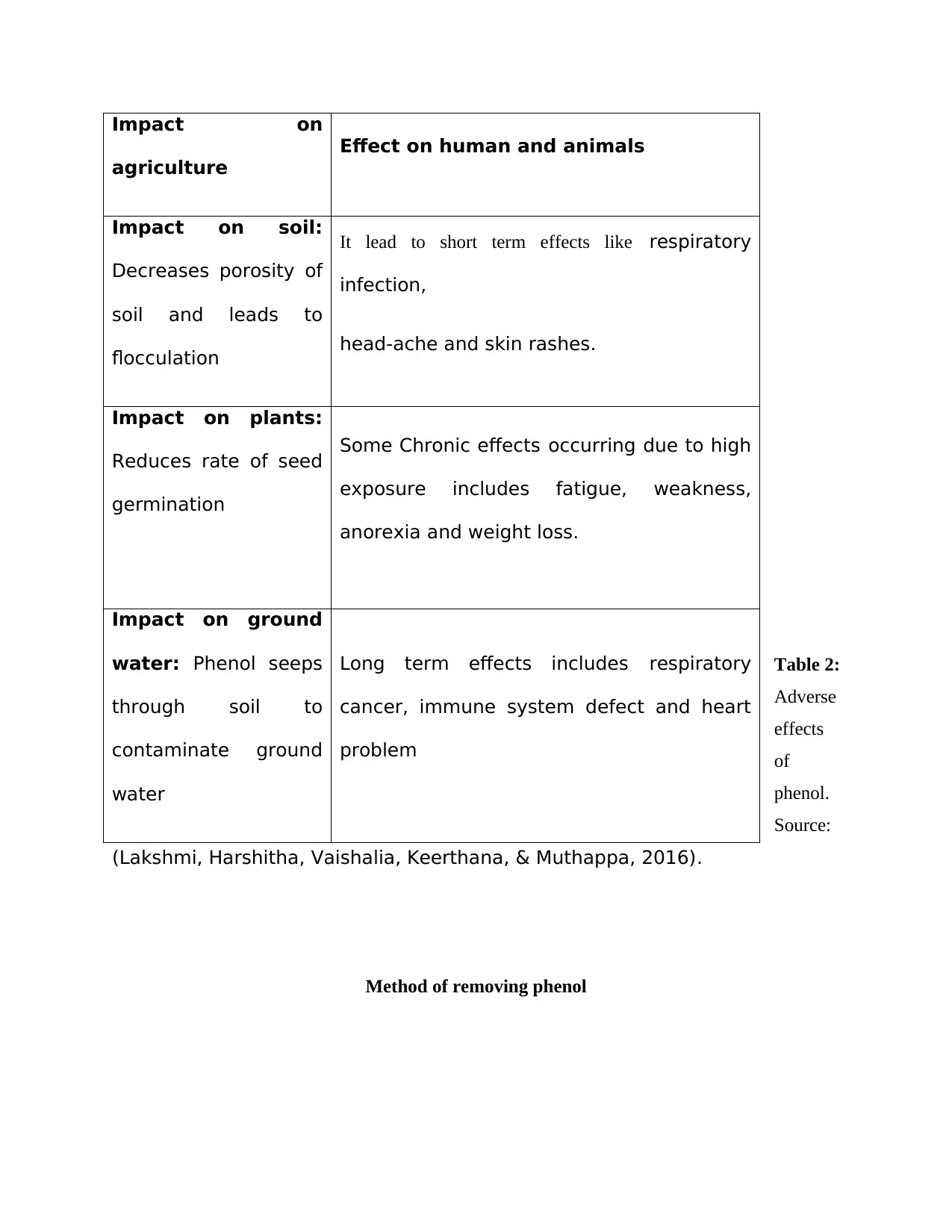
Table 2:
Adverse
effects
of
phenol.
Source:
(Lakshmi, Harshitha, Vaishalia, Keerthana, & Muthappa, 2016).
Method of removing phenol
Effect on human and animals
Impact on
agriculture
It lead to short term effects like respiratory
infection,
head-ache and skin rashes.
Impact on soil:
Decreases porosity of
soil and leads to
flocculation
Some Chronic effects occurring due to high
exposure includes fatigue, weakness,
anorexia and weight loss.
Impact on plants:
Reduces rate of seed
germination
Long term effects includes respiratory
cancer, immune system defect and heart
problem
Impact on ground
water: Phenol seeps
through soil to
contaminate ground
water
Adverse
effects
of
phenol.
Source:
(Lakshmi, Harshitha, Vaishalia, Keerthana, & Muthappa, 2016).
Method of removing phenol
Effect on human and animals
Impact on
agriculture
It lead to short term effects like respiratory
infection,
head-ache and skin rashes.
Impact on soil:
Decreases porosity of
soil and leads to
flocculation
Some Chronic effects occurring due to high
exposure includes fatigue, weakness,
anorexia and weight loss.
Impact on plants:
Reduces rate of seed
germination
Long term effects includes respiratory
cancer, immune system defect and heart
problem
Impact on ground
water: Phenol seeps
through soil to
contaminate ground
water
Paraphrase This Document
Need a fresh take? Get an instant paraphrase of this document with our AI Paraphraser
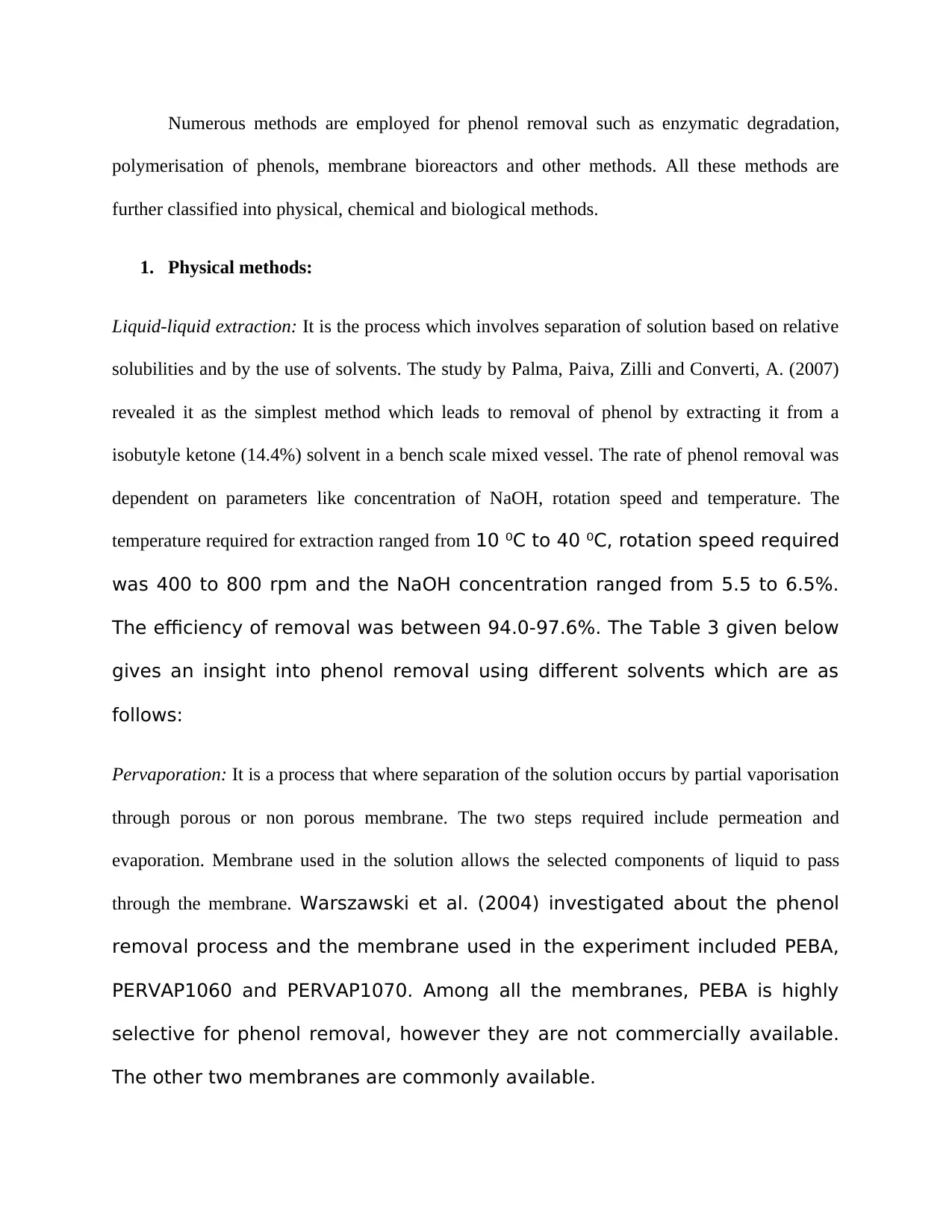
Numerous methods are employed for phenol removal such as enzymatic degradation,
polymerisation of phenols, membrane bioreactors and other methods. All these methods are
further classified into physical, chemical and biological methods.
1. Physical methods:
Liquid-liquid extraction: It is the process which involves separation of solution based on relative
solubilities and by the use of solvents. The study by Palma, Paiva, Zilli and Converti, A. (2007)
revealed it as the simplest method which leads to removal of phenol by extracting it from a
isobutyle ketone (14.4%) solvent in a bench scale mixed vessel. The rate of phenol removal was
dependent on parameters like concentration of NaOH, rotation speed and temperature. The
temperature required for extraction ranged from 10 ⁰C to 40 ⁰C, rotation speed required
was 400 to 800 rpm and the NaOH concentration ranged from 5.5 to 6.5%.
The efficiency of removal was between 94.0-97.6%. The Table 3 given below
gives an insight into phenol removal using different solvents which are as
follows:
Pervaporation: It is a process that where separation of the solution occurs by partial vaporisation
through porous or non porous membrane. The two steps required include permeation and
evaporation. Membrane used in the solution allows the selected components of liquid to pass
through the membrane. Warszawski et al. (2004) investigated about the phenol
removal process and the membrane used in the experiment included PEBA,
PERVAP1060 and PERVAP1070. Among all the membranes, PEBA is highly
selective for phenol removal, however they are not commercially available.
The other two membranes are commonly available.
polymerisation of phenols, membrane bioreactors and other methods. All these methods are
further classified into physical, chemical and biological methods.
1. Physical methods:
Liquid-liquid extraction: It is the process which involves separation of solution based on relative
solubilities and by the use of solvents. The study by Palma, Paiva, Zilli and Converti, A. (2007)
revealed it as the simplest method which leads to removal of phenol by extracting it from a
isobutyle ketone (14.4%) solvent in a bench scale mixed vessel. The rate of phenol removal was
dependent on parameters like concentration of NaOH, rotation speed and temperature. The
temperature required for extraction ranged from 10 ⁰C to 40 ⁰C, rotation speed required
was 400 to 800 rpm and the NaOH concentration ranged from 5.5 to 6.5%.
The efficiency of removal was between 94.0-97.6%. The Table 3 given below
gives an insight into phenol removal using different solvents which are as
follows:
Pervaporation: It is a process that where separation of the solution occurs by partial vaporisation
through porous or non porous membrane. The two steps required include permeation and
evaporation. Membrane used in the solution allows the selected components of liquid to pass
through the membrane. Warszawski et al. (2004) investigated about the phenol
removal process and the membrane used in the experiment included PEBA,
PERVAP1060 and PERVAP1070. Among all the membranes, PEBA is highly
selective for phenol removal, however they are not commercially available.
The other two membranes are commonly available.
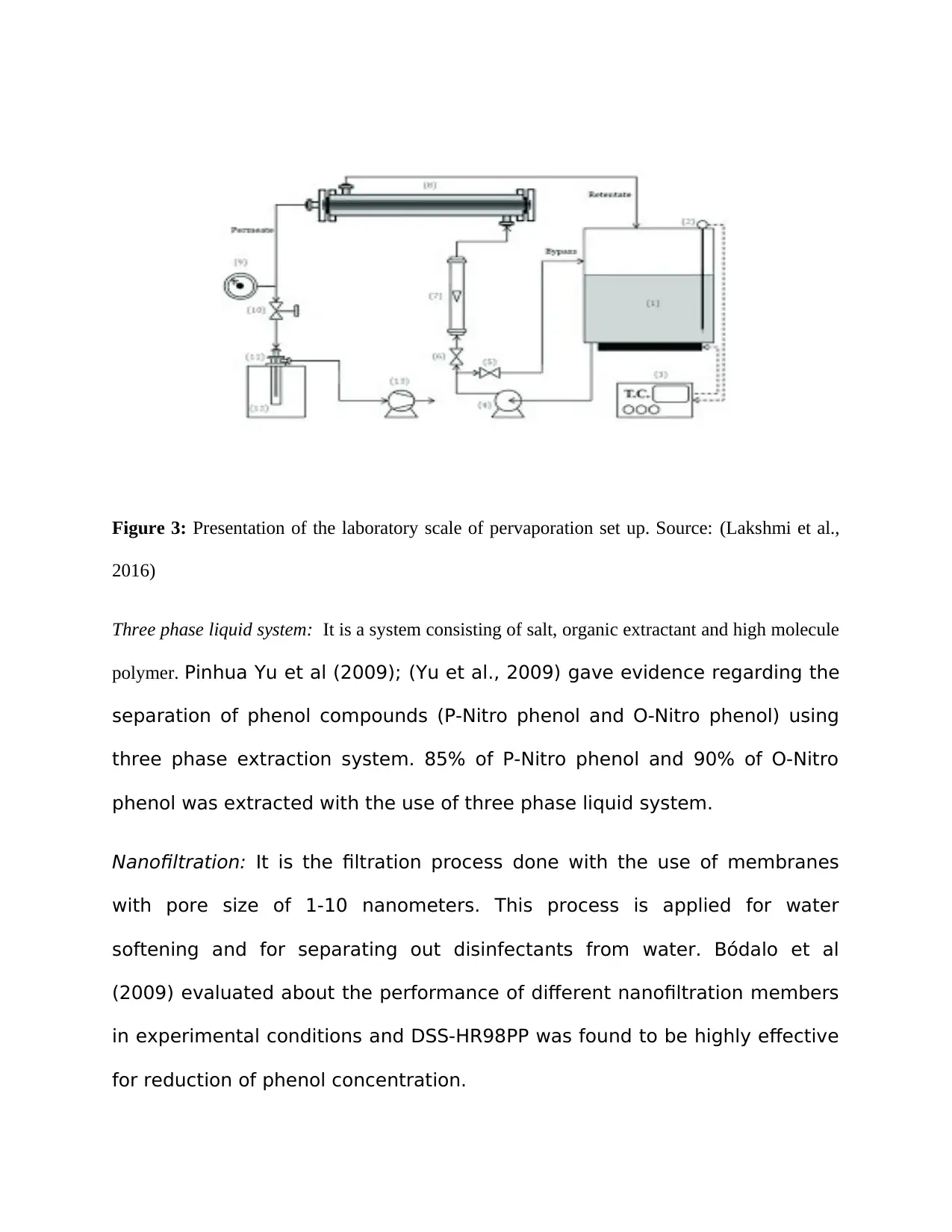
Figure 3: Presentation of the laboratory scale of pervaporation set up. Source: (Lakshmi et al.,
2016)
Three phase liquid system: It is a system consisting of salt, organic extractant and high molecule
polymer. Pinhua Yu et al (2009); (Yu et al., 2009) gave evidence regarding the
separation of phenol compounds (P-Nitro phenol and O-Nitro phenol) using
three phase extraction system. 85% of P-Nitro phenol and 90% of O-Nitro
phenol was extracted with the use of three phase liquid system.
Nanofiltration: It is the filtration process done with the use of membranes
with pore size of 1-10 nanometers. This process is applied for water
softening and for separating out disinfectants from water. Bódalo et al
(2009) evaluated about the performance of different nanofiltration members
in experimental conditions and DSS-HR98PP was found to be highly effective
for reduction of phenol concentration.
2016)
Three phase liquid system: It is a system consisting of salt, organic extractant and high molecule
polymer. Pinhua Yu et al (2009); (Yu et al., 2009) gave evidence regarding the
separation of phenol compounds (P-Nitro phenol and O-Nitro phenol) using
three phase extraction system. 85% of P-Nitro phenol and 90% of O-Nitro
phenol was extracted with the use of three phase liquid system.
Nanofiltration: It is the filtration process done with the use of membranes
with pore size of 1-10 nanometers. This process is applied for water
softening and for separating out disinfectants from water. Bódalo et al
(2009) evaluated about the performance of different nanofiltration members
in experimental conditions and DSS-HR98PP was found to be highly effective
for reduction of phenol concentration.
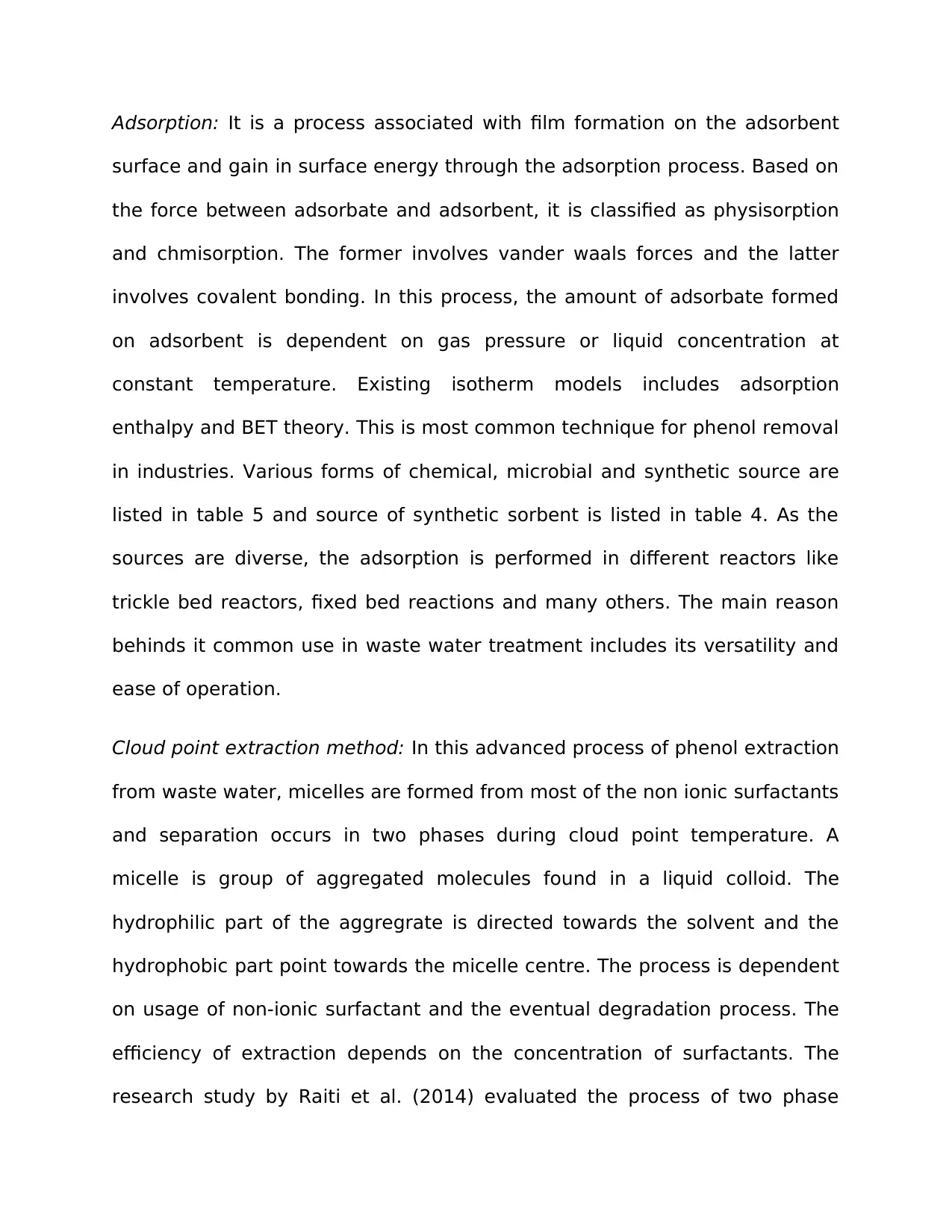
Adsorption: It is a process associated with film formation on the adsorbent
surface and gain in surface energy through the adsorption process. Based on
the force between adsorbate and adsorbent, it is classified as physisorption
and chmisorption. The former involves vander waals forces and the latter
involves covalent bonding. In this process, the amount of adsorbate formed
on adsorbent is dependent on gas pressure or liquid concentration at
constant temperature. Existing isotherm models includes adsorption
enthalpy and BET theory. This is most common technique for phenol removal
in industries. Various forms of chemical, microbial and synthetic source are
listed in table 5 and source of synthetic sorbent is listed in table 4. As the
sources are diverse, the adsorption is performed in different reactors like
trickle bed reactors, fixed bed reactions and many others. The main reason
behinds it common use in waste water treatment includes its versatility and
ease of operation.
Cloud point extraction method: In this advanced process of phenol extraction
from waste water, micelles are formed from most of the non ionic surfactants
and separation occurs in two phases during cloud point temperature. A
micelle is group of aggregated molecules found in a liquid colloid. The
hydrophilic part of the aggregrate is directed towards the solvent and the
hydrophobic part point towards the micelle centre. The process is dependent
on usage of non-ionic surfactant and the eventual degradation process. The
efficiency of extraction depends on the concentration of surfactants. The
research study by Raiti et al. (2014) evaluated the process of two phase
surface and gain in surface energy through the adsorption process. Based on
the force between adsorbate and adsorbent, it is classified as physisorption
and chmisorption. The former involves vander waals forces and the latter
involves covalent bonding. In this process, the amount of adsorbate formed
on adsorbent is dependent on gas pressure or liquid concentration at
constant temperature. Existing isotherm models includes adsorption
enthalpy and BET theory. This is most common technique for phenol removal
in industries. Various forms of chemical, microbial and synthetic source are
listed in table 5 and source of synthetic sorbent is listed in table 4. As the
sources are diverse, the adsorption is performed in different reactors like
trickle bed reactors, fixed bed reactions and many others. The main reason
behinds it common use in waste water treatment includes its versatility and
ease of operation.
Cloud point extraction method: In this advanced process of phenol extraction
from waste water, micelles are formed from most of the non ionic surfactants
and separation occurs in two phases during cloud point temperature. A
micelle is group of aggregated molecules found in a liquid colloid. The
hydrophilic part of the aggregrate is directed towards the solvent and the
hydrophobic part point towards the micelle centre. The process is dependent
on usage of non-ionic surfactant and the eventual degradation process. The
efficiency of extraction depends on the concentration of surfactants. The
research study by Raiti et al. (2014) evaluated the process of two phase
Secure Best Marks with AI Grader
Need help grading? Try our AI Grader for instant feedback on your assignments.
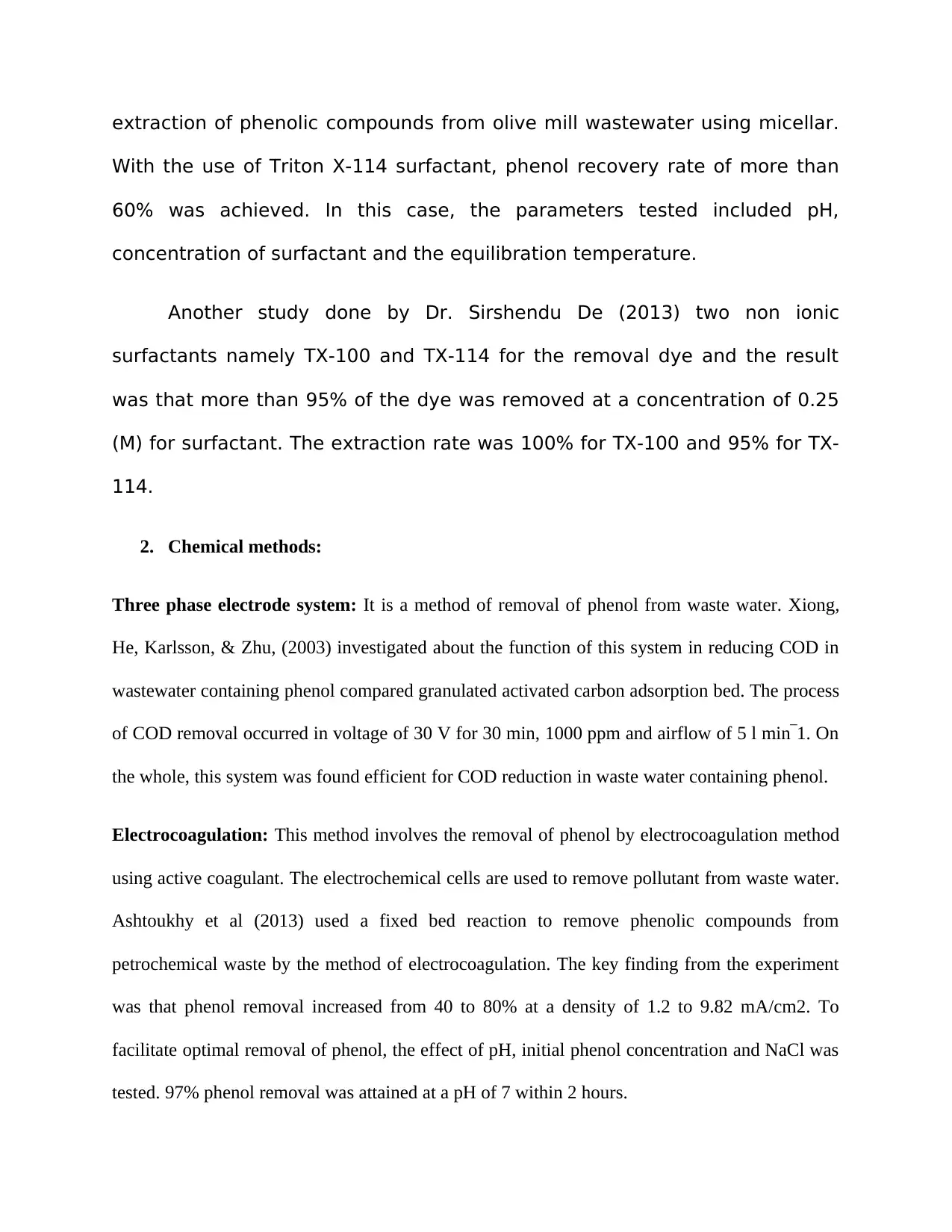
extraction of phenolic compounds from olive mill wastewater using micellar.
With the use of Triton X-114 surfactant, phenol recovery rate of more than
60% was achieved. In this case, the parameters tested included pH,
concentration of surfactant and the equilibration temperature.
Another study done by Dr. Sirshendu De (2013) two non ionic
surfactants namely TX-100 and TX-114 for the removal dye and the result
was that more than 95% of the dye was removed at a concentration of 0.25
(M) for surfactant. The extraction rate was 100% for TX-100 and 95% for TX-
114.
2. Chemical methods:
Three phase electrode system: It is a method of removal of phenol from waste water. Xiong,
He, Karlsson, & Zhu, (2003) investigated about the function of this system in reducing COD in
wastewater containing phenol compared granulated activated carbon adsorption bed. The process
of COD removal occurred in voltage of 30 V for 30 min, 1000 ppm and airflow of 5 l min‾1. On
the whole, this system was found efficient for COD reduction in waste water containing phenol.
Electrocoagulation: This method involves the removal of phenol by electrocoagulation method
using active coagulant. The electrochemical cells are used to remove pollutant from waste water.
Ashtoukhy et al (2013) used a fixed bed reaction to remove phenolic compounds from
petrochemical waste by the method of electrocoagulation. The key finding from the experiment
was that phenol removal increased from 40 to 80% at a density of 1.2 to 9.82 mA/cm2. To
facilitate optimal removal of phenol, the effect of pH, initial phenol concentration and NaCl was
tested. 97% phenol removal was attained at a pH of 7 within 2 hours.
With the use of Triton X-114 surfactant, phenol recovery rate of more than
60% was achieved. In this case, the parameters tested included pH,
concentration of surfactant and the equilibration temperature.
Another study done by Dr. Sirshendu De (2013) two non ionic
surfactants namely TX-100 and TX-114 for the removal dye and the result
was that more than 95% of the dye was removed at a concentration of 0.25
(M) for surfactant. The extraction rate was 100% for TX-100 and 95% for TX-
114.
2. Chemical methods:
Three phase electrode system: It is a method of removal of phenol from waste water. Xiong,
He, Karlsson, & Zhu, (2003) investigated about the function of this system in reducing COD in
wastewater containing phenol compared granulated activated carbon adsorption bed. The process
of COD removal occurred in voltage of 30 V for 30 min, 1000 ppm and airflow of 5 l min‾1. On
the whole, this system was found efficient for COD reduction in waste water containing phenol.
Electrocoagulation: This method involves the removal of phenol by electrocoagulation method
using active coagulant. The electrochemical cells are used to remove pollutant from waste water.
Ashtoukhy et al (2013) used a fixed bed reaction to remove phenolic compounds from
petrochemical waste by the method of electrocoagulation. The key finding from the experiment
was that phenol removal increased from 40 to 80% at a density of 1.2 to 9.82 mA/cm2. To
facilitate optimal removal of phenol, the effect of pH, initial phenol concentration and NaCl was
tested. 97% phenol removal was attained at a pH of 7 within 2 hours.
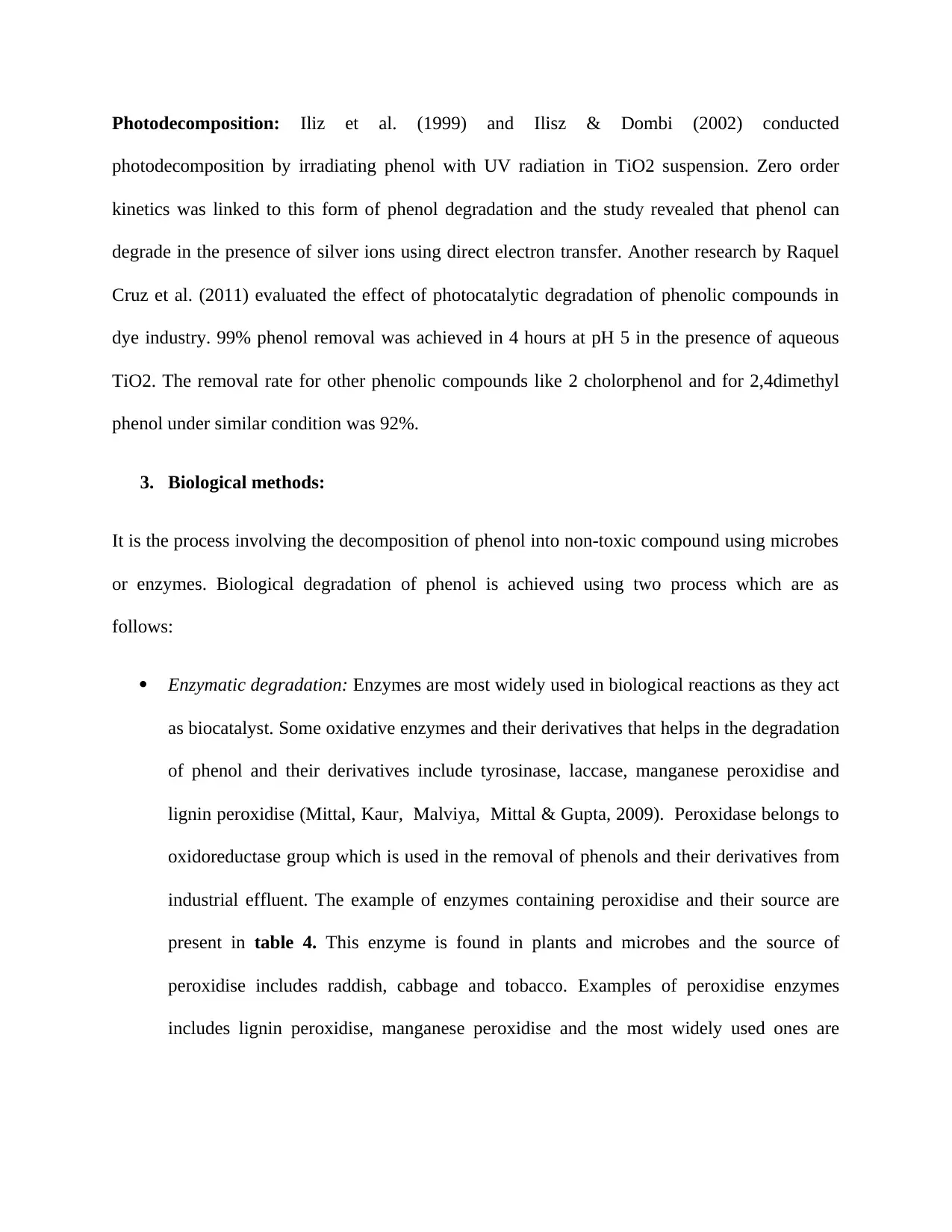
Photodecomposition: Iliz et al. (1999) and Ilisz & Dombi (2002) conducted
photodecomposition by irradiating phenol with UV radiation in TiO2 suspension. Zero order
kinetics was linked to this form of phenol degradation and the study revealed that phenol can
degrade in the presence of silver ions using direct electron transfer. Another research by Raquel
Cruz et al. (2011) evaluated the effect of photocatalytic degradation of phenolic compounds in
dye industry. 99% phenol removal was achieved in 4 hours at pH 5 in the presence of aqueous
TiO2. The removal rate for other phenolic compounds like 2 cholorphenol and for 2,4dimethyl
phenol under similar condition was 92%.
3. Biological methods:
It is the process involving the decomposition of phenol into non-toxic compound using microbes
or enzymes. Biological degradation of phenol is achieved using two process which are as
follows:
Enzymatic degradation: Enzymes are most widely used in biological reactions as they act
as biocatalyst. Some oxidative enzymes and their derivatives that helps in the degradation
of phenol and their derivatives include tyrosinase, laccase, manganese peroxidise and
lignin peroxidise (Mittal, Kaur, Malviya, Mittal & Gupta, 2009). Peroxidase belongs to
oxidoreductase group which is used in the removal of phenols and their derivatives from
industrial effluent. The example of enzymes containing peroxidise and their source are
present in table 4. This enzyme is found in plants and microbes and the source of
peroxidise includes raddish, cabbage and tobacco. Examples of peroxidise enzymes
includes lignin peroxidise, manganese peroxidise and the most widely used ones are
photodecomposition by irradiating phenol with UV radiation in TiO2 suspension. Zero order
kinetics was linked to this form of phenol degradation and the study revealed that phenol can
degrade in the presence of silver ions using direct electron transfer. Another research by Raquel
Cruz et al. (2011) evaluated the effect of photocatalytic degradation of phenolic compounds in
dye industry. 99% phenol removal was achieved in 4 hours at pH 5 in the presence of aqueous
TiO2. The removal rate for other phenolic compounds like 2 cholorphenol and for 2,4dimethyl
phenol under similar condition was 92%.
3. Biological methods:
It is the process involving the decomposition of phenol into non-toxic compound using microbes
or enzymes. Biological degradation of phenol is achieved using two process which are as
follows:
Enzymatic degradation: Enzymes are most widely used in biological reactions as they act
as biocatalyst. Some oxidative enzymes and their derivatives that helps in the degradation
of phenol and their derivatives include tyrosinase, laccase, manganese peroxidise and
lignin peroxidise (Mittal, Kaur, Malviya, Mittal & Gupta, 2009). Peroxidase belongs to
oxidoreductase group which is used in the removal of phenols and their derivatives from
industrial effluent. The example of enzymes containing peroxidise and their source are
present in table 4. This enzyme is found in plants and microbes and the source of
peroxidise includes raddish, cabbage and tobacco. Examples of peroxidise enzymes
includes lignin peroxidise, manganese peroxidise and the most widely used ones are
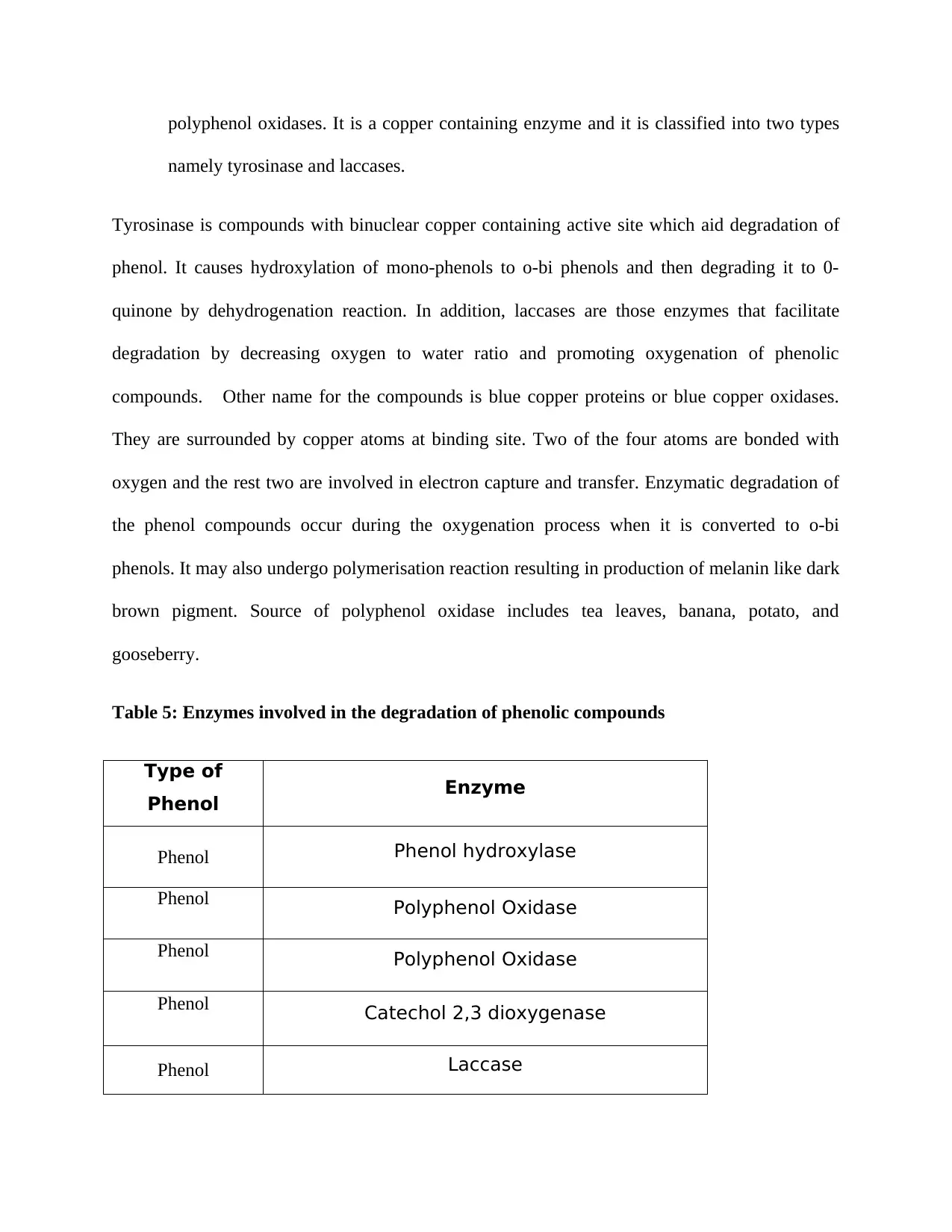
polyphenol oxidases. It is a copper containing enzyme and it is classified into two types
namely tyrosinase and laccases.
Tyrosinase is compounds with binuclear copper containing active site which aid degradation of
phenol. It causes hydroxylation of mono-phenols to o-bi phenols and then degrading it to 0-
quinone by dehydrogenation reaction. In addition, laccases are those enzymes that facilitate
degradation by decreasing oxygen to water ratio and promoting oxygenation of phenolic
compounds. Other name for the compounds is blue copper proteins or blue copper oxidases.
They are surrounded by copper atoms at binding site. Two of the four atoms are bonded with
oxygen and the rest two are involved in electron capture and transfer. Enzymatic degradation of
the phenol compounds occur during the oxygenation process when it is converted to o-bi
phenols. It may also undergo polymerisation reaction resulting in production of melanin like dark
brown pigment. Source of polyphenol oxidase includes tea leaves, banana, potato, and
gooseberry.
Table 5: Enzymes involved in the degradation of phenolic compounds
Type of
Phenol Enzyme
Phenol Phenol hydroxylase
Phenol Polyphenol Oxidase
Phenol Polyphenol Oxidase
Phenol Catechol 2,3 dioxygenase
Phenol Laccase
namely tyrosinase and laccases.
Tyrosinase is compounds with binuclear copper containing active site which aid degradation of
phenol. It causes hydroxylation of mono-phenols to o-bi phenols and then degrading it to 0-
quinone by dehydrogenation reaction. In addition, laccases are those enzymes that facilitate
degradation by decreasing oxygen to water ratio and promoting oxygenation of phenolic
compounds. Other name for the compounds is blue copper proteins or blue copper oxidases.
They are surrounded by copper atoms at binding site. Two of the four atoms are bonded with
oxygen and the rest two are involved in electron capture and transfer. Enzymatic degradation of
the phenol compounds occur during the oxygenation process when it is converted to o-bi
phenols. It may also undergo polymerisation reaction resulting in production of melanin like dark
brown pigment. Source of polyphenol oxidase includes tea leaves, banana, potato, and
gooseberry.
Table 5: Enzymes involved in the degradation of phenolic compounds
Type of
Phenol Enzyme
Phenol Phenol hydroxylase
Phenol Polyphenol Oxidase
Phenol Polyphenol Oxidase
Phenol Catechol 2,3 dioxygenase
Phenol Laccase
Paraphrase This Document
Need a fresh take? Get an instant paraphrase of this document with our AI Paraphraser
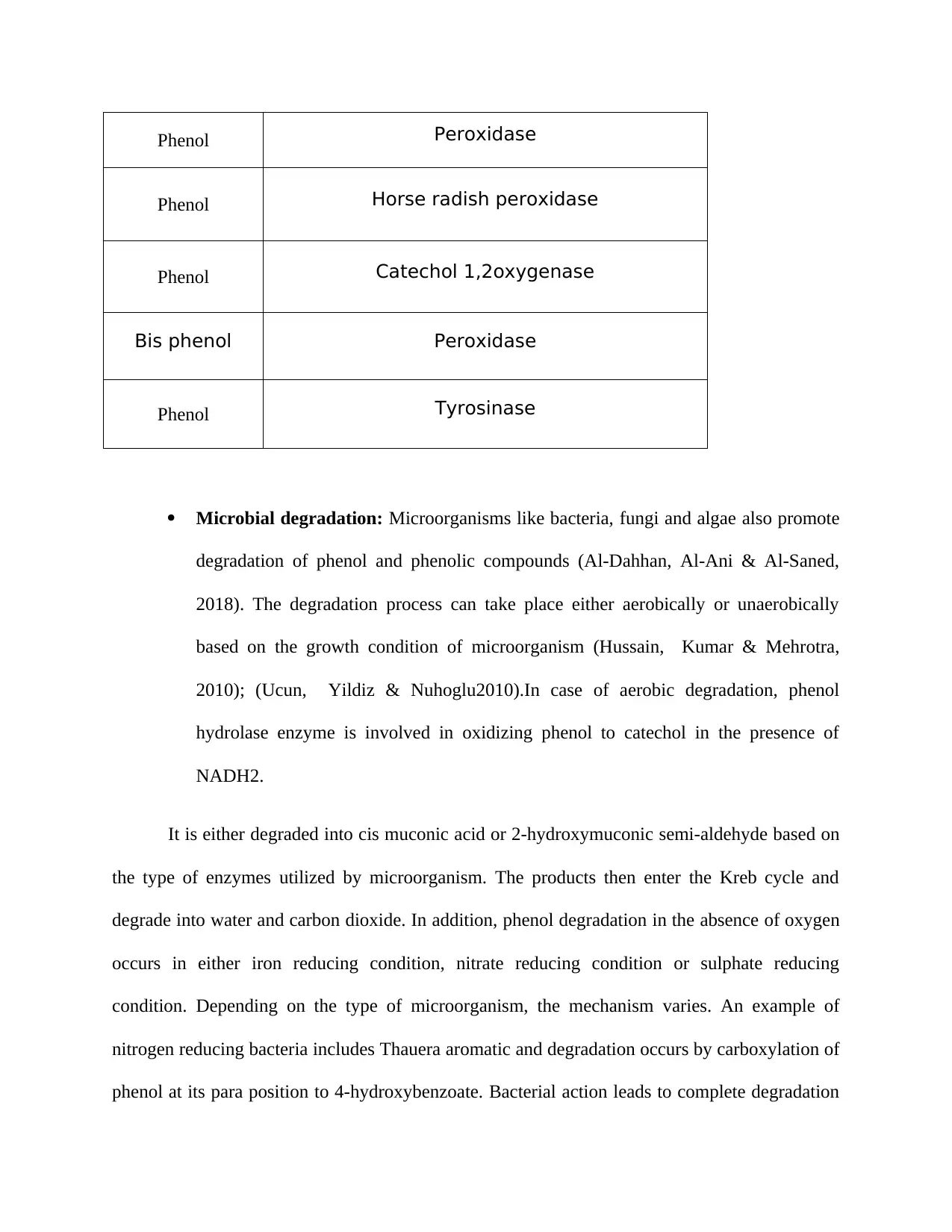
Phenol Peroxidase
Phenol Horse radish peroxidase
Phenol Catechol 1,2oxygenase
Bis phenol Peroxidase
Phenol Tyrosinase
Microbial degradation: Microorganisms like bacteria, fungi and algae also promote
degradation of phenol and phenolic compounds (Al-Dahhan, Al-Ani & Al-Saned,
2018). The degradation process can take place either aerobically or unaerobically
based on the growth condition of microorganism (Hussain, Kumar & Mehrotra,
2010); (Ucun, Yildiz & Nuhoglu2010).In case of aerobic degradation, phenol
hydrolase enzyme is involved in oxidizing phenol to catechol in the presence of
NADH2.
It is either degraded into cis muconic acid or 2-hydroxymuconic semi-aldehyde based on
the type of enzymes utilized by microorganism. The products then enter the Kreb cycle and
degrade into water and carbon dioxide. In addition, phenol degradation in the absence of oxygen
occurs in either iron reducing condition, nitrate reducing condition or sulphate reducing
condition. Depending on the type of microorganism, the mechanism varies. An example of
nitrogen reducing bacteria includes Thauera aromatic and degradation occurs by carboxylation of
phenol at its para position to 4-hydroxybenzoate. Bacterial action leads to complete degradation
Phenol Horse radish peroxidase
Phenol Catechol 1,2oxygenase
Bis phenol Peroxidase
Phenol Tyrosinase
Microbial degradation: Microorganisms like bacteria, fungi and algae also promote
degradation of phenol and phenolic compounds (Al-Dahhan, Al-Ani & Al-Saned,
2018). The degradation process can take place either aerobically or unaerobically
based on the growth condition of microorganism (Hussain, Kumar & Mehrotra,
2010); (Ucun, Yildiz & Nuhoglu2010).In case of aerobic degradation, phenol
hydrolase enzyme is involved in oxidizing phenol to catechol in the presence of
NADH2.
It is either degraded into cis muconic acid or 2-hydroxymuconic semi-aldehyde based on
the type of enzymes utilized by microorganism. The products then enter the Kreb cycle and
degrade into water and carbon dioxide. In addition, phenol degradation in the absence of oxygen
occurs in either iron reducing condition, nitrate reducing condition or sulphate reducing
condition. Depending on the type of microorganism, the mechanism varies. An example of
nitrogen reducing bacteria includes Thauera aromatic and degradation occurs by carboxylation of
phenol at its para position to 4-hydroxybenzoate. Bacterial action leads to complete degradation
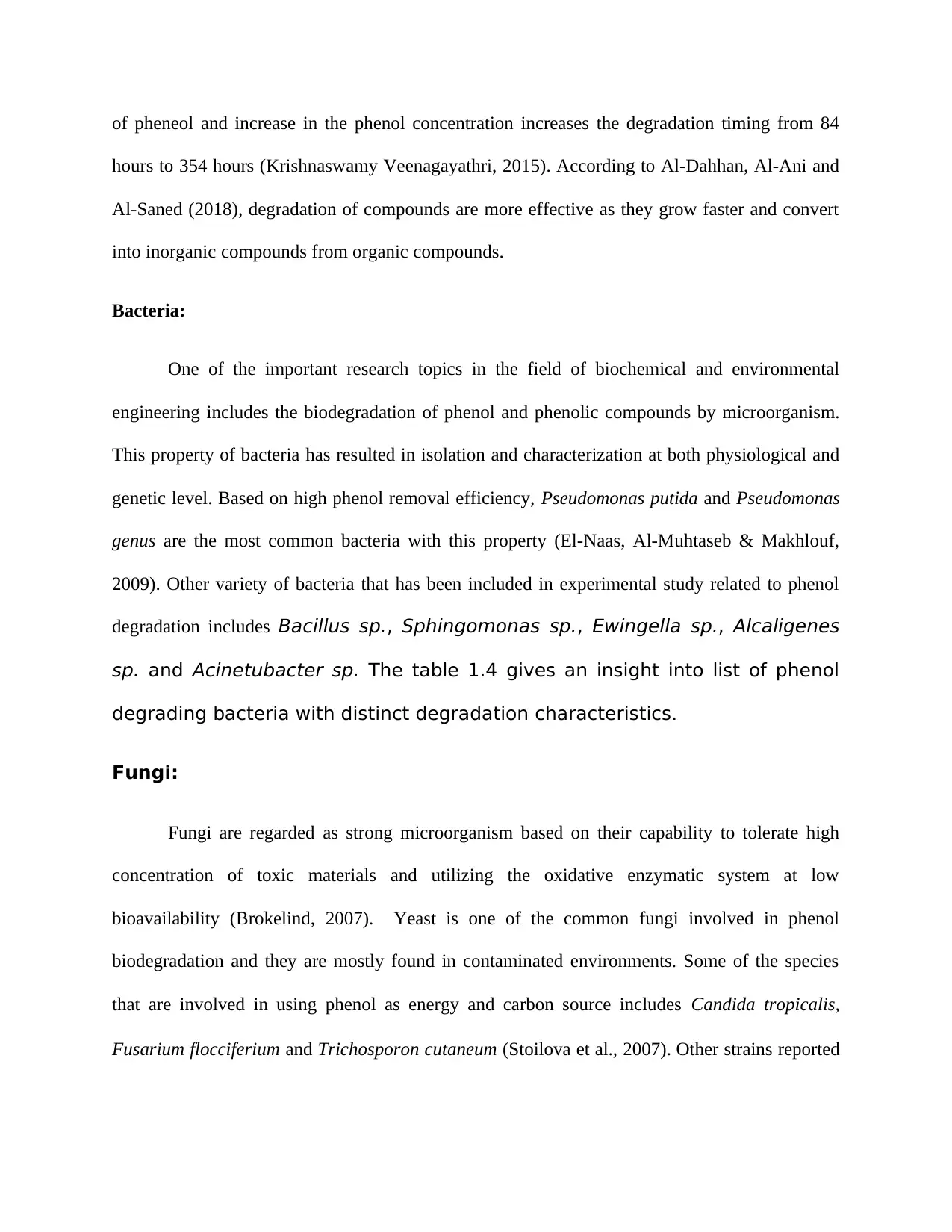
of pheneol and increase in the phenol concentration increases the degradation timing from 84
hours to 354 hours (Krishnaswamy Veenagayathri, 2015). According to Al-Dahhan, Al-Ani and
Al-Saned (2018), degradation of compounds are more effective as they grow faster and convert
into inorganic compounds from organic compounds.
Bacteria:
One of the important research topics in the field of biochemical and environmental
engineering includes the biodegradation of phenol and phenolic compounds by microorganism.
This property of bacteria has resulted in isolation and characterization at both physiological and
genetic level. Based on high phenol removal efficiency, Pseudomonas putida and Pseudomonas
genus are the most common bacteria with this property (El-Naas, Al-Muhtaseb & Makhlouf,
2009). Other variety of bacteria that has been included in experimental study related to phenol
degradation includes Bacillus sp., Sphingomonas sp., Ewingella sp., Alcaligenes
sp. and Acinetubacter sp. The table 1.4 gives an insight into list of phenol
degrading bacteria with distinct degradation characteristics.
Fungi:
Fungi are regarded as strong microorganism based on their capability to tolerate high
concentration of toxic materials and utilizing the oxidative enzymatic system at low
bioavailability (Brokelind, 2007). Yeast is one of the common fungi involved in phenol
biodegradation and they are mostly found in contaminated environments. Some of the species
that are involved in using phenol as energy and carbon source includes Candida tropicalis,
Fusarium flocciferium and Trichosporon cutaneum (Stoilova et al., 2007). Other strains reported
hours to 354 hours (Krishnaswamy Veenagayathri, 2015). According to Al-Dahhan, Al-Ani and
Al-Saned (2018), degradation of compounds are more effective as they grow faster and convert
into inorganic compounds from organic compounds.
Bacteria:
One of the important research topics in the field of biochemical and environmental
engineering includes the biodegradation of phenol and phenolic compounds by microorganism.
This property of bacteria has resulted in isolation and characterization at both physiological and
genetic level. Based on high phenol removal efficiency, Pseudomonas putida and Pseudomonas
genus are the most common bacteria with this property (El-Naas, Al-Muhtaseb & Makhlouf,
2009). Other variety of bacteria that has been included in experimental study related to phenol
degradation includes Bacillus sp., Sphingomonas sp., Ewingella sp., Alcaligenes
sp. and Acinetubacter sp. The table 1.4 gives an insight into list of phenol
degrading bacteria with distinct degradation characteristics.
Fungi:
Fungi are regarded as strong microorganism based on their capability to tolerate high
concentration of toxic materials and utilizing the oxidative enzymatic system at low
bioavailability (Brokelind, 2007). Yeast is one of the common fungi involved in phenol
biodegradation and they are mostly found in contaminated environments. Some of the species
that are involved in using phenol as energy and carbon source includes Candida tropicalis,
Fusarium flocciferium and Trichosporon cutaneum (Stoilova et al., 2007). Other strains reported
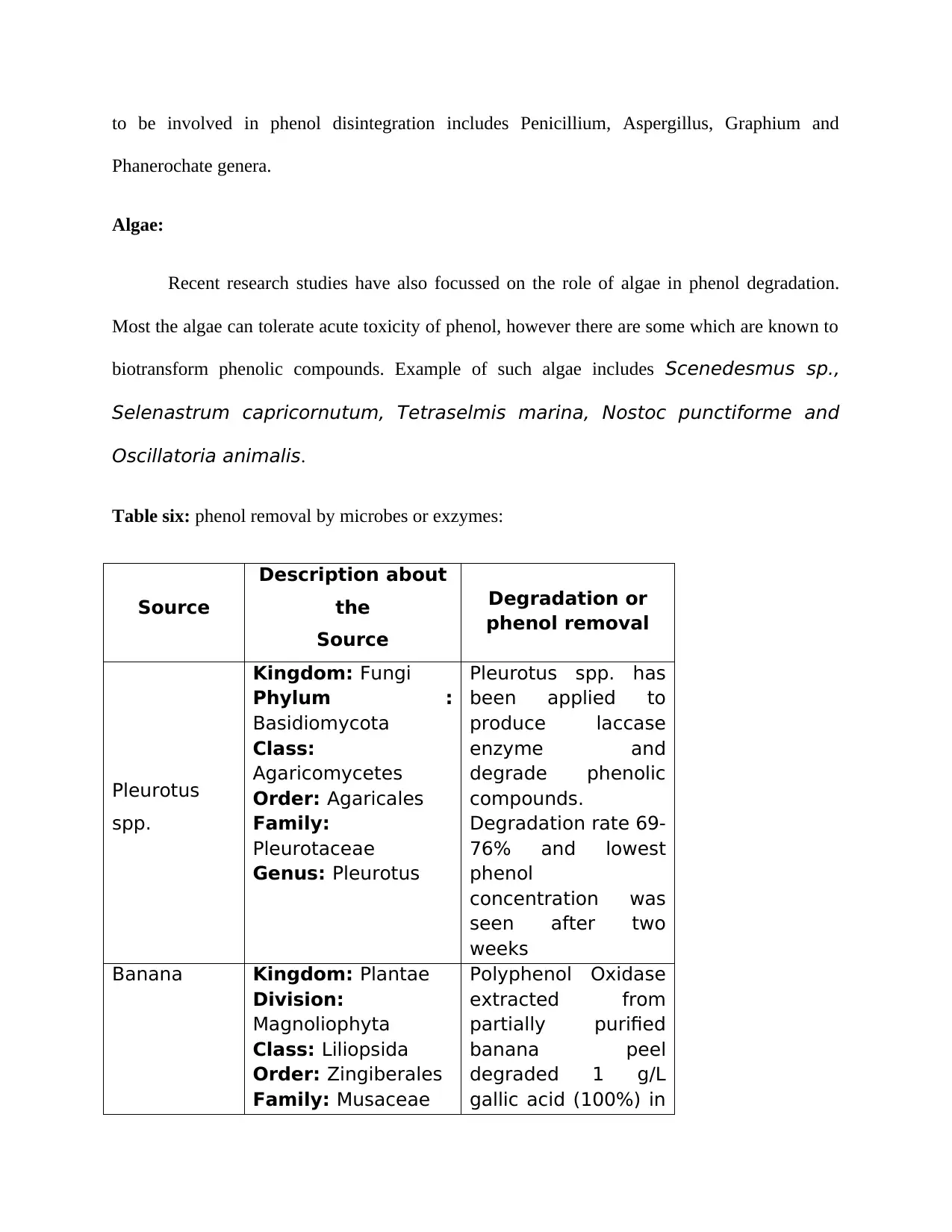
to be involved in phenol disintegration includes Penicillium, Aspergillus, Graphium and
Phanerochate genera.
Algae:
Recent research studies have also focussed on the role of algae in phenol degradation.
Most the algae can tolerate acute toxicity of phenol, however there are some which are known to
biotransform phenolic compounds. Example of such algae includes Scenedesmus sp.,
Selenastrum capricornutum, Tetraselmis marina, Nostoc punctiforme and
Oscillatoria animalis.
Table six: phenol removal by microbes or exzymes:
Source
Description about
the
Source
Degradation or
phenol removal
Pleurotus
spp.
Kingdom: Fungi
Phylum :
Basidiomycota
Class:
Agaricomycetes
Order: Agaricales
Family:
Pleurotaceae
Genus: Pleurotus
Pleurotus spp. has
been applied to
produce laccase
enzyme and
degrade phenolic
compounds.
Degradation rate 69-
76% and lowest
phenol
concentration was
seen after two
weeks
Banana Kingdom: Plantae
Division:
Magnoliophyta
Class: Liliopsida
Order: Zingiberales
Family: Musaceae
Polyphenol Oxidase
extracted from
partially purified
banana peel
degraded 1 g/L
gallic acid (100%) in
Phanerochate genera.
Algae:
Recent research studies have also focussed on the role of algae in phenol degradation.
Most the algae can tolerate acute toxicity of phenol, however there are some which are known to
biotransform phenolic compounds. Example of such algae includes Scenedesmus sp.,
Selenastrum capricornutum, Tetraselmis marina, Nostoc punctiforme and
Oscillatoria animalis.
Table six: phenol removal by microbes or exzymes:
Source
Description about
the
Source
Degradation or
phenol removal
Pleurotus
spp.
Kingdom: Fungi
Phylum :
Basidiomycota
Class:
Agaricomycetes
Order: Agaricales
Family:
Pleurotaceae
Genus: Pleurotus
Pleurotus spp. has
been applied to
produce laccase
enzyme and
degrade phenolic
compounds.
Degradation rate 69-
76% and lowest
phenol
concentration was
seen after two
weeks
Banana Kingdom: Plantae
Division:
Magnoliophyta
Class: Liliopsida
Order: Zingiberales
Family: Musaceae
Polyphenol Oxidase
extracted from
partially purified
banana peel
degraded 1 g/L
gallic acid (100%) in
Secure Best Marks with AI Grader
Need help grading? Try our AI Grader for instant feedback on your assignments.
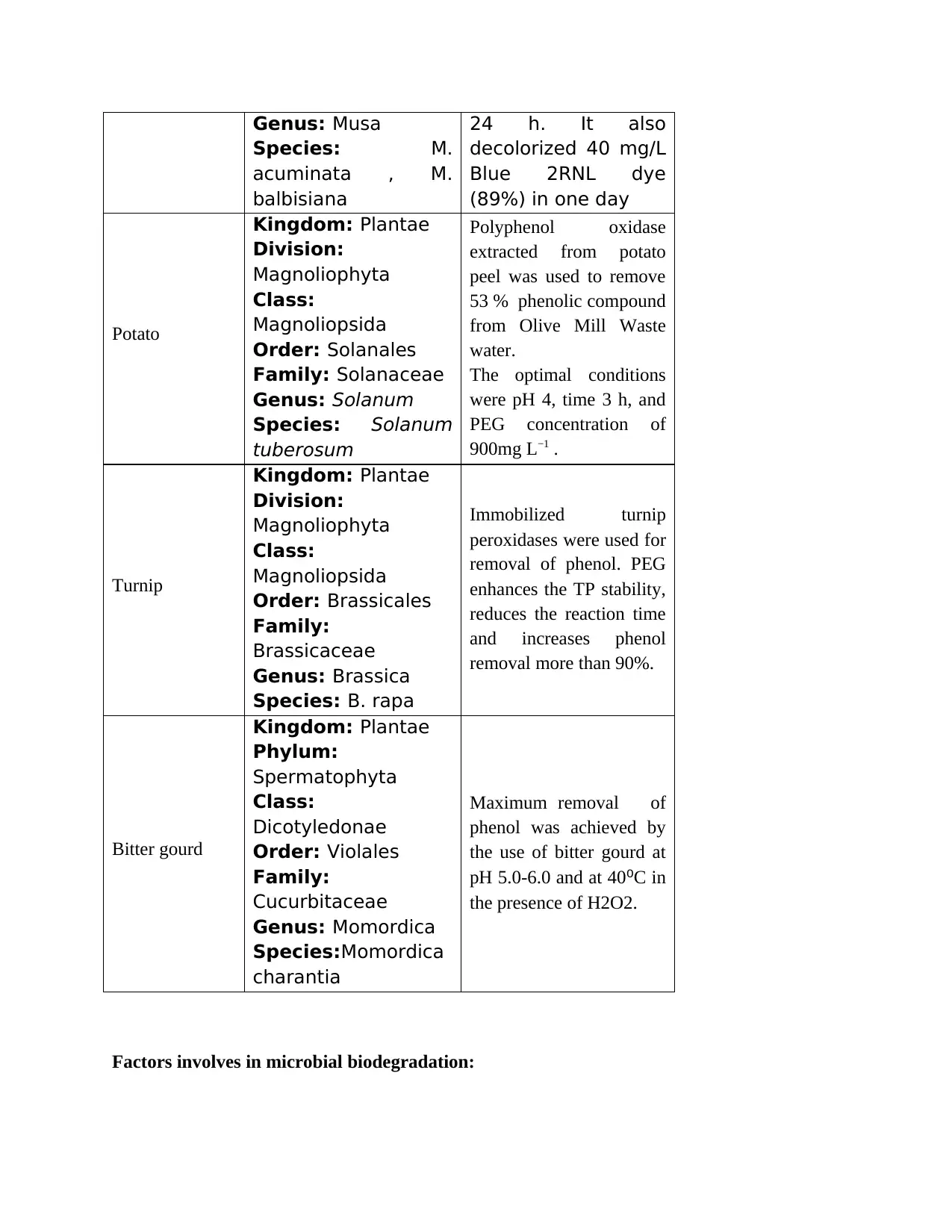
Genus: Musa
Species: M.
acuminata , M.
balbisiana
24 h. It also
decolorized 40 mg/L
Blue 2RNL dye
(89%) in one day
Potato
Kingdom: Plantae
Division:
Magnoliophyta
Class:
Magnoliopsida
Order: Solanales
Family: Solanaceae
Genus: Solanum
Species: Solanum
tuberosum
Polyphenol oxidase
extracted from potato
peel was used to remove
53 % phenolic compound
from Olive Mill Waste
water.
The optimal conditions
were pH 4, time 3 h, and
PEG concentration of
900mg L−1 .
Turnip
Kingdom: Plantae
Division:
Magnoliophyta
Class:
Magnoliopsida
Order: Brassicales
Family:
Brassicaceae
Genus: Brassica
Species: B. rapa
Immobilized turnip
peroxidases were used for
removal of phenol. PEG
enhances the TP stability,
reduces the reaction time
and increases phenol
removal more than 90%.
Bitter gourd
Kingdom: Plantae
Phylum:
Spermatophyta
Class:
Dicotyledonae
Order: Violales
Family:
Cucurbitaceae
Genus: Momordica
Species:Momordica
charantia
Maximum removal of
phenol was achieved by
the use of bitter gourd at
pH 5.0-6.0 and at 40⁰C in
the presence of H2O2.
Factors involves in microbial biodegradation:
Species: M.
acuminata , M.
balbisiana
24 h. It also
decolorized 40 mg/L
Blue 2RNL dye
(89%) in one day
Potato
Kingdom: Plantae
Division:
Magnoliophyta
Class:
Magnoliopsida
Order: Solanales
Family: Solanaceae
Genus: Solanum
Species: Solanum
tuberosum
Polyphenol oxidase
extracted from potato
peel was used to remove
53 % phenolic compound
from Olive Mill Waste
water.
The optimal conditions
were pH 4, time 3 h, and
PEG concentration of
900mg L−1 .
Turnip
Kingdom: Plantae
Division:
Magnoliophyta
Class:
Magnoliopsida
Order: Brassicales
Family:
Brassicaceae
Genus: Brassica
Species: B. rapa
Immobilized turnip
peroxidases were used for
removal of phenol. PEG
enhances the TP stability,
reduces the reaction time
and increases phenol
removal more than 90%.
Bitter gourd
Kingdom: Plantae
Phylum:
Spermatophyta
Class:
Dicotyledonae
Order: Violales
Family:
Cucurbitaceae
Genus: Momordica
Species:Momordica
charantia
Maximum removal of
phenol was achieved by
the use of bitter gourd at
pH 5.0-6.0 and at 40⁰C in
the presence of H2O2.
Factors involves in microbial biodegradation:
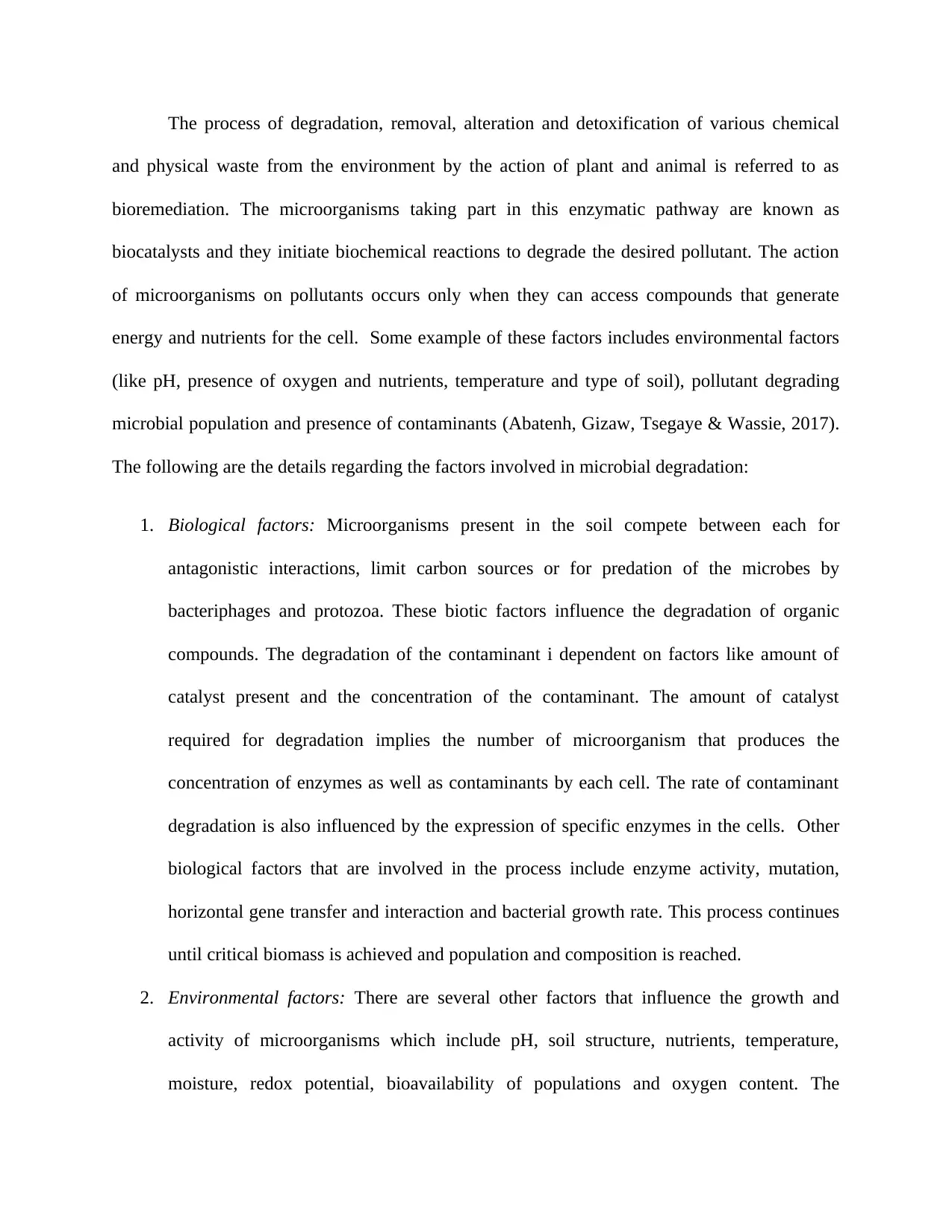
The process of degradation, removal, alteration and detoxification of various chemical
and physical waste from the environment by the action of plant and animal is referred to as
bioremediation. The microorganisms taking part in this enzymatic pathway are known as
biocatalysts and they initiate biochemical reactions to degrade the desired pollutant. The action
of microorganisms on pollutants occurs only when they can access compounds that generate
energy and nutrients for the cell. Some example of these factors includes environmental factors
(like pH, presence of oxygen and nutrients, temperature and type of soil), pollutant degrading
microbial population and presence of contaminants (Abatenh, Gizaw, Tsegaye & Wassie, 2017).
The following are the details regarding the factors involved in microbial degradation:
1. Biological factors: Microorganisms present in the soil compete between each for
antagonistic interactions, limit carbon sources or for predation of the microbes by
bacteriphages and protozoa. These biotic factors influence the degradation of organic
compounds. The degradation of the contaminant i dependent on factors like amount of
catalyst present and the concentration of the contaminant. The amount of catalyst
required for degradation implies the number of microorganism that produces the
concentration of enzymes as well as contaminants by each cell. The rate of contaminant
degradation is also influenced by the expression of specific enzymes in the cells. Other
biological factors that are involved in the process include enzyme activity, mutation,
horizontal gene transfer and interaction and bacterial growth rate. This process continues
until critical biomass is achieved and population and composition is reached.
2. Environmental factors: There are several other factors that influence the growth and
activity of microorganisms which include pH, soil structure, nutrients, temperature,
moisture, redox potential, bioavailability of populations and oxygen content. The
and physical waste from the environment by the action of plant and animal is referred to as
bioremediation. The microorganisms taking part in this enzymatic pathway are known as
biocatalysts and they initiate biochemical reactions to degrade the desired pollutant. The action
of microorganisms on pollutants occurs only when they can access compounds that generate
energy and nutrients for the cell. Some example of these factors includes environmental factors
(like pH, presence of oxygen and nutrients, temperature and type of soil), pollutant degrading
microbial population and presence of contaminants (Abatenh, Gizaw, Tsegaye & Wassie, 2017).
The following are the details regarding the factors involved in microbial degradation:
1. Biological factors: Microorganisms present in the soil compete between each for
antagonistic interactions, limit carbon sources or for predation of the microbes by
bacteriphages and protozoa. These biotic factors influence the degradation of organic
compounds. The degradation of the contaminant i dependent on factors like amount of
catalyst present and the concentration of the contaminant. The amount of catalyst
required for degradation implies the number of microorganism that produces the
concentration of enzymes as well as contaminants by each cell. The rate of contaminant
degradation is also influenced by the expression of specific enzymes in the cells. Other
biological factors that are involved in the process include enzyme activity, mutation,
horizontal gene transfer and interaction and bacterial growth rate. This process continues
until critical biomass is achieved and population and composition is reached.
2. Environmental factors: There are several other factors that influence the growth and
activity of microorganisms which include pH, soil structure, nutrients, temperature,
moisture, redox potential, bioavailability of populations and oxygen content. The
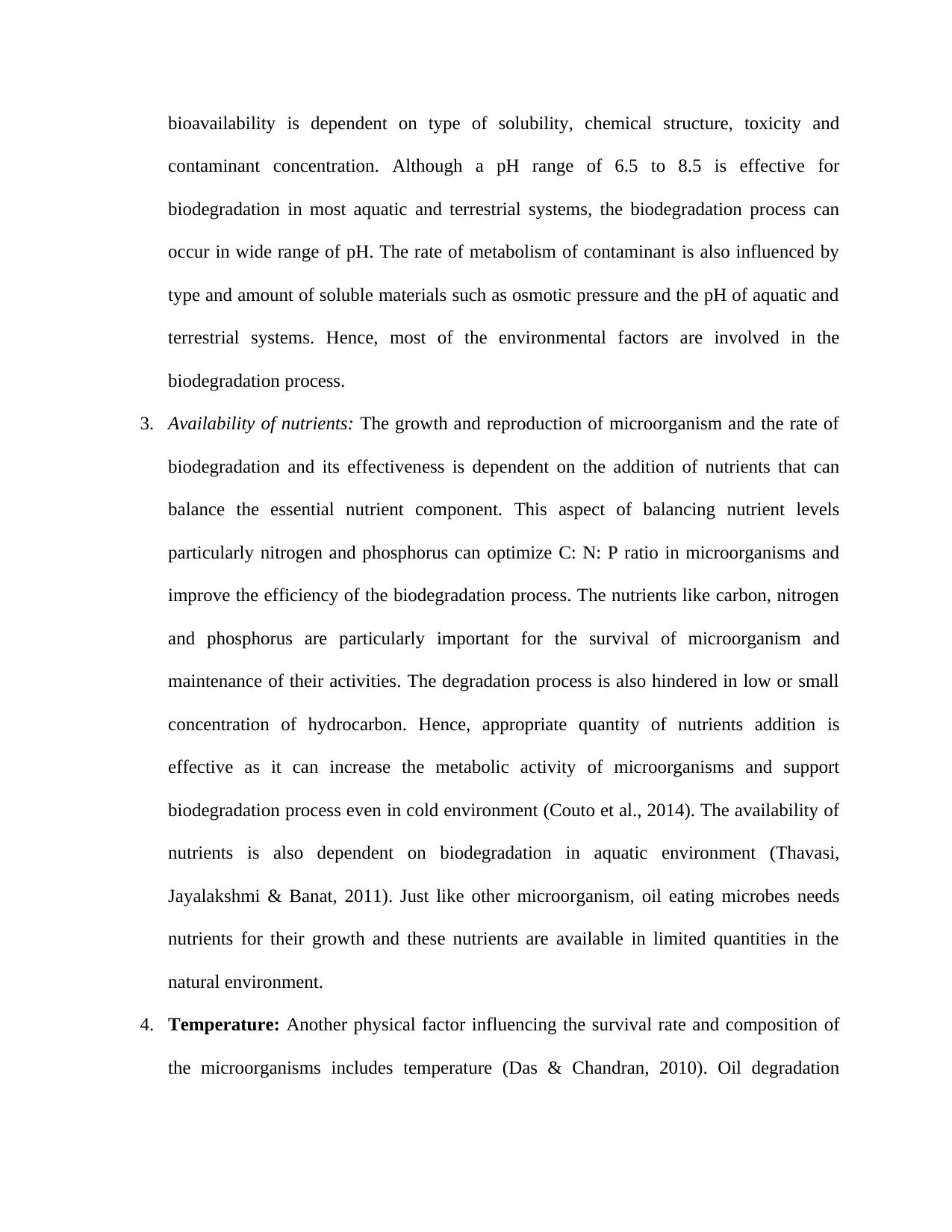
bioavailability is dependent on type of solubility, chemical structure, toxicity and
contaminant concentration. Although a pH range of 6.5 to 8.5 is effective for
biodegradation in most aquatic and terrestrial systems, the biodegradation process can
occur in wide range of pH. The rate of metabolism of contaminant is also influenced by
type and amount of soluble materials such as osmotic pressure and the pH of aquatic and
terrestrial systems. Hence, most of the environmental factors are involved in the
biodegradation process.
3. Availability of nutrients: The growth and reproduction of microorganism and the rate of
biodegradation and its effectiveness is dependent on the addition of nutrients that can
balance the essential nutrient component. This aspect of balancing nutrient levels
particularly nitrogen and phosphorus can optimize C: N: P ratio in microorganisms and
improve the efficiency of the biodegradation process. The nutrients like carbon, nitrogen
and phosphorus are particularly important for the survival of microorganism and
maintenance of their activities. The degradation process is also hindered in low or small
concentration of hydrocarbon. Hence, appropriate quantity of nutrients addition is
effective as it can increase the metabolic activity of microorganisms and support
biodegradation process even in cold environment (Couto et al., 2014). The availability of
nutrients is also dependent on biodegradation in aquatic environment (Thavasi,
Jayalakshmi & Banat, 2011). Just like other microorganism, oil eating microbes needs
nutrients for their growth and these nutrients are available in limited quantities in the
natural environment.
4. Temperature: Another physical factor influencing the survival rate and composition of
the microorganisms includes temperature (Das & Chandran, 2010). Oil degradation
contaminant concentration. Although a pH range of 6.5 to 8.5 is effective for
biodegradation in most aquatic and terrestrial systems, the biodegradation process can
occur in wide range of pH. The rate of metabolism of contaminant is also influenced by
type and amount of soluble materials such as osmotic pressure and the pH of aquatic and
terrestrial systems. Hence, most of the environmental factors are involved in the
biodegradation process.
3. Availability of nutrients: The growth and reproduction of microorganism and the rate of
biodegradation and its effectiveness is dependent on the addition of nutrients that can
balance the essential nutrient component. This aspect of balancing nutrient levels
particularly nitrogen and phosphorus can optimize C: N: P ratio in microorganisms and
improve the efficiency of the biodegradation process. The nutrients like carbon, nitrogen
and phosphorus are particularly important for the survival of microorganism and
maintenance of their activities. The degradation process is also hindered in low or small
concentration of hydrocarbon. Hence, appropriate quantity of nutrients addition is
effective as it can increase the metabolic activity of microorganisms and support
biodegradation process even in cold environment (Couto et al., 2014). The availability of
nutrients is also dependent on biodegradation in aquatic environment (Thavasi,
Jayalakshmi & Banat, 2011). Just like other microorganism, oil eating microbes needs
nutrients for their growth and these nutrients are available in limited quantities in the
natural environment.
4. Temperature: Another physical factor influencing the survival rate and composition of
the microorganisms includes temperature (Das & Chandran, 2010). Oil degradation
Paraphrase This Document
Need a fresh take? Get an instant paraphrase of this document with our AI Paraphraser
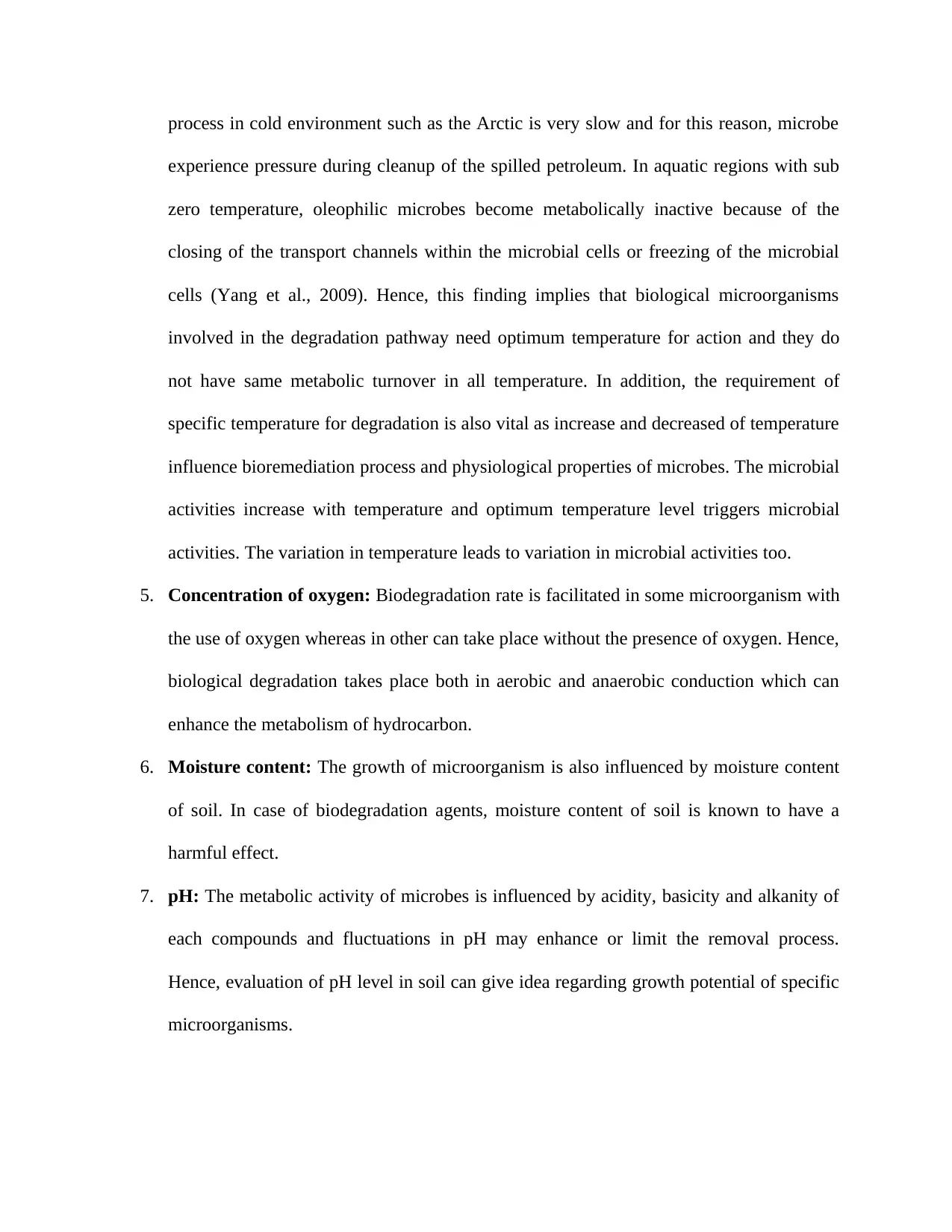
process in cold environment such as the Arctic is very slow and for this reason, microbe
experience pressure during cleanup of the spilled petroleum. In aquatic regions with sub
zero temperature, oleophilic microbes become metabolically inactive because of the
closing of the transport channels within the microbial cells or freezing of the microbial
cells (Yang et al., 2009). Hence, this finding implies that biological microorganisms
involved in the degradation pathway need optimum temperature for action and they do
not have same metabolic turnover in all temperature. In addition, the requirement of
specific temperature for degradation is also vital as increase and decreased of temperature
influence bioremediation process and physiological properties of microbes. The microbial
activities increase with temperature and optimum temperature level triggers microbial
activities. The variation in temperature leads to variation in microbial activities too.
5. Concentration of oxygen: Biodegradation rate is facilitated in some microorganism with
the use of oxygen whereas in other can take place without the presence of oxygen. Hence,
biological degradation takes place both in aerobic and anaerobic conduction which can
enhance the metabolism of hydrocarbon.
6. Moisture content: The growth of microorganism is also influenced by moisture content
of soil. In case of biodegradation agents, moisture content of soil is known to have a
harmful effect.
7. pH: The metabolic activity of microbes is influenced by acidity, basicity and alkanity of
each compounds and fluctuations in pH may enhance or limit the removal process.
Hence, evaluation of pH level in soil can give idea regarding growth potential of specific
microorganisms.
experience pressure during cleanup of the spilled petroleum. In aquatic regions with sub
zero temperature, oleophilic microbes become metabolically inactive because of the
closing of the transport channels within the microbial cells or freezing of the microbial
cells (Yang et al., 2009). Hence, this finding implies that biological microorganisms
involved in the degradation pathway need optimum temperature for action and they do
not have same metabolic turnover in all temperature. In addition, the requirement of
specific temperature for degradation is also vital as increase and decreased of temperature
influence bioremediation process and physiological properties of microbes. The microbial
activities increase with temperature and optimum temperature level triggers microbial
activities. The variation in temperature leads to variation in microbial activities too.
5. Concentration of oxygen: Biodegradation rate is facilitated in some microorganism with
the use of oxygen whereas in other can take place without the presence of oxygen. Hence,
biological degradation takes place both in aerobic and anaerobic conduction which can
enhance the metabolism of hydrocarbon.
6. Moisture content: The growth of microorganism is also influenced by moisture content
of soil. In case of biodegradation agents, moisture content of soil is known to have a
harmful effect.
7. pH: The metabolic activity of microbes is influenced by acidity, basicity and alkanity of
each compounds and fluctuations in pH may enhance or limit the removal process.
Hence, evaluation of pH level in soil can give idea regarding growth potential of specific
microorganisms.
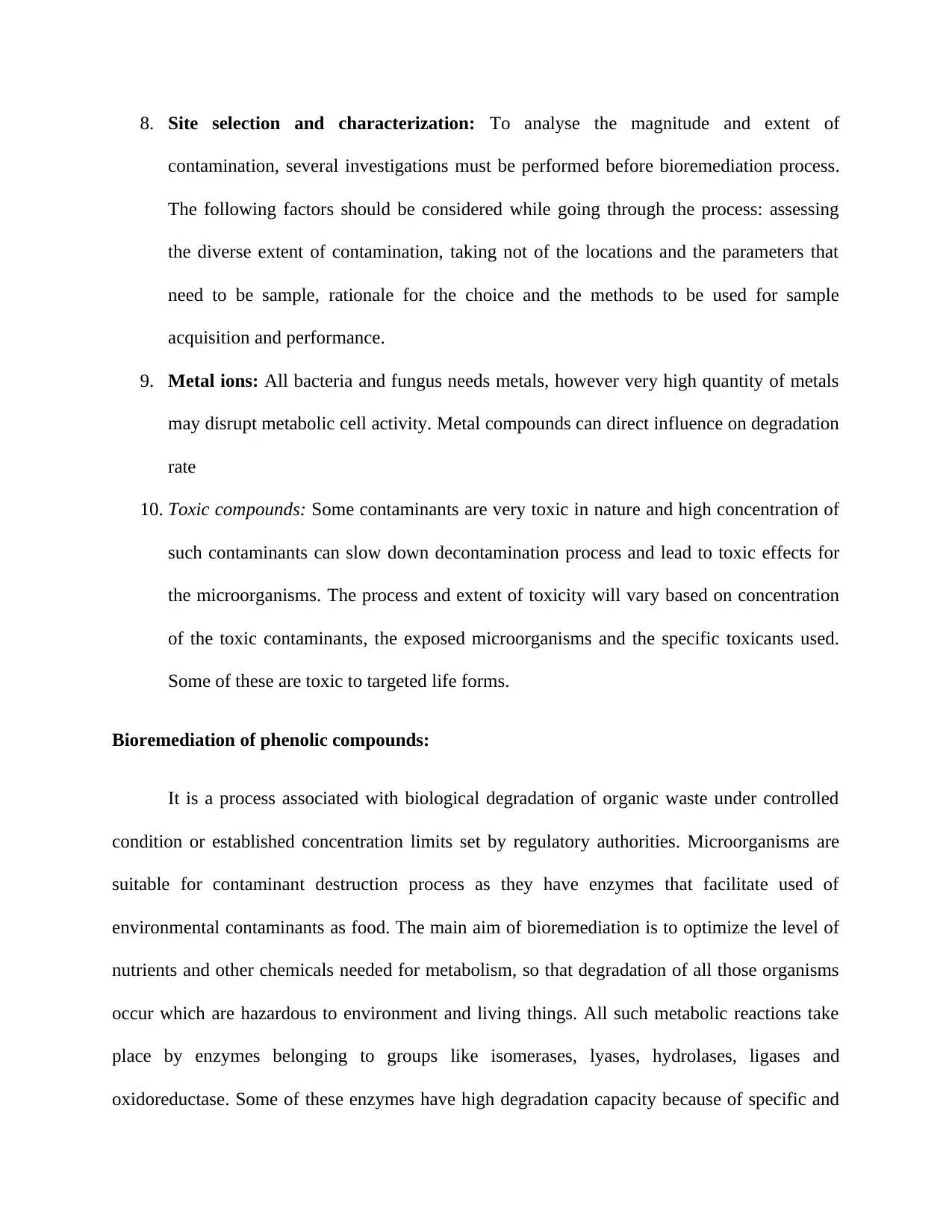
8. Site selection and characterization: To analyse the magnitude and extent of
contamination, several investigations must be performed before bioremediation process.
The following factors should be considered while going through the process: assessing
the diverse extent of contamination, taking not of the locations and the parameters that
need to be sample, rationale for the choice and the methods to be used for sample
acquisition and performance.
9. Metal ions: All bacteria and fungus needs metals, however very high quantity of metals
may disrupt metabolic cell activity. Metal compounds can direct influence on degradation
rate
10. Toxic compounds: Some contaminants are very toxic in nature and high concentration of
such contaminants can slow down decontamination process and lead to toxic effects for
the microorganisms. The process and extent of toxicity will vary based on concentration
of the toxic contaminants, the exposed microorganisms and the specific toxicants used.
Some of these are toxic to targeted life forms.
Bioremediation of phenolic compounds:
It is a process associated with biological degradation of organic waste under controlled
condition or established concentration limits set by regulatory authorities. Microorganisms are
suitable for contaminant destruction process as they have enzymes that facilitate used of
environmental contaminants as food. The main aim of bioremediation is to optimize the level of
nutrients and other chemicals needed for metabolism, so that degradation of all those organisms
occur which are hazardous to environment and living things. All such metabolic reactions take
place by enzymes belonging to groups like isomerases, lyases, hydrolases, ligases and
oxidoreductase. Some of these enzymes have high degradation capacity because of specific and
contamination, several investigations must be performed before bioremediation process.
The following factors should be considered while going through the process: assessing
the diverse extent of contamination, taking not of the locations and the parameters that
need to be sample, rationale for the choice and the methods to be used for sample
acquisition and performance.
9. Metal ions: All bacteria and fungus needs metals, however very high quantity of metals
may disrupt metabolic cell activity. Metal compounds can direct influence on degradation
rate
10. Toxic compounds: Some contaminants are very toxic in nature and high concentration of
such contaminants can slow down decontamination process and lead to toxic effects for
the microorganisms. The process and extent of toxicity will vary based on concentration
of the toxic contaminants, the exposed microorganisms and the specific toxicants used.
Some of these are toxic to targeted life forms.
Bioremediation of phenolic compounds:
It is a process associated with biological degradation of organic waste under controlled
condition or established concentration limits set by regulatory authorities. Microorganisms are
suitable for contaminant destruction process as they have enzymes that facilitate used of
environmental contaminants as food. The main aim of bioremediation is to optimize the level of
nutrients and other chemicals needed for metabolism, so that degradation of all those organisms
occur which are hazardous to environment and living things. All such metabolic reactions take
place by enzymes belonging to groups like isomerases, lyases, hydrolases, ligases and
oxidoreductase. Some of these enzymes have high degradation capacity because of specific and
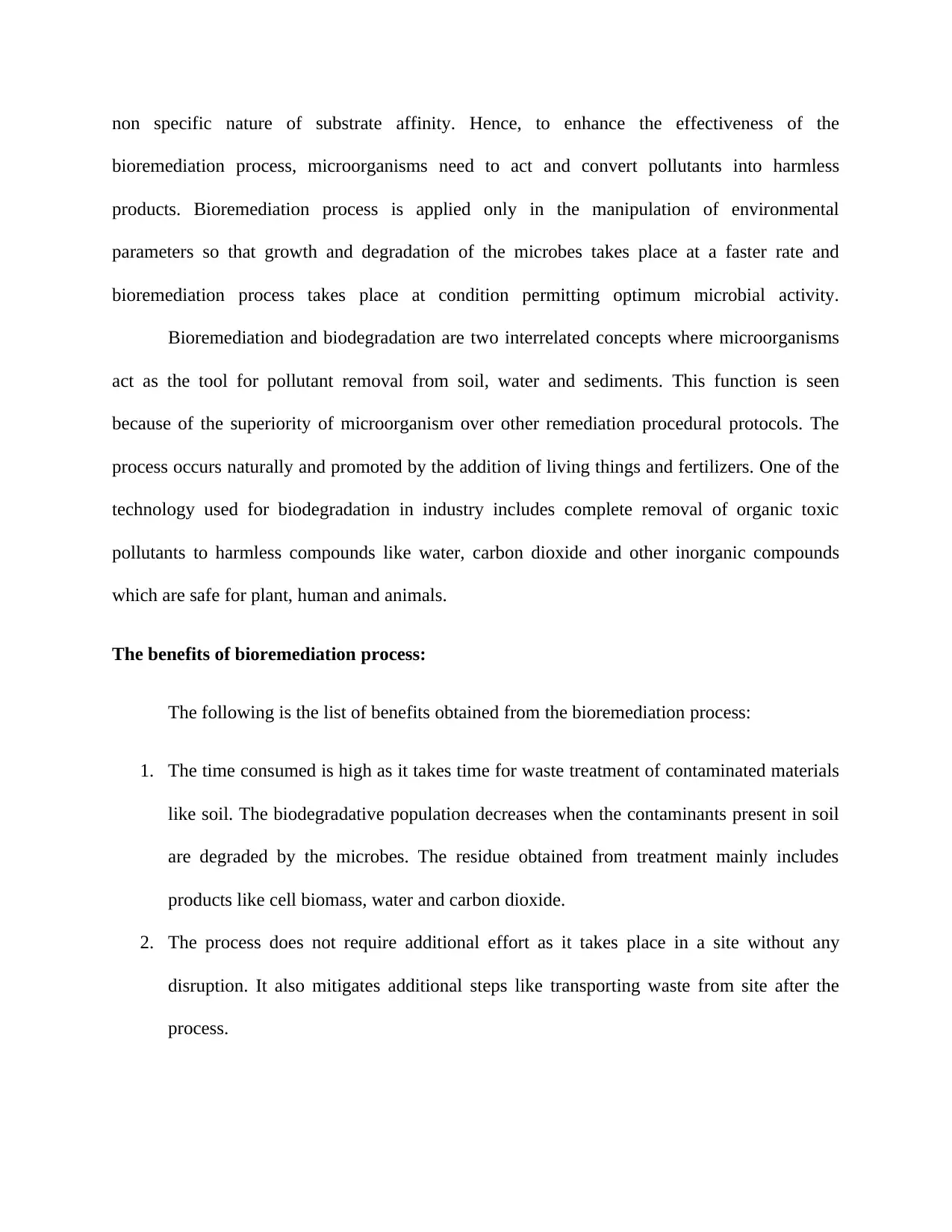
non specific nature of substrate affinity. Hence, to enhance the effectiveness of the
bioremediation process, microorganisms need to act and convert pollutants into harmless
products. Bioremediation process is applied only in the manipulation of environmental
parameters so that growth and degradation of the microbes takes place at a faster rate and
bioremediation process takes place at condition permitting optimum microbial activity.
Bioremediation and biodegradation are two interrelated concepts where microorganisms
act as the tool for pollutant removal from soil, water and sediments. This function is seen
because of the superiority of microorganism over other remediation procedural protocols. The
process occurs naturally and promoted by the addition of living things and fertilizers. One of the
technology used for biodegradation in industry includes complete removal of organic toxic
pollutants to harmless compounds like water, carbon dioxide and other inorganic compounds
which are safe for plant, human and animals.
The benefits of bioremediation process:
The following is the list of benefits obtained from the bioremediation process:
1. The time consumed is high as it takes time for waste treatment of contaminated materials
like soil. The biodegradative population decreases when the contaminants present in soil
are degraded by the microbes. The residue obtained from treatment mainly includes
products like cell biomass, water and carbon dioxide.
2. The process does not require additional effort as it takes place in a site without any
disruption. It also mitigates additional steps like transporting waste from site after the
process.
bioremediation process, microorganisms need to act and convert pollutants into harmless
products. Bioremediation process is applied only in the manipulation of environmental
parameters so that growth and degradation of the microbes takes place at a faster rate and
bioremediation process takes place at condition permitting optimum microbial activity.
Bioremediation and biodegradation are two interrelated concepts where microorganisms
act as the tool for pollutant removal from soil, water and sediments. This function is seen
because of the superiority of microorganism over other remediation procedural protocols. The
process occurs naturally and promoted by the addition of living things and fertilizers. One of the
technology used for biodegradation in industry includes complete removal of organic toxic
pollutants to harmless compounds like water, carbon dioxide and other inorganic compounds
which are safe for plant, human and animals.
The benefits of bioremediation process:
The following is the list of benefits obtained from the bioremediation process:
1. The time consumed is high as it takes time for waste treatment of contaminated materials
like soil. The biodegradative population decreases when the contaminants present in soil
are degraded by the microbes. The residue obtained from treatment mainly includes
products like cell biomass, water and carbon dioxide.
2. The process does not require additional effort as it takes place in a site without any
disruption. It also mitigates additional steps like transporting waste from site after the
process.
Secure Best Marks with AI Grader
Need help grading? Try our AI Grader for instant feedback on your assignments.
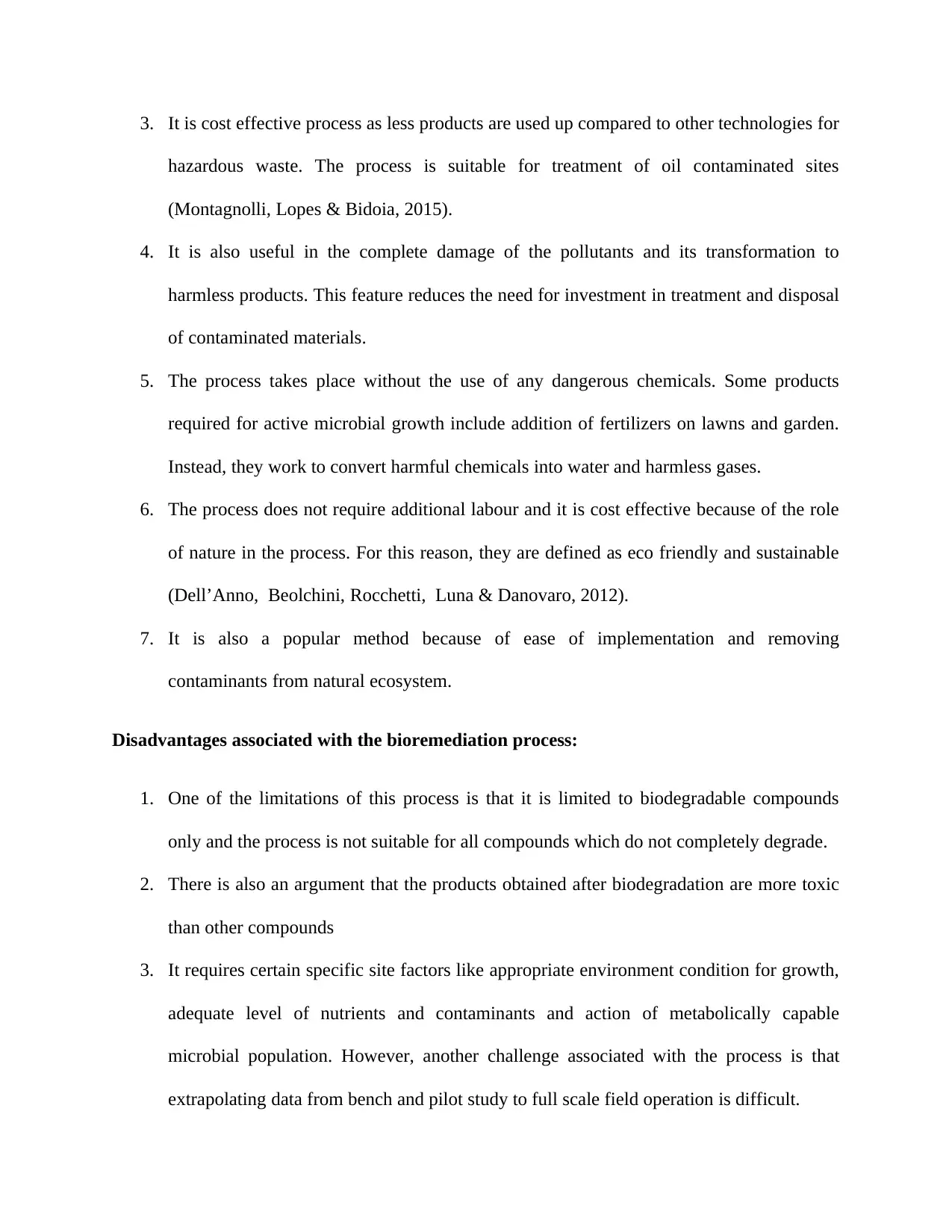
3. It is cost effective process as less products are used up compared to other technologies for
hazardous waste. The process is suitable for treatment of oil contaminated sites
(Montagnolli, Lopes & Bidoia, 2015).
4. It is also useful in the complete damage of the pollutants and its transformation to
harmless products. This feature reduces the need for investment in treatment and disposal
of contaminated materials.
5. The process takes place without the use of any dangerous chemicals. Some products
required for active microbial growth include addition of fertilizers on lawns and garden.
Instead, they work to convert harmful chemicals into water and harmless gases.
6. The process does not require additional labour and it is cost effective because of the role
of nature in the process. For this reason, they are defined as eco friendly and sustainable
(Dell’Anno, Beolchini, Rocchetti, Luna & Danovaro, 2012).
7. It is also a popular method because of ease of implementation and removing
contaminants from natural ecosystem.
Disadvantages associated with the bioremediation process:
1. One of the limitations of this process is that it is limited to biodegradable compounds
only and the process is not suitable for all compounds which do not completely degrade.
2. There is also an argument that the products obtained after biodegradation are more toxic
than other compounds
3. It requires certain specific site factors like appropriate environment condition for growth,
adequate level of nutrients and contaminants and action of metabolically capable
microbial population. However, another challenge associated with the process is that
extrapolating data from bench and pilot study to full scale field operation is difficult.
hazardous waste. The process is suitable for treatment of oil contaminated sites
(Montagnolli, Lopes & Bidoia, 2015).
4. It is also useful in the complete damage of the pollutants and its transformation to
harmless products. This feature reduces the need for investment in treatment and disposal
of contaminated materials.
5. The process takes place without the use of any dangerous chemicals. Some products
required for active microbial growth include addition of fertilizers on lawns and garden.
Instead, they work to convert harmful chemicals into water and harmless gases.
6. The process does not require additional labour and it is cost effective because of the role
of nature in the process. For this reason, they are defined as eco friendly and sustainable
(Dell’Anno, Beolchini, Rocchetti, Luna & Danovaro, 2012).
7. It is also a popular method because of ease of implementation and removing
contaminants from natural ecosystem.
Disadvantages associated with the bioremediation process:
1. One of the limitations of this process is that it is limited to biodegradable compounds
only and the process is not suitable for all compounds which do not completely degrade.
2. There is also an argument that the products obtained after biodegradation are more toxic
than other compounds
3. It requires certain specific site factors like appropriate environment condition for growth,
adequate level of nutrients and contaminants and action of metabolically capable
microbial population. However, another challenge associated with the process is that
extrapolating data from bench and pilot study to full scale field operation is difficult.
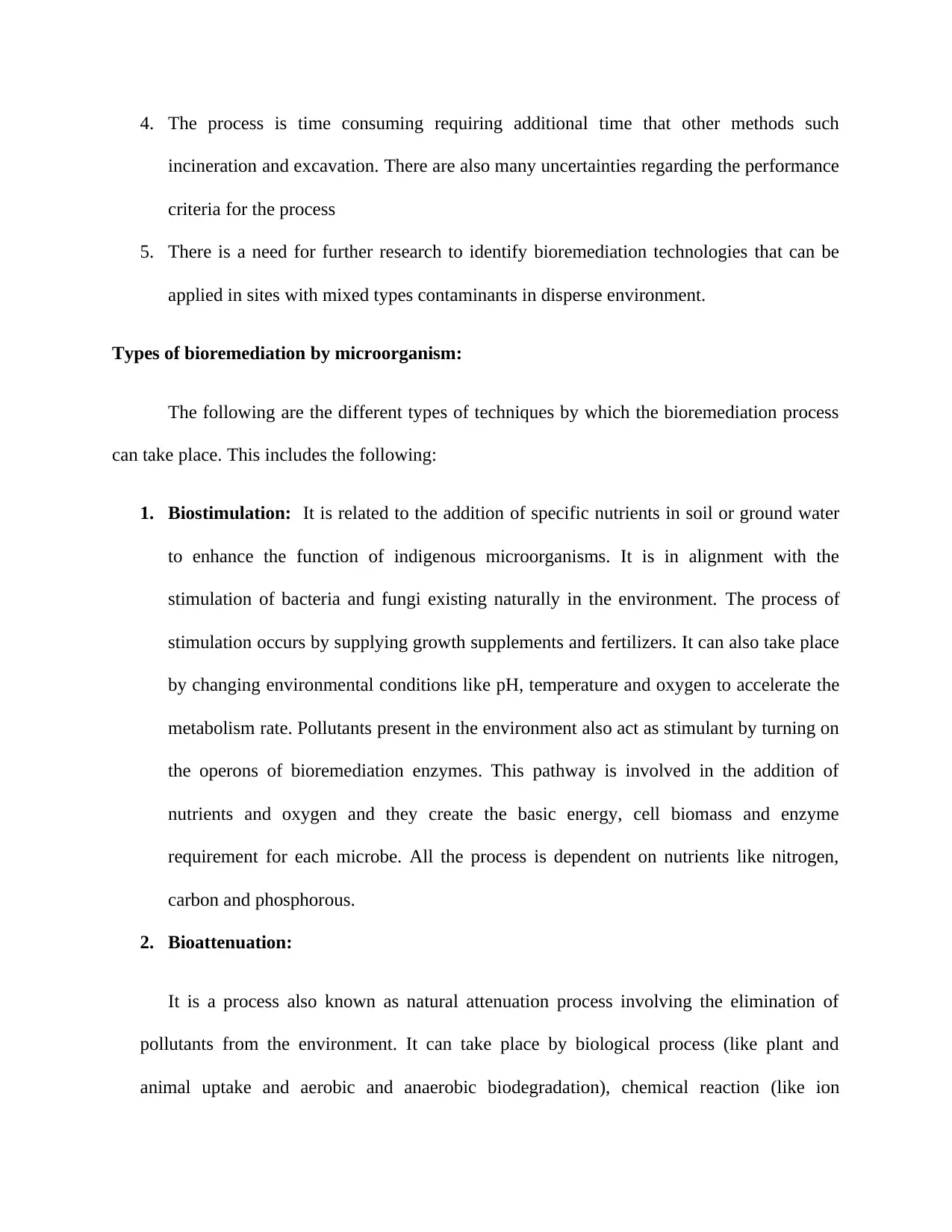
4. The process is time consuming requiring additional time that other methods such
incineration and excavation. There are also many uncertainties regarding the performance
criteria for the process
5. There is a need for further research to identify bioremediation technologies that can be
applied in sites with mixed types contaminants in disperse environment.
Types of bioremediation by microorganism:
The following are the different types of techniques by which the bioremediation process
can take place. This includes the following:
1. Biostimulation: It is related to the addition of specific nutrients in soil or ground water
to enhance the function of indigenous microorganisms. It is in alignment with the
stimulation of bacteria and fungi existing naturally in the environment. The process of
stimulation occurs by supplying growth supplements and fertilizers. It can also take place
by changing environmental conditions like pH, temperature and oxygen to accelerate the
metabolism rate. Pollutants present in the environment also act as stimulant by turning on
the operons of bioremediation enzymes. This pathway is involved in the addition of
nutrients and oxygen and they create the basic energy, cell biomass and enzyme
requirement for each microbe. All the process is dependent on nutrients like nitrogen,
carbon and phosphorous.
2. Bioattenuation:
It is a process also known as natural attenuation process involving the elimination of
pollutants from the environment. It can take place by biological process (like plant and
animal uptake and aerobic and anaerobic biodegradation), chemical reaction (like ion
incineration and excavation. There are also many uncertainties regarding the performance
criteria for the process
5. There is a need for further research to identify bioremediation technologies that can be
applied in sites with mixed types contaminants in disperse environment.
Types of bioremediation by microorganism:
The following are the different types of techniques by which the bioremediation process
can take place. This includes the following:
1. Biostimulation: It is related to the addition of specific nutrients in soil or ground water
to enhance the function of indigenous microorganisms. It is in alignment with the
stimulation of bacteria and fungi existing naturally in the environment. The process of
stimulation occurs by supplying growth supplements and fertilizers. It can also take place
by changing environmental conditions like pH, temperature and oxygen to accelerate the
metabolism rate. Pollutants present in the environment also act as stimulant by turning on
the operons of bioremediation enzymes. This pathway is involved in the addition of
nutrients and oxygen and they create the basic energy, cell biomass and enzyme
requirement for each microbe. All the process is dependent on nutrients like nitrogen,
carbon and phosphorous.
2. Bioattenuation:
It is a process also known as natural attenuation process involving the elimination of
pollutants from the environment. It can take place by biological process (like plant and
animal uptake and aerobic and anaerobic biodegradation), chemical reaction (like ion
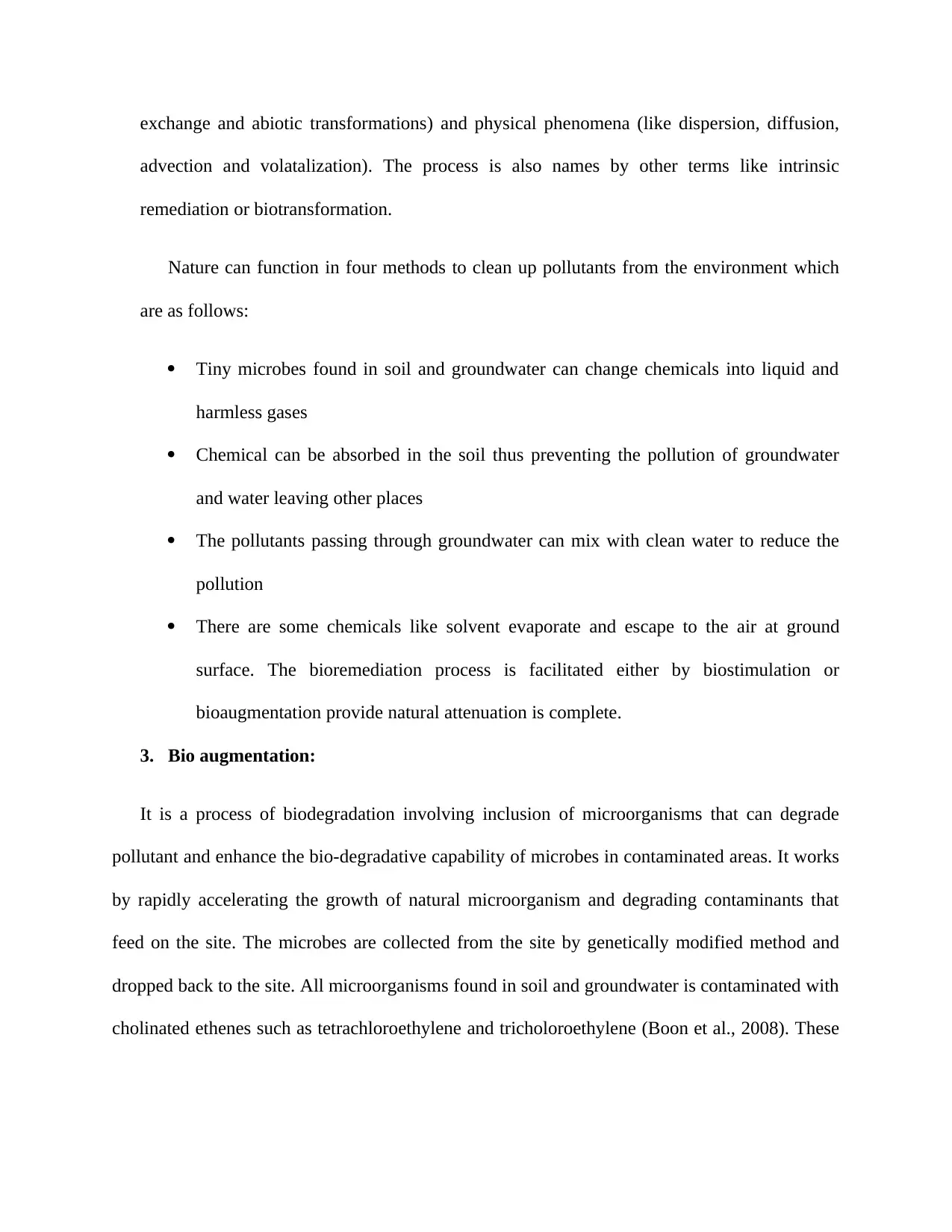
exchange and abiotic transformations) and physical phenomena (like dispersion, diffusion,
advection and volatalization). The process is also names by other terms like intrinsic
remediation or biotransformation.
Nature can function in four methods to clean up pollutants from the environment which
are as follows:
Tiny microbes found in soil and groundwater can change chemicals into liquid and
harmless gases
Chemical can be absorbed in the soil thus preventing the pollution of groundwater
and water leaving other places
The pollutants passing through groundwater can mix with clean water to reduce the
pollution
There are some chemicals like solvent evaporate and escape to the air at ground
surface. The bioremediation process is facilitated either by biostimulation or
bioaugmentation provide natural attenuation is complete.
3. Bio augmentation:
It is a process of biodegradation involving inclusion of microorganisms that can degrade
pollutant and enhance the bio-degradative capability of microbes in contaminated areas. It works
by rapidly accelerating the growth of natural microorganism and degrading contaminants that
feed on the site. The microbes are collected from the site by genetically modified method and
dropped back to the site. All microorganisms found in soil and groundwater is contaminated with
cholinated ethenes such as tetrachloroethylene and tricholoroethylene (Boon et al., 2008). These
advection and volatalization). The process is also names by other terms like intrinsic
remediation or biotransformation.
Nature can function in four methods to clean up pollutants from the environment which
are as follows:
Tiny microbes found in soil and groundwater can change chemicals into liquid and
harmless gases
Chemical can be absorbed in the soil thus preventing the pollution of groundwater
and water leaving other places
The pollutants passing through groundwater can mix with clean water to reduce the
pollution
There are some chemicals like solvent evaporate and escape to the air at ground
surface. The bioremediation process is facilitated either by biostimulation or
bioaugmentation provide natural attenuation is complete.
3. Bio augmentation:
It is a process of biodegradation involving inclusion of microorganisms that can degrade
pollutant and enhance the bio-degradative capability of microbes in contaminated areas. It works
by rapidly accelerating the growth of natural microorganism and degrading contaminants that
feed on the site. The microbes are collected from the site by genetically modified method and
dropped back to the site. All microorganisms found in soil and groundwater is contaminated with
cholinated ethenes such as tetrachloroethylene and tricholoroethylene (Boon et al., 2008). These
Paraphrase This Document
Need a fresh take? Get an instant paraphrase of this document with our AI Paraphraser
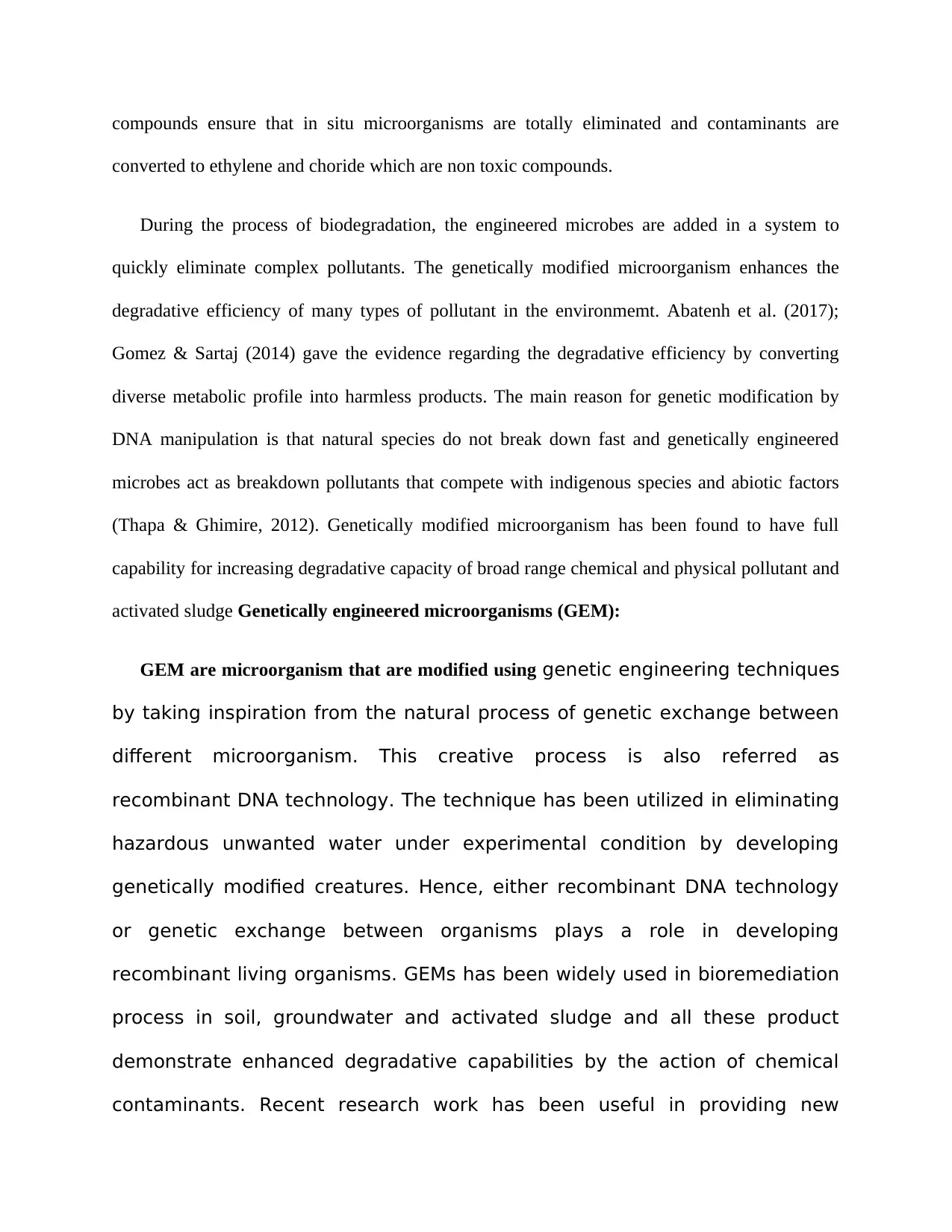
compounds ensure that in situ microorganisms are totally eliminated and contaminants are
converted to ethylene and choride which are non toxic compounds.
During the process of biodegradation, the engineered microbes are added in a system to
quickly eliminate complex pollutants. The genetically modified microorganism enhances the
degradative efficiency of many types of pollutant in the environmemt. Abatenh et al. (2017);
Gomez & Sartaj (2014) gave the evidence regarding the degradative efficiency by converting
diverse metabolic profile into harmless products. The main reason for genetic modification by
DNA manipulation is that natural species do not break down fast and genetically engineered
microbes act as breakdown pollutants that compete with indigenous species and abiotic factors
(Thapa & Ghimire, 2012). Genetically modified microorganism has been found to have full
capability for increasing degradative capacity of broad range chemical and physical pollutant and
activated sludge Genetically engineered microorganisms (GEM):
GEM are microorganism that are modified using genetic engineering techniques
by taking inspiration from the natural process of genetic exchange between
different microorganism. This creative process is also referred as
recombinant DNA technology. The technique has been utilized in eliminating
hazardous unwanted water under experimental condition by developing
genetically modified creatures. Hence, either recombinant DNA technology
or genetic exchange between organisms plays a role in developing
recombinant living organisms. GEMs has been widely used in bioremediation
process in soil, groundwater and activated sludge and all these product
demonstrate enhanced degradative capabilities by the action of chemical
contaminants. Recent research work has been useful in providing new
converted to ethylene and choride which are non toxic compounds.
During the process of biodegradation, the engineered microbes are added in a system to
quickly eliminate complex pollutants. The genetically modified microorganism enhances the
degradative efficiency of many types of pollutant in the environmemt. Abatenh et al. (2017);
Gomez & Sartaj (2014) gave the evidence regarding the degradative efficiency by converting
diverse metabolic profile into harmless products. The main reason for genetic modification by
DNA manipulation is that natural species do not break down fast and genetically engineered
microbes act as breakdown pollutants that compete with indigenous species and abiotic factors
(Thapa & Ghimire, 2012). Genetically modified microorganism has been found to have full
capability for increasing degradative capacity of broad range chemical and physical pollutant and
activated sludge Genetically engineered microorganisms (GEM):
GEM are microorganism that are modified using genetic engineering techniques
by taking inspiration from the natural process of genetic exchange between
different microorganism. This creative process is also referred as
recombinant DNA technology. The technique has been utilized in eliminating
hazardous unwanted water under experimental condition by developing
genetically modified creatures. Hence, either recombinant DNA technology
or genetic exchange between organisms plays a role in developing
recombinant living organisms. GEMs has been widely used in bioremediation
process in soil, groundwater and activated sludge and all these product
demonstrate enhanced degradative capabilities by the action of chemical
contaminants. Recent research work has been useful in providing new
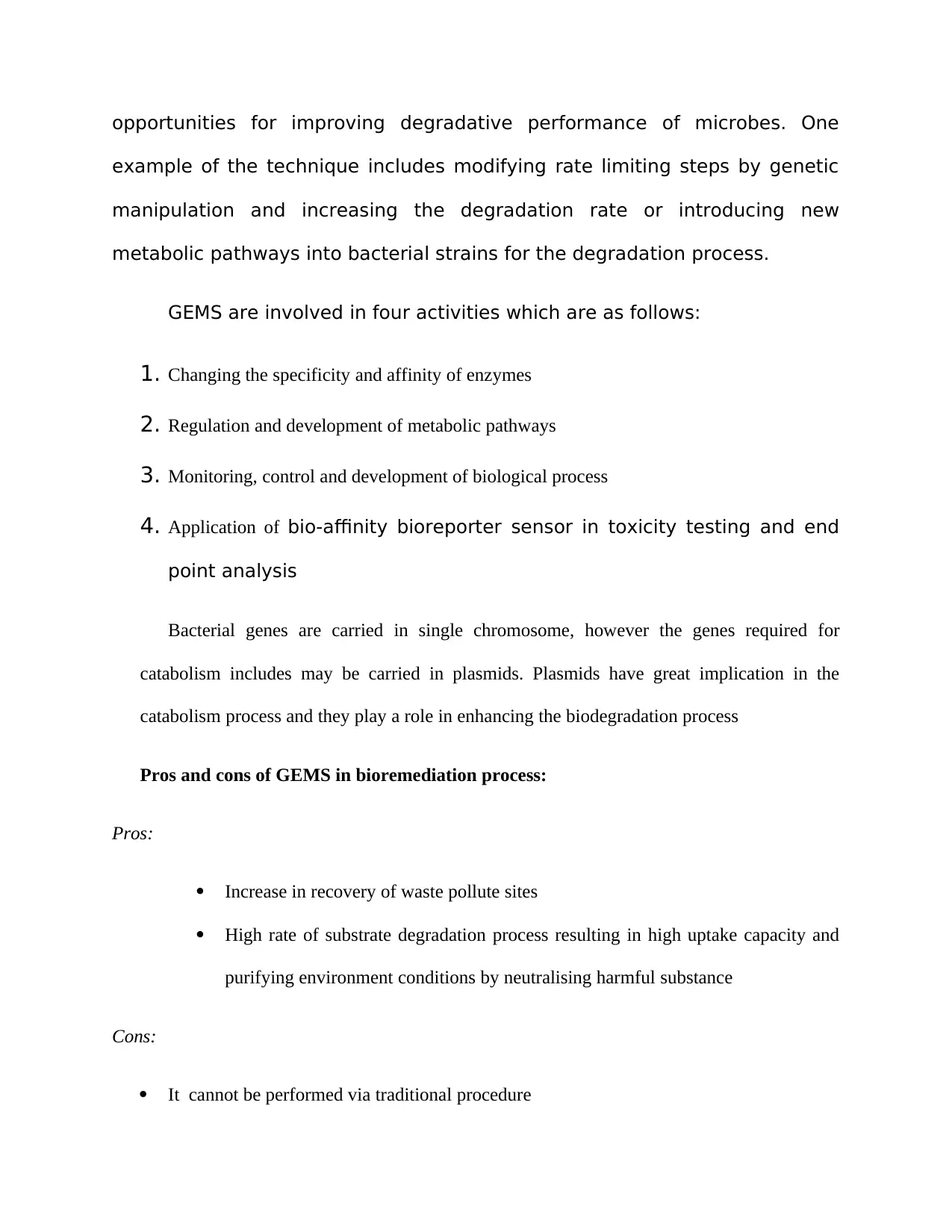
opportunities for improving degradative performance of microbes. One
example of the technique includes modifying rate limiting steps by genetic
manipulation and increasing the degradation rate or introducing new
metabolic pathways into bacterial strains for the degradation process.
GEMS are involved in four activities which are as follows:
1. Changing the specificity and affinity of enzymes
2. Regulation and development of metabolic pathways
3. Monitoring, control and development of biological process
4. Application of bio-affinity bioreporter sensor in toxicity testing and end
point analysis
Bacterial genes are carried in single chromosome, however the genes required for
catabolism includes may be carried in plasmids. Plasmids have great implication in the
catabolism process and they play a role in enhancing the biodegradation process
Pros and cons of GEMS in bioremediation process:
Pros:
Increase in recovery of waste pollute sites
High rate of substrate degradation process resulting in high uptake capacity and
purifying environment conditions by neutralising harmful substance
Cons:
It cannot be performed via traditional procedure
example of the technique includes modifying rate limiting steps by genetic
manipulation and increasing the degradation rate or introducing new
metabolic pathways into bacterial strains for the degradation process.
GEMS are involved in four activities which are as follows:
1. Changing the specificity and affinity of enzymes
2. Regulation and development of metabolic pathways
3. Monitoring, control and development of biological process
4. Application of bio-affinity bioreporter sensor in toxicity testing and end
point analysis
Bacterial genes are carried in single chromosome, however the genes required for
catabolism includes may be carried in plasmids. Plasmids have great implication in the
catabolism process and they play a role in enhancing the biodegradation process
Pros and cons of GEMS in bioremediation process:
Pros:
Increase in recovery of waste pollute sites
High rate of substrate degradation process resulting in high uptake capacity and
purifying environment conditions by neutralising harmful substance
Cons:
It cannot be performed via traditional procedure
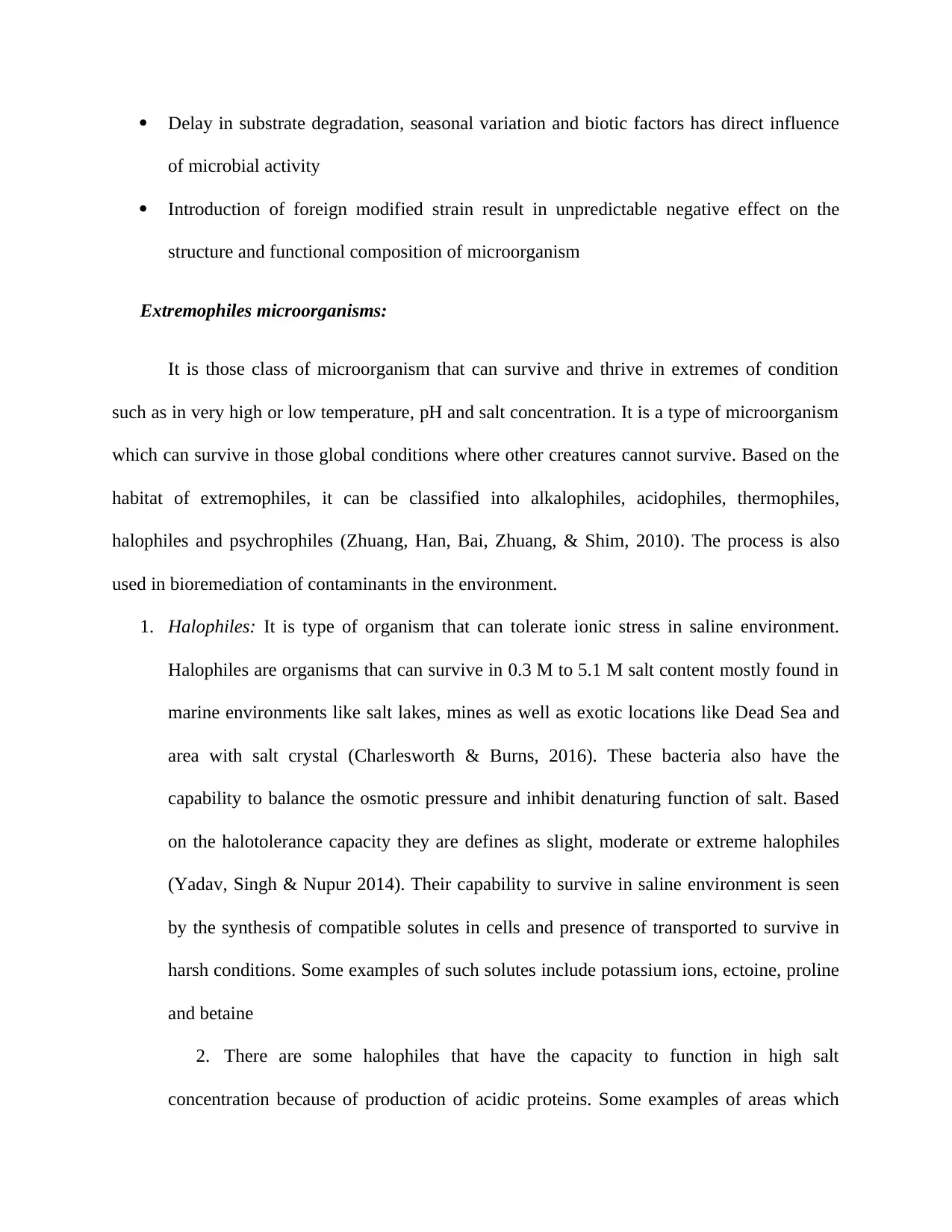
Delay in substrate degradation, seasonal variation and biotic factors has direct influence
of microbial activity
Introduction of foreign modified strain result in unpredictable negative effect on the
structure and functional composition of microorganism
Extremophiles microorganisms:
It is those class of microorganism that can survive and thrive in extremes of condition
such as in very high or low temperature, pH and salt concentration. It is a type of microorganism
which can survive in those global conditions where other creatures cannot survive. Based on the
habitat of extremophiles, it can be classified into alkalophiles, acidophiles, thermophiles,
halophiles and psychrophiles (Zhuang, Han, Bai, Zhuang, & Shim, 2010). The process is also
used in bioremediation of contaminants in the environment.
1. Halophiles: It is type of organism that can tolerate ionic stress in saline environment.
Halophiles are organisms that can survive in 0.3 M to 5.1 M salt content mostly found in
marine environments like salt lakes, mines as well as exotic locations like Dead Sea and
area with salt crystal (Charlesworth & Burns, 2016). These bacteria also have the
capability to balance the osmotic pressure and inhibit denaturing function of salt. Based
on the halotolerance capacity they are defines as slight, moderate or extreme halophiles
(Yadav, Singh & Nupur 2014). Their capability to survive in saline environment is seen
by the synthesis of compatible solutes in cells and presence of transported to survive in
harsh conditions. Some examples of such solutes include potassium ions, ectoine, proline
and betaine
2. There are some halophiles that have the capacity to function in high salt
concentration because of production of acidic proteins. Some examples of areas which
of microbial activity
Introduction of foreign modified strain result in unpredictable negative effect on the
structure and functional composition of microorganism
Extremophiles microorganisms:
It is those class of microorganism that can survive and thrive in extremes of condition
such as in very high or low temperature, pH and salt concentration. It is a type of microorganism
which can survive in those global conditions where other creatures cannot survive. Based on the
habitat of extremophiles, it can be classified into alkalophiles, acidophiles, thermophiles,
halophiles and psychrophiles (Zhuang, Han, Bai, Zhuang, & Shim, 2010). The process is also
used in bioremediation of contaminants in the environment.
1. Halophiles: It is type of organism that can tolerate ionic stress in saline environment.
Halophiles are organisms that can survive in 0.3 M to 5.1 M salt content mostly found in
marine environments like salt lakes, mines as well as exotic locations like Dead Sea and
area with salt crystal (Charlesworth & Burns, 2016). These bacteria also have the
capability to balance the osmotic pressure and inhibit denaturing function of salt. Based
on the halotolerance capacity they are defines as slight, moderate or extreme halophiles
(Yadav, Singh & Nupur 2014). Their capability to survive in saline environment is seen
by the synthesis of compatible solutes in cells and presence of transported to survive in
harsh conditions. Some examples of such solutes include potassium ions, ectoine, proline
and betaine
2. There are some halophiles that have the capacity to function in high salt
concentration because of production of acidic proteins. Some examples of areas which
Secure Best Marks with AI Grader
Need help grading? Try our AI Grader for instant feedback on your assignments.
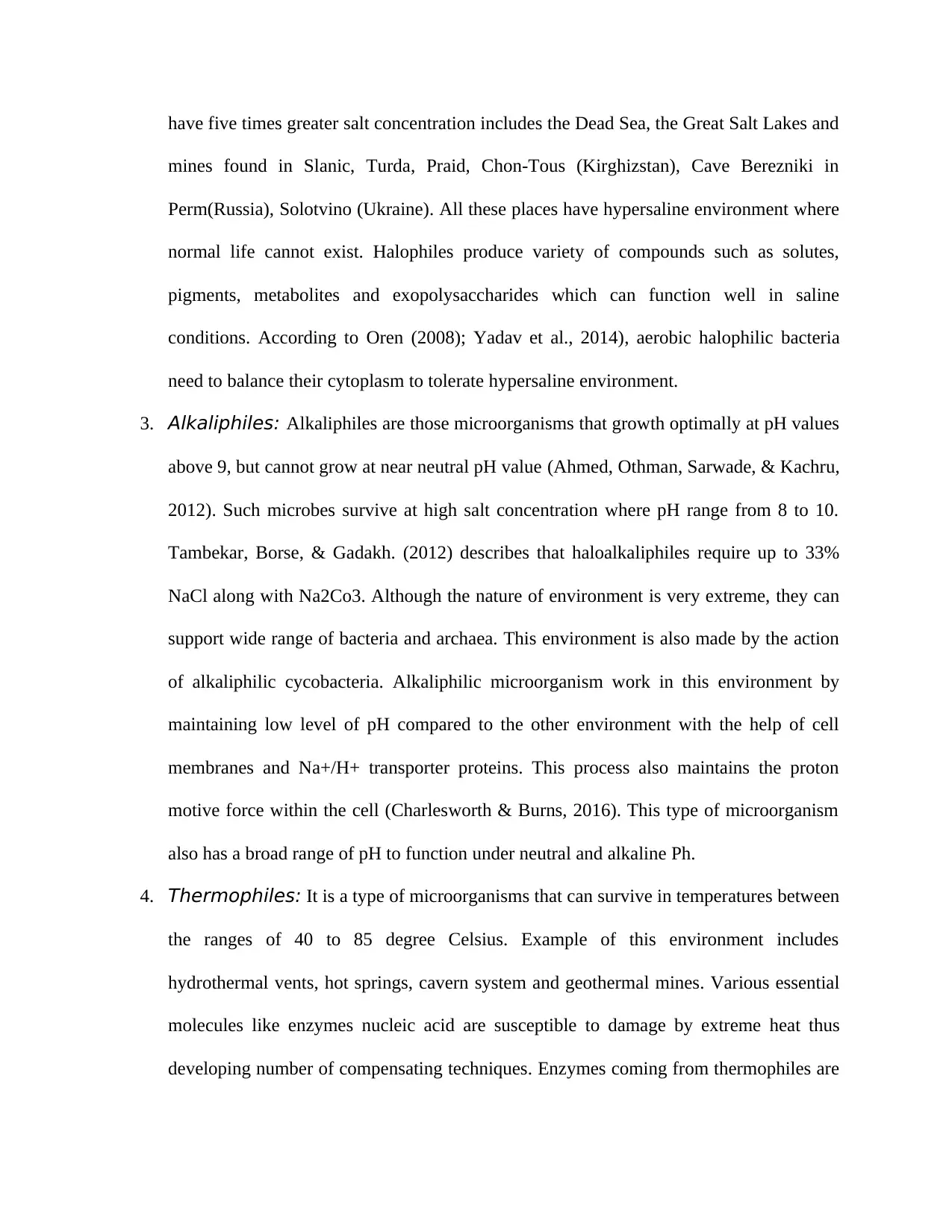
have five times greater salt concentration includes the Dead Sea, the Great Salt Lakes and
mines found in Slanic, Turda, Praid, Chon-Tous (Kirghizstan), Cave Berezniki in
Perm(Russia), Solotvino (Ukraine). All these places have hypersaline environment where
normal life cannot exist. Halophiles produce variety of compounds such as solutes,
pigments, metabolites and exopolysaccharides which can function well in saline
conditions. According to Oren (2008); Yadav et al., 2014), aerobic halophilic bacteria
need to balance their cytoplasm to tolerate hypersaline environment.
3. Alkaliphiles: Alkaliphiles are those microorganisms that growth optimally at pH values
above 9, but cannot grow at near neutral pH value (Ahmed, Othman, Sarwade, & Kachru,
2012). Such microbes survive at high salt concentration where pH range from 8 to 10.
Tambekar, Borse, & Gadakh. (2012) describes that haloalkaliphiles require up to 33%
NaCl along with Na2Co3. Although the nature of environment is very extreme, they can
support wide range of bacteria and archaea. This environment is also made by the action
of alkaliphilic cycobacteria. Alkaliphilic microorganism work in this environment by
maintaining low level of pH compared to the other environment with the help of cell
membranes and Na+/H+ transporter proteins. This process also maintains the proton
motive force within the cell (Charlesworth & Burns, 2016). This type of microorganism
also has a broad range of pH to function under neutral and alkaline Ph.
4. Thermophiles: It is a type of microorganisms that can survive in temperatures between
the ranges of 40 to 85 degree Celsius. Example of this environment includes
hydrothermal vents, hot springs, cavern system and geothermal mines. Various essential
molecules like enzymes nucleic acid are susceptible to damage by extreme heat thus
developing number of compensating techniques. Enzymes coming from thermophiles are
mines found in Slanic, Turda, Praid, Chon-Tous (Kirghizstan), Cave Berezniki in
Perm(Russia), Solotvino (Ukraine). All these places have hypersaline environment where
normal life cannot exist. Halophiles produce variety of compounds such as solutes,
pigments, metabolites and exopolysaccharides which can function well in saline
conditions. According to Oren (2008); Yadav et al., 2014), aerobic halophilic bacteria
need to balance their cytoplasm to tolerate hypersaline environment.
3. Alkaliphiles: Alkaliphiles are those microorganisms that growth optimally at pH values
above 9, but cannot grow at near neutral pH value (Ahmed, Othman, Sarwade, & Kachru,
2012). Such microbes survive at high salt concentration where pH range from 8 to 10.
Tambekar, Borse, & Gadakh. (2012) describes that haloalkaliphiles require up to 33%
NaCl along with Na2Co3. Although the nature of environment is very extreme, they can
support wide range of bacteria and archaea. This environment is also made by the action
of alkaliphilic cycobacteria. Alkaliphilic microorganism work in this environment by
maintaining low level of pH compared to the other environment with the help of cell
membranes and Na+/H+ transporter proteins. This process also maintains the proton
motive force within the cell (Charlesworth & Burns, 2016). This type of microorganism
also has a broad range of pH to function under neutral and alkaline Ph.
4. Thermophiles: It is a type of microorganisms that can survive in temperatures between
the ranges of 40 to 85 degree Celsius. Example of this environment includes
hydrothermal vents, hot springs, cavern system and geothermal mines. Various essential
molecules like enzymes nucleic acid are susceptible to damage by extreme heat thus
developing number of compensating techniques. Enzymes coming from thermophiles are
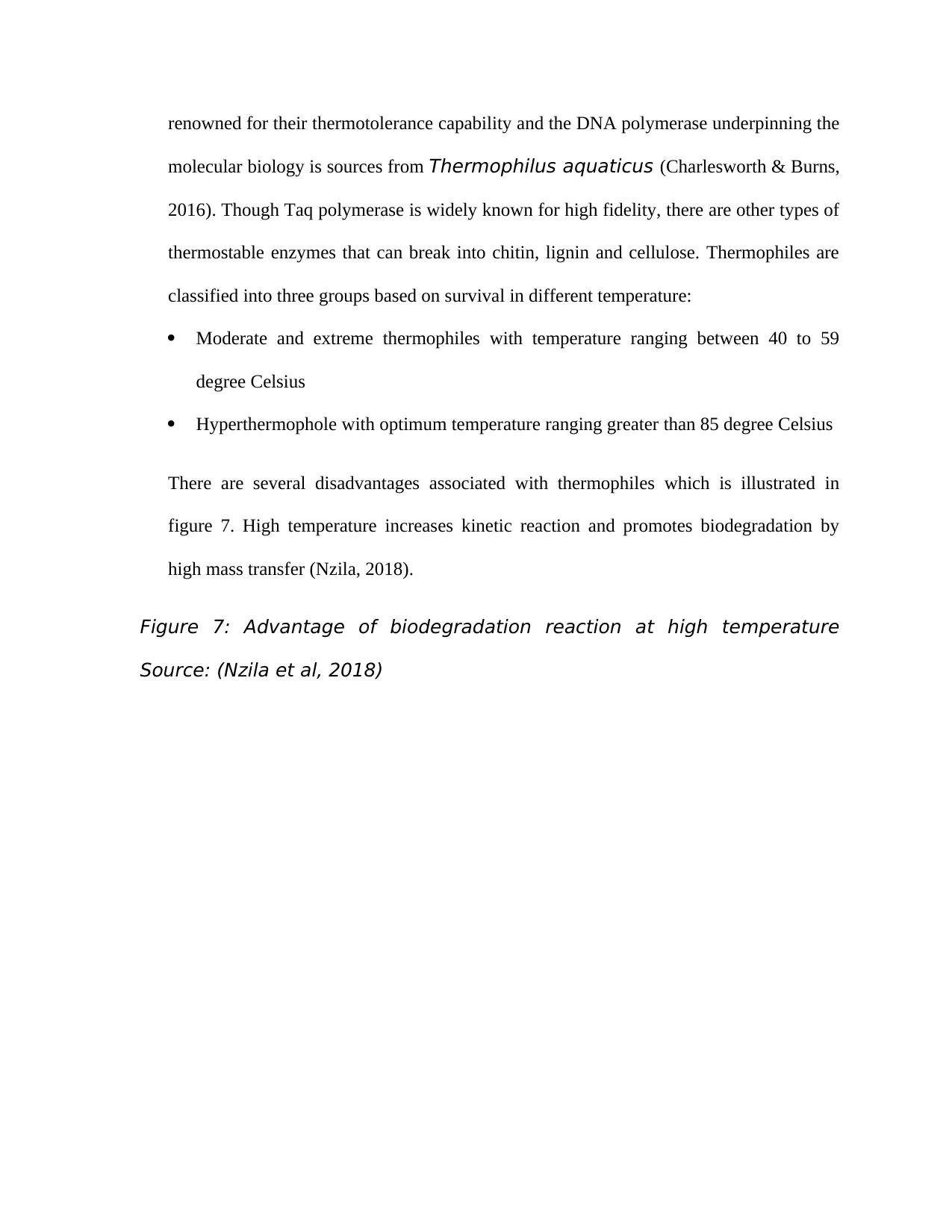
renowned for their thermotolerance capability and the DNA polymerase underpinning the
molecular biology is sources from Thermophilus aquaticus (Charlesworth & Burns,
2016). Though Taq polymerase is widely known for high fidelity, there are other types of
thermostable enzymes that can break into chitin, lignin and cellulose. Thermophiles are
classified into three groups based on survival in different temperature:
Moderate and extreme thermophiles with temperature ranging between 40 to 59
degree Celsius
Hyperthermophole with optimum temperature ranging greater than 85 degree Celsius
There are several disadvantages associated with thermophiles which is illustrated in
figure 7. High temperature increases kinetic reaction and promotes biodegradation by
high mass transfer (Nzila, 2018).
Figure 7: Advantage of biodegradation reaction at high temperature
Source: (Nzila et al, 2018)
molecular biology is sources from Thermophilus aquaticus (Charlesworth & Burns,
2016). Though Taq polymerase is widely known for high fidelity, there are other types of
thermostable enzymes that can break into chitin, lignin and cellulose. Thermophiles are
classified into three groups based on survival in different temperature:
Moderate and extreme thermophiles with temperature ranging between 40 to 59
degree Celsius
Hyperthermophole with optimum temperature ranging greater than 85 degree Celsius
There are several disadvantages associated with thermophiles which is illustrated in
figure 7. High temperature increases kinetic reaction and promotes biodegradation by
high mass transfer (Nzila, 2018).
Figure 7: Advantage of biodegradation reaction at high temperature
Source: (Nzila et al, 2018)
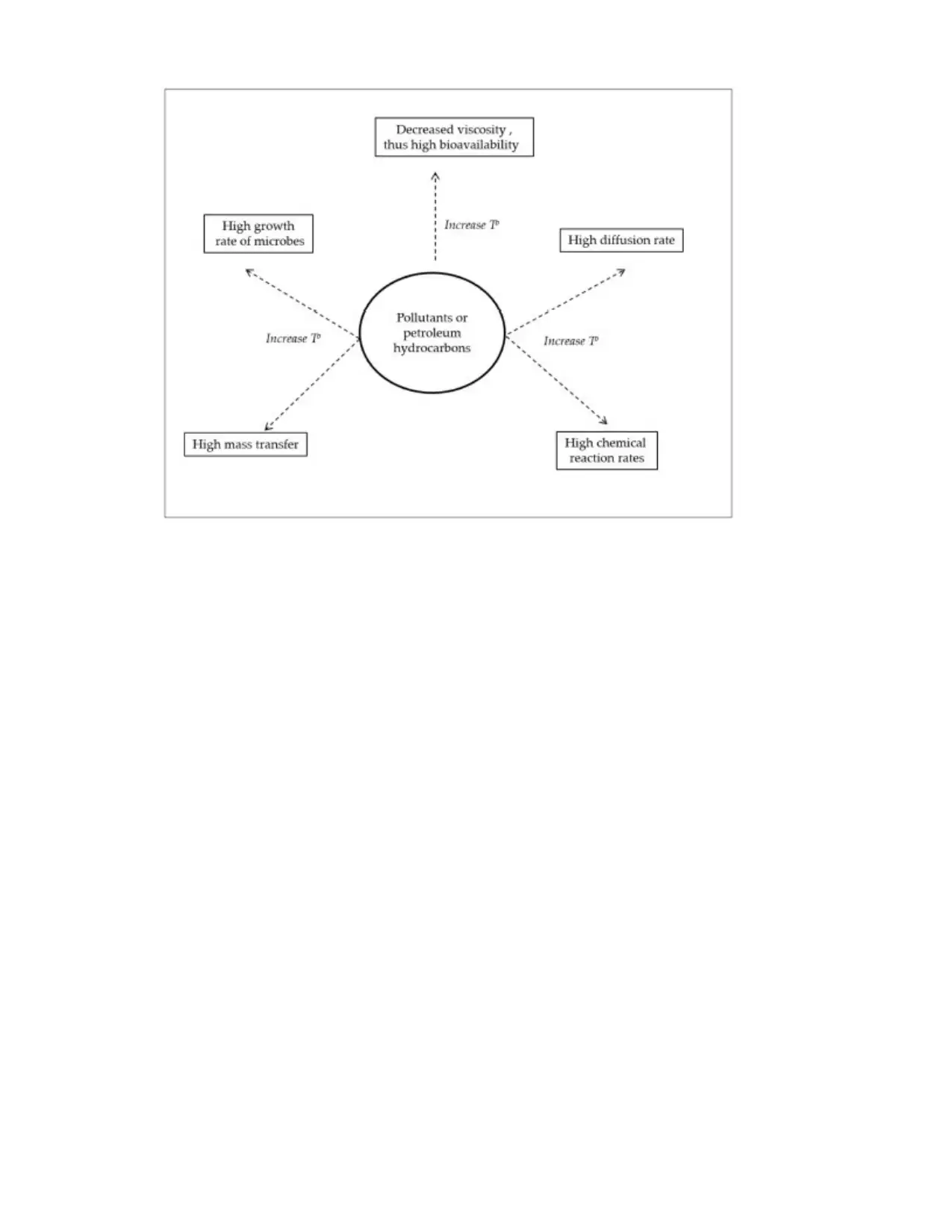
Paraphrase This Document
Need a fresh take? Get an instant paraphrase of this document with our AI Paraphraser
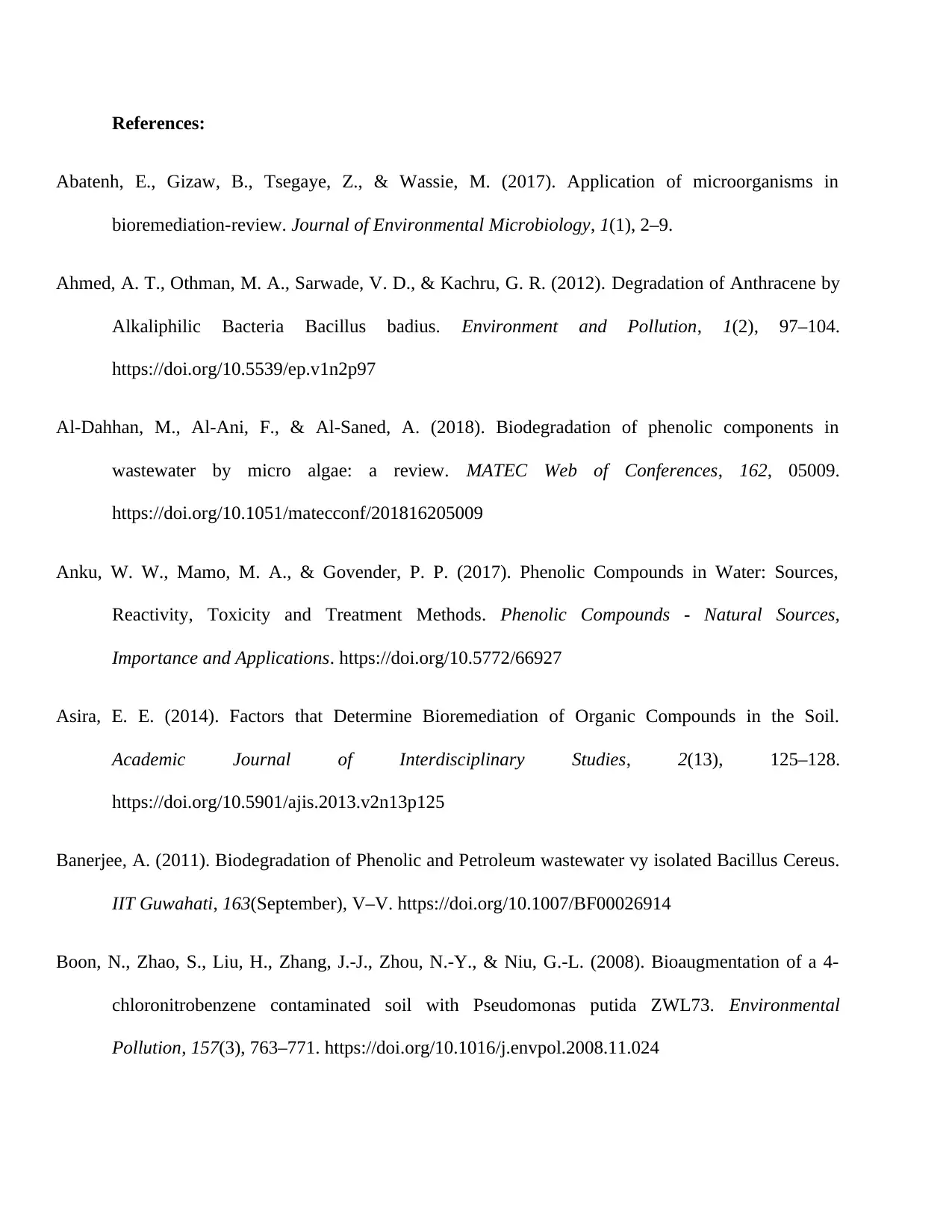
References:
Abatenh, E., Gizaw, B., Tsegaye, Z., & Wassie, M. (2017). Application of microorganisms in
bioremediation-review. Journal of Environmental Microbiology, 1(1), 2–9.
Ahmed, A. T., Othman, M. A., Sarwade, V. D., & Kachru, G. R. (2012). Degradation of Anthracene by
Alkaliphilic Bacteria Bacillus badius. Environment and Pollution, 1(2), 97–104.
https://doi.org/10.5539/ep.v1n2p97
Al-Dahhan, M., Al-Ani, F., & Al-Saned, A. (2018). Biodegradation of phenolic components in
wastewater by micro algae: a review. MATEC Web of Conferences, 162, 05009.
https://doi.org/10.1051/matecconf/201816205009
Anku, W. W., Mamo, M. A., & Govender, P. P. (2017). Phenolic Compounds in Water: Sources,
Reactivity, Toxicity and Treatment Methods. Phenolic Compounds - Natural Sources,
Importance and Applications. https://doi.org/10.5772/66927
Asira, E. E. (2014). Factors that Determine Bioremediation of Organic Compounds in the Soil.
Academic Journal of Interdisciplinary Studies, 2(13), 125–128.
https://doi.org/10.5901/ajis.2013.v2n13p125
Banerjee, A. (2011). Biodegradation of Phenolic and Petroleum wastewater vy isolated Bacillus Cereus.
IIT Guwahati, 163(September), V–V. https://doi.org/10.1007/BF00026914
Boon, N., Zhao, S., Liu, H., Zhang, J.-J., Zhou, N.-Y., & Niu, G.-L. (2008). Bioaugmentation of a 4-
chloronitrobenzene contaminated soil with Pseudomonas putida ZWL73. Environmental
Pollution, 157(3), 763–771. https://doi.org/10.1016/j.envpol.2008.11.024
Abatenh, E., Gizaw, B., Tsegaye, Z., & Wassie, M. (2017). Application of microorganisms in
bioremediation-review. Journal of Environmental Microbiology, 1(1), 2–9.
Ahmed, A. T., Othman, M. A., Sarwade, V. D., & Kachru, G. R. (2012). Degradation of Anthracene by
Alkaliphilic Bacteria Bacillus badius. Environment and Pollution, 1(2), 97–104.
https://doi.org/10.5539/ep.v1n2p97
Al-Dahhan, M., Al-Ani, F., & Al-Saned, A. (2018). Biodegradation of phenolic components in
wastewater by micro algae: a review. MATEC Web of Conferences, 162, 05009.
https://doi.org/10.1051/matecconf/201816205009
Anku, W. W., Mamo, M. A., & Govender, P. P. (2017). Phenolic Compounds in Water: Sources,
Reactivity, Toxicity and Treatment Methods. Phenolic Compounds - Natural Sources,
Importance and Applications. https://doi.org/10.5772/66927
Asira, E. E. (2014). Factors that Determine Bioremediation of Organic Compounds in the Soil.
Academic Journal of Interdisciplinary Studies, 2(13), 125–128.
https://doi.org/10.5901/ajis.2013.v2n13p125
Banerjee, A. (2011). Biodegradation of Phenolic and Petroleum wastewater vy isolated Bacillus Cereus.
IIT Guwahati, 163(September), V–V. https://doi.org/10.1007/BF00026914
Boon, N., Zhao, S., Liu, H., Zhang, J.-J., Zhou, N.-Y., & Niu, G.-L. (2008). Bioaugmentation of a 4-
chloronitrobenzene contaminated soil with Pseudomonas putida ZWL73. Environmental
Pollution, 157(3), 763–771. https://doi.org/10.1016/j.envpol.2008.11.024
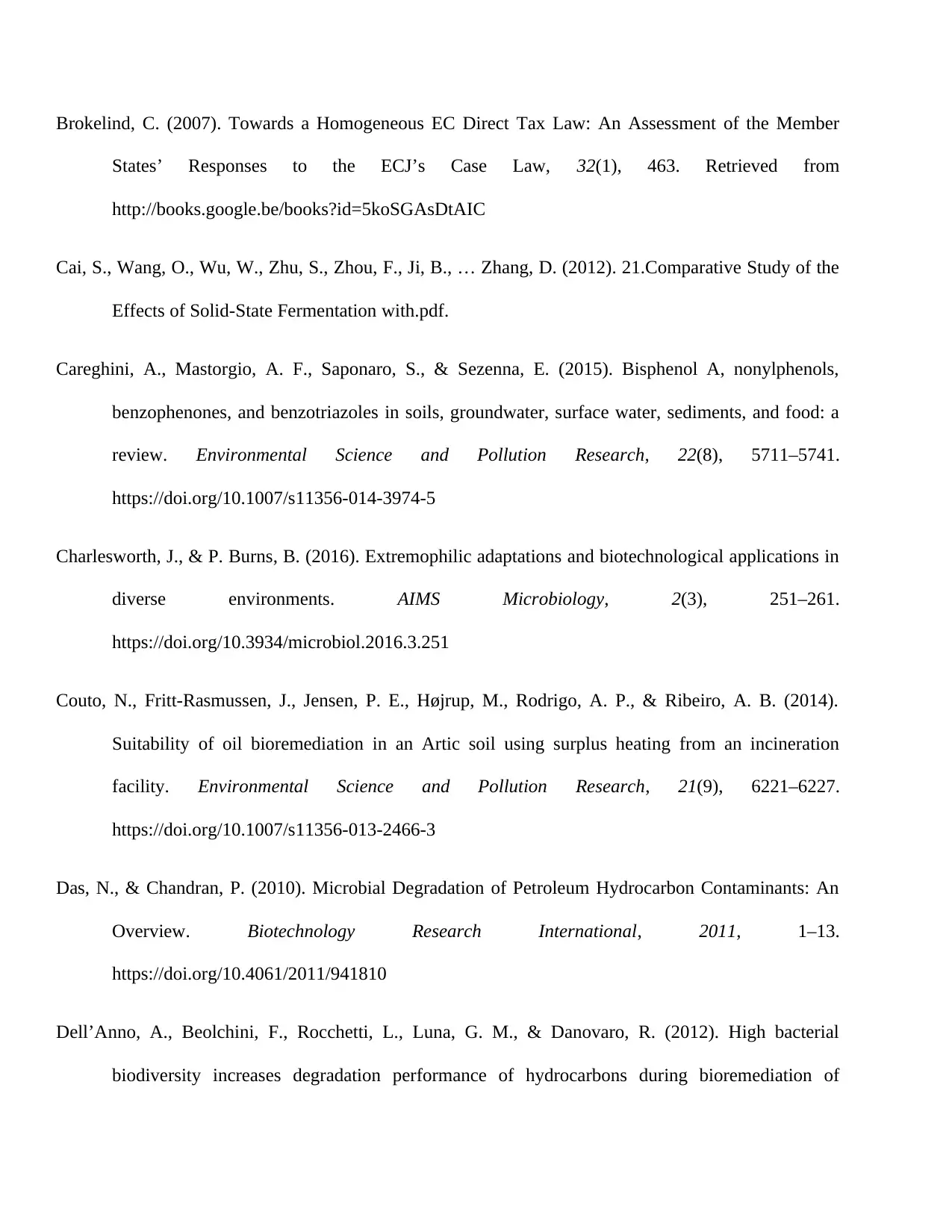
Brokelind, C. (2007). Towards a Homogeneous EC Direct Tax Law: An Assessment of the Member
States’ Responses to the ECJ’s Case Law, 32(1), 463. Retrieved from
http://books.google.be/books?id=5koSGAsDtAIC
Cai, S., Wang, O., Wu, W., Zhu, S., Zhou, F., Ji, B., … Zhang, D. (2012). 21.Comparative Study of the
Effects of Solid-State Fermentation with.pdf.
Careghini, A., Mastorgio, A. F., Saponaro, S., & Sezenna, E. (2015). Bisphenol A, nonylphenols,
benzophenones, and benzotriazoles in soils, groundwater, surface water, sediments, and food: a
review. Environmental Science and Pollution Research, 22(8), 5711–5741.
https://doi.org/10.1007/s11356-014-3974-5
Charlesworth, J., & P. Burns, B. (2016). Extremophilic adaptations and biotechnological applications in
diverse environments. AIMS Microbiology, 2(3), 251–261.
https://doi.org/10.3934/microbiol.2016.3.251
Couto, N., Fritt-Rasmussen, J., Jensen, P. E., Højrup, M., Rodrigo, A. P., & Ribeiro, A. B. (2014).
Suitability of oil bioremediation in an Artic soil using surplus heating from an incineration
facility. Environmental Science and Pollution Research, 21(9), 6221–6227.
https://doi.org/10.1007/s11356-013-2466-3
Das, N., & Chandran, P. (2010). Microbial Degradation of Petroleum Hydrocarbon Contaminants: An
Overview. Biotechnology Research International, 2011, 1–13.
https://doi.org/10.4061/2011/941810
Dell’Anno, A., Beolchini, F., Rocchetti, L., Luna, G. M., & Danovaro, R. (2012). High bacterial
biodiversity increases degradation performance of hydrocarbons during bioremediation of
States’ Responses to the ECJ’s Case Law, 32(1), 463. Retrieved from
http://books.google.be/books?id=5koSGAsDtAIC
Cai, S., Wang, O., Wu, W., Zhu, S., Zhou, F., Ji, B., … Zhang, D. (2012). 21.Comparative Study of the
Effects of Solid-State Fermentation with.pdf.
Careghini, A., Mastorgio, A. F., Saponaro, S., & Sezenna, E. (2015). Bisphenol A, nonylphenols,
benzophenones, and benzotriazoles in soils, groundwater, surface water, sediments, and food: a
review. Environmental Science and Pollution Research, 22(8), 5711–5741.
https://doi.org/10.1007/s11356-014-3974-5
Charlesworth, J., & P. Burns, B. (2016). Extremophilic adaptations and biotechnological applications in
diverse environments. AIMS Microbiology, 2(3), 251–261.
https://doi.org/10.3934/microbiol.2016.3.251
Couto, N., Fritt-Rasmussen, J., Jensen, P. E., Højrup, M., Rodrigo, A. P., & Ribeiro, A. B. (2014).
Suitability of oil bioremediation in an Artic soil using surplus heating from an incineration
facility. Environmental Science and Pollution Research, 21(9), 6221–6227.
https://doi.org/10.1007/s11356-013-2466-3
Das, N., & Chandran, P. (2010). Microbial Degradation of Petroleum Hydrocarbon Contaminants: An
Overview. Biotechnology Research International, 2011, 1–13.
https://doi.org/10.4061/2011/941810
Dell’Anno, A., Beolchini, F., Rocchetti, L., Luna, G. M., & Danovaro, R. (2012). High bacterial
biodiversity increases degradation performance of hydrocarbons during bioremediation of
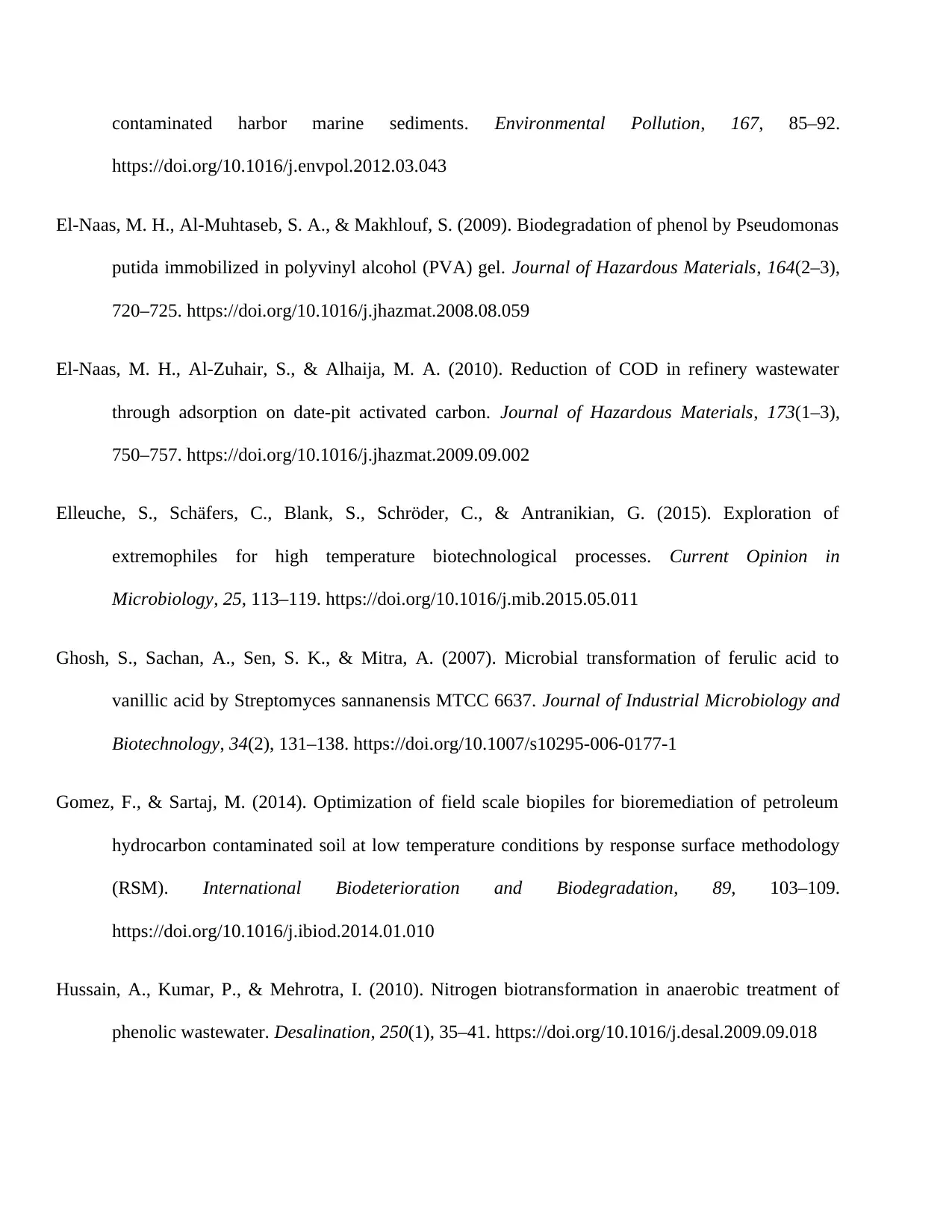
contaminated harbor marine sediments. Environmental Pollution, 167, 85–92.
https://doi.org/10.1016/j.envpol.2012.03.043
El-Naas, M. H., Al-Muhtaseb, S. A., & Makhlouf, S. (2009). Biodegradation of phenol by Pseudomonas
putida immobilized in polyvinyl alcohol (PVA) gel. Journal of Hazardous Materials, 164(2–3),
720–725. https://doi.org/10.1016/j.jhazmat.2008.08.059
El-Naas, M. H., Al-Zuhair, S., & Alhaija, M. A. (2010). Reduction of COD in refinery wastewater
through adsorption on date-pit activated carbon. Journal of Hazardous Materials, 173(1–3),
750–757. https://doi.org/10.1016/j.jhazmat.2009.09.002
Elleuche, S., Schäfers, C., Blank, S., Schröder, C., & Antranikian, G. (2015). Exploration of
extremophiles for high temperature biotechnological processes. Current Opinion in
Microbiology, 25, 113–119. https://doi.org/10.1016/j.mib.2015.05.011
Ghosh, S., Sachan, A., Sen, S. K., & Mitra, A. (2007). Microbial transformation of ferulic acid to
vanillic acid by Streptomyces sannanensis MTCC 6637. Journal of Industrial Microbiology and
Biotechnology, 34(2), 131–138. https://doi.org/10.1007/s10295-006-0177-1
Gomez, F., & Sartaj, M. (2014). Optimization of field scale biopiles for bioremediation of petroleum
hydrocarbon contaminated soil at low temperature conditions by response surface methodology
(RSM). International Biodeterioration and Biodegradation, 89, 103–109.
https://doi.org/10.1016/j.ibiod.2014.01.010
Hussain, A., Kumar, P., & Mehrotra, I. (2010). Nitrogen biotransformation in anaerobic treatment of
phenolic wastewater. Desalination, 250(1), 35–41. https://doi.org/10.1016/j.desal.2009.09.018
https://doi.org/10.1016/j.envpol.2012.03.043
El-Naas, M. H., Al-Muhtaseb, S. A., & Makhlouf, S. (2009). Biodegradation of phenol by Pseudomonas
putida immobilized in polyvinyl alcohol (PVA) gel. Journal of Hazardous Materials, 164(2–3),
720–725. https://doi.org/10.1016/j.jhazmat.2008.08.059
El-Naas, M. H., Al-Zuhair, S., & Alhaija, M. A. (2010). Reduction of COD in refinery wastewater
through adsorption on date-pit activated carbon. Journal of Hazardous Materials, 173(1–3),
750–757. https://doi.org/10.1016/j.jhazmat.2009.09.002
Elleuche, S., Schäfers, C., Blank, S., Schröder, C., & Antranikian, G. (2015). Exploration of
extremophiles for high temperature biotechnological processes. Current Opinion in
Microbiology, 25, 113–119. https://doi.org/10.1016/j.mib.2015.05.011
Ghosh, S., Sachan, A., Sen, S. K., & Mitra, A. (2007). Microbial transformation of ferulic acid to
vanillic acid by Streptomyces sannanensis MTCC 6637. Journal of Industrial Microbiology and
Biotechnology, 34(2), 131–138. https://doi.org/10.1007/s10295-006-0177-1
Gomez, F., & Sartaj, M. (2014). Optimization of field scale biopiles for bioremediation of petroleum
hydrocarbon contaminated soil at low temperature conditions by response surface methodology
(RSM). International Biodeterioration and Biodegradation, 89, 103–109.
https://doi.org/10.1016/j.ibiod.2014.01.010
Hussain, A., Kumar, P., & Mehrotra, I. (2010). Nitrogen biotransformation in anaerobic treatment of
phenolic wastewater. Desalination, 250(1), 35–41. https://doi.org/10.1016/j.desal.2009.09.018
Secure Best Marks with AI Grader
Need help grading? Try our AI Grader for instant feedback on your assignments.
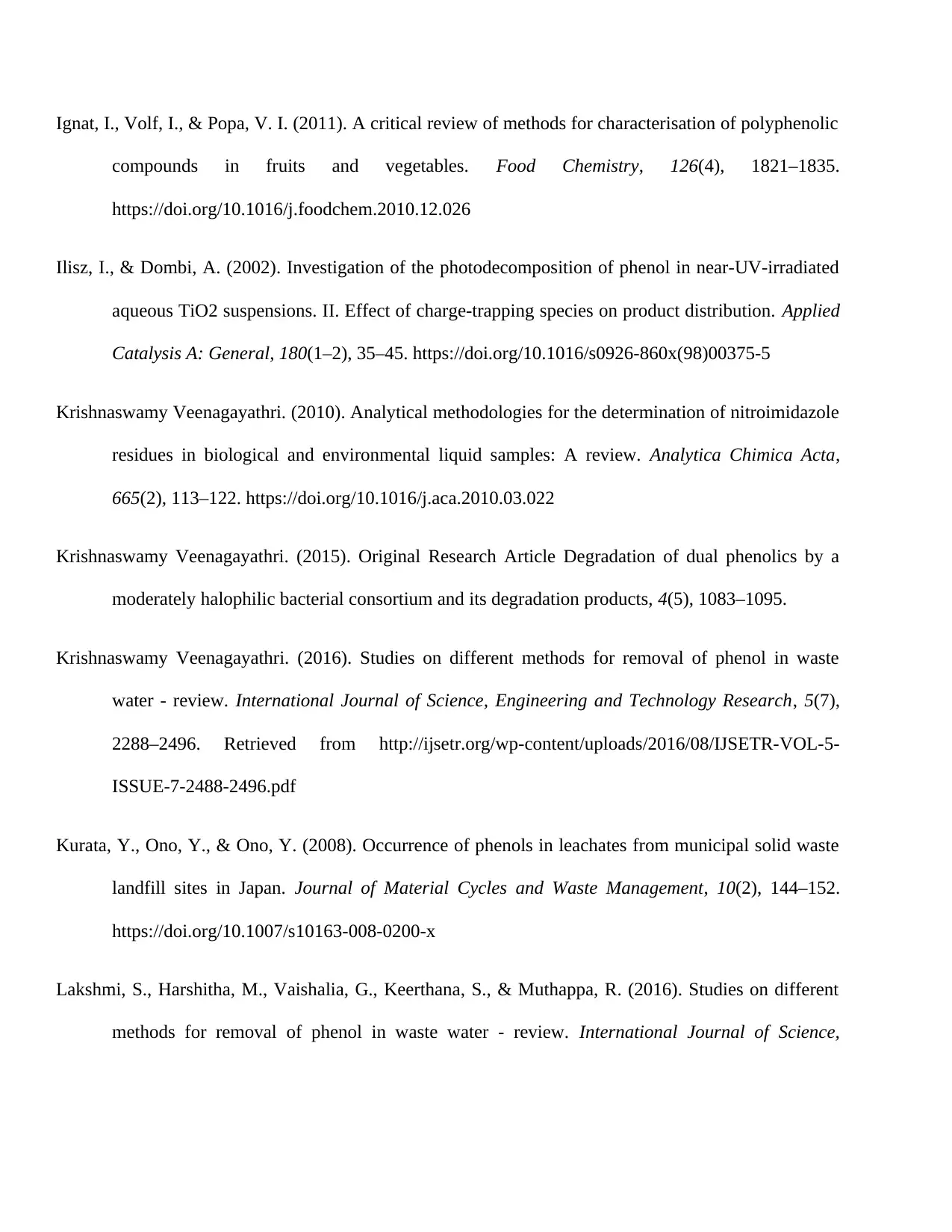
Ignat, I., Volf, I., & Popa, V. I. (2011). A critical review of methods for characterisation of polyphenolic
compounds in fruits and vegetables. Food Chemistry, 126(4), 1821–1835.
https://doi.org/10.1016/j.foodchem.2010.12.026
Ilisz, I., & Dombi, A. (2002). Investigation of the photodecomposition of phenol in near-UV-irradiated
aqueous TiO2 suspensions. II. Effect of charge-trapping species on product distribution. Applied
Catalysis A: General, 180(1–2), 35–45. https://doi.org/10.1016/s0926-860x(98)00375-5
Krishnaswamy Veenagayathri. (2010). Analytical methodologies for the determination of nitroimidazole
residues in biological and environmental liquid samples: A review. Analytica Chimica Acta,
665(2), 113–122. https://doi.org/10.1016/j.aca.2010.03.022
Krishnaswamy Veenagayathri. (2015). Original Research Article Degradation of dual phenolics by a
moderately halophilic bacterial consortium and its degradation products, 4(5), 1083–1095.
Krishnaswamy Veenagayathri. (2016). Studies on different methods for removal of phenol in waste
water - review. International Journal of Science, Engineering and Technology Research, 5(7),
2288–2496. Retrieved from http://ijsetr.org/wp-content/uploads/2016/08/IJSETR-VOL-5-
ISSUE-7-2488-2496.pdf
Kurata, Y., Ono, Y., & Ono, Y. (2008). Occurrence of phenols in leachates from municipal solid waste
landfill sites in Japan. Journal of Material Cycles and Waste Management, 10(2), 144–152.
https://doi.org/10.1007/s10163-008-0200-x
Lakshmi, S., Harshitha, M., Vaishalia, G., Keerthana, S., & Muthappa, R. (2016). Studies on different
methods for removal of phenol in waste water - review. International Journal of Science,
compounds in fruits and vegetables. Food Chemistry, 126(4), 1821–1835.
https://doi.org/10.1016/j.foodchem.2010.12.026
Ilisz, I., & Dombi, A. (2002). Investigation of the photodecomposition of phenol in near-UV-irradiated
aqueous TiO2 suspensions. II. Effect of charge-trapping species on product distribution. Applied
Catalysis A: General, 180(1–2), 35–45. https://doi.org/10.1016/s0926-860x(98)00375-5
Krishnaswamy Veenagayathri. (2010). Analytical methodologies for the determination of nitroimidazole
residues in biological and environmental liquid samples: A review. Analytica Chimica Acta,
665(2), 113–122. https://doi.org/10.1016/j.aca.2010.03.022
Krishnaswamy Veenagayathri. (2015). Original Research Article Degradation of dual phenolics by a
moderately halophilic bacterial consortium and its degradation products, 4(5), 1083–1095.
Krishnaswamy Veenagayathri. (2016). Studies on different methods for removal of phenol in waste
water - review. International Journal of Science, Engineering and Technology Research, 5(7),
2288–2496. Retrieved from http://ijsetr.org/wp-content/uploads/2016/08/IJSETR-VOL-5-
ISSUE-7-2488-2496.pdf
Kurata, Y., Ono, Y., & Ono, Y. (2008). Occurrence of phenols in leachates from municipal solid waste
landfill sites in Japan. Journal of Material Cycles and Waste Management, 10(2), 144–152.
https://doi.org/10.1007/s10163-008-0200-x
Lakshmi, S., Harshitha, M., Vaishalia, G., Keerthana, S., & Muthappa, R. (2016). Studies on different
methods for removal of phenol in waste water - review. International Journal of Science,
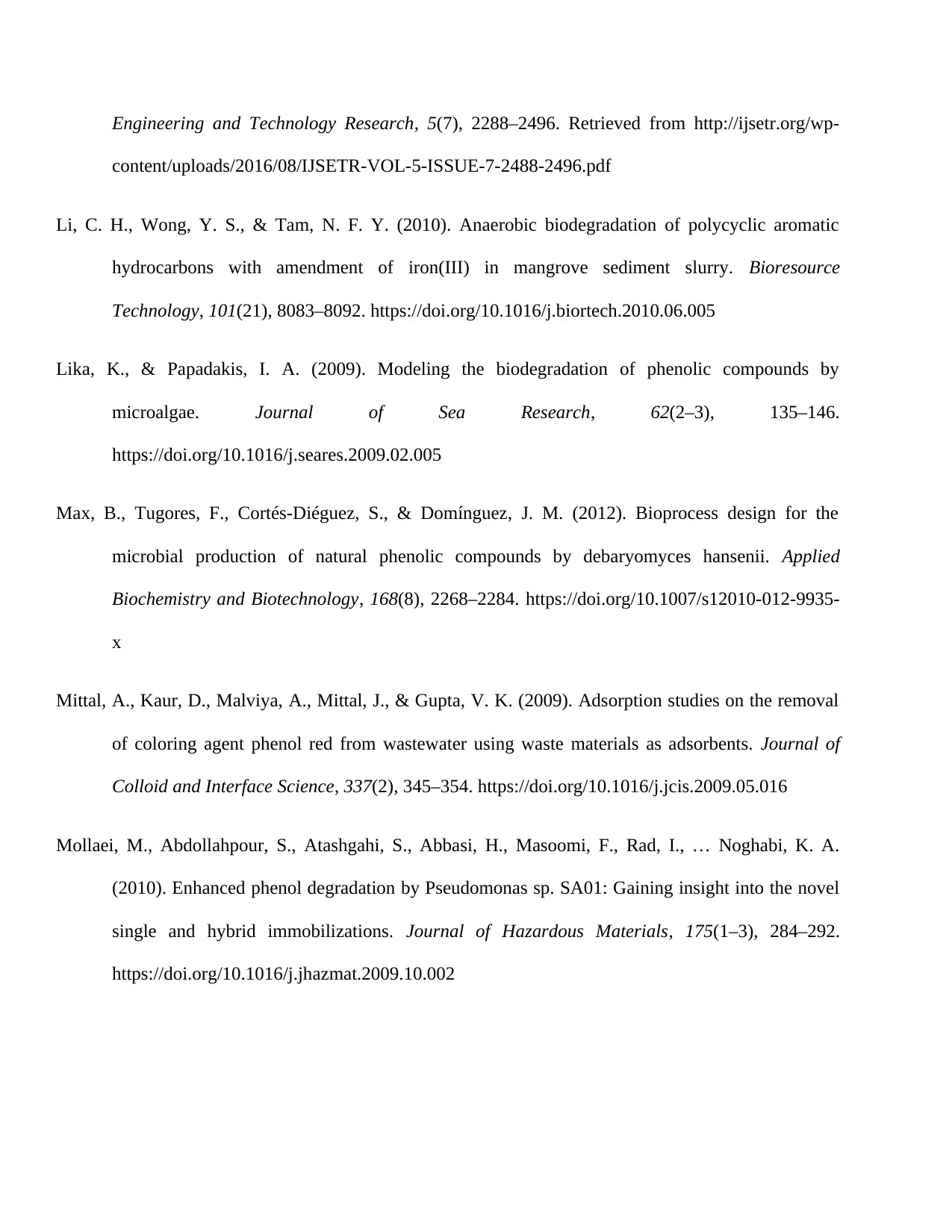
Engineering and Technology Research, 5(7), 2288–2496. Retrieved from http://ijsetr.org/wp-
content/uploads/2016/08/IJSETR-VOL-5-ISSUE-7-2488-2496.pdf
Li, C. H., Wong, Y. S., & Tam, N. F. Y. (2010). Anaerobic biodegradation of polycyclic aromatic
hydrocarbons with amendment of iron(III) in mangrove sediment slurry. Bioresource
Technology, 101(21), 8083–8092. https://doi.org/10.1016/j.biortech.2010.06.005
Lika, K., & Papadakis, I. A. (2009). Modeling the biodegradation of phenolic compounds by
microalgae. Journal of Sea Research, 62(2–3), 135–146.
https://doi.org/10.1016/j.seares.2009.02.005
Max, B., Tugores, F., Cortés-Diéguez, S., & Domínguez, J. M. (2012). Bioprocess design for the
microbial production of natural phenolic compounds by debaryomyces hansenii. Applied
Biochemistry and Biotechnology, 168(8), 2268–2284. https://doi.org/10.1007/s12010-012-9935-
x
Mittal, A., Kaur, D., Malviya, A., Mittal, J., & Gupta, V. K. (2009). Adsorption studies on the removal
of coloring agent phenol red from wastewater using waste materials as adsorbents. Journal of
Colloid and Interface Science, 337(2), 345–354. https://doi.org/10.1016/j.jcis.2009.05.016
Mollaei, M., Abdollahpour, S., Atashgahi, S., Abbasi, H., Masoomi, F., Rad, I., … Noghabi, K. A.
(2010). Enhanced phenol degradation by Pseudomonas sp. SA01: Gaining insight into the novel
single and hybrid immobilizations. Journal of Hazardous Materials, 175(1–3), 284–292.
https://doi.org/10.1016/j.jhazmat.2009.10.002
content/uploads/2016/08/IJSETR-VOL-5-ISSUE-7-2488-2496.pdf
Li, C. H., Wong, Y. S., & Tam, N. F. Y. (2010). Anaerobic biodegradation of polycyclic aromatic
hydrocarbons with amendment of iron(III) in mangrove sediment slurry. Bioresource
Technology, 101(21), 8083–8092. https://doi.org/10.1016/j.biortech.2010.06.005
Lika, K., & Papadakis, I. A. (2009). Modeling the biodegradation of phenolic compounds by
microalgae. Journal of Sea Research, 62(2–3), 135–146.
https://doi.org/10.1016/j.seares.2009.02.005
Max, B., Tugores, F., Cortés-Diéguez, S., & Domínguez, J. M. (2012). Bioprocess design for the
microbial production of natural phenolic compounds by debaryomyces hansenii. Applied
Biochemistry and Biotechnology, 168(8), 2268–2284. https://doi.org/10.1007/s12010-012-9935-
x
Mittal, A., Kaur, D., Malviya, A., Mittal, J., & Gupta, V. K. (2009). Adsorption studies on the removal
of coloring agent phenol red from wastewater using waste materials as adsorbents. Journal of
Colloid and Interface Science, 337(2), 345–354. https://doi.org/10.1016/j.jcis.2009.05.016
Mollaei, M., Abdollahpour, S., Atashgahi, S., Abbasi, H., Masoomi, F., Rad, I., … Noghabi, K. A.
(2010). Enhanced phenol degradation by Pseudomonas sp. SA01: Gaining insight into the novel
single and hybrid immobilizations. Journal of Hazardous Materials, 175(1–3), 284–292.
https://doi.org/10.1016/j.jhazmat.2009.10.002
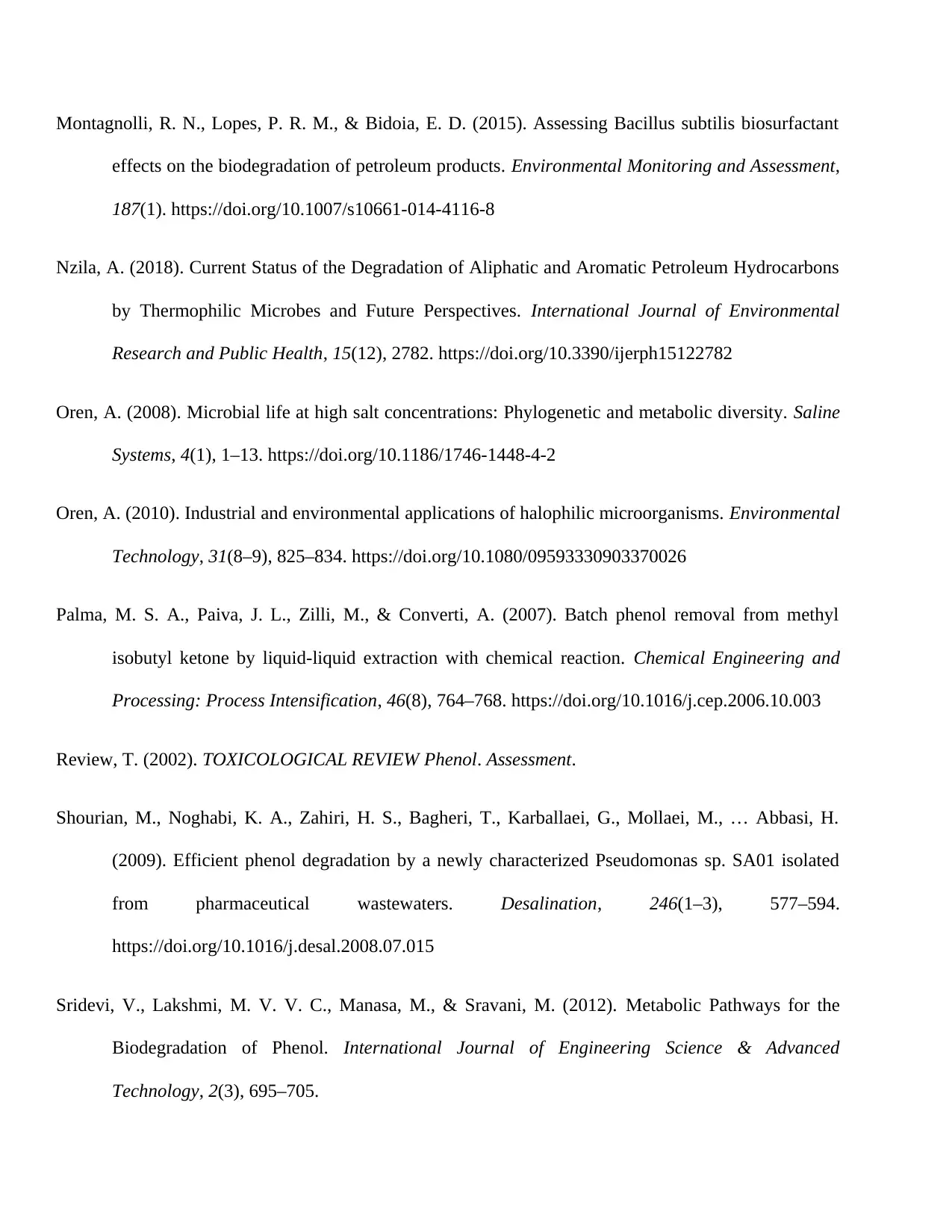
Montagnolli, R. N., Lopes, P. R. M., & Bidoia, E. D. (2015). Assessing Bacillus subtilis biosurfactant
effects on the biodegradation of petroleum products. Environmental Monitoring and Assessment,
187(1). https://doi.org/10.1007/s10661-014-4116-8
Nzila, A. (2018). Current Status of the Degradation of Aliphatic and Aromatic Petroleum Hydrocarbons
by Thermophilic Microbes and Future Perspectives. International Journal of Environmental
Research and Public Health, 15(12), 2782. https://doi.org/10.3390/ijerph15122782
Oren, A. (2008). Microbial life at high salt concentrations: Phylogenetic and metabolic diversity. Saline
Systems, 4(1), 1–13. https://doi.org/10.1186/1746-1448-4-2
Oren, A. (2010). Industrial and environmental applications of halophilic microorganisms. Environmental
Technology, 31(8–9), 825–834. https://doi.org/10.1080/09593330903370026
Palma, M. S. A., Paiva, J. L., Zilli, M., & Converti, A. (2007). Batch phenol removal from methyl
isobutyl ketone by liquid-liquid extraction with chemical reaction. Chemical Engineering and
Processing: Process Intensification, 46(8), 764–768. https://doi.org/10.1016/j.cep.2006.10.003
Review, T. (2002). TOXICOLOGICAL REVIEW Phenol. Assessment.
Shourian, M., Noghabi, K. A., Zahiri, H. S., Bagheri, T., Karballaei, G., Mollaei, M., … Abbasi, H.
(2009). Efficient phenol degradation by a newly characterized Pseudomonas sp. SA01 isolated
from pharmaceutical wastewaters. Desalination, 246(1–3), 577–594.
https://doi.org/10.1016/j.desal.2008.07.015
Sridevi, V., Lakshmi, M. V. V. C., Manasa, M., & Sravani, M. (2012). Metabolic Pathways for the
Biodegradation of Phenol. International Journal of Engineering Science & Advanced
Technology, 2(3), 695–705.
effects on the biodegradation of petroleum products. Environmental Monitoring and Assessment,
187(1). https://doi.org/10.1007/s10661-014-4116-8
Nzila, A. (2018). Current Status of the Degradation of Aliphatic and Aromatic Petroleum Hydrocarbons
by Thermophilic Microbes and Future Perspectives. International Journal of Environmental
Research and Public Health, 15(12), 2782. https://doi.org/10.3390/ijerph15122782
Oren, A. (2008). Microbial life at high salt concentrations: Phylogenetic and metabolic diversity. Saline
Systems, 4(1), 1–13. https://doi.org/10.1186/1746-1448-4-2
Oren, A. (2010). Industrial and environmental applications of halophilic microorganisms. Environmental
Technology, 31(8–9), 825–834. https://doi.org/10.1080/09593330903370026
Palma, M. S. A., Paiva, J. L., Zilli, M., & Converti, A. (2007). Batch phenol removal from methyl
isobutyl ketone by liquid-liquid extraction with chemical reaction. Chemical Engineering and
Processing: Process Intensification, 46(8), 764–768. https://doi.org/10.1016/j.cep.2006.10.003
Review, T. (2002). TOXICOLOGICAL REVIEW Phenol. Assessment.
Shourian, M., Noghabi, K. A., Zahiri, H. S., Bagheri, T., Karballaei, G., Mollaei, M., … Abbasi, H.
(2009). Efficient phenol degradation by a newly characterized Pseudomonas sp. SA01 isolated
from pharmaceutical wastewaters. Desalination, 246(1–3), 577–594.
https://doi.org/10.1016/j.desal.2008.07.015
Sridevi, V., Lakshmi, M. V. V. C., Manasa, M., & Sravani, M. (2012). Metabolic Pathways for the
Biodegradation of Phenol. International Journal of Engineering Science & Advanced
Technology, 2(3), 695–705.
Paraphrase This Document
Need a fresh take? Get an instant paraphrase of this document with our AI Paraphraser
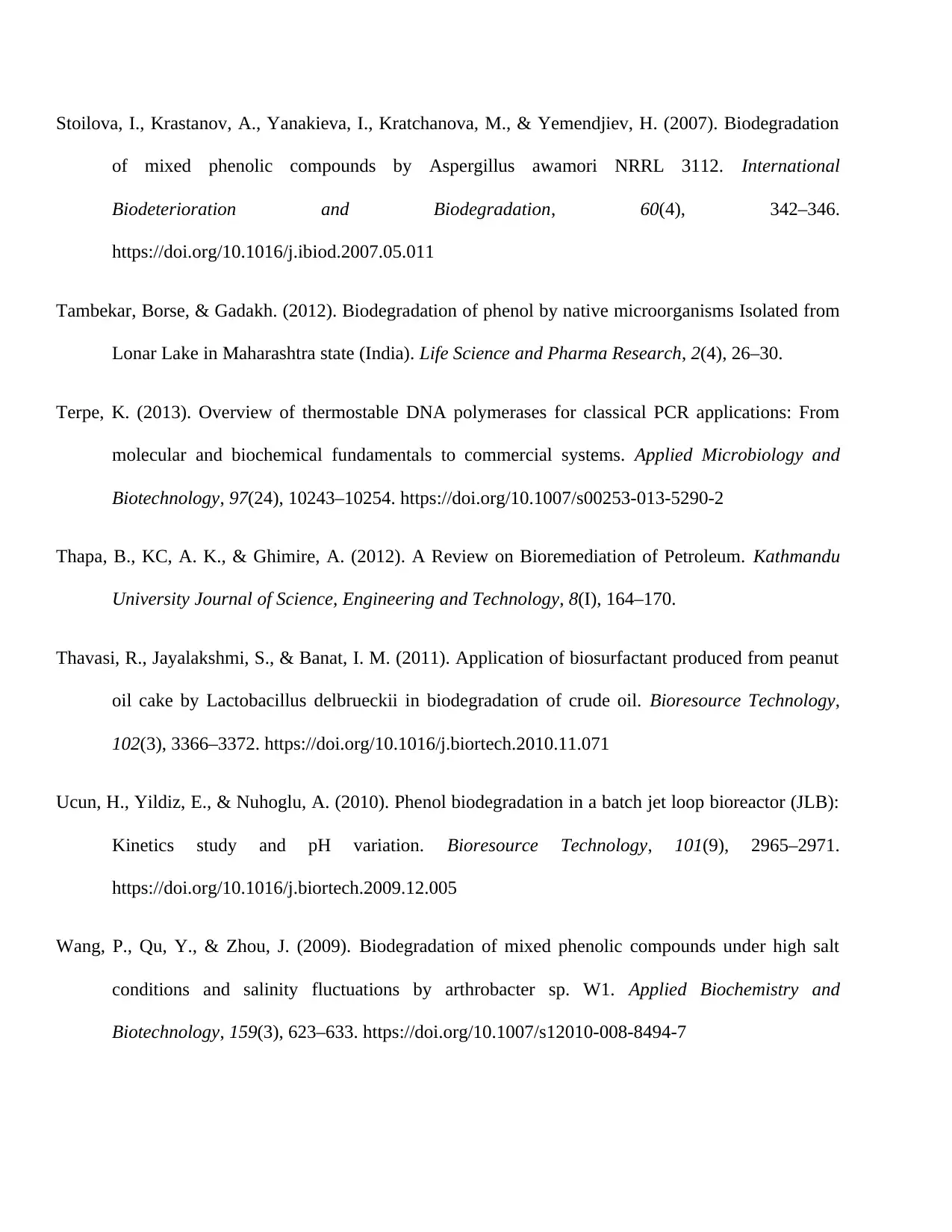
Stoilova, I., Krastanov, A., Yanakieva, I., Kratchanova, M., & Yemendjiev, H. (2007). Biodegradation
of mixed phenolic compounds by Aspergillus awamori NRRL 3112. International
Biodeterioration and Biodegradation, 60(4), 342–346.
https://doi.org/10.1016/j.ibiod.2007.05.011
Tambekar, Borse, & Gadakh. (2012). Biodegradation of phenol by native microorganisms Isolated from
Lonar Lake in Maharashtra state (India). Life Science and Pharma Research, 2(4), 26–30.
Terpe, K. (2013). Overview of thermostable DNA polymerases for classical PCR applications: From
molecular and biochemical fundamentals to commercial systems. Applied Microbiology and
Biotechnology, 97(24), 10243–10254. https://doi.org/10.1007/s00253-013-5290-2
Thapa, B., KC, A. K., & Ghimire, A. (2012). A Review on Bioremediation of Petroleum. Kathmandu
University Journal of Science, Engineering and Technology, 8(I), 164–170.
Thavasi, R., Jayalakshmi, S., & Banat, I. M. (2011). Application of biosurfactant produced from peanut
oil cake by Lactobacillus delbrueckii in biodegradation of crude oil. Bioresource Technology,
102(3), 3366–3372. https://doi.org/10.1016/j.biortech.2010.11.071
Ucun, H., Yildiz, E., & Nuhoglu, A. (2010). Phenol biodegradation in a batch jet loop bioreactor (JLB):
Kinetics study and pH variation. Bioresource Technology, 101(9), 2965–2971.
https://doi.org/10.1016/j.biortech.2009.12.005
Wang, P., Qu, Y., & Zhou, J. (2009). Biodegradation of mixed phenolic compounds under high salt
conditions and salinity fluctuations by arthrobacter sp. W1. Applied Biochemistry and
Biotechnology, 159(3), 623–633. https://doi.org/10.1007/s12010-008-8494-7
of mixed phenolic compounds by Aspergillus awamori NRRL 3112. International
Biodeterioration and Biodegradation, 60(4), 342–346.
https://doi.org/10.1016/j.ibiod.2007.05.011
Tambekar, Borse, & Gadakh. (2012). Biodegradation of phenol by native microorganisms Isolated from
Lonar Lake in Maharashtra state (India). Life Science and Pharma Research, 2(4), 26–30.
Terpe, K. (2013). Overview of thermostable DNA polymerases for classical PCR applications: From
molecular and biochemical fundamentals to commercial systems. Applied Microbiology and
Biotechnology, 97(24), 10243–10254. https://doi.org/10.1007/s00253-013-5290-2
Thapa, B., KC, A. K., & Ghimire, A. (2012). A Review on Bioremediation of Petroleum. Kathmandu
University Journal of Science, Engineering and Technology, 8(I), 164–170.
Thavasi, R., Jayalakshmi, S., & Banat, I. M. (2011). Application of biosurfactant produced from peanut
oil cake by Lactobacillus delbrueckii in biodegradation of crude oil. Bioresource Technology,
102(3), 3366–3372. https://doi.org/10.1016/j.biortech.2010.11.071
Ucun, H., Yildiz, E., & Nuhoglu, A. (2010). Phenol biodegradation in a batch jet loop bioreactor (JLB):
Kinetics study and pH variation. Bioresource Technology, 101(9), 2965–2971.
https://doi.org/10.1016/j.biortech.2009.12.005
Wang, P., Qu, Y., & Zhou, J. (2009). Biodegradation of mixed phenolic compounds under high salt
conditions and salinity fluctuations by arthrobacter sp. W1. Applied Biochemistry and
Biotechnology, 159(3), 623–633. https://doi.org/10.1007/s12010-008-8494-7
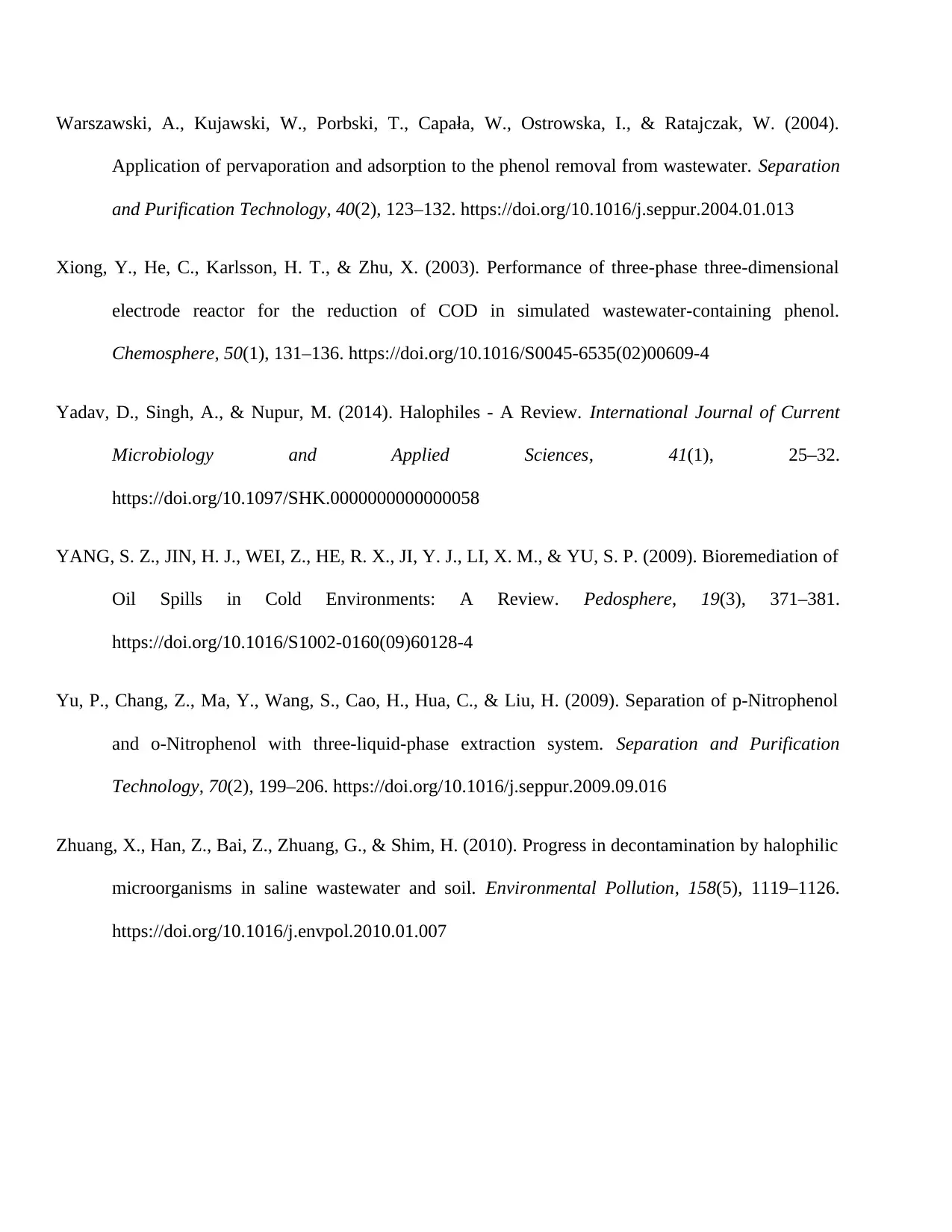
Warszawski, A., Kujawski, W., Porbski, T., Capała, W., Ostrowska, I., & Ratajczak, W. (2004).
Application of pervaporation and adsorption to the phenol removal from wastewater. Separation
and Purification Technology, 40(2), 123–132. https://doi.org/10.1016/j.seppur.2004.01.013
Xiong, Y., He, C., Karlsson, H. T., & Zhu, X. (2003). Performance of three-phase three-dimensional
electrode reactor for the reduction of COD in simulated wastewater-containing phenol.
Chemosphere, 50(1), 131–136. https://doi.org/10.1016/S0045-6535(02)00609-4
Yadav, D., Singh, A., & Nupur, M. (2014). Halophiles - A Review. International Journal of Current
Microbiology and Applied Sciences, 41(1), 25–32.
https://doi.org/10.1097/SHK.0000000000000058
YANG, S. Z., JIN, H. J., WEI, Z., HE, R. X., JI, Y. J., LI, X. M., & YU, S. P. (2009). Bioremediation of
Oil Spills in Cold Environments: A Review. Pedosphere, 19(3), 371–381.
https://doi.org/10.1016/S1002-0160(09)60128-4
Yu, P., Chang, Z., Ma, Y., Wang, S., Cao, H., Hua, C., & Liu, H. (2009). Separation of p-Nitrophenol
and o-Nitrophenol with three-liquid-phase extraction system. Separation and Purification
Technology, 70(2), 199–206. https://doi.org/10.1016/j.seppur.2009.09.016
Zhuang, X., Han, Z., Bai, Z., Zhuang, G., & Shim, H. (2010). Progress in decontamination by halophilic
microorganisms in saline wastewater and soil. Environmental Pollution, 158(5), 1119–1126.
https://doi.org/10.1016/j.envpol.2010.01.007
Application of pervaporation and adsorption to the phenol removal from wastewater. Separation
and Purification Technology, 40(2), 123–132. https://doi.org/10.1016/j.seppur.2004.01.013
Xiong, Y., He, C., Karlsson, H. T., & Zhu, X. (2003). Performance of three-phase three-dimensional
electrode reactor for the reduction of COD in simulated wastewater-containing phenol.
Chemosphere, 50(1), 131–136. https://doi.org/10.1016/S0045-6535(02)00609-4
Yadav, D., Singh, A., & Nupur, M. (2014). Halophiles - A Review. International Journal of Current
Microbiology and Applied Sciences, 41(1), 25–32.
https://doi.org/10.1097/SHK.0000000000000058
YANG, S. Z., JIN, H. J., WEI, Z., HE, R. X., JI, Y. J., LI, X. M., & YU, S. P. (2009). Bioremediation of
Oil Spills in Cold Environments: A Review. Pedosphere, 19(3), 371–381.
https://doi.org/10.1016/S1002-0160(09)60128-4
Yu, P., Chang, Z., Ma, Y., Wang, S., Cao, H., Hua, C., & Liu, H. (2009). Separation of p-Nitrophenol
and o-Nitrophenol with three-liquid-phase extraction system. Separation and Purification
Technology, 70(2), 199–206. https://doi.org/10.1016/j.seppur.2009.09.016
Zhuang, X., Han, Z., Bai, Z., Zhuang, G., & Shim, H. (2010). Progress in decontamination by halophilic
microorganisms in saline wastewater and soil. Environmental Pollution, 158(5), 1119–1126.
https://doi.org/10.1016/j.envpol.2010.01.007
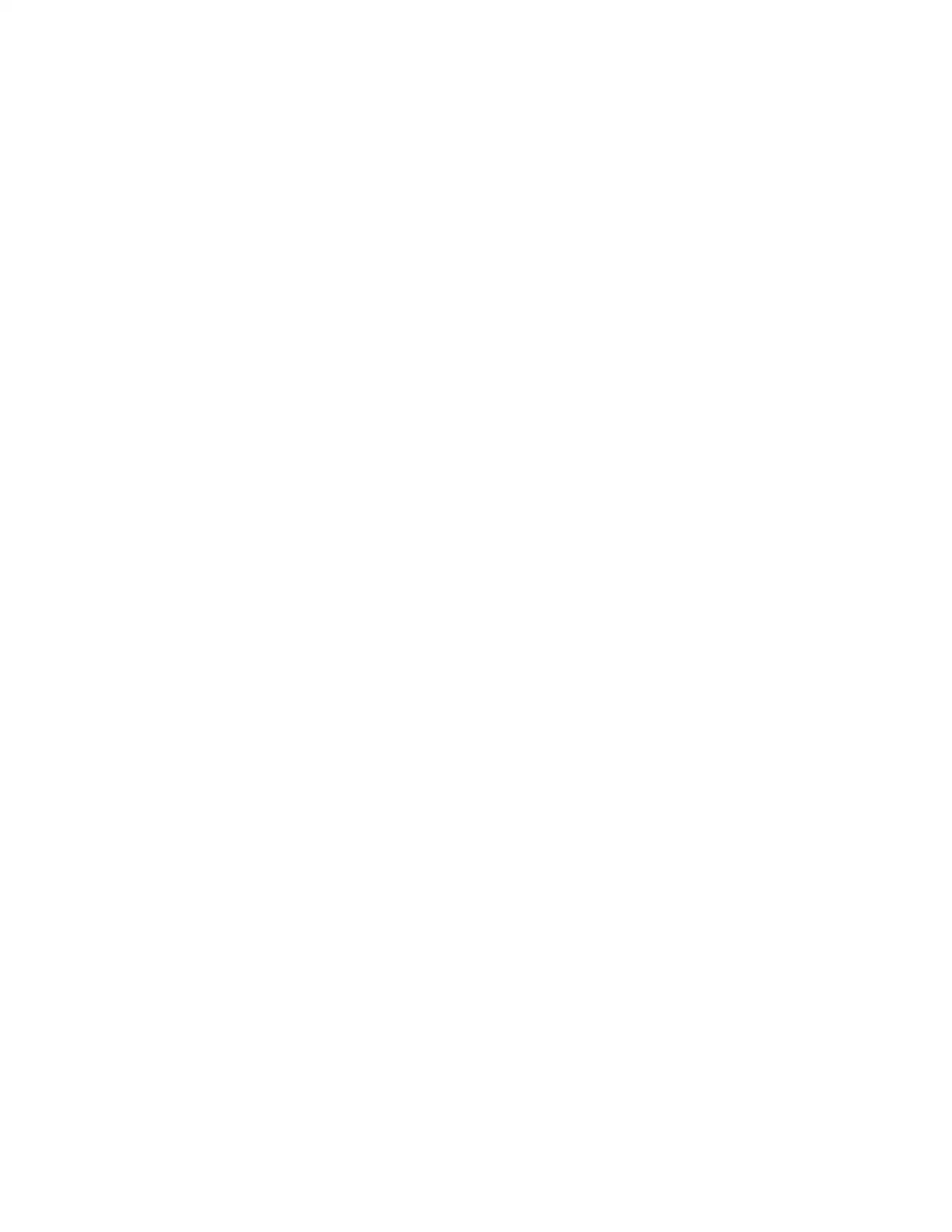
1 out of 46
![[object Object]](/_next/image/?url=%2F_next%2Fstatic%2Fmedia%2Flogo.6d15ce61.png&w=640&q=75)
Your All-in-One AI-Powered Toolkit for Academic Success.
+13062052269
info@desklib.com
Available 24*7 on WhatsApp / Email
Unlock your academic potential
© 2024 | Zucol Services PVT LTD | All rights reserved.