Study of Pyrheliometer to Calculate Direct Normal Irradiance and Global Horizontal Irradiance
VerifiedAdded on 2024/01/18
|71
|15066
|477
AI Summary
This experiment focuses on studying the working of a pyrheliometer and calculating direct normal irradiance and global horizontal irradiance. The experiment was conducted at the University of Engineering and Technology, Lahore.
Contribute Materials
Your contribution can guide someone’s learning journey. Share your
documents today.
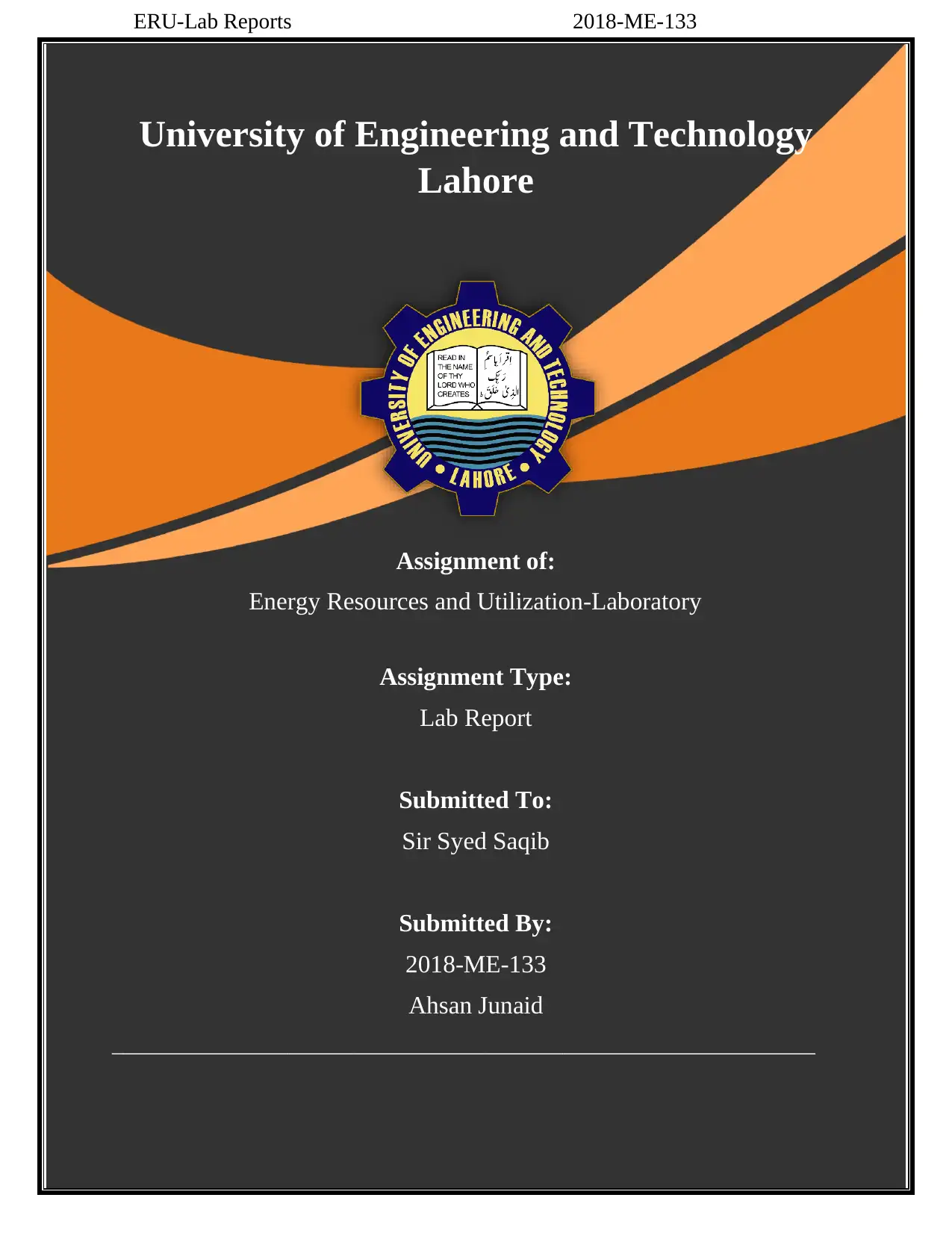
ERU-Lab Reports 2018-ME-133
University of Engineering and Technology
Lahore
Assignment of:
Energy Resources and Utilization-Laboratory
Assignment Type:
Lab Report
Submitted To:
Sir Syed Saqib
Submitted By:
2018-ME-133
Ahsan Junaid
________________________________________________________________
University of Engineering and Technology
Lahore
Assignment of:
Energy Resources and Utilization-Laboratory
Assignment Type:
Lab Report
Submitted To:
Sir Syed Saqib
Submitted By:
2018-ME-133
Ahsan Junaid
________________________________________________________________
Secure Best Marks with AI Grader
Need help grading? Try our AI Grader for instant feedback on your assignments.
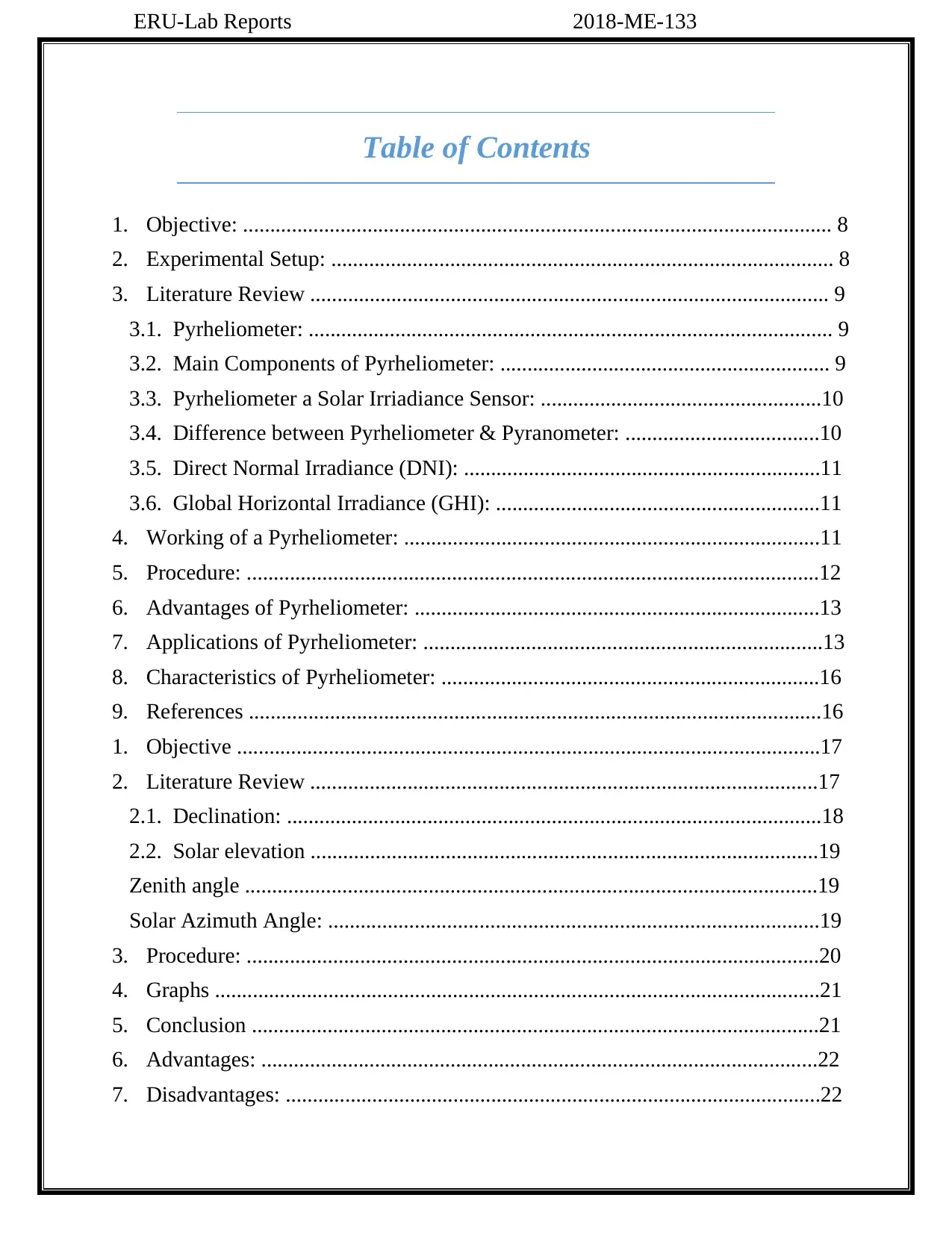
ERU-Lab Reports 2018-ME-133
Table of Contents
1. Objective: ............................................................................................................. 8
2. Experimental Setup: ............................................................................................. 8
3. Literature Review ................................................................................................ 9
3.1. Pyrheliometer: ................................................................................................. 9
3.2. Main Components of Pyrheliometer: ............................................................. 9
3.3. Pyrheliometer a Solar Irriadiance Sensor: ....................................................10
3.4. Difference between Pyrheliometer & Pyranometer: ....................................10
3.5. Direct Normal Irradiance (DNI): ..................................................................11
3.6. Global Horizontal Irradiance (GHI): ............................................................11
4. Working of a Pyrheliometer: .............................................................................11
5. Procedure: ..........................................................................................................12
6. Advantages of Pyrheliometer: ...........................................................................13
7. Applications of Pyrheliometer: ..........................................................................13
8. Characteristics of Pyrheliometer: ......................................................................16
9. References ..........................................................................................................16
1. Objective ............................................................................................................17
2. Literature Review ..............................................................................................17
2.1. Declination: ...................................................................................................18
2.2. Solar elevation ..............................................................................................19
Zenith angle ..........................................................................................................19
Solar Azimuth Angle: ...........................................................................................19
3. Procedure: ..........................................................................................................20
4. Graphs ................................................................................................................21
5. Conclusion .........................................................................................................21
6. Advantages: .......................................................................................................22
7. Disadvantages: ...................................................................................................22
Table of Contents
1. Objective: ............................................................................................................. 8
2. Experimental Setup: ............................................................................................. 8
3. Literature Review ................................................................................................ 9
3.1. Pyrheliometer: ................................................................................................. 9
3.2. Main Components of Pyrheliometer: ............................................................. 9
3.3. Pyrheliometer a Solar Irriadiance Sensor: ....................................................10
3.4. Difference between Pyrheliometer & Pyranometer: ....................................10
3.5. Direct Normal Irradiance (DNI): ..................................................................11
3.6. Global Horizontal Irradiance (GHI): ............................................................11
4. Working of a Pyrheliometer: .............................................................................11
5. Procedure: ..........................................................................................................12
6. Advantages of Pyrheliometer: ...........................................................................13
7. Applications of Pyrheliometer: ..........................................................................13
8. Characteristics of Pyrheliometer: ......................................................................16
9. References ..........................................................................................................16
1. Objective ............................................................................................................17
2. Literature Review ..............................................................................................17
2.1. Declination: ...................................................................................................18
2.2. Solar elevation ..............................................................................................19
Zenith angle ..........................................................................................................19
Solar Azimuth Angle: ...........................................................................................19
3. Procedure: ..........................................................................................................20
4. Graphs ................................................................................................................21
5. Conclusion .........................................................................................................21
6. Advantages: .......................................................................................................22
7. Disadvantages: ...................................................................................................22
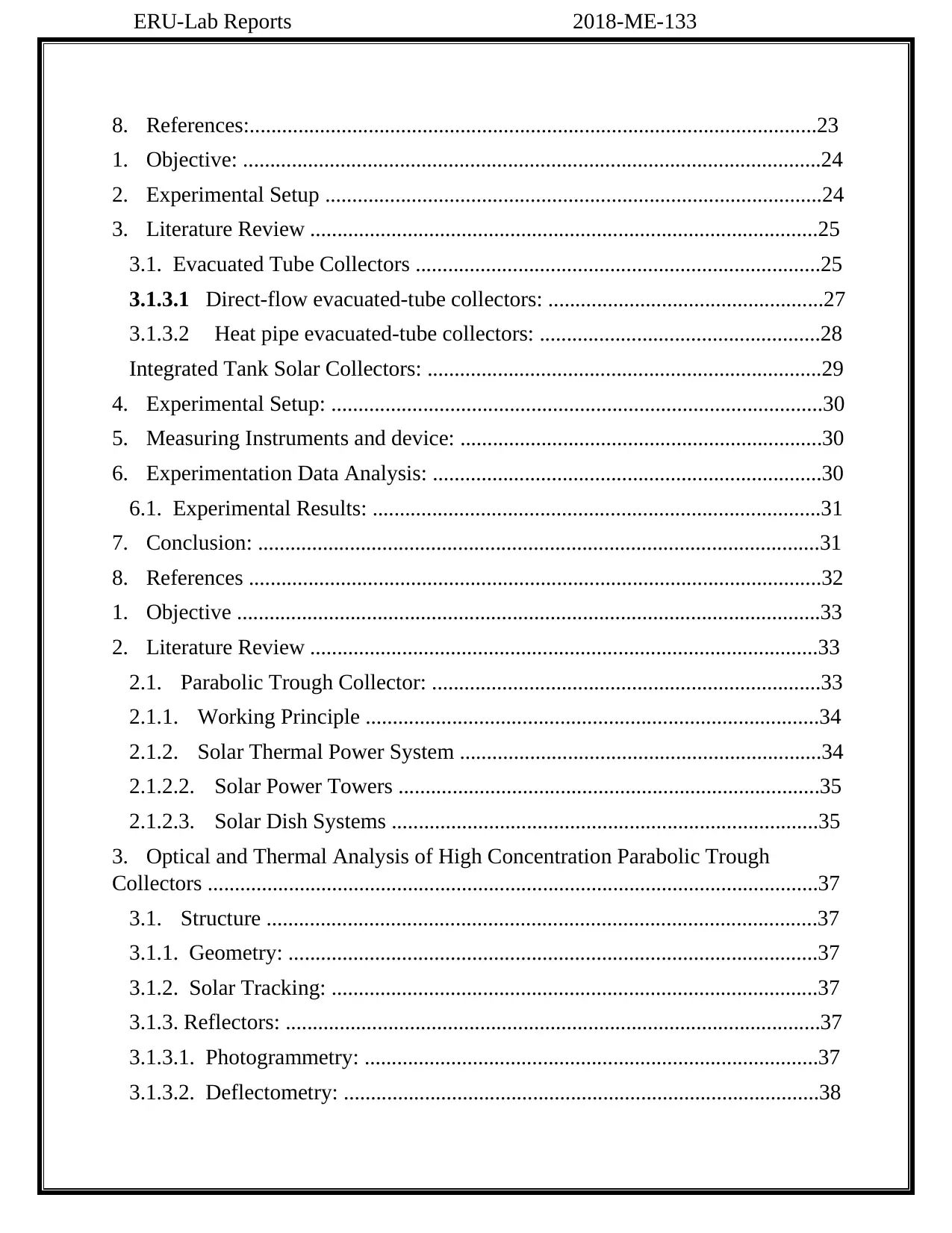
ERU-Lab Reports 2018-ME-133
8. References:.........................................................................................................23
1. Objective: ...........................................................................................................24
2. Experimental Setup ............................................................................................24
3. Literature Review ..............................................................................................25
3.1. Evacuated Tube Collectors ...........................................................................25
3.1.3.1 Direct-flow evacuated-tube collectors: ...................................................27
3.1.3.2 Heat pipe evacuated-tube collectors: ....................................................28
Integrated Tank Solar Collectors: .........................................................................29
4. Experimental Setup: ...........................................................................................30
5. Measuring Instruments and device: ...................................................................30
6. Experimentation Data Analysis: ........................................................................30
6.1. Experimental Results: ...................................................................................31
7. Conclusion: ........................................................................................................31
8. References ..........................................................................................................32
1. Objective ............................................................................................................33
2. Literature Review ..............................................................................................33
2.1. Parabolic Trough Collector: ........................................................................33
2.1.1. Working Principle ....................................................................................34
2.1.2. Solar Thermal Power System ...................................................................34
2.1.2.2. Solar Power Towers ..............................................................................35
2.1.2.3. Solar Dish Systems ...............................................................................35
3. Optical and Thermal Analysis of High Concentration Parabolic Trough
Collectors .................................................................................................................37
3.1. Structure ......................................................................................................37
3.1.1. Geometry: ..................................................................................................37
3.1.2. Solar Tracking: ..........................................................................................37
3.1.3. Reflectors: ...................................................................................................37
3.1.3.1. Photogrammetry: ....................................................................................37
3.1.3.2. Deflectometry: ........................................................................................38
8. References:.........................................................................................................23
1. Objective: ...........................................................................................................24
2. Experimental Setup ............................................................................................24
3. Literature Review ..............................................................................................25
3.1. Evacuated Tube Collectors ...........................................................................25
3.1.3.1 Direct-flow evacuated-tube collectors: ...................................................27
3.1.3.2 Heat pipe evacuated-tube collectors: ....................................................28
Integrated Tank Solar Collectors: .........................................................................29
4. Experimental Setup: ...........................................................................................30
5. Measuring Instruments and device: ...................................................................30
6. Experimentation Data Analysis: ........................................................................30
6.1. Experimental Results: ...................................................................................31
7. Conclusion: ........................................................................................................31
8. References ..........................................................................................................32
1. Objective ............................................................................................................33
2. Literature Review ..............................................................................................33
2.1. Parabolic Trough Collector: ........................................................................33
2.1.1. Working Principle ....................................................................................34
2.1.2. Solar Thermal Power System ...................................................................34
2.1.2.2. Solar Power Towers ..............................................................................35
2.1.2.3. Solar Dish Systems ...............................................................................35
3. Optical and Thermal Analysis of High Concentration Parabolic Trough
Collectors .................................................................................................................37
3.1. Structure ......................................................................................................37
3.1.1. Geometry: ..................................................................................................37
3.1.2. Solar Tracking: ..........................................................................................37
3.1.3. Reflectors: ...................................................................................................37
3.1.3.1. Photogrammetry: ....................................................................................37
3.1.3.2. Deflectometry: ........................................................................................38
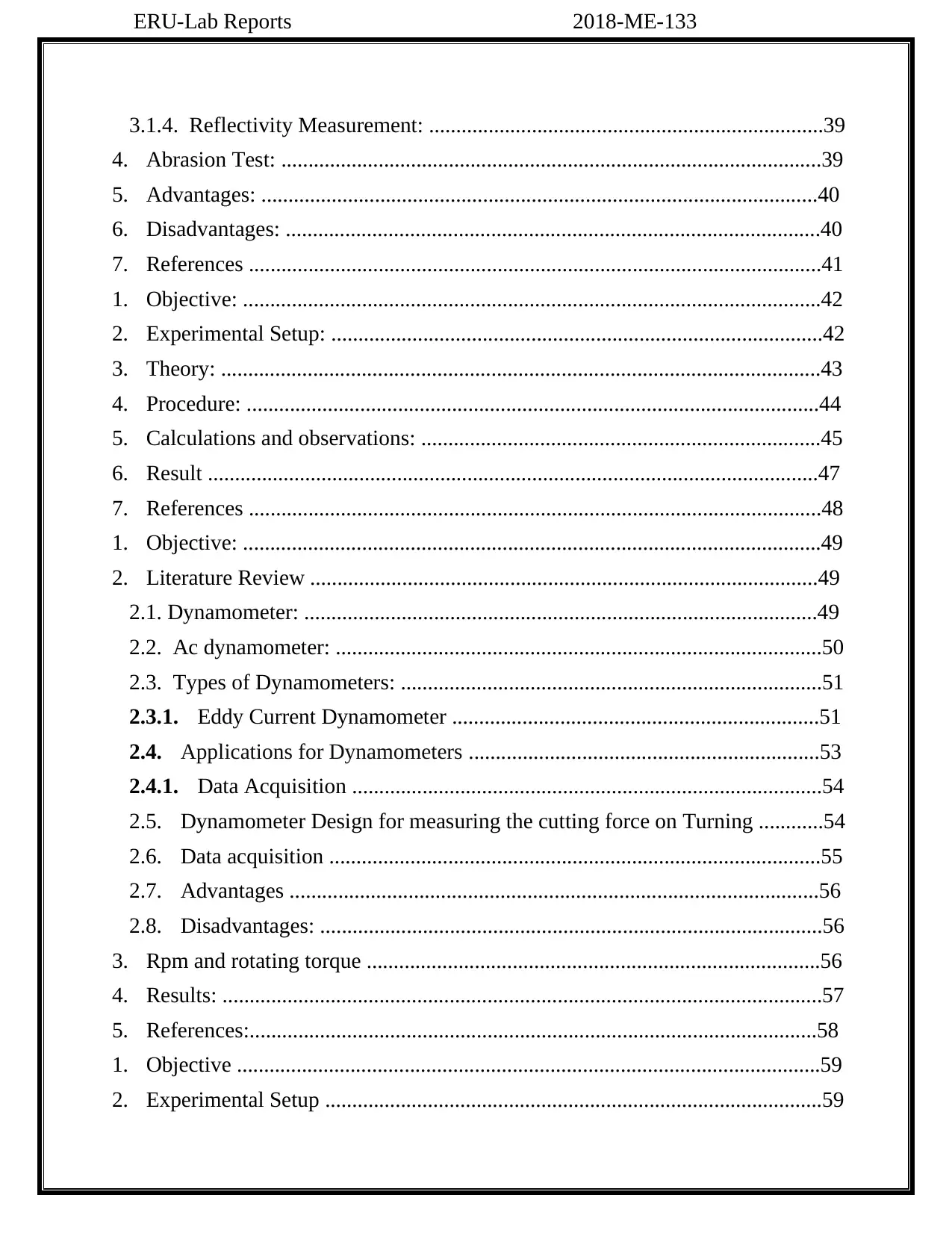
ERU-Lab Reports 2018-ME-133
3.1.4. Reflectivity Measurement: .........................................................................39
4. Abrasion Test: ....................................................................................................39
5. Advantages: .......................................................................................................40
6. Disadvantages: ...................................................................................................40
7. References ..........................................................................................................41
1. Objective: ...........................................................................................................42
2. Experimental Setup: ...........................................................................................42
3. Theory: ...............................................................................................................43
4. Procedure: ..........................................................................................................44
5. Calculations and observations: ..........................................................................45
6. Result .................................................................................................................47
7. References ..........................................................................................................48
1. Objective: ...........................................................................................................49
2. Literature Review ..............................................................................................49
2.1. Dynamometer: ...............................................................................................49
2.2. Ac dynamometer: ..........................................................................................50
2.3. Types of Dynamometers: ..............................................................................51
2.3.1. Eddy Current Dynamometer ....................................................................51
2.4. Applications for Dynamometers .................................................................53
2.4.1. Data Acquisition .......................................................................................54
2.5. Dynamometer Design for measuring the cutting force on Turning ............54
2.6. Data acquisition ...........................................................................................55
2.7. Advantages ..................................................................................................56
2.8. Disadvantages: .............................................................................................56
3. Rpm and rotating torque ....................................................................................56
4. Results: ...............................................................................................................57
5. References:.........................................................................................................58
1. Objective ............................................................................................................59
2. Experimental Setup ............................................................................................59
3.1.4. Reflectivity Measurement: .........................................................................39
4. Abrasion Test: ....................................................................................................39
5. Advantages: .......................................................................................................40
6. Disadvantages: ...................................................................................................40
7. References ..........................................................................................................41
1. Objective: ...........................................................................................................42
2. Experimental Setup: ...........................................................................................42
3. Theory: ...............................................................................................................43
4. Procedure: ..........................................................................................................44
5. Calculations and observations: ..........................................................................45
6. Result .................................................................................................................47
7. References ..........................................................................................................48
1. Objective: ...........................................................................................................49
2. Literature Review ..............................................................................................49
2.1. Dynamometer: ...............................................................................................49
2.2. Ac dynamometer: ..........................................................................................50
2.3. Types of Dynamometers: ..............................................................................51
2.3.1. Eddy Current Dynamometer ....................................................................51
2.4. Applications for Dynamometers .................................................................53
2.4.1. Data Acquisition .......................................................................................54
2.5. Dynamometer Design for measuring the cutting force on Turning ............54
2.6. Data acquisition ...........................................................................................55
2.7. Advantages ..................................................................................................56
2.8. Disadvantages: .............................................................................................56
3. Rpm and rotating torque ....................................................................................56
4. Results: ...............................................................................................................57
5. References:.........................................................................................................58
1. Objective ............................................................................................................59
2. Experimental Setup ............................................................................................59
Secure Best Marks with AI Grader
Need help grading? Try our AI Grader for instant feedback on your assignments.
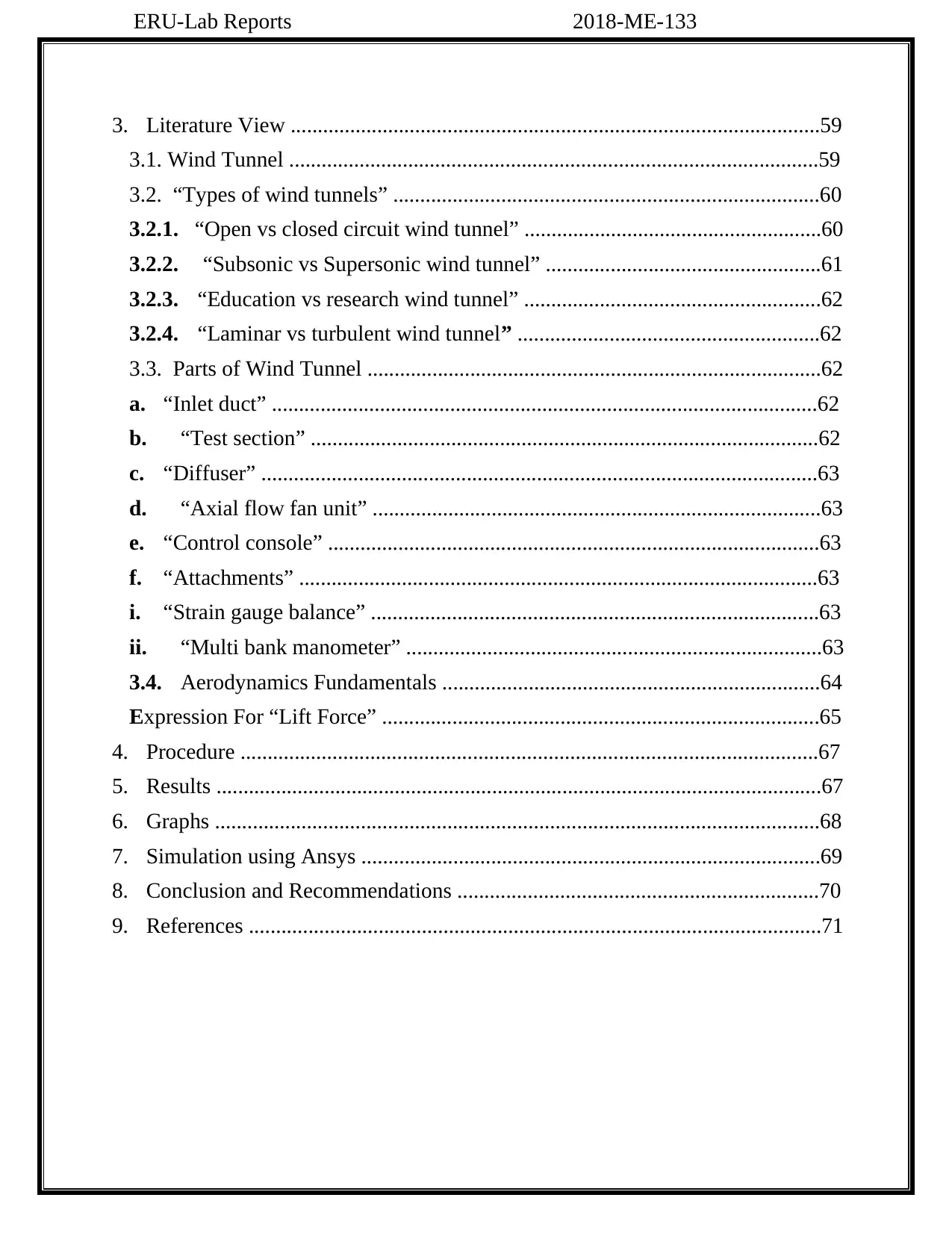
ERU-Lab Reports 2018-ME-133
3. Literature View ..................................................................................................59
3.1. Wind Tunnel ..................................................................................................59
3.2. “Types of wind tunnels” ...............................................................................60
3.2.1. “Open vs closed circuit wind tunnel” .......................................................60
3.2.2. “Subsonic vs Supersonic wind tunnel” ...................................................61
3.2.3. “Education vs research wind tunnel” .......................................................62
3.2.4. “Laminar vs turbulent wind tunnel” ........................................................62
3.3. Parts of Wind Tunnel ....................................................................................62
a. “Inlet duct” .....................................................................................................62
b. “Test section” ..............................................................................................62
c. “Diffuser” .......................................................................................................63
d. “Axial flow fan unit” ...................................................................................63
e. “Control console” ...........................................................................................63
f. “Attachments” ................................................................................................63
i. “Strain gauge balance” ...................................................................................63
ii. “Multi bank manometer” .............................................................................63
3.4. Aerodynamics Fundamentals ......................................................................64
Expression For “Lift Force” .................................................................................65
4. Procedure ...........................................................................................................67
5. Results ................................................................................................................67
6. Graphs ................................................................................................................68
7. Simulation using Ansys .....................................................................................69
8. Conclusion and Recommendations ...................................................................70
9. References ..........................................................................................................71
3. Literature View ..................................................................................................59
3.1. Wind Tunnel ..................................................................................................59
3.2. “Types of wind tunnels” ...............................................................................60
3.2.1. “Open vs closed circuit wind tunnel” .......................................................60
3.2.2. “Subsonic vs Supersonic wind tunnel” ...................................................61
3.2.3. “Education vs research wind tunnel” .......................................................62
3.2.4. “Laminar vs turbulent wind tunnel” ........................................................62
3.3. Parts of Wind Tunnel ....................................................................................62
a. “Inlet duct” .....................................................................................................62
b. “Test section” ..............................................................................................62
c. “Diffuser” .......................................................................................................63
d. “Axial flow fan unit” ...................................................................................63
e. “Control console” ...........................................................................................63
f. “Attachments” ................................................................................................63
i. “Strain gauge balance” ...................................................................................63
ii. “Multi bank manometer” .............................................................................63
3.4. Aerodynamics Fundamentals ......................................................................64
Expression For “Lift Force” .................................................................................65
4. Procedure ...........................................................................................................67
5. Results ................................................................................................................67
6. Graphs ................................................................................................................68
7. Simulation using Ansys .....................................................................................69
8. Conclusion and Recommendations ...................................................................70
9. References ..........................................................................................................71
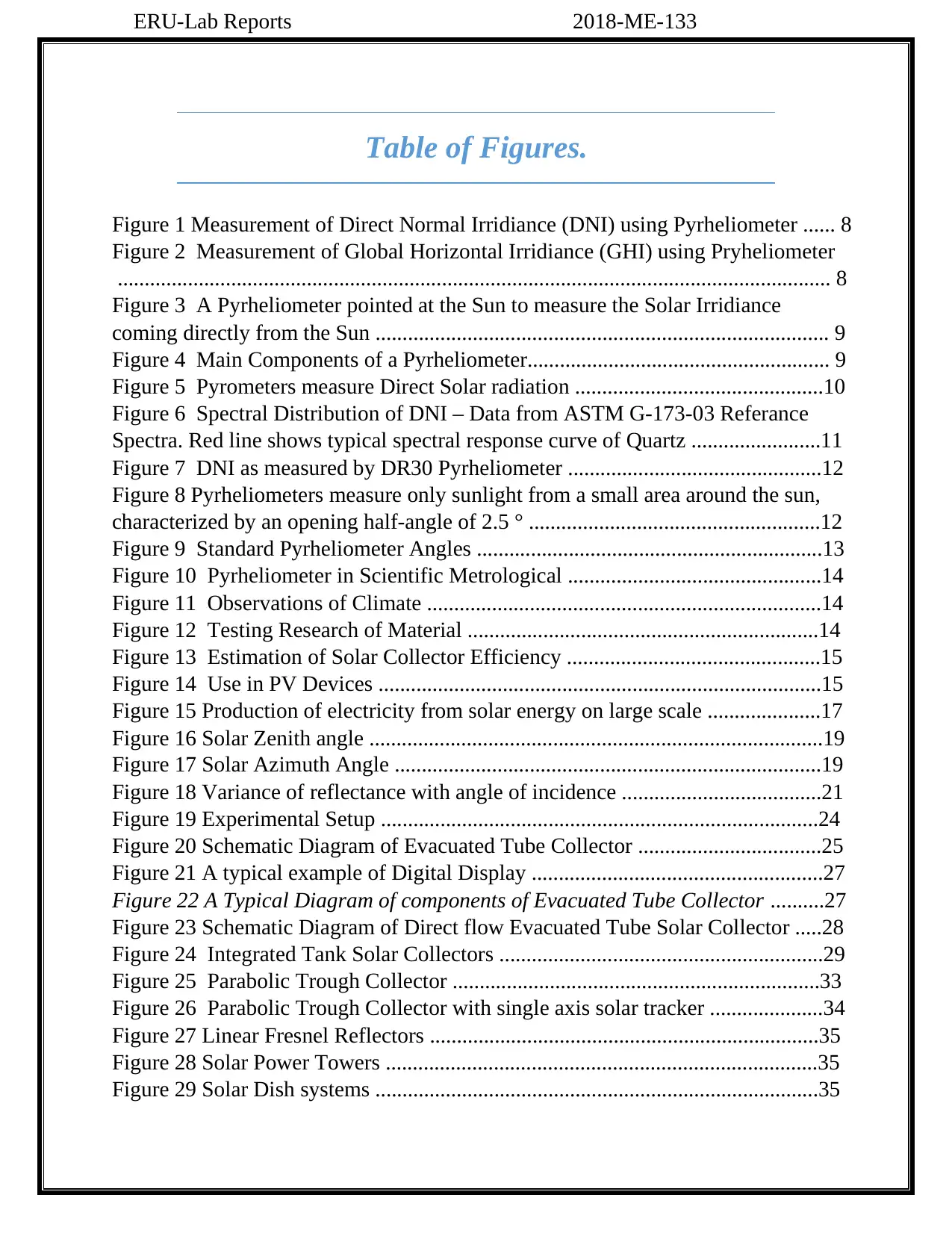
ERU-Lab Reports 2018-ME-133
Table of Figures.
Figure 1 Measurement of Direct Normal Irridiance (DNI) using Pyrheliometer ...... 8
Figure 2 Measurement of Global Horizontal Irridiance (GHI) using Pryheliometer
.................................................................................................................................... 8
Figure 3 A Pyrheliometer pointed at the Sun to measure the Solar Irridiance
coming directly from the Sun .................................................................................... 9
Figure 4 Main Components of a Pyrheliometer........................................................ 9
Figure 5 Pyrometers measure Direct Solar radiation ..............................................10
Figure 6 Spectral Distribution of DNI – Data from ASTM G-173-03 Referance
Spectra. Red line shows typical spectral response curve of Quartz ........................11
Figure 7 DNI as measured by DR30 Pyrheliometer ...............................................12
Figure 8 Pyrheliometers measure only sunlight from a small area around the sun,
characterized by an opening half-angle of 2.5 ° ......................................................12
Figure 9 Standard Pyrheliometer Angles ................................................................13
Figure 10 Pyrheliometer in Scientific Metrological ...............................................14
Figure 11 Observations of Climate .........................................................................14
Figure 12 Testing Research of Material .................................................................14
Figure 13 Estimation of Solar Collector Efficiency ...............................................15
Figure 14 Use in PV Devices ..................................................................................15
Figure 15 Production of electricity from solar energy on large scale .....................17
Figure 16 Solar Zenith angle ....................................................................................19
Figure 17 Solar Azimuth Angle ...............................................................................19
Figure 18 Variance of reflectance with angle of incidence .....................................21
Figure 19 Experimental Setup .................................................................................24
Figure 20 Schematic Diagram of Evacuated Tube Collector ..................................25
Figure 21 A typical example of Digital Display ......................................................27
Figure 22 A Typical Diagram of components of Evacuated Tube Collector ..........27
Figure 23 Schematic Diagram of Direct flow Evacuated Tube Solar Collector .....28
Figure 24 Integrated Tank Solar Collectors ............................................................29
Figure 25 Parabolic Trough Collector ....................................................................33
Figure 26 Parabolic Trough Collector with single axis solar tracker .....................34
Figure 27 Linear Fresnel Reflectors ........................................................................35
Figure 28 Solar Power Towers ................................................................................35
Figure 29 Solar Dish systems ..................................................................................35
Table of Figures.
Figure 1 Measurement of Direct Normal Irridiance (DNI) using Pyrheliometer ...... 8
Figure 2 Measurement of Global Horizontal Irridiance (GHI) using Pryheliometer
.................................................................................................................................... 8
Figure 3 A Pyrheliometer pointed at the Sun to measure the Solar Irridiance
coming directly from the Sun .................................................................................... 9
Figure 4 Main Components of a Pyrheliometer........................................................ 9
Figure 5 Pyrometers measure Direct Solar radiation ..............................................10
Figure 6 Spectral Distribution of DNI – Data from ASTM G-173-03 Referance
Spectra. Red line shows typical spectral response curve of Quartz ........................11
Figure 7 DNI as measured by DR30 Pyrheliometer ...............................................12
Figure 8 Pyrheliometers measure only sunlight from a small area around the sun,
characterized by an opening half-angle of 2.5 ° ......................................................12
Figure 9 Standard Pyrheliometer Angles ................................................................13
Figure 10 Pyrheliometer in Scientific Metrological ...............................................14
Figure 11 Observations of Climate .........................................................................14
Figure 12 Testing Research of Material .................................................................14
Figure 13 Estimation of Solar Collector Efficiency ...............................................15
Figure 14 Use in PV Devices ..................................................................................15
Figure 15 Production of electricity from solar energy on large scale .....................17
Figure 16 Solar Zenith angle ....................................................................................19
Figure 17 Solar Azimuth Angle ...............................................................................19
Figure 18 Variance of reflectance with angle of incidence .....................................21
Figure 19 Experimental Setup .................................................................................24
Figure 20 Schematic Diagram of Evacuated Tube Collector ..................................25
Figure 21 A typical example of Digital Display ......................................................27
Figure 22 A Typical Diagram of components of Evacuated Tube Collector ..........27
Figure 23 Schematic Diagram of Direct flow Evacuated Tube Solar Collector .....28
Figure 24 Integrated Tank Solar Collectors ............................................................29
Figure 25 Parabolic Trough Collector ....................................................................33
Figure 26 Parabolic Trough Collector with single axis solar tracker .....................34
Figure 27 Linear Fresnel Reflectors ........................................................................35
Figure 28 Solar Power Towers ................................................................................35
Figure 29 Solar Dish systems ..................................................................................35
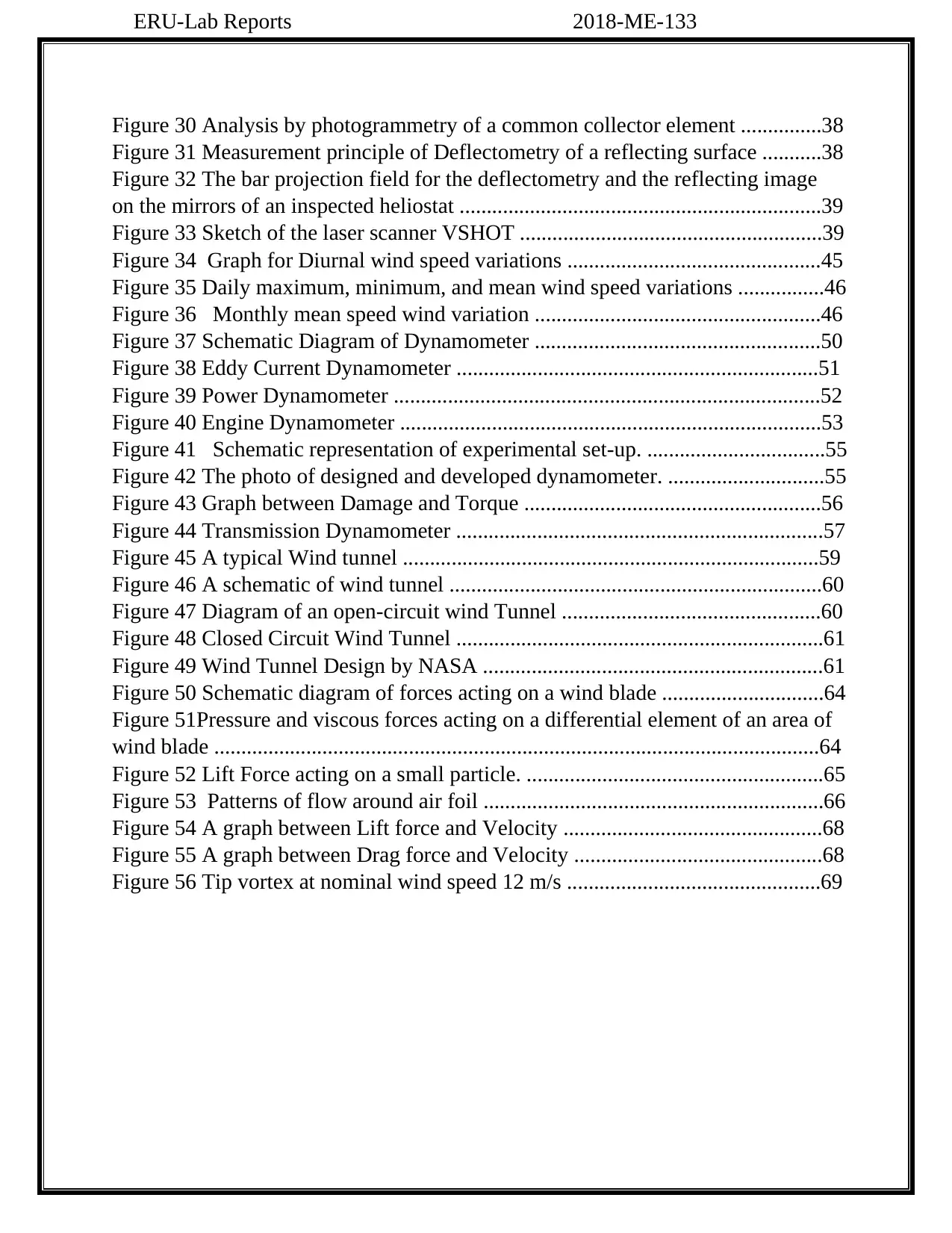
ERU-Lab Reports 2018-ME-133
Figure 30 Analysis by photogrammetry of a common collector element ...............38
Figure 31 Measurement principle of Deflectometry of a reflecting surface ...........38
Figure 32 The bar projection field for the deflectometry and the reflecting image
on the mirrors of an inspected heliostat ...................................................................39
Figure 33 Sketch of the laser scanner VSHOT ........................................................39
Figure 34 Graph for Diurnal wind speed variations ...............................................45
Figure 35 Daily maximum, minimum, and mean wind speed variations ................46
Figure 36 Monthly mean speed wind variation .....................................................46
Figure 37 Schematic Diagram of Dynamometer .....................................................50
Figure 38 Eddy Current Dynamometer ...................................................................51
Figure 39 Power Dynamometer ...............................................................................52
Figure 40 Engine Dynamometer ..............................................................................53
Figure 41 Schematic representation of experimental set-up. .................................55
Figure 42 The photo of designed and developed dynamometer. .............................55
Figure 43 Graph between Damage and Torque .......................................................56
Figure 44 Transmission Dynamometer ....................................................................57
Figure 45 A typical Wind tunnel .............................................................................59
Figure 46 A schematic of wind tunnel .....................................................................60
Figure 47 Diagram of an open-circuit wind Tunnel ................................................60
Figure 48 Closed Circuit Wind Tunnel ....................................................................61
Figure 49 Wind Tunnel Design by NASA ...............................................................61
Figure 50 Schematic diagram of forces acting on a wind blade ..............................64
Figure 51Pressure and viscous forces acting on a differential element of an area of
wind blade ................................................................................................................64
Figure 52 Lift Force acting on a small particle. .......................................................65
Figure 53 Patterns of flow around air foil ...............................................................66
Figure 54 A graph between Lift force and Velocity ................................................68
Figure 55 A graph between Drag force and Velocity ..............................................68
Figure 56 Tip vortex at nominal wind speed 12 m/s ...............................................69
University of Engineering & Technology, Lahore
Figure 30 Analysis by photogrammetry of a common collector element ...............38
Figure 31 Measurement principle of Deflectometry of a reflecting surface ...........38
Figure 32 The bar projection field for the deflectometry and the reflecting image
on the mirrors of an inspected heliostat ...................................................................39
Figure 33 Sketch of the laser scanner VSHOT ........................................................39
Figure 34 Graph for Diurnal wind speed variations ...............................................45
Figure 35 Daily maximum, minimum, and mean wind speed variations ................46
Figure 36 Monthly mean speed wind variation .....................................................46
Figure 37 Schematic Diagram of Dynamometer .....................................................50
Figure 38 Eddy Current Dynamometer ...................................................................51
Figure 39 Power Dynamometer ...............................................................................52
Figure 40 Engine Dynamometer ..............................................................................53
Figure 41 Schematic representation of experimental set-up. .................................55
Figure 42 The photo of designed and developed dynamometer. .............................55
Figure 43 Graph between Damage and Torque .......................................................56
Figure 44 Transmission Dynamometer ....................................................................57
Figure 45 A typical Wind tunnel .............................................................................59
Figure 46 A schematic of wind tunnel .....................................................................60
Figure 47 Diagram of an open-circuit wind Tunnel ................................................60
Figure 48 Closed Circuit Wind Tunnel ....................................................................61
Figure 49 Wind Tunnel Design by NASA ...............................................................61
Figure 50 Schematic diagram of forces acting on a wind blade ..............................64
Figure 51Pressure and viscous forces acting on a differential element of an area of
wind blade ................................................................................................................64
Figure 52 Lift Force acting on a small particle. .......................................................65
Figure 53 Patterns of flow around air foil ...............................................................66
Figure 54 A graph between Lift force and Velocity ................................................68
Figure 55 A graph between Drag force and Velocity ..............................................68
Figure 56 Tip vortex at nominal wind speed 12 m/s ...............................................69
University of Engineering & Technology, Lahore
Paraphrase This Document
Need a fresh take? Get an instant paraphrase of this document with our AI Paraphraser
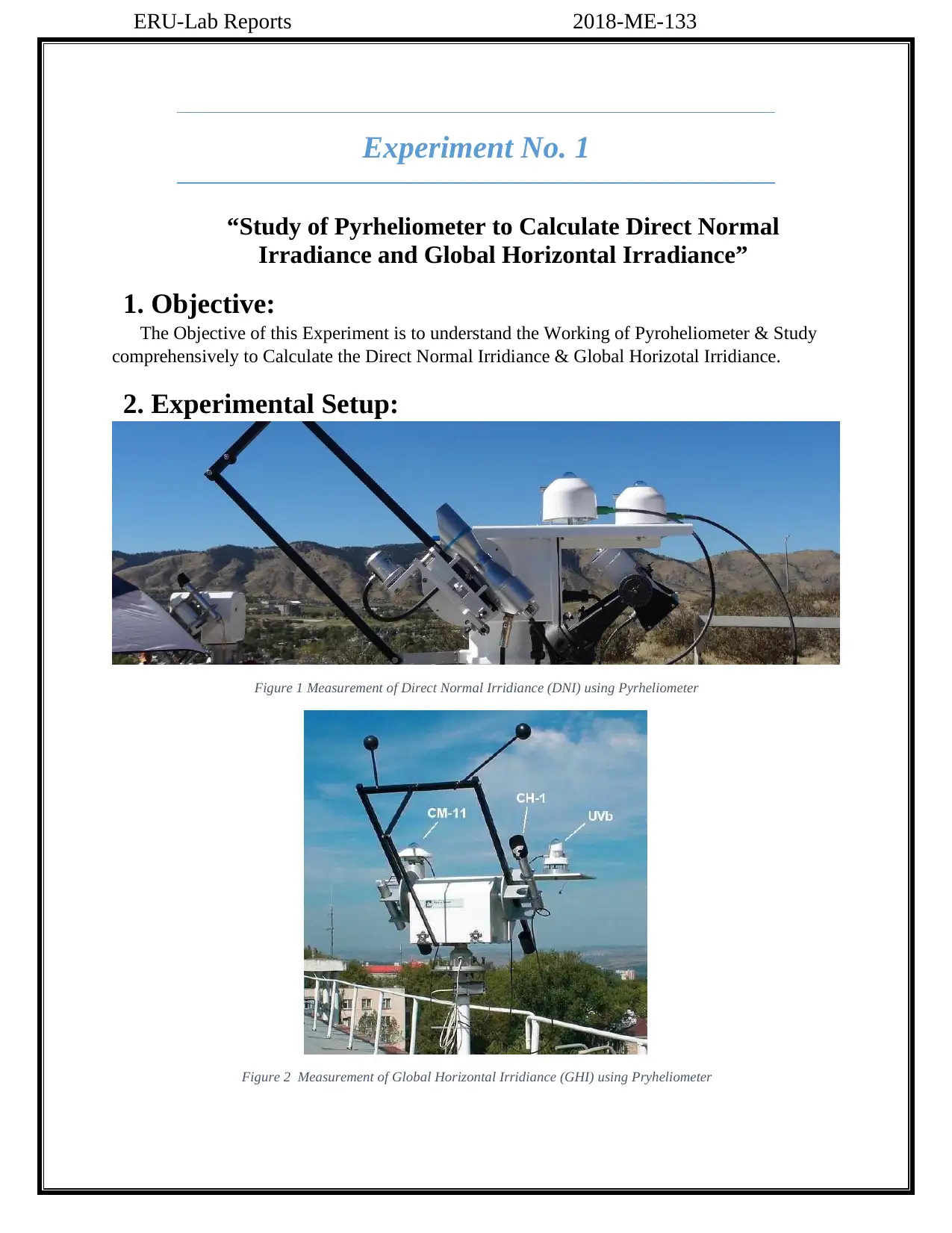
ERU-Lab Reports 2018-ME-133
Experiment No. 1
“Study of Pyrheliometer to Calculate Direct Normal
Irradiance and Global Horizontal Irradiance”
1. Objective:
The Objective of this Experiment is to understand the Working of Pyroheliometer & Study
comprehensively to Calculate the Direct Normal Irridiance & Global Horizotal Irridiance.
2. Experimental Setup:
Figure 1 Measurement of Direct Normal Irridiance (DNI) using Pyrheliometer
Figure 2 Measurement of Global Horizontal Irridiance (GHI) using Pryheliometer
Experiment No. 1
“Study of Pyrheliometer to Calculate Direct Normal
Irradiance and Global Horizontal Irradiance”
1. Objective:
The Objective of this Experiment is to understand the Working of Pyroheliometer & Study
comprehensively to Calculate the Direct Normal Irridiance & Global Horizotal Irridiance.
2. Experimental Setup:
Figure 1 Measurement of Direct Normal Irridiance (DNI) using Pyrheliometer
Figure 2 Measurement of Global Horizontal Irridiance (GHI) using Pryheliometer
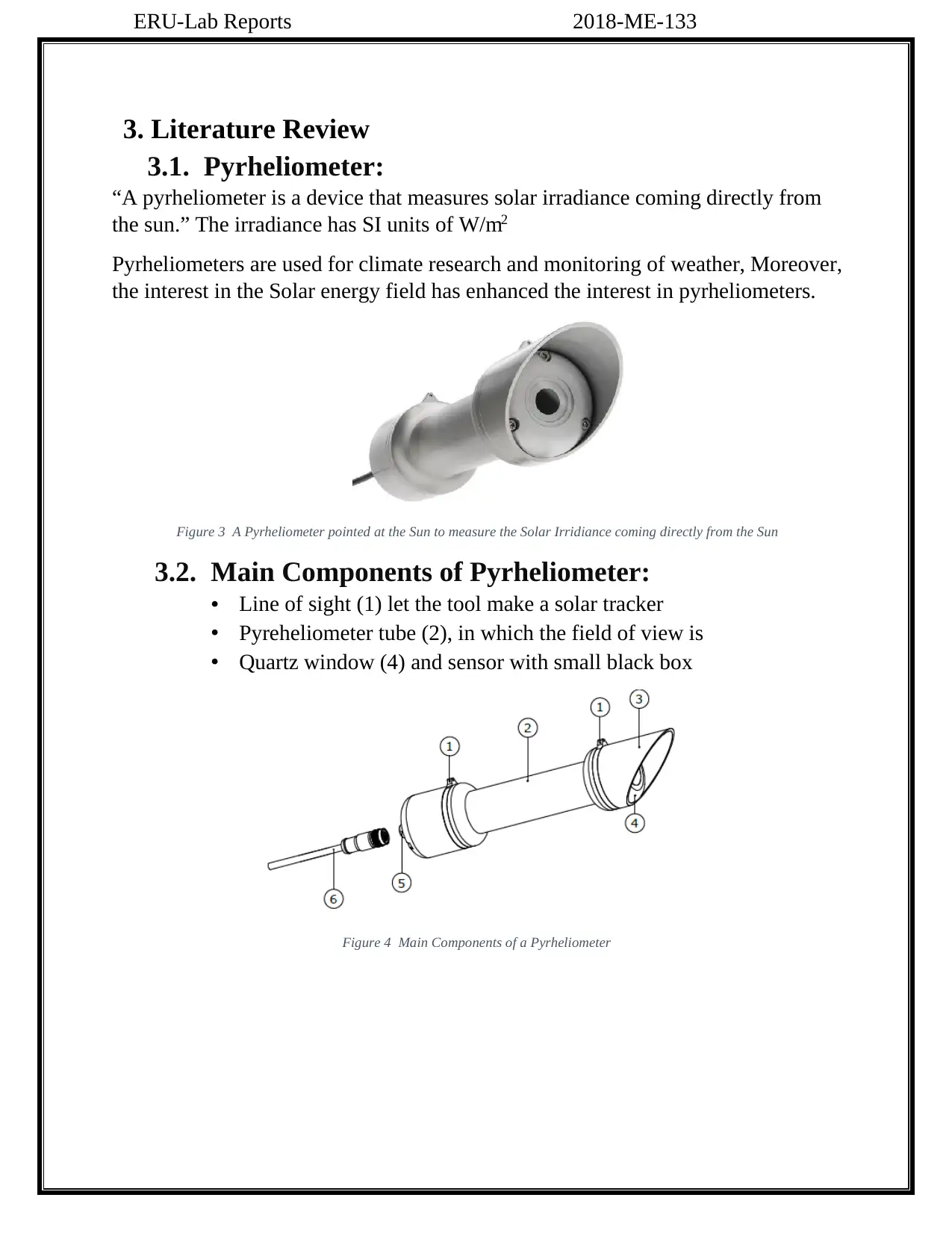
ERU-Lab Reports 2018-ME-133
3. Literature Review
3.1. Pyrheliometer:
“A pyrheliometer is a device that measures solar irradiance coming directly from
the sun.” The irradiance has SI units of W/m2
Pyrheliometers are used for climate research and monitoring of weather, Moreover,
the interest in the Solar energy field has enhanced the interest in pyrheliometers.
Figure 3 A Pyrheliometer pointed at the Sun to measure the Solar Irridiance coming directly from the Sun
3.2. Main Components of Pyrheliometer:
• Line of sight (1) let the tool make a solar tracker
• Pyreheliometer tube (2), in which the field of view is
• Quartz window (4) and sensor with small black box
Figure 4 Main Components of a Pyrheliometer
3. Literature Review
3.1. Pyrheliometer:
“A pyrheliometer is a device that measures solar irradiance coming directly from
the sun.” The irradiance has SI units of W/m2
Pyrheliometers are used for climate research and monitoring of weather, Moreover,
the interest in the Solar energy field has enhanced the interest in pyrheliometers.
Figure 3 A Pyrheliometer pointed at the Sun to measure the Solar Irridiance coming directly from the Sun
3.2. Main Components of Pyrheliometer:
• Line of sight (1) let the tool make a solar tracker
• Pyreheliometer tube (2), in which the field of view is
• Quartz window (4) and sensor with small black box
Figure 4 Main Components of a Pyrheliometer
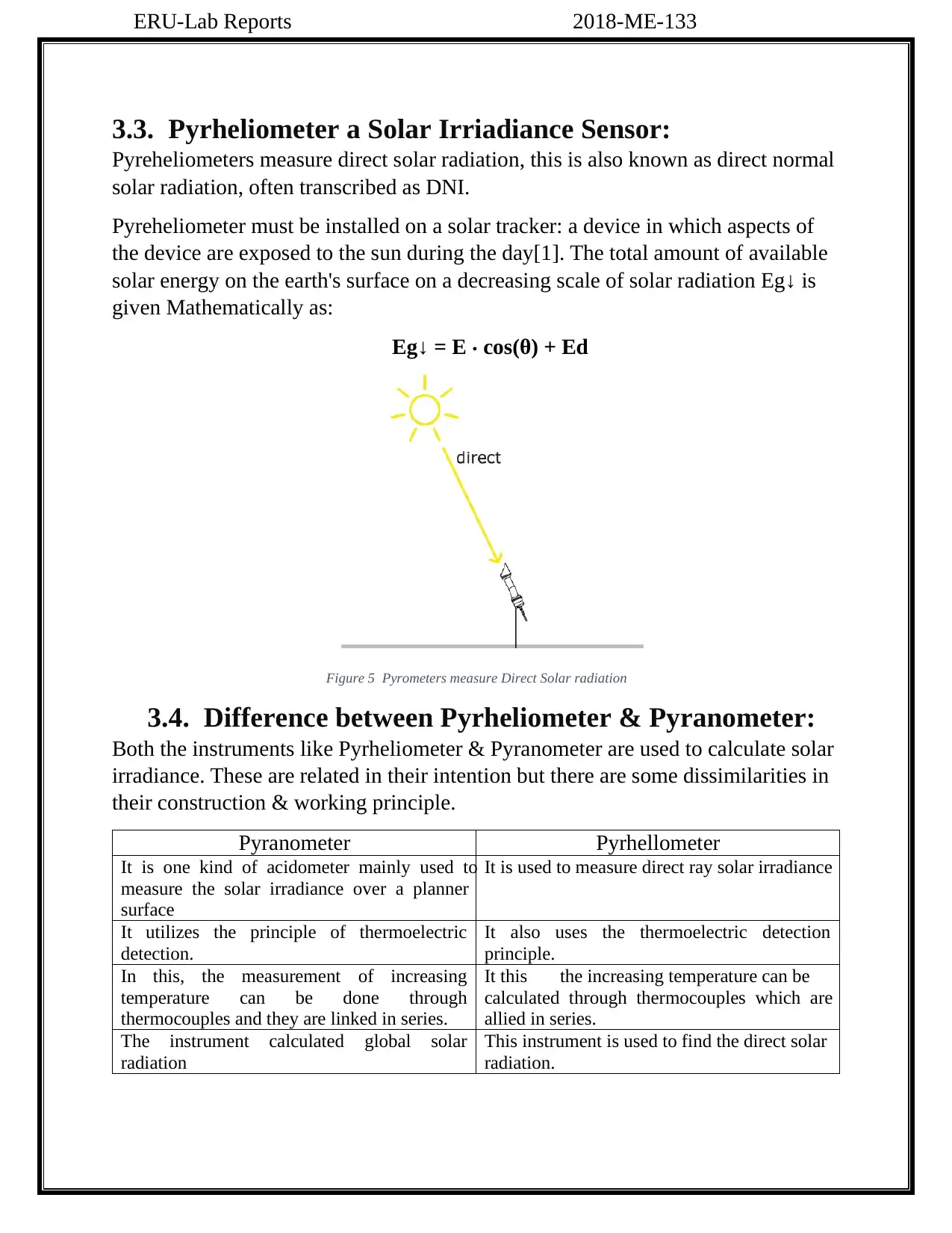
ERU-Lab Reports 2018-ME-133
3.3. Pyrheliometer a Solar Irriadiance Sensor:
Pyreheliometers measure direct solar radiation, this is also known as direct normal
solar radiation, often transcribed as DNI.
Pyreheliometer must be installed on a solar tracker: a device in which aspects of
the device are exposed to the sun during the day[1]. The total amount of available
solar energy on the earth's surface on a decreasing scale of solar radiation Eg↓ is
given Mathematically as:
Eg↓ = E ⋅ cos(θ) + Ed
Figure 5 Pyrometers measure Direct Solar radiation
3.4. Difference between Pyrheliometer & Pyranometer:
Both the instruments like Pyrheliometer & Pyranometer are used to calculate solar
irradiance. These are related in their intention but there are some dissimilarities in
their construction & working principle.
Pyranometer Pyrhellometer
It is one kind of acidometer mainly used to
measure the solar irradiance over a planner
surface
It is used to measure direct ray solar irradiance
It utilizes the principle of thermoelectric
detection.
It also uses the thermoelectric detection
principle.
In this, the measurement of increasing
temperature can be done through
thermocouples and they are linked in series.
It this the increasing temperature can be
calculated through thermocouples which are
allied in series.
The instrument calculated global solar
radiation
This instrument is used to find the direct solar
radiation.
3.3. Pyrheliometer a Solar Irriadiance Sensor:
Pyreheliometers measure direct solar radiation, this is also known as direct normal
solar radiation, often transcribed as DNI.
Pyreheliometer must be installed on a solar tracker: a device in which aspects of
the device are exposed to the sun during the day[1]. The total amount of available
solar energy on the earth's surface on a decreasing scale of solar radiation Eg↓ is
given Mathematically as:
Eg↓ = E ⋅ cos(θ) + Ed
Figure 5 Pyrometers measure Direct Solar radiation
3.4. Difference between Pyrheliometer & Pyranometer:
Both the instruments like Pyrheliometer & Pyranometer are used to calculate solar
irradiance. These are related in their intention but there are some dissimilarities in
their construction & working principle.
Pyranometer Pyrhellometer
It is one kind of acidometer mainly used to
measure the solar irradiance over a planner
surface
It is used to measure direct ray solar irradiance
It utilizes the principle of thermoelectric
detection.
It also uses the thermoelectric detection
principle.
In this, the measurement of increasing
temperature can be done through
thermocouples and they are linked in series.
It this the increasing temperature can be
calculated through thermocouples which are
allied in series.
The instrument calculated global solar
radiation
This instrument is used to find the direct solar
radiation.
Secure Best Marks with AI Grader
Need help grading? Try our AI Grader for instant feedback on your assignments.
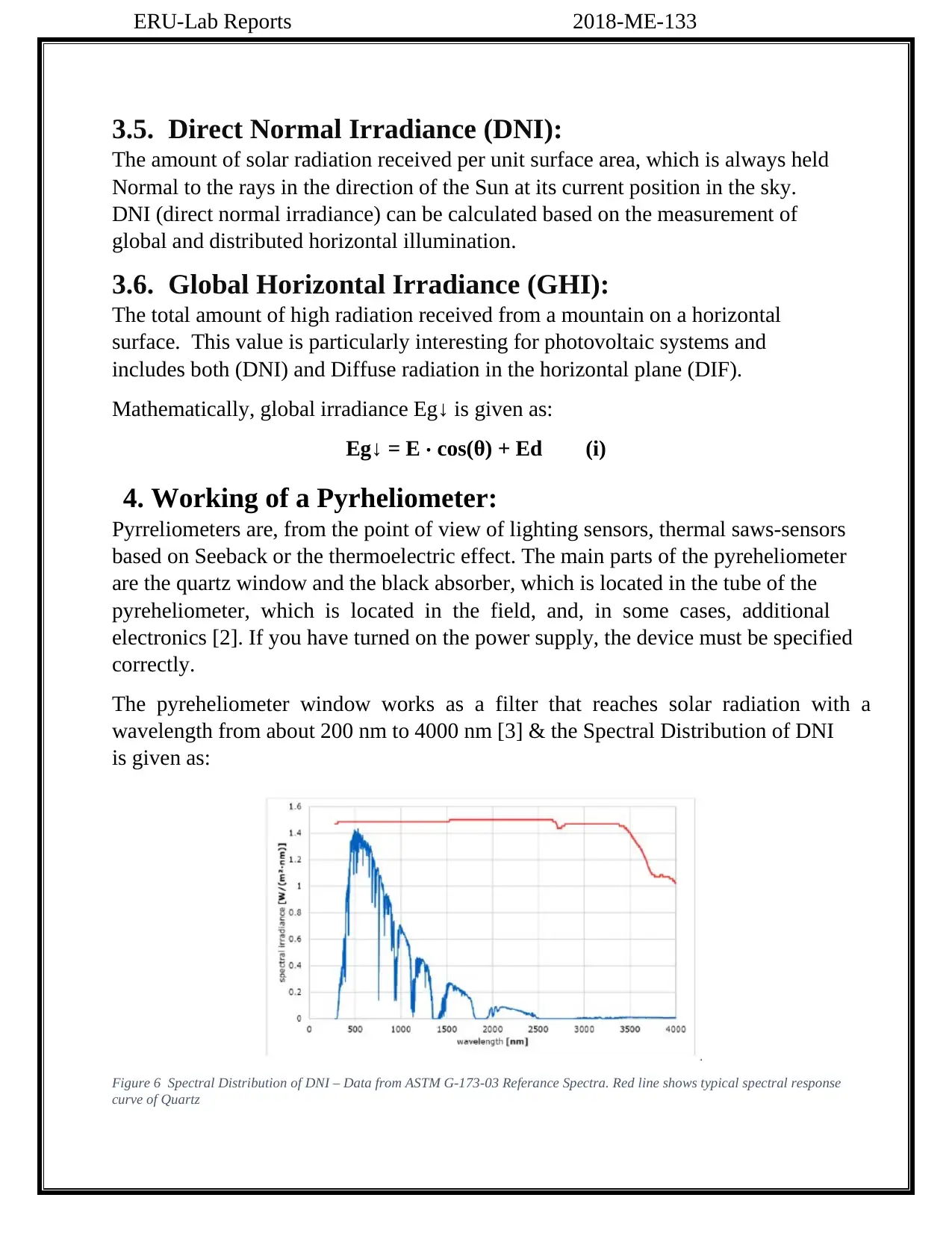
ERU-Lab Reports 2018-ME-133
3.5. Direct Normal Irradiance (DNI):
The amount of solar radiation received per unit surface area, which is always held
Normal to the rays in the direction of the Sun at its current position in the sky.
DNI (direct normal irradiance) can be calculated based on the measurement of
global and distributed horizontal illumination.
3.6. Global Horizontal Irradiance (GHI):
The total amount of high radiation received from a mountain on a horizontal
surface. This value is particularly interesting for photovoltaic systems and
includes both (DNI) and Diffuse radiation in the horizontal plane (DIF).
Mathematically, global irradiance Eg↓ is given as:
Eg↓ = E ⋅ cos(θ) + Ed (i)
4. Working of a Pyrheliometer:
Pyrreliometers are, from the point of view of lighting sensors, thermal saws-sensors
based on Seeback or the thermoelectric effect. The main parts of the pyreheliometer
are the quartz window and the black absorber, which is located in the tube of the
pyreheliometer, which is located in the field, and, in some cases, additional
electronics [2]. If you have turned on the power supply, the device must be specified
correctly.
The pyreheliometer window works as a filter that reaches solar radiation with a
wavelength from about 200 nm to 4000 nm [3] & the Spectral Distribution of DNI
is given as:
Figure 6 Spectral Distribution of DNI – Data from ASTM G-173-03 Referance Spectra. Red line shows typical spectral response
curve of Quartz
3.5. Direct Normal Irradiance (DNI):
The amount of solar radiation received per unit surface area, which is always held
Normal to the rays in the direction of the Sun at its current position in the sky.
DNI (direct normal irradiance) can be calculated based on the measurement of
global and distributed horizontal illumination.
3.6. Global Horizontal Irradiance (GHI):
The total amount of high radiation received from a mountain on a horizontal
surface. This value is particularly interesting for photovoltaic systems and
includes both (DNI) and Diffuse radiation in the horizontal plane (DIF).
Mathematically, global irradiance Eg↓ is given as:
Eg↓ = E ⋅ cos(θ) + Ed (i)
4. Working of a Pyrheliometer:
Pyrreliometers are, from the point of view of lighting sensors, thermal saws-sensors
based on Seeback or the thermoelectric effect. The main parts of the pyreheliometer
are the quartz window and the black absorber, which is located in the tube of the
pyreheliometer, which is located in the field, and, in some cases, additional
electronics [2]. If you have turned on the power supply, the device must be specified
correctly.
The pyreheliometer window works as a filter that reaches solar radiation with a
wavelength from about 200 nm to 4000 nm [3] & the Spectral Distribution of DNI
is given as:
Figure 6 Spectral Distribution of DNI – Data from ASTM G-173-03 Referance Spectra. Red line shows typical spectral response
curve of Quartz
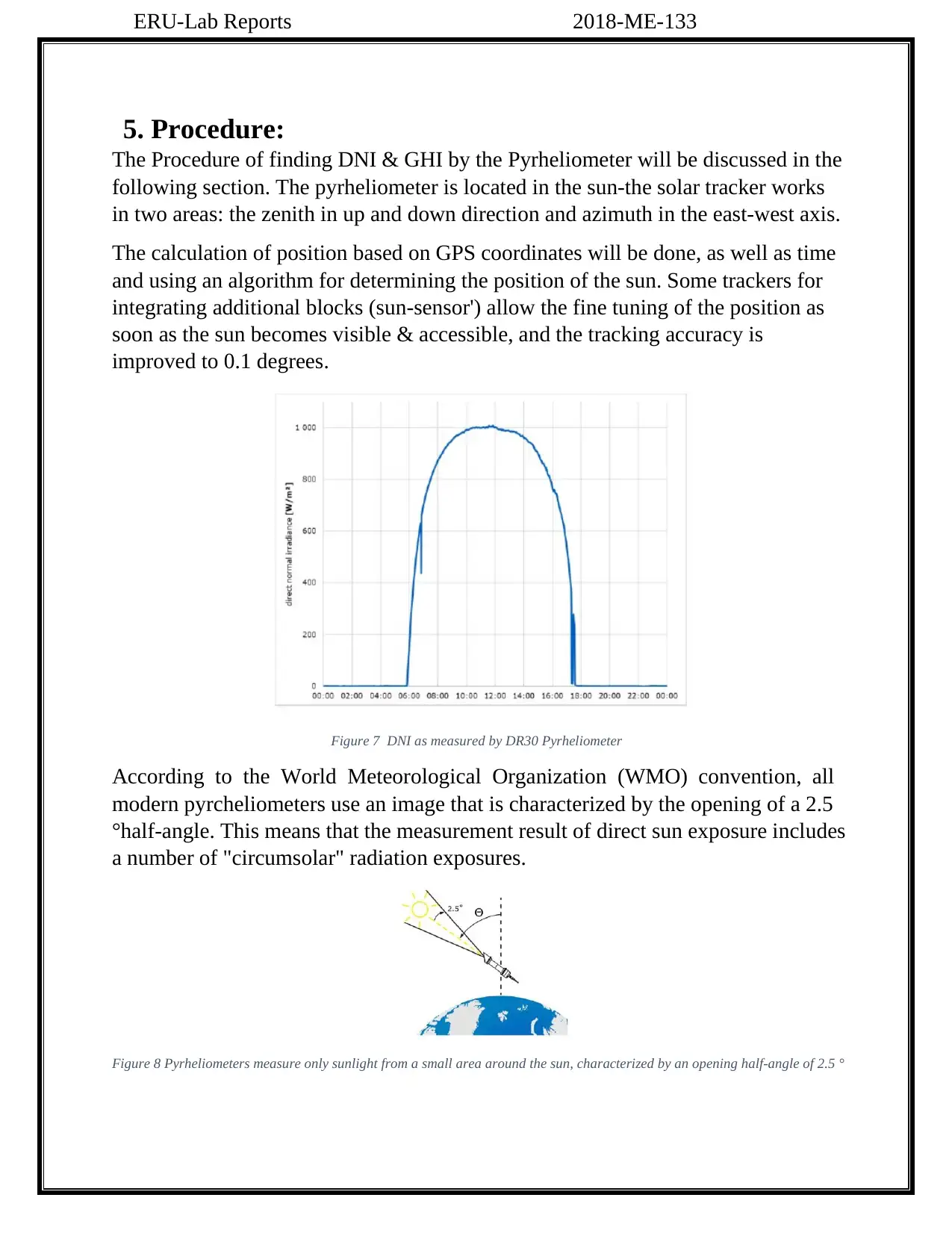
ERU-Lab Reports 2018-ME-133
5. Procedure:
The Procedure of finding DNI & GHI by the Pyrheliometer will be discussed in the
following section. The pyrheliometer is located in the sun-the solar tracker works
in two areas: the zenith in up and down direction and azimuth in the east-west axis.
The calculation of position based on GPS coordinates will be done, as well as time
and using an algorithm for determining the position of the sun. Some trackers for
integrating additional blocks (sun-sensor') allow the fine tuning of the position as
soon as the sun becomes visible & accessible, and the tracking accuracy is
improved to 0.1 degrees.
Figure 7 DNI as measured by DR30 Pyrheliometer
According to the World Meteorological Organization (WMO) convention, all
modern pyrcheliometers use an image that is characterized by the opening of a 2.5
°half-angle. This means that the measurement result of direct sun exposure includes
a number of "circumsolar" radiation exposures.
Figure 8 Pyrheliometers measure only sunlight from a small area around the sun, characterized by an opening half-angle of 2.5 °
5. Procedure:
The Procedure of finding DNI & GHI by the Pyrheliometer will be discussed in the
following section. The pyrheliometer is located in the sun-the solar tracker works
in two areas: the zenith in up and down direction and azimuth in the east-west axis.
The calculation of position based on GPS coordinates will be done, as well as time
and using an algorithm for determining the position of the sun. Some trackers for
integrating additional blocks (sun-sensor') allow the fine tuning of the position as
soon as the sun becomes visible & accessible, and the tracking accuracy is
improved to 0.1 degrees.
Figure 7 DNI as measured by DR30 Pyrheliometer
According to the World Meteorological Organization (WMO) convention, all
modern pyrcheliometers use an image that is characterized by the opening of a 2.5
°half-angle. This means that the measurement result of direct sun exposure includes
a number of "circumsolar" radiation exposures.
Figure 8 Pyrheliometers measure only sunlight from a small area around the sun, characterized by an opening half-angle of 2.5 °
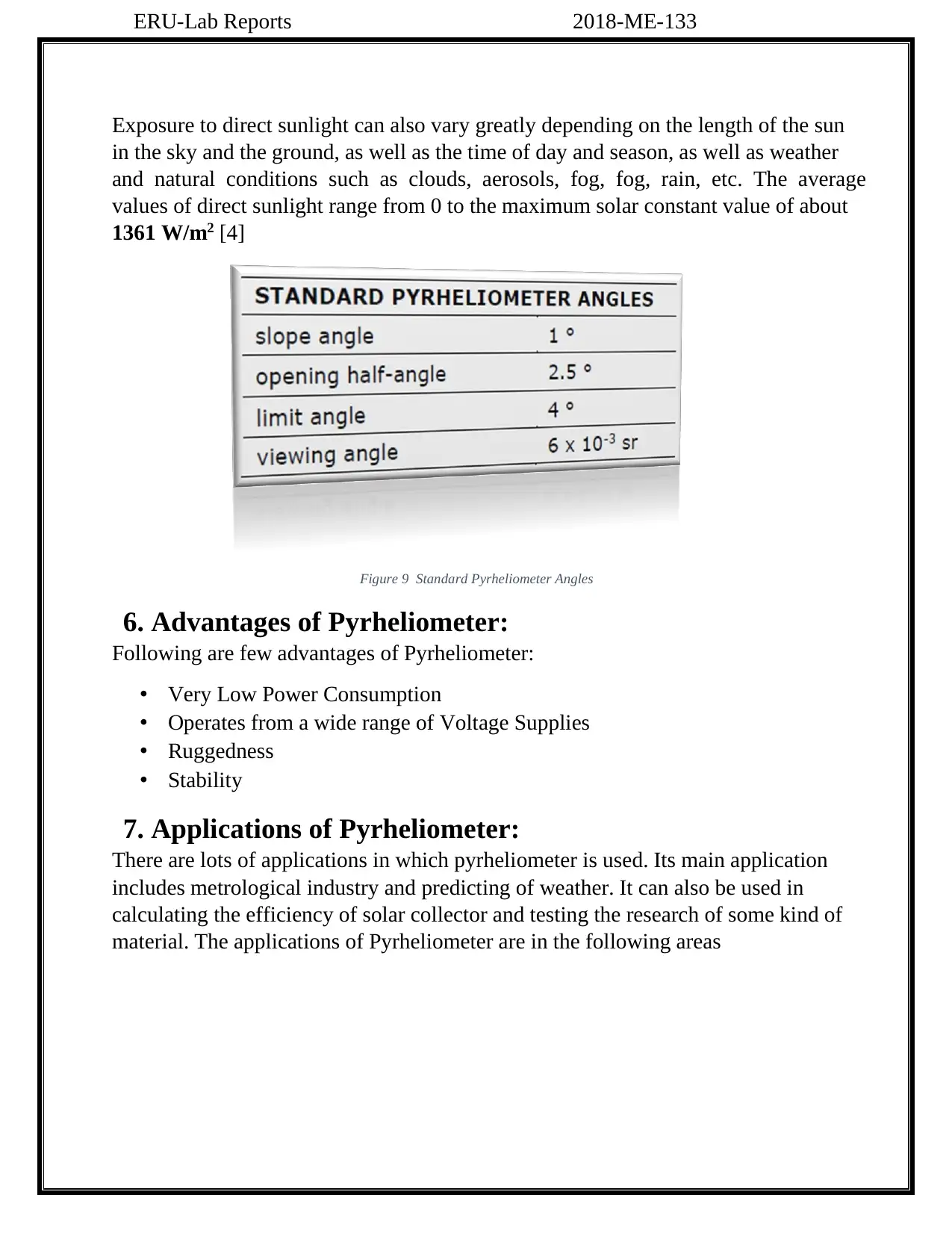
ERU-Lab Reports 2018-ME-133
Exposure to direct sunlight can also vary greatly depending on the length of the sun
in the sky and the ground, as well as the time of day and season, as well as weather
and natural conditions such as clouds, aerosols, fog, fog, rain, etc. The average
values of direct sunlight range from 0 to the maximum solar constant value of about
1361 W/m2 [4]
Figure 9 Standard Pyrheliometer Angles
6. Advantages of Pyrheliometer:
Following are few advantages of Pyrheliometer:
• Very Low Power Consumption
• Operates from a wide range of Voltage Supplies
• Ruggedness
• Stability
7. Applications of Pyrheliometer:
There are lots of applications in which pyrheliometer is used. Its main application
includes metrological industry and predicting of weather. It can also be used in
calculating the efficiency of solar collector and testing the research of some kind of
material. The applications of Pyrheliometer are in the following areas
Exposure to direct sunlight can also vary greatly depending on the length of the sun
in the sky and the ground, as well as the time of day and season, as well as weather
and natural conditions such as clouds, aerosols, fog, fog, rain, etc. The average
values of direct sunlight range from 0 to the maximum solar constant value of about
1361 W/m2 [4]
Figure 9 Standard Pyrheliometer Angles
6. Advantages of Pyrheliometer:
Following are few advantages of Pyrheliometer:
• Very Low Power Consumption
• Operates from a wide range of Voltage Supplies
• Ruggedness
• Stability
7. Applications of Pyrheliometer:
There are lots of applications in which pyrheliometer is used. Its main application
includes metrological industry and predicting of weather. It can also be used in
calculating the efficiency of solar collector and testing the research of some kind of
material. The applications of Pyrheliometer are in the following areas
Paraphrase This Document
Need a fresh take? Get an instant paraphrase of this document with our AI Paraphraser
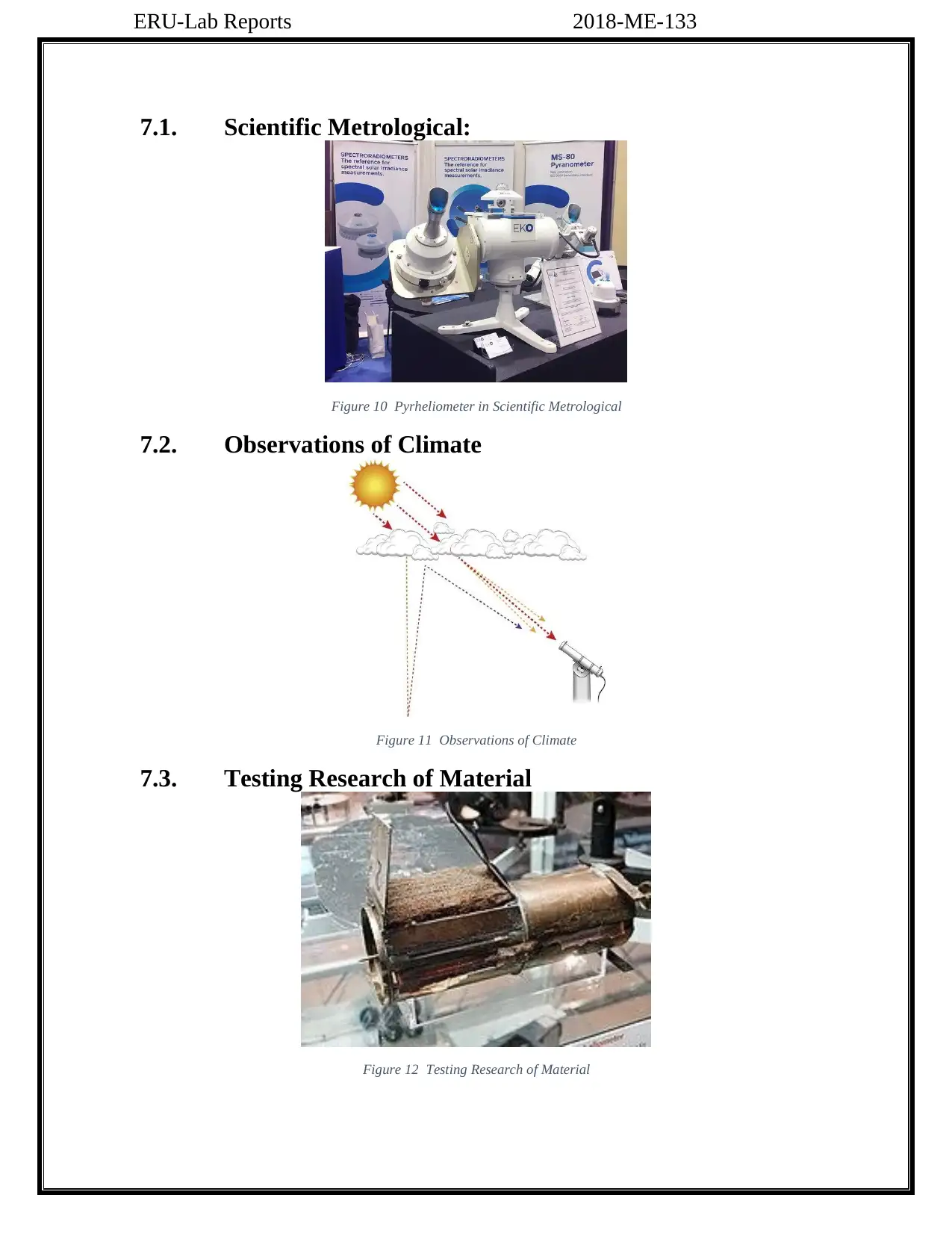
ERU-Lab Reports 2018-ME-133
7.1. Scientific Metrological:
Figure 10 Pyrheliometer in Scientific Metrological
7.2. Observations of Climate
Figure 11 Observations of Climate
7.3. Testing Research of Material
Figure 12 Testing Research of Material
7.1. Scientific Metrological:
Figure 10 Pyrheliometer in Scientific Metrological
7.2. Observations of Climate
Figure 11 Observations of Climate
7.3. Testing Research of Material
Figure 12 Testing Research of Material
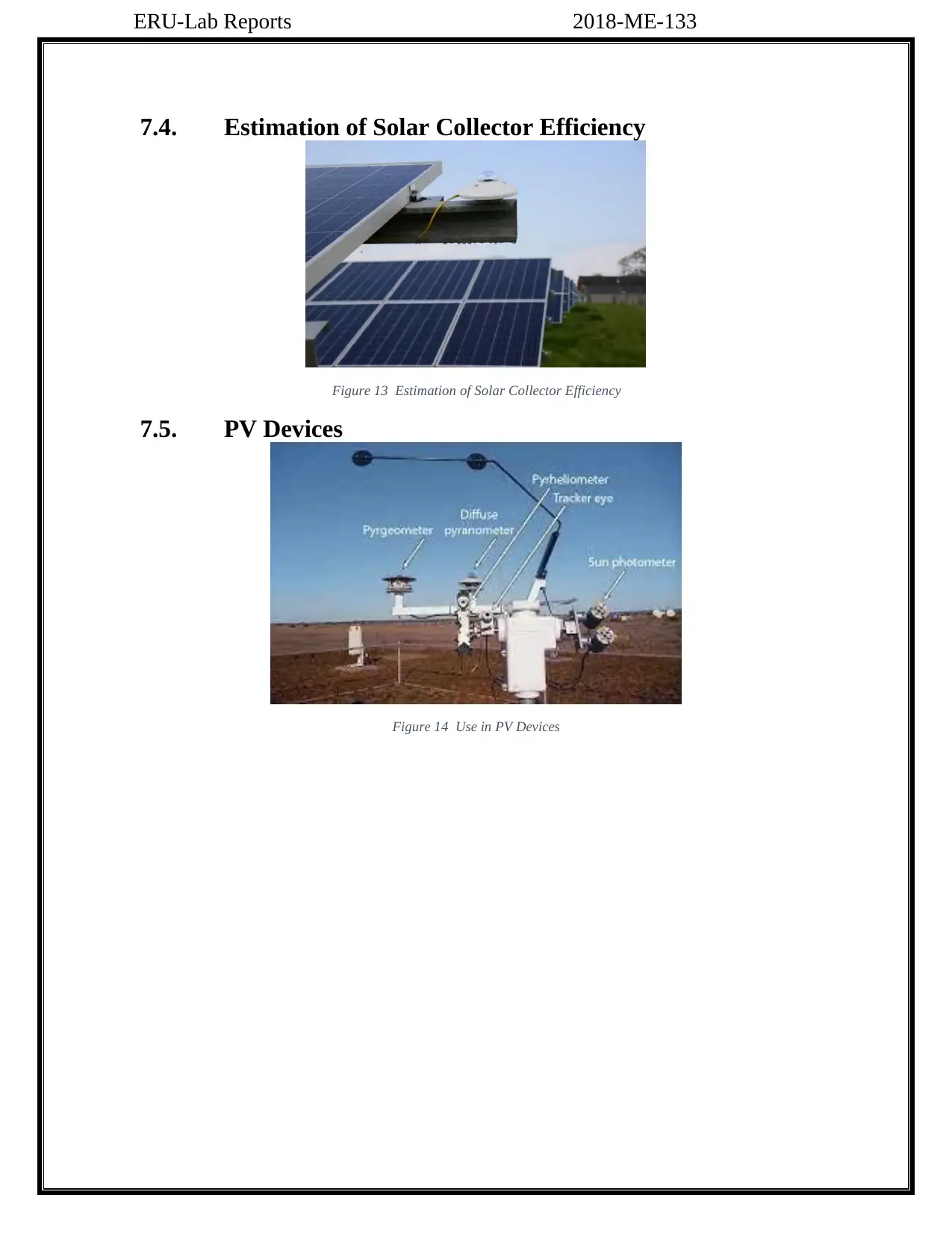
ERU-Lab Reports 2018-ME-133
7.4. Estimation of Solar Collector Efficiency
Figure 13 Estimation of Solar Collector Efficiency
7.5. PV Devices
Figure 14 Use in PV Devices
7.4. Estimation of Solar Collector Efficiency
Figure 13 Estimation of Solar Collector Efficiency
7.5. PV Devices
Figure 14 Use in PV Devices
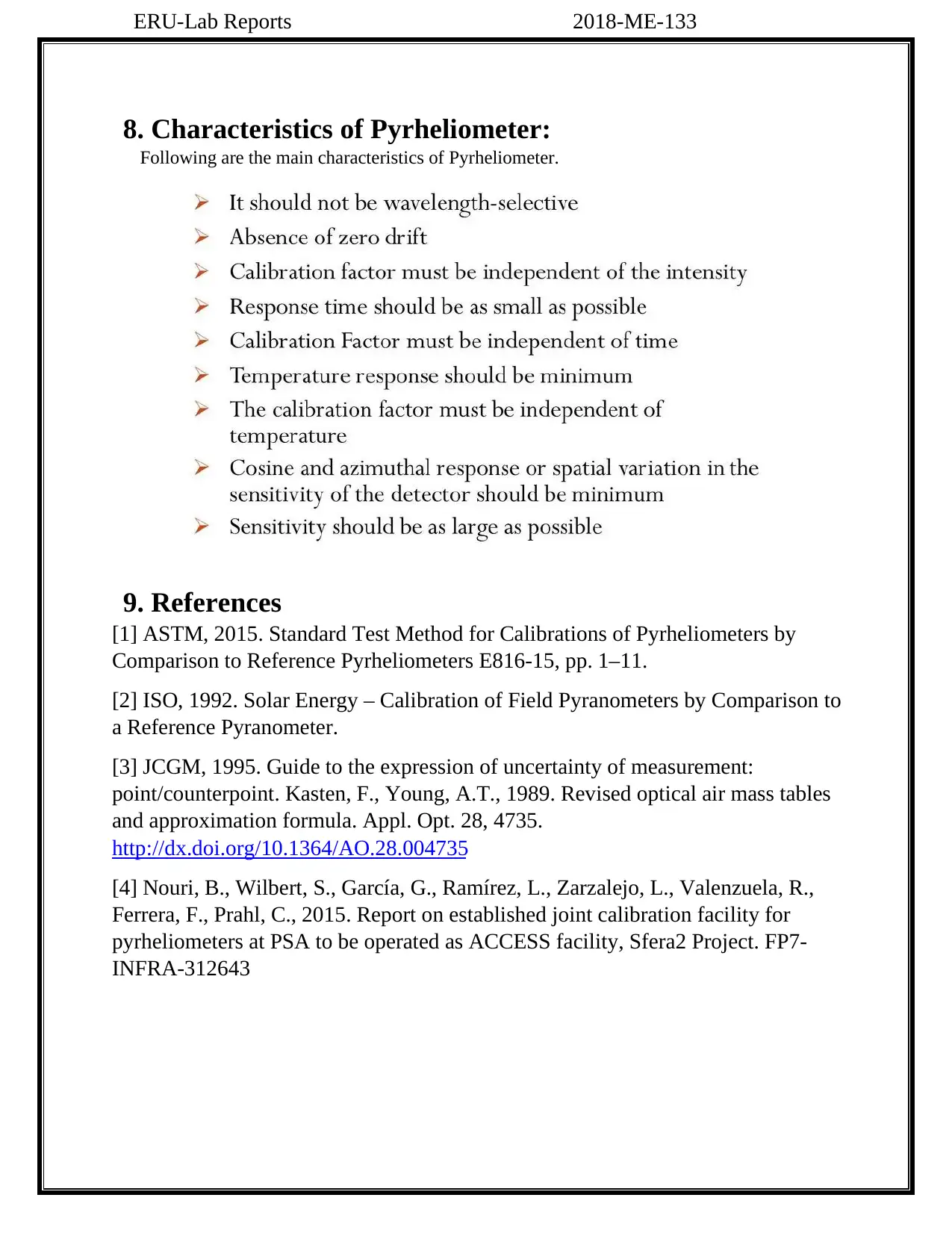
ERU-Lab Reports 2018-ME-133
8. Characteristics of Pyrheliometer:
Following are the main characteristics of Pyrheliometer.
9. References
[1] ASTM, 2015. Standard Test Method for Calibrations of Pyrheliometers by
Comparison to Reference Pyrheliometers E816-15, pp. 1–11.
[2] ISO, 1992. Solar Energy – Calibration of Field Pyranometers by Comparison to
a Reference Pyranometer.
[3] JCGM, 1995. Guide to the expression of uncertainty of measurement:
point/counterpoint. Kasten, F., Young, A.T., 1989. Revised optical air mass tables
and approximation formula. Appl. Opt. 28, 4735.
http://dx.doi.org/10.1364/AO.28.004735
[4] Nouri, B., Wilbert, S., García, G., Ramírez, L., Zarzalejo, L., Valenzuela, R.,
Ferrera, F., Prahl, C., 2015. Report on established joint calibration facility for
pyrheliometers at PSA to be operated as ACCESS facility, Sfera2 Project. FP7-
INFRA-312643
8. Characteristics of Pyrheliometer:
Following are the main characteristics of Pyrheliometer.
9. References
[1] ASTM, 2015. Standard Test Method for Calibrations of Pyrheliometers by
Comparison to Reference Pyrheliometers E816-15, pp. 1–11.
[2] ISO, 1992. Solar Energy – Calibration of Field Pyranometers by Comparison to
a Reference Pyranometer.
[3] JCGM, 1995. Guide to the expression of uncertainty of measurement:
point/counterpoint. Kasten, F., Young, A.T., 1989. Revised optical air mass tables
and approximation formula. Appl. Opt. 28, 4735.
http://dx.doi.org/10.1364/AO.28.004735
[4] Nouri, B., Wilbert, S., García, G., Ramírez, L., Zarzalejo, L., Valenzuela, R.,
Ferrera, F., Prahl, C., 2015. Report on established joint calibration facility for
pyrheliometers at PSA to be operated as ACCESS facility, Sfera2 Project. FP7-
INFRA-312643
Secure Best Marks with AI Grader
Need help grading? Try our AI Grader for instant feedback on your assignments.
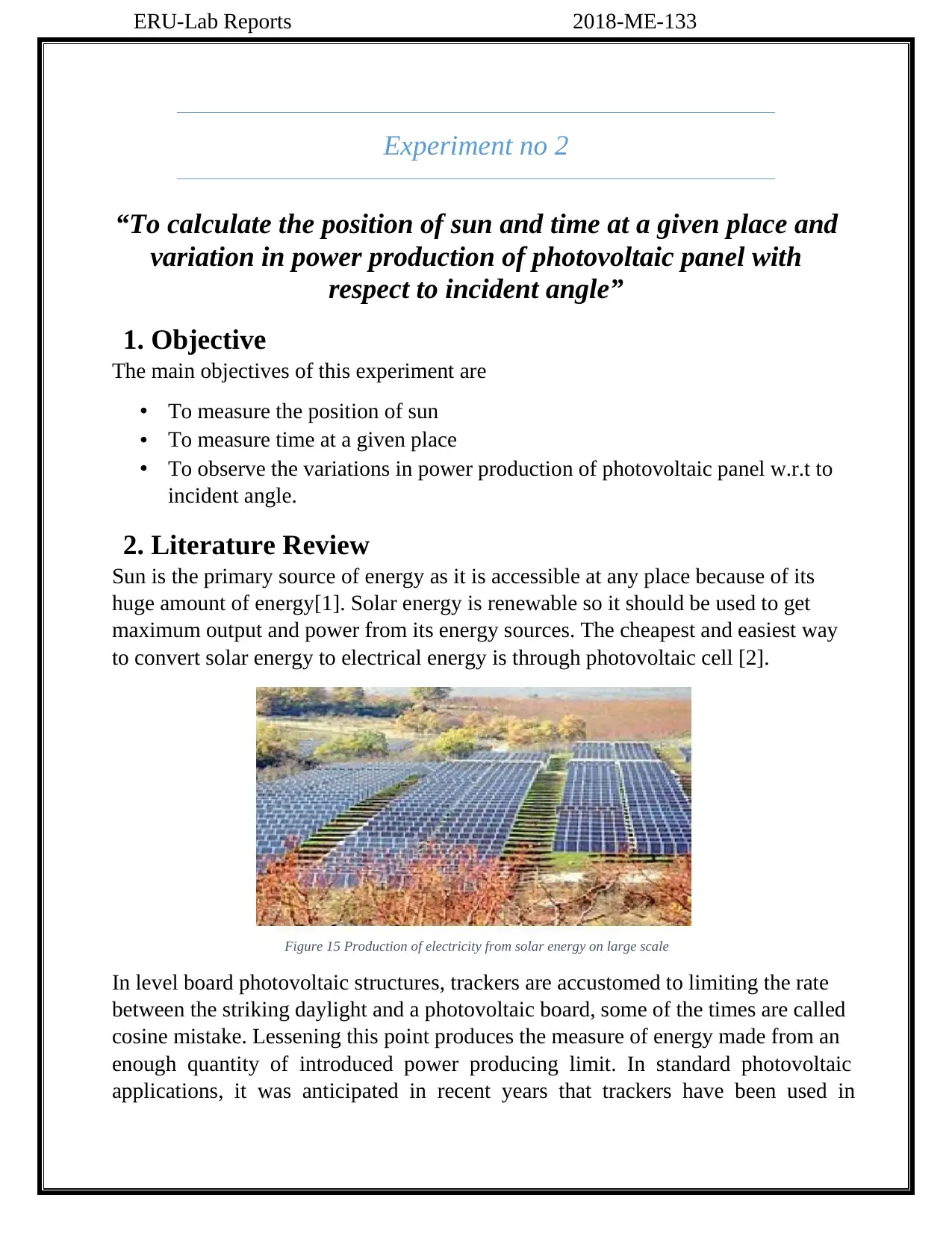
ERU-Lab Reports 2018-ME-133
Experiment no 2
“To calculate the position of sun and time at a given place and
variation in power production of photovoltaic panel with
respect to incident angle”
1. Objective
The main objectives of this experiment are
• To measure the position of sun
• To measure time at a given place
• To observe the variations in power production of photovoltaic panel w.r.t to
incident angle.
2. Literature Review
Sun is the primary source of energy as it is accessible at any place because of its
huge amount of energy[1]. Solar energy is renewable so it should be used to get
maximum output and power from its energy sources. The cheapest and easiest way
to convert solar energy to electrical energy is through photovoltaic cell [2].
Figure 15 Production of electricity from solar energy on large scale
In level board photovoltaic structures, trackers are accustomed to limiting the rate
between the striking daylight and a photovoltaic board, some of the times are called
cosine mistake. Lessening this point produces the measure of energy made from an
enough quantity of introduced power producing limit. In standard photovoltaic
applications, it was anticipated in recent years that trackers have been used in
Experiment no 2
“To calculate the position of sun and time at a given place and
variation in power production of photovoltaic panel with
respect to incident angle”
1. Objective
The main objectives of this experiment are
• To measure the position of sun
• To measure time at a given place
• To observe the variations in power production of photovoltaic panel w.r.t to
incident angle.
2. Literature Review
Sun is the primary source of energy as it is accessible at any place because of its
huge amount of energy[1]. Solar energy is renewable so it should be used to get
maximum output and power from its energy sources. The cheapest and easiest way
to convert solar energy to electrical energy is through photovoltaic cell [2].
Figure 15 Production of electricity from solar energy on large scale
In level board photovoltaic structures, trackers are accustomed to limiting the rate
between the striking daylight and a photovoltaic board, some of the times are called
cosine mistake. Lessening this point produces the measure of energy made from an
enough quantity of introduced power producing limit. In standard photovoltaic
applications, it was anticipated in recent years that trackers have been used in
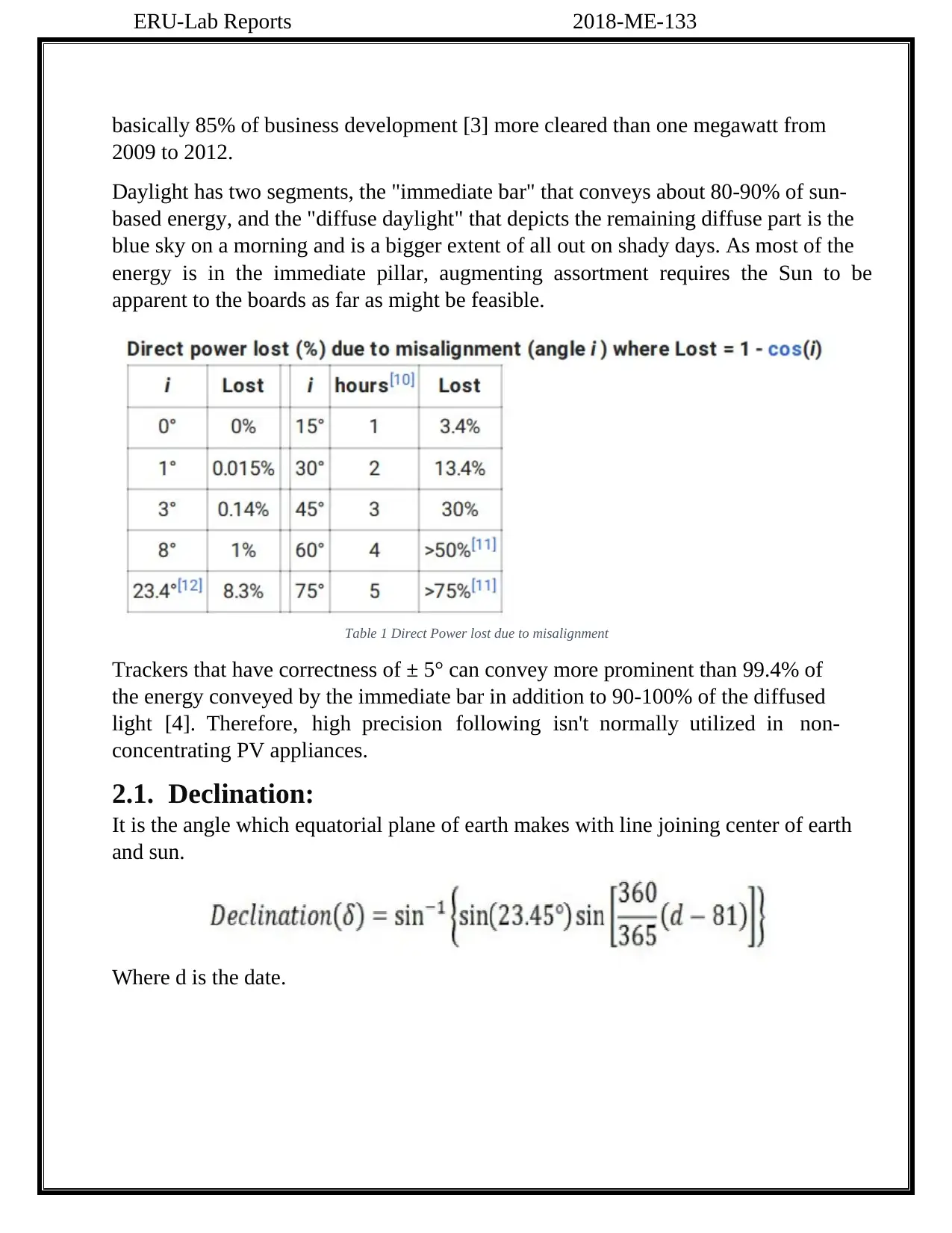
ERU-Lab Reports 2018-ME-133
basically 85% of business development [3] more cleared than one megawatt from
2009 to 2012.
Daylight has two segments, the "immediate bar" that conveys about 80-90% of sun-
based energy, and the "diffuse daylight" that depicts the remaining diffuse part is the
blue sky on a morning and is a bigger extent of all out on shady days. As most of the
energy is in the immediate pillar, augmenting assortment requires the Sun to be
apparent to the boards as far as might be feasible.
Table 1 Direct Power lost due to misalignment
Trackers that have correctness of ± 5° can convey more prominent than 99.4% of
the energy conveyed by the immediate bar in addition to 90-100% of the diffused
light [4]. Therefore, high precision following isn't normally utilized in non-
concentrating PV appliances.
2.1. Declination:
It is the angle which equatorial plane of earth makes with line joining center of earth
and sun.
Where d is the date.
basically 85% of business development [3] more cleared than one megawatt from
2009 to 2012.
Daylight has two segments, the "immediate bar" that conveys about 80-90% of sun-
based energy, and the "diffuse daylight" that depicts the remaining diffuse part is the
blue sky on a morning and is a bigger extent of all out on shady days. As most of the
energy is in the immediate pillar, augmenting assortment requires the Sun to be
apparent to the boards as far as might be feasible.
Table 1 Direct Power lost due to misalignment
Trackers that have correctness of ± 5° can convey more prominent than 99.4% of
the energy conveyed by the immediate bar in addition to 90-100% of the diffused
light [4]. Therefore, high precision following isn't normally utilized in non-
concentrating PV appliances.
2.1. Declination:
It is the angle which equatorial plane of earth makes with line joining center of earth
and sun.
Where d is the date.
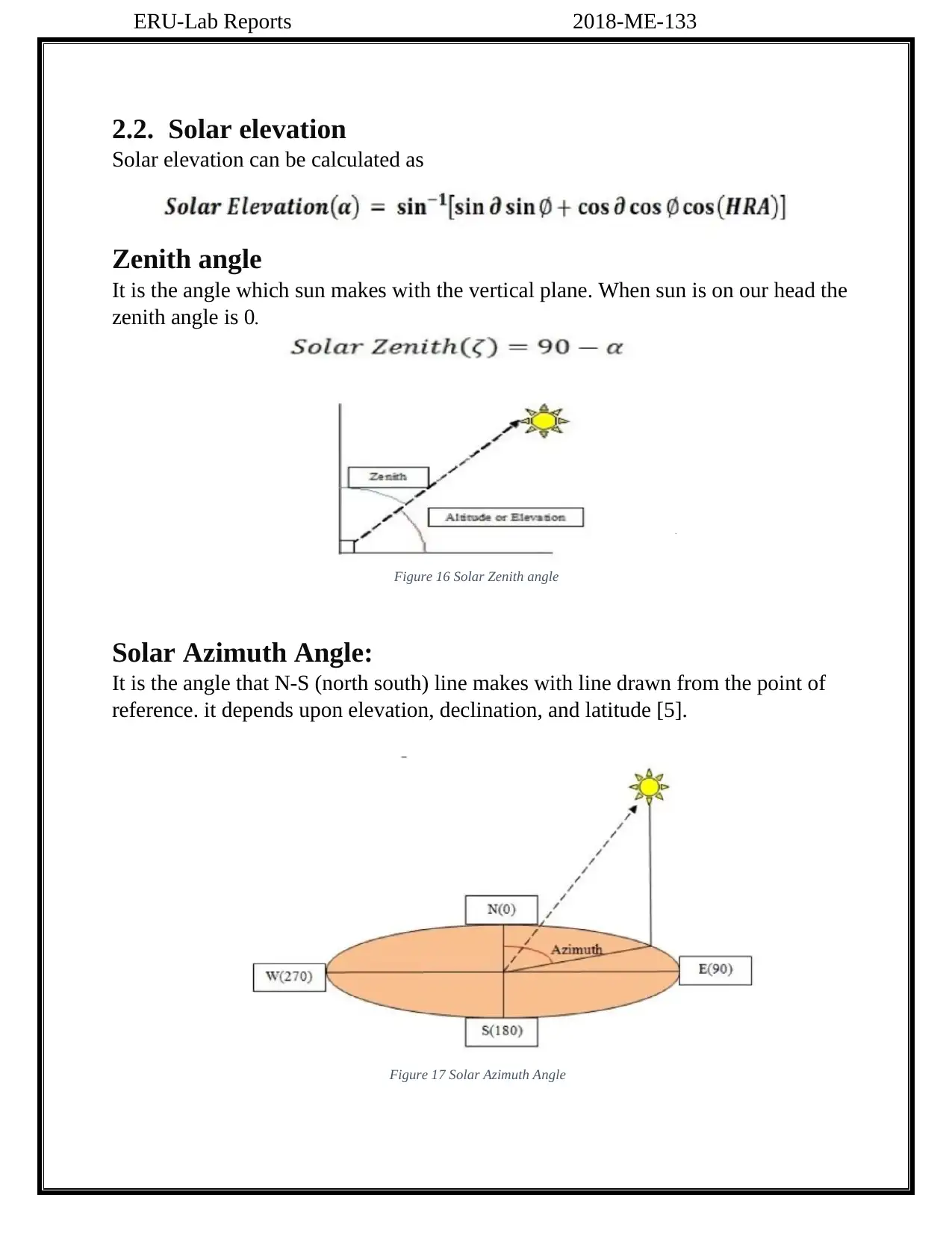
ERU-Lab Reports 2018-ME-133
2.2. Solar elevation
Solar elevation can be calculated as
Zenith angle
It is the angle which sun makes with the vertical plane. When sun is on our head the
zenith angle is 0.
Solar Azimuth Angle:
It is the angle that N-S (north south) line makes with line drawn from the point of
reference. it depends upon elevation, declination, and latitude [5].
Figure 16 Solar Zenith angle
Figure 17 Solar Azimuth Angle
2.2. Solar elevation
Solar elevation can be calculated as
Zenith angle
It is the angle which sun makes with the vertical plane. When sun is on our head the
zenith angle is 0.
Solar Azimuth Angle:
It is the angle that N-S (north south) line makes with line drawn from the point of
reference. it depends upon elevation, declination, and latitude [5].
Figure 16 Solar Zenith angle
Figure 17 Solar Azimuth Angle
Paraphrase This Document
Need a fresh take? Get an instant paraphrase of this document with our AI Paraphraser
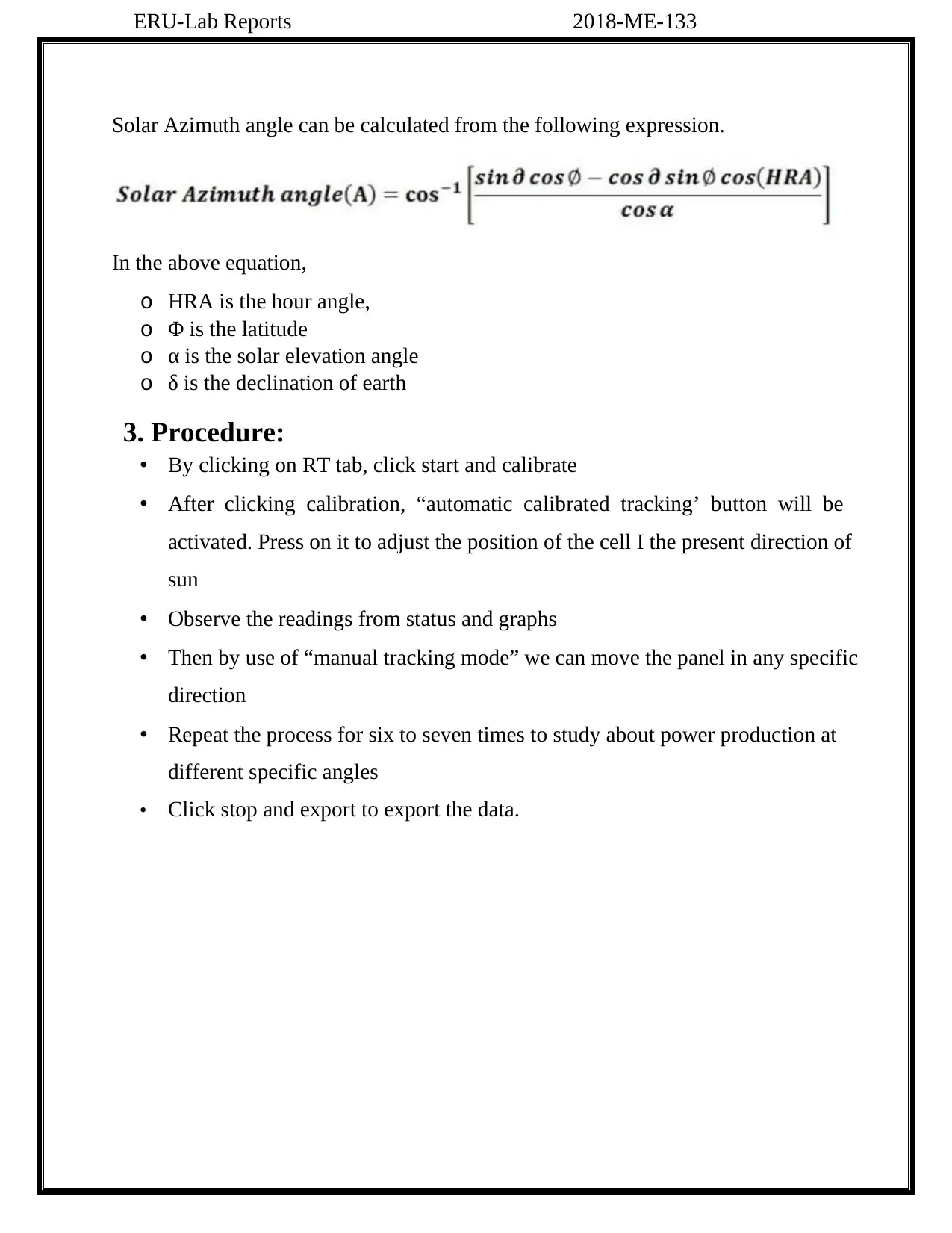
ERU-Lab Reports 2018-ME-133
Solar Azimuth angle can be calculated from the following expression.
In the above equation,
o HRA is the hour angle,
o Φ is the latitude
o α is the solar elevation angle
o δ is the declination of earth
3. Procedure:
• By clicking on RT tab, click start and calibrate
• After clicking calibration, “automatic calibrated tracking’ button will be
activated. Press on it to adjust the position of the cell I the present direction of
sun
• Observe the readings from status and graphs
• Then by use of “manual tracking mode” we can move the panel in any specific
direction
• Repeat the process for six to seven times to study about power production at
different specific angles
• Click stop and export to export the data.
Solar Azimuth angle can be calculated from the following expression.
In the above equation,
o HRA is the hour angle,
o Φ is the latitude
o α is the solar elevation angle
o δ is the declination of earth
3. Procedure:
• By clicking on RT tab, click start and calibrate
• After clicking calibration, “automatic calibrated tracking’ button will be
activated. Press on it to adjust the position of the cell I the present direction of
sun
• Observe the readings from status and graphs
• Then by use of “manual tracking mode” we can move the panel in any specific
direction
• Repeat the process for six to seven times to study about power production at
different specific angles
• Click stop and export to export the data.
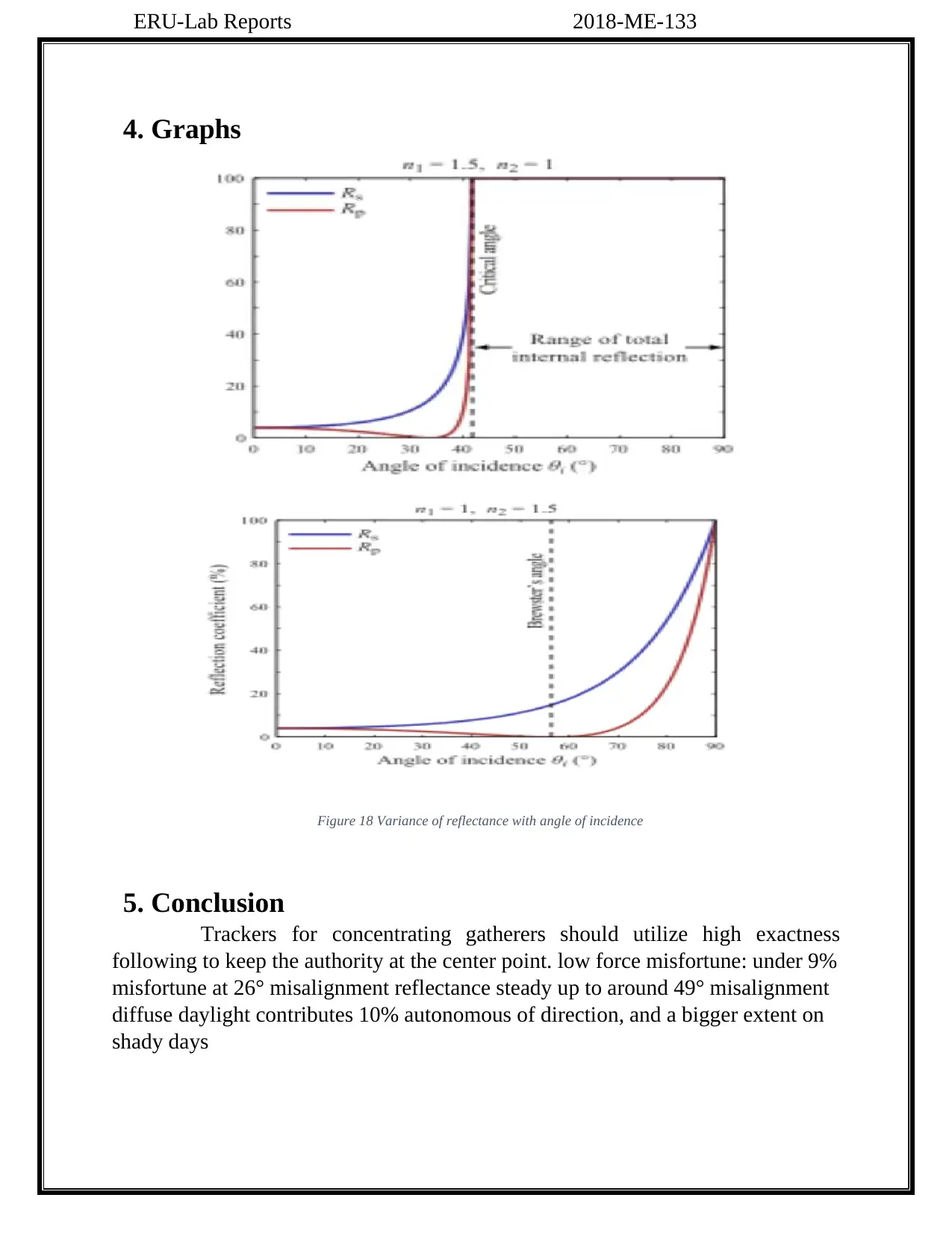
ERU-Lab Reports 2018-ME-133
4. Graphs
Figure 18 Variance of reflectance with angle of incidence
5. Conclusion
Trackers for concentrating gatherers should utilize high exactness
following to keep the authority at the center point. low force misfortune: under 9%
misfortune at 26° misalignment reflectance steady up to around 49° misalignment
diffuse daylight contributes 10% autonomous of direction, and a bigger extent on
shady days
4. Graphs
Figure 18 Variance of reflectance with angle of incidence
5. Conclusion
Trackers for concentrating gatherers should utilize high exactness
following to keep the authority at the center point. low force misfortune: under 9%
misfortune at 26° misalignment reflectance steady up to around 49° misalignment
diffuse daylight contributes 10% autonomous of direction, and a bigger extent on
shady days
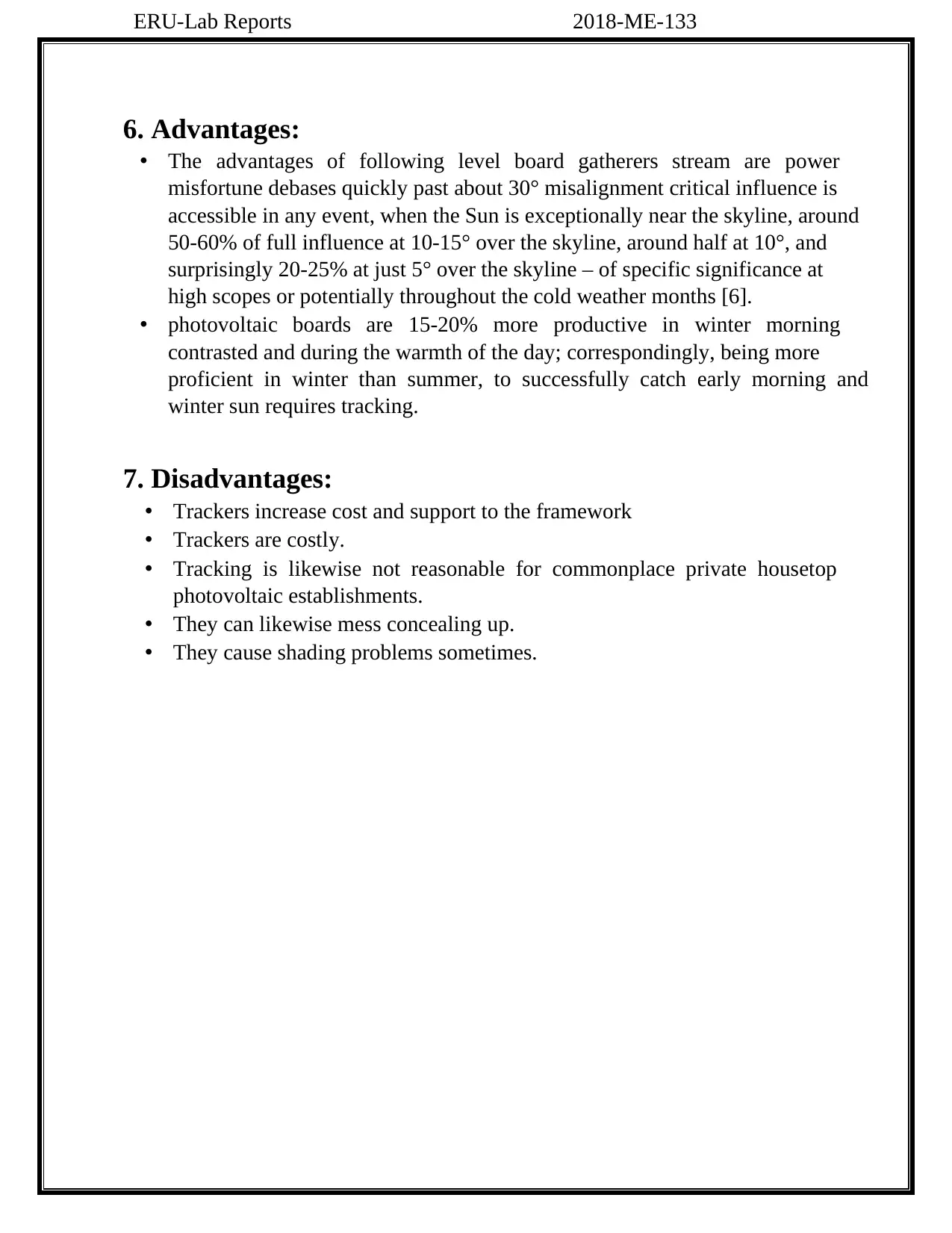
ERU-Lab Reports 2018-ME-133
6. Advantages:
• The advantages of following level board gatherers stream are power
misfortune debases quickly past about 30° misalignment critical influence is
accessible in any event, when the Sun is exceptionally near the skyline, around
50-60% of full influence at 10-15° over the skyline, around half at 10°, and
surprisingly 20-25% at just 5° over the skyline – of specific significance at
high scopes or potentially throughout the cold weather months [6].
• photovoltaic boards are 15-20% more productive in winter morning
contrasted and during the warmth of the day; correspondingly, being more
proficient in winter than summer, to successfully catch early morning and
winter sun requires tracking.
7. Disadvantages:
• Trackers increase cost and support to the framework
• Trackers are costly.
• Tracking is likewise not reasonable for commonplace private housetop
photovoltaic establishments.
• They can likewise mess concealing up.
• They cause shading problems sometimes.
6. Advantages:
• The advantages of following level board gatherers stream are power
misfortune debases quickly past about 30° misalignment critical influence is
accessible in any event, when the Sun is exceptionally near the skyline, around
50-60% of full influence at 10-15° over the skyline, around half at 10°, and
surprisingly 20-25% at just 5° over the skyline – of specific significance at
high scopes or potentially throughout the cold weather months [6].
• photovoltaic boards are 15-20% more productive in winter morning
contrasted and during the warmth of the day; correspondingly, being more
proficient in winter than summer, to successfully catch early morning and
winter sun requires tracking.
7. Disadvantages:
• Trackers increase cost and support to the framework
• Trackers are costly.
• Tracking is likewise not reasonable for commonplace private housetop
photovoltaic establishments.
• They can likewise mess concealing up.
• They cause shading problems sometimes.
Secure Best Marks with AI Grader
Need help grading? Try our AI Grader for instant feedback on your assignments.
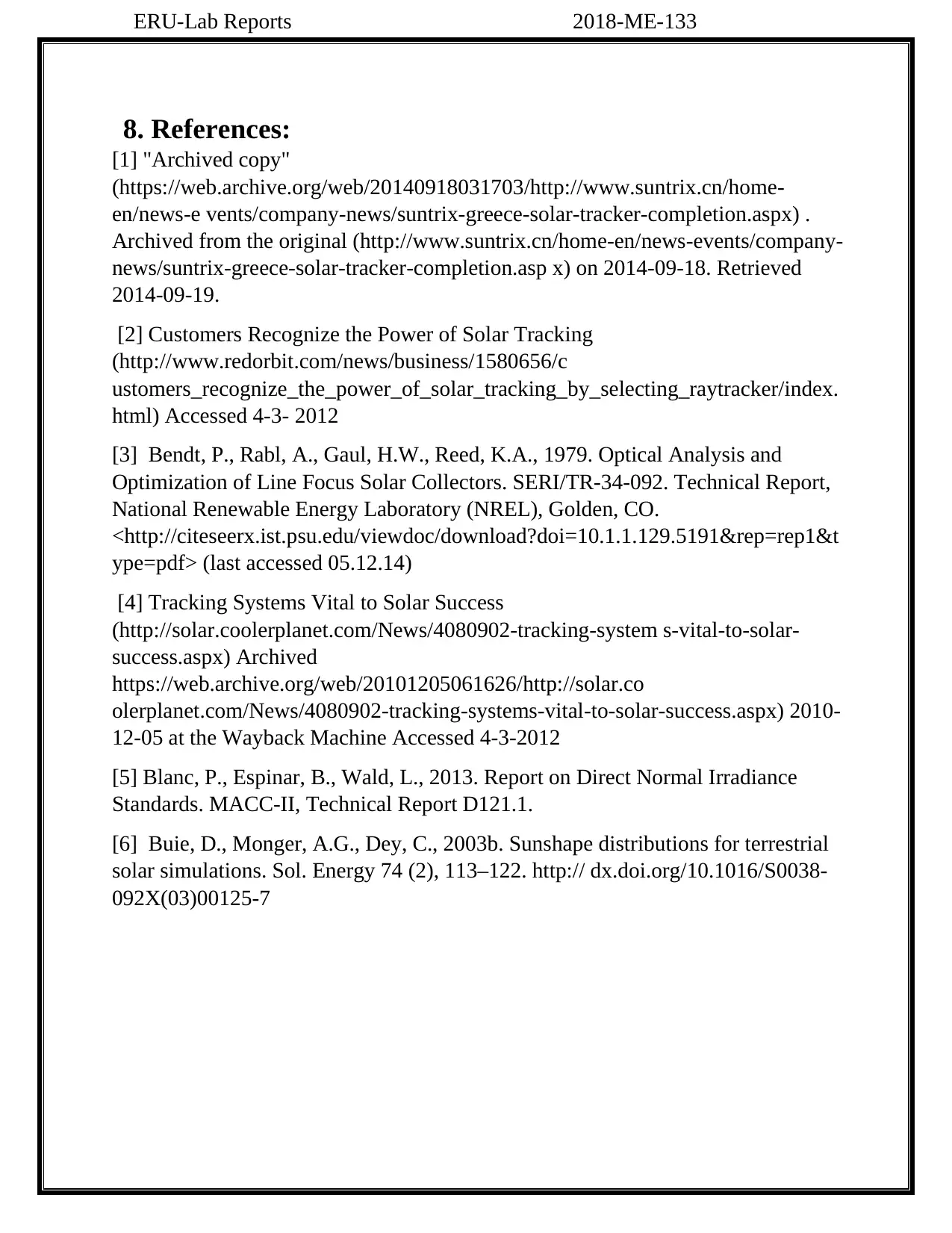
ERU-Lab Reports 2018-ME-133
8. References:
[1] "Archived copy"
(https://web.archive.org/web/20140918031703/http://www.suntrix.cn/home-
en/news-e vents/company-news/suntrix-greece-solar-tracker-completion.aspx) .
Archived from the original (http://www.suntrix.cn/home-en/news-events/company-
news/suntrix-greece-solar-tracker-completion.asp x) on 2014-09-18. Retrieved
2014-09-19.
[2] Customers Recognize the Power of Solar Tracking
(http://www.redorbit.com/news/business/1580656/c
ustomers_recognize_the_power_of_solar_tracking_by_selecting_raytracker/index.
html) Accessed 4-3- 2012
[3] Bendt, P., Rabl, A., Gaul, H.W., Reed, K.A., 1979. Optical Analysis and
Optimization of Line Focus Solar Collectors. SERI/TR-34-092. Technical Report,
National Renewable Energy Laboratory (NREL), Golden, CO.
<http://citeseerx.ist.psu.edu/viewdoc/download?doi=10.1.1.129.5191&rep=rep1&t
ype=pdf> (last accessed 05.12.14)
[4] Tracking Systems Vital to Solar Success
(http://solar.coolerplanet.com/News/4080902-tracking-system s-vital-to-solar-
success.aspx) Archived
https://web.archive.org/web/20101205061626/http://solar.co
olerplanet.com/News/4080902-tracking-systems-vital-to-solar-success.aspx) 2010-
12-05 at the Wayback Machine Accessed 4-3-2012
[5] Blanc, P., Espinar, B., Wald, L., 2013. Report on Direct Normal Irradiance
Standards. MACC-II, Technical Report D121.1.
[6] Buie, D., Monger, A.G., Dey, C., 2003b. Sunshape distributions for terrestrial
solar simulations. Sol. Energy 74 (2), 113–122. http:// dx.doi.org/10.1016/S0038-
092X(03)00125-7
8. References:
[1] "Archived copy"
(https://web.archive.org/web/20140918031703/http://www.suntrix.cn/home-
en/news-e vents/company-news/suntrix-greece-solar-tracker-completion.aspx) .
Archived from the original (http://www.suntrix.cn/home-en/news-events/company-
news/suntrix-greece-solar-tracker-completion.asp x) on 2014-09-18. Retrieved
2014-09-19.
[2] Customers Recognize the Power of Solar Tracking
(http://www.redorbit.com/news/business/1580656/c
ustomers_recognize_the_power_of_solar_tracking_by_selecting_raytracker/index.
html) Accessed 4-3- 2012
[3] Bendt, P., Rabl, A., Gaul, H.W., Reed, K.A., 1979. Optical Analysis and
Optimization of Line Focus Solar Collectors. SERI/TR-34-092. Technical Report,
National Renewable Energy Laboratory (NREL), Golden, CO.
<http://citeseerx.ist.psu.edu/viewdoc/download?doi=10.1.1.129.5191&rep=rep1&t
ype=pdf> (last accessed 05.12.14)
[4] Tracking Systems Vital to Solar Success
(http://solar.coolerplanet.com/News/4080902-tracking-system s-vital-to-solar-
success.aspx) Archived
https://web.archive.org/web/20101205061626/http://solar.co
olerplanet.com/News/4080902-tracking-systems-vital-to-solar-success.aspx) 2010-
12-05 at the Wayback Machine Accessed 4-3-2012
[5] Blanc, P., Espinar, B., Wald, L., 2013. Report on Direct Normal Irradiance
Standards. MACC-II, Technical Report D121.1.
[6] Buie, D., Monger, A.G., Dey, C., 2003b. Sunshape distributions for terrestrial
solar simulations. Sol. Energy 74 (2), 113–122. http:// dx.doi.org/10.1016/S0038-
092X(03)00125-7
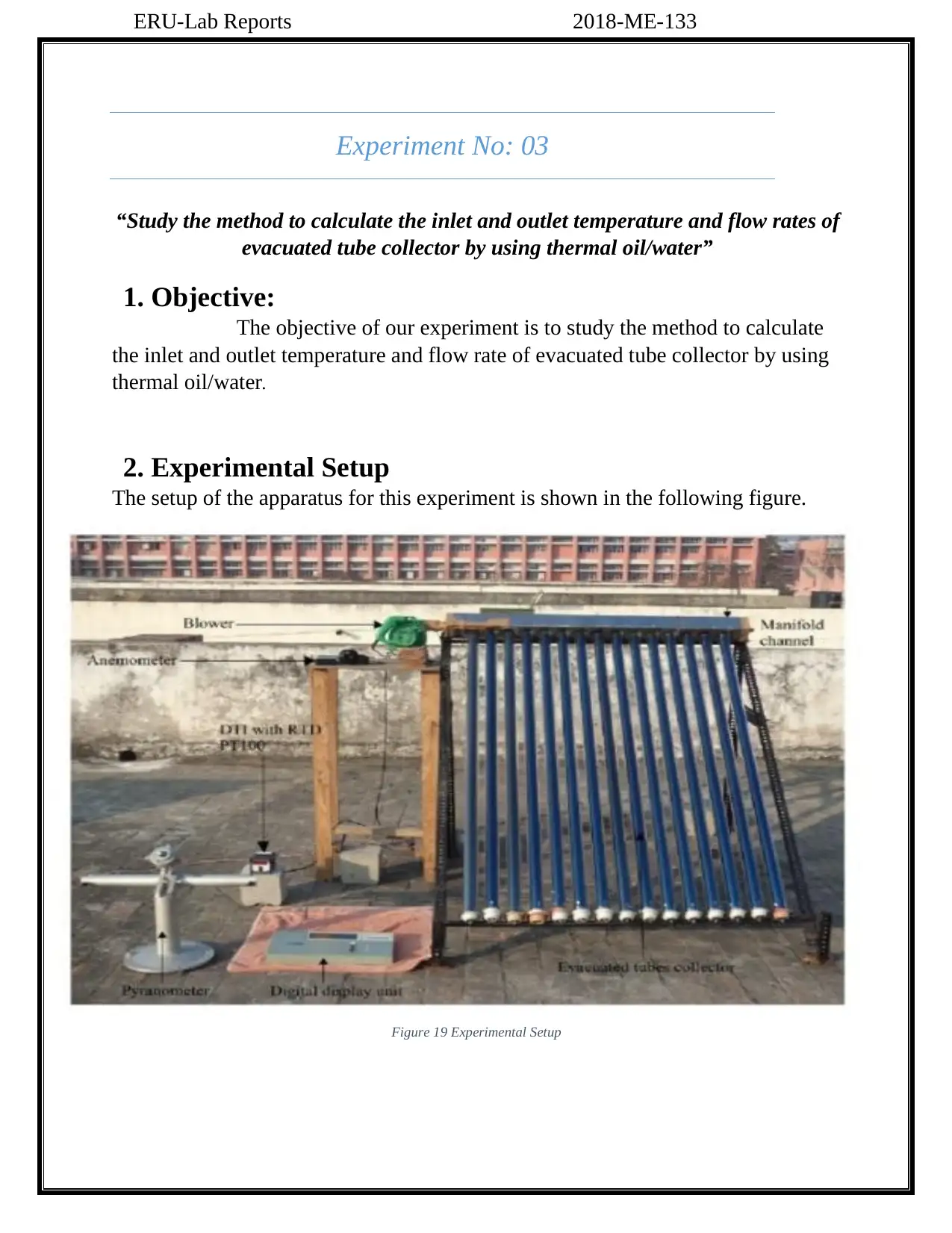
ERU-Lab Reports 2018-ME-133
Experiment No: 03
“Study the method to calculate the inlet and outlet temperature and flow rates of
evacuated tube collector by using thermal oil/water”
1. Objective:
The objective of our experiment is to study the method to calculate
the inlet and outlet temperature and flow rate of evacuated tube collector by using
thermal oil/water.
2. Experimental Setup
The setup of the apparatus for this experiment is shown in the following figure.
Figure 19 Experimental Setup
Experiment No: 03
“Study the method to calculate the inlet and outlet temperature and flow rates of
evacuated tube collector by using thermal oil/water”
1. Objective:
The objective of our experiment is to study the method to calculate
the inlet and outlet temperature and flow rate of evacuated tube collector by using
thermal oil/water.
2. Experimental Setup
The setup of the apparatus for this experiment is shown in the following figure.
Figure 19 Experimental Setup
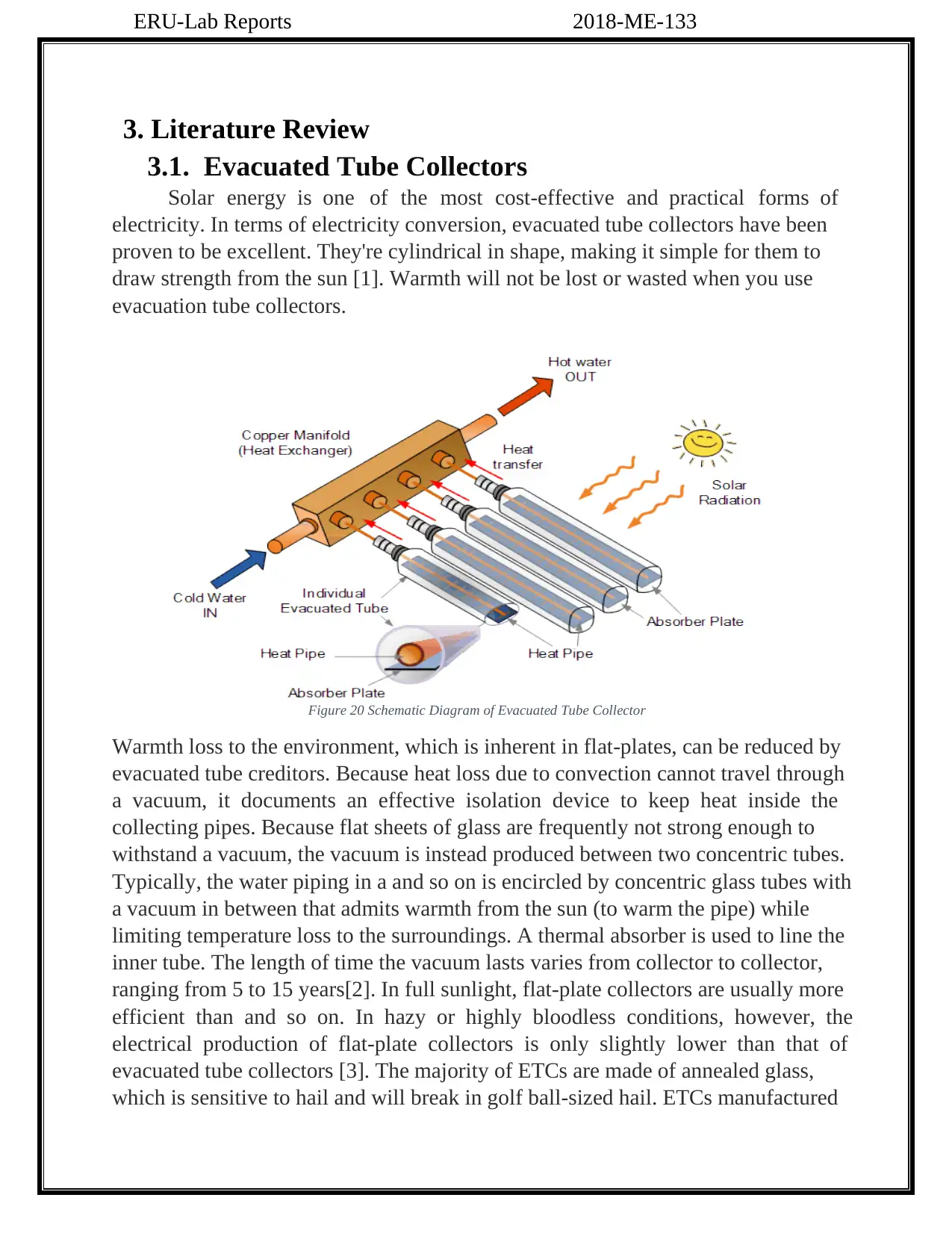
ERU-Lab Reports 2018-ME-133
3. Literature Review
3.1. Evacuated Tube Collectors
Solar energy is one of the most cost-effective and practical forms of
electricity. In terms of electricity conversion, evacuated tube collectors have been
proven to be excellent. They're cylindrical in shape, making it simple for them to
draw strength from the sun [1]. Warmth will not be lost or wasted when you use
evacuation tube collectors.
Figure 20 Schematic Diagram of Evacuated Tube Collector
Warmth loss to the environment, which is inherent in flat-plates, can be reduced by
evacuated tube creditors. Because heat loss due to convection cannot travel through
a vacuum, it documents an effective isolation device to keep heat inside the
collecting pipes. Because flat sheets of glass are frequently not strong enough to
withstand a vacuum, the vacuum is instead produced between two concentric tubes.
Typically, the water piping in a and so on is encircled by concentric glass tubes with
a vacuum in between that admits warmth from the sun (to warm the pipe) while
limiting temperature loss to the surroundings. A thermal absorber is used to line the
inner tube. The length of time the vacuum lasts varies from collector to collector,
ranging from 5 to 15 years[2]. In full sunlight, flat-plate collectors are usually more
efficient than and so on. In hazy or highly bloodless conditions, however, the
electrical production of flat-plate collectors is only slightly lower than that of
evacuated tube collectors [3]. The majority of ETCs are made of annealed glass,
which is sensitive to hail and will break in golf ball-sized hail. ETCs manufactured
3. Literature Review
3.1. Evacuated Tube Collectors
Solar energy is one of the most cost-effective and practical forms of
electricity. In terms of electricity conversion, evacuated tube collectors have been
proven to be excellent. They're cylindrical in shape, making it simple for them to
draw strength from the sun [1]. Warmth will not be lost or wasted when you use
evacuation tube collectors.
Figure 20 Schematic Diagram of Evacuated Tube Collector
Warmth loss to the environment, which is inherent in flat-plates, can be reduced by
evacuated tube creditors. Because heat loss due to convection cannot travel through
a vacuum, it documents an effective isolation device to keep heat inside the
collecting pipes. Because flat sheets of glass are frequently not strong enough to
withstand a vacuum, the vacuum is instead produced between two concentric tubes.
Typically, the water piping in a and so on is encircled by concentric glass tubes with
a vacuum in between that admits warmth from the sun (to warm the pipe) while
limiting temperature loss to the surroundings. A thermal absorber is used to line the
inner tube. The length of time the vacuum lasts varies from collector to collector,
ranging from 5 to 15 years[2]. In full sunlight, flat-plate collectors are usually more
efficient than and so on. In hazy or highly bloodless conditions, however, the
electrical production of flat-plate collectors is only slightly lower than that of
evacuated tube collectors [3]. The majority of ETCs are made of annealed glass,
which is sensitive to hail and will break in golf ball-sized hail. ETCs manufactured
Paraphrase This Document
Need a fresh take? Get an instant paraphrase of this document with our AI Paraphraser
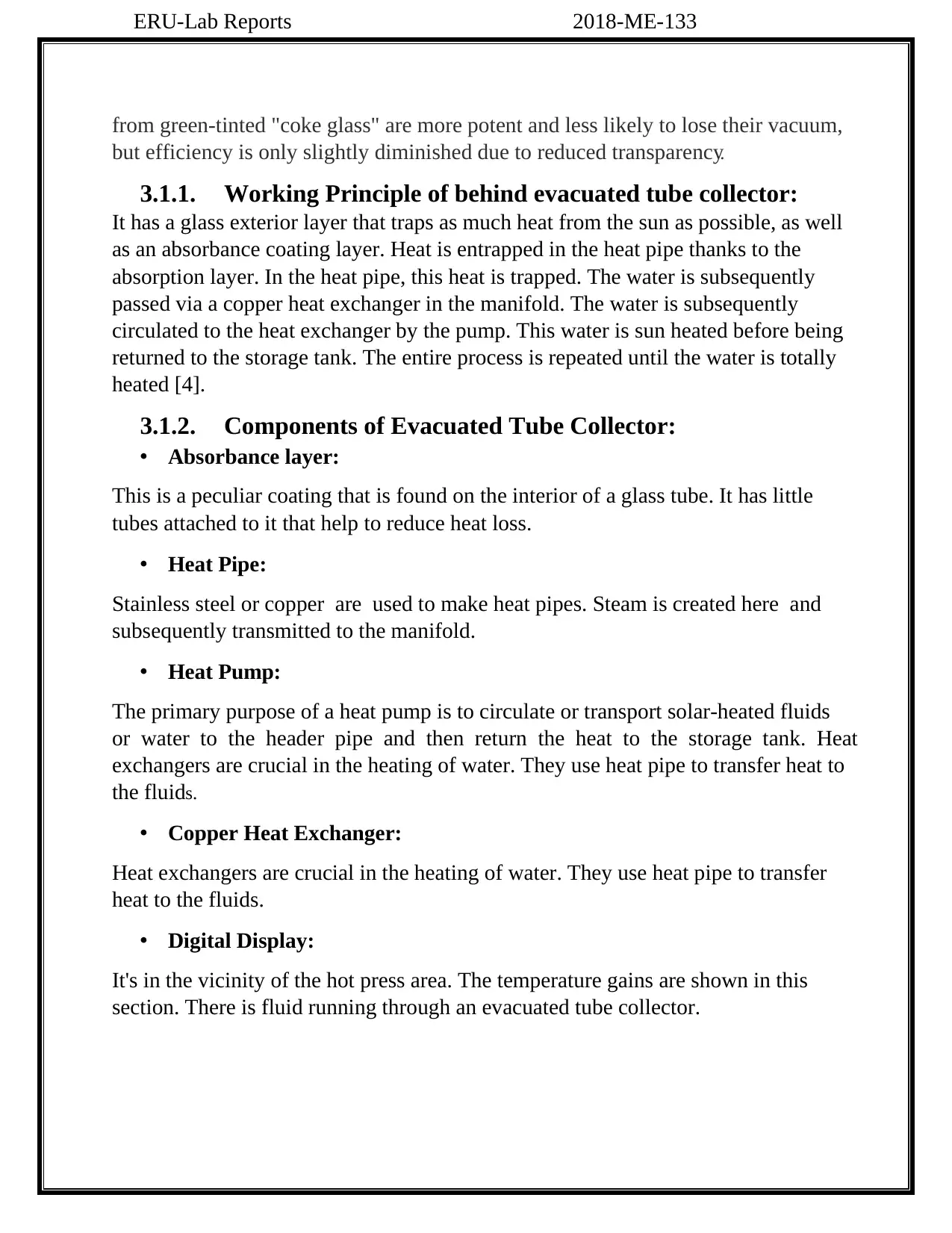
ERU-Lab Reports 2018-ME-133
from green-tinted "coke glass" are more potent and less likely to lose their vacuum,
but efficiency is only slightly diminished due to reduced transparency.
3.1.1. Working Principle of behind evacuated tube collector:
It has a glass exterior layer that traps as much heat from the sun as possible, as well
as an absorbance coating layer. Heat is entrapped in the heat pipe thanks to the
absorption layer. In the heat pipe, this heat is trapped. The water is subsequently
passed via a copper heat exchanger in the manifold. The water is subsequently
circulated to the heat exchanger by the pump. This water is sun heated before being
returned to the storage tank. The entire process is repeated until the water is totally
heated [4].
3.1.2. Components of Evacuated Tube Collector:
• Absorbance layer:
This is a peculiar coating that is found on the interior of a glass tube. It has little
tubes attached to it that help to reduce heat loss.
• Heat Pipe:
Stainless steel or copper are used to make heat pipes. Steam is created here and
subsequently transmitted to the manifold.
• Heat Pump:
The primary purpose of a heat pump is to circulate or transport solar-heated fluids
or water to the header pipe and then return the heat to the storage tank. Heat
exchangers are crucial in the heating of water. They use heat pipe to transfer heat to
the fluids.
• Copper Heat Exchanger:
Heat exchangers are crucial in the heating of water. They use heat pipe to transfer
heat to the fluids.
• Digital Display:
It's in the vicinity of the hot press area. The temperature gains are shown in this
section. There is fluid running through an evacuated tube collector.
from green-tinted "coke glass" are more potent and less likely to lose their vacuum,
but efficiency is only slightly diminished due to reduced transparency.
3.1.1. Working Principle of behind evacuated tube collector:
It has a glass exterior layer that traps as much heat from the sun as possible, as well
as an absorbance coating layer. Heat is entrapped in the heat pipe thanks to the
absorption layer. In the heat pipe, this heat is trapped. The water is subsequently
passed via a copper heat exchanger in the manifold. The water is subsequently
circulated to the heat exchanger by the pump. This water is sun heated before being
returned to the storage tank. The entire process is repeated until the water is totally
heated [4].
3.1.2. Components of Evacuated Tube Collector:
• Absorbance layer:
This is a peculiar coating that is found on the interior of a glass tube. It has little
tubes attached to it that help to reduce heat loss.
• Heat Pipe:
Stainless steel or copper are used to make heat pipes. Steam is created here and
subsequently transmitted to the manifold.
• Heat Pump:
The primary purpose of a heat pump is to circulate or transport solar-heated fluids
or water to the header pipe and then return the heat to the storage tank. Heat
exchangers are crucial in the heating of water. They use heat pipe to transfer heat to
the fluids.
• Copper Heat Exchanger:
Heat exchangers are crucial in the heating of water. They use heat pipe to transfer
heat to the fluids.
• Digital Display:
It's in the vicinity of the hot press area. The temperature gains are shown in this
section. There is fluid running through an evacuated tube collector.
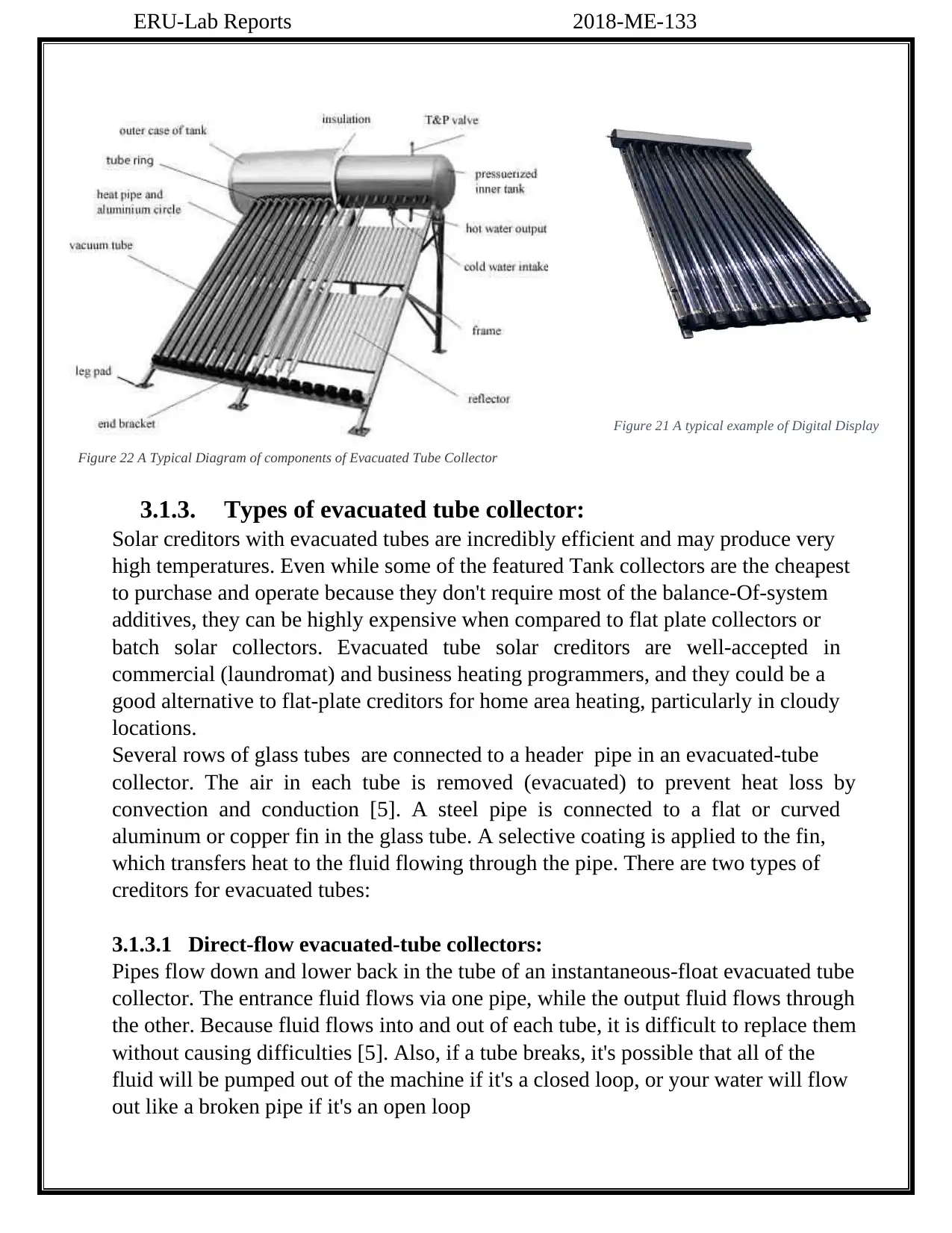
ERU-Lab Reports 2018-ME-133
3.1.3. Types of evacuated tube collector:
Solar creditors with evacuated tubes are incredibly efficient and may produce very
high temperatures. Even while some of the featured Tank collectors are the cheapest
to purchase and operate because they don't require most of the balance-Of-system
additives, they can be highly expensive when compared to flat plate collectors or
batch solar collectors. Evacuated tube solar creditors are well-accepted in
commercial (laundromat) and business heating programmers, and they could be a
good alternative to flat-plate creditors for home area heating, particularly in cloudy
locations.
Several rows of glass tubes are connected to a header pipe in an evacuated-tube
collector. The air in each tube is removed (evacuated) to prevent heat loss by
convection and conduction [5]. A steel pipe is connected to a flat or curved
aluminum or copper fin in the glass tube. A selective coating is applied to the fin,
which transfers heat to the fluid flowing through the pipe. There are two types of
creditors for evacuated tubes:
3.1.3.1 Direct-flow evacuated-tube collectors:
Pipes flow down and lower back in the tube of an instantaneous-float evacuated tube
collector. The entrance fluid flows via one pipe, while the output fluid flows through
the other. Because fluid flows into and out of each tube, it is difficult to replace them
without causing difficulties [5]. Also, if a tube breaks, it's possible that all of the
fluid will be pumped out of the machine if it's a closed loop, or your water will flow
out like a broken pipe if it's an open loop
Figure 21 A typical example of Digital Display
Figure 22 A Typical Diagram of components of Evacuated Tube Collector
3.1.3. Types of evacuated tube collector:
Solar creditors with evacuated tubes are incredibly efficient and may produce very
high temperatures. Even while some of the featured Tank collectors are the cheapest
to purchase and operate because they don't require most of the balance-Of-system
additives, they can be highly expensive when compared to flat plate collectors or
batch solar collectors. Evacuated tube solar creditors are well-accepted in
commercial (laundromat) and business heating programmers, and they could be a
good alternative to flat-plate creditors for home area heating, particularly in cloudy
locations.
Several rows of glass tubes are connected to a header pipe in an evacuated-tube
collector. The air in each tube is removed (evacuated) to prevent heat loss by
convection and conduction [5]. A steel pipe is connected to a flat or curved
aluminum or copper fin in the glass tube. A selective coating is applied to the fin,
which transfers heat to the fluid flowing through the pipe. There are two types of
creditors for evacuated tubes:
3.1.3.1 Direct-flow evacuated-tube collectors:
Pipes flow down and lower back in the tube of an instantaneous-float evacuated tube
collector. The entrance fluid flows via one pipe, while the output fluid flows through
the other. Because fluid flows into and out of each tube, it is difficult to replace them
without causing difficulties [5]. Also, if a tube breaks, it's possible that all of the
fluid will be pumped out of the machine if it's a closed loop, or your water will flow
out like a broken pipe if it's an open loop
Figure 21 A typical example of Digital Display
Figure 22 A Typical Diagram of components of Evacuated Tube Collector
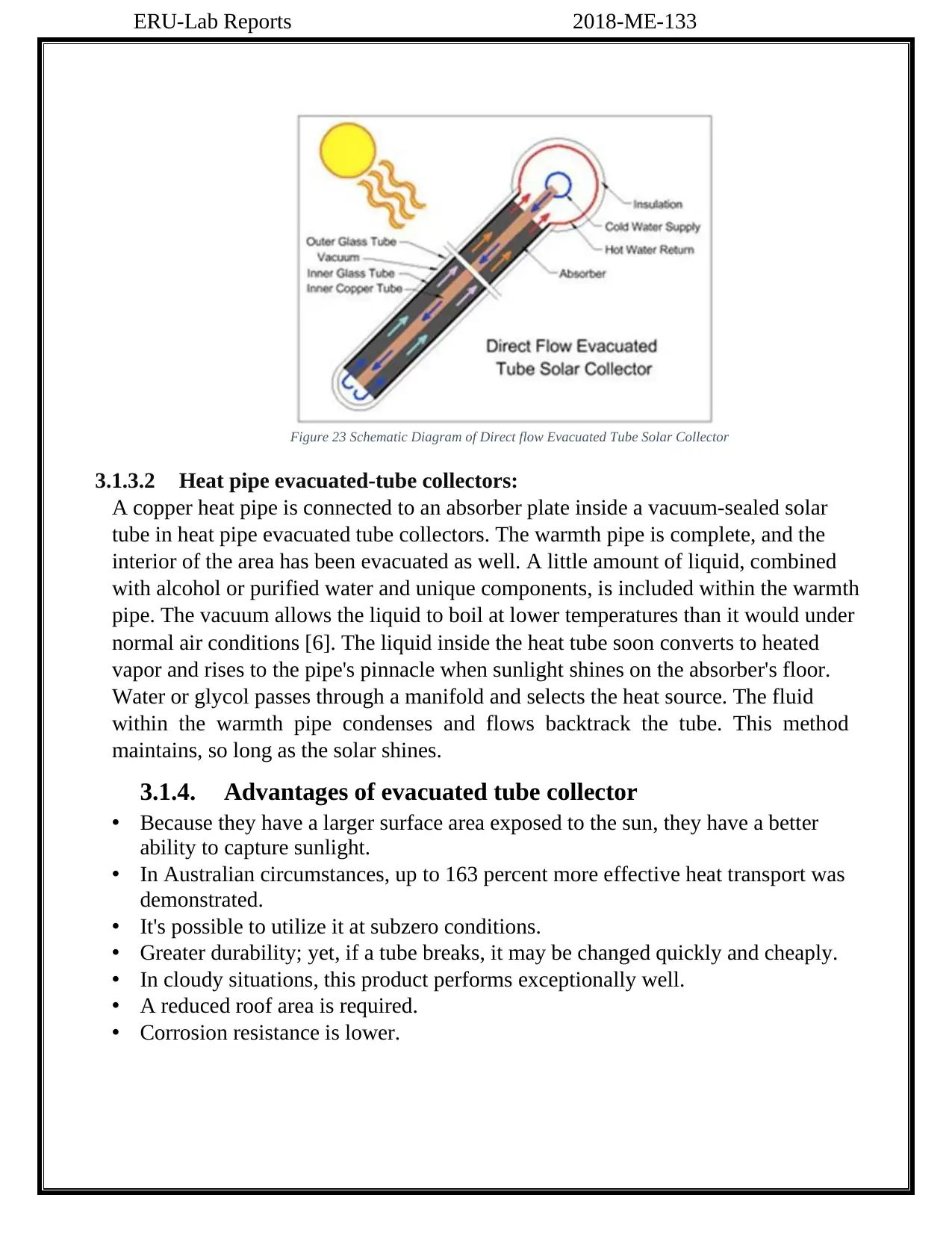
ERU-Lab Reports 2018-ME-133
3.1.3.2 Heat pipe evacuated-tube collectors:
A copper heat pipe is connected to an absorber plate inside a vacuum-sealed solar
tube in heat pipe evacuated tube collectors. The warmth pipe is complete, and the
interior of the area has been evacuated as well. A little amount of liquid, combined
with alcohol or purified water and unique components, is included within the warmth
pipe. The vacuum allows the liquid to boil at lower temperatures than it would under
normal air conditions [6]. The liquid inside the heat tube soon converts to heated
vapor and rises to the pipe's pinnacle when sunlight shines on the absorber's floor.
Water or glycol passes through a manifold and selects the heat source. The fluid
within the warmth pipe condenses and flows backtrack the tube. This method
maintains, so long as the solar shines.
3.1.4. Advantages of evacuated tube collector
• Because they have a larger surface area exposed to the sun, they have a better
ability to capture sunlight.
• In Australian circumstances, up to 163 percent more effective heat transport was
demonstrated.
• It's possible to utilize it at subzero conditions.
• Greater durability; yet, if a tube breaks, it may be changed quickly and cheaply.
• In cloudy situations, this product performs exceptionally well.
• A reduced roof area is required.
• Corrosion resistance is lower.
Figure 23 Schematic Diagram of Direct flow Evacuated Tube Solar Collector
3.1.3.2 Heat pipe evacuated-tube collectors:
A copper heat pipe is connected to an absorber plate inside a vacuum-sealed solar
tube in heat pipe evacuated tube collectors. The warmth pipe is complete, and the
interior of the area has been evacuated as well. A little amount of liquid, combined
with alcohol or purified water and unique components, is included within the warmth
pipe. The vacuum allows the liquid to boil at lower temperatures than it would under
normal air conditions [6]. The liquid inside the heat tube soon converts to heated
vapor and rises to the pipe's pinnacle when sunlight shines on the absorber's floor.
Water or glycol passes through a manifold and selects the heat source. The fluid
within the warmth pipe condenses and flows backtrack the tube. This method
maintains, so long as the solar shines.
3.1.4. Advantages of evacuated tube collector
• Because they have a larger surface area exposed to the sun, they have a better
ability to capture sunlight.
• In Australian circumstances, up to 163 percent more effective heat transport was
demonstrated.
• It's possible to utilize it at subzero conditions.
• Greater durability; yet, if a tube breaks, it may be changed quickly and cheaply.
• In cloudy situations, this product performs exceptionally well.
• A reduced roof area is required.
• Corrosion resistance is lower.
Figure 23 Schematic Diagram of Direct flow Evacuated Tube Solar Collector
Secure Best Marks with AI Grader
Need help grading? Try our AI Grader for instant feedback on your assignments.
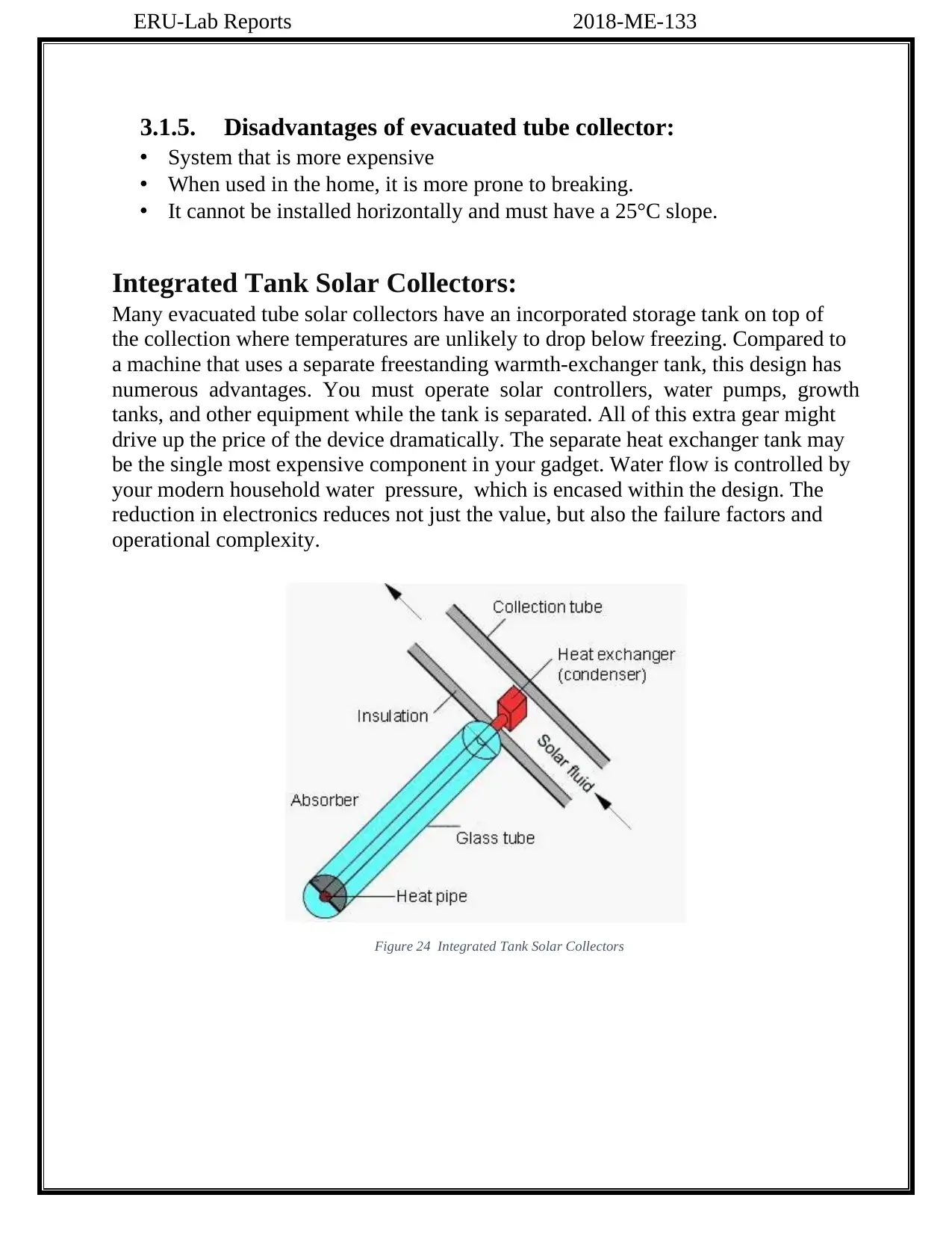
ERU-Lab Reports 2018-ME-133
3.1.5. Disadvantages of evacuated tube collector:
• System that is more expensive
• When used in the home, it is more prone to breaking.
• It cannot be installed horizontally and must have a 25°C slope.
Integrated Tank Solar Collectors:
Many evacuated tube solar collectors have an incorporated storage tank on top of
the collection where temperatures are unlikely to drop below freezing. Compared to
a machine that uses a separate freestanding warmth-exchanger tank, this design has
numerous advantages. You must operate solar controllers, water pumps, growth
tanks, and other equipment while the tank is separated. All of this extra gear might
drive up the price of the device dramatically. The separate heat exchanger tank may
be the single most expensive component in your gadget. Water flow is controlled by
your modern household water pressure, which is encased within the design. The
reduction in electronics reduces not just the value, but also the failure factors and
operational complexity.
Figure 24 Integrated Tank Solar Collectors
3.1.5. Disadvantages of evacuated tube collector:
• System that is more expensive
• When used in the home, it is more prone to breaking.
• It cannot be installed horizontally and must have a 25°C slope.
Integrated Tank Solar Collectors:
Many evacuated tube solar collectors have an incorporated storage tank on top of
the collection where temperatures are unlikely to drop below freezing. Compared to
a machine that uses a separate freestanding warmth-exchanger tank, this design has
numerous advantages. You must operate solar controllers, water pumps, growth
tanks, and other equipment while the tank is separated. All of this extra gear might
drive up the price of the device dramatically. The separate heat exchanger tank may
be the single most expensive component in your gadget. Water flow is controlled by
your modern household water pressure, which is encased within the design. The
reduction in electronics reduces not just the value, but also the failure factors and
operational complexity.
Figure 24 Integrated Tank Solar Collectors
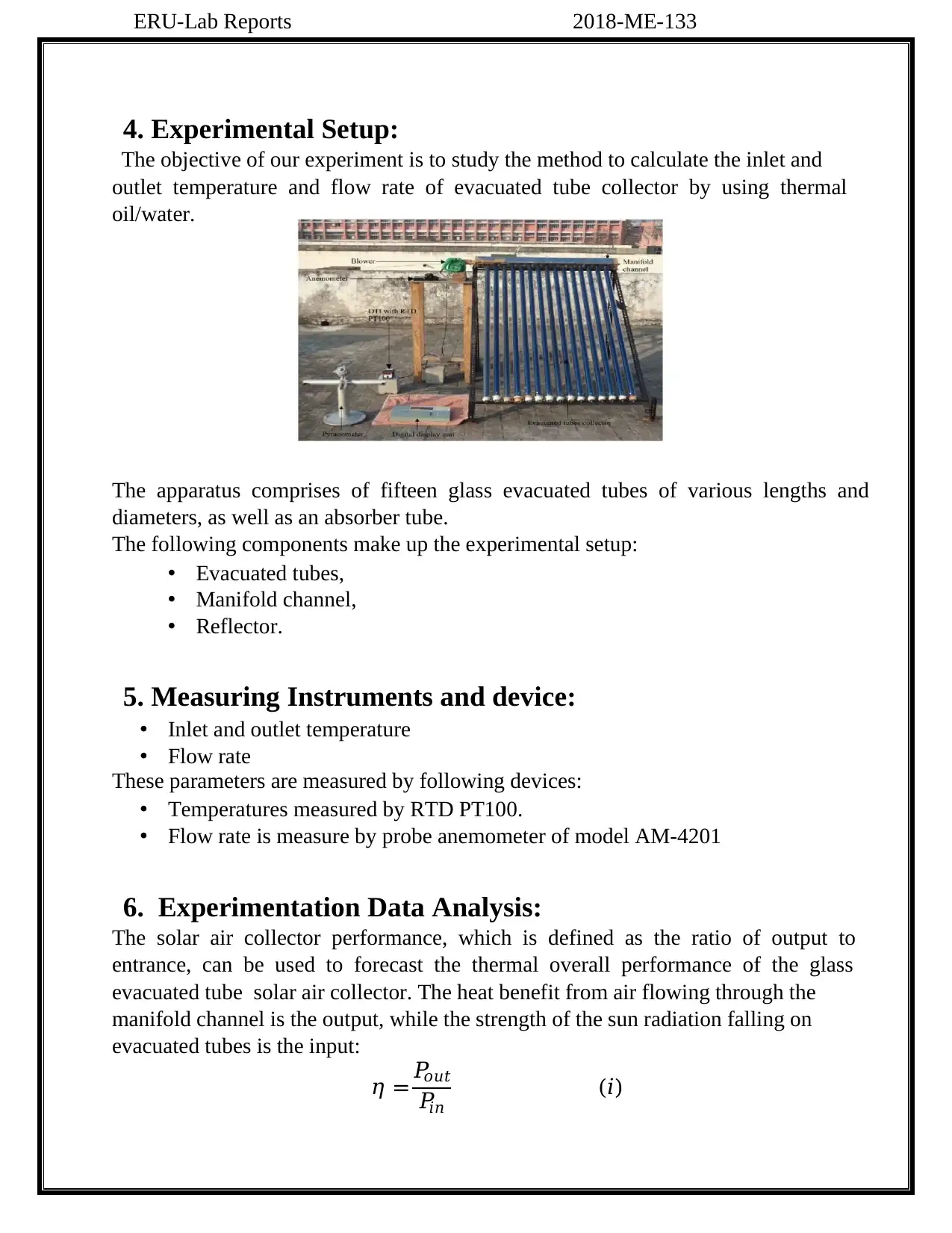
ERU-Lab Reports 2018-ME-133
4. Experimental Setup:
The objective of our experiment is to study the method to calculate the inlet and
outlet temperature and flow rate of evacuated tube collector by using thermal
oil/water.
The apparatus comprises of fifteen glass evacuated tubes of various lengths and
diameters, as well as an absorber tube.
The following components make up the experimental setup:
• Evacuated tubes,
• Manifold channel,
• Reflector.
5. Measuring Instruments and device:
• Inlet and outlet temperature
• Flow rate
These parameters are measured by following devices:
• Temperatures measured by RTD PT100.
• Flow rate is measure by probe anemometer of model AM-4201
6. Experimentation Data Analysis:
The solar air collector performance, which is defined as the ratio of output to
entrance, can be used to forecast the thermal overall performance of the glass
evacuated tube solar air collector. The heat benefit from air flowing through the
manifold channel is the output, while the strength of the sun radiation falling on
evacuated tubes is the input:
𝜂 =𝑃𝑜𝑢𝑡
𝑃𝑖𝑛
(𝑖)
4. Experimental Setup:
The objective of our experiment is to study the method to calculate the inlet and
outlet temperature and flow rate of evacuated tube collector by using thermal
oil/water.
The apparatus comprises of fifteen glass evacuated tubes of various lengths and
diameters, as well as an absorber tube.
The following components make up the experimental setup:
• Evacuated tubes,
• Manifold channel,
• Reflector.
5. Measuring Instruments and device:
• Inlet and outlet temperature
• Flow rate
These parameters are measured by following devices:
• Temperatures measured by RTD PT100.
• Flow rate is measure by probe anemometer of model AM-4201
6. Experimentation Data Analysis:
The solar air collector performance, which is defined as the ratio of output to
entrance, can be used to forecast the thermal overall performance of the glass
evacuated tube solar air collector. The heat benefit from air flowing through the
manifold channel is the output, while the strength of the sun radiation falling on
evacuated tubes is the input:
𝜂 =𝑃𝑜𝑢𝑡
𝑃𝑖𝑛
(𝑖)
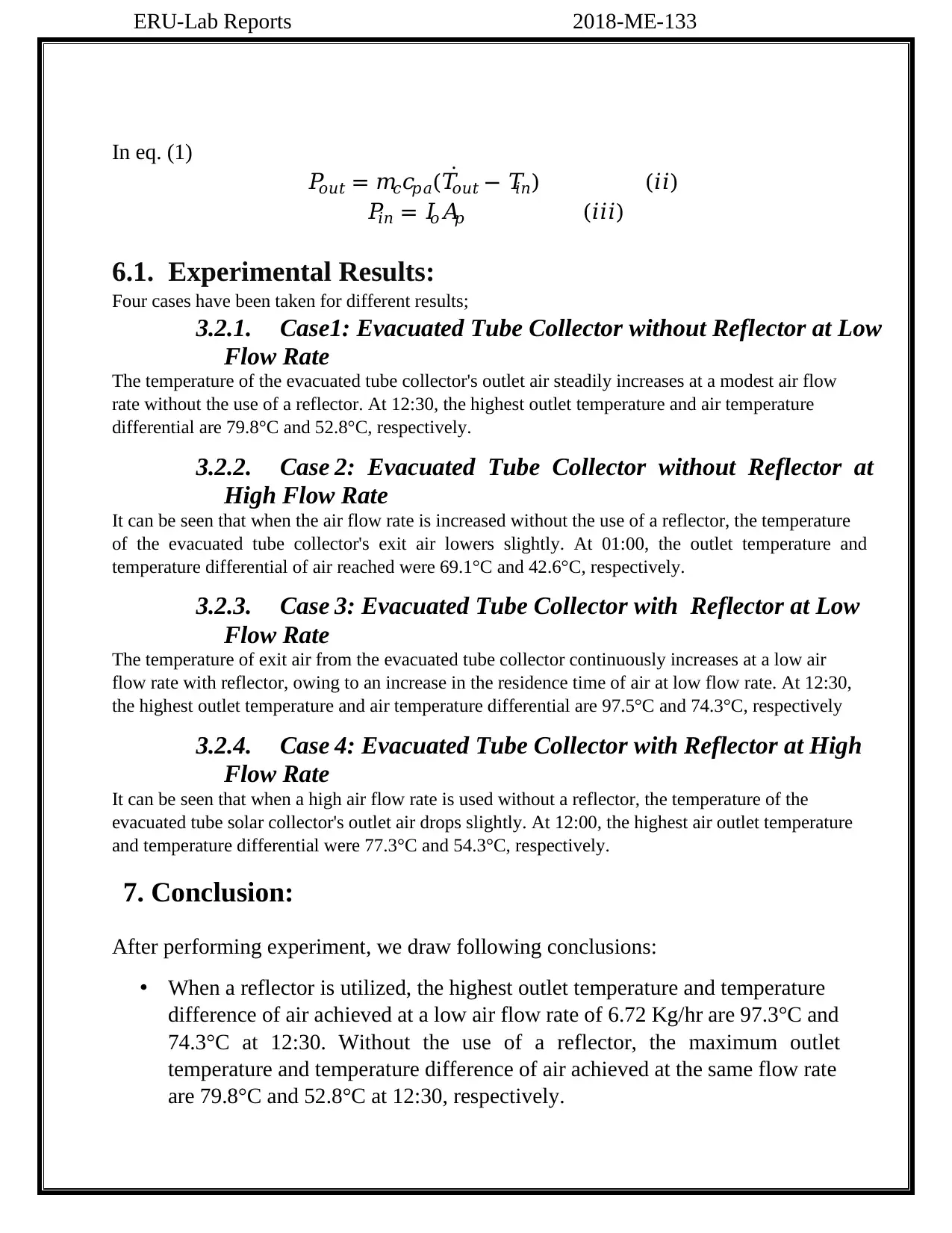
ERU-Lab Reports 2018-ME-133
In eq. (1)
𝑃𝑜𝑢𝑡 = 𝑚𝑐𝑐𝑝𝑎(𝑇𝑜𝑢𝑡 − 𝑇𝑖𝑛)̇ (𝑖𝑖)
𝑃𝑖𝑛 = 𝐼𝑜𝐴𝑝 (𝑖𝑖𝑖)
6.1. Experimental Results:
Four cases have been taken for different results;
3.2.1. Case1: Evacuated Tube Collector without Reflector at Low
Flow Rate
The temperature of the evacuated tube collector's outlet air steadily increases at a modest air flow
rate without the use of a reflector. At 12:30, the highest outlet temperature and air temperature
differential are 79.8°C and 52.8°C, respectively.
3.2.2. Case 2: Evacuated Tube Collector without Reflector at
High Flow Rate
It can be seen that when the air flow rate is increased without the use of a reflector, the temperature
of the evacuated tube collector's exit air lowers slightly. At 01:00, the outlet temperature and
temperature differential of air reached were 69.1°C and 42.6°C, respectively.
3.2.3. Case 3: Evacuated Tube Collector with Reflector at Low
Flow Rate
The temperature of exit air from the evacuated tube collector continuously increases at a low air
flow rate with reflector, owing to an increase in the residence time of air at low flow rate. At 12:30,
the highest outlet temperature and air temperature differential are 97.5°C and 74.3°C, respectively
3.2.4. Case 4: Evacuated Tube Collector with Reflector at High
Flow Rate
It can be seen that when a high air flow rate is used without a reflector, the temperature of the
evacuated tube solar collector's outlet air drops slightly. At 12:00, the highest air outlet temperature
and temperature differential were 77.3°C and 54.3°C, respectively.
7. Conclusion:
After performing experiment, we draw following conclusions:
• When a reflector is utilized, the highest outlet temperature and temperature
difference of air achieved at a low air flow rate of 6.72 Kg/hr are 97.3°C and
74.3°C at 12:30. Without the use of a reflector, the maximum outlet
temperature and temperature difference of air achieved at the same flow rate
are 79.8°C and 52.8°C at 12:30, respectively.
In eq. (1)
𝑃𝑜𝑢𝑡 = 𝑚𝑐𝑐𝑝𝑎(𝑇𝑜𝑢𝑡 − 𝑇𝑖𝑛)̇ (𝑖𝑖)
𝑃𝑖𝑛 = 𝐼𝑜𝐴𝑝 (𝑖𝑖𝑖)
6.1. Experimental Results:
Four cases have been taken for different results;
3.2.1. Case1: Evacuated Tube Collector without Reflector at Low
Flow Rate
The temperature of the evacuated tube collector's outlet air steadily increases at a modest air flow
rate without the use of a reflector. At 12:30, the highest outlet temperature and air temperature
differential are 79.8°C and 52.8°C, respectively.
3.2.2. Case 2: Evacuated Tube Collector without Reflector at
High Flow Rate
It can be seen that when the air flow rate is increased without the use of a reflector, the temperature
of the evacuated tube collector's exit air lowers slightly. At 01:00, the outlet temperature and
temperature differential of air reached were 69.1°C and 42.6°C, respectively.
3.2.3. Case 3: Evacuated Tube Collector with Reflector at Low
Flow Rate
The temperature of exit air from the evacuated tube collector continuously increases at a low air
flow rate with reflector, owing to an increase in the residence time of air at low flow rate. At 12:30,
the highest outlet temperature and air temperature differential are 97.5°C and 74.3°C, respectively
3.2.4. Case 4: Evacuated Tube Collector with Reflector at High
Flow Rate
It can be seen that when a high air flow rate is used without a reflector, the temperature of the
evacuated tube solar collector's outlet air drops slightly. At 12:00, the highest air outlet temperature
and temperature differential were 77.3°C and 54.3°C, respectively.
7. Conclusion:
After performing experiment, we draw following conclusions:
• When a reflector is utilized, the highest outlet temperature and temperature
difference of air achieved at a low air flow rate of 6.72 Kg/hr are 97.3°C and
74.3°C at 12:30. Without the use of a reflector, the maximum outlet
temperature and temperature difference of air achieved at the same flow rate
are 79.8°C and 52.8°C at 12:30, respectively.
Paraphrase This Document
Need a fresh take? Get an instant paraphrase of this document with our AI Paraphraser
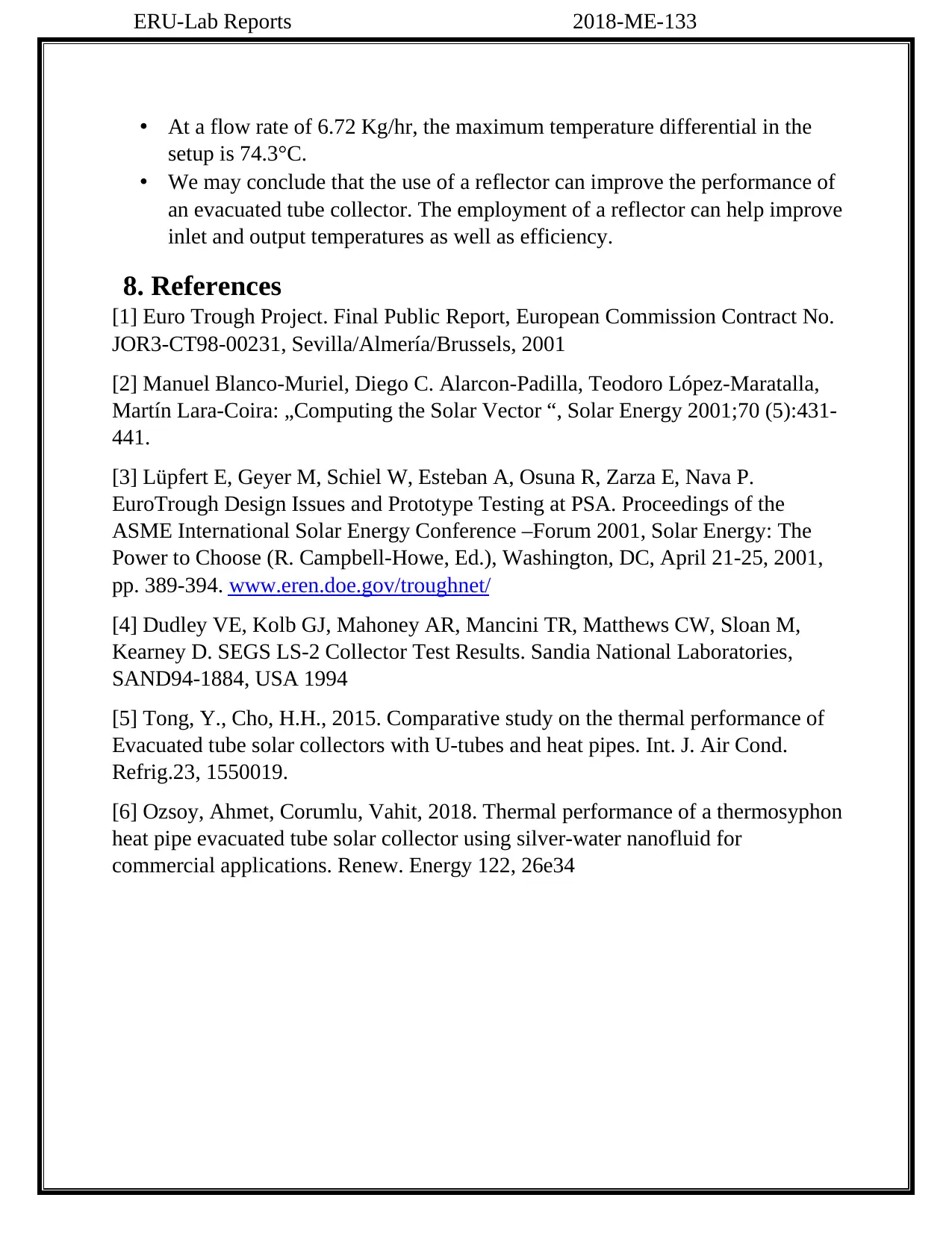
ERU-Lab Reports 2018-ME-133
• At a flow rate of 6.72 Kg/hr, the maximum temperature differential in the
setup is 74.3°C.
• We may conclude that the use of a reflector can improve the performance of
an evacuated tube collector. The employment of a reflector can help improve
inlet and output temperatures as well as efficiency.
8. References
[1] Euro Trough Project. Final Public Report, European Commission Contract No.
JOR3-CT98-00231, Sevilla/Almería/Brussels, 2001
[2] Manuel Blanco-Muriel, Diego C. Alarcon-Padilla, Teodoro López-Maratalla,
Martín Lara-Coira: „Computing the Solar Vector “, Solar Energy 2001;70 (5):431-
441.
[3] Lüpfert E, Geyer M, Schiel W, Esteban A, Osuna R, Zarza E, Nava P.
EuroTrough Design Issues and Prototype Testing at PSA. Proceedings of the
ASME International Solar Energy Conference –Forum 2001, Solar Energy: The
Power to Choose (R. Campbell-Howe, Ed.), Washington, DC, April 21-25, 2001,
pp. 389-394. www.eren.doe.gov/troughnet/
[4] Dudley VE, Kolb GJ, Mahoney AR, Mancini TR, Matthews CW, Sloan M,
Kearney D. SEGS LS-2 Collector Test Results. Sandia National Laboratories,
SAND94-1884, USA 1994
[5] Tong, Y., Cho, H.H., 2015. Comparative study on the thermal performance of
Evacuated tube solar collectors with U-tubes and heat pipes. Int. J. Air Cond.
Refrig.23, 1550019.
[6] Ozsoy, Ahmet, Corumlu, Vahit, 2018. Thermal performance of a thermosyphon
heat pipe evacuated tube solar collector using silver-water nanofluid for
commercial applications. Renew. Energy 122, 26e34
• At a flow rate of 6.72 Kg/hr, the maximum temperature differential in the
setup is 74.3°C.
• We may conclude that the use of a reflector can improve the performance of
an evacuated tube collector. The employment of a reflector can help improve
inlet and output temperatures as well as efficiency.
8. References
[1] Euro Trough Project. Final Public Report, European Commission Contract No.
JOR3-CT98-00231, Sevilla/Almería/Brussels, 2001
[2] Manuel Blanco-Muriel, Diego C. Alarcon-Padilla, Teodoro López-Maratalla,
Martín Lara-Coira: „Computing the Solar Vector “, Solar Energy 2001;70 (5):431-
441.
[3] Lüpfert E, Geyer M, Schiel W, Esteban A, Osuna R, Zarza E, Nava P.
EuroTrough Design Issues and Prototype Testing at PSA. Proceedings of the
ASME International Solar Energy Conference –Forum 2001, Solar Energy: The
Power to Choose (R. Campbell-Howe, Ed.), Washington, DC, April 21-25, 2001,
pp. 389-394. www.eren.doe.gov/troughnet/
[4] Dudley VE, Kolb GJ, Mahoney AR, Mancini TR, Matthews CW, Sloan M,
Kearney D. SEGS LS-2 Collector Test Results. Sandia National Laboratories,
SAND94-1884, USA 1994
[5] Tong, Y., Cho, H.H., 2015. Comparative study on the thermal performance of
Evacuated tube solar collectors with U-tubes and heat pipes. Int. J. Air Cond.
Refrig.23, 1550019.
[6] Ozsoy, Ahmet, Corumlu, Vahit, 2018. Thermal performance of a thermosyphon
heat pipe evacuated tube solar collector using silver-water nanofluid for
commercial applications. Renew. Energy 122, 26e34
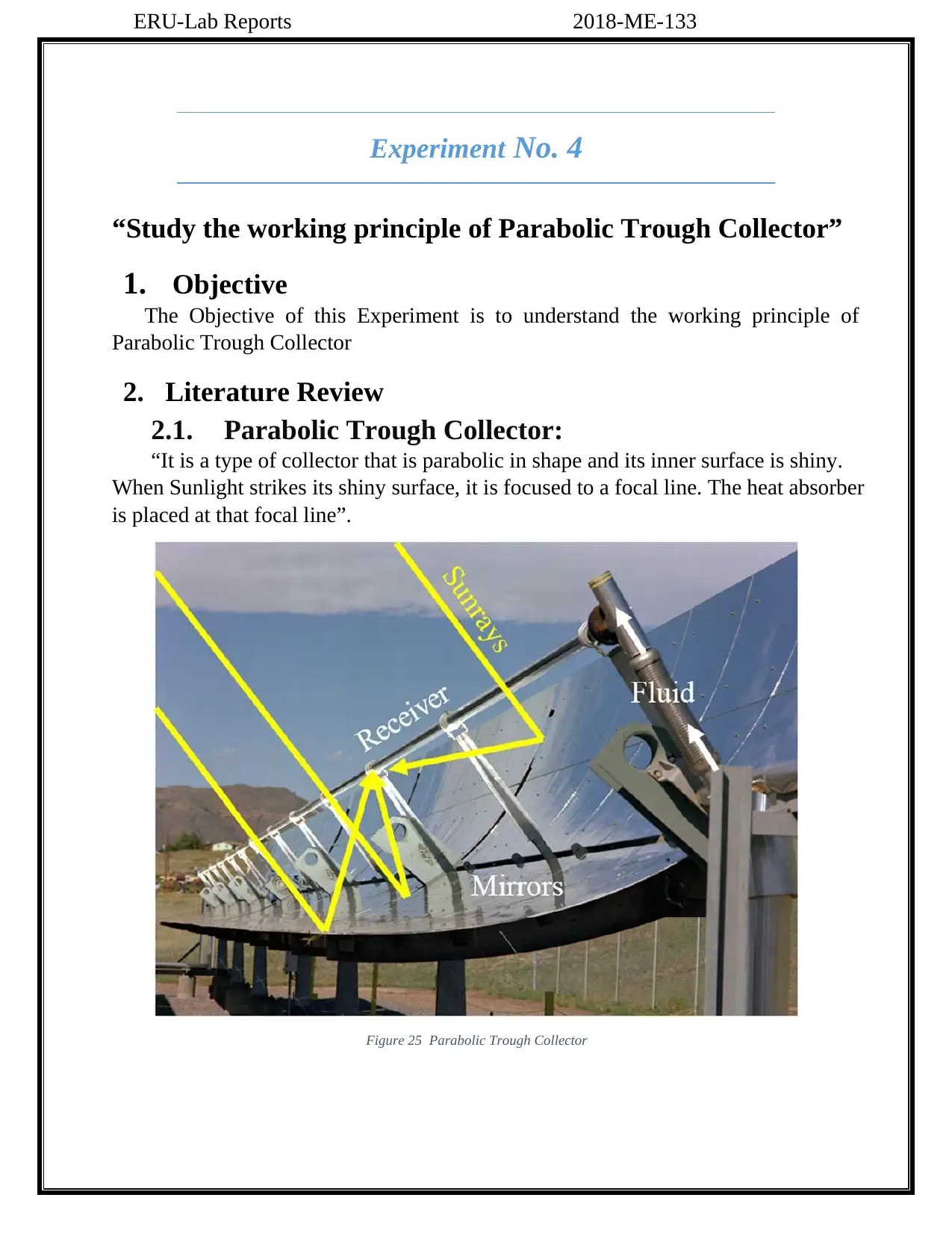
ERU-Lab Reports 2018-ME-133
Experiment No. 4
“Study the working principle of Parabolic Trough Collector”
1. Objective
The Objective of this Experiment is to understand the working principle of
Parabolic Trough Collector
2. Literature Review
2.1. Parabolic Trough Collector:
“It is a type of collector that is parabolic in shape and its inner surface is shiny.
When Sunlight strikes its shiny surface, it is focused to a focal line. The heat absorber
is placed at that focal line”.
Figure 25 Parabolic Trough Collector
Experiment No. 4
“Study the working principle of Parabolic Trough Collector”
1. Objective
The Objective of this Experiment is to understand the working principle of
Parabolic Trough Collector
2. Literature Review
2.1. Parabolic Trough Collector:
“It is a type of collector that is parabolic in shape and its inner surface is shiny.
When Sunlight strikes its shiny surface, it is focused to a focal line. The heat absorber
is placed at that focal line”.
Figure 25 Parabolic Trough Collector
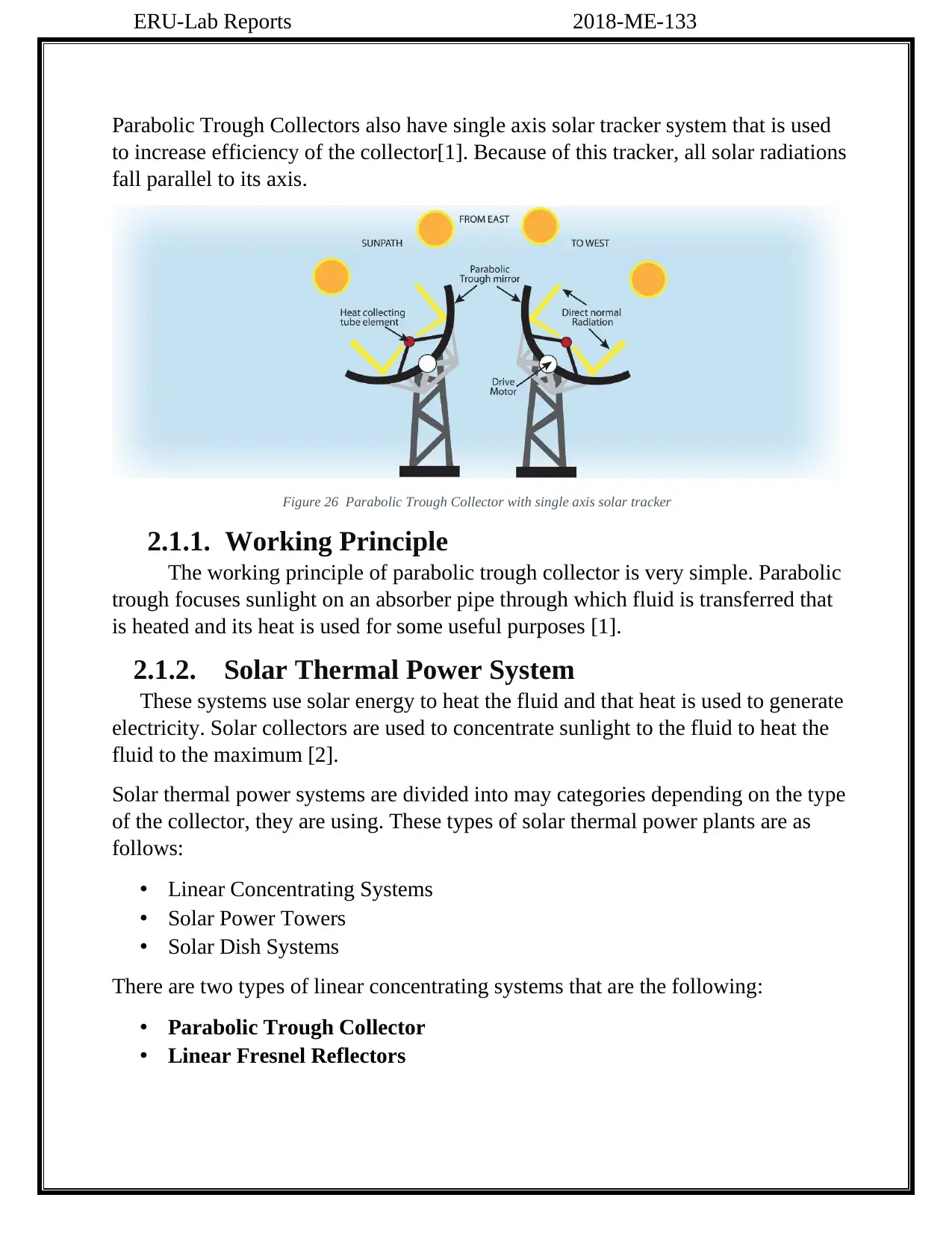
ERU-Lab Reports 2018-ME-133
Parabolic Trough Collectors also have single axis solar tracker system that is used
to increase efficiency of the collector[1]. Because of this tracker, all solar radiations
fall parallel to its axis.
Figure 26 Parabolic Trough Collector with single axis solar tracker
2.1.1. Working Principle
The working principle of parabolic trough collector is very simple. Parabolic
trough focuses sunlight on an absorber pipe through which fluid is transferred that
is heated and its heat is used for some useful purposes [1].
2.1.2. Solar Thermal Power System
These systems use solar energy to heat the fluid and that heat is used to generate
electricity. Solar collectors are used to concentrate sunlight to the fluid to heat the
fluid to the maximum [2].
Solar thermal power systems are divided into may categories depending on the type
of the collector, they are using. These types of solar thermal power plants are as
follows:
• Linear Concentrating Systems
• Solar Power Towers
• Solar Dish Systems
There are two types of linear concentrating systems that are the following:
• Parabolic Trough Collector
• Linear Fresnel Reflectors
Parabolic Trough Collectors also have single axis solar tracker system that is used
to increase efficiency of the collector[1]. Because of this tracker, all solar radiations
fall parallel to its axis.
Figure 26 Parabolic Trough Collector with single axis solar tracker
2.1.1. Working Principle
The working principle of parabolic trough collector is very simple. Parabolic
trough focuses sunlight on an absorber pipe through which fluid is transferred that
is heated and its heat is used for some useful purposes [1].
2.1.2. Solar Thermal Power System
These systems use solar energy to heat the fluid and that heat is used to generate
electricity. Solar collectors are used to concentrate sunlight to the fluid to heat the
fluid to the maximum [2].
Solar thermal power systems are divided into may categories depending on the type
of the collector, they are using. These types of solar thermal power plants are as
follows:
• Linear Concentrating Systems
• Solar Power Towers
• Solar Dish Systems
There are two types of linear concentrating systems that are the following:
• Parabolic Trough Collector
• Linear Fresnel Reflectors
Secure Best Marks with AI Grader
Need help grading? Try our AI Grader for instant feedback on your assignments.
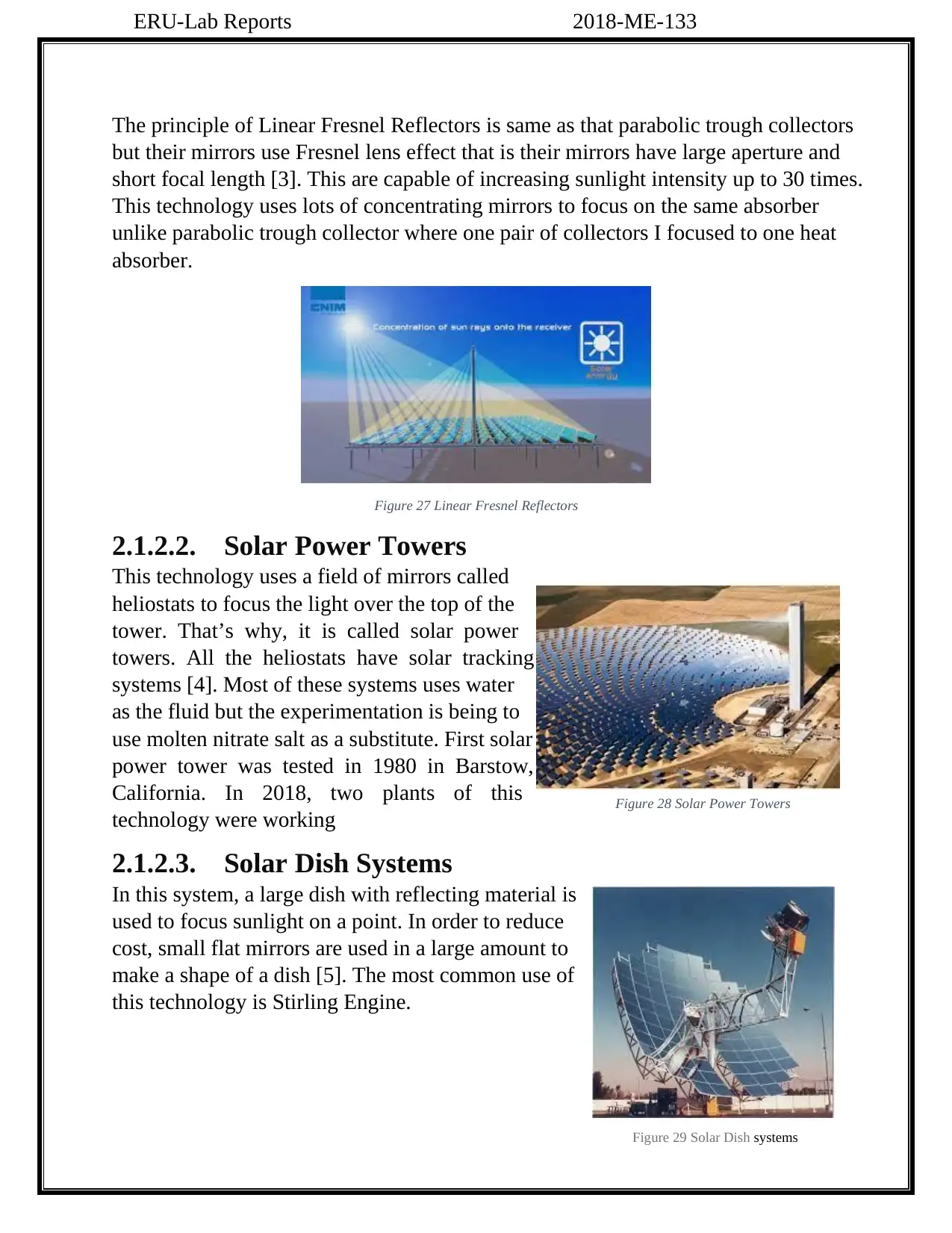
ERU-Lab Reports 2018-ME-133
The principle of Linear Fresnel Reflectors is same as that parabolic trough collectors
but their mirrors use Fresnel lens effect that is their mirrors have large aperture and
short focal length [3]. This are capable of increasing sunlight intensity up to 30 times.
This technology uses lots of concentrating mirrors to focus on the same absorber
unlike parabolic trough collector where one pair of collectors I focused to one heat
absorber.
Figure 27 Linear Fresnel Reflectors
2.1.2.2. Solar Power Towers
This technology uses a field of mirrors called
heliostats to focus the light over the top of the
tower. That’s why, it is called solar power
towers. All the heliostats have solar tracking
systems [4]. Most of these systems uses water
as the fluid but the experimentation is being to
use molten nitrate salt as a substitute. First solar
power tower was tested in 1980 in Barstow,
California. In 2018, two plants of this
technology were working
2.1.2.3. Solar Dish Systems
In this system, a large dish with reflecting material is
used to focus sunlight on a point. In order to reduce
cost, small flat mirrors are used in a large amount to
make a shape of a dish [5]. The most common use of
this technology is Stirling Engine.
Figure 28 Solar Power Towers
Figure 29 Solar Dish systems
The principle of Linear Fresnel Reflectors is same as that parabolic trough collectors
but their mirrors use Fresnel lens effect that is their mirrors have large aperture and
short focal length [3]. This are capable of increasing sunlight intensity up to 30 times.
This technology uses lots of concentrating mirrors to focus on the same absorber
unlike parabolic trough collector where one pair of collectors I focused to one heat
absorber.
Figure 27 Linear Fresnel Reflectors
2.1.2.2. Solar Power Towers
This technology uses a field of mirrors called
heliostats to focus the light over the top of the
tower. That’s why, it is called solar power
towers. All the heliostats have solar tracking
systems [4]. Most of these systems uses water
as the fluid but the experimentation is being to
use molten nitrate salt as a substitute. First solar
power tower was tested in 1980 in Barstow,
California. In 2018, two plants of this
technology were working
2.1.2.3. Solar Dish Systems
In this system, a large dish with reflecting material is
used to focus sunlight on a point. In order to reduce
cost, small flat mirrors are used in a large amount to
make a shape of a dish [5]. The most common use of
this technology is Stirling Engine.
Figure 28 Solar Power Towers
Figure 29 Solar Dish systems
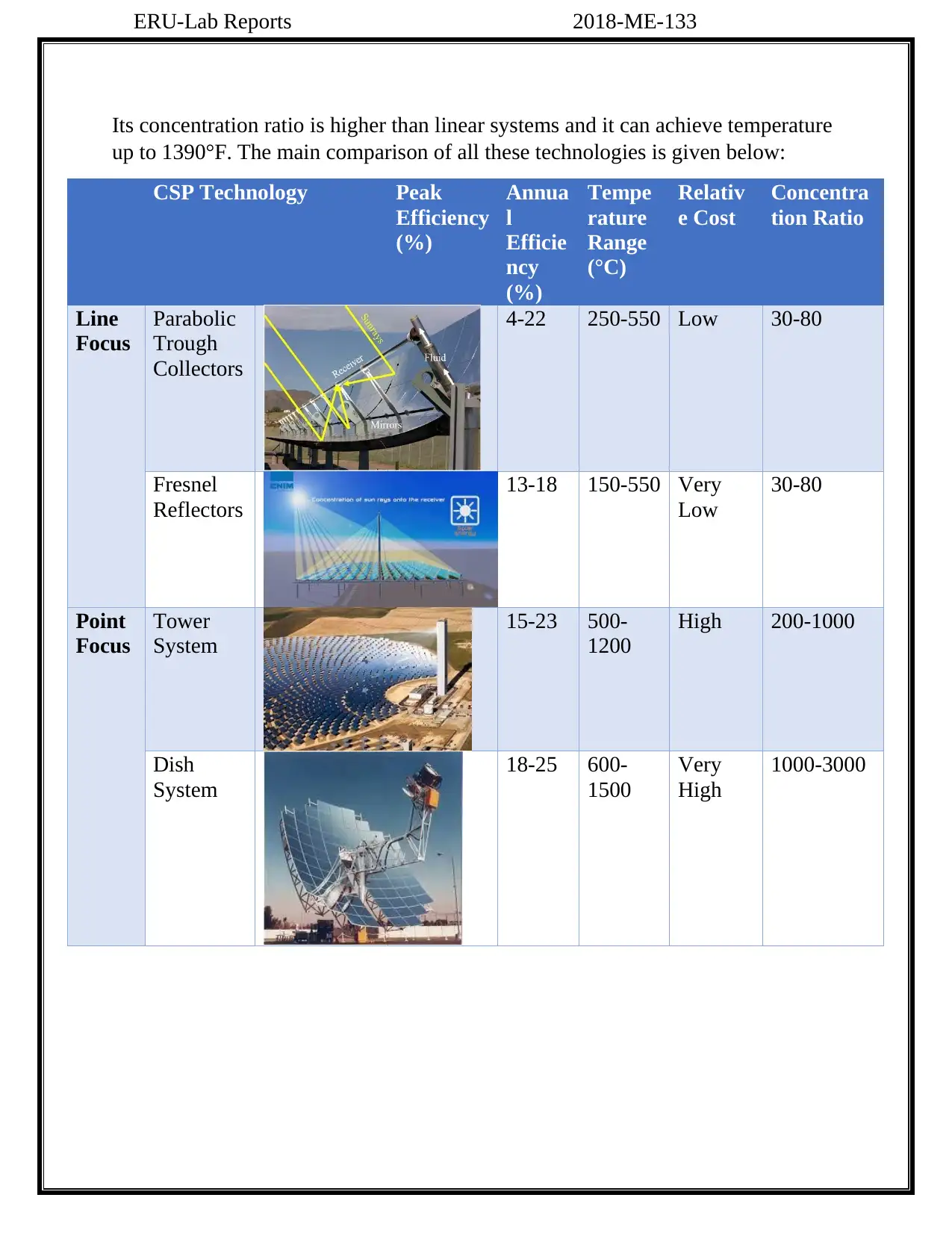
ERU-Lab Reports 2018-ME-133
Its concentration ratio is higher than linear systems and it can achieve temperature
up to 1390°F. The main comparison of all these technologies is given below:
CSP Technology Peak
Efficiency
(%)
Annua
l
Efficie
ncy
(%)
Tempe
rature
Range
(°C)
Relativ
e Cost
Concentra
tion Ratio
Line
Focus
Parabolic
Trough
Collectors
20-25 4-22 250-550 Low 30-80
Fresnel
Reflectors
18-20 13-18 150-550 Very
Low
30-80
Point
Focus
Tower
System
22-24 15-23 500-
1200
High 200-1000
Dish
System
28-32 18-25 600-
1500
Very
High
1000-3000
Its concentration ratio is higher than linear systems and it can achieve temperature
up to 1390°F. The main comparison of all these technologies is given below:
CSP Technology Peak
Efficiency
(%)
Annua
l
Efficie
ncy
(%)
Tempe
rature
Range
(°C)
Relativ
e Cost
Concentra
tion Ratio
Line
Focus
Parabolic
Trough
Collectors
20-25 4-22 250-550 Low 30-80
Fresnel
Reflectors
18-20 13-18 150-550 Very
Low
30-80
Point
Focus
Tower
System
22-24 15-23 500-
1200
High 200-1000
Dish
System
28-32 18-25 600-
1500
Very
High
1000-3000
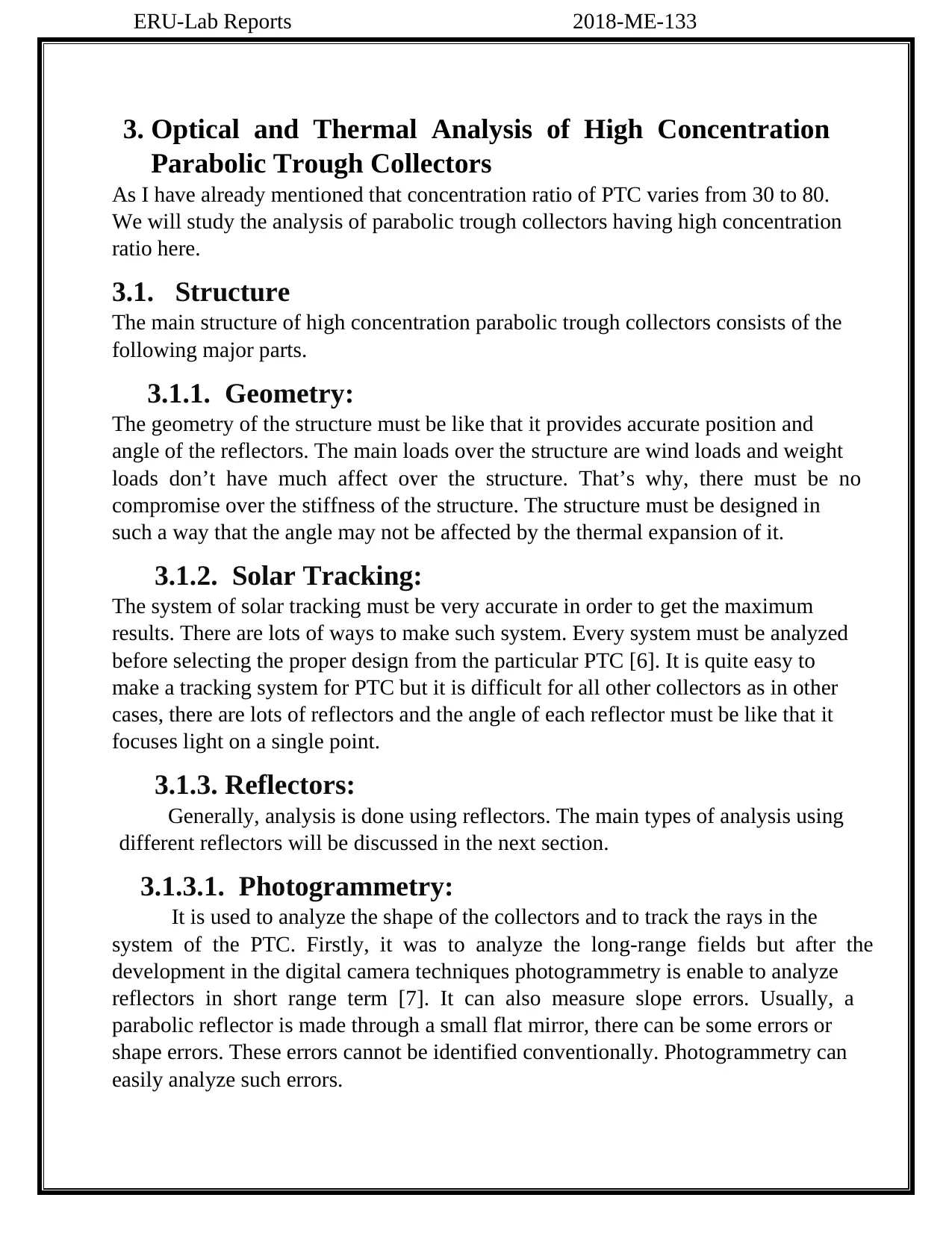
ERU-Lab Reports 2018-ME-133
3. Optical and Thermal Analysis of High Concentration
Parabolic Trough Collectors
As I have already mentioned that concentration ratio of PTC varies from 30 to 80.
We will study the analysis of parabolic trough collectors having high concentration
ratio here.
3.1. Structure
The main structure of high concentration parabolic trough collectors consists of the
following major parts.
3.1.1. Geometry:
The geometry of the structure must be like that it provides accurate position and
angle of the reflectors. The main loads over the structure are wind loads and weight
loads don’t have much affect over the structure. That’s why, there must be no
compromise over the stiffness of the structure. The structure must be designed in
such a way that the angle may not be affected by the thermal expansion of it.
3.1.2. Solar Tracking:
The system of solar tracking must be very accurate in order to get the maximum
results. There are lots of ways to make such system. Every system must be analyzed
before selecting the proper design from the particular PTC [6]. It is quite easy to
make a tracking system for PTC but it is difficult for all other collectors as in other
cases, there are lots of reflectors and the angle of each reflector must be like that it
focuses light on a single point.
3.1.3. Reflectors:
Generally, analysis is done using reflectors. The main types of analysis using
different reflectors will be discussed in the next section.
3.1.3.1. Photogrammetry:
It is used to analyze the shape of the collectors and to track the rays in the
system of the PTC. Firstly, it was to analyze the long-range fields but after the
development in the digital camera techniques photogrammetry is enable to analyze
reflectors in short range term [7]. It can also measure slope errors. Usually, a
parabolic reflector is made through a small flat mirror, there can be some errors or
shape errors. These errors cannot be identified conventionally. Photogrammetry can
easily analyze such errors.
3. Optical and Thermal Analysis of High Concentration
Parabolic Trough Collectors
As I have already mentioned that concentration ratio of PTC varies from 30 to 80.
We will study the analysis of parabolic trough collectors having high concentration
ratio here.
3.1. Structure
The main structure of high concentration parabolic trough collectors consists of the
following major parts.
3.1.1. Geometry:
The geometry of the structure must be like that it provides accurate position and
angle of the reflectors. The main loads over the structure are wind loads and weight
loads don’t have much affect over the structure. That’s why, there must be no
compromise over the stiffness of the structure. The structure must be designed in
such a way that the angle may not be affected by the thermal expansion of it.
3.1.2. Solar Tracking:
The system of solar tracking must be very accurate in order to get the maximum
results. There are lots of ways to make such system. Every system must be analyzed
before selecting the proper design from the particular PTC [6]. It is quite easy to
make a tracking system for PTC but it is difficult for all other collectors as in other
cases, there are lots of reflectors and the angle of each reflector must be like that it
focuses light on a single point.
3.1.3. Reflectors:
Generally, analysis is done using reflectors. The main types of analysis using
different reflectors will be discussed in the next section.
3.1.3.1. Photogrammetry:
It is used to analyze the shape of the collectors and to track the rays in the
system of the PTC. Firstly, it was to analyze the long-range fields but after the
development in the digital camera techniques photogrammetry is enable to analyze
reflectors in short range term [7]. It can also measure slope errors. Usually, a
parabolic reflector is made through a small flat mirror, there can be some errors or
shape errors. These errors cannot be identified conventionally. Photogrammetry can
easily analyze such errors.
Paraphrase This Document
Need a fresh take? Get an instant paraphrase of this document with our AI Paraphraser
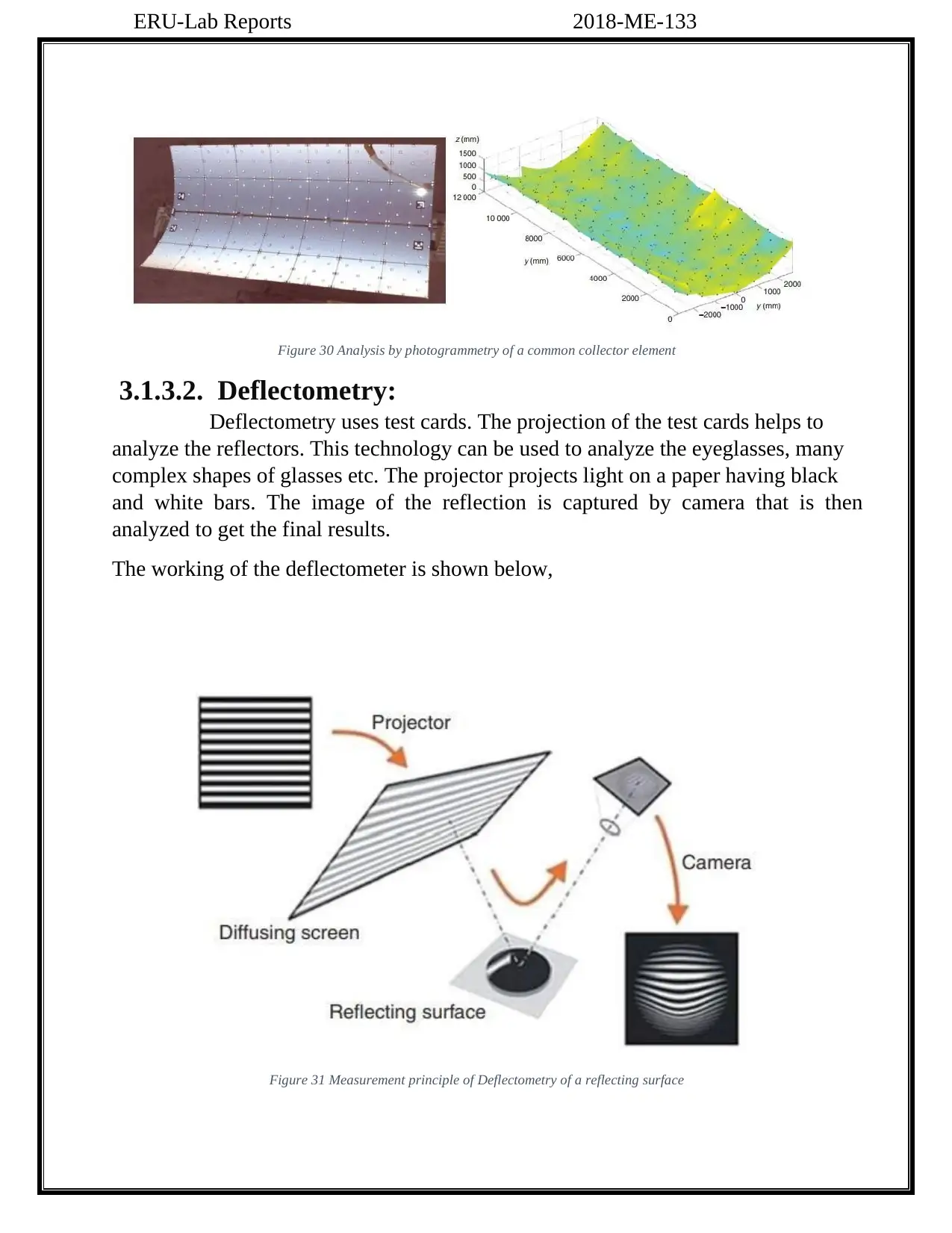
ERU-Lab Reports 2018-ME-133
Figure 30 Analysis by photogrammetry of a common collector element
3.1.3.2. Deflectometry:
Deflectometry uses test cards. The projection of the test cards helps to
analyze the reflectors. This technology can be used to analyze the eyeglasses, many
complex shapes of glasses etc. The projector projects light on a paper having black
and white bars. The image of the reflection is captured by camera that is then
analyzed to get the final results.
The working of the deflectometer is shown below,
Figure 31 Measurement principle of Deflectometry of a reflecting surface
Figure 30 Analysis by photogrammetry of a common collector element
3.1.3.2. Deflectometry:
Deflectometry uses test cards. The projection of the test cards helps to
analyze the reflectors. This technology can be used to analyze the eyeglasses, many
complex shapes of glasses etc. The projector projects light on a paper having black
and white bars. The image of the reflection is captured by camera that is then
analyzed to get the final results.
The working of the deflectometer is shown below,
Figure 31 Measurement principle of Deflectometry of a reflecting surface
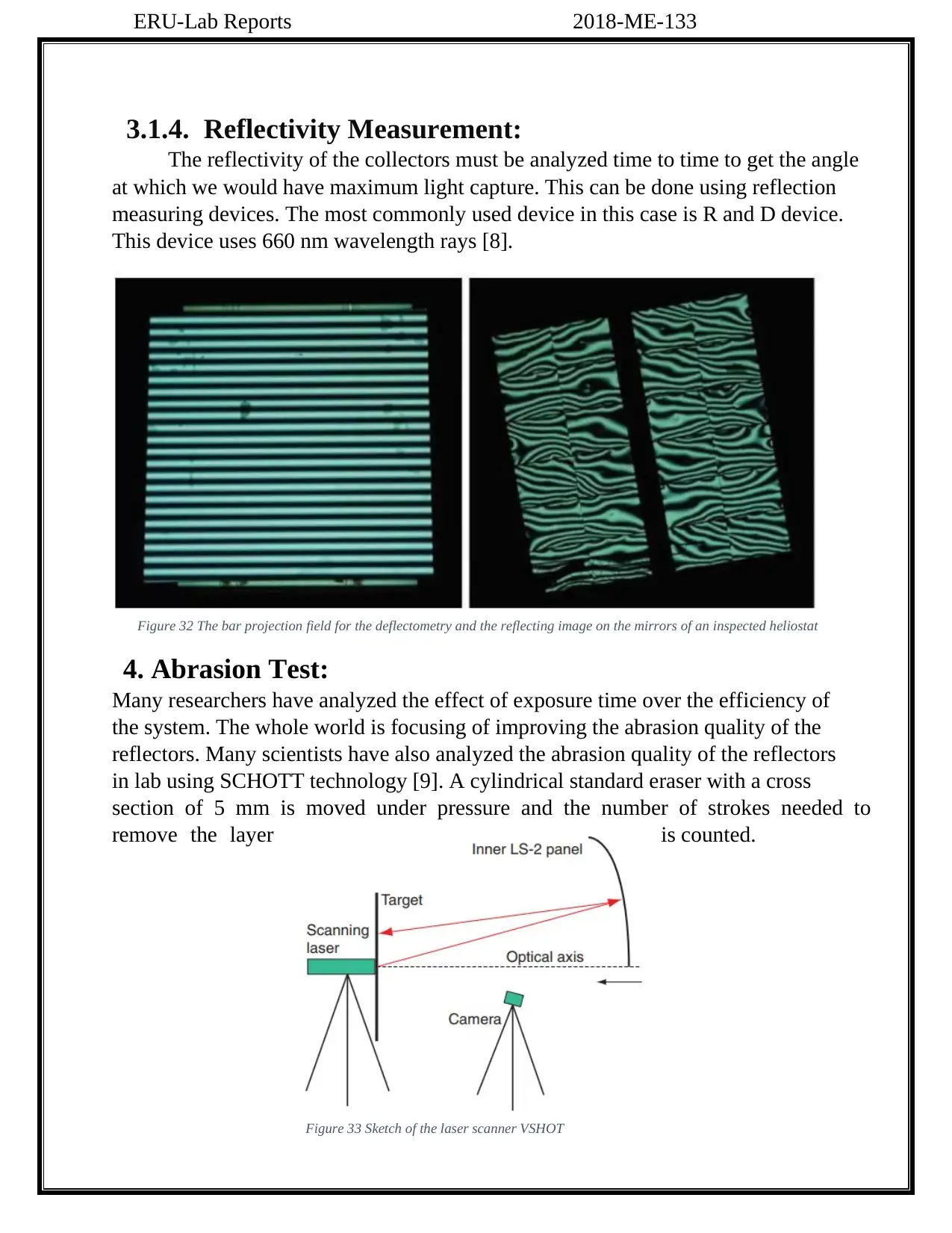
ERU-Lab Reports 2018-ME-133
3.1.4. Reflectivity Measurement:
The reflectivity of the collectors must be analyzed time to time to get the angle
at which we would have maximum light capture. This can be done using reflection
measuring devices. The most commonly used device in this case is R and D device.
This device uses 660 nm wavelength rays [8].
Figure 32 The bar projection field for the deflectometry and the reflecting image on the mirrors of an inspected heliostat
4. Abrasion Test:
Many researchers have analyzed the effect of exposure time over the efficiency of
the system. The whole world is focusing of improving the abrasion quality of the
reflectors. Many scientists have also analyzed the abrasion quality of the reflectors
in lab using SCHOTT technology [9]. A cylindrical standard eraser with a cross
section of 5 mm is moved under pressure and the number of strokes needed to
remove the layer is counted.
Figure 33 Sketch of the laser scanner VSHOT
3.1.4. Reflectivity Measurement:
The reflectivity of the collectors must be analyzed time to time to get the angle
at which we would have maximum light capture. This can be done using reflection
measuring devices. The most commonly used device in this case is R and D device.
This device uses 660 nm wavelength rays [8].
Figure 32 The bar projection field for the deflectometry and the reflecting image on the mirrors of an inspected heliostat
4. Abrasion Test:
Many researchers have analyzed the effect of exposure time over the efficiency of
the system. The whole world is focusing of improving the abrasion quality of the
reflectors. Many scientists have also analyzed the abrasion quality of the reflectors
in lab using SCHOTT technology [9]. A cylindrical standard eraser with a cross
section of 5 mm is moved under pressure and the number of strokes needed to
remove the layer is counted.
Figure 33 Sketch of the laser scanner VSHOT
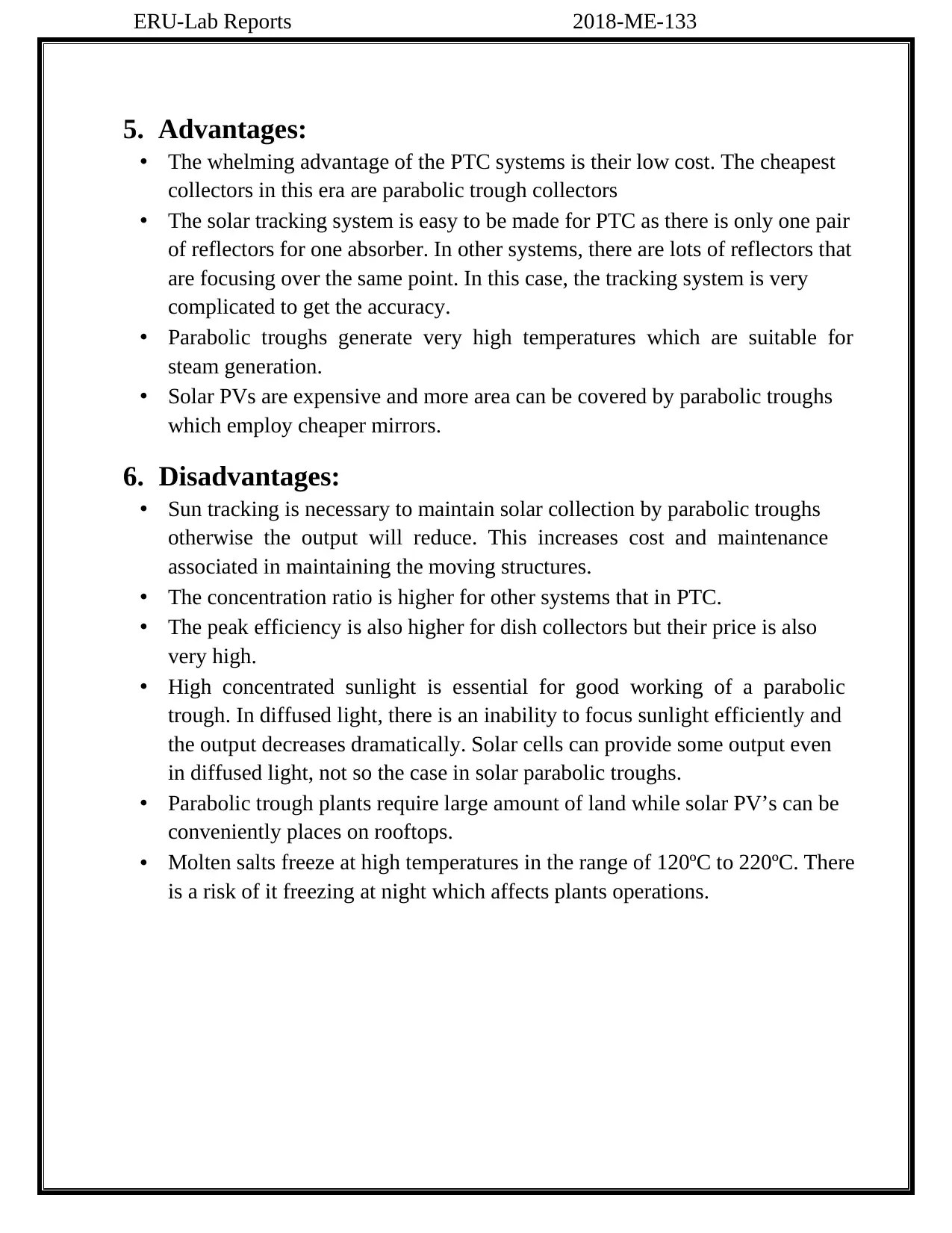
ERU-Lab Reports 2018-ME-133
5. Advantages:
• The whelming advantage of the PTC systems is their low cost. The cheapest
collectors in this era are parabolic trough collectors
• The solar tracking system is easy to be made for PTC as there is only one pair
of reflectors for one absorber. In other systems, there are lots of reflectors that
are focusing over the same point. In this case, the tracking system is very
complicated to get the accuracy.
• Parabolic troughs generate very high temperatures which are suitable for
steam generation.
• Solar PVs are expensive and more area can be covered by parabolic troughs
which employ cheaper mirrors.
6. Disadvantages:
• Sun tracking is necessary to maintain solar collection by parabolic troughs
otherwise the output will reduce. This increases cost and maintenance
associated in maintaining the moving structures.
• The concentration ratio is higher for other systems that in PTC.
• The peak efficiency is also higher for dish collectors but their price is also
very high.
• High concentrated sunlight is essential for good working of a parabolic
trough. In diffused light, there is an inability to focus sunlight efficiently and
the output decreases dramatically. Solar cells can provide some output even
in diffused light, not so the case in solar parabolic troughs.
• Parabolic trough plants require large amount of land while solar PV’s can be
conveniently places on rooftops.
• Molten salts freeze at high temperatures in the range of 120ºC to 220ºC. There
is a risk of it freezing at night which affects plants operations.
5. Advantages:
• The whelming advantage of the PTC systems is their low cost. The cheapest
collectors in this era are parabolic trough collectors
• The solar tracking system is easy to be made for PTC as there is only one pair
of reflectors for one absorber. In other systems, there are lots of reflectors that
are focusing over the same point. In this case, the tracking system is very
complicated to get the accuracy.
• Parabolic troughs generate very high temperatures which are suitable for
steam generation.
• Solar PVs are expensive and more area can be covered by parabolic troughs
which employ cheaper mirrors.
6. Disadvantages:
• Sun tracking is necessary to maintain solar collection by parabolic troughs
otherwise the output will reduce. This increases cost and maintenance
associated in maintaining the moving structures.
• The concentration ratio is higher for other systems that in PTC.
• The peak efficiency is also higher for dish collectors but their price is also
very high.
• High concentrated sunlight is essential for good working of a parabolic
trough. In diffused light, there is an inability to focus sunlight efficiently and
the output decreases dramatically. Solar cells can provide some output even
in diffused light, not so the case in solar parabolic troughs.
• Parabolic trough plants require large amount of land while solar PV’s can be
conveniently places on rooftops.
• Molten salts freeze at high temperatures in the range of 120ºC to 220ºC. There
is a risk of it freezing at night which affects plants operations.
Secure Best Marks with AI Grader
Need help grading? Try our AI Grader for instant feedback on your assignments.
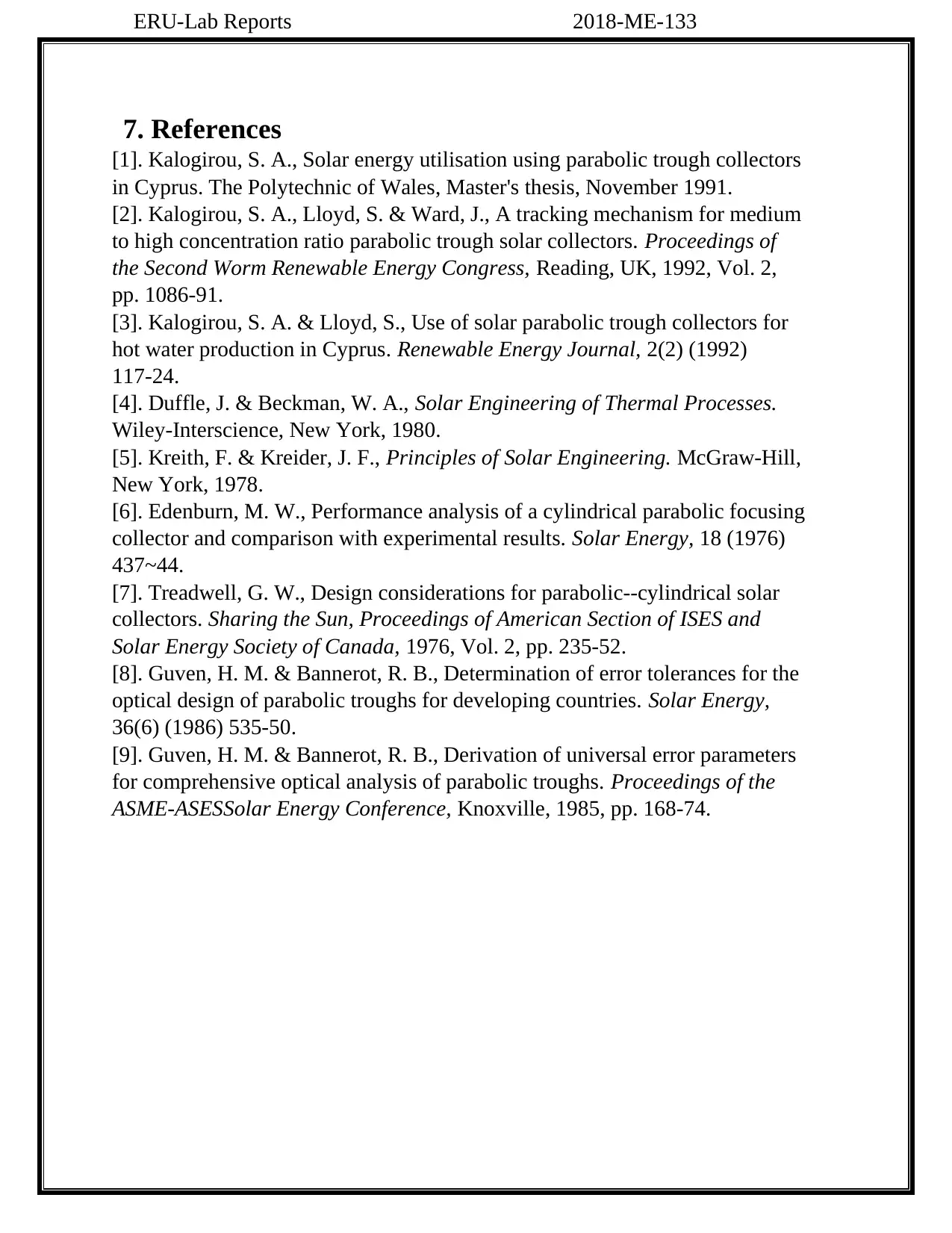
ERU-Lab Reports 2018-ME-133
7. References
[1]. Kalogirou, S. A., Solar energy utilisation using parabolic trough collectors
in Cyprus. The Polytechnic of Wales, Master's thesis, November 1991.
[2]. Kalogirou, S. A., Lloyd, S. & Ward, J., A tracking mechanism for medium
to high concentration ratio parabolic trough solar collectors. Proceedings of
the Second Worm Renewable Energy Congress, Reading, UK, 1992, Vol. 2,
pp. 1086-91.
[3]. Kalogirou, S. A. & Lloyd, S., Use of solar parabolic trough collectors for
hot water production in Cyprus. Renewable Energy Journal, 2(2) (1992)
117-24.
[4]. Duffle, J. & Beckman, W. A., Solar Engineering of Thermal Processes.
Wiley-Interscience, New York, 1980.
[5]. Kreith, F. & Kreider, J. F., Principles of Solar Engineering. McGraw-Hill,
New York, 1978.
[6]. Edenburn, M. W., Performance analysis of a cylindrical parabolic focusing
collector and comparison with experimental results. Solar Energy, 18 (1976)
437~44.
[7]. Treadwell, G. W., Design considerations for parabolic--cylindrical solar
collectors. Sharing the Sun, Proceedings of American Section of ISES and
Solar Energy Society of Canada, 1976, Vol. 2, pp. 235-52.
[8]. Guven, H. M. & Bannerot, R. B., Determination of error tolerances for the
optical design of parabolic troughs for developing countries. Solar Energy,
36(6) (1986) 535-50.
[9]. Guven, H. M. & Bannerot, R. B., Derivation of universal error parameters
for comprehensive optical analysis of parabolic troughs. Proceedings of the
ASME-ASESSolar Energy Conference, Knoxville, 1985, pp. 168-74.
7. References
[1]. Kalogirou, S. A., Solar energy utilisation using parabolic trough collectors
in Cyprus. The Polytechnic of Wales, Master's thesis, November 1991.
[2]. Kalogirou, S. A., Lloyd, S. & Ward, J., A tracking mechanism for medium
to high concentration ratio parabolic trough solar collectors. Proceedings of
the Second Worm Renewable Energy Congress, Reading, UK, 1992, Vol. 2,
pp. 1086-91.
[3]. Kalogirou, S. A. & Lloyd, S., Use of solar parabolic trough collectors for
hot water production in Cyprus. Renewable Energy Journal, 2(2) (1992)
117-24.
[4]. Duffle, J. & Beckman, W. A., Solar Engineering of Thermal Processes.
Wiley-Interscience, New York, 1980.
[5]. Kreith, F. & Kreider, J. F., Principles of Solar Engineering. McGraw-Hill,
New York, 1978.
[6]. Edenburn, M. W., Performance analysis of a cylindrical parabolic focusing
collector and comparison with experimental results. Solar Energy, 18 (1976)
437~44.
[7]. Treadwell, G. W., Design considerations for parabolic--cylindrical solar
collectors. Sharing the Sun, Proceedings of American Section of ISES and
Solar Energy Society of Canada, 1976, Vol. 2, pp. 235-52.
[8]. Guven, H. M. & Bannerot, R. B., Determination of error tolerances for the
optical design of parabolic troughs for developing countries. Solar Energy,
36(6) (1986) 535-50.
[9]. Guven, H. M. & Bannerot, R. B., Derivation of universal error parameters
for comprehensive optical analysis of parabolic troughs. Proceedings of the
ASME-ASESSolar Energy Conference, Knoxville, 1985, pp. 168-74.
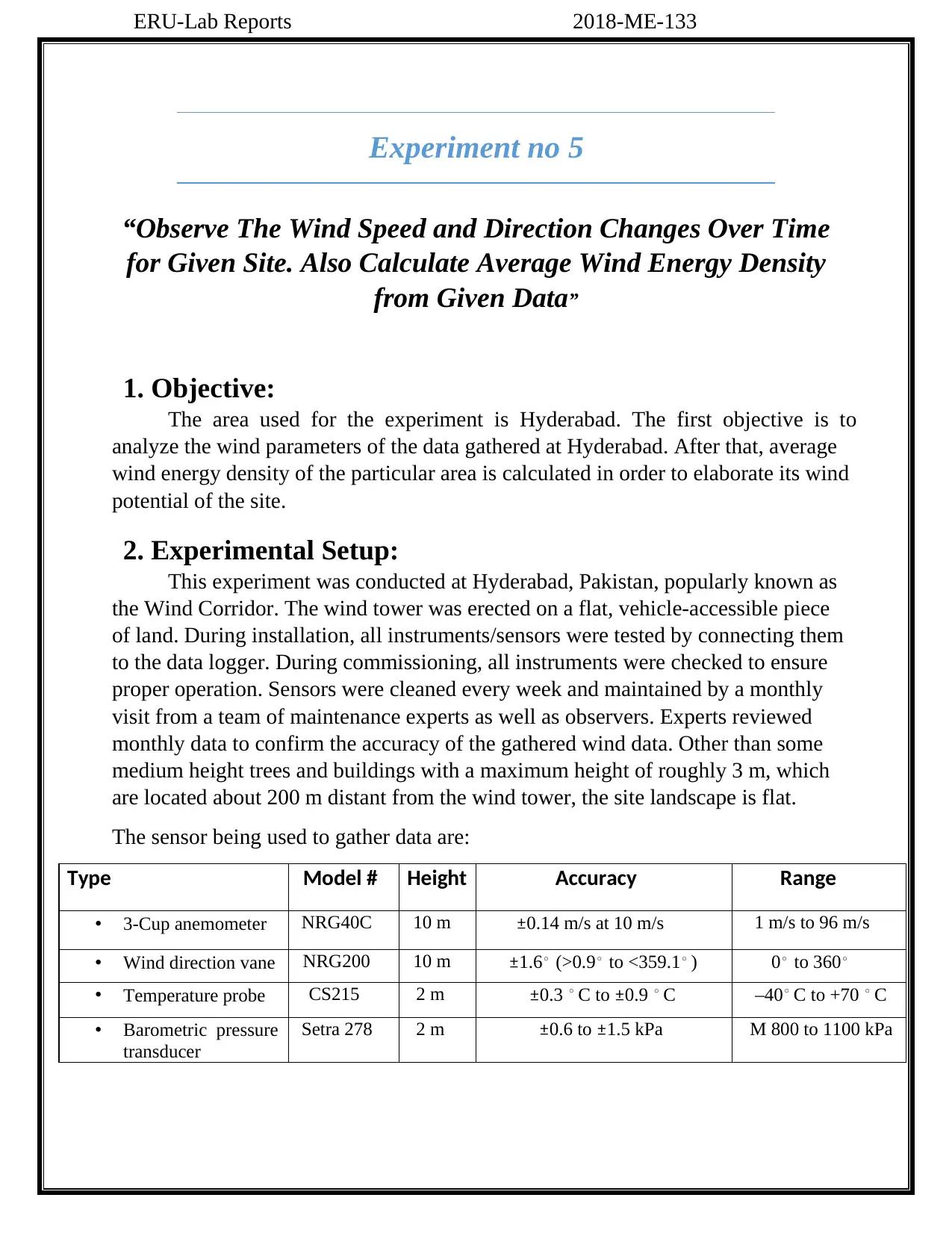
ERU-Lab Reports 2018-ME-133
Experiment no 5
“Observe The Wind Speed and Direction Changes Over Time
for Given Site. Also Calculate Average Wind Energy Density
from Given Data”
1. Objective:
The area used for the experiment is Hyderabad. The first objective is to
analyze the wind parameters of the data gathered at Hyderabad. After that, average
wind energy density of the particular area is calculated in order to elaborate its wind
potential of the site.
2. Experimental Setup:
This experiment was conducted at Hyderabad, Pakistan, popularly known as
the Wind Corridor. The wind tower was erected on a flat, vehicle-accessible piece
of land. During installation, all instruments/sensors were tested by connecting them
to the data logger. During commissioning, all instruments were checked to ensure
proper operation. Sensors were cleaned every week and maintained by a monthly
visit from a team of maintenance experts as well as observers. Experts reviewed
monthly data to confirm the accuracy of the gathered wind data. Other than some
medium height trees and buildings with a maximum height of roughly 3 m, which
are located about 200 m distant from the wind tower, the site landscape is flat.
The sensor being used to gather data are:
Type Model # Height Accuracy Range
• 3-Cup anemometer NRG40C 10 m ±0.14 m/s at 10 m/s 1 m/s to 96 m/s
• Wind direction vane NRG200 10 m ±1.6◦ (>0.9◦ to <359.1◦ ) 0◦ to 360◦
• Temperature probe CS215 2 m ±0.3 ◦ C to ±0.9 ◦ C –40◦ C to +70 ◦ C
• Barometric pressure
transducer
Setra 278 2 m ±0.6 to ±1.5 kPa M 800 to 1100 kPa
Experiment no 5
“Observe The Wind Speed and Direction Changes Over Time
for Given Site. Also Calculate Average Wind Energy Density
from Given Data”
1. Objective:
The area used for the experiment is Hyderabad. The first objective is to
analyze the wind parameters of the data gathered at Hyderabad. After that, average
wind energy density of the particular area is calculated in order to elaborate its wind
potential of the site.
2. Experimental Setup:
This experiment was conducted at Hyderabad, Pakistan, popularly known as
the Wind Corridor. The wind tower was erected on a flat, vehicle-accessible piece
of land. During installation, all instruments/sensors were tested by connecting them
to the data logger. During commissioning, all instruments were checked to ensure
proper operation. Sensors were cleaned every week and maintained by a monthly
visit from a team of maintenance experts as well as observers. Experts reviewed
monthly data to confirm the accuracy of the gathered wind data. Other than some
medium height trees and buildings with a maximum height of roughly 3 m, which
are located about 200 m distant from the wind tower, the site landscape is flat.
The sensor being used to gather data are:
Type Model # Height Accuracy Range
• 3-Cup anemometer NRG40C 10 m ±0.14 m/s at 10 m/s 1 m/s to 96 m/s
• Wind direction vane NRG200 10 m ±1.6◦ (>0.9◦ to <359.1◦ ) 0◦ to 360◦
• Temperature probe CS215 2 m ±0.3 ◦ C to ±0.9 ◦ C –40◦ C to +70 ◦ C
• Barometric pressure
transducer
Setra 278 2 m ±0.6 to ±1.5 kPa M 800 to 1100 kPa
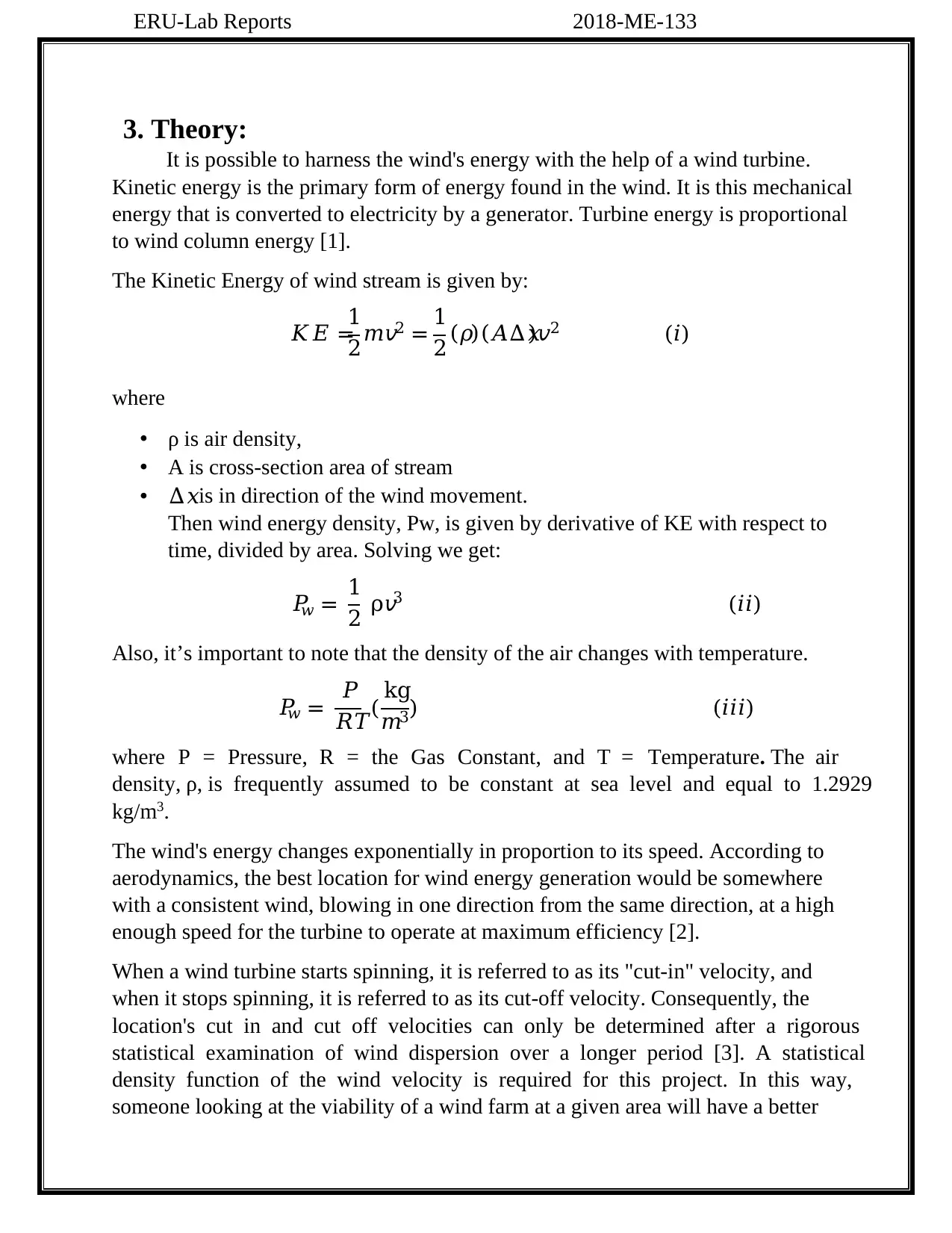
ERU-Lab Reports 2018-ME-133
3. Theory:
It is possible to harness the wind's energy with the help of a wind turbine.
Kinetic energy is the primary form of energy found in the wind. It is this mechanical
energy that is converted to electricity by a generator. Turbine energy is proportional
to wind column energy [1].
The Kinetic Energy of wind stream is given by:
𝐾𝐸 =
1
2𝑚𝑣2 = 1
2(𝜌)(𝐴∆𝑥)𝑣2 (𝑖)
where
• ρ is air density,
• A is cross-section area of stream
• ∆𝑥is in direction of the wind movement.
Then wind energy density, Pw, is given by derivative of KE with respect to
time, divided by area. Solving we get:
𝑃𝑤 = 1
2 ρ𝑣3 (𝑖𝑖)
Also, it’s important to note that the density of the air changes with temperature.
𝑃𝑤 = 𝑃
𝑅𝑇( kg
𝑚3) (𝑖𝑖𝑖)
where P = Pressure, R = the Gas Constant, and T = Temperature. The air
density, ρ, is frequently assumed to be constant at sea level and equal to 1.2929
kg/m3.
The wind's energy changes exponentially in proportion to its speed. According to
aerodynamics, the best location for wind energy generation would be somewhere
with a consistent wind, blowing in one direction from the same direction, at a high
enough speed for the turbine to operate at maximum efficiency [2].
When a wind turbine starts spinning, it is referred to as its "cut-in" velocity, and
when it stops spinning, it is referred to as its cut-off velocity. Consequently, the
location's cut in and cut off velocities can only be determined after a rigorous
statistical examination of wind dispersion over a longer period [3]. A statistical
density function of the wind velocity is required for this project. In this way,
someone looking at the viability of a wind farm at a given area will have a better
3. Theory:
It is possible to harness the wind's energy with the help of a wind turbine.
Kinetic energy is the primary form of energy found in the wind. It is this mechanical
energy that is converted to electricity by a generator. Turbine energy is proportional
to wind column energy [1].
The Kinetic Energy of wind stream is given by:
𝐾𝐸 =
1
2𝑚𝑣2 = 1
2(𝜌)(𝐴∆𝑥)𝑣2 (𝑖)
where
• ρ is air density,
• A is cross-section area of stream
• ∆𝑥is in direction of the wind movement.
Then wind energy density, Pw, is given by derivative of KE with respect to
time, divided by area. Solving we get:
𝑃𝑤 = 1
2 ρ𝑣3 (𝑖𝑖)
Also, it’s important to note that the density of the air changes with temperature.
𝑃𝑤 = 𝑃
𝑅𝑇( kg
𝑚3) (𝑖𝑖𝑖)
where P = Pressure, R = the Gas Constant, and T = Temperature. The air
density, ρ, is frequently assumed to be constant at sea level and equal to 1.2929
kg/m3.
The wind's energy changes exponentially in proportion to its speed. According to
aerodynamics, the best location for wind energy generation would be somewhere
with a consistent wind, blowing in one direction from the same direction, at a high
enough speed for the turbine to operate at maximum efficiency [2].
When a wind turbine starts spinning, it is referred to as its "cut-in" velocity, and
when it stops spinning, it is referred to as its cut-off velocity. Consequently, the
location's cut in and cut off velocities can only be determined after a rigorous
statistical examination of wind dispersion over a longer period [3]. A statistical
density function of the wind velocity is required for this project. In this way,
someone looking at the viability of a wind farm at a given area will have a better
Paraphrase This Document
Need a fresh take? Get an instant paraphrase of this document with our AI Paraphraser
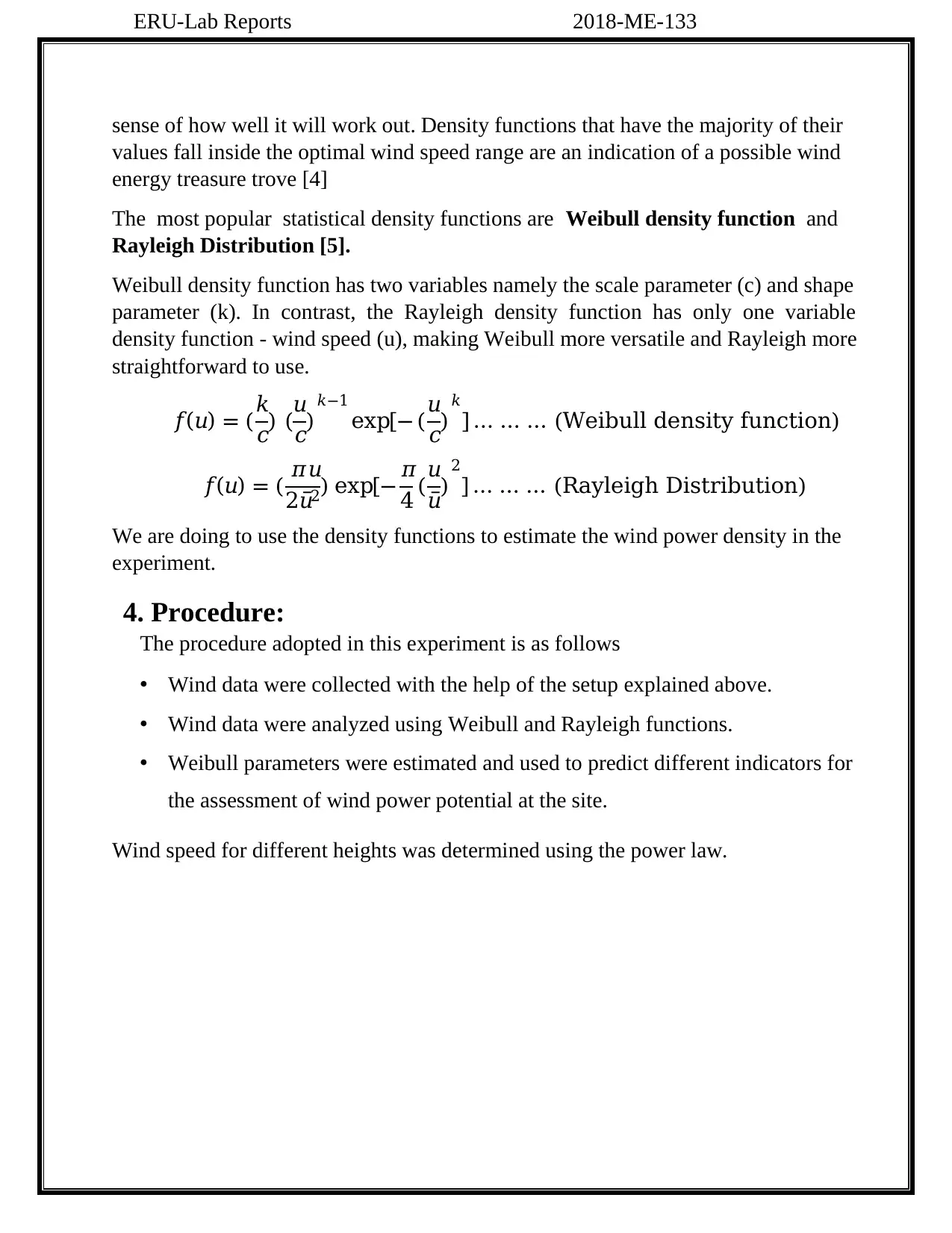
ERU-Lab Reports 2018-ME-133
sense of how well it will work out. Density functions that have the majority of their
values fall inside the optimal wind speed range are an indication of a possible wind
energy treasure trove [4]
The most popular statistical density functions are Weibull density function and
Rayleigh Distribution [5].
Weibull density function has two variables namely the scale parameter (c) and shape
parameter (k). In contrast, the Rayleigh density function has only one variable
density function - wind speed (u), making Weibull more versatile and Rayleigh more
straightforward to use.
𝑓(𝑢) = (𝑘
𝑐) (𝑢
𝑐)
𝑘−1
exp[− (𝑢
𝑐)
𝑘
] … … … (Weibull density function)
𝑓(𝑢) = ( 𝜋𝑢
2𝑢̅2) exp[− 𝜋
4 (𝑢
𝑢̅)
2
] … … … (Rayleigh Distribution)
We are doing to use the density functions to estimate the wind power density in the
experiment.
4. Procedure:
The procedure adopted in this experiment is as follows
• Wind data were collected with the help of the setup explained above.
• Wind data were analyzed using Weibull and Rayleigh functions.
• Weibull parameters were estimated and used to predict different indicators for
the assessment of wind power potential at the site.
Wind speed for different heights was determined using the power law.
sense of how well it will work out. Density functions that have the majority of their
values fall inside the optimal wind speed range are an indication of a possible wind
energy treasure trove [4]
The most popular statistical density functions are Weibull density function and
Rayleigh Distribution [5].
Weibull density function has two variables namely the scale parameter (c) and shape
parameter (k). In contrast, the Rayleigh density function has only one variable
density function - wind speed (u), making Weibull more versatile and Rayleigh more
straightforward to use.
𝑓(𝑢) = (𝑘
𝑐) (𝑢
𝑐)
𝑘−1
exp[− (𝑢
𝑐)
𝑘
] … … … (Weibull density function)
𝑓(𝑢) = ( 𝜋𝑢
2𝑢̅2) exp[− 𝜋
4 (𝑢
𝑢̅)
2
] … … … (Rayleigh Distribution)
We are doing to use the density functions to estimate the wind power density in the
experiment.
4. Procedure:
The procedure adopted in this experiment is as follows
• Wind data were collected with the help of the setup explained above.
• Wind data were analyzed using Weibull and Rayleigh functions.
• Weibull parameters were estimated and used to predict different indicators for
the assessment of wind power potential at the site.
Wind speed for different heights was determined using the power law.
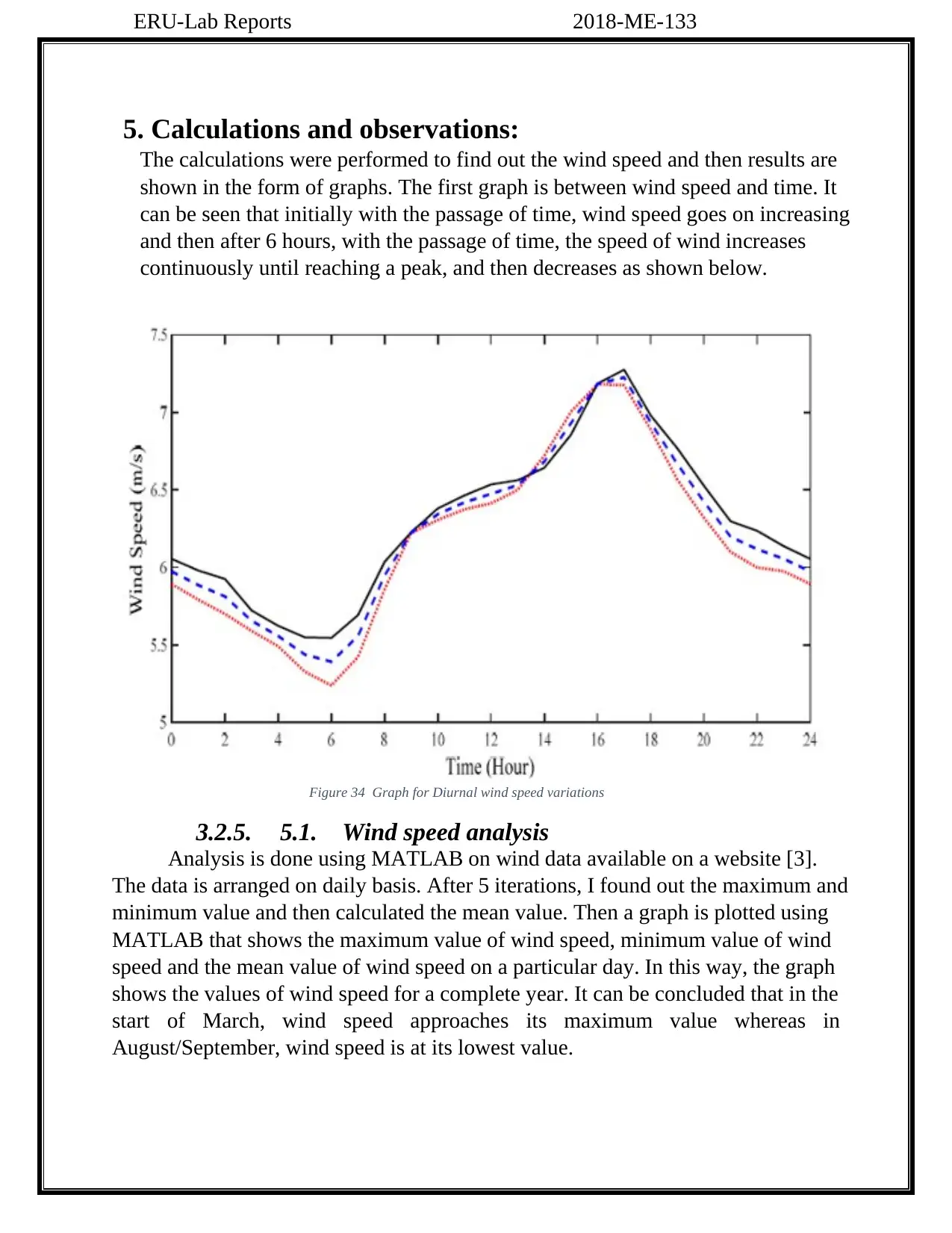
ERU-Lab Reports 2018-ME-133
5. Calculations and observations:
The calculations were performed to find out the wind speed and then results are
shown in the form of graphs. The first graph is between wind speed and time. It
can be seen that initially with the passage of time, wind speed goes on increasing
and then after 6 hours, with the passage of time, the speed of wind increases
continuously until reaching a peak, and then decreases as shown below.
3.2.5. 5.1. Wind speed analysis
Analysis is done using MATLAB on wind data available on a website [3].
The data is arranged on daily basis. After 5 iterations, I found out the maximum and
minimum value and then calculated the mean value. Then a graph is plotted using
MATLAB that shows the maximum value of wind speed, minimum value of wind
speed and the mean value of wind speed on a particular day. In this way, the graph
shows the values of wind speed for a complete year. It can be concluded that in the
start of March, wind speed approaches its maximum value whereas in
August/September, wind speed is at its lowest value.
Figure 34 Graph for Diurnal wind speed variations
5. Calculations and observations:
The calculations were performed to find out the wind speed and then results are
shown in the form of graphs. The first graph is between wind speed and time. It
can be seen that initially with the passage of time, wind speed goes on increasing
and then after 6 hours, with the passage of time, the speed of wind increases
continuously until reaching a peak, and then decreases as shown below.
3.2.5. 5.1. Wind speed analysis
Analysis is done using MATLAB on wind data available on a website [3].
The data is arranged on daily basis. After 5 iterations, I found out the maximum and
minimum value and then calculated the mean value. Then a graph is plotted using
MATLAB that shows the maximum value of wind speed, minimum value of wind
speed and the mean value of wind speed on a particular day. In this way, the graph
shows the values of wind speed for a complete year. It can be concluded that in the
start of March, wind speed approaches its maximum value whereas in
August/September, wind speed is at its lowest value.
Figure 34 Graph for Diurnal wind speed variations
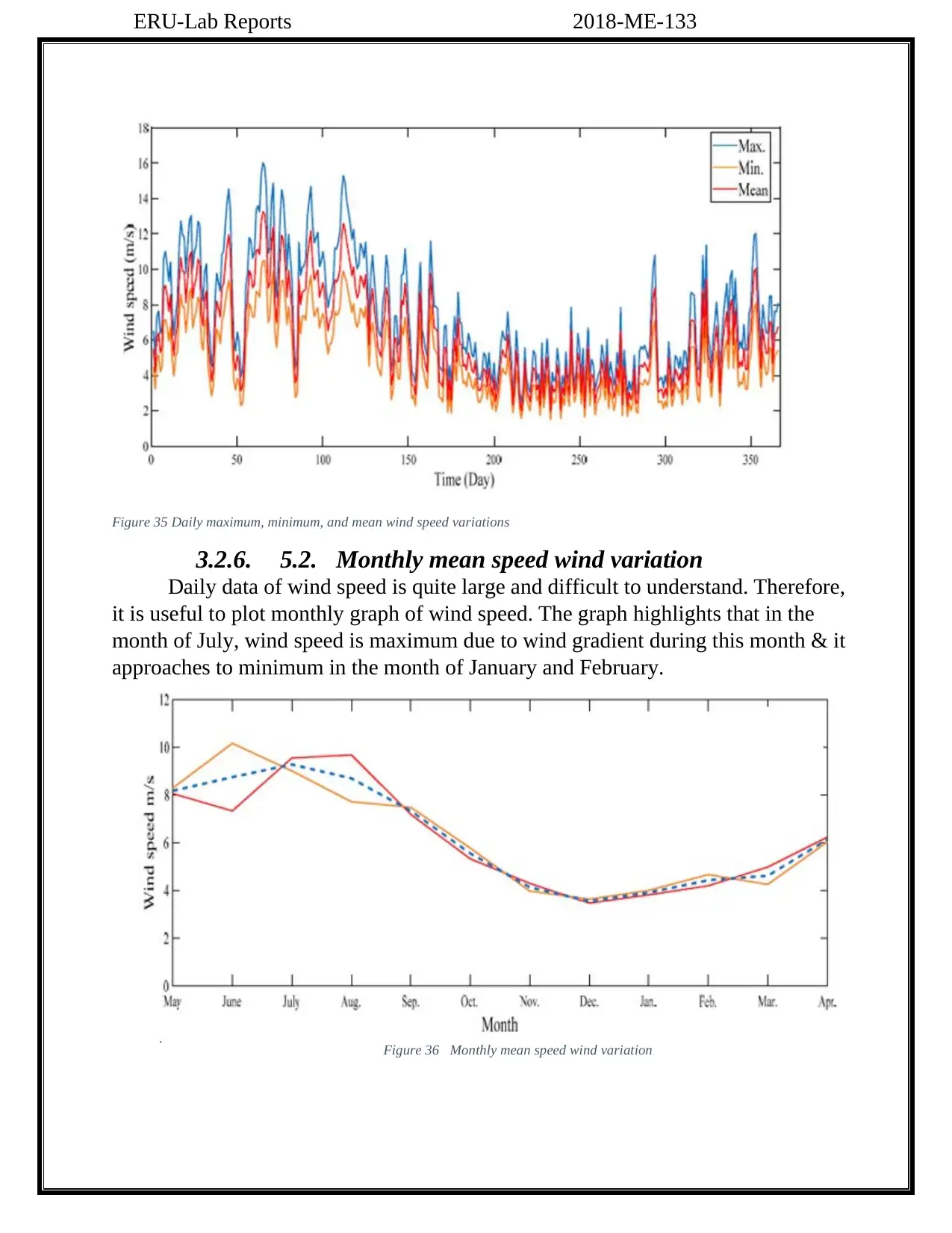
ERU-Lab Reports 2018-ME-133
Figure 35 Daily maximum, minimum, and mean wind speed variations
3.2.6. 5.2. Monthly mean speed wind variation
Daily data of wind speed is quite large and difficult to understand. Therefore,
it is useful to plot monthly graph of wind speed. The graph highlights that in the
month of July, wind speed is maximum due to wind gradient during this month & it
approaches to minimum in the month of January and February.
Figure 36 Monthly mean speed wind variation
Figure 35 Daily maximum, minimum, and mean wind speed variations
3.2.6. 5.2. Monthly mean speed wind variation
Daily data of wind speed is quite large and difficult to understand. Therefore,
it is useful to plot monthly graph of wind speed. The graph highlights that in the
month of July, wind speed is maximum due to wind gradient during this month & it
approaches to minimum in the month of January and February.
Figure 36 Monthly mean speed wind variation
Secure Best Marks with AI Grader
Need help grading? Try our AI Grader for instant feedback on your assignments.
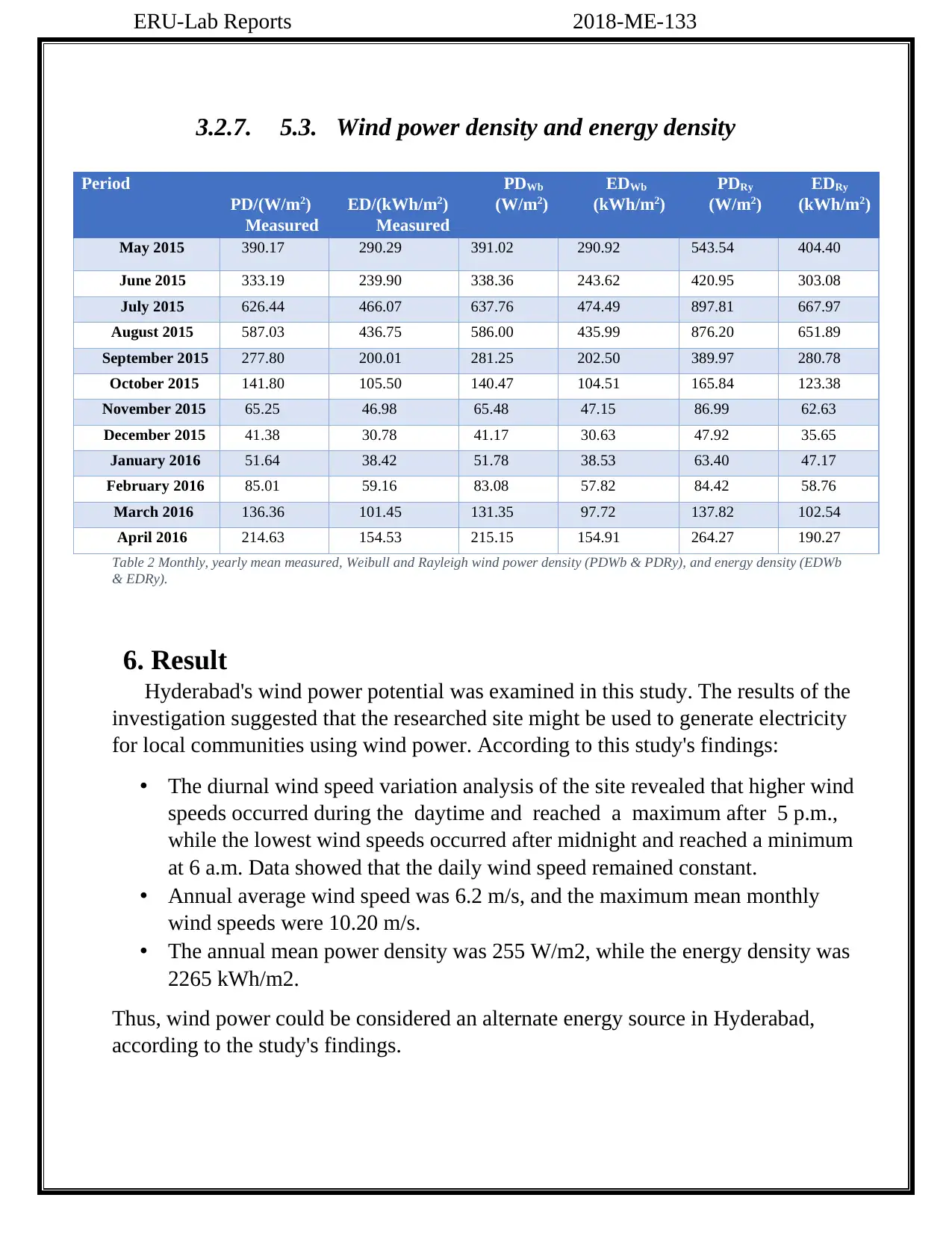
ERU-Lab Reports 2018-ME-133
3.2.7. 5.3. Wind power density and energy density
Table 2 Monthly, yearly mean measured, Weibull and Rayleigh wind power density (PDWb & PDRy), and energy density (EDWb
& EDRy).
6. Result
Hyderabad's wind power potential was examined in this study. The results of the
investigation suggested that the researched site might be used to generate electricity
for local communities using wind power. According to this study's findings:
• The diurnal wind speed variation analysis of the site revealed that higher wind
speeds occurred during the daytime and reached a maximum after 5 p.m.,
while the lowest wind speeds occurred after midnight and reached a minimum
at 6 a.m. Data showed that the daily wind speed remained constant.
• Annual average wind speed was 6.2 m/s, and the maximum mean monthly
wind speeds were 10.20 m/s.
• The annual mean power density was 255 W/m2, while the energy density was
2265 kWh/m2.
Thus, wind power could be considered an alternate energy source in Hyderabad,
according to the study's findings.
Period
PD/(W/m2)
Measured
ED/(kWh/m2)
Measured
PDWb
(W/m2)
EDWb
(kWh/m2)
PDRy
(W/m2)
EDRy
(kWh/m2)
May 2015 390.17 290.29 391.02 290.92 543.54 404.40
June 2015 333.19 239.90 338.36 243.62 420.95 303.08
July 2015 626.44 466.07 637.76 474.49 897.81 667.97
August 2015 587.03 436.75 586.00 435.99 876.20 651.89
September 2015 277.80 200.01 281.25 202.50 389.97 280.78
October 2015 141.80 105.50 140.47 104.51 165.84 123.38
November 2015 65.25 46.98 65.48 47.15 86.99 62.63
December 2015 41.38 30.78 41.17 30.63 47.92 35.65
January 2016 51.64 38.42 51.78 38.53 63.40 47.17
February 2016 85.01 59.16 83.08 57.82 84.42 58.76
March 2016 136.36 101.45 131.35 97.72 137.82 102.54
April 2016 214.63 154.53 215.15 154.91 264.27 190.27
3.2.7. 5.3. Wind power density and energy density
Table 2 Monthly, yearly mean measured, Weibull and Rayleigh wind power density (PDWb & PDRy), and energy density (EDWb
& EDRy).
6. Result
Hyderabad's wind power potential was examined in this study. The results of the
investigation suggested that the researched site might be used to generate electricity
for local communities using wind power. According to this study's findings:
• The diurnal wind speed variation analysis of the site revealed that higher wind
speeds occurred during the daytime and reached a maximum after 5 p.m.,
while the lowest wind speeds occurred after midnight and reached a minimum
at 6 a.m. Data showed that the daily wind speed remained constant.
• Annual average wind speed was 6.2 m/s, and the maximum mean monthly
wind speeds were 10.20 m/s.
• The annual mean power density was 255 W/m2, while the energy density was
2265 kWh/m2.
Thus, wind power could be considered an alternate energy source in Hyderabad,
according to the study's findings.
Period
PD/(W/m2)
Measured
ED/(kWh/m2)
Measured
PDWb
(W/m2)
EDWb
(kWh/m2)
PDRy
(W/m2)
EDRy
(kWh/m2)
May 2015 390.17 290.29 391.02 290.92 543.54 404.40
June 2015 333.19 239.90 338.36 243.62 420.95 303.08
July 2015 626.44 466.07 637.76 474.49 897.81 667.97
August 2015 587.03 436.75 586.00 435.99 876.20 651.89
September 2015 277.80 200.01 281.25 202.50 389.97 280.78
October 2015 141.80 105.50 140.47 104.51 165.84 123.38
November 2015 65.25 46.98 65.48 47.15 86.99 62.63
December 2015 41.38 30.78 41.17 30.63 47.92 35.65
January 2016 51.64 38.42 51.78 38.53 63.40 47.17
February 2016 85.01 59.16 83.08 57.82 84.42 58.76
March 2016 136.36 101.45 131.35 97.72 137.82 102.54
April 2016 214.63 154.53 215.15 154.91 264.27 190.27
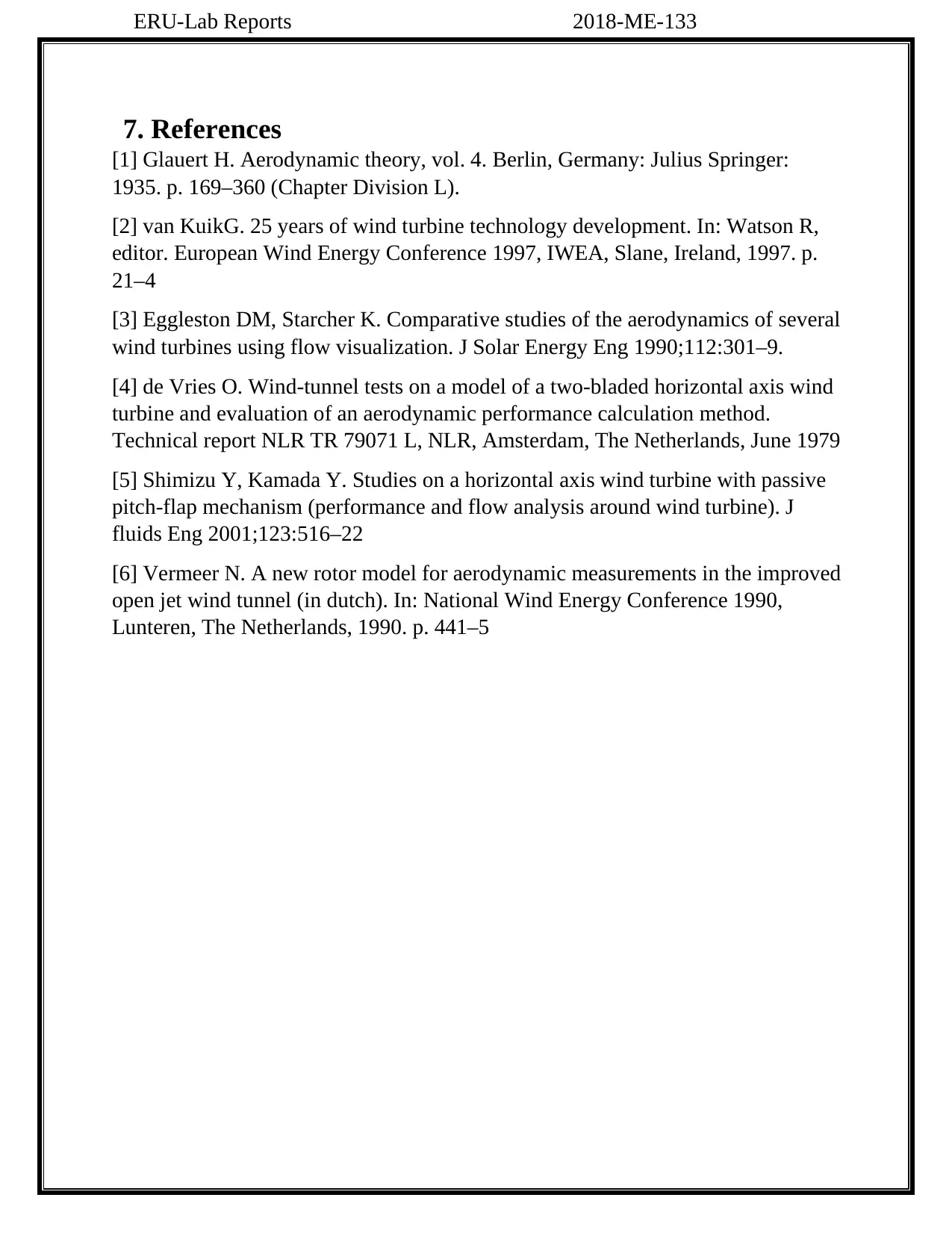
ERU-Lab Reports 2018-ME-133
7. References
[1] Glauert H. Aerodynamic theory, vol. 4. Berlin, Germany: Julius Springer:
1935. p. 169–360 (Chapter Division L).
[2] van KuikG. 25 years of wind turbine technology development. In: Watson R,
editor. European Wind Energy Conference 1997, IWEA, Slane, Ireland, 1997. p.
21–4
[3] Eggleston DM, Starcher K. Comparative studies of the aerodynamics of several
wind turbines using flow visualization. J Solar Energy Eng 1990;112:301–9.
[4] de Vries O. Wind-tunnel tests on a model of a two-bladed horizontal axis wind
turbine and evaluation of an aerodynamic performance calculation method.
Technical report NLR TR 79071 L, NLR, Amsterdam, The Netherlands, June 1979
[5] Shimizu Y, Kamada Y. Studies on a horizontal axis wind turbine with passive
pitch-flap mechanism (performance and flow analysis around wind turbine). J
fluids Eng 2001;123:516–22
[6] Vermeer N. A new rotor model for aerodynamic measurements in the improved
open jet wind tunnel (in dutch). In: National Wind Energy Conference 1990,
Lunteren, The Netherlands, 1990. p. 441–5
7. References
[1] Glauert H. Aerodynamic theory, vol. 4. Berlin, Germany: Julius Springer:
1935. p. 169–360 (Chapter Division L).
[2] van KuikG. 25 years of wind turbine technology development. In: Watson R,
editor. European Wind Energy Conference 1997, IWEA, Slane, Ireland, 1997. p.
21–4
[3] Eggleston DM, Starcher K. Comparative studies of the aerodynamics of several
wind turbines using flow visualization. J Solar Energy Eng 1990;112:301–9.
[4] de Vries O. Wind-tunnel tests on a model of a two-bladed horizontal axis wind
turbine and evaluation of an aerodynamic performance calculation method.
Technical report NLR TR 79071 L, NLR, Amsterdam, The Netherlands, June 1979
[5] Shimizu Y, Kamada Y. Studies on a horizontal axis wind turbine with passive
pitch-flap mechanism (performance and flow analysis around wind turbine). J
fluids Eng 2001;123:516–22
[6] Vermeer N. A new rotor model for aerodynamic measurements in the improved
open jet wind tunnel (in dutch). In: National Wind Energy Conference 1990,
Lunteren, The Netherlands, 1990. p. 441–5
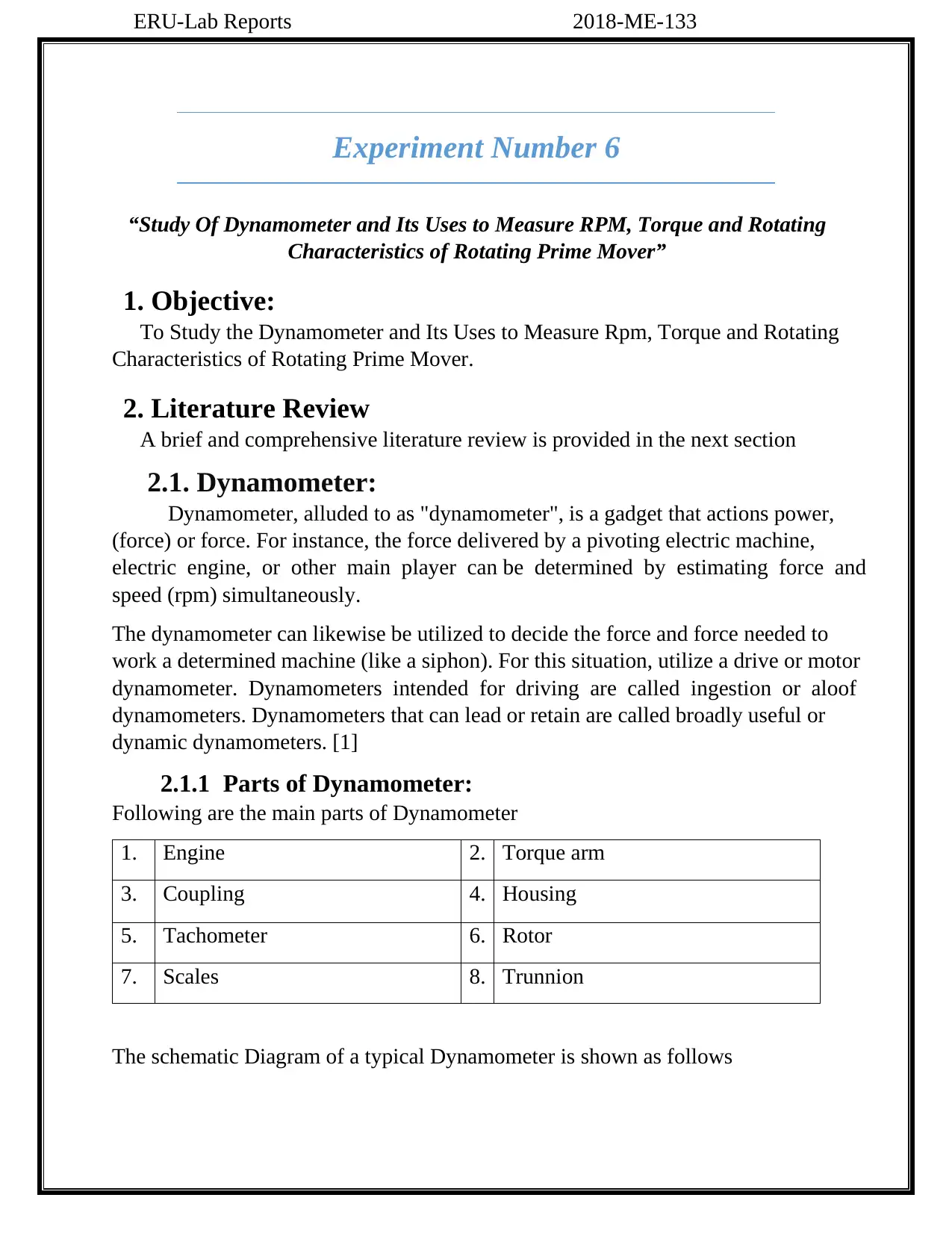
ERU-Lab Reports 2018-ME-133
Experiment Number 6
“Study Of Dynamometer and Its Uses to Measure RPM, Torque and Rotating
Characteristics of Rotating Prime Mover”
1. Objective:
To Study the Dynamometer and Its Uses to Measure Rpm, Torque and Rotating
Characteristics of Rotating Prime Mover.
2. Literature Review
A brief and comprehensive literature review is provided in the next section
2.1. Dynamometer:
Dynamometer, alluded to as "dynamometer", is a gadget that actions power,
(force) or force. For instance, the force delivered by a pivoting electric machine,
electric engine, or other main player can be determined by estimating force and
speed (rpm) simultaneously.
The dynamometer can likewise be utilized to decide the force and force needed to
work a determined machine (like a siphon). For this situation, utilize a drive or motor
dynamometer. Dynamometers intended for driving are called ingestion or aloof
dynamometers. Dynamometers that can lead or retain are called broadly useful or
dynamic dynamometers. [1]
2.1.1 Parts of Dynamometer:
Following are the main parts of Dynamometer
1. Engine 2. Torque arm
3. Coupling 4. Housing
5. Tachometer 6. Rotor
7. Scales 8. Trunnion
The schematic Diagram of a typical Dynamometer is shown as follows
Experiment Number 6
“Study Of Dynamometer and Its Uses to Measure RPM, Torque and Rotating
Characteristics of Rotating Prime Mover”
1. Objective:
To Study the Dynamometer and Its Uses to Measure Rpm, Torque and Rotating
Characteristics of Rotating Prime Mover.
2. Literature Review
A brief and comprehensive literature review is provided in the next section
2.1. Dynamometer:
Dynamometer, alluded to as "dynamometer", is a gadget that actions power,
(force) or force. For instance, the force delivered by a pivoting electric machine,
electric engine, or other main player can be determined by estimating force and
speed (rpm) simultaneously.
The dynamometer can likewise be utilized to decide the force and force needed to
work a determined machine (like a siphon). For this situation, utilize a drive or motor
dynamometer. Dynamometers intended for driving are called ingestion or aloof
dynamometers. Dynamometers that can lead or retain are called broadly useful or
dynamic dynamometers. [1]
2.1.1 Parts of Dynamometer:
Following are the main parts of Dynamometer
1. Engine 2. Torque arm
3. Coupling 4. Housing
5. Tachometer 6. Rotor
7. Scales 8. Trunnion
The schematic Diagram of a typical Dynamometer is shown as follows
Paraphrase This Document
Need a fresh take? Get an instant paraphrase of this document with our AI Paraphraser
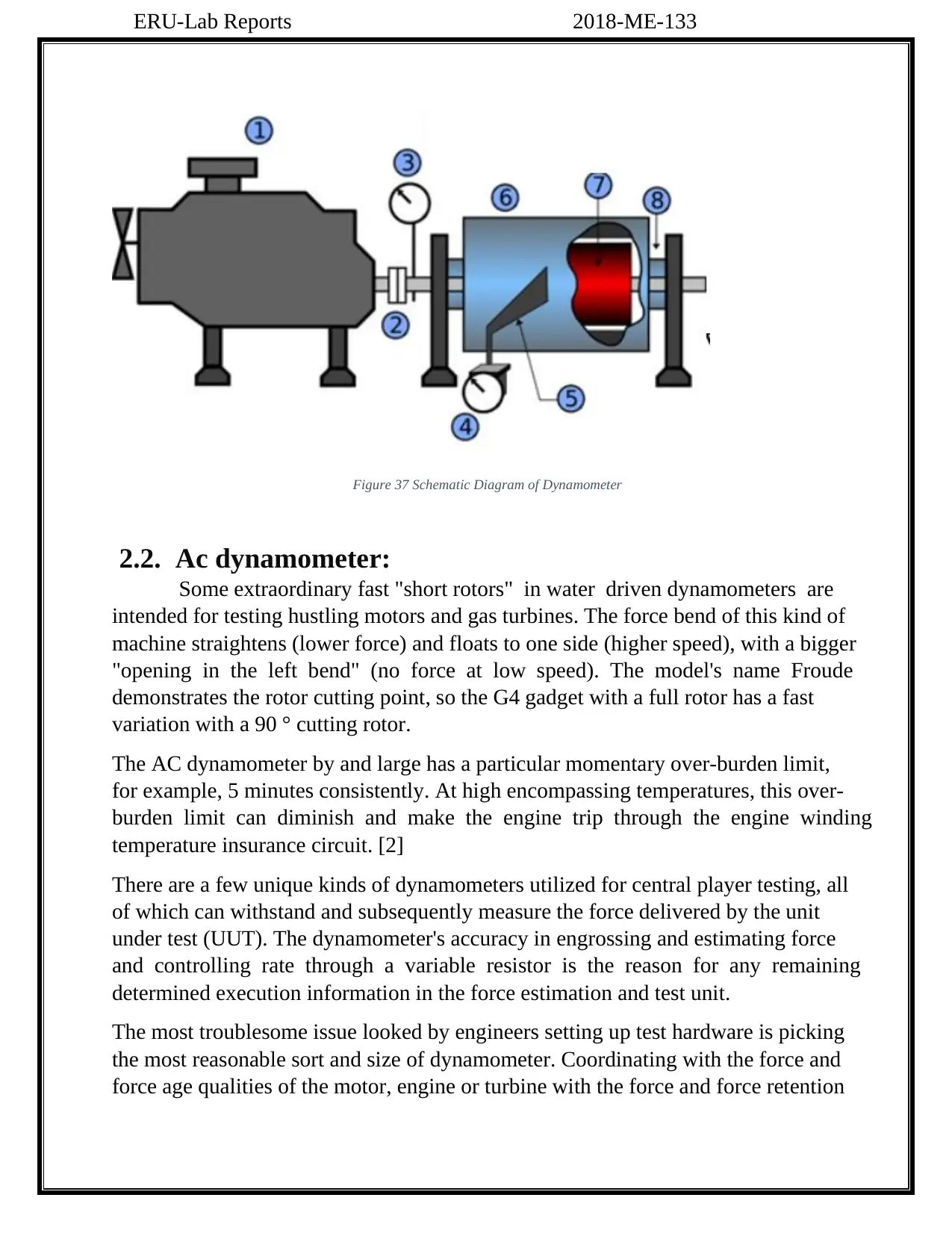
ERU-Lab Reports 2018-ME-133
2.2. Ac dynamometer:
Some extraordinary fast "short rotors" in water driven dynamometers are
intended for testing hustling motors and gas turbines. The force bend of this kind of
machine straightens (lower force) and floats to one side (higher speed), with a bigger
"opening in the left bend" (no force at low speed). The model's name Froude
demonstrates the rotor cutting point, so the G4 gadget with a full rotor has a fast
variation with a 90 ° cutting rotor.
The AC dynamometer by and large has a particular momentary over-burden limit,
for example, 5 minutes consistently. At high encompassing temperatures, this over-
burden limit can diminish and make the engine trip through the engine winding
temperature insurance circuit. [2]
There are a few unique kinds of dynamometers utilized for central player testing, all
of which can withstand and subsequently measure the force delivered by the unit
under test (UUT). The dynamometer's accuracy in engrossing and estimating force
and controlling rate through a variable resistor is the reason for any remaining
determined execution information in the force estimation and test unit.
The most troublesome issue looked by engineers setting up test hardware is picking
the most reasonable sort and size of dynamometer. Coordinating with the force and
force age qualities of the motor, engine or turbine with the force and force retention
Figure 37 Schematic Diagram of Dynamometer
2.2. Ac dynamometer:
Some extraordinary fast "short rotors" in water driven dynamometers are
intended for testing hustling motors and gas turbines. The force bend of this kind of
machine straightens (lower force) and floats to one side (higher speed), with a bigger
"opening in the left bend" (no force at low speed). The model's name Froude
demonstrates the rotor cutting point, so the G4 gadget with a full rotor has a fast
variation with a 90 ° cutting rotor.
The AC dynamometer by and large has a particular momentary over-burden limit,
for example, 5 minutes consistently. At high encompassing temperatures, this over-
burden limit can diminish and make the engine trip through the engine winding
temperature insurance circuit. [2]
There are a few unique kinds of dynamometers utilized for central player testing, all
of which can withstand and subsequently measure the force delivered by the unit
under test (UUT). The dynamometer's accuracy in engrossing and estimating force
and controlling rate through a variable resistor is the reason for any remaining
determined execution information in the force estimation and test unit.
The most troublesome issue looked by engineers setting up test hardware is picking
the most reasonable sort and size of dynamometer. Coordinating with the force and
force age qualities of the motor, engine or turbine with the force and force retention
Figure 37 Schematic Diagram of Dynamometer
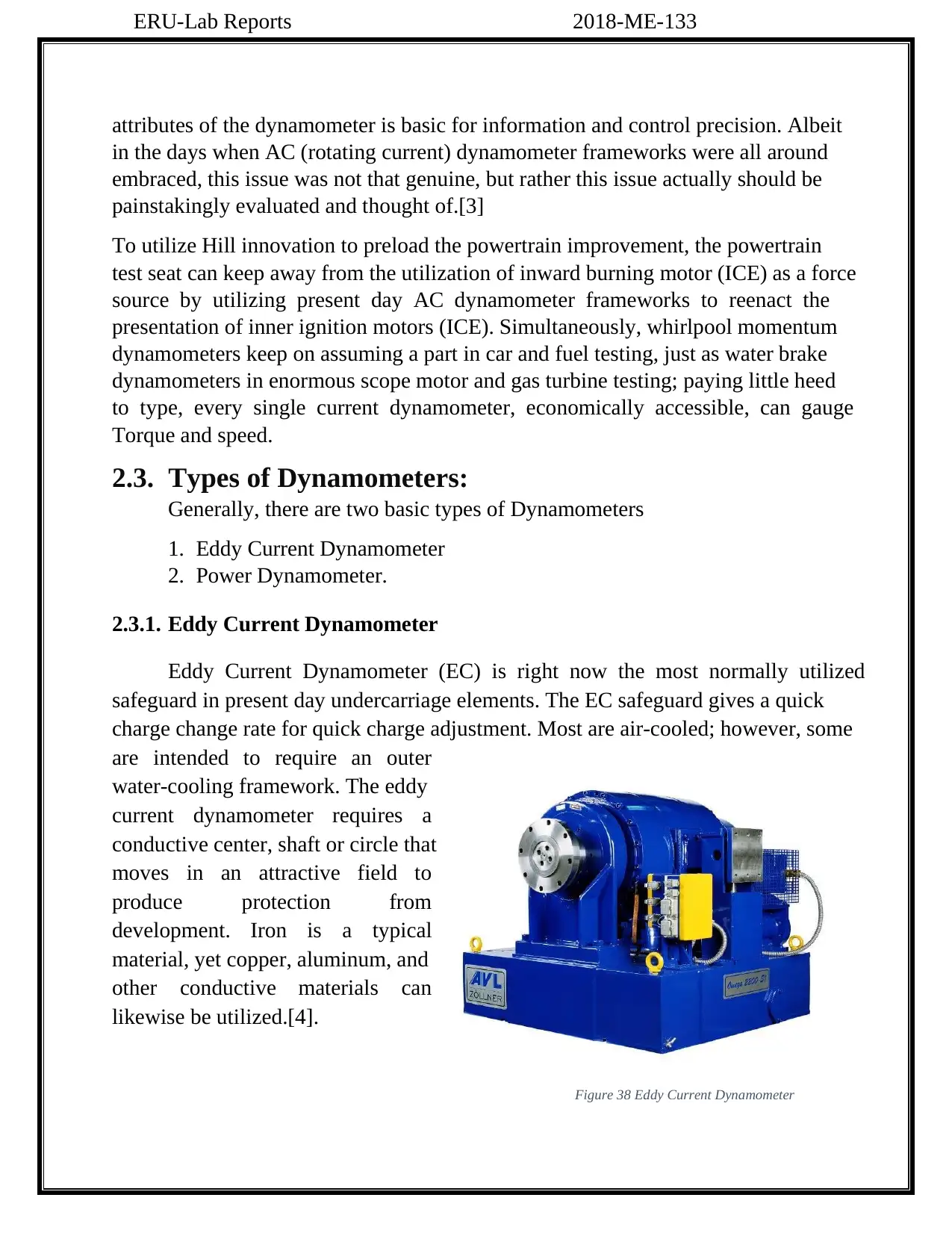
ERU-Lab Reports 2018-ME-133
attributes of the dynamometer is basic for information and control precision. Albeit
in the days when AC (rotating current) dynamometer frameworks were all around
embraced, this issue was not that genuine, but rather this issue actually should be
painstakingly evaluated and thought of.[3]
To utilize Hill innovation to preload the powertrain improvement, the powertrain
test seat can keep away from the utilization of inward burning motor (ICE) as a force
source by utilizing present day AC dynamometer frameworks to reenact the
presentation of inner ignition motors (ICE). Simultaneously, whirlpool momentum
dynamometers keep on assuming a part in car and fuel testing, just as water brake
dynamometers in enormous scope motor and gas turbine testing; paying little heed
to type, every single current dynamometer, economically accessible, can gauge
Torque and speed.
2.3. Types of Dynamometers:
Generally, there are two basic types of Dynamometers
1. Eddy Current Dynamometer
2. Power Dynamometer.
2.3.1. Eddy Current Dynamometer
Eddy Current Dynamometer (EC) is right now the most normally utilized
safeguard in present day undercarriage elements. The EC safeguard gives a quick
charge change rate for quick charge adjustment. Most are air-cooled; however, some
are intended to require an outer
water-cooling framework. The eddy
current dynamometer requires a
conductive center, shaft or circle that
moves in an attractive field to
produce protection from
development. Iron is a typical
material, yet copper, aluminum, and
other conductive materials can
likewise be utilized.[4].
Figure 38 Eddy Current Dynamometer
attributes of the dynamometer is basic for information and control precision. Albeit
in the days when AC (rotating current) dynamometer frameworks were all around
embraced, this issue was not that genuine, but rather this issue actually should be
painstakingly evaluated and thought of.[3]
To utilize Hill innovation to preload the powertrain improvement, the powertrain
test seat can keep away from the utilization of inward burning motor (ICE) as a force
source by utilizing present day AC dynamometer frameworks to reenact the
presentation of inner ignition motors (ICE). Simultaneously, whirlpool momentum
dynamometers keep on assuming a part in car and fuel testing, just as water brake
dynamometers in enormous scope motor and gas turbine testing; paying little heed
to type, every single current dynamometer, economically accessible, can gauge
Torque and speed.
2.3. Types of Dynamometers:
Generally, there are two basic types of Dynamometers
1. Eddy Current Dynamometer
2. Power Dynamometer.
2.3.1. Eddy Current Dynamometer
Eddy Current Dynamometer (EC) is right now the most normally utilized
safeguard in present day undercarriage elements. The EC safeguard gives a quick
charge change rate for quick charge adjustment. Most are air-cooled; however, some
are intended to require an outer
water-cooling framework. The eddy
current dynamometer requires a
conductive center, shaft or circle that
moves in an attractive field to
produce protection from
development. Iron is a typical
material, yet copper, aluminum, and
other conductive materials can
likewise be utilized.[4].
Figure 38 Eddy Current Dynamometer
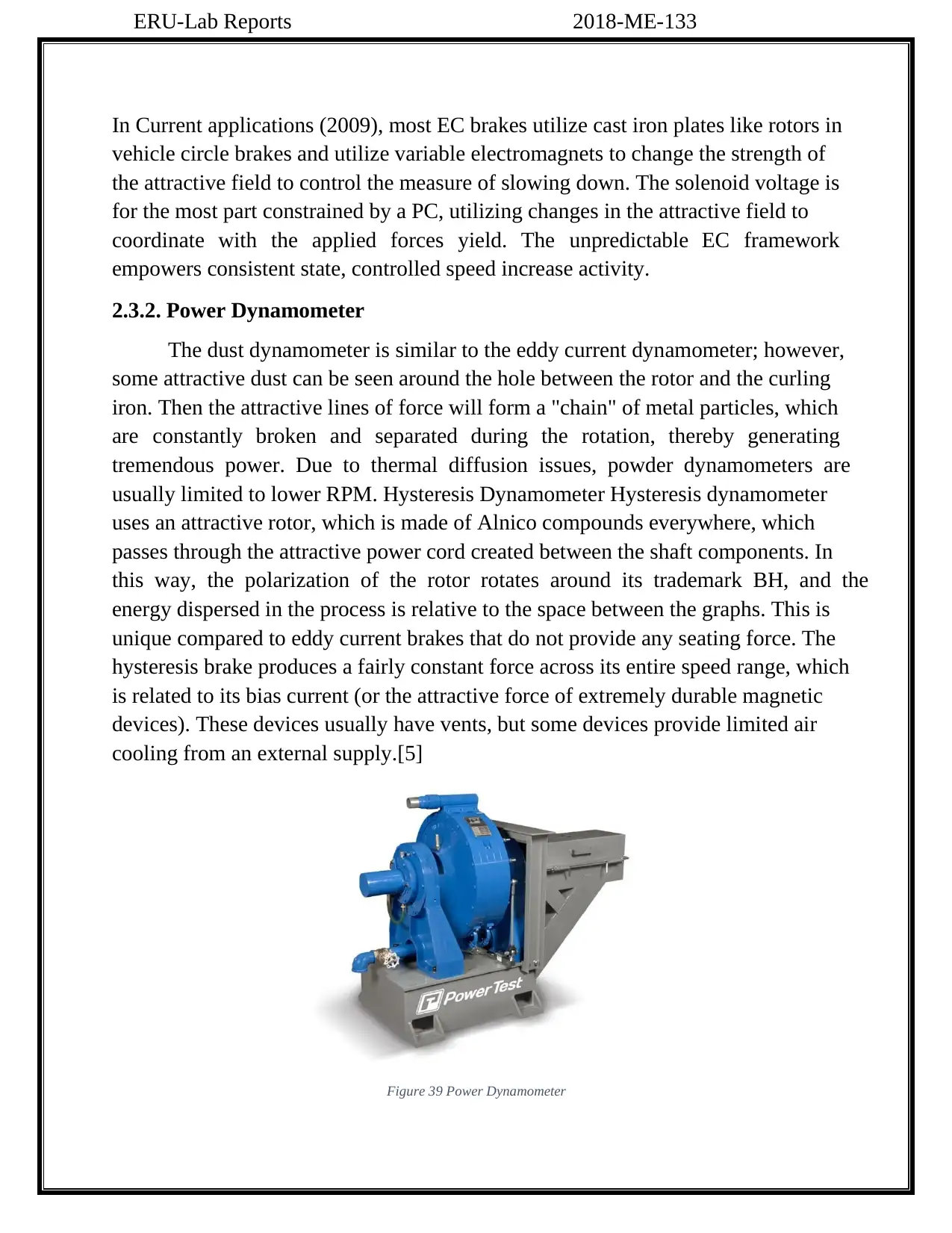
ERU-Lab Reports 2018-ME-133
In Current applications (2009), most EC brakes utilize cast iron plates like rotors in
vehicle circle brakes and utilize variable electromagnets to change the strength of
the attractive field to control the measure of slowing down. The solenoid voltage is
for the most part constrained by a PC, utilizing changes in the attractive field to
coordinate with the applied forces yield. The unpredictable EC framework
empowers consistent state, controlled speed increase activity.
2.3.2. Power Dynamometer
The dust dynamometer is similar to the eddy current dynamometer; however,
some attractive dust can be seen around the hole between the rotor and the curling
iron. Then the attractive lines of force will form a "chain" of metal particles, which
are constantly broken and separated during the rotation, thereby generating
tremendous power. Due to thermal diffusion issues, powder dynamometers are
usually limited to lower RPM. Hysteresis Dynamometer Hysteresis dynamometer
uses an attractive rotor, which is made of Alnico compounds everywhere, which
passes through the attractive power cord created between the shaft components. In
this way, the polarization of the rotor rotates around its trademark BH, and the
energy dispersed in the process is relative to the space between the graphs. This is
unique compared to eddy current brakes that do not provide any seating force. The
hysteresis brake produces a fairly constant force across its entire speed range, which
is related to its bias current (or the attractive force of extremely durable magnetic
devices). These devices usually have vents, but some devices provide limited air
cooling from an external supply.[5]
Figure 39 Power Dynamometer
In Current applications (2009), most EC brakes utilize cast iron plates like rotors in
vehicle circle brakes and utilize variable electromagnets to change the strength of
the attractive field to control the measure of slowing down. The solenoid voltage is
for the most part constrained by a PC, utilizing changes in the attractive field to
coordinate with the applied forces yield. The unpredictable EC framework
empowers consistent state, controlled speed increase activity.
2.3.2. Power Dynamometer
The dust dynamometer is similar to the eddy current dynamometer; however,
some attractive dust can be seen around the hole between the rotor and the curling
iron. Then the attractive lines of force will form a "chain" of metal particles, which
are constantly broken and separated during the rotation, thereby generating
tremendous power. Due to thermal diffusion issues, powder dynamometers are
usually limited to lower RPM. Hysteresis Dynamometer Hysteresis dynamometer
uses an attractive rotor, which is made of Alnico compounds everywhere, which
passes through the attractive power cord created between the shaft components. In
this way, the polarization of the rotor rotates around its trademark BH, and the
energy dispersed in the process is relative to the space between the graphs. This is
unique compared to eddy current brakes that do not provide any seating force. The
hysteresis brake produces a fairly constant force across its entire speed range, which
is related to its bias current (or the attractive force of extremely durable magnetic
devices). These devices usually have vents, but some devices provide limited air
cooling from an external supply.[5]
Figure 39 Power Dynamometer
Secure Best Marks with AI Grader
Need help grading? Try our AI Grader for instant feedback on your assignments.
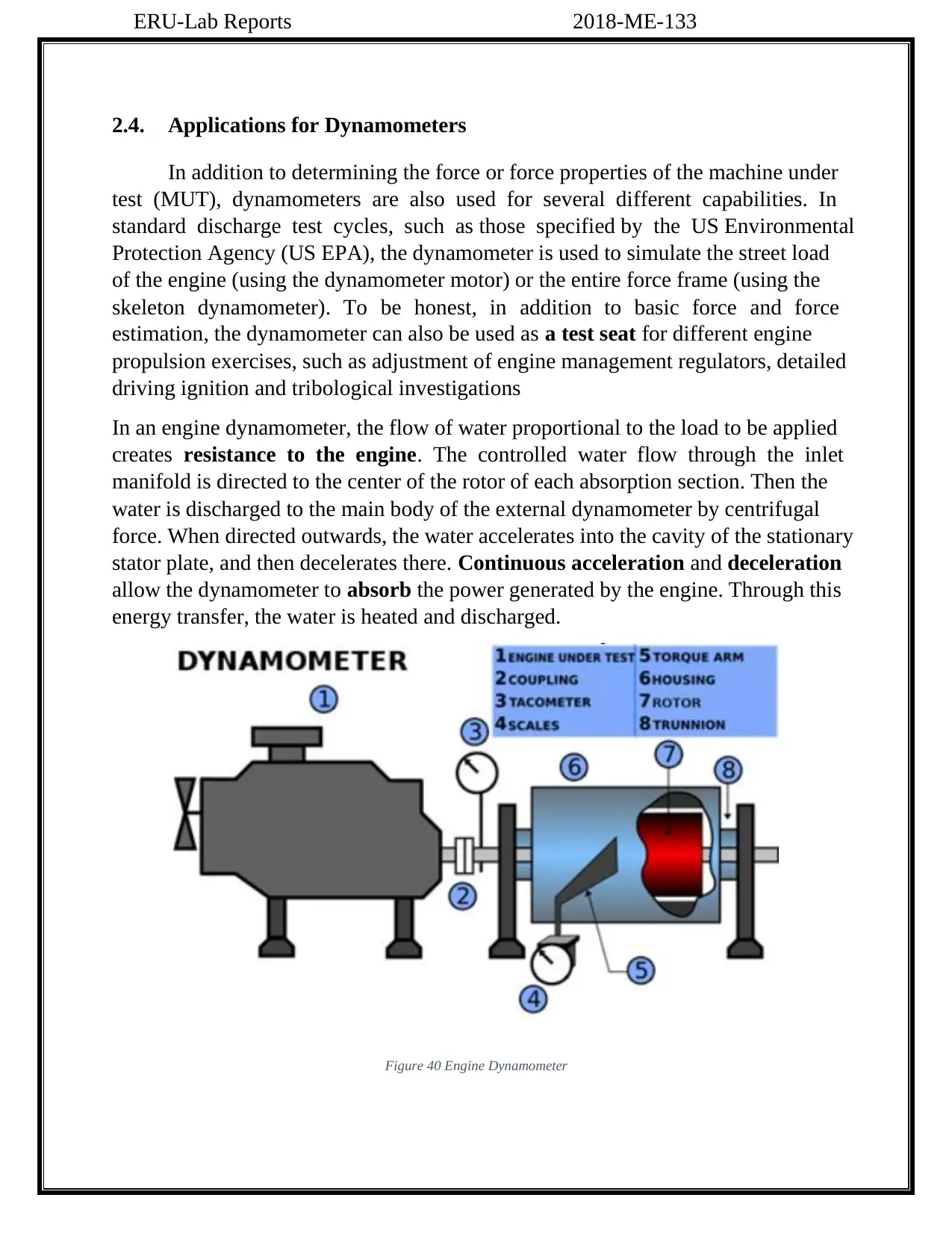
ERU-Lab Reports 2018-ME-133
2.4. Applications for Dynamometers
In addition to determining the force or force properties of the machine under
test (MUT), dynamometers are also used for several different capabilities. In
standard discharge test cycles, such as those specified by the US Environmental
Protection Agency (US EPA), the dynamometer is used to simulate the street load
of the engine (using the dynamometer motor) or the entire force frame (using the
skeleton dynamometer). To be honest, in addition to basic force and force
estimation, the dynamometer can also be used as a test seat for different engine
propulsion exercises, such as adjustment of engine management regulators, detailed
driving ignition and tribological investigations
In an engine dynamometer, the flow of water proportional to the load to be applied
creates resistance to the engine. The controlled water flow through the inlet
manifold is directed to the center of the rotor of each absorption section. Then the
water is discharged to the main body of the external dynamometer by centrifugal
force. When directed outwards, the water accelerates into the cavity of the stationary
stator plate, and then decelerates there. Continuous acceleration and deceleration
allow the dynamometer to absorb the power generated by the engine. Through this
energy transfer, the water is heated and discharged.
Figure 40 Engine Dynamometer
2.4. Applications for Dynamometers
In addition to determining the force or force properties of the machine under
test (MUT), dynamometers are also used for several different capabilities. In
standard discharge test cycles, such as those specified by the US Environmental
Protection Agency (US EPA), the dynamometer is used to simulate the street load
of the engine (using the dynamometer motor) or the entire force frame (using the
skeleton dynamometer). To be honest, in addition to basic force and force
estimation, the dynamometer can also be used as a test seat for different engine
propulsion exercises, such as adjustment of engine management regulators, detailed
driving ignition and tribological investigations
In an engine dynamometer, the flow of water proportional to the load to be applied
creates resistance to the engine. The controlled water flow through the inlet
manifold is directed to the center of the rotor of each absorption section. Then the
water is discharged to the main body of the external dynamometer by centrifugal
force. When directed outwards, the water accelerates into the cavity of the stationary
stator plate, and then decelerates there. Continuous acceleration and deceleration
allow the dynamometer to absorb the power generated by the engine. Through this
energy transfer, the water is heated and discharged.
Figure 40 Engine Dynamometer
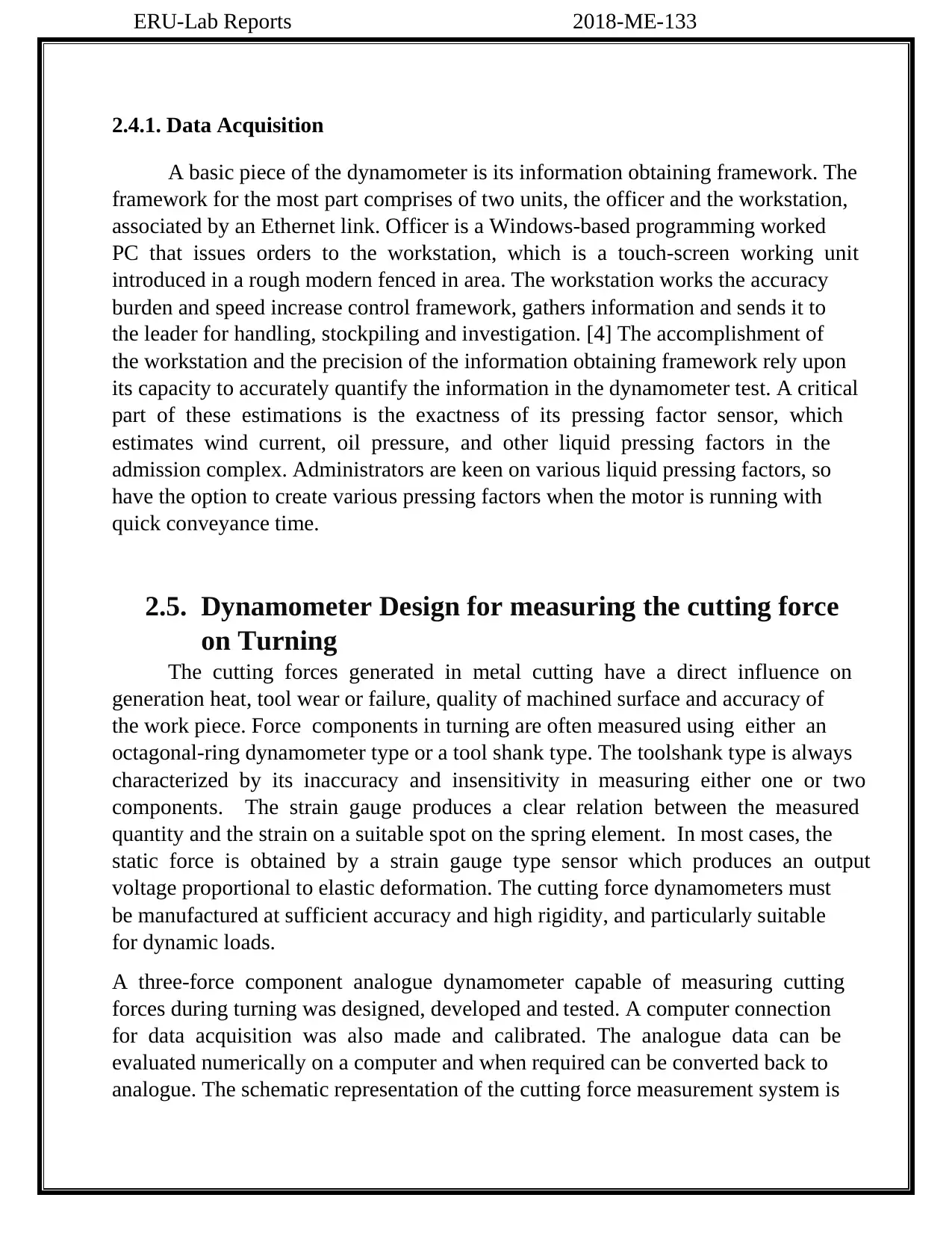
ERU-Lab Reports 2018-ME-133
2.4.1. Data Acquisition
A basic piece of the dynamometer is its information obtaining framework. The
framework for the most part comprises of two units, the officer and the workstation,
associated by an Ethernet link. Officer is a Windows-based programming worked
PC that issues orders to the workstation, which is a touch-screen working unit
introduced in a rough modern fenced in area. The workstation works the accuracy
burden and speed increase control framework, gathers information and sends it to
the leader for handling, stockpiling and investigation. [4] The accomplishment of
the workstation and the precision of the information obtaining framework rely upon
its capacity to accurately quantify the information in the dynamometer test. A critical
part of these estimations is the exactness of its pressing factor sensor, which
estimates wind current, oil pressure, and other liquid pressing factors in the
admission complex. Administrators are keen on various liquid pressing factors, so
have the option to create various pressing factors when the motor is running with
quick conveyance time.
2.5. Dynamometer Design for measuring the cutting force
on Turning
The cutting forces generated in metal cutting have a direct influence on
generation heat, tool wear or failure, quality of machined surface and accuracy of
the work piece. Force components in turning are often measured using either an
octagonal-ring dynamometer type or a tool shank type. The toolshank type is always
characterized by its inaccuracy and insensitivity in measuring either one or two
components. The strain gauge produces a clear relation between the measured
quantity and the strain on a suitable spot on the spring element. In most cases, the
static force is obtained by a strain gauge type sensor which produces an output
voltage proportional to elastic deformation. The cutting force dynamometers must
be manufactured at sufficient accuracy and high rigidity, and particularly suitable
for dynamic loads.
A three-force component analogue dynamometer capable of measuring cutting
forces during turning was designed, developed and tested. A computer connection
for data acquisition was also made and calibrated. The analogue data can be
evaluated numerically on a computer and when required can be converted back to
analogue. The schematic representation of the cutting force measurement system is
2.4.1. Data Acquisition
A basic piece of the dynamometer is its information obtaining framework. The
framework for the most part comprises of two units, the officer and the workstation,
associated by an Ethernet link. Officer is a Windows-based programming worked
PC that issues orders to the workstation, which is a touch-screen working unit
introduced in a rough modern fenced in area. The workstation works the accuracy
burden and speed increase control framework, gathers information and sends it to
the leader for handling, stockpiling and investigation. [4] The accomplishment of
the workstation and the precision of the information obtaining framework rely upon
its capacity to accurately quantify the information in the dynamometer test. A critical
part of these estimations is the exactness of its pressing factor sensor, which
estimates wind current, oil pressure, and other liquid pressing factors in the
admission complex. Administrators are keen on various liquid pressing factors, so
have the option to create various pressing factors when the motor is running with
quick conveyance time.
2.5. Dynamometer Design for measuring the cutting force
on Turning
The cutting forces generated in metal cutting have a direct influence on
generation heat, tool wear or failure, quality of machined surface and accuracy of
the work piece. Force components in turning are often measured using either an
octagonal-ring dynamometer type or a tool shank type. The toolshank type is always
characterized by its inaccuracy and insensitivity in measuring either one or two
components. The strain gauge produces a clear relation between the measured
quantity and the strain on a suitable spot on the spring element. In most cases, the
static force is obtained by a strain gauge type sensor which produces an output
voltage proportional to elastic deformation. The cutting force dynamometers must
be manufactured at sufficient accuracy and high rigidity, and particularly suitable
for dynamic loads.
A three-force component analogue dynamometer capable of measuring cutting
forces during turning was designed, developed and tested. A computer connection
for data acquisition was also made and calibrated. The analogue data can be
evaluated numerically on a computer and when required can be converted back to
analogue. The schematic representation of the cutting force measurement system is
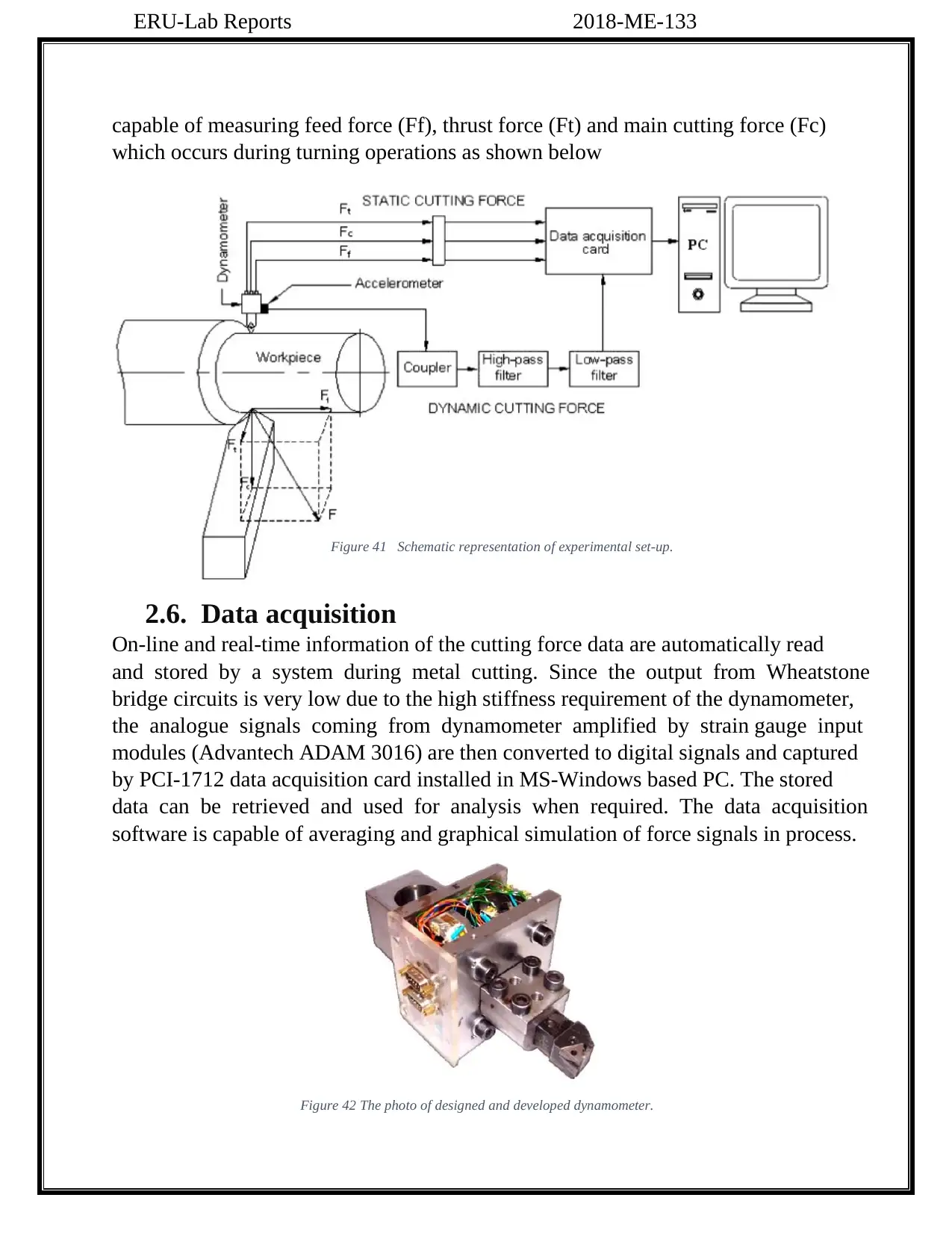
ERU-Lab Reports 2018-ME-133
capable of measuring feed force (Ff), thrust force (Ft) and main cutting force (Fc)
which occurs during turning operations as shown below
2.6. Data acquisition
On-line and real-time information of the cutting force data are automatically read
and stored by a system during metal cutting. Since the output from Wheatstone
bridge circuits is very low due to the high stiffness requirement of the dynamometer,
the analogue signals coming from dynamometer amplified by strain gauge input
modules (Advantech ADAM 3016) are then converted to digital signals and captured
by PCI-1712 data acquisition card installed in MS-Windows based PC. The stored
data can be retrieved and used for analysis when required. The data acquisition
software is capable of averaging and graphical simulation of force signals in process.
Figure 42 The photo of designed and developed dynamometer.
Figure 41 Schematic representation of experimental set-up.
capable of measuring feed force (Ff), thrust force (Ft) and main cutting force (Fc)
which occurs during turning operations as shown below
2.6. Data acquisition
On-line and real-time information of the cutting force data are automatically read
and stored by a system during metal cutting. Since the output from Wheatstone
bridge circuits is very low due to the high stiffness requirement of the dynamometer,
the analogue signals coming from dynamometer amplified by strain gauge input
modules (Advantech ADAM 3016) are then converted to digital signals and captured
by PCI-1712 data acquisition card installed in MS-Windows based PC. The stored
data can be retrieved and used for analysis when required. The data acquisition
software is capable of averaging and graphical simulation of force signals in process.
Figure 42 The photo of designed and developed dynamometer.
Figure 41 Schematic representation of experimental set-up.
Paraphrase This Document
Need a fresh take? Get an instant paraphrase of this document with our AI Paraphraser
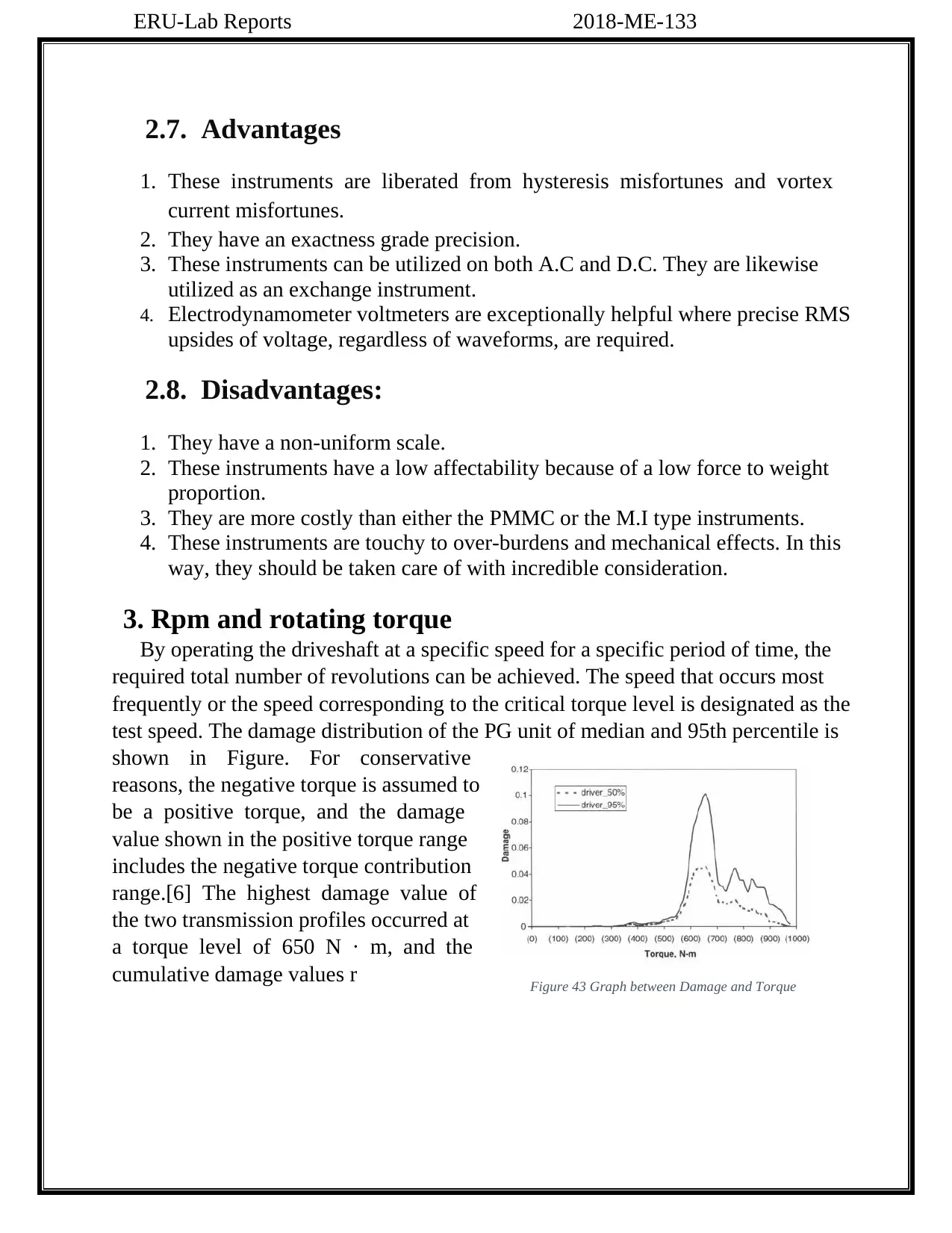
ERU-Lab Reports 2018-ME-133
2.7. Advantages
1. These instruments are liberated from hysteresis misfortunes and vortex
current misfortunes.
2. They have an exactness grade precision.
3. These instruments can be utilized on both A.C and D.C. They are likewise
utilized as an exchange instrument.
4. Electrodynamometer voltmeters are exceptionally helpful where precise RMS
upsides of voltage, regardless of waveforms, are required.
2.8. Disadvantages:
1. They have a non-uniform scale.
2. These instruments have a low affectability because of a low force to weight
proportion.
3. They are more costly than either the PMMC or the M.I type instruments.
4. These instruments are touchy to over-burdens and mechanical effects. In this
way, they should be taken care of with incredible consideration.
3. Rpm and rotating torque
By operating the driveshaft at a specific speed for a specific period of time, the
required total number of revolutions can be achieved. The speed that occurs most
frequently or the speed corresponding to the critical torque level is designated as the
test speed. The damage distribution of the PG unit of median and 95th percentile is
shown in Figure. For conservative
reasons, the negative torque is assumed to
be a positive torque, and the damage
value shown in the positive torque range
includes the negative torque contribution
range.[6] The highest damage value of
the two transmission profiles occurred at
a torque level of 650 N · m, and the
cumulative damage values r Figure 43 Graph between Damage and Torque
2.7. Advantages
1. These instruments are liberated from hysteresis misfortunes and vortex
current misfortunes.
2. They have an exactness grade precision.
3. These instruments can be utilized on both A.C and D.C. They are likewise
utilized as an exchange instrument.
4. Electrodynamometer voltmeters are exceptionally helpful where precise RMS
upsides of voltage, regardless of waveforms, are required.
2.8. Disadvantages:
1. They have a non-uniform scale.
2. These instruments have a low affectability because of a low force to weight
proportion.
3. They are more costly than either the PMMC or the M.I type instruments.
4. These instruments are touchy to over-burdens and mechanical effects. In this
way, they should be taken care of with incredible consideration.
3. Rpm and rotating torque
By operating the driveshaft at a specific speed for a specific period of time, the
required total number of revolutions can be achieved. The speed that occurs most
frequently or the speed corresponding to the critical torque level is designated as the
test speed. The damage distribution of the PG unit of median and 95th percentile is
shown in Figure. For conservative
reasons, the negative torque is assumed to
be a positive torque, and the damage
value shown in the positive torque range
includes the negative torque contribution
range.[6] The highest damage value of
the two transmission profiles occurred at
a torque level of 650 N · m, and the
cumulative damage values r Figure 43 Graph between Damage and Torque
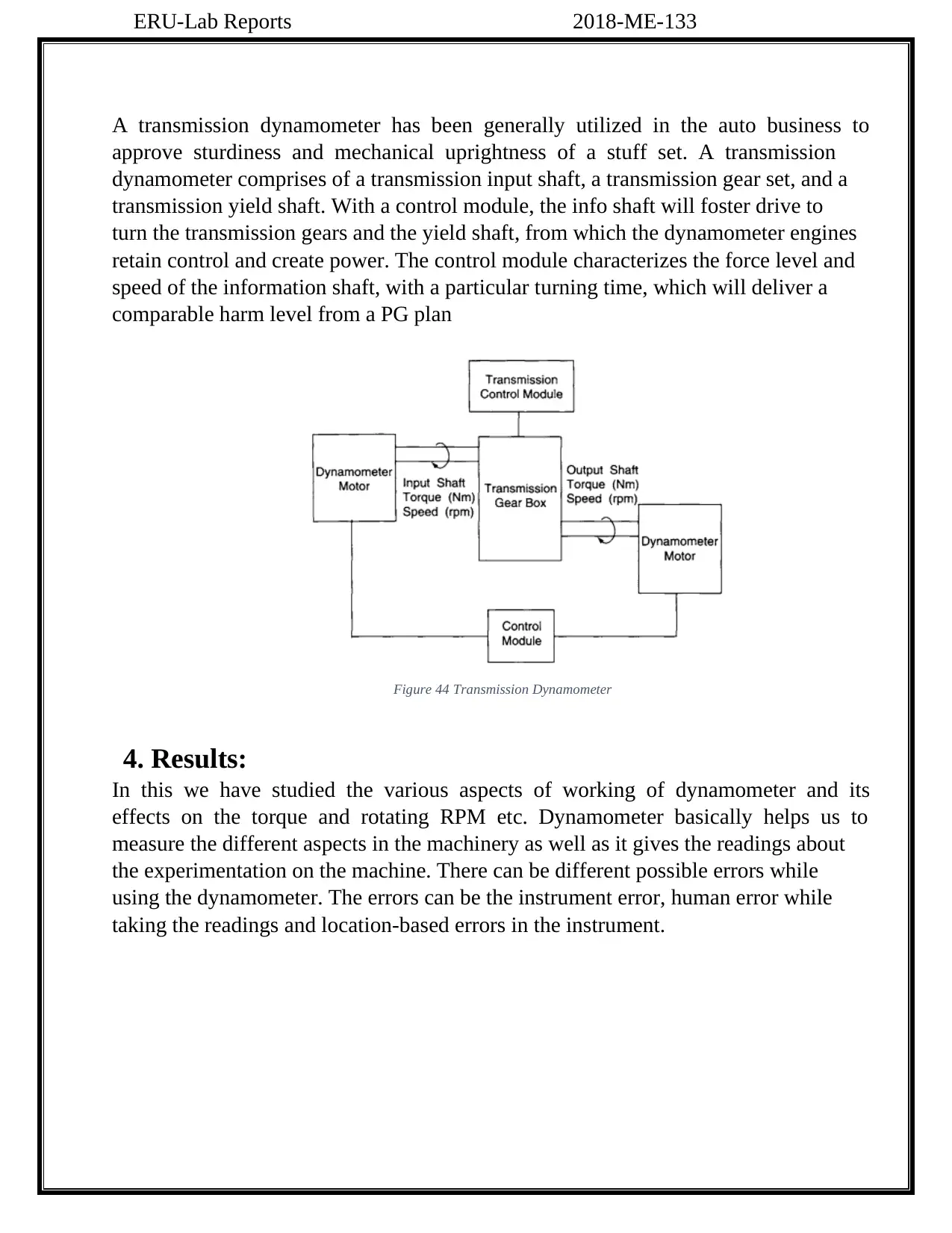
ERU-Lab Reports 2018-ME-133
A transmission dynamometer has been generally utilized in the auto business to
approve sturdiness and mechanical uprightness of a stuff set. A transmission
dynamometer comprises of a transmission input shaft, a transmission gear set, and a
transmission yield shaft. With a control module, the info shaft will foster drive to
turn the transmission gears and the yield shaft, from which the dynamometer engines
retain control and create power. The control module characterizes the force level and
speed of the information shaft, with a particular turning time, which will deliver a
comparable harm level from a PG plan
4. Results:
In this we have studied the various aspects of working of dynamometer and its
effects on the torque and rotating RPM etc. Dynamometer basically helps us to
measure the different aspects in the machinery as well as it gives the readings about
the experimentation on the machine. There can be different possible errors while
using the dynamometer. The errors can be the instrument error, human error while
taking the readings and location-based errors in the instrument.
Figure 44 Transmission Dynamometer
A transmission dynamometer has been generally utilized in the auto business to
approve sturdiness and mechanical uprightness of a stuff set. A transmission
dynamometer comprises of a transmission input shaft, a transmission gear set, and a
transmission yield shaft. With a control module, the info shaft will foster drive to
turn the transmission gears and the yield shaft, from which the dynamometer engines
retain control and create power. The control module characterizes the force level and
speed of the information shaft, with a particular turning time, which will deliver a
comparable harm level from a PG plan
4. Results:
In this we have studied the various aspects of working of dynamometer and its
effects on the torque and rotating RPM etc. Dynamometer basically helps us to
measure the different aspects in the machinery as well as it gives the readings about
the experimentation on the machine. There can be different possible errors while
using the dynamometer. The errors can be the instrument error, human error while
taking the readings and location-based errors in the instrument.
Figure 44 Transmission Dynamometer
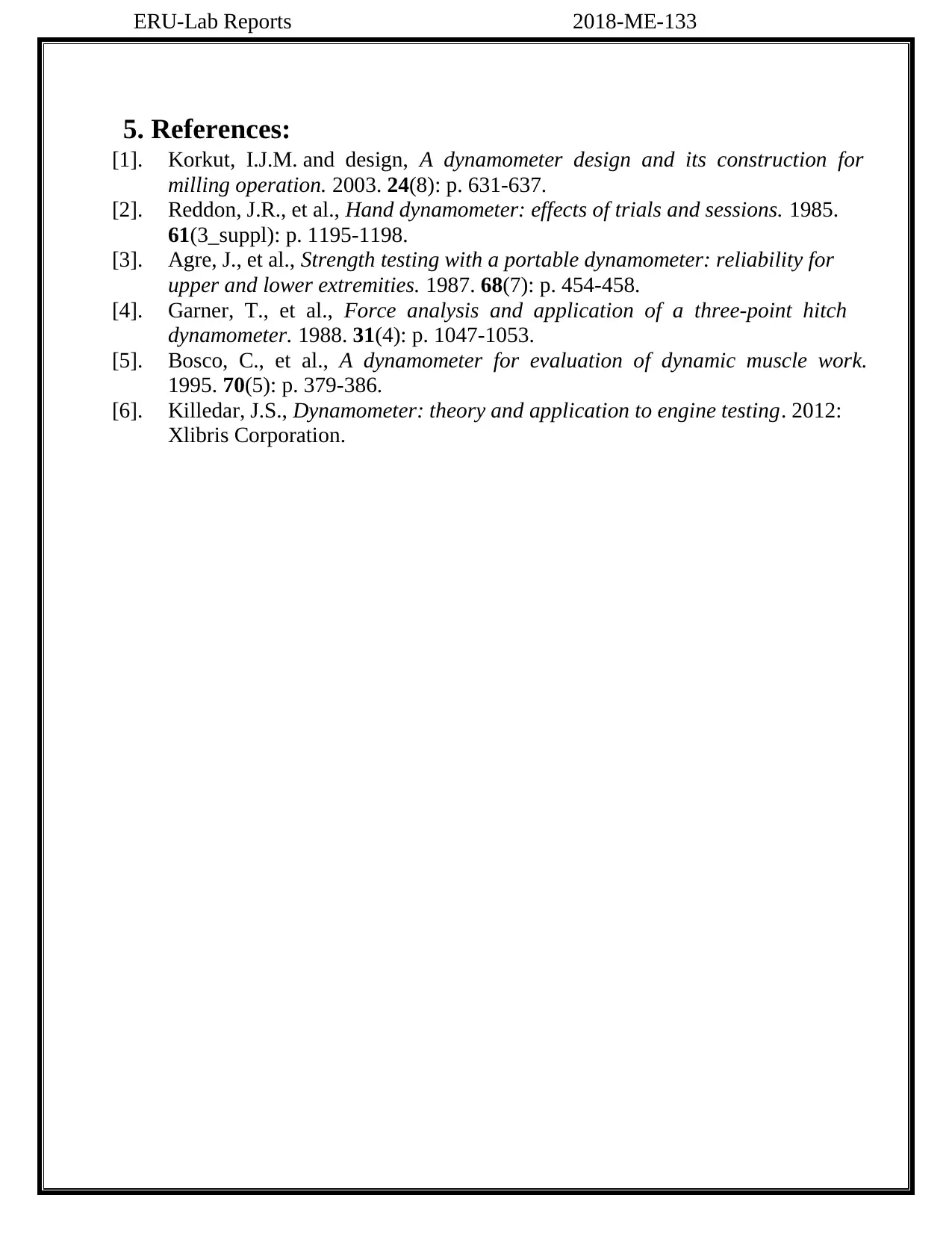
ERU-Lab Reports 2018-ME-133
5. References:
[1]. Korkut, I.J.M. and design, A dynamometer design and its construction for
milling operation. 2003. 24(8): p. 631-637.
[2]. Reddon, J.R., et al., Hand dynamometer: effects of trials and sessions. 1985.
61(3_suppl): p. 1195-1198.
[3]. Agre, J., et al., Strength testing with a portable dynamometer: reliability for
upper and lower extremities. 1987. 68(7): p. 454-458.
[4]. Garner, T., et al., Force analysis and application of a three-point hitch
dynamometer. 1988. 31(4): p. 1047-1053.
[5]. Bosco, C., et al., A dynamometer for evaluation of dynamic muscle work.
1995. 70(5): p. 379-386.
[6]. Killedar, J.S., Dynamometer: theory and application to engine testing. 2012:
Xlibris Corporation.
5. References:
[1]. Korkut, I.J.M. and design, A dynamometer design and its construction for
milling operation. 2003. 24(8): p. 631-637.
[2]. Reddon, J.R., et al., Hand dynamometer: effects of trials and sessions. 1985.
61(3_suppl): p. 1195-1198.
[3]. Agre, J., et al., Strength testing with a portable dynamometer: reliability for
upper and lower extremities. 1987. 68(7): p. 454-458.
[4]. Garner, T., et al., Force analysis and application of a three-point hitch
dynamometer. 1988. 31(4): p. 1047-1053.
[5]. Bosco, C., et al., A dynamometer for evaluation of dynamic muscle work.
1995. 70(5): p. 379-386.
[6]. Killedar, J.S., Dynamometer: theory and application to engine testing. 2012:
Xlibris Corporation.
Secure Best Marks with AI Grader
Need help grading? Try our AI Grader for instant feedback on your assignments.
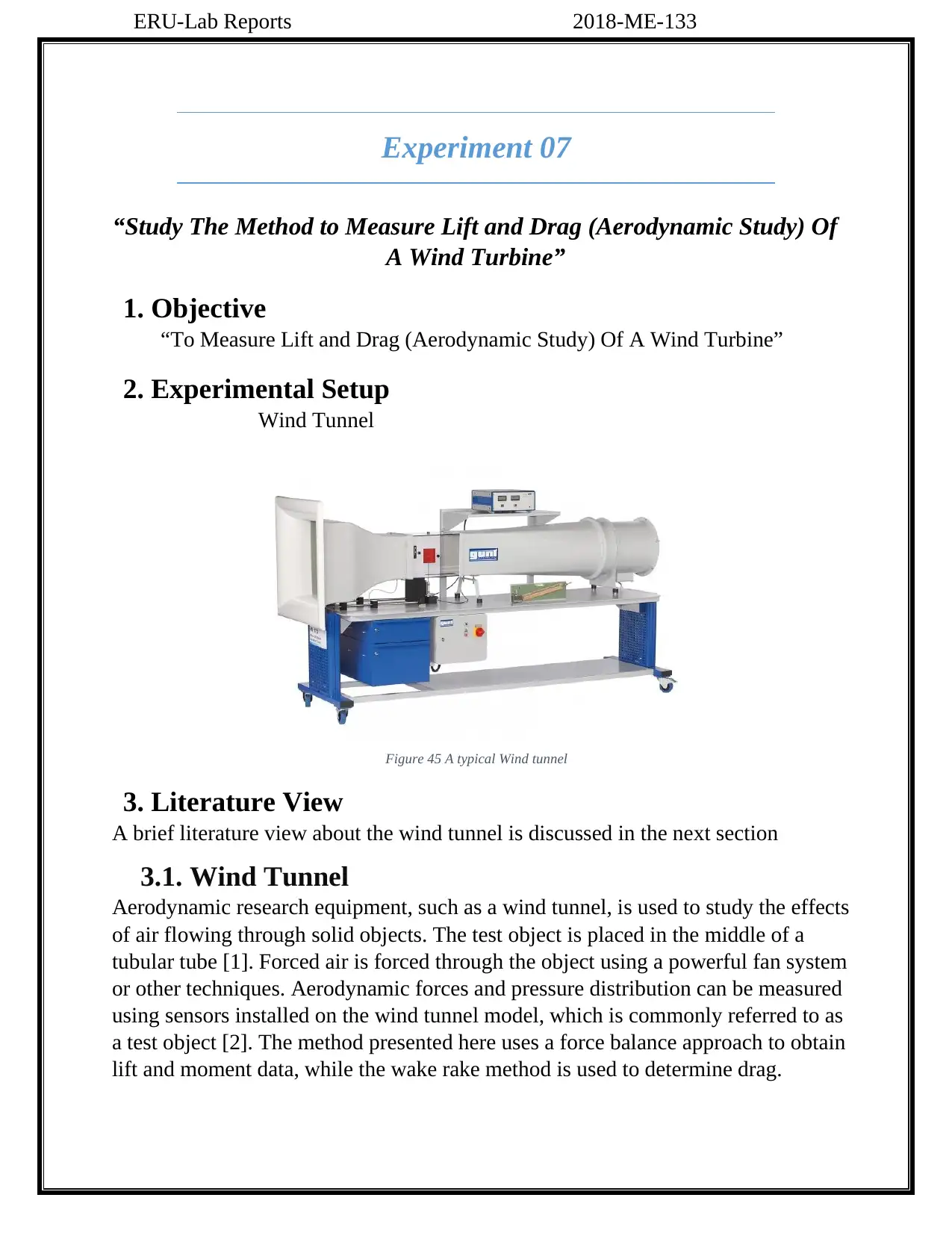
ERU-Lab Reports 2018-ME-133
Experiment 07
“Study The Method to Measure Lift and Drag (Aerodynamic Study) Of
A Wind Turbine”
1. Objective
“To Measure Lift and Drag (Aerodynamic Study) Of A Wind Turbine”
2. Experimental Setup
Wind Tunnel
Figure 45 A typical Wind tunnel
3. Literature View
A brief literature view about the wind tunnel is discussed in the next section
3.1. Wind Tunnel
Aerodynamic research equipment, such as a wind tunnel, is used to study the effects
of air flowing through solid objects. The test object is placed in the middle of a
tubular tube [1]. Forced air is forced through the object using a powerful fan system
or other techniques. Aerodynamic forces and pressure distribution can be measured
using sensors installed on the wind tunnel model, which is commonly referred to as
a test object [2]. The method presented here uses a force balance approach to obtain
lift and moment data, while the wake rake method is used to determine drag.
Experiment 07
“Study The Method to Measure Lift and Drag (Aerodynamic Study) Of
A Wind Turbine”
1. Objective
“To Measure Lift and Drag (Aerodynamic Study) Of A Wind Turbine”
2. Experimental Setup
Wind Tunnel
Figure 45 A typical Wind tunnel
3. Literature View
A brief literature view about the wind tunnel is discussed in the next section
3.1. Wind Tunnel
Aerodynamic research equipment, such as a wind tunnel, is used to study the effects
of air flowing through solid objects. The test object is placed in the middle of a
tubular tube [1]. Forced air is forced through the object using a powerful fan system
or other techniques. Aerodynamic forces and pressure distribution can be measured
using sensors installed on the wind tunnel model, which is commonly referred to as
a test object [2]. The method presented here uses a force balance approach to obtain
lift and moment data, while the wake rake method is used to determine drag.
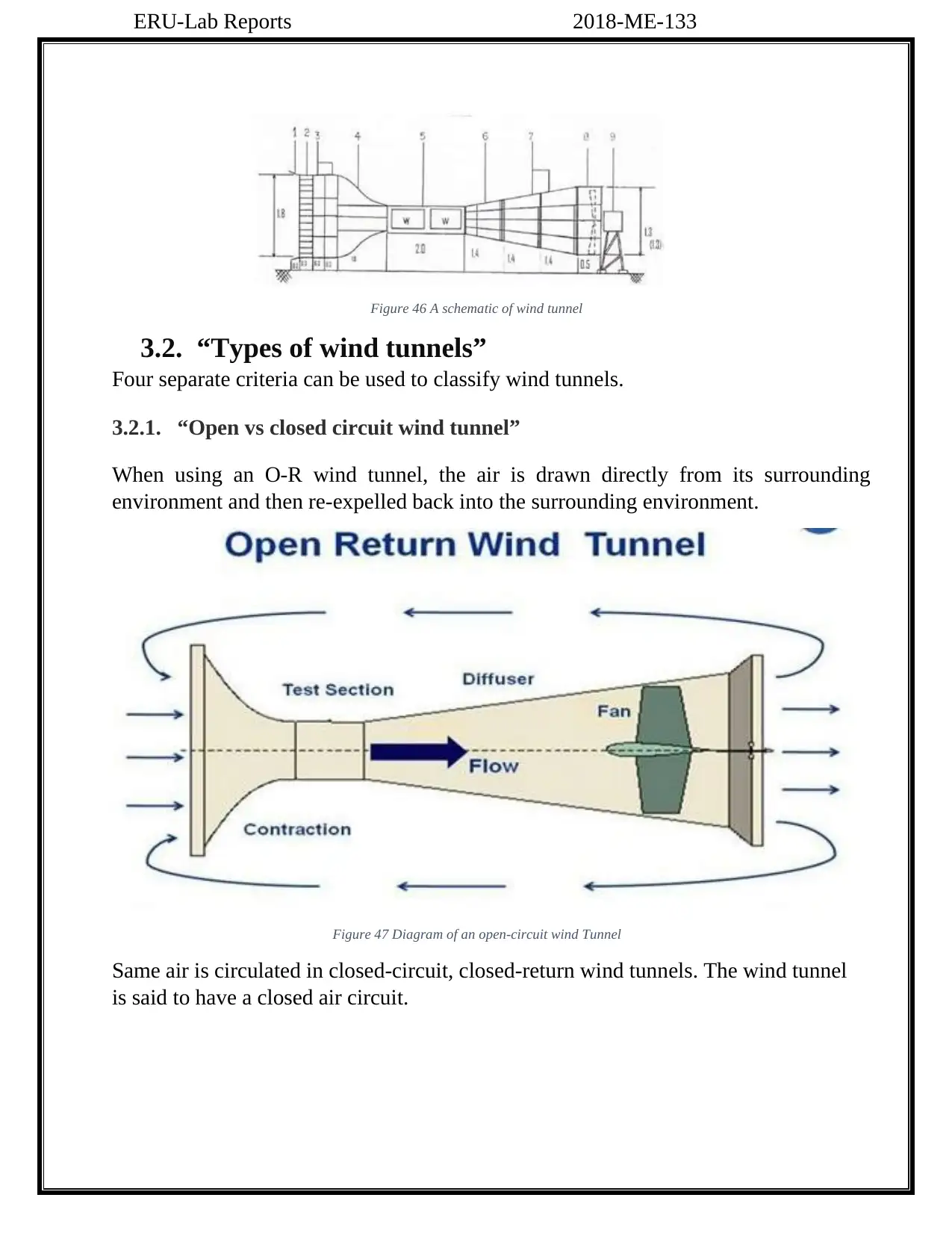
ERU-Lab Reports 2018-ME-133
Figure 46 A schematic of wind tunnel
3.2. “Types of wind tunnels”
Four separate criteria can be used to classify wind tunnels.
3.2.1. “Open vs closed circuit wind tunnel”
When using an O-R wind tunnel, the air is drawn directly from its surrounding
environment and then re-expelled back into the surrounding environment.
Figure 47 Diagram of an open-circuit wind Tunnel
Same air is circulated in closed-circuit, closed-return wind tunnels. The wind tunnel
is said to have a closed air circuit.
Figure 46 A schematic of wind tunnel
3.2. “Types of wind tunnels”
Four separate criteria can be used to classify wind tunnels.
3.2.1. “Open vs closed circuit wind tunnel”
When using an O-R wind tunnel, the air is drawn directly from its surrounding
environment and then re-expelled back into the surrounding environment.
Figure 47 Diagram of an open-circuit wind Tunnel
Same air is circulated in closed-circuit, closed-return wind tunnels. The wind tunnel
is said to have a closed air circuit.
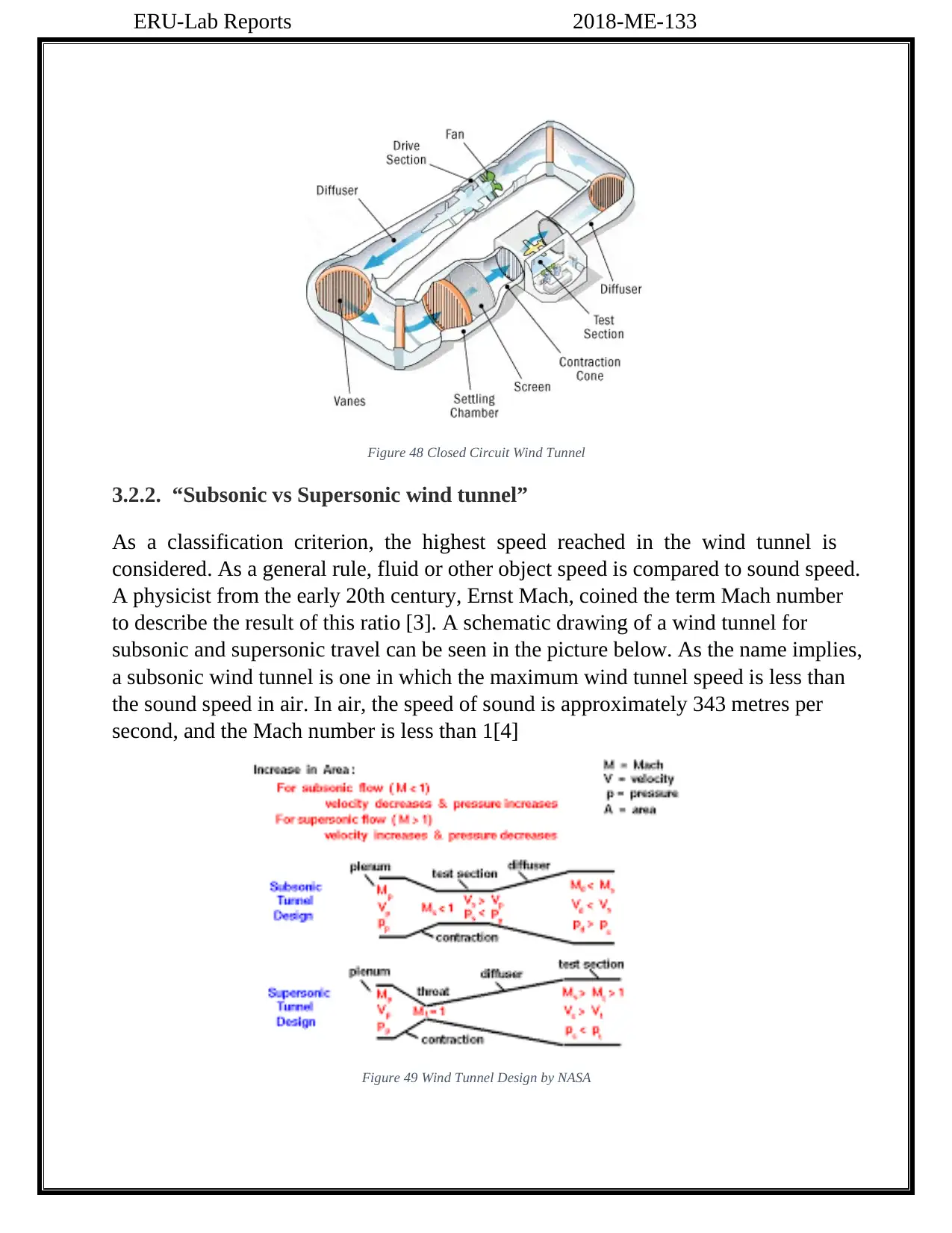
ERU-Lab Reports 2018-ME-133
Figure 48 Closed Circuit Wind Tunnel
3.2.2. “Subsonic vs Supersonic wind tunnel”
As a classification criterion, the highest speed reached in the wind tunnel is
considered. As a general rule, fluid or other object speed is compared to sound speed.
A physicist from the early 20th century, Ernst Mach, coined the term Mach number
to describe the result of this ratio [3]. A schematic drawing of a wind tunnel for
subsonic and supersonic travel can be seen in the picture below. As the name implies,
a subsonic wind tunnel is one in which the maximum wind tunnel speed is less than
the sound speed in air. In air, the speed of sound is approximately 343 metres per
second, and the Mach number is less than 1[4]
Figure 49 Wind Tunnel Design by NASA
Figure 48 Closed Circuit Wind Tunnel
3.2.2. “Subsonic vs Supersonic wind tunnel”
As a classification criterion, the highest speed reached in the wind tunnel is
considered. As a general rule, fluid or other object speed is compared to sound speed.
A physicist from the early 20th century, Ernst Mach, coined the term Mach number
to describe the result of this ratio [3]. A schematic drawing of a wind tunnel for
subsonic and supersonic travel can be seen in the picture below. As the name implies,
a subsonic wind tunnel is one in which the maximum wind tunnel speed is less than
the sound speed in air. In air, the speed of sound is approximately 343 metres per
second, and the Mach number is less than 1[4]
Figure 49 Wind Tunnel Design by NASA
Paraphrase This Document
Need a fresh take? Get an instant paraphrase of this document with our AI Paraphraser
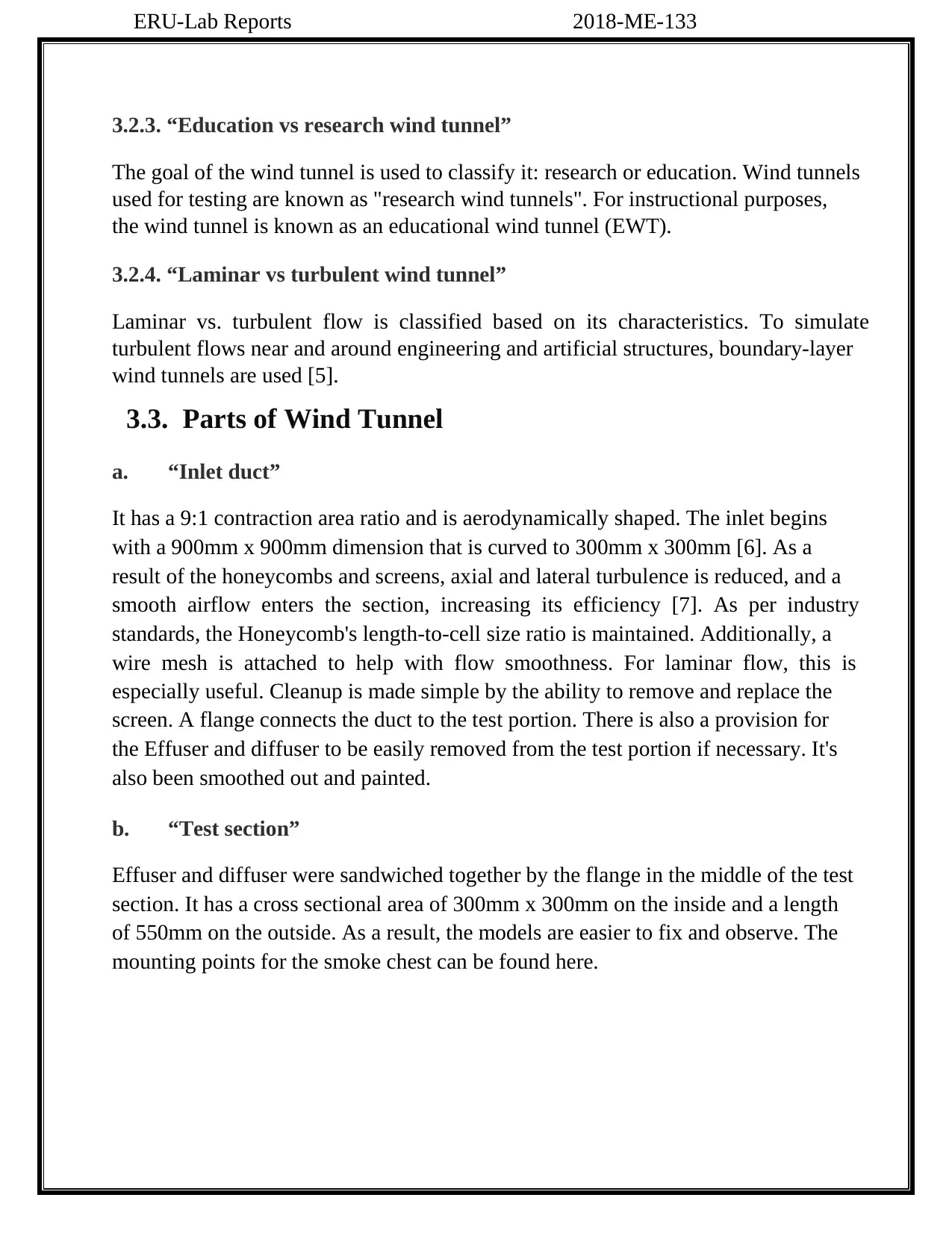
ERU-Lab Reports 2018-ME-133
3.2.3. “Education vs research wind tunnel”
The goal of the wind tunnel is used to classify it: research or education. Wind tunnels
used for testing are known as "research wind tunnels". For instructional purposes,
the wind tunnel is known as an educational wind tunnel (EWT).
3.2.4. “Laminar vs turbulent wind tunnel”
Laminar vs. turbulent flow is classified based on its characteristics. To simulate
turbulent flows near and around engineering and artificial structures, boundary-layer
wind tunnels are used [5].
3.3. Parts of Wind Tunnel
a. “Inlet duct”
It has a 9:1 contraction area ratio and is aerodynamically shaped. The inlet begins
with a 900mm x 900mm dimension that is curved to 300mm x 300mm [6]. As a
result of the honeycombs and screens, axial and lateral turbulence is reduced, and a
smooth airflow enters the section, increasing its efficiency [7]. As per industry
standards, the Honeycomb's length-to-cell size ratio is maintained. Additionally, a
wire mesh is attached to help with flow smoothness. For laminar flow, this is
especially useful. Cleanup is made simple by the ability to remove and replace the
screen. A flange connects the duct to the test portion. There is also a provision for
the Effuser and diffuser to be easily removed from the test portion if necessary. It's
also been smoothed out and painted.
b. “Test section”
Effuser and diffuser were sandwiched together by the flange in the middle of the test
section. It has a cross sectional area of 300mm x 300mm on the inside and a length
of 550mm on the outside. As a result, the models are easier to fix and observe. The
mounting points for the smoke chest can be found here.
3.2.3. “Education vs research wind tunnel”
The goal of the wind tunnel is used to classify it: research or education. Wind tunnels
used for testing are known as "research wind tunnels". For instructional purposes,
the wind tunnel is known as an educational wind tunnel (EWT).
3.2.4. “Laminar vs turbulent wind tunnel”
Laminar vs. turbulent flow is classified based on its characteristics. To simulate
turbulent flows near and around engineering and artificial structures, boundary-layer
wind tunnels are used [5].
3.3. Parts of Wind Tunnel
a. “Inlet duct”
It has a 9:1 contraction area ratio and is aerodynamically shaped. The inlet begins
with a 900mm x 900mm dimension that is curved to 300mm x 300mm [6]. As a
result of the honeycombs and screens, axial and lateral turbulence is reduced, and a
smooth airflow enters the section, increasing its efficiency [7]. As per industry
standards, the Honeycomb's length-to-cell size ratio is maintained. Additionally, a
wire mesh is attached to help with flow smoothness. For laminar flow, this is
especially useful. Cleanup is made simple by the ability to remove and replace the
screen. A flange connects the duct to the test portion. There is also a provision for
the Effuser and diffuser to be easily removed from the test portion if necessary. It's
also been smoothed out and painted.
b. “Test section”
Effuser and diffuser were sandwiched together by the flange in the middle of the test
section. It has a cross sectional area of 300mm x 300mm on the inside and a length
of 550mm on the outside. As a result, the models are easier to fix and observe. The
mounting points for the smoke chest can be found here.
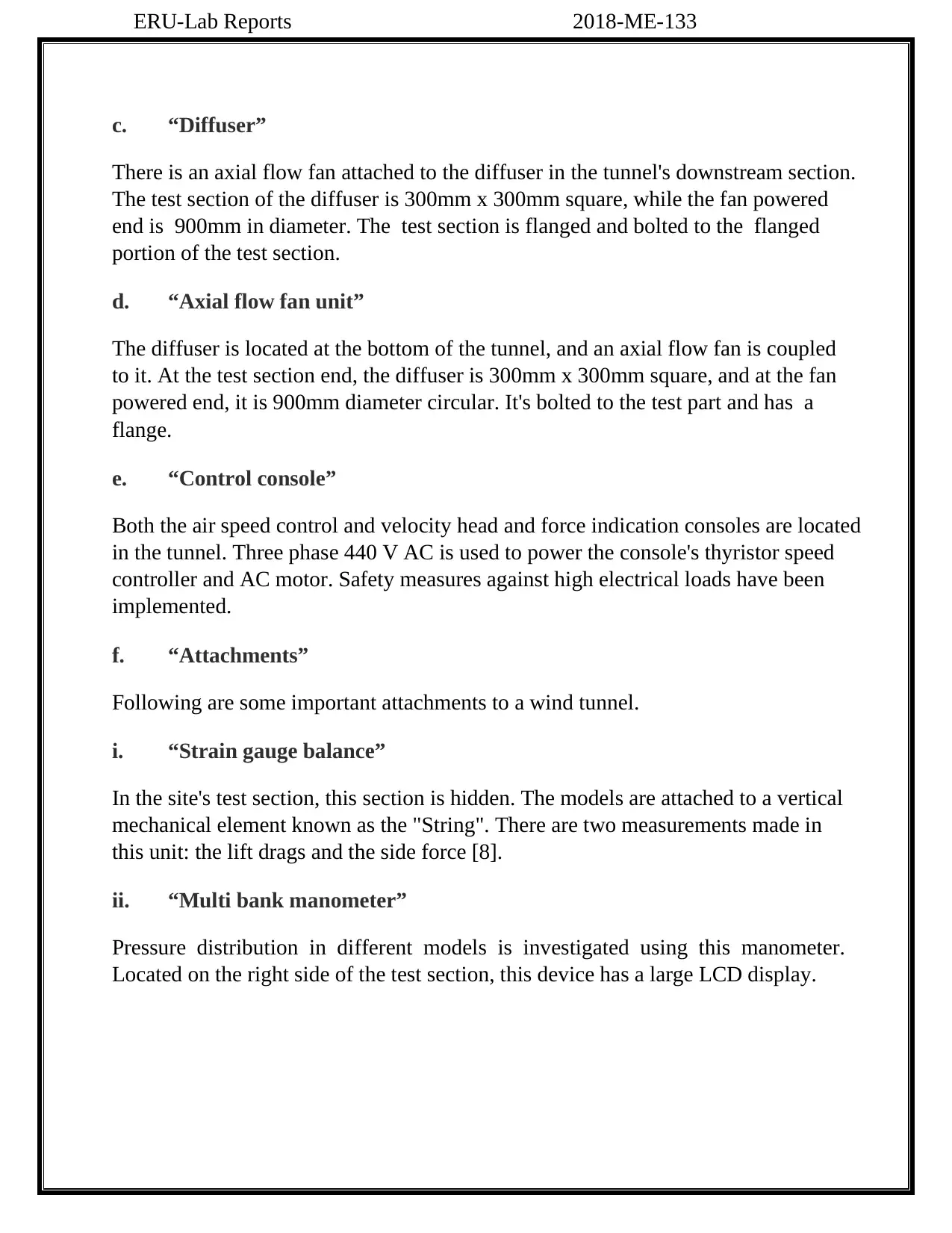
ERU-Lab Reports 2018-ME-133
c. “Diffuser”
There is an axial flow fan attached to the diffuser in the tunnel's downstream section.
The test section of the diffuser is 300mm x 300mm square, while the fan powered
end is 900mm in diameter. The test section is flanged and bolted to the flanged
portion of the test section.
d. “Axial flow fan unit”
The diffuser is located at the bottom of the tunnel, and an axial flow fan is coupled
to it. At the test section end, the diffuser is 300mm x 300mm square, and at the fan
powered end, it is 900mm diameter circular. It's bolted to the test part and has a
flange.
e. “Control console”
Both the air speed control and velocity head and force indication consoles are located
in the tunnel. Three phase 440 V AC is used to power the console's thyristor speed
controller and AC motor. Safety measures against high electrical loads have been
implemented.
f. “Attachments”
Following are some important attachments to a wind tunnel.
i. “Strain gauge balance”
In the site's test section, this section is hidden. The models are attached to a vertical
mechanical element known as the "String". There are two measurements made in
this unit: the lift drags and the side force [8].
ii. “Multi bank manometer”
Pressure distribution in different models is investigated using this manometer.
Located on the right side of the test section, this device has a large LCD display.
c. “Diffuser”
There is an axial flow fan attached to the diffuser in the tunnel's downstream section.
The test section of the diffuser is 300mm x 300mm square, while the fan powered
end is 900mm in diameter. The test section is flanged and bolted to the flanged
portion of the test section.
d. “Axial flow fan unit”
The diffuser is located at the bottom of the tunnel, and an axial flow fan is coupled
to it. At the test section end, the diffuser is 300mm x 300mm square, and at the fan
powered end, it is 900mm diameter circular. It's bolted to the test part and has a
flange.
e. “Control console”
Both the air speed control and velocity head and force indication consoles are located
in the tunnel. Three phase 440 V AC is used to power the console's thyristor speed
controller and AC motor. Safety measures against high electrical loads have been
implemented.
f. “Attachments”
Following are some important attachments to a wind tunnel.
i. “Strain gauge balance”
In the site's test section, this section is hidden. The models are attached to a vertical
mechanical element known as the "String". There are two measurements made in
this unit: the lift drags and the side force [8].
ii. “Multi bank manometer”
Pressure distribution in different models is investigated using this manometer.
Located on the right side of the test section, this device has a large LCD display.
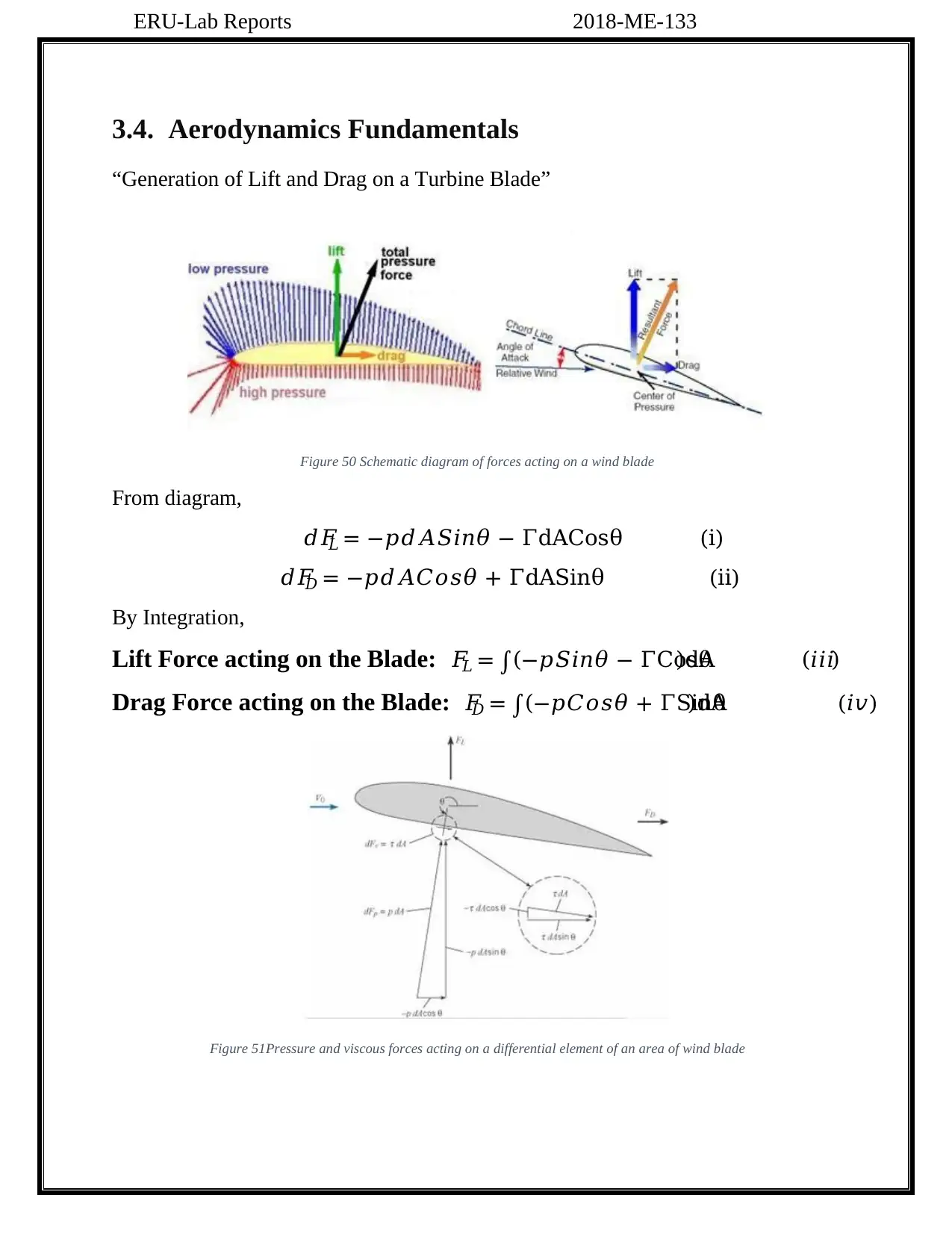
ERU-Lab Reports 2018-ME-133
3.4. Aerodynamics Fundamentals
“Generation of Lift and Drag on a Turbine Blade”
Figure 50 Schematic diagram of forces acting on a wind blade
From diagram,
𝑑𝐹𝐿 = −𝑝𝑑𝐴𝑆𝑖𝑛𝜃 − ΓdACosθ (i)
𝑑𝐹𝐷 = −𝑝𝑑𝐴𝐶𝑜𝑠𝜃 + ΓdASinθ (ii)
By Integration,
Lift Force acting on the Blade: 𝐹𝐿 = ∫(−𝑝𝑆𝑖𝑛𝜃 − ΓCosθ)dA (𝑖𝑖𝑖)
Drag Force acting on the Blade: 𝐹𝐷 = ∫(−𝑝𝐶𝑜𝑠𝜃 + ΓSinθ)dA (𝑖𝑣)
Figure 51Pressure and viscous forces acting on a differential element of an area of wind blade
3.4. Aerodynamics Fundamentals
“Generation of Lift and Drag on a Turbine Blade”
Figure 50 Schematic diagram of forces acting on a wind blade
From diagram,
𝑑𝐹𝐿 = −𝑝𝑑𝐴𝑆𝑖𝑛𝜃 − ΓdACosθ (i)
𝑑𝐹𝐷 = −𝑝𝑑𝐴𝐶𝑜𝑠𝜃 + ΓdASinθ (ii)
By Integration,
Lift Force acting on the Blade: 𝐹𝐿 = ∫(−𝑝𝑆𝑖𝑛𝜃 − ΓCosθ)dA (𝑖𝑖𝑖)
Drag Force acting on the Blade: 𝐹𝐷 = ∫(−𝑝𝐶𝑜𝑠𝜃 + ΓSinθ)dA (𝑖𝑣)
Figure 51Pressure and viscous forces acting on a differential element of an area of wind blade
Secure Best Marks with AI Grader
Need help grading? Try our AI Grader for instant feedback on your assignments.
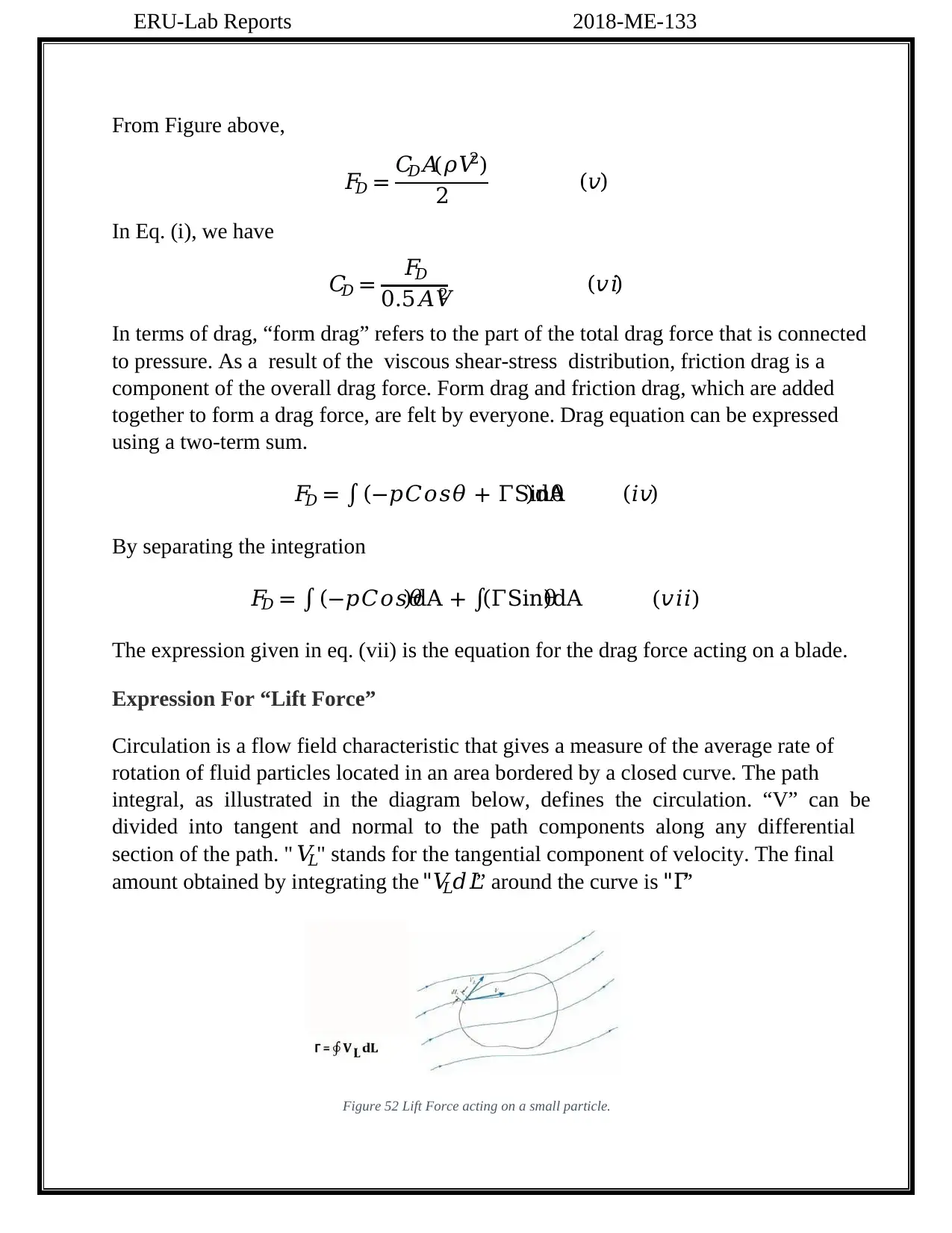
ERU-Lab Reports 2018-ME-133
From Figure above,
𝐹𝐷 = 𝐶𝐷𝐴(𝜌𝑉2)
2 (𝑣)
In Eq. (i), we have
𝐶𝐷 = 𝐹𝐷
0.5𝐴𝑉2 (𝑣𝑖)
In terms of drag, “form drag” refers to the part of the total drag force that is connected
to pressure. As a result of the viscous shear-stress distribution, friction drag is a
component of the overall drag force. Form drag and friction drag, which are added
together to form a drag force, are felt by everyone. Drag equation can be expressed
using a two-term sum.
𝐹𝐷 = ∫ (−𝑝𝐶𝑜𝑠𝜃 + ΓSinθ)dA (𝑖𝑣)
By separating the integration
𝐹𝐷 = ∫ (−𝑝𝐶𝑜𝑠𝜃)dA + ∫(ΓSinθ)dA (𝑣𝑖𝑖)
The expression given in eq. (vii) is the equation for the drag force acting on a blade.
Expression For “Lift Force”
Circulation is a flow field characteristic that gives a measure of the average rate of
rotation of fluid particles located in an area bordered by a closed curve. The path
integral, as illustrated in the diagram below, defines the circulation. “V” can be
divided into tangent and normal to the path components along any differential
section of the path. " 𝑉𝐿" stands for the tangential component of velocity. The final
amount obtained by integrating the "𝑉𝐿𝑑𝐿” around the curve is "Γ”
Figure 52 Lift Force acting on a small particle.
From Figure above,
𝐹𝐷 = 𝐶𝐷𝐴(𝜌𝑉2)
2 (𝑣)
In Eq. (i), we have
𝐶𝐷 = 𝐹𝐷
0.5𝐴𝑉2 (𝑣𝑖)
In terms of drag, “form drag” refers to the part of the total drag force that is connected
to pressure. As a result of the viscous shear-stress distribution, friction drag is a
component of the overall drag force. Form drag and friction drag, which are added
together to form a drag force, are felt by everyone. Drag equation can be expressed
using a two-term sum.
𝐹𝐷 = ∫ (−𝑝𝐶𝑜𝑠𝜃 + ΓSinθ)dA (𝑖𝑣)
By separating the integration
𝐹𝐷 = ∫ (−𝑝𝐶𝑜𝑠𝜃)dA + ∫(ΓSinθ)dA (𝑣𝑖𝑖)
The expression given in eq. (vii) is the equation for the drag force acting on a blade.
Expression For “Lift Force”
Circulation is a flow field characteristic that gives a measure of the average rate of
rotation of fluid particles located in an area bordered by a closed curve. The path
integral, as illustrated in the diagram below, defines the circulation. “V” can be
divided into tangent and normal to the path components along any differential
section of the path. " 𝑉𝐿" stands for the tangential component of velocity. The final
amount obtained by integrating the "𝑉𝐿𝑑𝐿” around the curve is "Γ”
Figure 52 Lift Force acting on a small particle.
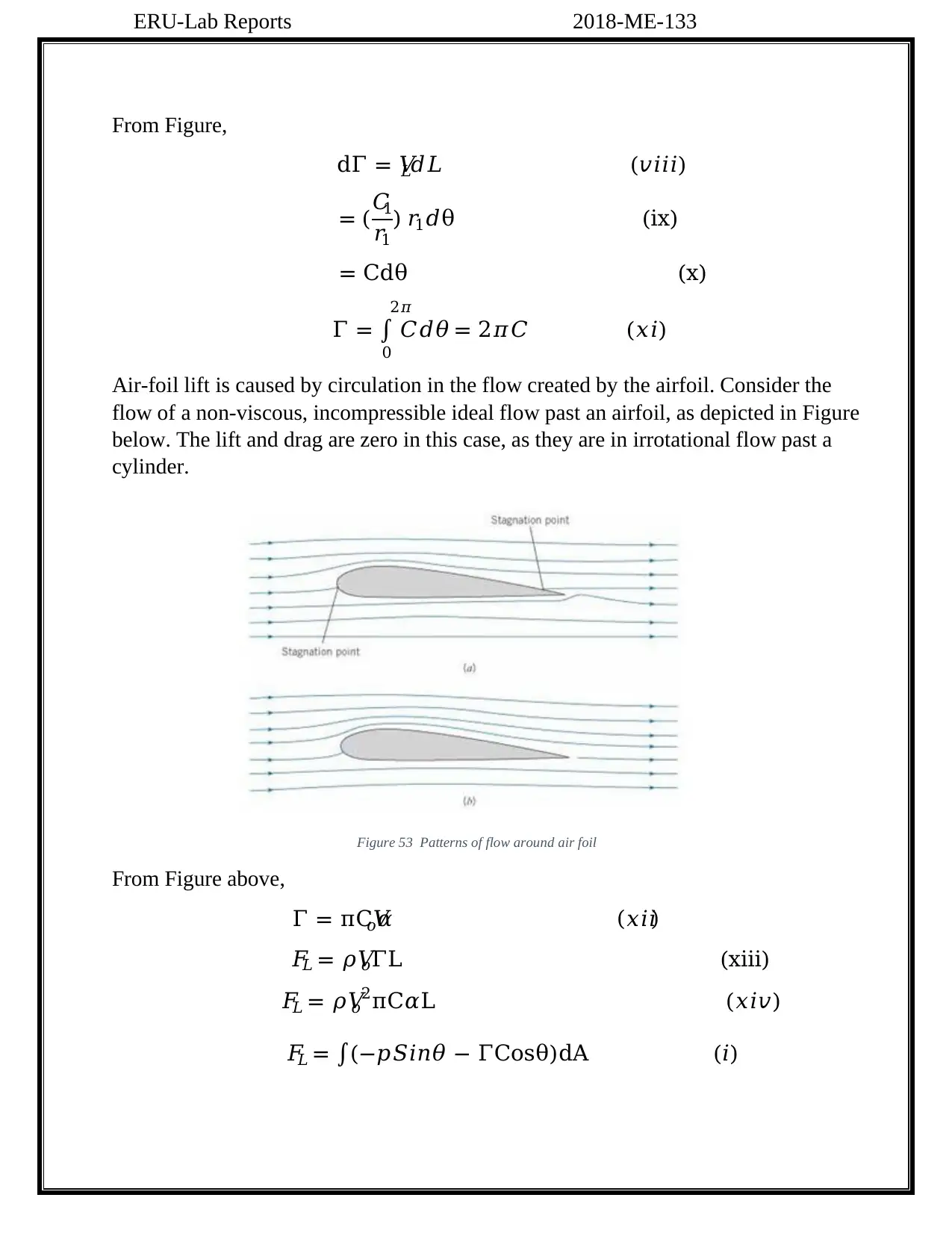
ERU-Lab Reports 2018-ME-133
From Figure,
dΓ = 𝑉𝐿𝑑𝐿 (𝑣𝑖𝑖𝑖)
= (𝐶1
𝑟1
) 𝑟1𝑑θ (ix)
= Cdθ (x)
Γ = ∫ 𝐶𝑑𝜃
2𝜋
0
= 2𝜋𝐶 (𝑥𝑖)
Air-foil lift is caused by circulation in the flow created by the airfoil. Consider the
flow of a non-viscous, incompressible ideal flow past an airfoil, as depicted in Figure
below. The lift and drag are zero in this case, as they are in irrotational flow past a
cylinder.
Figure 53 Patterns of flow around air foil
From Figure above,
Γ = πC𝑉𝑜𝛼 (𝑥𝑖𝑖)
𝐹𝐿 = 𝜌𝑉𝑜ΓL (xiii)
𝐹𝐿 = 𝜌𝑉𝑜2πC𝛼L (𝑥𝑖𝑣)
𝐹𝐿 = ∫(−𝑝𝑆𝑖𝑛𝜃 − ΓCosθ)dA (𝑖)
From Figure,
dΓ = 𝑉𝐿𝑑𝐿 (𝑣𝑖𝑖𝑖)
= (𝐶1
𝑟1
) 𝑟1𝑑θ (ix)
= Cdθ (x)
Γ = ∫ 𝐶𝑑𝜃
2𝜋
0
= 2𝜋𝐶 (𝑥𝑖)
Air-foil lift is caused by circulation in the flow created by the airfoil. Consider the
flow of a non-viscous, incompressible ideal flow past an airfoil, as depicted in Figure
below. The lift and drag are zero in this case, as they are in irrotational flow past a
cylinder.
Figure 53 Patterns of flow around air foil
From Figure above,
Γ = πC𝑉𝑜𝛼 (𝑥𝑖𝑖)
𝐹𝐿 = 𝜌𝑉𝑜ΓL (xiii)
𝐹𝐿 = 𝜌𝑉𝑜2πC𝛼L (𝑥𝑖𝑣)
𝐹𝐿 = ∫(−𝑝𝑆𝑖𝑛𝜃 − ΓCosθ)dA (𝑖)
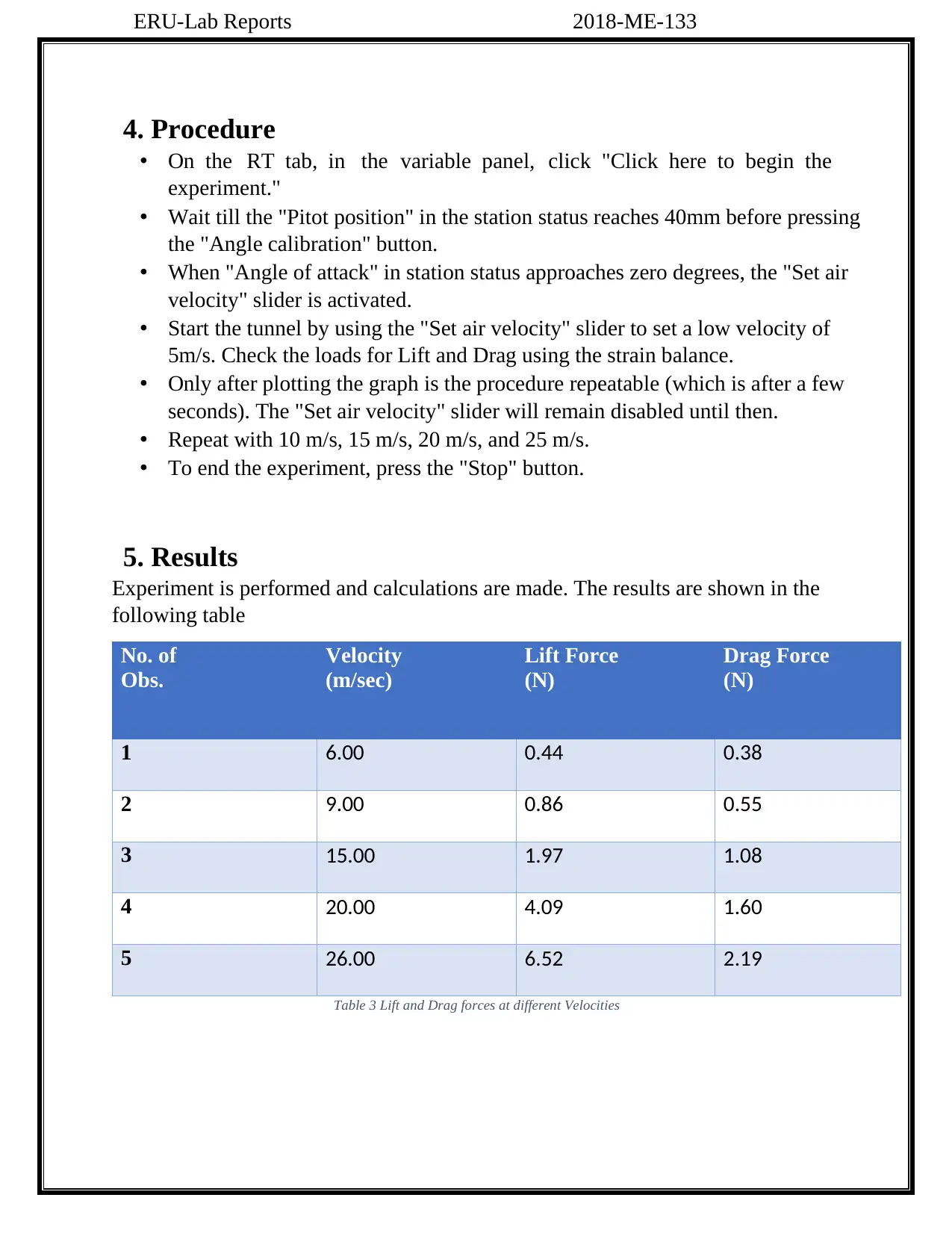
ERU-Lab Reports 2018-ME-133
4. Procedure
• On the RT tab, in the variable panel, click "Click here to begin the
experiment."
• Wait till the "Pitot position" in the station status reaches 40mm before pressing
the "Angle calibration" button.
• When "Angle of attack" in station status approaches zero degrees, the "Set air
velocity" slider is activated.
• Start the tunnel by using the "Set air velocity" slider to set a low velocity of
5m/s. Check the loads for Lift and Drag using the strain balance.
• Only after plotting the graph is the procedure repeatable (which is after a few
seconds). The "Set air velocity" slider will remain disabled until then.
• Repeat with 10 m/s, 15 m/s, 20 m/s, and 25 m/s.
• To end the experiment, press the "Stop" button.
5. Results
Experiment is performed and calculations are made. The results are shown in the
following table
No. of
Obs.
Velocity
(m/sec)
Lift Force
(N)
Drag Force
(N)
1 6.00 0.44 0.38
2 9.00 0.86 0.55
3 15.00 1.97 1.08
4 20.00 4.09 1.60
5 26.00 6.52 2.19
Table 3 Lift and Drag forces at different Velocities
4. Procedure
• On the RT tab, in the variable panel, click "Click here to begin the
experiment."
• Wait till the "Pitot position" in the station status reaches 40mm before pressing
the "Angle calibration" button.
• When "Angle of attack" in station status approaches zero degrees, the "Set air
velocity" slider is activated.
• Start the tunnel by using the "Set air velocity" slider to set a low velocity of
5m/s. Check the loads for Lift and Drag using the strain balance.
• Only after plotting the graph is the procedure repeatable (which is after a few
seconds). The "Set air velocity" slider will remain disabled until then.
• Repeat with 10 m/s, 15 m/s, 20 m/s, and 25 m/s.
• To end the experiment, press the "Stop" button.
5. Results
Experiment is performed and calculations are made. The results are shown in the
following table
No. of
Obs.
Velocity
(m/sec)
Lift Force
(N)
Drag Force
(N)
1 6.00 0.44 0.38
2 9.00 0.86 0.55
3 15.00 1.97 1.08
4 20.00 4.09 1.60
5 26.00 6.52 2.19
Table 3 Lift and Drag forces at different Velocities
Paraphrase This Document
Need a fresh take? Get an instant paraphrase of this document with our AI Paraphraser
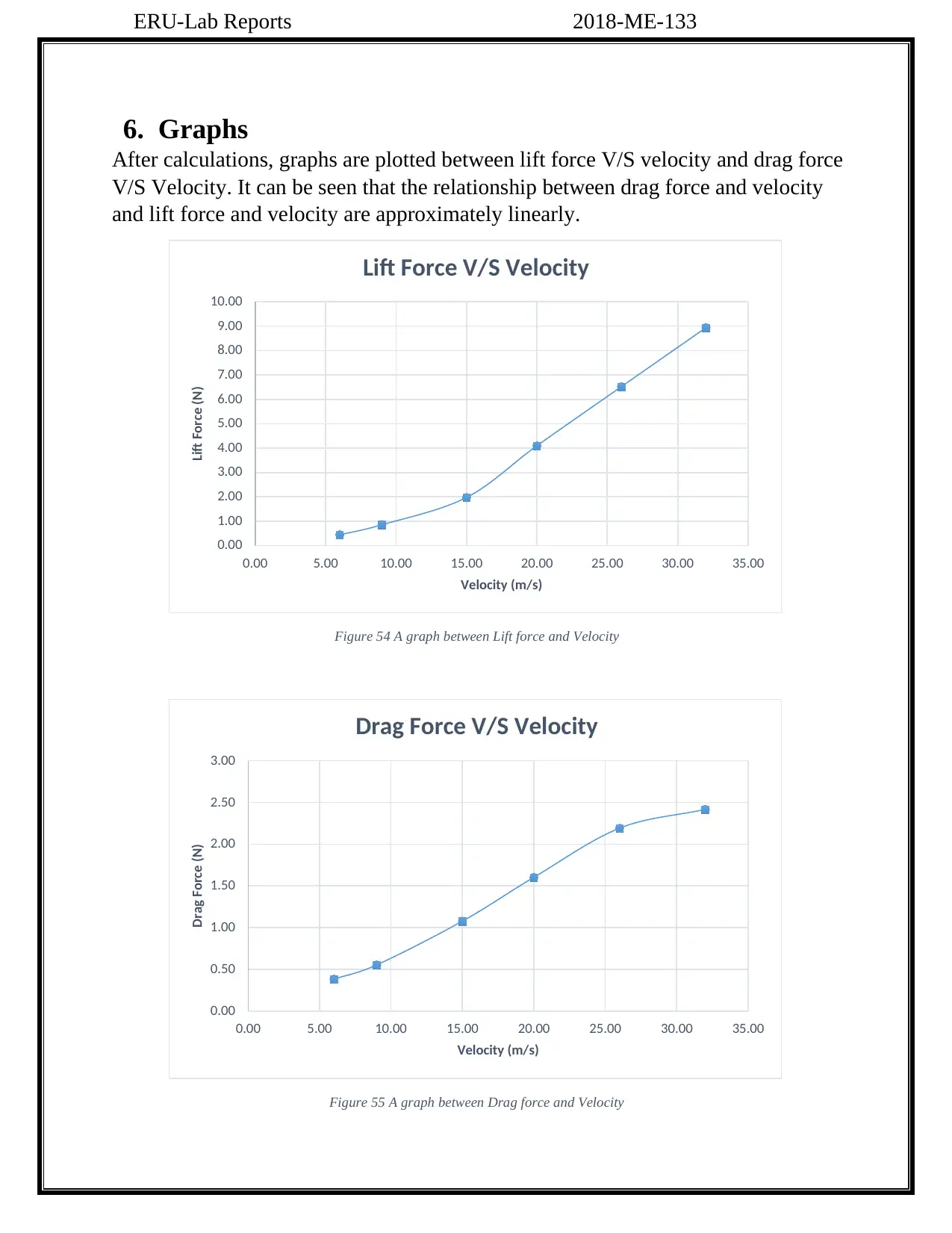
ERU-Lab Reports 2018-ME-133
6. Graphs
After calculations, graphs are plotted between lift force V/S velocity and drag force
V/S Velocity. It can be seen that the relationship between drag force and velocity
and lift force and velocity are approximately linearly.
Figure 54 A graph between Lift force and Velocity
Figure 55 A graph between Drag force and Velocity
0.00
1.00
2.00
3.00
4.00
5.00
6.00
7.00
8.00
9.00
10.00
0.00 5.00 10.00 15.00 20.00 25.00 30.00 35.00
Lift Force (N)
Velocity (m/s)
Lift Force V/S Velocity
0.00
0.50
1.00
1.50
2.00
2.50
3.00
0.00 5.00 10.00 15.00 20.00 25.00 30.00 35.00
Drag Force (N)
Velocity (m/s)
Drag Force V/S Velocity
6. Graphs
After calculations, graphs are plotted between lift force V/S velocity and drag force
V/S Velocity. It can be seen that the relationship between drag force and velocity
and lift force and velocity are approximately linearly.
Figure 54 A graph between Lift force and Velocity
Figure 55 A graph between Drag force and Velocity
0.00
1.00
2.00
3.00
4.00
5.00
6.00
7.00
8.00
9.00
10.00
0.00 5.00 10.00 15.00 20.00 25.00 30.00 35.00
Lift Force (N)
Velocity (m/s)
Lift Force V/S Velocity
0.00
0.50
1.00
1.50
2.00
2.50
3.00
0.00 5.00 10.00 15.00 20.00 25.00 30.00 35.00
Drag Force (N)
Velocity (m/s)
Drag Force V/S Velocity
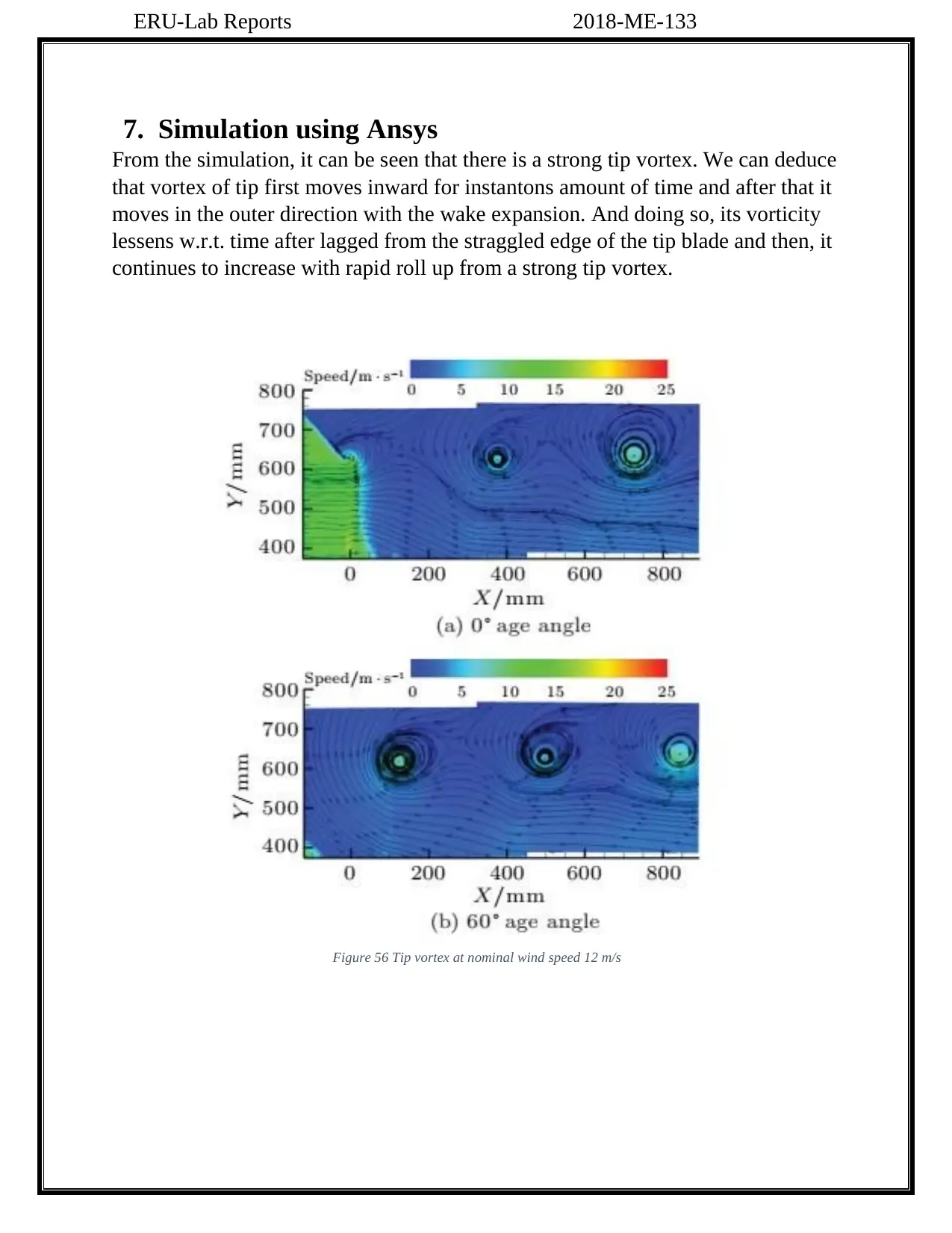
ERU-Lab Reports 2018-ME-133
7. Simulation using Ansys
From the simulation, it can be seen that there is a strong tip vortex. We can deduce
that vortex of tip first moves inward for instantons amount of time and after that it
moves in the outer direction with the wake expansion. And doing so, its vorticity
lessens w.r.t. time after lagged from the straggled edge of the tip blade and then, it
continues to increase with rapid roll up from a strong tip vortex.
Figure 56 Tip vortex at nominal wind speed 12 m/s
7. Simulation using Ansys
From the simulation, it can be seen that there is a strong tip vortex. We can deduce
that vortex of tip first moves inward for instantons amount of time and after that it
moves in the outer direction with the wake expansion. And doing so, its vorticity
lessens w.r.t. time after lagged from the straggled edge of the tip blade and then, it
continues to increase with rapid roll up from a strong tip vortex.
Figure 56 Tip vortex at nominal wind speed 12 m/s
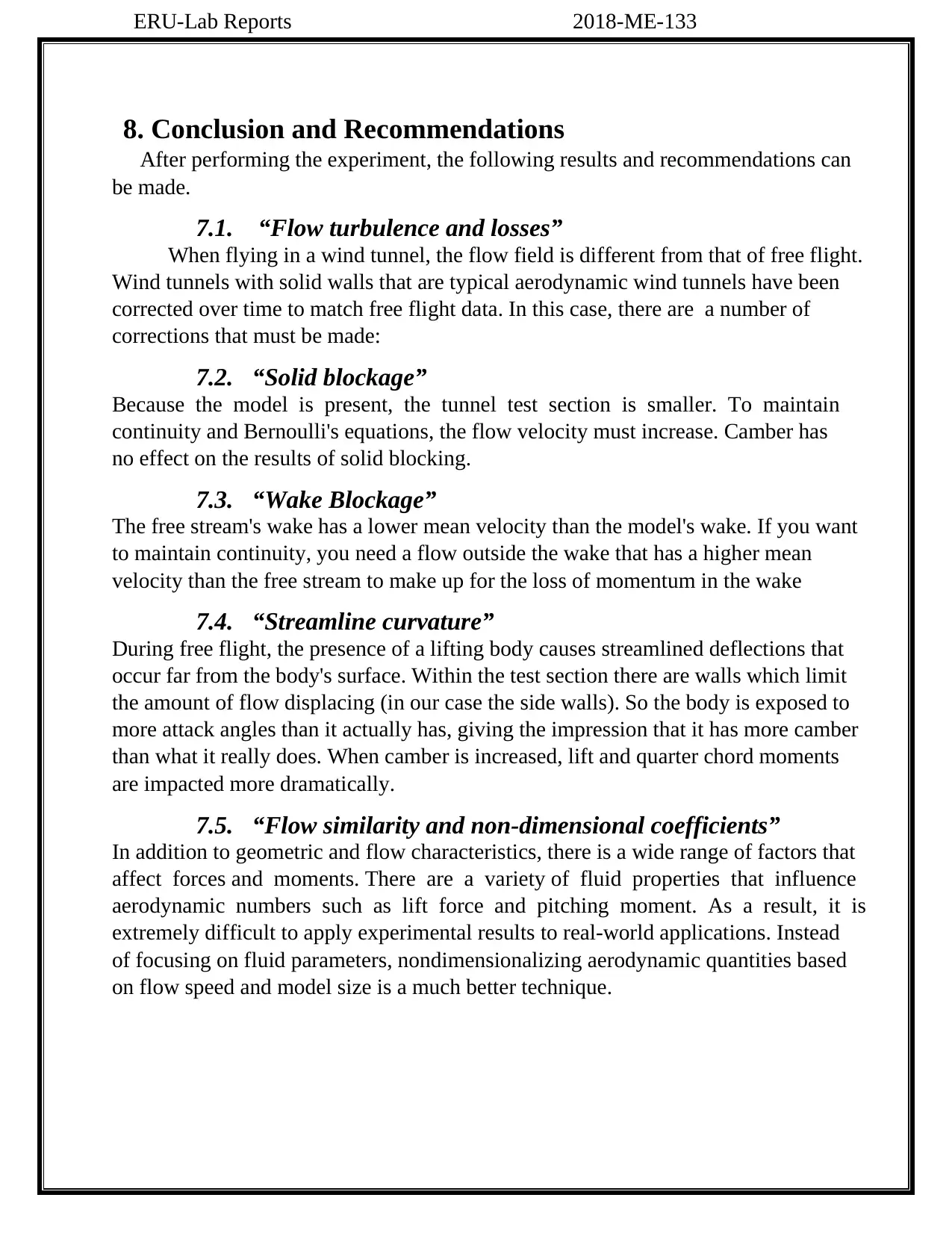
ERU-Lab Reports 2018-ME-133
8. Conclusion and Recommendations
After performing the experiment, the following results and recommendations can
be made.
7.1. “Flow turbulence and losses”
When flying in a wind tunnel, the flow field is different from that of free flight.
Wind tunnels with solid walls that are typical aerodynamic wind tunnels have been
corrected over time to match free flight data. In this case, there are a number of
corrections that must be made:
7.2. “Solid blockage”
Because the model is present, the tunnel test section is smaller. To maintain
continuity and Bernoulli's equations, the flow velocity must increase. Camber has
no effect on the results of solid blocking.
7.3. “Wake Blockage”
The free stream's wake has a lower mean velocity than the model's wake. If you want
to maintain continuity, you need a flow outside the wake that has a higher mean
velocity than the free stream to make up for the loss of momentum in the wake
7.4. “Streamline curvature”
During free flight, the presence of a lifting body causes streamlined deflections that
occur far from the body's surface. Within the test section there are walls which limit
the amount of flow displacing (in our case the side walls). So the body is exposed to
more attack angles than it actually has, giving the impression that it has more camber
than what it really does. When camber is increased, lift and quarter chord moments
are impacted more dramatically.
7.5. “Flow similarity and non-dimensional coefficients”
In addition to geometric and flow characteristics, there is a wide range of factors that
affect forces and moments. There are a variety of fluid properties that influence
aerodynamic numbers such as lift force and pitching moment. As a result, it is
extremely difficult to apply experimental results to real-world applications. Instead
of focusing on fluid parameters, nondimensionalizing aerodynamic quantities based
on flow speed and model size is a much better technique.
8. Conclusion and Recommendations
After performing the experiment, the following results and recommendations can
be made.
7.1. “Flow turbulence and losses”
When flying in a wind tunnel, the flow field is different from that of free flight.
Wind tunnels with solid walls that are typical aerodynamic wind tunnels have been
corrected over time to match free flight data. In this case, there are a number of
corrections that must be made:
7.2. “Solid blockage”
Because the model is present, the tunnel test section is smaller. To maintain
continuity and Bernoulli's equations, the flow velocity must increase. Camber has
no effect on the results of solid blocking.
7.3. “Wake Blockage”
The free stream's wake has a lower mean velocity than the model's wake. If you want
to maintain continuity, you need a flow outside the wake that has a higher mean
velocity than the free stream to make up for the loss of momentum in the wake
7.4. “Streamline curvature”
During free flight, the presence of a lifting body causes streamlined deflections that
occur far from the body's surface. Within the test section there are walls which limit
the amount of flow displacing (in our case the side walls). So the body is exposed to
more attack angles than it actually has, giving the impression that it has more camber
than what it really does. When camber is increased, lift and quarter chord moments
are impacted more dramatically.
7.5. “Flow similarity and non-dimensional coefficients”
In addition to geometric and flow characteristics, there is a wide range of factors that
affect forces and moments. There are a variety of fluid properties that influence
aerodynamic numbers such as lift force and pitching moment. As a result, it is
extremely difficult to apply experimental results to real-world applications. Instead
of focusing on fluid parameters, nondimensionalizing aerodynamic quantities based
on flow speed and model size is a much better technique.
Secure Best Marks with AI Grader
Need help grading? Try our AI Grader for instant feedback on your assignments.
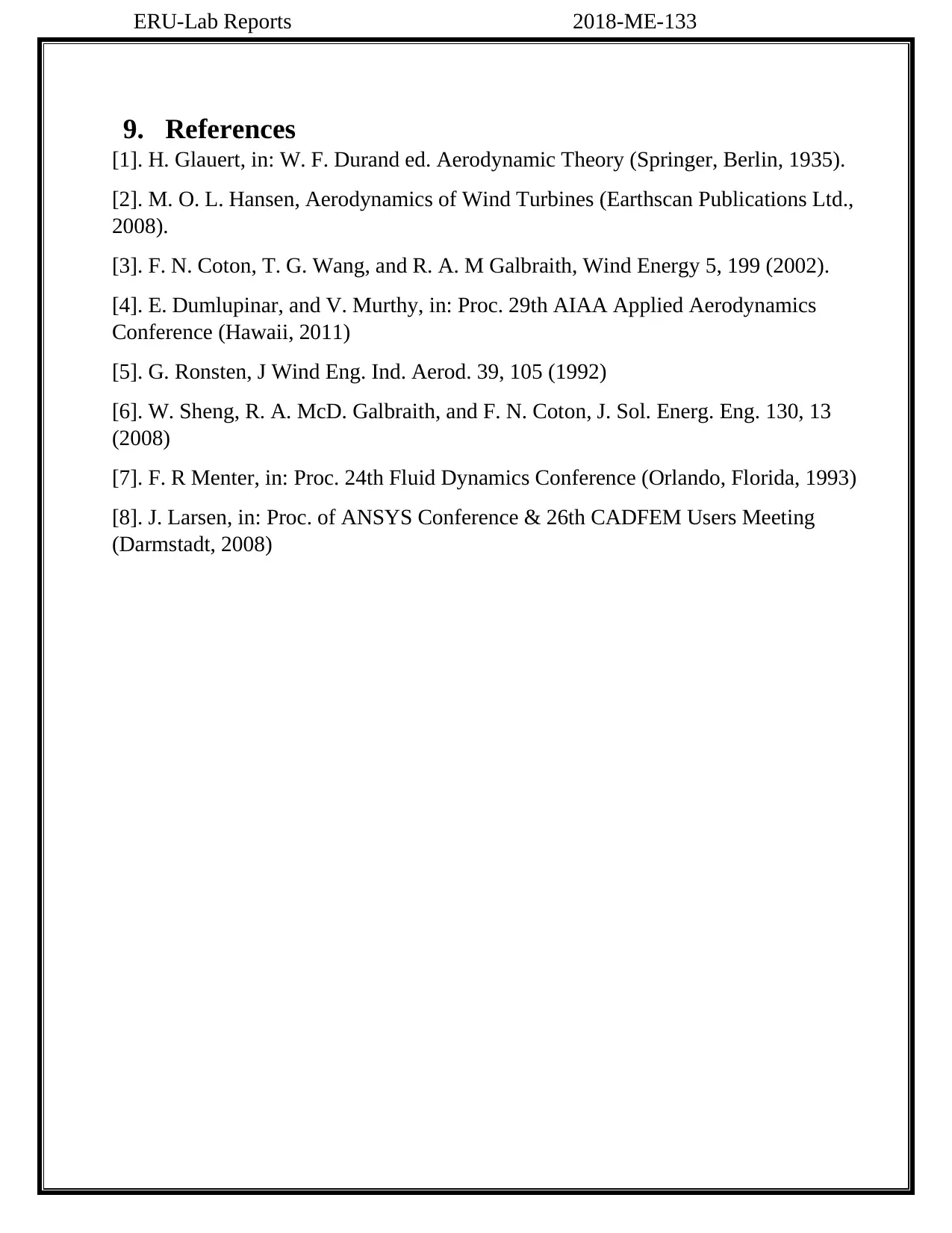
ERU-Lab Reports 2018-ME-133
9. References
[1]. H. Glauert, in: W. F. Durand ed. Aerodynamic Theory (Springer, Berlin, 1935).
[2]. M. O. L. Hansen, Aerodynamics of Wind Turbines (Earthscan Publications Ltd.,
2008).
[3]. F. N. Coton, T. G. Wang, and R. A. M Galbraith, Wind Energy 5, 199 (2002).
[4]. E. Dumlupinar, and V. Murthy, in: Proc. 29th AIAA Applied Aerodynamics
Conference (Hawaii, 2011)
[5]. G. Ronsten, J Wind Eng. Ind. Aerod. 39, 105 (1992)
[6]. W. Sheng, R. A. McD. Galbraith, and F. N. Coton, J. Sol. Energ. Eng. 130, 13
(2008)
[7]. F. R Menter, in: Proc. 24th Fluid Dynamics Conference (Orlando, Florida, 1993)
[8]. J. Larsen, in: Proc. of ANSYS Conference & 26th CADFEM Users Meeting
(Darmstadt, 2008)
9. References
[1]. H. Glauert, in: W. F. Durand ed. Aerodynamic Theory (Springer, Berlin, 1935).
[2]. M. O. L. Hansen, Aerodynamics of Wind Turbines (Earthscan Publications Ltd.,
2008).
[3]. F. N. Coton, T. G. Wang, and R. A. M Galbraith, Wind Energy 5, 199 (2002).
[4]. E. Dumlupinar, and V. Murthy, in: Proc. 29th AIAA Applied Aerodynamics
Conference (Hawaii, 2011)
[5]. G. Ronsten, J Wind Eng. Ind. Aerod. 39, 105 (1992)
[6]. W. Sheng, R. A. McD. Galbraith, and F. N. Coton, J. Sol. Energ. Eng. 130, 13
(2008)
[7]. F. R Menter, in: Proc. 24th Fluid Dynamics Conference (Orlando, Florida, 1993)
[8]. J. Larsen, in: Proc. of ANSYS Conference & 26th CADFEM Users Meeting
(Darmstadt, 2008)
1 out of 71
![[object Object]](/_next/image/?url=%2F_next%2Fstatic%2Fmedia%2Flogo.6d15ce61.png&w=640&q=75)
Your All-in-One AI-Powered Toolkit for Academic Success.
+13062052269
info@desklib.com
Available 24*7 on WhatsApp / Email
Unlock your academic potential
© 2024 | Zucol Services PVT LTD | All rights reserved.