Everything You Wanted to Know about Smart Healthcare
VerifiedAdded on 2023/03/23
|11
|7313
|61
AI Summary
This article discusses the importance, requirements and applications of smart healthcare along with the current industry trends and products. It gives a deeper insight about the different platforms across which more research can be pursued in this dynamic domain.
Contribute Materials
Your contribution can guide someone’s learning journey. Share your
documents today.
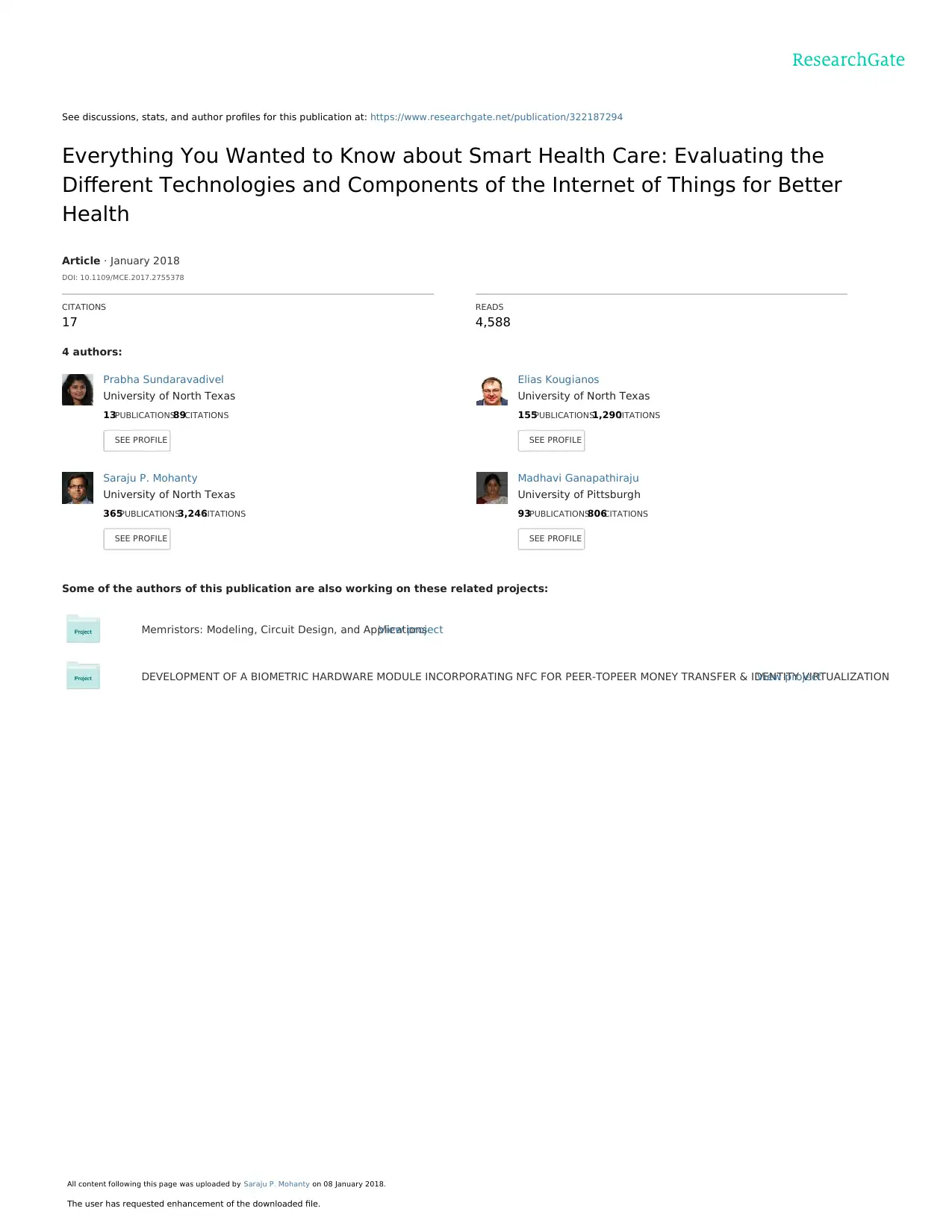
See discussions, stats, and author profiles for this publication at: https://www.researchgate.net/publication/322187294
Everything You Wanted to Know about Smart Health Care: Evaluating the
Different Technologies and Components of the Internet of Things for Better
Health
Article · January 2018
DOI: 10.1109/MCE.2017.2755378
CITATIONS
17
READS
4,588
4 authors:
Some of the authors of this publication are also working on these related projects:
Memristors: Modeling, Circuit Design, and ApplicationsView project
DEVELOPMENT OF A BIOMETRIC HARDWARE MODULE INCORPORATING NFC FOR PEER-TOPEER MONEY TRANSFER & IDENTITY VIRTUALIZATIONView project
Prabha Sundaravadivel
University of North Texas
13PUBLICATIONS89CITATIONS
SEE PROFILE
Elias Kougianos
University of North Texas
155PUBLICATIONS1,290CITATIONS
SEE PROFILE
Saraju P. Mohanty
University of North Texas
365PUBLICATIONS3,246CITATIONS
SEE PROFILE
Madhavi Ganapathiraju
University of Pittsburgh
93PUBLICATIONS806CITATIONS
SEE PROFILE
All content following this page was uploaded by Saraju P. Mohanty on 08 January 2018.
The user has requested enhancement of the downloaded file.
Everything You Wanted to Know about Smart Health Care: Evaluating the
Different Technologies and Components of the Internet of Things for Better
Health
Article · January 2018
DOI: 10.1109/MCE.2017.2755378
CITATIONS
17
READS
4,588
4 authors:
Some of the authors of this publication are also working on these related projects:
Memristors: Modeling, Circuit Design, and ApplicationsView project
DEVELOPMENT OF A BIOMETRIC HARDWARE MODULE INCORPORATING NFC FOR PEER-TOPEER MONEY TRANSFER & IDENTITY VIRTUALIZATIONView project
Prabha Sundaravadivel
University of North Texas
13PUBLICATIONS89CITATIONS
SEE PROFILE
Elias Kougianos
University of North Texas
155PUBLICATIONS1,290CITATIONS
SEE PROFILE
Saraju P. Mohanty
University of North Texas
365PUBLICATIONS3,246CITATIONS
SEE PROFILE
Madhavi Ganapathiraju
University of Pittsburgh
93PUBLICATIONS806CITATIONS
SEE PROFILE
All content following this page was uploaded by Saraju P. Mohanty on 08 January 2018.
The user has requested enhancement of the downloaded file.
Secure Best Marks with AI Grader
Need help grading? Try our AI Grader for instant feedback on your assignments.
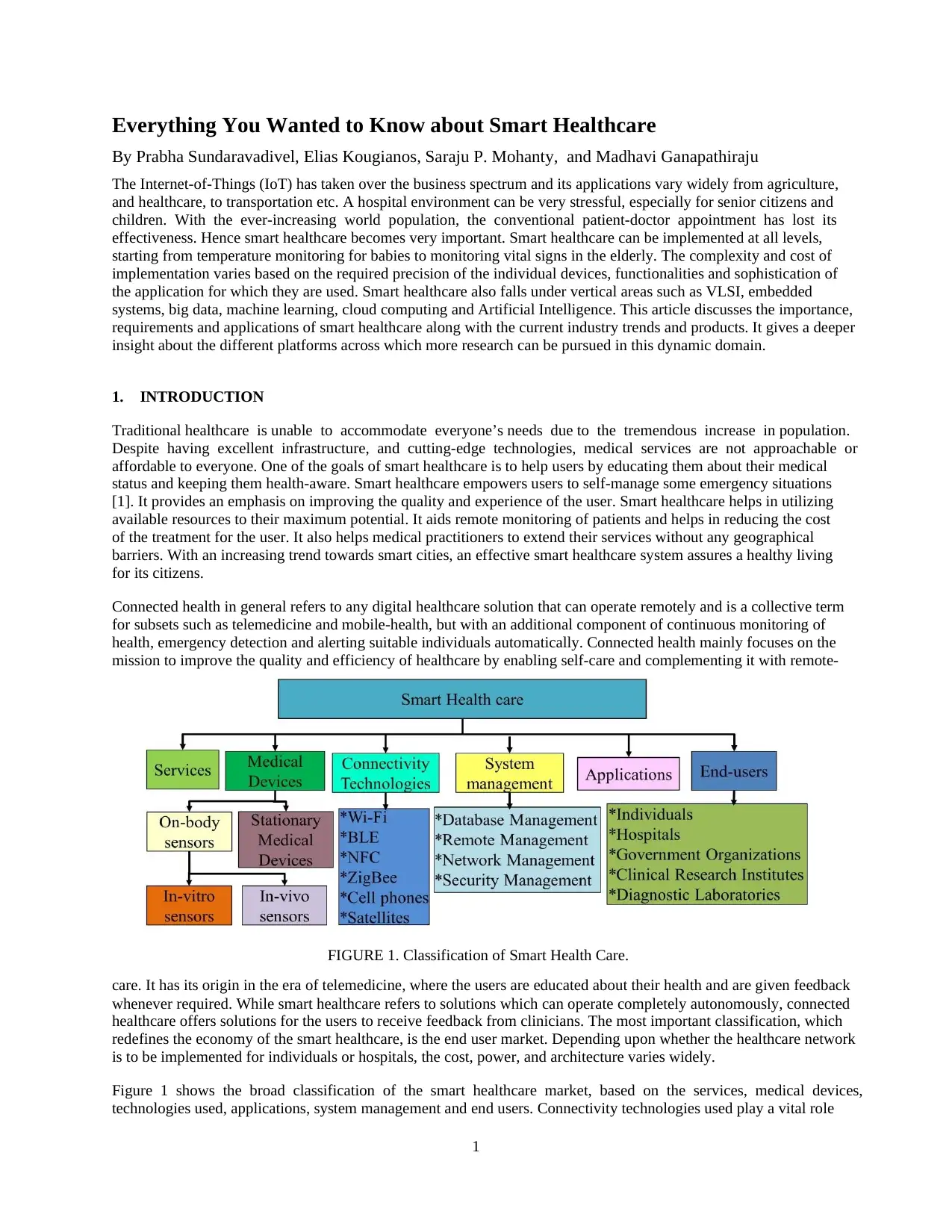
1
Everything You Wanted to Know about Smart Healthcare
By Prabha Sundaravadivel, Elias Kougianos, Saraju P. Mohanty, and Madhavi Ganapathiraju
The Internet-of-Things (IoT) has taken over the business spectrum and its applications vary widely from agriculture,
and healthcare, to transportation etc. A hospital environment can be very stressful, especially for senior citizens and
children. With the ever-increasing world population, the conventional patient-doctor appointment has lost its
effectiveness. Hence smart healthcare becomes very important. Smart healthcare can be implemented at all levels,
starting from temperature monitoring for babies to monitoring vital signs in the elderly. The complexity and cost of
implementation varies based on the required precision of the individual devices, functionalities and sophistication of
the application for which they are used. Smart healthcare also falls under vertical areas such as VLSI, embedded
systems, big data, machine learning, cloud computing and Artificial Intelligence. This article discusses the importance,
requirements and applications of smart healthcare along with the current industry trends and products. It gives a deeper
insight about the different platforms across which more research can be pursued in this dynamic domain.
1. INTRODUCTION
Traditional healthcare is unable to accommodate everyone’s needs due to the tremendous increase in population.
Despite having excellent infrastructure, and cutting-edge technologies, medical services are not approachable or
affordable to everyone. One of the goals of smart healthcare is to help users by educating them about their medical
status and keeping them health-aware. Smart healthcare empowers users to self-manage some emergency situations
[1]. It provides an emphasis on improving the quality and experience of the user. Smart healthcare helps in utilizing
available resources to their maximum potential. It aids remote monitoring of patients and helps in reducing the cost
of the treatment for the user. It also helps medical practitioners to extend their services without any geographical
barriers. With an increasing trend towards smart cities, an effective smart healthcare system assures a healthy living
for its citizens.
Connected health in general refers to any digital healthcare solution that can operate remotely and is a collective term
for subsets such as telemedicine and mobile-health, but with an additional component of continuous monitoring of
health, emergency detection and alerting suitable individuals automatically. Connected health mainly focuses on the
mission to improve the quality and efficiency of healthcare by enabling self-care and complementing it with remote-
care. It has its origin in the era of telemedicine, where the users are educated about their health and are given feedback
whenever required. While smart healthcare refers to solutions which can operate completely autonomously, connected
healthcare offers solutions for the users to receive feedback from clinicians. The most important classification, which
redefines the economy of the smart healthcare, is the end user market. Depending upon whether the healthcare network
is to be implemented for individuals or hospitals, the cost, power, and architecture varies widely.
Figure 1 shows the broad classification of the smart healthcare market, based on the services, medical devices,
technologies used, applications, system management and end users. Connectivity technologies used play a vital role
FIGURE 1. Classification of Smart Health Care.
Everything You Wanted to Know about Smart Healthcare
By Prabha Sundaravadivel, Elias Kougianos, Saraju P. Mohanty, and Madhavi Ganapathiraju
The Internet-of-Things (IoT) has taken over the business spectrum and its applications vary widely from agriculture,
and healthcare, to transportation etc. A hospital environment can be very stressful, especially for senior citizens and
children. With the ever-increasing world population, the conventional patient-doctor appointment has lost its
effectiveness. Hence smart healthcare becomes very important. Smart healthcare can be implemented at all levels,
starting from temperature monitoring for babies to monitoring vital signs in the elderly. The complexity and cost of
implementation varies based on the required precision of the individual devices, functionalities and sophistication of
the application for which they are used. Smart healthcare also falls under vertical areas such as VLSI, embedded
systems, big data, machine learning, cloud computing and Artificial Intelligence. This article discusses the importance,
requirements and applications of smart healthcare along with the current industry trends and products. It gives a deeper
insight about the different platforms across which more research can be pursued in this dynamic domain.
1. INTRODUCTION
Traditional healthcare is unable to accommodate everyone’s needs due to the tremendous increase in population.
Despite having excellent infrastructure, and cutting-edge technologies, medical services are not approachable or
affordable to everyone. One of the goals of smart healthcare is to help users by educating them about their medical
status and keeping them health-aware. Smart healthcare empowers users to self-manage some emergency situations
[1]. It provides an emphasis on improving the quality and experience of the user. Smart healthcare helps in utilizing
available resources to their maximum potential. It aids remote monitoring of patients and helps in reducing the cost
of the treatment for the user. It also helps medical practitioners to extend their services without any geographical
barriers. With an increasing trend towards smart cities, an effective smart healthcare system assures a healthy living
for its citizens.
Connected health in general refers to any digital healthcare solution that can operate remotely and is a collective term
for subsets such as telemedicine and mobile-health, but with an additional component of continuous monitoring of
health, emergency detection and alerting suitable individuals automatically. Connected health mainly focuses on the
mission to improve the quality and efficiency of healthcare by enabling self-care and complementing it with remote-
care. It has its origin in the era of telemedicine, where the users are educated about their health and are given feedback
whenever required. While smart healthcare refers to solutions which can operate completely autonomously, connected
healthcare offers solutions for the users to receive feedback from clinicians. The most important classification, which
redefines the economy of the smart healthcare, is the end user market. Depending upon whether the healthcare network
is to be implemented for individuals or hospitals, the cost, power, and architecture varies widely.
Figure 1 shows the broad classification of the smart healthcare market, based on the services, medical devices,
technologies used, applications, system management and end users. Connectivity technologies used play a vital role
FIGURE 1. Classification of Smart Health Care.
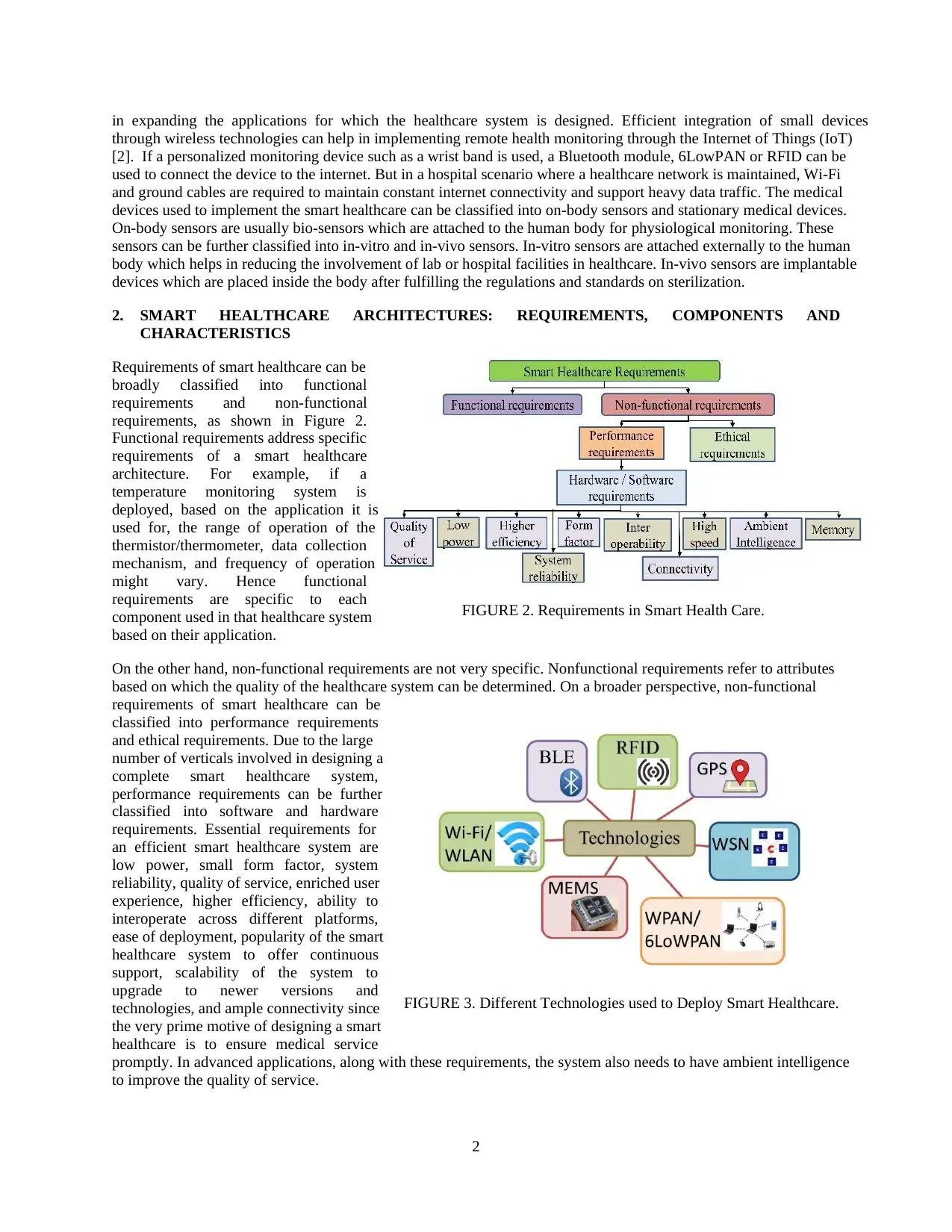
2
in expanding the applications for which the healthcare system is designed. Efficient integration of small devices
through wireless technologies can help in implementing remote health monitoring through the Internet of Things (IoT)
[2]. If a personalized monitoring device such as a wrist band is used, a Bluetooth module, 6LowPAN or RFID can be
used to connect the device to the internet. But in a hospital scenario where a healthcare network is maintained, Wi-Fi
and ground cables are required to maintain constant internet connectivity and support heavy data traffic. The medical
devices used to implement the smart healthcare can be classified into on-body sensors and stationary medical devices.
On-body sensors are usually bio-sensors which are attached to the human body for physiological monitoring. These
sensors can be further classified into in-vitro and in-vivo sensors. In-vitro sensors are attached externally to the human
body which helps in reducing the involvement of lab or hospital facilities in healthcare. In-vivo sensors are implantable
devices which are placed inside the body after fulfilling the regulations and standards on sterilization.
2. SMART HEALTHCARE ARCHITECTURES: REQUIREMENTS, COMPONENTS AND
CHARACTERISTICS
Requirements of smart healthcare can be
broadly classified into functional
requirements and non-functional
requirements, as shown in Figure 2.
Functional requirements address specific
requirements of a smart healthcare
architecture. For example, if a
temperature monitoring system is
deployed, based on the application it is
used for, the range of operation of the
thermistor/thermometer, data collection
mechanism, and frequency of operation
might vary. Hence functional
requirements are specific to each
component used in that healthcare system
based on their application.
On the other hand, non-functional requirements are not very specific. Nonfunctional requirements refer to attributes
based on which the quality of the healthcare system can be determined. On a broader perspective, non-functional
requirements of smart healthcare can be
classified into performance requirements
and ethical requirements. Due to the large
number of verticals involved in designing a
complete smart healthcare system,
performance requirements can be further
classified into software and hardware
requirements. Essential requirements for
an efficient smart healthcare system are
low power, small form factor, system
reliability, quality of service, enriched user
experience, higher efficiency, ability to
interoperate across different platforms,
ease of deployment, popularity of the smart
healthcare system to offer continuous
support, scalability of the system to
upgrade to newer versions and
technologies, and ample connectivity since
the very prime motive of designing a smart
healthcare is to ensure medical service
promptly. In advanced applications, along with these requirements, the system also needs to have ambient intelligence
to improve the quality of service.
FIGURE 2. Requirements in Smart Health Care.
FIGURE 3. Different Technologies used to Deploy Smart Healthcare.
in expanding the applications for which the healthcare system is designed. Efficient integration of small devices
through wireless technologies can help in implementing remote health monitoring through the Internet of Things (IoT)
[2]. If a personalized monitoring device such as a wrist band is used, a Bluetooth module, 6LowPAN or RFID can be
used to connect the device to the internet. But in a hospital scenario where a healthcare network is maintained, Wi-Fi
and ground cables are required to maintain constant internet connectivity and support heavy data traffic. The medical
devices used to implement the smart healthcare can be classified into on-body sensors and stationary medical devices.
On-body sensors are usually bio-sensors which are attached to the human body for physiological monitoring. These
sensors can be further classified into in-vitro and in-vivo sensors. In-vitro sensors are attached externally to the human
body which helps in reducing the involvement of lab or hospital facilities in healthcare. In-vivo sensors are implantable
devices which are placed inside the body after fulfilling the regulations and standards on sterilization.
2. SMART HEALTHCARE ARCHITECTURES: REQUIREMENTS, COMPONENTS AND
CHARACTERISTICS
Requirements of smart healthcare can be
broadly classified into functional
requirements and non-functional
requirements, as shown in Figure 2.
Functional requirements address specific
requirements of a smart healthcare
architecture. For example, if a
temperature monitoring system is
deployed, based on the application it is
used for, the range of operation of the
thermistor/thermometer, data collection
mechanism, and frequency of operation
might vary. Hence functional
requirements are specific to each
component used in that healthcare system
based on their application.
On the other hand, non-functional requirements are not very specific. Nonfunctional requirements refer to attributes
based on which the quality of the healthcare system can be determined. On a broader perspective, non-functional
requirements of smart healthcare can be
classified into performance requirements
and ethical requirements. Due to the large
number of verticals involved in designing a
complete smart healthcare system,
performance requirements can be further
classified into software and hardware
requirements. Essential requirements for
an efficient smart healthcare system are
low power, small form factor, system
reliability, quality of service, enriched user
experience, higher efficiency, ability to
interoperate across different platforms,
ease of deployment, popularity of the smart
healthcare system to offer continuous
support, scalability of the system to
upgrade to newer versions and
technologies, and ample connectivity since
the very prime motive of designing a smart
healthcare is to ensure medical service
promptly. In advanced applications, along with these requirements, the system also needs to have ambient intelligence
to improve the quality of service.
FIGURE 2. Requirements in Smart Health Care.
FIGURE 3. Different Technologies used to Deploy Smart Healthcare.
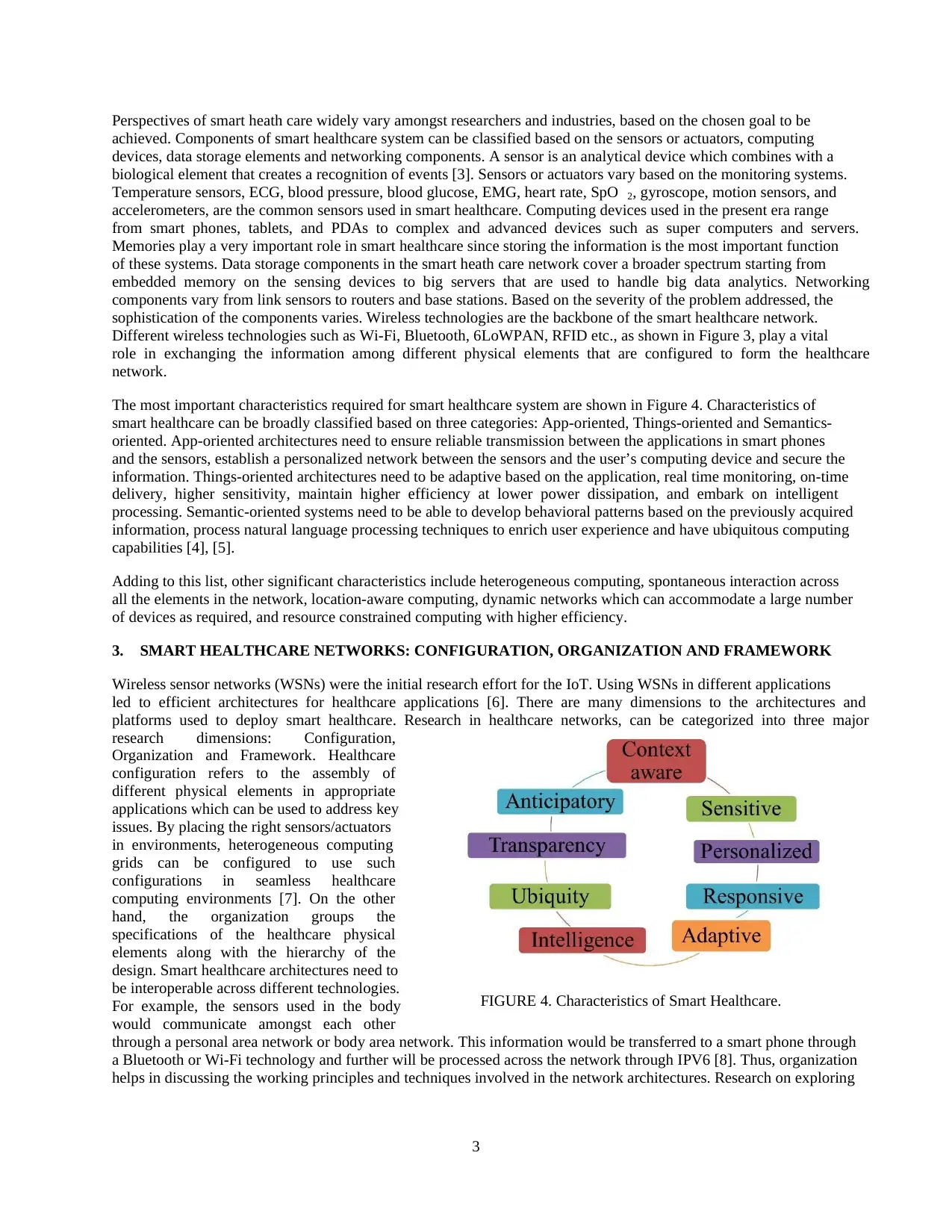
3
Perspectives of smart heath care widely vary amongst researchers and industries, based on the chosen goal to be
achieved. Components of smart healthcare system can be classified based on the sensors or actuators, computing
devices, data storage elements and networking components. A sensor is an analytical device which combines with a
biological element that creates a recognition of events [3]. Sensors or actuators vary based on the monitoring systems.
Temperature sensors, ECG, blood pressure, blood glucose, EMG, heart rate, SpO 2, gyroscope, motion sensors, and
accelerometers, are the common sensors used in smart healthcare. Computing devices used in the present era range
from smart phones, tablets, and PDAs to complex and advanced devices such as super computers and servers.
Memories play a very important role in smart healthcare since storing the information is the most important function
of these systems. Data storage components in the smart heath care network cover a broader spectrum starting from
embedded memory on the sensing devices to big servers that are used to handle big data analytics. Networking
components vary from link sensors to routers and base stations. Based on the severity of the problem addressed, the
sophistication of the components varies. Wireless technologies are the backbone of the smart healthcare network.
Different wireless technologies such as Wi-Fi, Bluetooth, 6LoWPAN, RFID etc., as shown in Figure 3, play a vital
role in exchanging the information among different physical elements that are configured to form the healthcare
network.
The most important characteristics required for smart healthcare system are shown in Figure 4. Characteristics of
smart healthcare can be broadly classified based on three categories: App-oriented, Things-oriented and Semantics-
oriented. App-oriented architectures need to ensure reliable transmission between the applications in smart phones
and the sensors, establish a personalized network between the sensors and the user’s computing device and secure the
information. Things-oriented architectures need to be adaptive based on the application, real time monitoring, on-time
delivery, higher sensitivity, maintain higher efficiency at lower power dissipation, and embark on intelligent
processing. Semantic-oriented systems need to be able to develop behavioral patterns based on the previously acquired
information, process natural language processing techniques to enrich user experience and have ubiquitous computing
capabilities [4], [5].
Adding to this list, other significant characteristics include heterogeneous computing, spontaneous interaction across
all the elements in the network, location-aware computing, dynamic networks which can accommodate a large number
of devices as required, and resource constrained computing with higher efficiency.
3. SMART HEALTHCARE NETWORKS: CONFIGURATION, ORGANIZATION AND FRAMEWORK
Wireless sensor networks (WSNs) were the initial research effort for the IoT. Using WSNs in different applications
led to efficient architectures for healthcare applications [6]. There are many dimensions to the architectures and
platforms used to deploy smart healthcare. Research in healthcare networks, can be categorized into three major
research dimensions: Configuration,
Organization and Framework. Healthcare
configuration refers to the assembly of
different physical elements in appropriate
applications which can be used to address key
issues. By placing the right sensors/actuators
in environments, heterogeneous computing
grids can be configured to use such
configurations in seamless healthcare
computing environments [7]. On the other
hand, the organization groups the
specifications of the healthcare physical
elements along with the hierarchy of the
design. Smart healthcare architectures need to
be interoperable across different technologies.
For example, the sensors used in the body
would communicate amongst each other
through a personal area network or body area network. This information would be transferred to a smart phone through
a Bluetooth or Wi-Fi technology and further will be processed across the network through IPV6 [8]. Thus, organization
helps in discussing the working principles and techniques involved in the network architectures. Research on exploring
FIGURE 4. Characteristics of Smart Healthcare.
Perspectives of smart heath care widely vary amongst researchers and industries, based on the chosen goal to be
achieved. Components of smart healthcare system can be classified based on the sensors or actuators, computing
devices, data storage elements and networking components. A sensor is an analytical device which combines with a
biological element that creates a recognition of events [3]. Sensors or actuators vary based on the monitoring systems.
Temperature sensors, ECG, blood pressure, blood glucose, EMG, heart rate, SpO 2, gyroscope, motion sensors, and
accelerometers, are the common sensors used in smart healthcare. Computing devices used in the present era range
from smart phones, tablets, and PDAs to complex and advanced devices such as super computers and servers.
Memories play a very important role in smart healthcare since storing the information is the most important function
of these systems. Data storage components in the smart heath care network cover a broader spectrum starting from
embedded memory on the sensing devices to big servers that are used to handle big data analytics. Networking
components vary from link sensors to routers and base stations. Based on the severity of the problem addressed, the
sophistication of the components varies. Wireless technologies are the backbone of the smart healthcare network.
Different wireless technologies such as Wi-Fi, Bluetooth, 6LoWPAN, RFID etc., as shown in Figure 3, play a vital
role in exchanging the information among different physical elements that are configured to form the healthcare
network.
The most important characteristics required for smart healthcare system are shown in Figure 4. Characteristics of
smart healthcare can be broadly classified based on three categories: App-oriented, Things-oriented and Semantics-
oriented. App-oriented architectures need to ensure reliable transmission between the applications in smart phones
and the sensors, establish a personalized network between the sensors and the user’s computing device and secure the
information. Things-oriented architectures need to be adaptive based on the application, real time monitoring, on-time
delivery, higher sensitivity, maintain higher efficiency at lower power dissipation, and embark on intelligent
processing. Semantic-oriented systems need to be able to develop behavioral patterns based on the previously acquired
information, process natural language processing techniques to enrich user experience and have ubiquitous computing
capabilities [4], [5].
Adding to this list, other significant characteristics include heterogeneous computing, spontaneous interaction across
all the elements in the network, location-aware computing, dynamic networks which can accommodate a large number
of devices as required, and resource constrained computing with higher efficiency.
3. SMART HEALTHCARE NETWORKS: CONFIGURATION, ORGANIZATION AND FRAMEWORK
Wireless sensor networks (WSNs) were the initial research effort for the IoT. Using WSNs in different applications
led to efficient architectures for healthcare applications [6]. There are many dimensions to the architectures and
platforms used to deploy smart healthcare. Research in healthcare networks, can be categorized into three major
research dimensions: Configuration,
Organization and Framework. Healthcare
configuration refers to the assembly of
different physical elements in appropriate
applications which can be used to address key
issues. By placing the right sensors/actuators
in environments, heterogeneous computing
grids can be configured to use such
configurations in seamless healthcare
computing environments [7]. On the other
hand, the organization groups the
specifications of the healthcare physical
elements along with the hierarchy of the
design. Smart healthcare architectures need to
be interoperable across different technologies.
For example, the sensors used in the body
would communicate amongst each other
through a personal area network or body area network. This information would be transferred to a smart phone through
a Bluetooth or Wi-Fi technology and further will be processed across the network through IPV6 [8]. Thus, organization
helps in discussing the working principles and techniques involved in the network architectures. Research on exploring
FIGURE 4. Characteristics of Smart Healthcare.
Secure Best Marks with AI Grader
Need help grading? Try our AI Grader for instant feedback on your assignments.
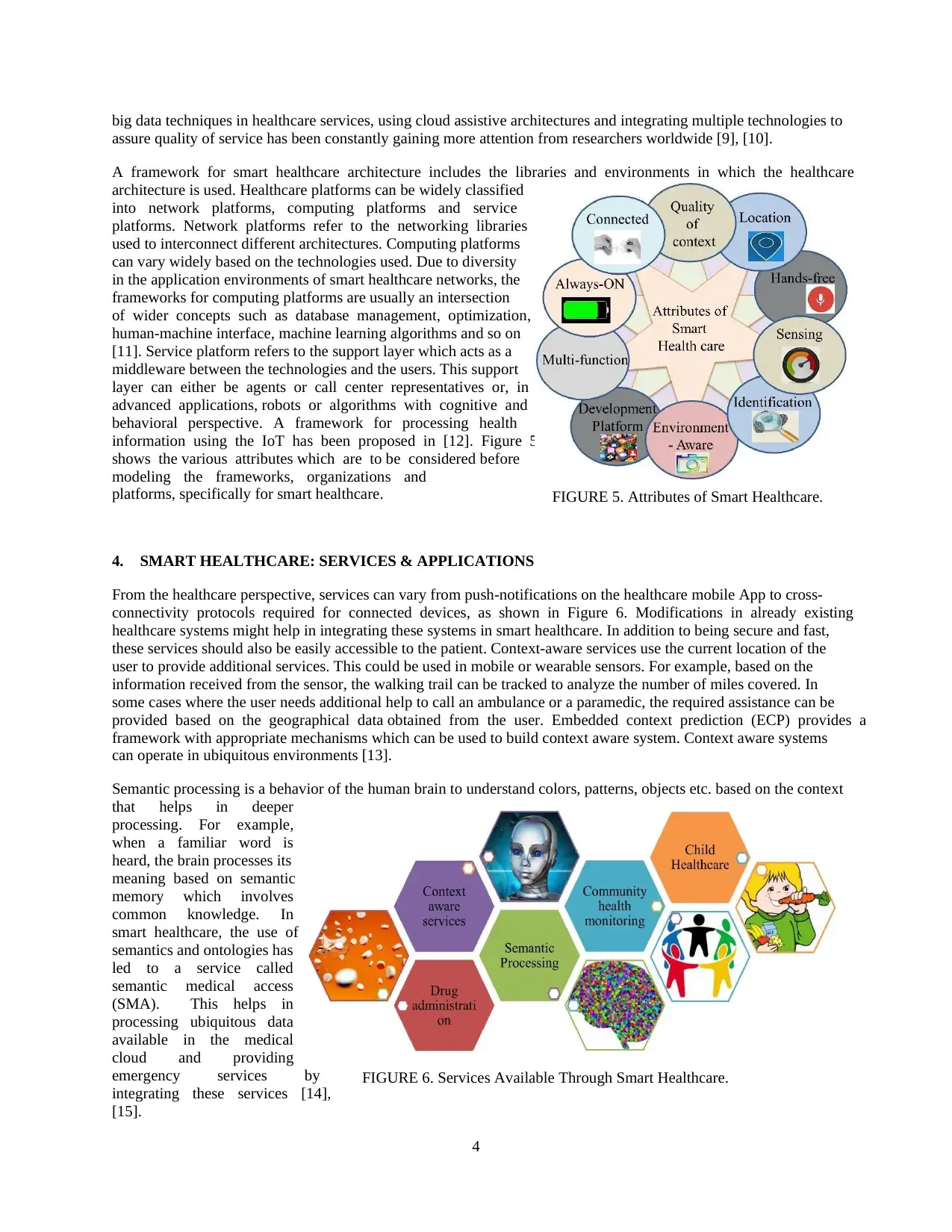
4
big data techniques in healthcare services, using cloud assistive architectures and integrating multiple technologies to
assure quality of service has been constantly gaining more attention from researchers worldwide [9], [10].
A framework for smart healthcare architecture includes the libraries and environments in which the healthcare
architecture is used. Healthcare platforms can be widely classified
into network platforms, computing platforms and service
platforms. Network platforms refer to the networking libraries
used to interconnect different architectures. Computing platforms
can vary widely based on the technologies used. Due to diversity
in the application environments of smart healthcare networks, the
frameworks for computing platforms are usually an intersection
of wider concepts such as database management, optimization,
human-machine interface, machine learning algorithms and so on
[11]. Service platform refers to the support layer which acts as a
middleware between the technologies and the users. This support
layer can either be agents or call center representatives or, in
advanced applications, robots or algorithms with cognitive and
behavioral perspective. A framework for processing health
information using the IoT has been proposed in [12]. Figure 5
shows the various attributes which are to be considered before
modeling the frameworks, organizations and
platforms, specifically for smart healthcare.
4. SMART HEALTHCARE: SERVICES & APPLICATIONS
From the healthcare perspective, services can vary from push-notifications on the healthcare mobile App to cross-
connectivity protocols required for connected devices, as shown in Figure 6. Modifications in already existing
healthcare systems might help in integrating these systems in smart healthcare. In addition to being secure and fast,
these services should also be easily accessible to the patient. Context-aware services use the current location of the
user to provide additional services. This could be used in mobile or wearable sensors. For example, based on the
information received from the sensor, the walking trail can be tracked to analyze the number of miles covered. In
some cases where the user needs additional help to call an ambulance or a paramedic, the required assistance can be
provided based on the geographical data obtained from the user. Embedded context prediction (ECP) provides a
framework with appropriate mechanisms which can be used to build context aware system. Context aware systems
can operate in ubiquitous environments [13].
Semantic processing is a behavior of the human brain to understand colors, patterns, objects etc. based on the context
that helps in deeper
processing. For example,
when a familiar word is
heard, the brain processes its
meaning based on semantic
memory which involves
common knowledge. In
smart healthcare, the use of
semantics and ontologies has
led to a service called
semantic medical access
(SMA). This helps in
processing ubiquitous data
available in the medical
cloud and providing
emergency services by
integrating these services [14],
[15].
FIGURE 5. Attributes of Smart Healthcare.
FIGURE 6. Services Available Through Smart Healthcare.
big data techniques in healthcare services, using cloud assistive architectures and integrating multiple technologies to
assure quality of service has been constantly gaining more attention from researchers worldwide [9], [10].
A framework for smart healthcare architecture includes the libraries and environments in which the healthcare
architecture is used. Healthcare platforms can be widely classified
into network platforms, computing platforms and service
platforms. Network platforms refer to the networking libraries
used to interconnect different architectures. Computing platforms
can vary widely based on the technologies used. Due to diversity
in the application environments of smart healthcare networks, the
frameworks for computing platforms are usually an intersection
of wider concepts such as database management, optimization,
human-machine interface, machine learning algorithms and so on
[11]. Service platform refers to the support layer which acts as a
middleware between the technologies and the users. This support
layer can either be agents or call center representatives or, in
advanced applications, robots or algorithms with cognitive and
behavioral perspective. A framework for processing health
information using the IoT has been proposed in [12]. Figure 5
shows the various attributes which are to be considered before
modeling the frameworks, organizations and
platforms, specifically for smart healthcare.
4. SMART HEALTHCARE: SERVICES & APPLICATIONS
From the healthcare perspective, services can vary from push-notifications on the healthcare mobile App to cross-
connectivity protocols required for connected devices, as shown in Figure 6. Modifications in already existing
healthcare systems might help in integrating these systems in smart healthcare. In addition to being secure and fast,
these services should also be easily accessible to the patient. Context-aware services use the current location of the
user to provide additional services. This could be used in mobile or wearable sensors. For example, based on the
information received from the sensor, the walking trail can be tracked to analyze the number of miles covered. In
some cases where the user needs additional help to call an ambulance or a paramedic, the required assistance can be
provided based on the geographical data obtained from the user. Embedded context prediction (ECP) provides a
framework with appropriate mechanisms which can be used to build context aware system. Context aware systems
can operate in ubiquitous environments [13].
Semantic processing is a behavior of the human brain to understand colors, patterns, objects etc. based on the context
that helps in deeper
processing. For example,
when a familiar word is
heard, the brain processes its
meaning based on semantic
memory which involves
common knowledge. In
smart healthcare, the use of
semantics and ontologies has
led to a service called
semantic medical access
(SMA). This helps in
processing ubiquitous data
available in the medical
cloud and providing
emergency services by
integrating these services [14],
[15].
FIGURE 5. Attributes of Smart Healthcare.
FIGURE 6. Services Available Through Smart Healthcare.
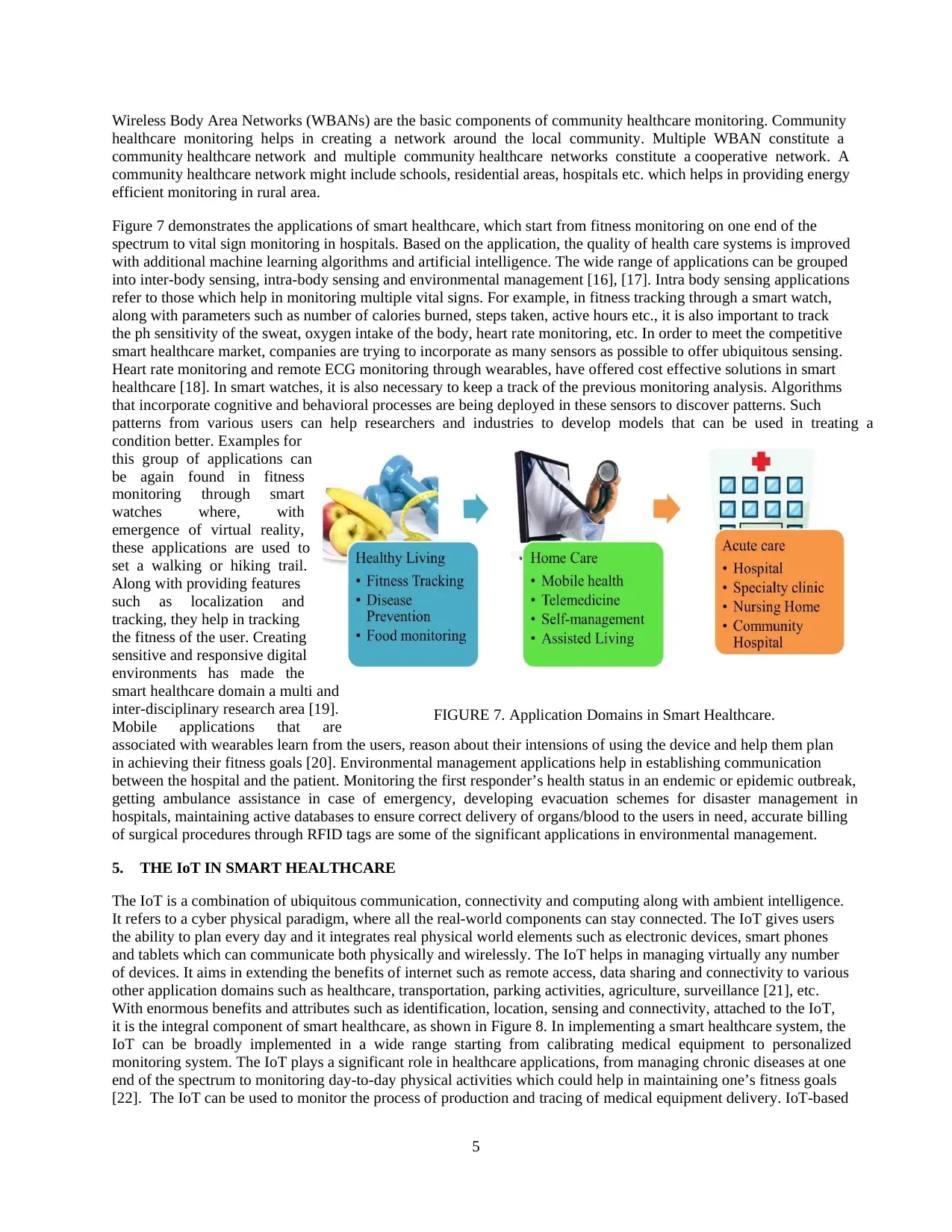
5
Wireless Body Area Networks (WBANs) are the basic components of community healthcare monitoring. Community
healthcare monitoring helps in creating a network around the local community. Multiple WBAN constitute a
community healthcare network and multiple community healthcare networks constitute a cooperative network. A
community healthcare network might include schools, residential areas, hospitals etc. which helps in providing energy
efficient monitoring in rural area.
Figure 7 demonstrates the applications of smart healthcare, which start from fitness monitoring on one end of the
spectrum to vital sign monitoring in hospitals. Based on the application, the quality of health care systems is improved
with additional machine learning algorithms and artificial intelligence. The wide range of applications can be grouped
into inter-body sensing, intra-body sensing and environmental management [16], [17]. Intra body sensing applications
refer to those which help in monitoring multiple vital signs. For example, in fitness tracking through a smart watch,
along with parameters such as number of calories burned, steps taken, active hours etc., it is also important to track
the ph sensitivity of the sweat, oxygen intake of the body, heart rate monitoring, etc. In order to meet the competitive
smart healthcare market, companies are trying to incorporate as many sensors as possible to offer ubiquitous sensing.
Heart rate monitoring and remote ECG monitoring through wearables, have offered cost effective solutions in smart
healthcare [18]. In smart watches, it is also necessary to keep a track of the previous monitoring analysis. Algorithms
that incorporate cognitive and behavioral processes are being deployed in these sensors to discover patterns. Such
patterns from various users can help researchers and industries to develop models that can be used in treating a
condition better. Examples for
this group of applications can
be again found in fitness
monitoring through smart
watches where, with
emergence of virtual reality,
these applications are used to
set a walking or hiking trail.
Along with providing features
such as localization and
tracking, they help in tracking
the fitness of the user. Creating
sensitive and responsive digital
environments has made the
smart healthcare domain a multi and
inter-disciplinary research area [19].
Mobile applications that are
associated with wearables learn from the users, reason about their intensions of using the device and help them plan
in achieving their fitness goals [20]. Environmental management applications help in establishing communication
between the hospital and the patient. Monitoring the first responder’s health status in an endemic or epidemic outbreak,
getting ambulance assistance in case of emergency, developing evacuation schemes for disaster management in
hospitals, maintaining active databases to ensure correct delivery of organs/blood to the users in need, accurate billing
of surgical procedures through RFID tags are some of the significant applications in environmental management.
5. THE IoT IN SMART HEALTHCARE
The IoT is a combination of ubiquitous communication, connectivity and computing along with ambient intelligence.
It refers to a cyber physical paradigm, where all the real-world components can stay connected. The IoT gives users
the ability to plan every day and it integrates real physical world elements such as electronic devices, smart phones
and tablets which can communicate both physically and wirelessly. The IoT helps in managing virtually any number
of devices. It aims in extending the benefits of internet such as remote access, data sharing and connectivity to various
other application domains such as healthcare, transportation, parking activities, agriculture, surveillance [21], etc.
With enormous benefits and attributes such as identification, location, sensing and connectivity, attached to the IoT,
it is the integral component of smart healthcare, as shown in Figure 8. In implementing a smart healthcare system, the
IoT can be broadly implemented in a wide range starting from calibrating medical equipment to personalized
monitoring system. The IoT plays a significant role in healthcare applications, from managing chronic diseases at one
end of the spectrum to monitoring day-to-day physical activities which could help in maintaining one’s fitness goals
[22]. The IoT can be used to monitor the process of production and tracing of medical equipment delivery. IoT-based
FIGURE 7. Application Domains in Smart Healthcare.
Wireless Body Area Networks (WBANs) are the basic components of community healthcare monitoring. Community
healthcare monitoring helps in creating a network around the local community. Multiple WBAN constitute a
community healthcare network and multiple community healthcare networks constitute a cooperative network. A
community healthcare network might include schools, residential areas, hospitals etc. which helps in providing energy
efficient monitoring in rural area.
Figure 7 demonstrates the applications of smart healthcare, which start from fitness monitoring on one end of the
spectrum to vital sign monitoring in hospitals. Based on the application, the quality of health care systems is improved
with additional machine learning algorithms and artificial intelligence. The wide range of applications can be grouped
into inter-body sensing, intra-body sensing and environmental management [16], [17]. Intra body sensing applications
refer to those which help in monitoring multiple vital signs. For example, in fitness tracking through a smart watch,
along with parameters such as number of calories burned, steps taken, active hours etc., it is also important to track
the ph sensitivity of the sweat, oxygen intake of the body, heart rate monitoring, etc. In order to meet the competitive
smart healthcare market, companies are trying to incorporate as many sensors as possible to offer ubiquitous sensing.
Heart rate monitoring and remote ECG monitoring through wearables, have offered cost effective solutions in smart
healthcare [18]. In smart watches, it is also necessary to keep a track of the previous monitoring analysis. Algorithms
that incorporate cognitive and behavioral processes are being deployed in these sensors to discover patterns. Such
patterns from various users can help researchers and industries to develop models that can be used in treating a
condition better. Examples for
this group of applications can
be again found in fitness
monitoring through smart
watches where, with
emergence of virtual reality,
these applications are used to
set a walking or hiking trail.
Along with providing features
such as localization and
tracking, they help in tracking
the fitness of the user. Creating
sensitive and responsive digital
environments has made the
smart healthcare domain a multi and
inter-disciplinary research area [19].
Mobile applications that are
associated with wearables learn from the users, reason about their intensions of using the device and help them plan
in achieving their fitness goals [20]. Environmental management applications help in establishing communication
between the hospital and the patient. Monitoring the first responder’s health status in an endemic or epidemic outbreak,
getting ambulance assistance in case of emergency, developing evacuation schemes for disaster management in
hospitals, maintaining active databases to ensure correct delivery of organs/blood to the users in need, accurate billing
of surgical procedures through RFID tags are some of the significant applications in environmental management.
5. THE IoT IN SMART HEALTHCARE
The IoT is a combination of ubiquitous communication, connectivity and computing along with ambient intelligence.
It refers to a cyber physical paradigm, where all the real-world components can stay connected. The IoT gives users
the ability to plan every day and it integrates real physical world elements such as electronic devices, smart phones
and tablets which can communicate both physically and wirelessly. The IoT helps in managing virtually any number
of devices. It aims in extending the benefits of internet such as remote access, data sharing and connectivity to various
other application domains such as healthcare, transportation, parking activities, agriculture, surveillance [21], etc.
With enormous benefits and attributes such as identification, location, sensing and connectivity, attached to the IoT,
it is the integral component of smart healthcare, as shown in Figure 8. In implementing a smart healthcare system, the
IoT can be broadly implemented in a wide range starting from calibrating medical equipment to personalized
monitoring system. The IoT plays a significant role in healthcare applications, from managing chronic diseases at one
end of the spectrum to monitoring day-to-day physical activities which could help in maintaining one’s fitness goals
[22]. The IoT can be used to monitor the process of production and tracing of medical equipment delivery. IoT-based
FIGURE 7. Application Domains in Smart Healthcare.
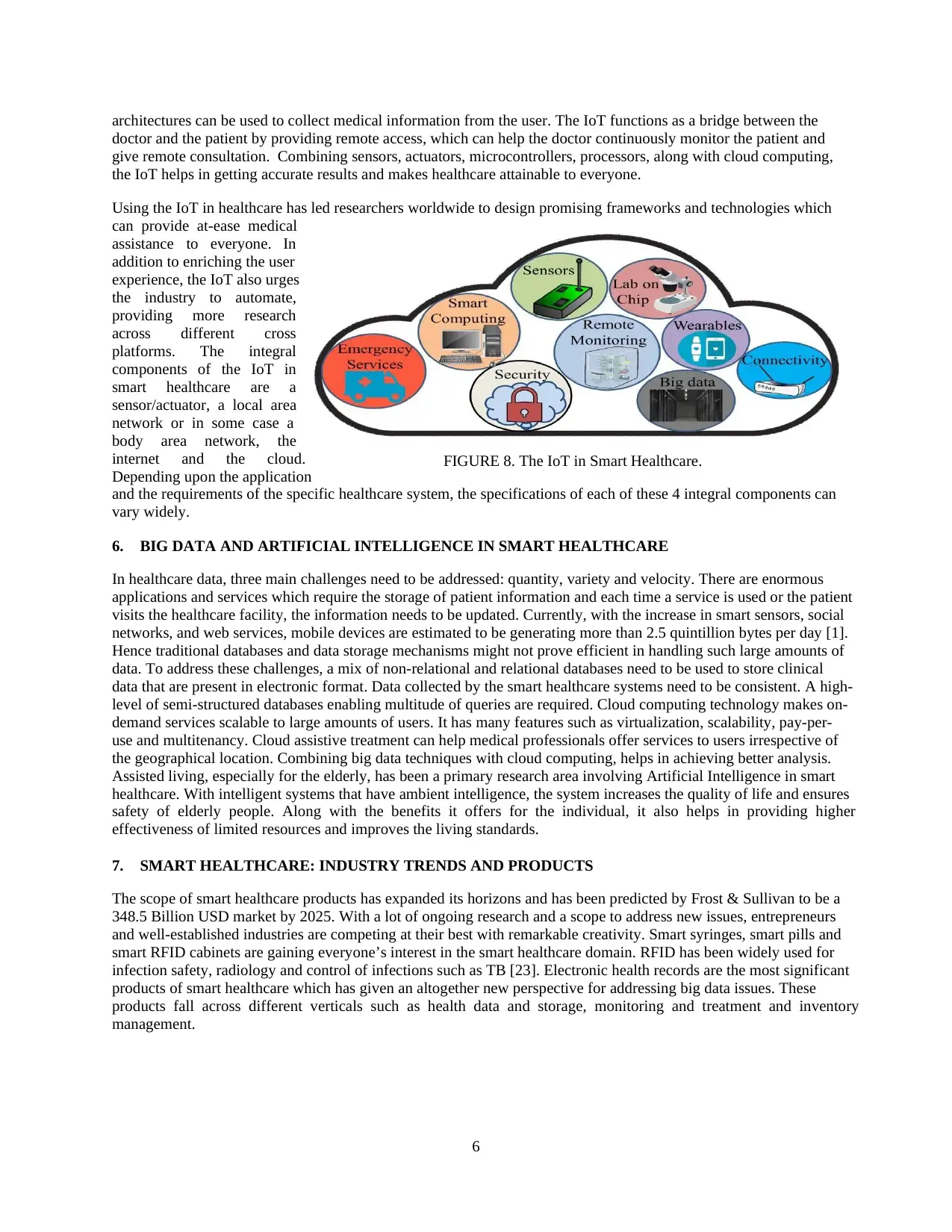
6
architectures can be used to collect medical information from the user. The IoT functions as a bridge between the
doctor and the patient by providing remote access, which can help the doctor continuously monitor the patient and
give remote consultation. Combining sensors, actuators, microcontrollers, processors, along with cloud computing,
the IoT helps in getting accurate results and makes healthcare attainable to everyone.
Using the IoT in healthcare has led researchers worldwide to design promising frameworks and technologies which
can provide at-ease medical
assistance to everyone. In
addition to enriching the user
experience, the IoT also urges
the industry to automate,
providing more research
across different cross
platforms. The integral
components of the IoT in
smart healthcare are a
sensor/actuator, a local area
network or in some case a
body area network, the
internet and the cloud.
Depending upon the application
and the requirements of the specific healthcare system, the specifications of each of these 4 integral components can
vary widely.
6. BIG DATA AND ARTIFICIAL INTELLIGENCE IN SMART HEALTHCARE
In healthcare data, three main challenges need to be addressed: quantity, variety and velocity. There are enormous
applications and services which require the storage of patient information and each time a service is used or the patient
visits the healthcare facility, the information needs to be updated. Currently, with the increase in smart sensors, social
networks, and web services, mobile devices are estimated to be generating more than 2.5 quintillion bytes per day [1].
Hence traditional databases and data storage mechanisms might not prove efficient in handling such large amounts of
data. To address these challenges, a mix of non-relational and relational databases need to be used to store clinical
data that are present in electronic format. Data collected by the smart healthcare systems need to be consistent. A high-
level of semi-structured databases enabling multitude of queries are required. Cloud computing technology makes on-
demand services scalable to large amounts of users. It has many features such as virtualization, scalability, pay-per-
use and multitenancy. Cloud assistive treatment can help medical professionals offer services to users irrespective of
the geographical location. Combining big data techniques with cloud computing, helps in achieving better analysis.
Assisted living, especially for the elderly, has been a primary research area involving Artificial Intelligence in smart
healthcare. With intelligent systems that have ambient intelligence, the system increases the quality of life and ensures
safety of elderly people. Along with the benefits it offers for the individual, it also helps in providing higher
effectiveness of limited resources and improves the living standards.
7. SMART HEALTHCARE: INDUSTRY TRENDS AND PRODUCTS
The scope of smart healthcare products has expanded its horizons and has been predicted by Frost & Sullivan to be a
348.5 Billion USD market by 2025. With a lot of ongoing research and a scope to address new issues, entrepreneurs
and well-established industries are competing at their best with remarkable creativity. Smart syringes, smart pills and
smart RFID cabinets are gaining everyone’s interest in the smart healthcare domain. RFID has been widely used for
infection safety, radiology and control of infections such as TB [23]. Electronic health records are the most significant
products of smart healthcare which has given an altogether new perspective for addressing big data issues. These
products fall across different verticals such as health data and storage, monitoring and treatment and inventory
management.
FIGURE 8. The IoT in Smart Healthcare.
architectures can be used to collect medical information from the user. The IoT functions as a bridge between the
doctor and the patient by providing remote access, which can help the doctor continuously monitor the patient and
give remote consultation. Combining sensors, actuators, microcontrollers, processors, along with cloud computing,
the IoT helps in getting accurate results and makes healthcare attainable to everyone.
Using the IoT in healthcare has led researchers worldwide to design promising frameworks and technologies which
can provide at-ease medical
assistance to everyone. In
addition to enriching the user
experience, the IoT also urges
the industry to automate,
providing more research
across different cross
platforms. The integral
components of the IoT in
smart healthcare are a
sensor/actuator, a local area
network or in some case a
body area network, the
internet and the cloud.
Depending upon the application
and the requirements of the specific healthcare system, the specifications of each of these 4 integral components can
vary widely.
6. BIG DATA AND ARTIFICIAL INTELLIGENCE IN SMART HEALTHCARE
In healthcare data, three main challenges need to be addressed: quantity, variety and velocity. There are enormous
applications and services which require the storage of patient information and each time a service is used or the patient
visits the healthcare facility, the information needs to be updated. Currently, with the increase in smart sensors, social
networks, and web services, mobile devices are estimated to be generating more than 2.5 quintillion bytes per day [1].
Hence traditional databases and data storage mechanisms might not prove efficient in handling such large amounts of
data. To address these challenges, a mix of non-relational and relational databases need to be used to store clinical
data that are present in electronic format. Data collected by the smart healthcare systems need to be consistent. A high-
level of semi-structured databases enabling multitude of queries are required. Cloud computing technology makes on-
demand services scalable to large amounts of users. It has many features such as virtualization, scalability, pay-per-
use and multitenancy. Cloud assistive treatment can help medical professionals offer services to users irrespective of
the geographical location. Combining big data techniques with cloud computing, helps in achieving better analysis.
Assisted living, especially for the elderly, has been a primary research area involving Artificial Intelligence in smart
healthcare. With intelligent systems that have ambient intelligence, the system increases the quality of life and ensures
safety of elderly people. Along with the benefits it offers for the individual, it also helps in providing higher
effectiveness of limited resources and improves the living standards.
7. SMART HEALTHCARE: INDUSTRY TRENDS AND PRODUCTS
The scope of smart healthcare products has expanded its horizons and has been predicted by Frost & Sullivan to be a
348.5 Billion USD market by 2025. With a lot of ongoing research and a scope to address new issues, entrepreneurs
and well-established industries are competing at their best with remarkable creativity. Smart syringes, smart pills and
smart RFID cabinets are gaining everyone’s interest in the smart healthcare domain. RFID has been widely used for
infection safety, radiology and control of infections such as TB [23]. Electronic health records are the most significant
products of smart healthcare which has given an altogether new perspective for addressing big data issues. These
products fall across different verticals such as health data and storage, monitoring and treatment and inventory
management.
FIGURE 8. The IoT in Smart Healthcare.
Paraphrase This Document
Need a fresh take? Get an instant paraphrase of this document with our AI Paraphraser
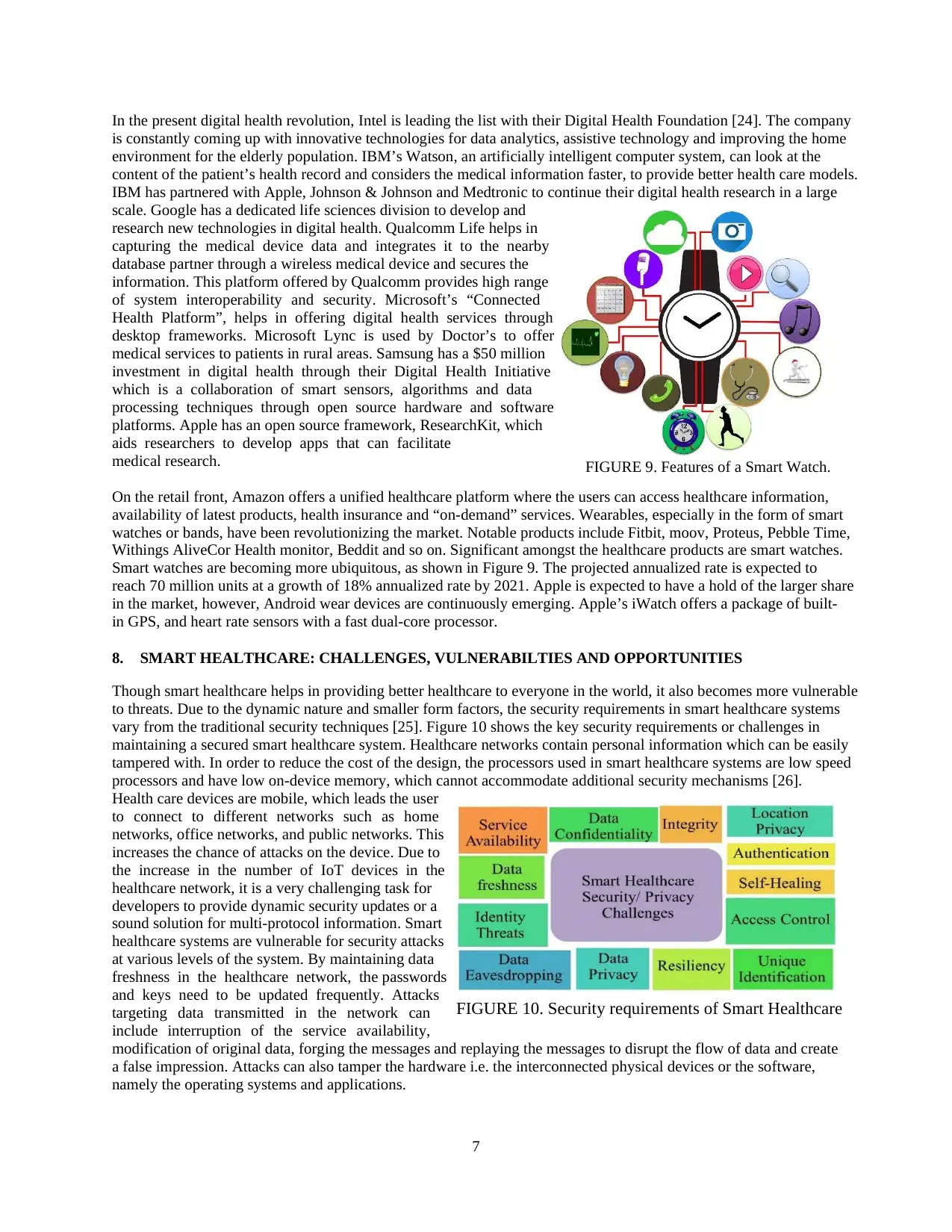
7
In the present digital health revolution, Intel is leading the list with their Digital Health Foundation [24]. The company
is constantly coming up with innovative technologies for data analytics, assistive technology and improving the home
environment for the elderly population. IBM’s Watson, an artificially intelligent computer system, can look at the
content of the patient’s health record and considers the medical information faster, to provide better health care models.
IBM has partnered with Apple, Johnson & Johnson and Medtronic to continue their digital health research in a large
scale. Google has a dedicated life sciences division to develop and
research new technologies in digital health. Qualcomm Life helps in
capturing the medical device data and integrates it to the nearby
database partner through a wireless medical device and secures the
information. This platform offered by Qualcomm provides high range
of system interoperability and security. Microsoft’s “Connected
Health Platform”, helps in offering digital health services through
desktop frameworks. Microsoft Lync is used by Doctor’s to offer
medical services to patients in rural areas. Samsung has a $50 million
investment in digital health through their Digital Health Initiative
which is a collaboration of smart sensors, algorithms and data
processing techniques through open source hardware and software
platforms. Apple has an open source framework, ResearchKit, which
aids researchers to develop apps that can facilitate
medical research.
On the retail front, Amazon offers a unified healthcare platform where the users can access healthcare information,
availability of latest products, health insurance and “on-demand” services. Wearables, especially in the form of smart
watches or bands, have been revolutionizing the market. Notable products include Fitbit, moov, Proteus, Pebble Time,
Withings AliveCor Health monitor, Beddit and so on. Significant amongst the healthcare products are smart watches.
Smart watches are becoming more ubiquitous, as shown in Figure 9. The projected annualized rate is expected to
reach 70 million units at a growth of 18% annualized rate by 2021. Apple is expected to have a hold of the larger share
in the market, however, Android wear devices are continuously emerging. Apple’s iWatch offers a package of built-
in GPS, and heart rate sensors with a fast dual-core processor.
8. SMART HEALTHCARE: CHALLENGES, VULNERABILTIES AND OPPORTUNITIES
Though smart healthcare helps in providing better healthcare to everyone in the world, it also becomes more vulnerable
to threats. Due to the dynamic nature and smaller form factors, the security requirements in smart healthcare systems
vary from the traditional security techniques [25]. Figure 10 shows the key security requirements or challenges in
maintaining a secured smart healthcare system. Healthcare networks contain personal information which can be easily
tampered with. In order to reduce the cost of the design, the processors used in smart healthcare systems are low speed
processors and have low on-device memory, which cannot accommodate additional security mechanisms [26].
Health care devices are mobile, which leads the user
to connect to different networks such as home
networks, office networks, and public networks. This
increases the chance of attacks on the device. Due to
the increase in the number of IoT devices in the
healthcare network, it is a very challenging task for
developers to provide dynamic security updates or a
sound solution for multi-protocol information. Smart
healthcare systems are vulnerable for security attacks
at various levels of the system. By maintaining data
freshness in the healthcare network, the passwords
and keys need to be updated frequently. Attacks
targeting data transmitted in the network can
include interruption of the service availability,
modification of original data, forging the messages and replaying the messages to disrupt the flow of data and create
a false impression. Attacks can also tamper the hardware i.e. the interconnected physical devices or the software,
namely the operating systems and applications.
FIGURE 9. Features of a Smart Watch.
FIGURE 10. Security requirements of Smart Healthcare
In the present digital health revolution, Intel is leading the list with their Digital Health Foundation [24]. The company
is constantly coming up with innovative technologies for data analytics, assistive technology and improving the home
environment for the elderly population. IBM’s Watson, an artificially intelligent computer system, can look at the
content of the patient’s health record and considers the medical information faster, to provide better health care models.
IBM has partnered with Apple, Johnson & Johnson and Medtronic to continue their digital health research in a large
scale. Google has a dedicated life sciences division to develop and
research new technologies in digital health. Qualcomm Life helps in
capturing the medical device data and integrates it to the nearby
database partner through a wireless medical device and secures the
information. This platform offered by Qualcomm provides high range
of system interoperability and security. Microsoft’s “Connected
Health Platform”, helps in offering digital health services through
desktop frameworks. Microsoft Lync is used by Doctor’s to offer
medical services to patients in rural areas. Samsung has a $50 million
investment in digital health through their Digital Health Initiative
which is a collaboration of smart sensors, algorithms and data
processing techniques through open source hardware and software
platforms. Apple has an open source framework, ResearchKit, which
aids researchers to develop apps that can facilitate
medical research.
On the retail front, Amazon offers a unified healthcare platform where the users can access healthcare information,
availability of latest products, health insurance and “on-demand” services. Wearables, especially in the form of smart
watches or bands, have been revolutionizing the market. Notable products include Fitbit, moov, Proteus, Pebble Time,
Withings AliveCor Health monitor, Beddit and so on. Significant amongst the healthcare products are smart watches.
Smart watches are becoming more ubiquitous, as shown in Figure 9. The projected annualized rate is expected to
reach 70 million units at a growth of 18% annualized rate by 2021. Apple is expected to have a hold of the larger share
in the market, however, Android wear devices are continuously emerging. Apple’s iWatch offers a package of built-
in GPS, and heart rate sensors with a fast dual-core processor.
8. SMART HEALTHCARE: CHALLENGES, VULNERABILTIES AND OPPORTUNITIES
Though smart healthcare helps in providing better healthcare to everyone in the world, it also becomes more vulnerable
to threats. Due to the dynamic nature and smaller form factors, the security requirements in smart healthcare systems
vary from the traditional security techniques [25]. Figure 10 shows the key security requirements or challenges in
maintaining a secured smart healthcare system. Healthcare networks contain personal information which can be easily
tampered with. In order to reduce the cost of the design, the processors used in smart healthcare systems are low speed
processors and have low on-device memory, which cannot accommodate additional security mechanisms [26].
Health care devices are mobile, which leads the user
to connect to different networks such as home
networks, office networks, and public networks. This
increases the chance of attacks on the device. Due to
the increase in the number of IoT devices in the
healthcare network, it is a very challenging task for
developers to provide dynamic security updates or a
sound solution for multi-protocol information. Smart
healthcare systems are vulnerable for security attacks
at various levels of the system. By maintaining data
freshness in the healthcare network, the passwords
and keys need to be updated frequently. Attacks
targeting data transmitted in the network can
include interruption of the service availability,
modification of original data, forging the messages and replaying the messages to disrupt the flow of data and create
a false impression. Attacks can also tamper the hardware i.e. the interconnected physical devices or the software,
namely the operating systems and applications.
FIGURE 9. Features of a Smart Watch.
FIGURE 10. Security requirements of Smart Healthcare
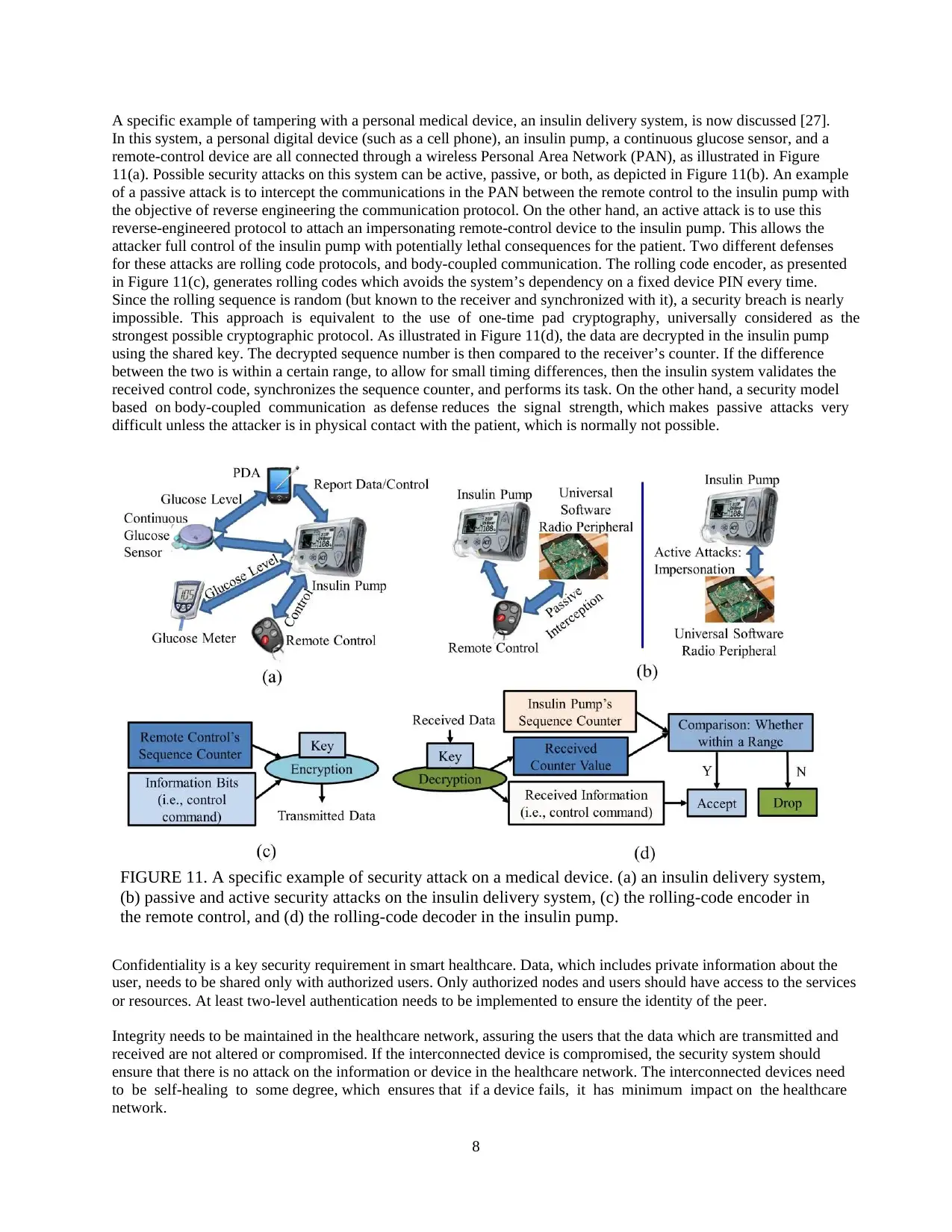
8
A specific example of tampering with a personal medical device, an insulin delivery system, is now discussed [27].
In this system, a personal digital device (such as a cell phone), an insulin pump, a continuous glucose sensor, and a
remote-control device are all connected through a wireless Personal Area Network (PAN), as illustrated in Figure
11(a). Possible security attacks on this system can be active, passive, or both, as depicted in Figure 11(b). An example
of a passive attack is to intercept the communications in the PAN between the remote control to the insulin pump with
the objective of reverse engineering the communication protocol. On the other hand, an active attack is to use this
reverse-engineered protocol to attach an impersonating remote-control device to the insulin pump. This allows the
attacker full control of the insulin pump with potentially lethal consequences for the patient. Two different defenses
for these attacks are rolling code protocols, and body-coupled communication. The rolling code encoder, as presented
in Figure 11(c), generates rolling codes which avoids the system’s dependency on a fixed device PIN every time.
Since the rolling sequence is random (but known to the receiver and synchronized with it), a security breach is nearly
impossible. This approach is equivalent to the use of one-time pad cryptography, universally considered as the
strongest possible cryptographic protocol. As illustrated in Figure 11(d), the data are decrypted in the insulin pump
using the shared key. The decrypted sequence number is then compared to the receiver’s counter. If the difference
between the two is within a certain range, to allow for small timing differences, then the insulin system validates the
received control code, synchronizes the sequence counter, and performs its task. On the other hand, a security model
based on body-coupled communication as defense reduces the signal strength, which makes passive attacks very
difficult unless the attacker is in physical contact with the patient, which is normally not possible.
Confidentiality is a key security requirement in smart healthcare. Data, which includes private information about the
user, needs to be shared only with authorized users. Only authorized nodes and users should have access to the services
or resources. At least two-level authentication needs to be implemented to ensure the identity of the peer.
Integrity needs to be maintained in the healthcare network, assuring the users that the data which are transmitted and
received are not altered or compromised. If the interconnected device is compromised, the security system should
ensure that there is no attack on the information or device in the healthcare network. The interconnected devices need
to be self-healing to some degree, which ensures that if a device fails, it has minimum impact on the healthcare
network.
FIGURE 11. A specific example of security attack on a medical device. (a) an insulin delivery system,
(b) passive and active security attacks on the insulin delivery system, (c) the rolling-code encoder in
the remote control, and (d) the rolling-code decoder in the insulin pump.
A specific example of tampering with a personal medical device, an insulin delivery system, is now discussed [27].
In this system, a personal digital device (such as a cell phone), an insulin pump, a continuous glucose sensor, and a
remote-control device are all connected through a wireless Personal Area Network (PAN), as illustrated in Figure
11(a). Possible security attacks on this system can be active, passive, or both, as depicted in Figure 11(b). An example
of a passive attack is to intercept the communications in the PAN between the remote control to the insulin pump with
the objective of reverse engineering the communication protocol. On the other hand, an active attack is to use this
reverse-engineered protocol to attach an impersonating remote-control device to the insulin pump. This allows the
attacker full control of the insulin pump with potentially lethal consequences for the patient. Two different defenses
for these attacks are rolling code protocols, and body-coupled communication. The rolling code encoder, as presented
in Figure 11(c), generates rolling codes which avoids the system’s dependency on a fixed device PIN every time.
Since the rolling sequence is random (but known to the receiver and synchronized with it), a security breach is nearly
impossible. This approach is equivalent to the use of one-time pad cryptography, universally considered as the
strongest possible cryptographic protocol. As illustrated in Figure 11(d), the data are decrypted in the insulin pump
using the shared key. The decrypted sequence number is then compared to the receiver’s counter. If the difference
between the two is within a certain range, to allow for small timing differences, then the insulin system validates the
received control code, synchronizes the sequence counter, and performs its task. On the other hand, a security model
based on body-coupled communication as defense reduces the signal strength, which makes passive attacks very
difficult unless the attacker is in physical contact with the patient, which is normally not possible.
Confidentiality is a key security requirement in smart healthcare. Data, which includes private information about the
user, needs to be shared only with authorized users. Only authorized nodes and users should have access to the services
or resources. At least two-level authentication needs to be implemented to ensure the identity of the peer.
Integrity needs to be maintained in the healthcare network, assuring the users that the data which are transmitted and
received are not altered or compromised. If the interconnected device is compromised, the security system should
ensure that there is no attack on the information or device in the healthcare network. The interconnected devices need
to be self-healing to some degree, which ensures that if a device fails, it has minimum impact on the healthcare
network.
FIGURE 11. A specific example of security attack on a medical device. (a) an insulin delivery system,
(b) passive and active security attacks on the insulin delivery system, (c) the rolling-code encoder in
the remote control, and (d) the rolling-code decoder in the insulin pump.
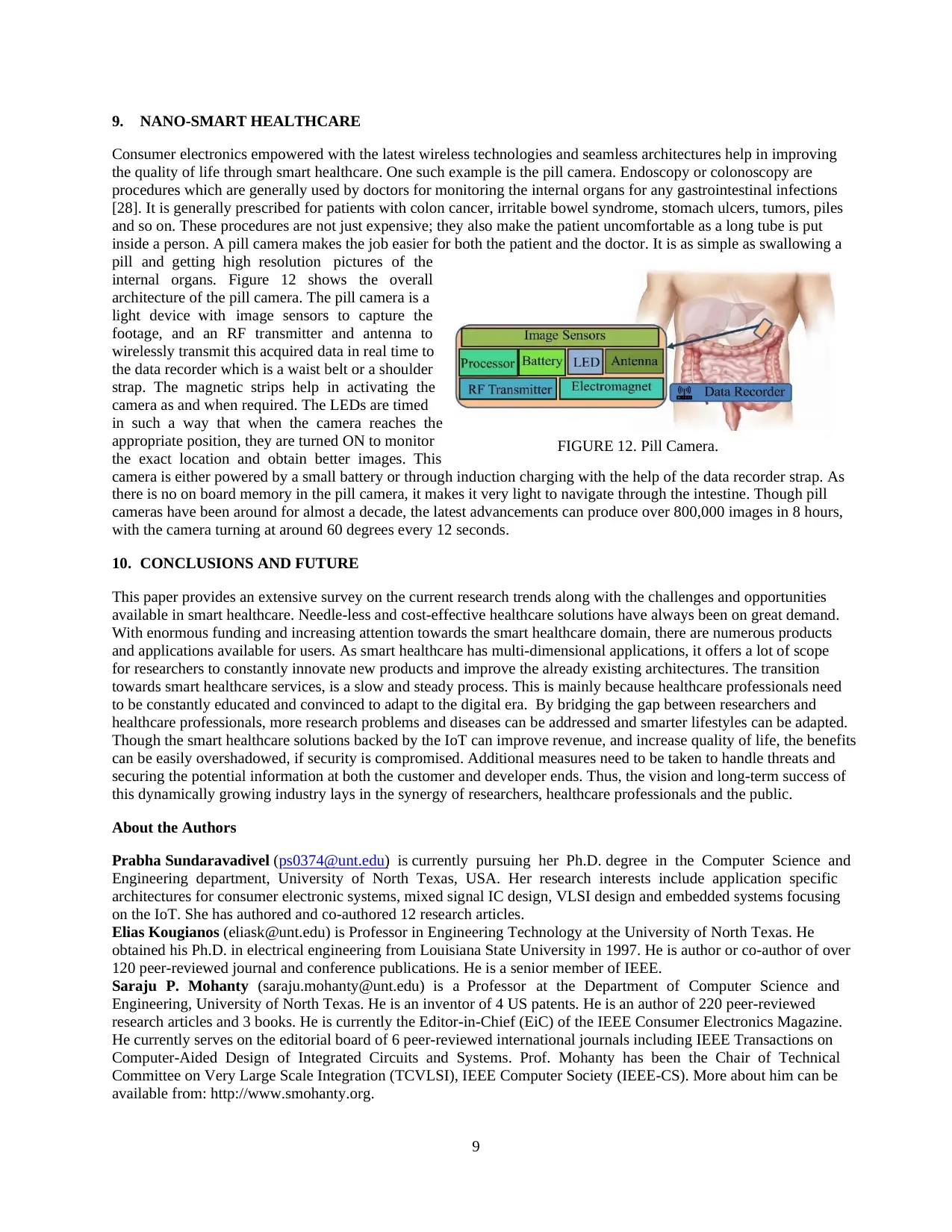
9
9. NANO-SMART HEALTHCARE
Consumer electronics empowered with the latest wireless technologies and seamless architectures help in improving
the quality of life through smart healthcare. One such example is the pill camera. Endoscopy or colonoscopy are
procedures which are generally used by doctors for monitoring the internal organs for any gastrointestinal infections
[28]. It is generally prescribed for patients with colon cancer, irritable bowel syndrome, stomach ulcers, tumors, piles
and so on. These procedures are not just expensive; they also make the patient uncomfortable as a long tube is put
inside a person. A pill camera makes the job easier for both the patient and the doctor. It is as simple as swallowing a
pill and getting high resolution pictures of the
internal organs. Figure 12 shows the overall
architecture of the pill camera. The pill camera is a
light device with image sensors to capture the
footage, and an RF transmitter and antenna to
wirelessly transmit this acquired data in real time to
the data recorder which is a waist belt or a shoulder
strap. The magnetic strips help in activating the
camera as and when required. The LEDs are timed
in such a way that when the camera reaches the
appropriate position, they are turned ON to monitor
the exact location and obtain better images. This
camera is either powered by a small battery or through induction charging with the help of the data recorder strap. As
there is no on board memory in the pill camera, it makes it very light to navigate through the intestine. Though pill
cameras have been around for almost a decade, the latest advancements can produce over 800,000 images in 8 hours,
with the camera turning at around 60 degrees every 12 seconds.
10. CONCLUSIONS AND FUTURE
This paper provides an extensive survey on the current research trends along with the challenges and opportunities
available in smart healthcare. Needle-less and cost-effective healthcare solutions have always been on great demand.
With enormous funding and increasing attention towards the smart healthcare domain, there are numerous products
and applications available for users. As smart healthcare has multi-dimensional applications, it offers a lot of scope
for researchers to constantly innovate new products and improve the already existing architectures. The transition
towards smart healthcare services, is a slow and steady process. This is mainly because healthcare professionals need
to be constantly educated and convinced to adapt to the digital era. By bridging the gap between researchers and
healthcare professionals, more research problems and diseases can be addressed and smarter lifestyles can be adapted.
Though the smart healthcare solutions backed by the IoT can improve revenue, and increase quality of life, the benefits
can be easily overshadowed, if security is compromised. Additional measures need to be taken to handle threats and
securing the potential information at both the customer and developer ends. Thus, the vision and long-term success of
this dynamically growing industry lays in the synergy of researchers, healthcare professionals and the public.
About the Authors
Prabha Sundaravadivel (ps0374@unt.edu) is currently pursuing her Ph.D. degree in the Computer Science and
Engineering department, University of North Texas, USA. Her research interests include application specific
architectures for consumer electronic systems, mixed signal IC design, VLSI design and embedded systems focusing
on the IoT. She has authored and co-authored 12 research articles.
Elias Kougianos (eliask@unt.edu) is Professor in Engineering Technology at the University of North Texas. He
obtained his Ph.D. in electrical engineering from Louisiana State University in 1997. He is author or co-author of over
120 peer-reviewed journal and conference publications. He is a senior member of IEEE.
Saraju P. Mohanty (saraju.mohanty@unt.edu) is a Professor at the Department of Computer Science and
Engineering, University of North Texas. He is an inventor of 4 US patents. He is an author of 220 peer-reviewed
research articles and 3 books. He is currently the Editor-in-Chief (EiC) of the IEEE Consumer Electronics Magazine.
He currently serves on the editorial board of 6 peer-reviewed international journals including IEEE Transactions on
Computer-Aided Design of Integrated Circuits and Systems. Prof. Mohanty has been the Chair of Technical
Committee on Very Large Scale Integration (TCVLSI), IEEE Computer Society (IEEE-CS). More about him can be
available from: http://www.smohanty.org.
FIGURE 12. Pill Camera.
9. NANO-SMART HEALTHCARE
Consumer electronics empowered with the latest wireless technologies and seamless architectures help in improving
the quality of life through smart healthcare. One such example is the pill camera. Endoscopy or colonoscopy are
procedures which are generally used by doctors for monitoring the internal organs for any gastrointestinal infections
[28]. It is generally prescribed for patients with colon cancer, irritable bowel syndrome, stomach ulcers, tumors, piles
and so on. These procedures are not just expensive; they also make the patient uncomfortable as a long tube is put
inside a person. A pill camera makes the job easier for both the patient and the doctor. It is as simple as swallowing a
pill and getting high resolution pictures of the
internal organs. Figure 12 shows the overall
architecture of the pill camera. The pill camera is a
light device with image sensors to capture the
footage, and an RF transmitter and antenna to
wirelessly transmit this acquired data in real time to
the data recorder which is a waist belt or a shoulder
strap. The magnetic strips help in activating the
camera as and when required. The LEDs are timed
in such a way that when the camera reaches the
appropriate position, they are turned ON to monitor
the exact location and obtain better images. This
camera is either powered by a small battery or through induction charging with the help of the data recorder strap. As
there is no on board memory in the pill camera, it makes it very light to navigate through the intestine. Though pill
cameras have been around for almost a decade, the latest advancements can produce over 800,000 images in 8 hours,
with the camera turning at around 60 degrees every 12 seconds.
10. CONCLUSIONS AND FUTURE
This paper provides an extensive survey on the current research trends along with the challenges and opportunities
available in smart healthcare. Needle-less and cost-effective healthcare solutions have always been on great demand.
With enormous funding and increasing attention towards the smart healthcare domain, there are numerous products
and applications available for users. As smart healthcare has multi-dimensional applications, it offers a lot of scope
for researchers to constantly innovate new products and improve the already existing architectures. The transition
towards smart healthcare services, is a slow and steady process. This is mainly because healthcare professionals need
to be constantly educated and convinced to adapt to the digital era. By bridging the gap between researchers and
healthcare professionals, more research problems and diseases can be addressed and smarter lifestyles can be adapted.
Though the smart healthcare solutions backed by the IoT can improve revenue, and increase quality of life, the benefits
can be easily overshadowed, if security is compromised. Additional measures need to be taken to handle threats and
securing the potential information at both the customer and developer ends. Thus, the vision and long-term success of
this dynamically growing industry lays in the synergy of researchers, healthcare professionals and the public.
About the Authors
Prabha Sundaravadivel (ps0374@unt.edu) is currently pursuing her Ph.D. degree in the Computer Science and
Engineering department, University of North Texas, USA. Her research interests include application specific
architectures for consumer electronic systems, mixed signal IC design, VLSI design and embedded systems focusing
on the IoT. She has authored and co-authored 12 research articles.
Elias Kougianos (eliask@unt.edu) is Professor in Engineering Technology at the University of North Texas. He
obtained his Ph.D. in electrical engineering from Louisiana State University in 1997. He is author or co-author of over
120 peer-reviewed journal and conference publications. He is a senior member of IEEE.
Saraju P. Mohanty (saraju.mohanty@unt.edu) is a Professor at the Department of Computer Science and
Engineering, University of North Texas. He is an inventor of 4 US patents. He is an author of 220 peer-reviewed
research articles and 3 books. He is currently the Editor-in-Chief (EiC) of the IEEE Consumer Electronics Magazine.
He currently serves on the editorial board of 6 peer-reviewed international journals including IEEE Transactions on
Computer-Aided Design of Integrated Circuits and Systems. Prof. Mohanty has been the Chair of Technical
Committee on Very Large Scale Integration (TCVLSI), IEEE Computer Society (IEEE-CS). More about him can be
available from: http://www.smohanty.org.
FIGURE 12. Pill Camera.
Secure Best Marks with AI Grader
Need help grading? Try our AI Grader for instant feedback on your assignments.
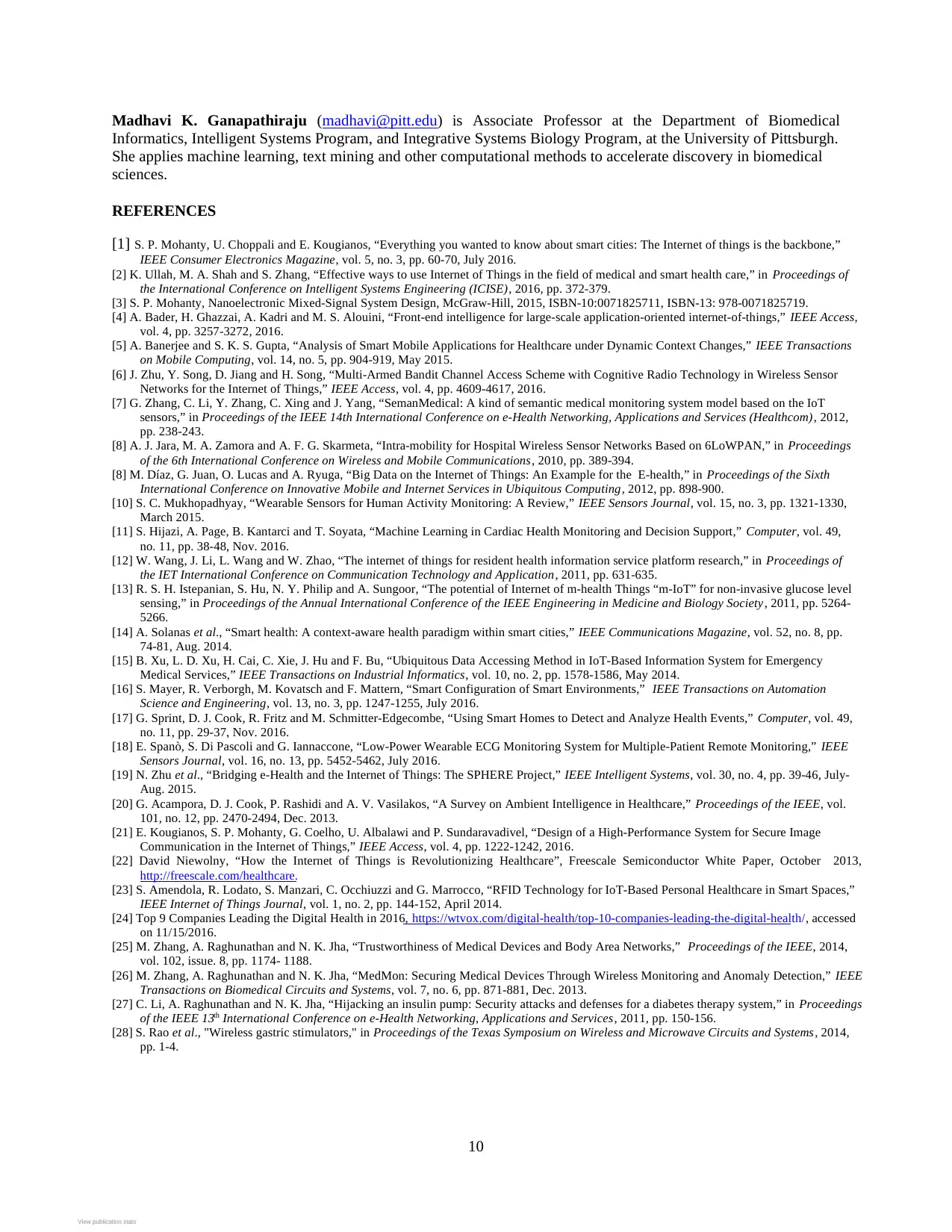
10
Madhavi K. Ganapathiraju (madhavi@pitt.edu) is Associate Professor at the Department of Biomedical
Informatics, Intelligent Systems Program, and Integrative Systems Biology Program, at the University of Pittsburgh.
She applies machine learning, text mining and other computational methods to accelerate discovery in biomedical
sciences.
REFERENCES
[1] S. P. Mohanty, U. Choppali and E. Kougianos, “Everything you wanted to know about smart cities: The Internet of things is the backbone,”
IEEE Consumer Electronics Magazine, vol. 5, no. 3, pp. 60-70, July 2016.
[2] K. Ullah, M. A. Shah and S. Zhang, “Effective ways to use Internet of Things in the field of medical and smart health care,” in Proceedings of
the International Conference on Intelligent Systems Engineering (ICISE), 2016, pp. 372-379.
[3] S. P. Mohanty, Nanoelectronic Mixed-Signal System Design, McGraw-Hill, 2015, ISBN-10:0071825711, ISBN-13: 978-0071825719.
[4] A. Bader, H. Ghazzai, A. Kadri and M. S. Alouini, “Front-end intelligence for large-scale application-oriented internet-of-things,” IEEE Access,
vol. 4, pp. 3257-3272, 2016.
[5] A. Banerjee and S. K. S. Gupta, “Analysis of Smart Mobile Applications for Healthcare under Dynamic Context Changes,” IEEE Transactions
on Mobile Computing, vol. 14, no. 5, pp. 904-919, May 2015.
[6] J. Zhu, Y. Song, D. Jiang and H. Song, “Multi-Armed Bandit Channel Access Scheme with Cognitive Radio Technology in Wireless Sensor
Networks for the Internet of Things,” IEEE Access, vol. 4, pp. 4609-4617, 2016.
[7] G. Zhang, C. Li, Y. Zhang, C. Xing and J. Yang, “SemanMedical: A kind of semantic medical monitoring system model based on the IoT
sensors,” in Proceedings of the IEEE 14th International Conference on e-Health Networking, Applications and Services (Healthcom), 2012,
pp. 238-243.
[8] A. J. Jara, M. A. Zamora and A. F. G. Skarmeta, “Intra-mobility for Hospital Wireless Sensor Networks Based on 6LoWPAN,” in Proceedings
of the 6th International Conference on Wireless and Mobile Communications, 2010, pp. 389-394.
[8] M. Díaz, G. Juan, O. Lucas and A. Ryuga, “Big Data on the Internet of Things: An Example for the E-health,” in Proceedings of the Sixth
International Conference on Innovative Mobile and Internet Services in Ubiquitous Computing, 2012, pp. 898-900.
[10] S. C. Mukhopadhyay, “Wearable Sensors for Human Activity Monitoring: A Review,” IEEE Sensors Journal, vol. 15, no. 3, pp. 1321-1330,
March 2015.
[11] S. Hijazi, A. Page, B. Kantarci and T. Soyata, “Machine Learning in Cardiac Health Monitoring and Decision Support,” Computer, vol. 49,
no. 11, pp. 38-48, Nov. 2016.
[12] W. Wang, J. Li, L. Wang and W. Zhao, “The internet of things for resident health information service platform research,” in Proceedings of
the IET International Conference on Communication Technology and Application, 2011, pp. 631-635.
[13] R. S. H. Istepanian, S. Hu, N. Y. Philip and A. Sungoor, “The potential of Internet of m-health Things “m-IoT” for non-invasive glucose level
sensing,” in Proceedings of the Annual International Conference of the IEEE Engineering in Medicine and Biology Society, 2011, pp. 5264-
5266.
[14] A. Solanas et al., “Smart health: A context-aware health paradigm within smart cities,” IEEE Communications Magazine, vol. 52, no. 8, pp.
74-81, Aug. 2014.
[15] B. Xu, L. D. Xu, H. Cai, C. Xie, J. Hu and F. Bu, “Ubiquitous Data Accessing Method in IoT-Based Information System for Emergency
Medical Services,” IEEE Transactions on Industrial Informatics, vol. 10, no. 2, pp. 1578-1586, May 2014.
[16] S. Mayer, R. Verborgh, M. Kovatsch and F. Mattern, “Smart Configuration of Smart Environments,” IEEE Transactions on Automation
Science and Engineering, vol. 13, no. 3, pp. 1247-1255, July 2016.
[17] G. Sprint, D. J. Cook, R. Fritz and M. Schmitter-Edgecombe, “Using Smart Homes to Detect and Analyze Health Events,” Computer, vol. 49,
no. 11, pp. 29-37, Nov. 2016.
[18] E. Spanò, S. Di Pascoli and G. Iannaccone, “Low-Power Wearable ECG Monitoring System for Multiple-Patient Remote Monitoring,” IEEE
Sensors Journal, vol. 16, no. 13, pp. 5452-5462, July 2016.
[19] N. Zhu et al., “Bridging e-Health and the Internet of Things: The SPHERE Project,” IEEE Intelligent Systems, vol. 30, no. 4, pp. 39-46, July-
Aug. 2015.
[20] G. Acampora, D. J. Cook, P. Rashidi and A. V. Vasilakos, “A Survey on Ambient Intelligence in Healthcare,” Proceedings of the IEEE, vol.
101, no. 12, pp. 2470-2494, Dec. 2013.
[21] E. Kougianos, S. P. Mohanty, G. Coelho, U. Albalawi and P. Sundaravadivel, “Design of a High-Performance System for Secure Image
Communication in the Internet of Things,” IEEE Access, vol. 4, pp. 1222-1242, 2016.
[22] David Niewolny, “How the Internet of Things is Revolutionizing Healthcare”, Freescale Semiconductor White Paper, October 2013,
http://freescale.com/healthcare.
[23] S. Amendola, R. Lodato, S. Manzari, C. Occhiuzzi and G. Marrocco, “RFID Technology for IoT-Based Personal Healthcare in Smart Spaces,”
IEEE Internet of Things Journal, vol. 1, no. 2, pp. 144-152, April 2014.
[24] Top 9 Companies Leading the Digital Health in 2016, https://wtvox.com/digital-health/top-10-companies-leading-the-digital-health/, accessed
on 11/15/2016.
[25] M. Zhang, A. Raghunathan and N. K. Jha, “Trustworthiness of Medical Devices and Body Area Networks,” Proceedings of the IEEE, 2014,
vol. 102, issue. 8, pp. 1174- 1188.
[26] M. Zhang, A. Raghunathan and N. K. Jha, “MedMon: Securing Medical Devices Through Wireless Monitoring and Anomaly Detection,” IEEE
Transactions on Biomedical Circuits and Systems, vol. 7, no. 6, pp. 871-881, Dec. 2013.
[27] C. Li, A. Raghunathan and N. K. Jha, “Hijacking an insulin pump: Security attacks and defenses for a diabetes therapy system,” in Proceedings
of the IEEE 13th International Conference on e-Health Networking, Applications and Services, 2011, pp. 150-156.
[28] S. Rao et al., "Wireless gastric stimulators," in Proceedings of the Texas Symposium on Wireless and Microwave Circuits and Systems, 2014,
pp. 1-4.
View publication statsView publication stats
Madhavi K. Ganapathiraju (madhavi@pitt.edu) is Associate Professor at the Department of Biomedical
Informatics, Intelligent Systems Program, and Integrative Systems Biology Program, at the University of Pittsburgh.
She applies machine learning, text mining and other computational methods to accelerate discovery in biomedical
sciences.
REFERENCES
[1] S. P. Mohanty, U. Choppali and E. Kougianos, “Everything you wanted to know about smart cities: The Internet of things is the backbone,”
IEEE Consumer Electronics Magazine, vol. 5, no. 3, pp. 60-70, July 2016.
[2] K. Ullah, M. A. Shah and S. Zhang, “Effective ways to use Internet of Things in the field of medical and smart health care,” in Proceedings of
the International Conference on Intelligent Systems Engineering (ICISE), 2016, pp. 372-379.
[3] S. P. Mohanty, Nanoelectronic Mixed-Signal System Design, McGraw-Hill, 2015, ISBN-10:0071825711, ISBN-13: 978-0071825719.
[4] A. Bader, H. Ghazzai, A. Kadri and M. S. Alouini, “Front-end intelligence for large-scale application-oriented internet-of-things,” IEEE Access,
vol. 4, pp. 3257-3272, 2016.
[5] A. Banerjee and S. K. S. Gupta, “Analysis of Smart Mobile Applications for Healthcare under Dynamic Context Changes,” IEEE Transactions
on Mobile Computing, vol. 14, no. 5, pp. 904-919, May 2015.
[6] J. Zhu, Y. Song, D. Jiang and H. Song, “Multi-Armed Bandit Channel Access Scheme with Cognitive Radio Technology in Wireless Sensor
Networks for the Internet of Things,” IEEE Access, vol. 4, pp. 4609-4617, 2016.
[7] G. Zhang, C. Li, Y. Zhang, C. Xing and J. Yang, “SemanMedical: A kind of semantic medical monitoring system model based on the IoT
sensors,” in Proceedings of the IEEE 14th International Conference on e-Health Networking, Applications and Services (Healthcom), 2012,
pp. 238-243.
[8] A. J. Jara, M. A. Zamora and A. F. G. Skarmeta, “Intra-mobility for Hospital Wireless Sensor Networks Based on 6LoWPAN,” in Proceedings
of the 6th International Conference on Wireless and Mobile Communications, 2010, pp. 389-394.
[8] M. Díaz, G. Juan, O. Lucas and A. Ryuga, “Big Data on the Internet of Things: An Example for the E-health,” in Proceedings of the Sixth
International Conference on Innovative Mobile and Internet Services in Ubiquitous Computing, 2012, pp. 898-900.
[10] S. C. Mukhopadhyay, “Wearable Sensors for Human Activity Monitoring: A Review,” IEEE Sensors Journal, vol. 15, no. 3, pp. 1321-1330,
March 2015.
[11] S. Hijazi, A. Page, B. Kantarci and T. Soyata, “Machine Learning in Cardiac Health Monitoring and Decision Support,” Computer, vol. 49,
no. 11, pp. 38-48, Nov. 2016.
[12] W. Wang, J. Li, L. Wang and W. Zhao, “The internet of things for resident health information service platform research,” in Proceedings of
the IET International Conference on Communication Technology and Application, 2011, pp. 631-635.
[13] R. S. H. Istepanian, S. Hu, N. Y. Philip and A. Sungoor, “The potential of Internet of m-health Things “m-IoT” for non-invasive glucose level
sensing,” in Proceedings of the Annual International Conference of the IEEE Engineering in Medicine and Biology Society, 2011, pp. 5264-
5266.
[14] A. Solanas et al., “Smart health: A context-aware health paradigm within smart cities,” IEEE Communications Magazine, vol. 52, no. 8, pp.
74-81, Aug. 2014.
[15] B. Xu, L. D. Xu, H. Cai, C. Xie, J. Hu and F. Bu, “Ubiquitous Data Accessing Method in IoT-Based Information System for Emergency
Medical Services,” IEEE Transactions on Industrial Informatics, vol. 10, no. 2, pp. 1578-1586, May 2014.
[16] S. Mayer, R. Verborgh, M. Kovatsch and F. Mattern, “Smart Configuration of Smart Environments,” IEEE Transactions on Automation
Science and Engineering, vol. 13, no. 3, pp. 1247-1255, July 2016.
[17] G. Sprint, D. J. Cook, R. Fritz and M. Schmitter-Edgecombe, “Using Smart Homes to Detect and Analyze Health Events,” Computer, vol. 49,
no. 11, pp. 29-37, Nov. 2016.
[18] E. Spanò, S. Di Pascoli and G. Iannaccone, “Low-Power Wearable ECG Monitoring System for Multiple-Patient Remote Monitoring,” IEEE
Sensors Journal, vol. 16, no. 13, pp. 5452-5462, July 2016.
[19] N. Zhu et al., “Bridging e-Health and the Internet of Things: The SPHERE Project,” IEEE Intelligent Systems, vol. 30, no. 4, pp. 39-46, July-
Aug. 2015.
[20] G. Acampora, D. J. Cook, P. Rashidi and A. V. Vasilakos, “A Survey on Ambient Intelligence in Healthcare,” Proceedings of the IEEE, vol.
101, no. 12, pp. 2470-2494, Dec. 2013.
[21] E. Kougianos, S. P. Mohanty, G. Coelho, U. Albalawi and P. Sundaravadivel, “Design of a High-Performance System for Secure Image
Communication in the Internet of Things,” IEEE Access, vol. 4, pp. 1222-1242, 2016.
[22] David Niewolny, “How the Internet of Things is Revolutionizing Healthcare”, Freescale Semiconductor White Paper, October 2013,
http://freescale.com/healthcare.
[23] S. Amendola, R. Lodato, S. Manzari, C. Occhiuzzi and G. Marrocco, “RFID Technology for IoT-Based Personal Healthcare in Smart Spaces,”
IEEE Internet of Things Journal, vol. 1, no. 2, pp. 144-152, April 2014.
[24] Top 9 Companies Leading the Digital Health in 2016, https://wtvox.com/digital-health/top-10-companies-leading-the-digital-health/, accessed
on 11/15/2016.
[25] M. Zhang, A. Raghunathan and N. K. Jha, “Trustworthiness of Medical Devices and Body Area Networks,” Proceedings of the IEEE, 2014,
vol. 102, issue. 8, pp. 1174- 1188.
[26] M. Zhang, A. Raghunathan and N. K. Jha, “MedMon: Securing Medical Devices Through Wireless Monitoring and Anomaly Detection,” IEEE
Transactions on Biomedical Circuits and Systems, vol. 7, no. 6, pp. 871-881, Dec. 2013.
[27] C. Li, A. Raghunathan and N. K. Jha, “Hijacking an insulin pump: Security attacks and defenses for a diabetes therapy system,” in Proceedings
of the IEEE 13th International Conference on e-Health Networking, Applications and Services, 2011, pp. 150-156.
[28] S. Rao et al., "Wireless gastric stimulators," in Proceedings of the Texas Symposium on Wireless and Microwave Circuits and Systems, 2014,
pp. 1-4.
View publication statsView publication stats
1 out of 11
![[object Object]](/_next/image/?url=%2F_next%2Fstatic%2Fmedia%2Flogo.6d15ce61.png&w=640&q=75)
Your All-in-One AI-Powered Toolkit for Academic Success.
+13062052269
info@desklib.com
Available 24*7 on WhatsApp / Email
Unlock your academic potential
© 2024 | Zucol Services PVT LTD | All rights reserved.