Alkali-Silica Reaction on Prestressed Concrete: Effects and Mitigation
VerifiedAdded on 2022/11/17
|53
|13202
|437
AI Summary
This report discusses the alkali-silica reaction (ASR) on prestressed concrete, its effects on concrete durability, and preventive measures. It includes a literature review on ASR, its mechanism, and factors responsible for it. The report also covers the detrimental effects of ASR, testing methods, and mitigation techniques.
Contribute Materials
Your contribution can guide someone’s learning journey. Share your
documents today.
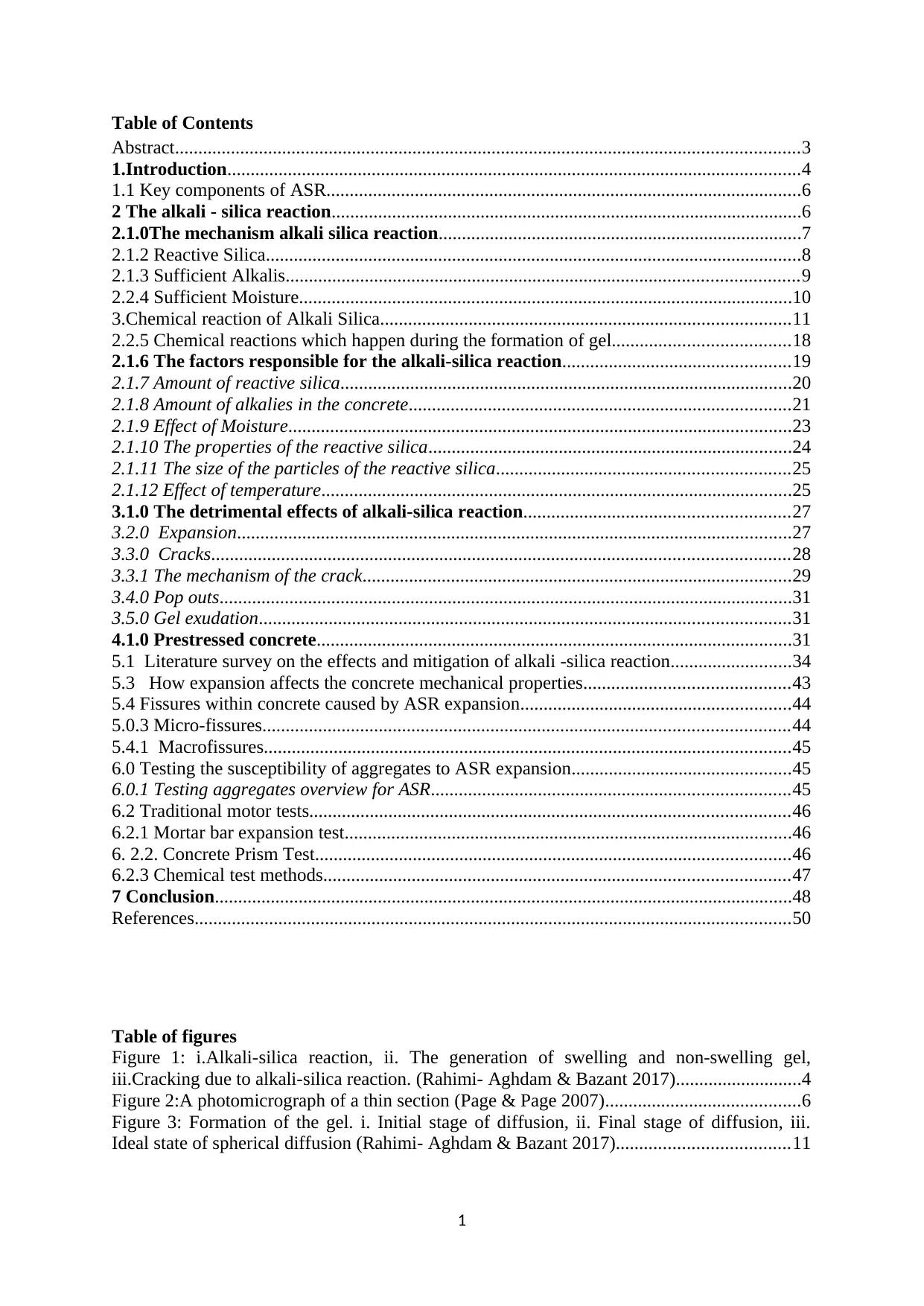
Table of Contents
Abstract......................................................................................................................................3
1.Introduction...........................................................................................................................4
1.1 Key components of ASR......................................................................................................6
2 The alkali - silica reaction.....................................................................................................6
2.1.0The mechanism alkali silica reaction..............................................................................7
2.1.2 Reactive Silica...................................................................................................................8
2.1.3 Sufficient Alkalis..............................................................................................................9
2.2.4 Sufficient Moisture..........................................................................................................10
3.Chemical reaction of Alkali Silica........................................................................................11
2.2.5 Chemical reactions which happen during the formation of gel......................................18
2.1.6 The factors responsible for the alkali-silica reaction.................................................19
2.1.7 Amount of reactive silica.................................................................................................20
2.1.8 Amount of alkalies in the concrete..................................................................................21
2.1.9 Effect of Moisture............................................................................................................23
2.1.10 The properties of the reactive silica..............................................................................24
2.1.11 The size of the particles of the reactive silica...............................................................25
2.1.12 Effect of temperature.....................................................................................................25
3.1.0 The detrimental effects of alkali-silica reaction.........................................................27
3.2.0 Expansion.......................................................................................................................27
3.3.0 Cracks............................................................................................................................28
3.3.1 The mechanism of the crack............................................................................................29
3.4.0 Pop outs...........................................................................................................................31
3.5.0 Gel exudation..................................................................................................................31
4.1.0 Prestressed concrete......................................................................................................31
5.1 Literature survey on the effects and mitigation of alkali -silica reaction..........................34
5.3 How expansion affects the concrete mechanical properties............................................43
5.4 Fissures within concrete caused by ASR expansion..........................................................44
5.0.3 Micro-fissures.................................................................................................................44
5.4.1 Macrofissures.................................................................................................................45
6.0 Testing the susceptibility of aggregates to ASR expansion...............................................45
6.0.1 Testing aggregates overview for ASR.............................................................................45
6.2 Traditional motor tests.......................................................................................................46
6.2.1 Mortar bar expansion test................................................................................................46
6. 2.2. Concrete Prism Test......................................................................................................46
6.2.3 Chemical test methods....................................................................................................47
7 Conclusion............................................................................................................................48
References................................................................................................................................50
Table of figures
Figure 1: i.Alkali-silica reaction, ii. The generation of swelling and non-swelling gel,
iii.Cracking due to alkali-silica reaction. (Rahimi- Aghdam & Bazant 2017)...........................4
Figure 2:A photomicrograph of a thin section (Page & Page 2007)..........................................6
Figure 3: Formation of the gel. i. Initial stage of diffusion, ii. Final stage of diffusion, iii.
Ideal state of spherical diffusion (Rahimi- Aghdam & Bazant 2017).....................................11
1
Abstract......................................................................................................................................3
1.Introduction...........................................................................................................................4
1.1 Key components of ASR......................................................................................................6
2 The alkali - silica reaction.....................................................................................................6
2.1.0The mechanism alkali silica reaction..............................................................................7
2.1.2 Reactive Silica...................................................................................................................8
2.1.3 Sufficient Alkalis..............................................................................................................9
2.2.4 Sufficient Moisture..........................................................................................................10
3.Chemical reaction of Alkali Silica........................................................................................11
2.2.5 Chemical reactions which happen during the formation of gel......................................18
2.1.6 The factors responsible for the alkali-silica reaction.................................................19
2.1.7 Amount of reactive silica.................................................................................................20
2.1.8 Amount of alkalies in the concrete..................................................................................21
2.1.9 Effect of Moisture............................................................................................................23
2.1.10 The properties of the reactive silica..............................................................................24
2.1.11 The size of the particles of the reactive silica...............................................................25
2.1.12 Effect of temperature.....................................................................................................25
3.1.0 The detrimental effects of alkali-silica reaction.........................................................27
3.2.0 Expansion.......................................................................................................................27
3.3.0 Cracks............................................................................................................................28
3.3.1 The mechanism of the crack............................................................................................29
3.4.0 Pop outs...........................................................................................................................31
3.5.0 Gel exudation..................................................................................................................31
4.1.0 Prestressed concrete......................................................................................................31
5.1 Literature survey on the effects and mitigation of alkali -silica reaction..........................34
5.3 How expansion affects the concrete mechanical properties............................................43
5.4 Fissures within concrete caused by ASR expansion..........................................................44
5.0.3 Micro-fissures.................................................................................................................44
5.4.1 Macrofissures.................................................................................................................45
6.0 Testing the susceptibility of aggregates to ASR expansion...............................................45
6.0.1 Testing aggregates overview for ASR.............................................................................45
6.2 Traditional motor tests.......................................................................................................46
6.2.1 Mortar bar expansion test................................................................................................46
6. 2.2. Concrete Prism Test......................................................................................................46
6.2.3 Chemical test methods....................................................................................................47
7 Conclusion............................................................................................................................48
References................................................................................................................................50
Table of figures
Figure 1: i.Alkali-silica reaction, ii. The generation of swelling and non-swelling gel,
iii.Cracking due to alkali-silica reaction. (Rahimi- Aghdam & Bazant 2017)...........................4
Figure 2:A photomicrograph of a thin section (Page & Page 2007)..........................................6
Figure 3: Formation of the gel. i. Initial stage of diffusion, ii. Final stage of diffusion, iii.
Ideal state of spherical diffusion (Rahimi- Aghdam & Bazant 2017).....................................11
1
Secure Best Marks with AI Grader
Need help grading? Try our AI Grader for instant feedback on your assignments.
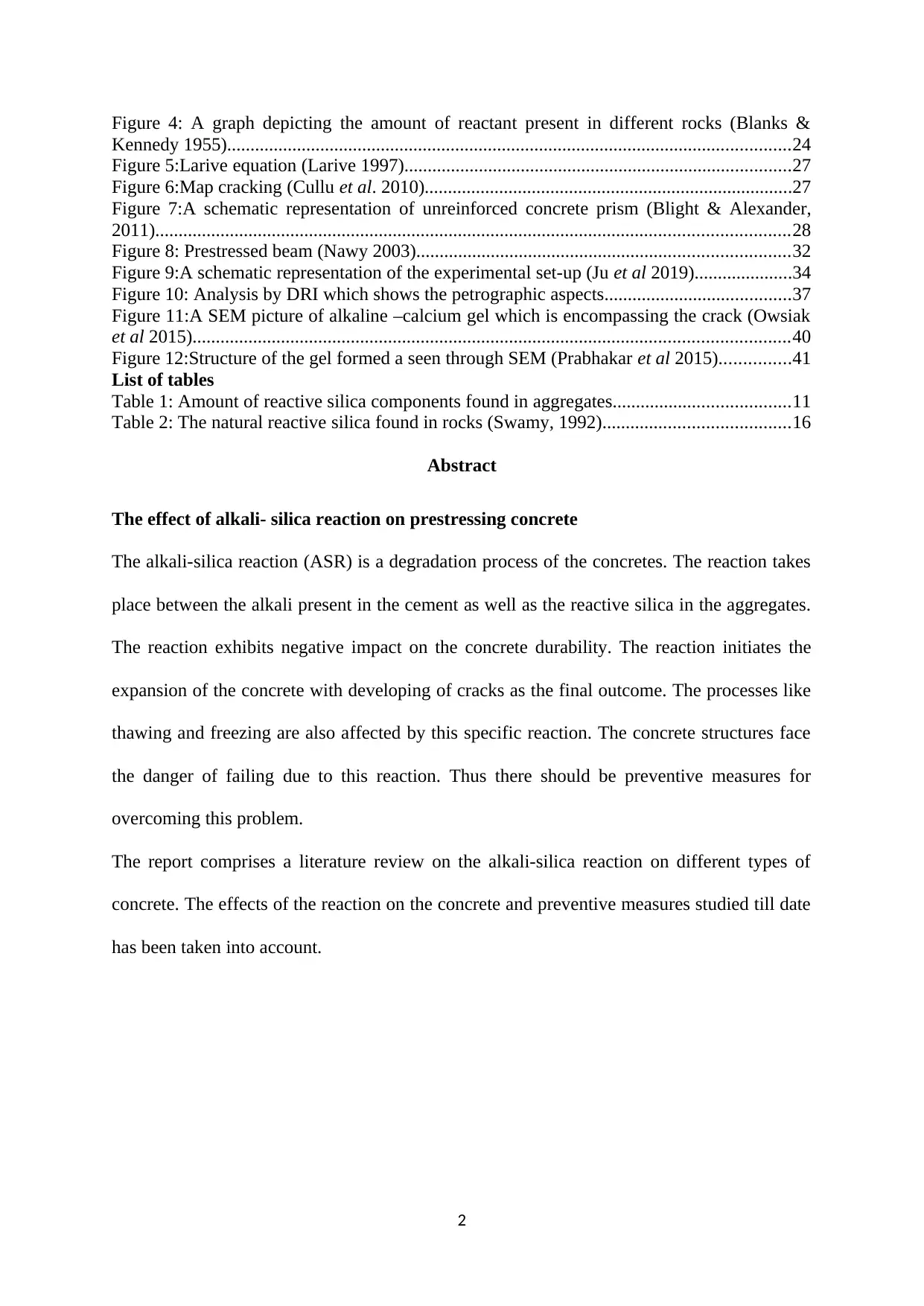
Figure 4: A graph depicting the amount of reactant present in different rocks (Blanks &
Kennedy 1955).........................................................................................................................24
Figure 5:Larive equation (Larive 1997)...................................................................................27
Figure 6:Map cracking (Cullu et al. 2010)...............................................................................27
Figure 7:A schematic representation of unreinforced concrete prism (Blight & Alexander,
2011)........................................................................................................................................28
Figure 8: Prestressed beam (Nawy 2003)................................................................................32
Figure 9:A schematic representation of the experimental set-up (Ju et al 2019).....................34
Figure 10: Analysis by DRI which shows the petrographic aspects........................................37
Figure 11:A SEM picture of alkaline –calcium gel which is encompassing the crack (Owsiak
et al 2015)................................................................................................................................40
Figure 12:Structure of the gel formed a seen through SEM (Prabhakar et al 2015)...............41
List of tables
Table 1: Amount of reactive silica components found in aggregates......................................11
Table 2: The natural reactive silica found in rocks (Swamy, 1992)........................................16
Abstract
The effect of alkali- silica reaction on prestressing concrete
The alkali-silica reaction (ASR) is a degradation process of the concretes. The reaction takes
place between the alkali present in the cement as well as the reactive silica in the aggregates.
The reaction exhibits negative impact on the concrete durability. The reaction initiates the
expansion of the concrete with developing of cracks as the final outcome. The processes like
thawing and freezing are also affected by this specific reaction. The concrete structures face
the danger of failing due to this reaction. Thus there should be preventive measures for
overcoming this problem.
The report comprises a literature review on the alkali-silica reaction on different types of
concrete. The effects of the reaction on the concrete and preventive measures studied till date
has been taken into account.
2
Kennedy 1955).........................................................................................................................24
Figure 5:Larive equation (Larive 1997)...................................................................................27
Figure 6:Map cracking (Cullu et al. 2010)...............................................................................27
Figure 7:A schematic representation of unreinforced concrete prism (Blight & Alexander,
2011)........................................................................................................................................28
Figure 8: Prestressed beam (Nawy 2003)................................................................................32
Figure 9:A schematic representation of the experimental set-up (Ju et al 2019).....................34
Figure 10: Analysis by DRI which shows the petrographic aspects........................................37
Figure 11:A SEM picture of alkaline –calcium gel which is encompassing the crack (Owsiak
et al 2015)................................................................................................................................40
Figure 12:Structure of the gel formed a seen through SEM (Prabhakar et al 2015)...............41
List of tables
Table 1: Amount of reactive silica components found in aggregates......................................11
Table 2: The natural reactive silica found in rocks (Swamy, 1992)........................................16
Abstract
The effect of alkali- silica reaction on prestressing concrete
The alkali-silica reaction (ASR) is a degradation process of the concretes. The reaction takes
place between the alkali present in the cement as well as the reactive silica in the aggregates.
The reaction exhibits negative impact on the concrete durability. The reaction initiates the
expansion of the concrete with developing of cracks as the final outcome. The processes like
thawing and freezing are also affected by this specific reaction. The concrete structures face
the danger of failing due to this reaction. Thus there should be preventive measures for
overcoming this problem.
The report comprises a literature review on the alkali-silica reaction on different types of
concrete. The effects of the reaction on the concrete and preventive measures studied till date
has been taken into account.
2
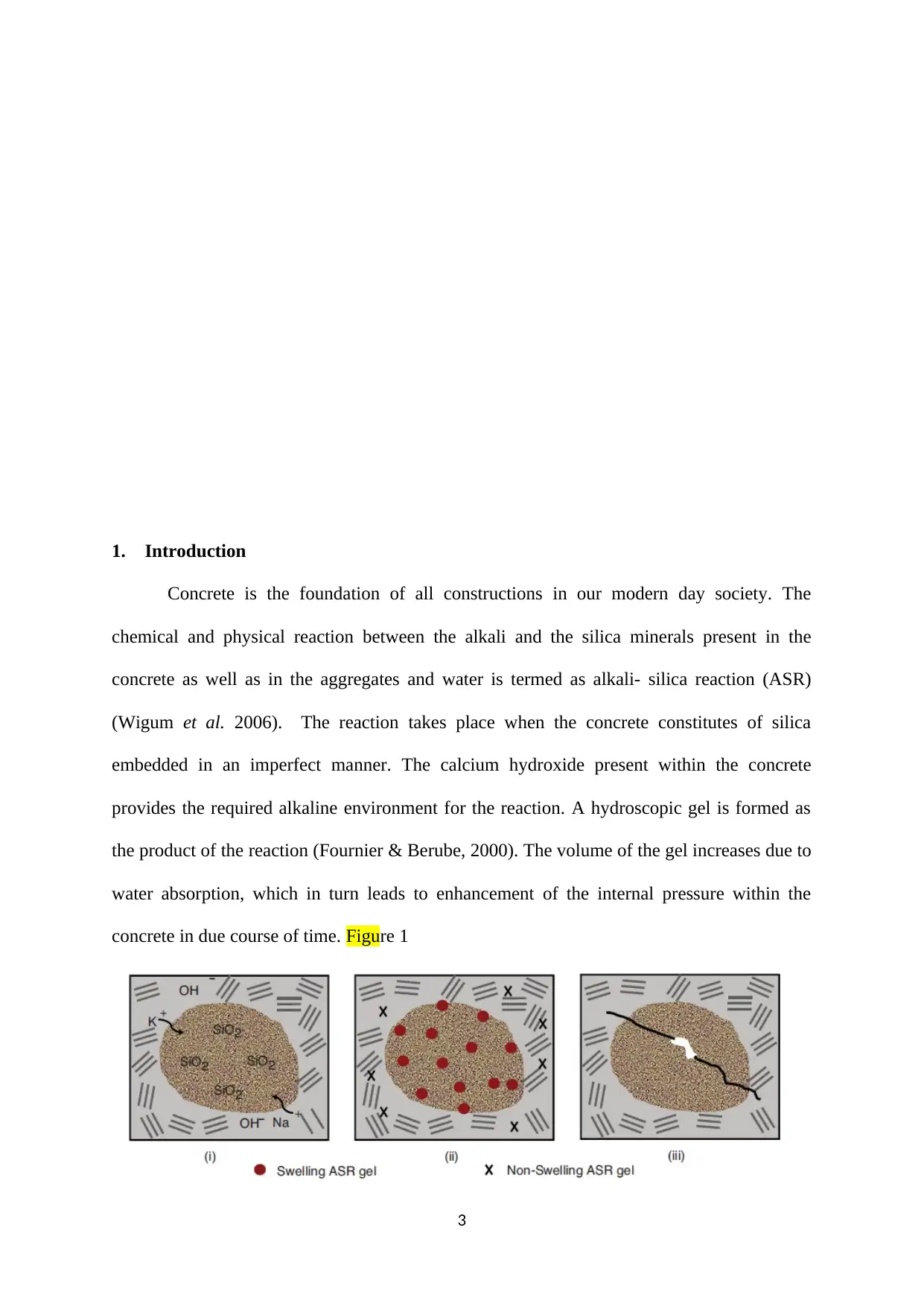
1. Introduction
Concrete is the foundation of all constructions in our modern day society. The
chemical and physical reaction between the alkali and the silica minerals present in the
concrete as well as in the aggregates and water is termed as alkali- silica reaction (ASR)
(Wigum et al. 2006). The reaction takes place when the concrete constitutes of silica
embedded in an imperfect manner. The calcium hydroxide present within the concrete
provides the required alkaline environment for the reaction. A hydroscopic gel is formed as
the product of the reaction (Fournier & Berube, 2000). The volume of the gel increases due to
water absorption, which in turn leads to enhancement of the internal pressure within the
concrete in due course of time. Figure 1
3
Concrete is the foundation of all constructions in our modern day society. The
chemical and physical reaction between the alkali and the silica minerals present in the
concrete as well as in the aggregates and water is termed as alkali- silica reaction (ASR)
(Wigum et al. 2006). The reaction takes place when the concrete constitutes of silica
embedded in an imperfect manner. The calcium hydroxide present within the concrete
provides the required alkaline environment for the reaction. A hydroscopic gel is formed as
the product of the reaction (Fournier & Berube, 2000). The volume of the gel increases due to
water absorption, which in turn leads to enhancement of the internal pressure within the
concrete in due course of time. Figure 1
3
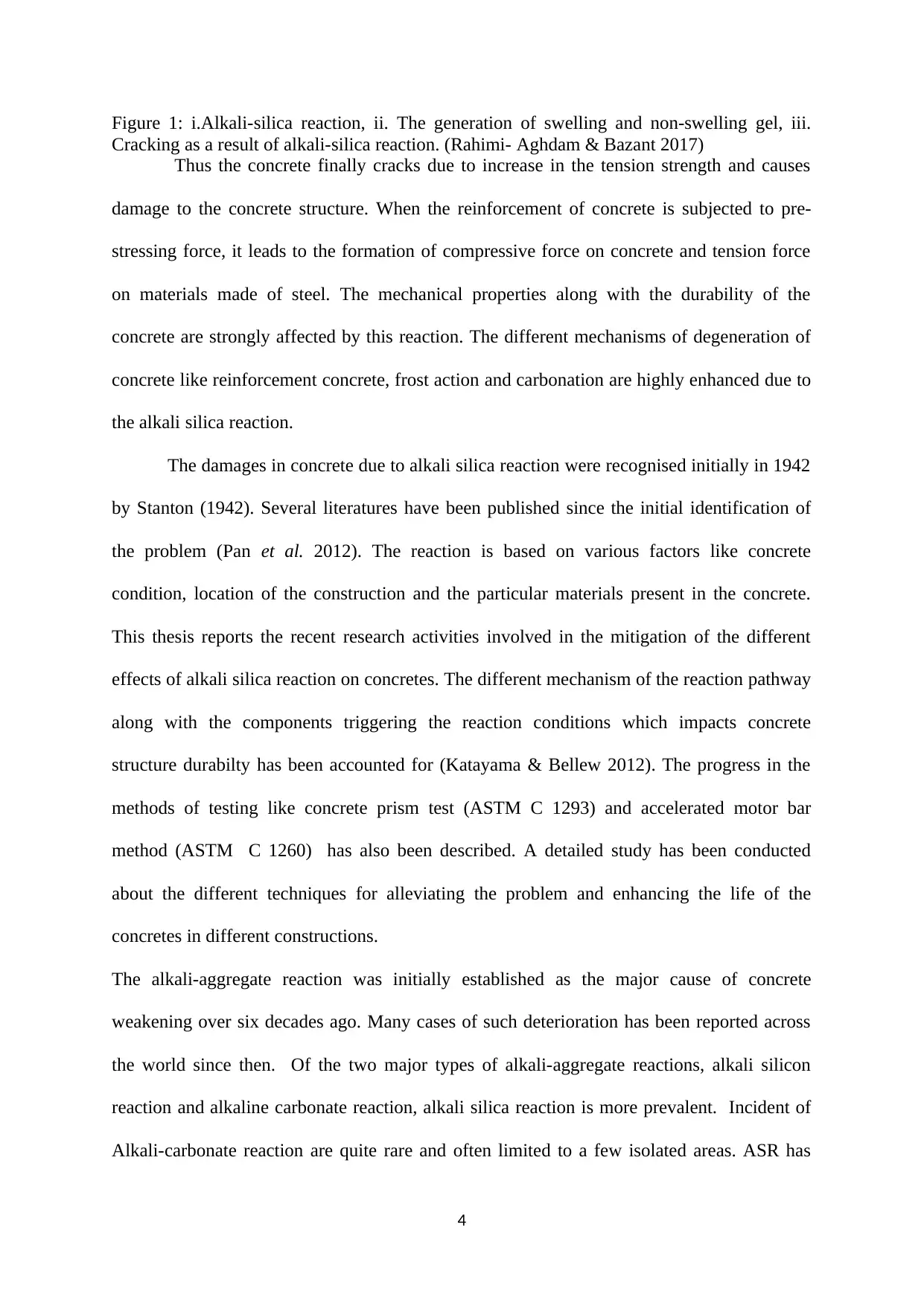
Figure 1: i.Alkali-silica reaction, ii. The generation of swelling and non-swelling gel, iii.
Cracking as a result of alkali-silica reaction. (Rahimi- Aghdam & Bazant 2017)
Thus the concrete finally cracks due to increase in the tension strength and causes
damage to the concrete structure. When the reinforcement of concrete is subjected to pre-
stressing force, it leads to the formation of compressive force on concrete and tension force
on materials made of steel. The mechanical properties along with the durability of the
concrete are strongly affected by this reaction. The different mechanisms of degeneration of
concrete like reinforcement concrete, frost action and carbonation are highly enhanced due to
the alkali silica reaction.
The damages in concrete due to alkali silica reaction were recognised initially in 1942
by Stanton (1942). Several literatures have been published since the initial identification of
the problem (Pan et al. 2012). The reaction is based on various factors like concrete
condition, location of the construction and the particular materials present in the concrete.
This thesis reports the recent research activities involved in the mitigation of the different
effects of alkali silica reaction on concretes. The different mechanism of the reaction pathway
along with the components triggering the reaction conditions which impacts concrete
structure durabilty has been accounted for (Katayama & Bellew 2012). The progress in the
methods of testing like concrete prism test (ASTM C 1293) and accelerated motor bar
method (ASTM C 1260) has also been described. A detailed study has been conducted
about the different techniques for alleviating the problem and enhancing the life of the
concretes in different constructions.
The alkali-aggregate reaction was initially established as the major cause of concrete
weakening over six decades ago. Many cases of such deterioration has been reported across
the world since then. Of the two major types of alkali-aggregate reactions, alkali silicon
reaction and alkaline carbonate reaction, alkali silica reaction is more prevalent. Incident of
Alkali-carbonate reaction are quite rare and often limited to a few isolated areas. ASR has
4
Cracking as a result of alkali-silica reaction. (Rahimi- Aghdam & Bazant 2017)
Thus the concrete finally cracks due to increase in the tension strength and causes
damage to the concrete structure. When the reinforcement of concrete is subjected to pre-
stressing force, it leads to the formation of compressive force on concrete and tension force
on materials made of steel. The mechanical properties along with the durability of the
concrete are strongly affected by this reaction. The different mechanisms of degeneration of
concrete like reinforcement concrete, frost action and carbonation are highly enhanced due to
the alkali silica reaction.
The damages in concrete due to alkali silica reaction were recognised initially in 1942
by Stanton (1942). Several literatures have been published since the initial identification of
the problem (Pan et al. 2012). The reaction is based on various factors like concrete
condition, location of the construction and the particular materials present in the concrete.
This thesis reports the recent research activities involved in the mitigation of the different
effects of alkali silica reaction on concretes. The different mechanism of the reaction pathway
along with the components triggering the reaction conditions which impacts concrete
structure durabilty has been accounted for (Katayama & Bellew 2012). The progress in the
methods of testing like concrete prism test (ASTM C 1293) and accelerated motor bar
method (ASTM C 1260) has also been described. A detailed study has been conducted
about the different techniques for alleviating the problem and enhancing the life of the
concretes in different constructions.
The alkali-aggregate reaction was initially established as the major cause of concrete
weakening over six decades ago. Many cases of such deterioration has been reported across
the world since then. Of the two major types of alkali-aggregate reactions, alkali silicon
reaction and alkaline carbonate reaction, alkali silica reaction is more prevalent. Incident of
Alkali-carbonate reaction are quite rare and often limited to a few isolated areas. ASR has
4
Secure Best Marks with AI Grader
Need help grading? Try our AI Grader for instant feedback on your assignments.
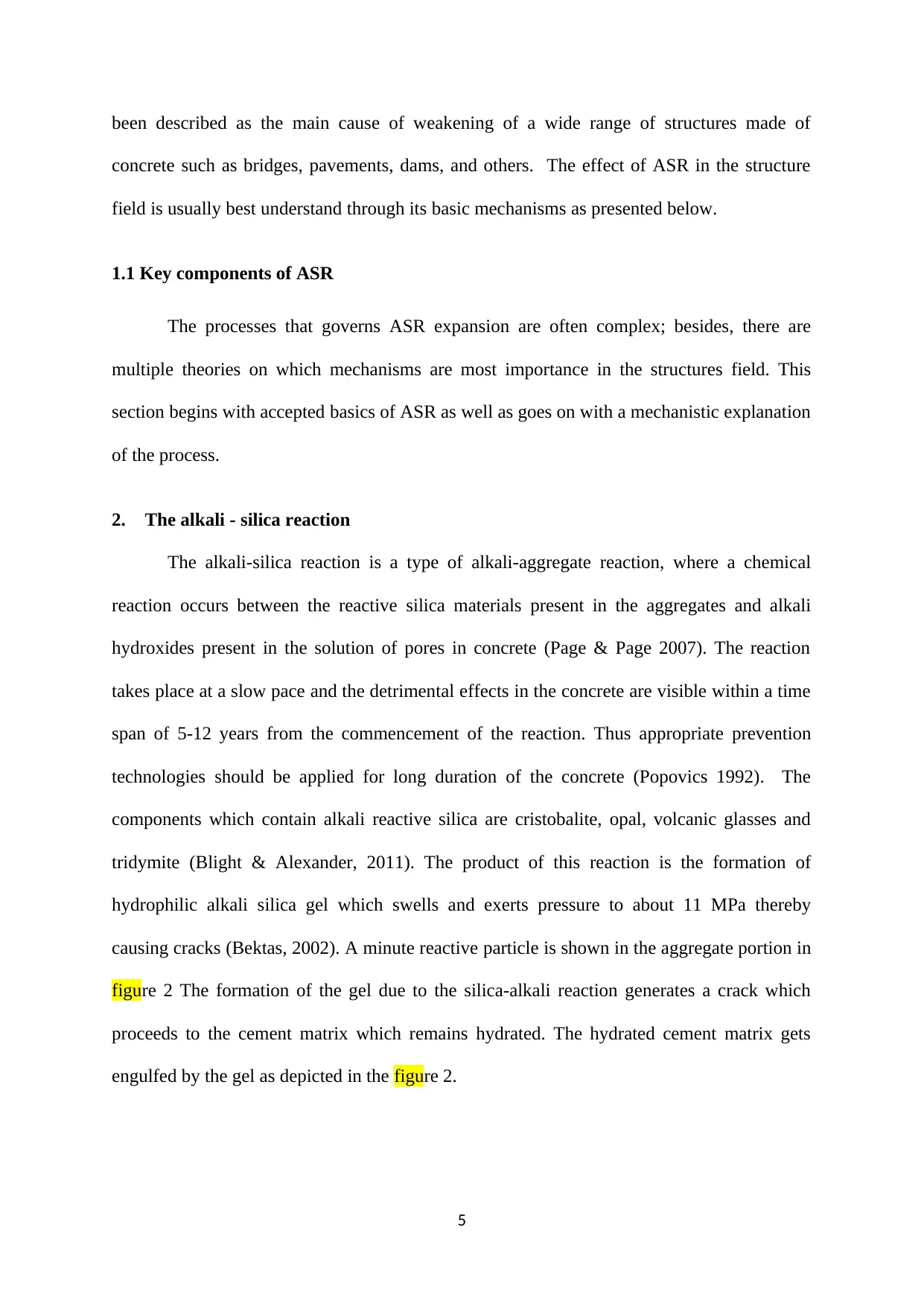
been described as the main cause of weakening of a wide range of structures made of
concrete such as bridges, pavements, dams, and others. The effect of ASR in the structure
field is usually best understand through its basic mechanisms as presented below.
1.1 Key components of ASR
The processes that governs ASR expansion are often complex; besides, there are
multiple theories on which mechanisms are most importance in the structures field. This
section begins with accepted basics of ASR as well as goes on with a mechanistic explanation
of the process.
2. The alkali - silica reaction
The alkali-silica reaction is a type of alkali-aggregate reaction, where a chemical
reaction occurs between the reactive silica materials present in the aggregates and alkali
hydroxides present in the solution of pores in concrete (Page & Page 2007). The reaction
takes place at a slow pace and the detrimental effects in the concrete are visible within a time
span of 5-12 years from the commencement of the reaction. Thus appropriate prevention
technologies should be applied for long duration of the concrete (Popovics 1992). The
components which contain alkali reactive silica are cristobalite, opal, volcanic glasses and
tridymite (Blight & Alexander, 2011). The product of this reaction is the formation of
hydrophilic alkali silica gel which swells and exerts pressure to about 11 MPa thereby
causing cracks (Bektas, 2002). A minute reactive particle is shown in the aggregate portion in
figure 2 The formation of the gel due to the silica-alkali reaction generates a crack which
proceeds to the cement matrix which remains hydrated. The hydrated cement matrix gets
engulfed by the gel as depicted in the figure 2.
5
concrete such as bridges, pavements, dams, and others. The effect of ASR in the structure
field is usually best understand through its basic mechanisms as presented below.
1.1 Key components of ASR
The processes that governs ASR expansion are often complex; besides, there are
multiple theories on which mechanisms are most importance in the structures field. This
section begins with accepted basics of ASR as well as goes on with a mechanistic explanation
of the process.
2. The alkali - silica reaction
The alkali-silica reaction is a type of alkali-aggregate reaction, where a chemical
reaction occurs between the reactive silica materials present in the aggregates and alkali
hydroxides present in the solution of pores in concrete (Page & Page 2007). The reaction
takes place at a slow pace and the detrimental effects in the concrete are visible within a time
span of 5-12 years from the commencement of the reaction. Thus appropriate prevention
technologies should be applied for long duration of the concrete (Popovics 1992). The
components which contain alkali reactive silica are cristobalite, opal, volcanic glasses and
tridymite (Blight & Alexander, 2011). The product of this reaction is the formation of
hydrophilic alkali silica gel which swells and exerts pressure to about 11 MPa thereby
causing cracks (Bektas, 2002). A minute reactive particle is shown in the aggregate portion in
figure 2 The formation of the gel due to the silica-alkali reaction generates a crack which
proceeds to the cement matrix which remains hydrated. The hydrated cement matrix gets
engulfed by the gel as depicted in the figure 2.
5
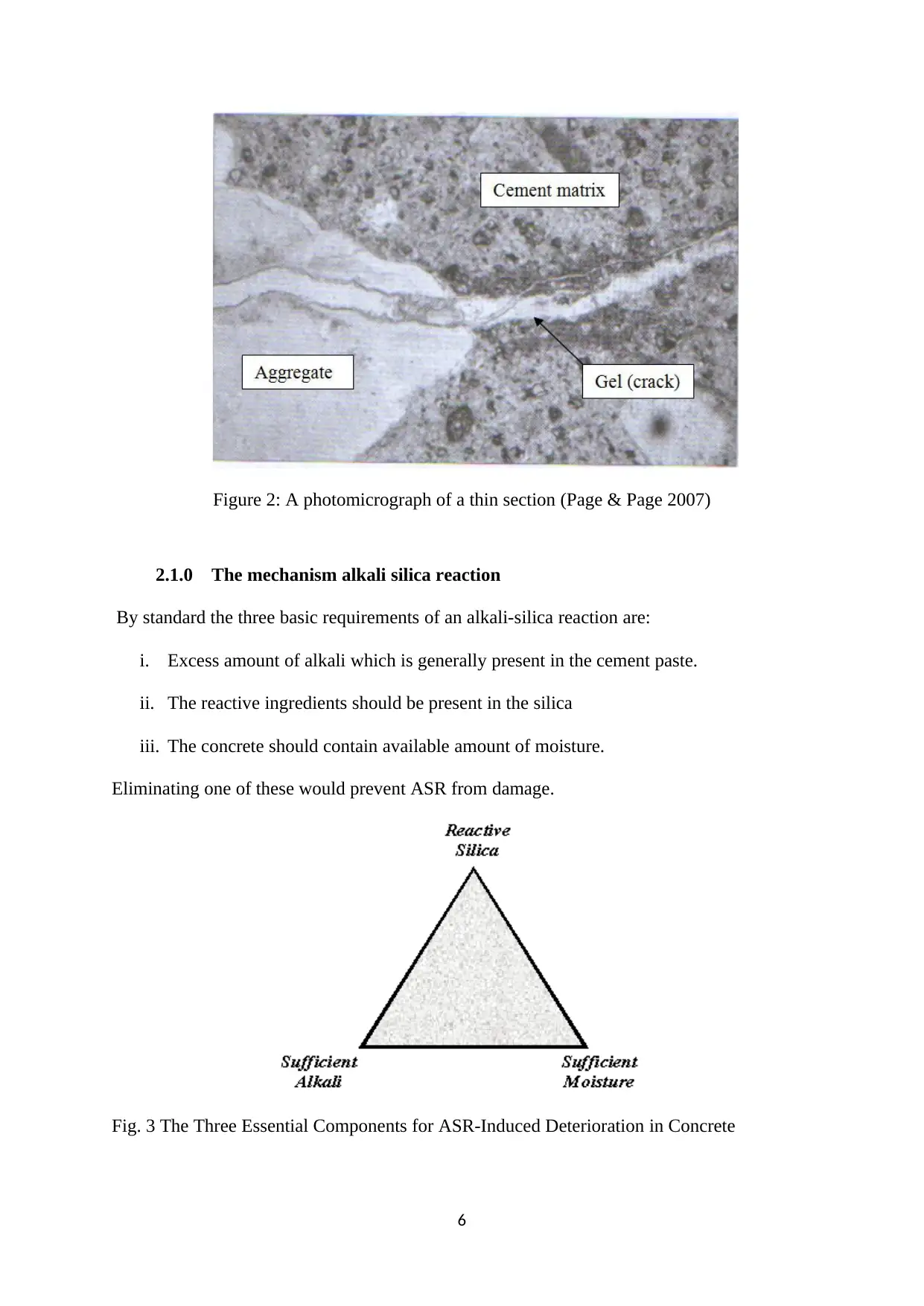
Figure 2: A photomicrograph of a thin section (Page & Page 2007)
2.1.0 The mechanism alkali silica reaction
By standard the three basic requirements of an alkali-silica reaction are:
i. Excess amount of alkali which is generally present in the cement paste.
ii. The reactive ingredients should be present in the silica
iii. The concrete should contain available amount of moisture.
Eliminating one of these would prevent ASR from damage.
Fig. 3 The Three Essential Components for ASR-Induced Deterioration in Concrete
6
2.1.0 The mechanism alkali silica reaction
By standard the three basic requirements of an alkali-silica reaction are:
i. Excess amount of alkali which is generally present in the cement paste.
ii. The reactive ingredients should be present in the silica
iii. The concrete should contain available amount of moisture.
Eliminating one of these would prevent ASR from damage.
Fig. 3 The Three Essential Components for ASR-Induced Deterioration in Concrete
6
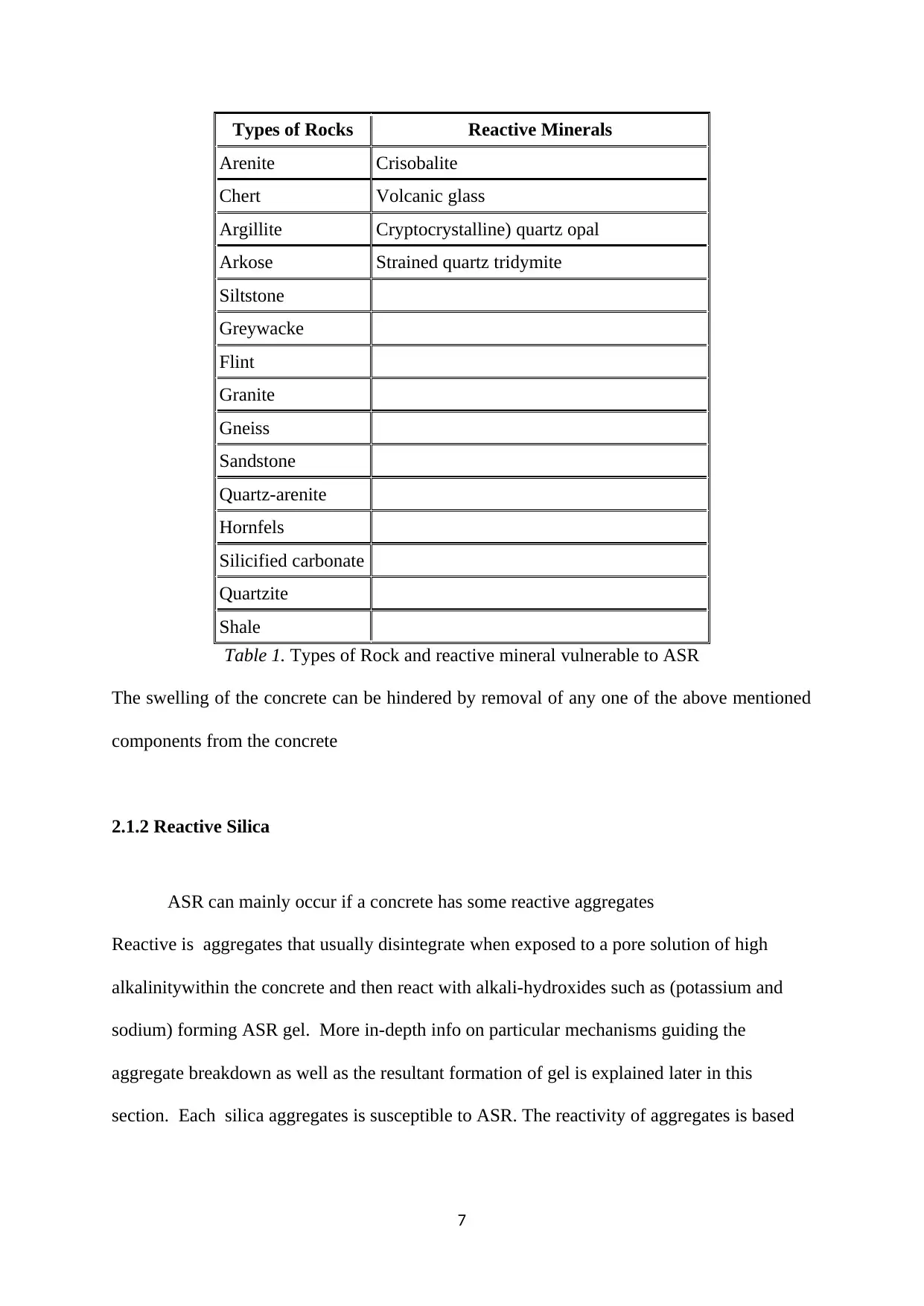
Types of Rocks Reactive Minerals
Arenite Crisobalite
Chert Volcanic glass
Argillite Cryptocrystalline) quartz opal
Arkose Strained quartz tridymite
Siltstone
Greywacke
Flint
Granite
Gneiss
Sandstone
Quartz-arenite
Hornfels
Silicified carbonate
Quartzite
Shale
Table 1. Types of Rock and reactive mineral vulnerable to ASR
The swelling of the concrete can be hindered by removal of any one of the above mentioned
components from the concrete
2.1.2 Reactive Silica
ASR can mainly occur if a concrete has some reactive aggregates
Reactive is aggregates that usually disintegrate when exposed to a pore solution of high
alkalinitywithin the concrete and then react with alkali-hydroxides such as (potassium and
sodium) forming ASR gel. More in-depth info on particular mechanisms guiding the
aggregate breakdown as well as the resultant formation of gel is explained later in this
section. Each silica aggregates is susceptible to ASR. The reactivity of aggregates is based
7
Arenite Crisobalite
Chert Volcanic glass
Argillite Cryptocrystalline) quartz opal
Arkose Strained quartz tridymite
Siltstone
Greywacke
Flint
Granite
Gneiss
Sandstone
Quartz-arenite
Hornfels
Silicified carbonate
Quartzite
Shale
Table 1. Types of Rock and reactive mineral vulnerable to ASR
The swelling of the concrete can be hindered by removal of any one of the above mentioned
components from the concrete
2.1.2 Reactive Silica
ASR can mainly occur if a concrete has some reactive aggregates
Reactive is aggregates that usually disintegrate when exposed to a pore solution of high
alkalinitywithin the concrete and then react with alkali-hydroxides such as (potassium and
sodium) forming ASR gel. More in-depth info on particular mechanisms guiding the
aggregate breakdown as well as the resultant formation of gel is explained later in this
section. Each silica aggregates is susceptible to ASR. The reactivity of aggregates is based
7
Paraphrase This Document
Need a fresh take? Get an instant paraphrase of this document with our AI Paraphraser
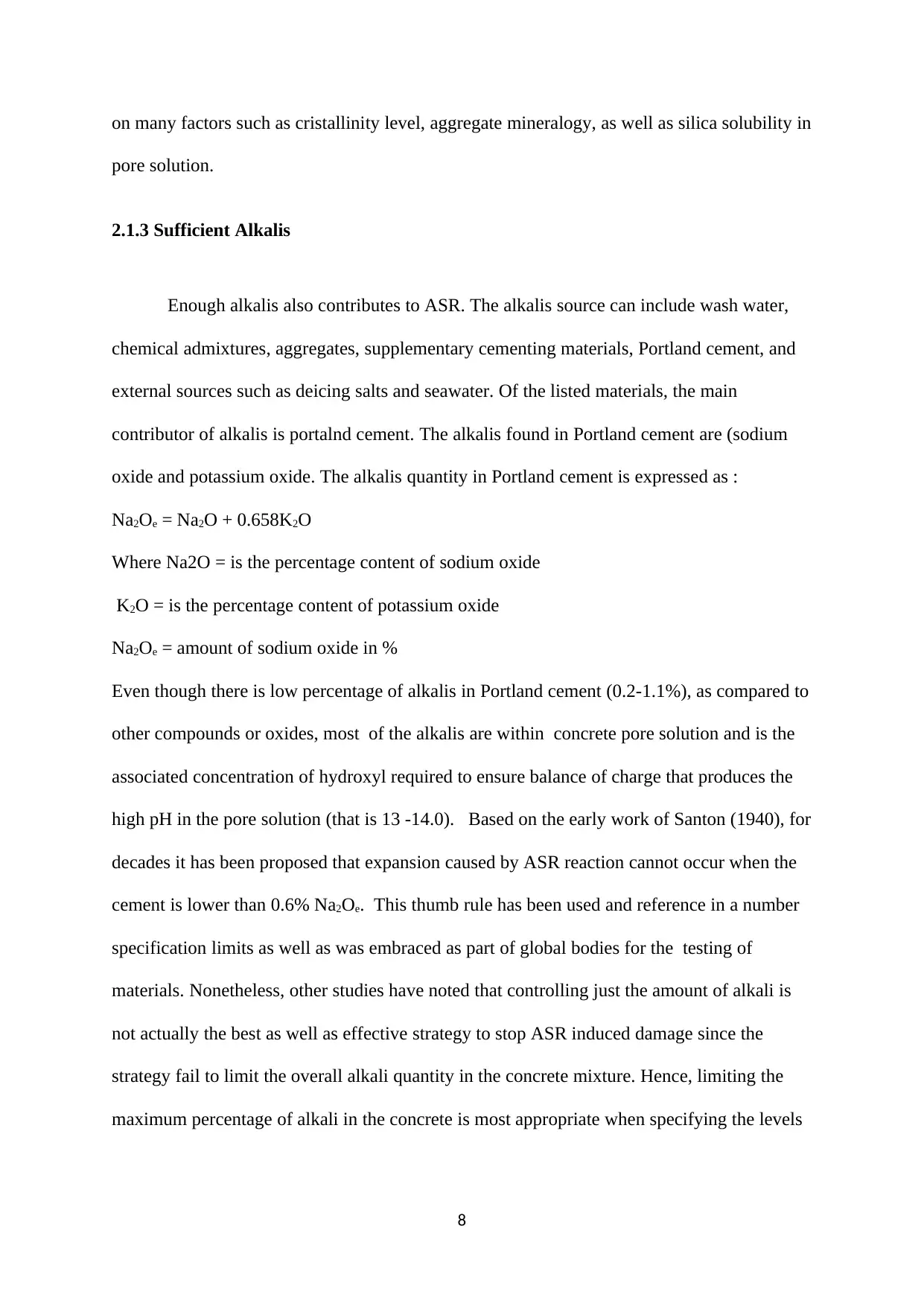
on many factors such as cristallinity level, aggregate mineralogy, as well as silica solubility in
pore solution.
2.1.3 Sufficient Alkalis
Enough alkalis also contributes to ASR. The alkalis source can include wash water,
chemical admixtures, aggregates, supplementary cementing materials, Portland cement, and
external sources such as deicing salts and seawater. Of the listed materials, the main
contributor of alkalis is portalnd cement. The alkalis found in Portland cement are (sodium
oxide and potassium oxide. The alkalis quantity in Portland cement is expressed as :
Na2Oe = Na2O + 0.658K2O
Where Na2O = is the percentage content of sodium oxide
K2O = is the percentage content of potassium oxide
Na2Oe = amount of sodium oxide in %
Even though there is low percentage of alkalis in Portland cement (0.2-1.1%), as compared to
other compounds or oxides, most of the alkalis are within concrete pore solution and is the
associated concentration of hydroxyl required to ensure balance of charge that produces the
high pH in the pore solution (that is 13 -14.0). Based on the early work of Santon (1940), for
decades it has been proposed that expansion caused by ASR reaction cannot occur when the
cement is lower than 0.6% Na2Oe. This thumb rule has been used and reference in a number
specification limits as well as was embraced as part of global bodies for the testing of
materials. Nonetheless, other studies have noted that controlling just the amount of alkali is
not actually the best as well as effective strategy to stop ASR induced damage since the
strategy fail to limit the overall alkali quantity in the concrete mixture. Hence, limiting the
maximum percentage of alkali in the concrete is most appropriate when specifying the levels
8
pore solution.
2.1.3 Sufficient Alkalis
Enough alkalis also contributes to ASR. The alkalis source can include wash water,
chemical admixtures, aggregates, supplementary cementing materials, Portland cement, and
external sources such as deicing salts and seawater. Of the listed materials, the main
contributor of alkalis is portalnd cement. The alkalis found in Portland cement are (sodium
oxide and potassium oxide. The alkalis quantity in Portland cement is expressed as :
Na2Oe = Na2O + 0.658K2O
Where Na2O = is the percentage content of sodium oxide
K2O = is the percentage content of potassium oxide
Na2Oe = amount of sodium oxide in %
Even though there is low percentage of alkalis in Portland cement (0.2-1.1%), as compared to
other compounds or oxides, most of the alkalis are within concrete pore solution and is the
associated concentration of hydroxyl required to ensure balance of charge that produces the
high pH in the pore solution (that is 13 -14.0). Based on the early work of Santon (1940), for
decades it has been proposed that expansion caused by ASR reaction cannot occur when the
cement is lower than 0.6% Na2Oe. This thumb rule has been used and reference in a number
specification limits as well as was embraced as part of global bodies for the testing of
materials. Nonetheless, other studies have noted that controlling just the amount of alkali is
not actually the best as well as effective strategy to stop ASR induced damage since the
strategy fail to limit the overall alkali quantity in the concrete mixture. Hence, limiting the
maximum percentage of alkali in the concrete is most appropriate when specifying the levels
8
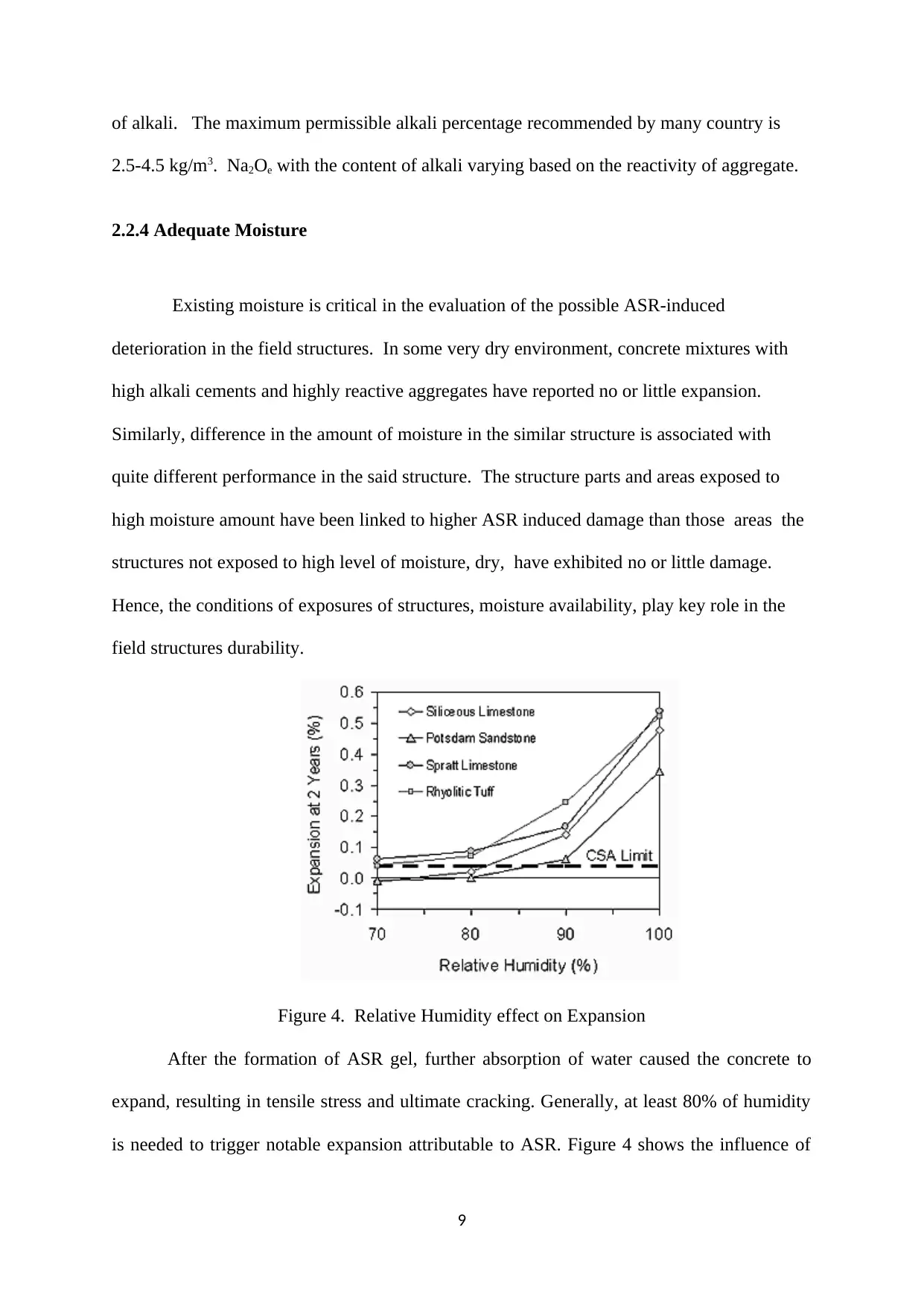
of alkali. The maximum permissible alkali percentage recommended by many country is
2.5-4.5 kg/m3. Na2Oe with the content of alkali varying based on the reactivity of aggregate.
2.2.4 Adequate Moisture
Existing moisture is critical in the evaluation of the possible ASR-induced
deterioration in the field structures. In some very dry environment, concrete mixtures with
high alkali cements and highly reactive aggregates have reported no or little expansion.
Similarly, difference in the amount of moisture in the similar structure is associated with
quite different performance in the said structure. The structure parts and areas exposed to
high moisture amount have been linked to higher ASR induced damage than those areas the
structures not exposed to high level of moisture, dry, have exhibited no or little damage.
Hence, the conditions of exposures of structures, moisture availability, play key role in the
field structures durability.
Figure 4. Relative Humidity effect on Expansion
After the formation of ASR gel, further absorption of water caused the concrete to
expand, resulting in tensile stress and ultimate cracking. Generally, at least 80% of humidity
is needed to trigger notable expansion attributable to ASR. Figure 4 shows the influence of
9
2.5-4.5 kg/m3. Na2Oe with the content of alkali varying based on the reactivity of aggregate.
2.2.4 Adequate Moisture
Existing moisture is critical in the evaluation of the possible ASR-induced
deterioration in the field structures. In some very dry environment, concrete mixtures with
high alkali cements and highly reactive aggregates have reported no or little expansion.
Similarly, difference in the amount of moisture in the similar structure is associated with
quite different performance in the said structure. The structure parts and areas exposed to
high moisture amount have been linked to higher ASR induced damage than those areas the
structures not exposed to high level of moisture, dry, have exhibited no or little damage.
Hence, the conditions of exposures of structures, moisture availability, play key role in the
field structures durability.
Figure 4. Relative Humidity effect on Expansion
After the formation of ASR gel, further absorption of water caused the concrete to
expand, resulting in tensile stress and ultimate cracking. Generally, at least 80% of humidity
is needed to trigger notable expansion attributable to ASR. Figure 4 shows the influence of
9
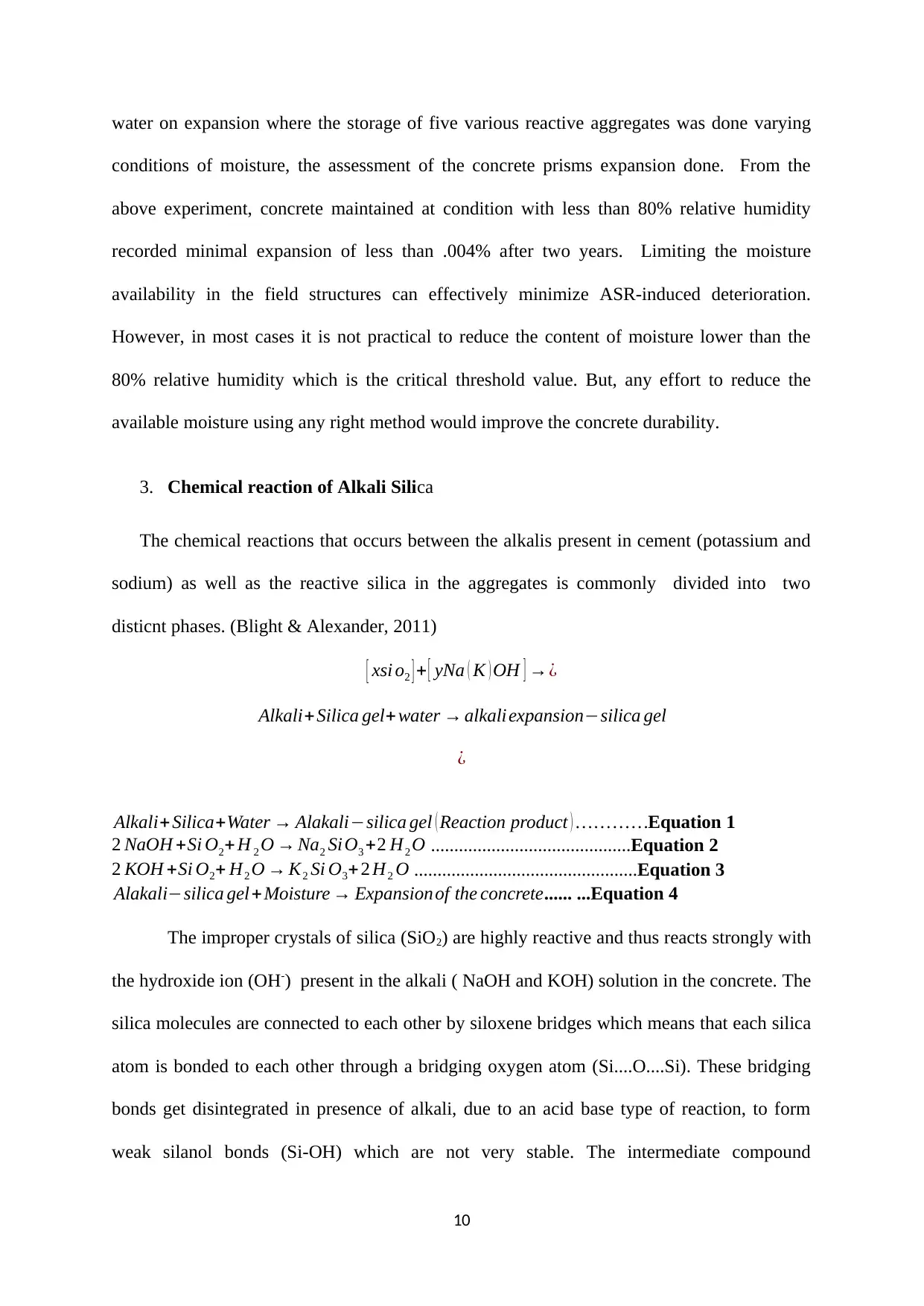
water on expansion where the storage of five various reactive aggregates was done varying
conditions of moisture, the assessment of the concrete prisms expansion done. From the
above experiment, concrete maintained at condition with less than 80% relative humidity
recorded minimal expansion of less than .004% after two years. Limiting the moisture
availability in the field structures can effectively minimize ASR-induced deterioration.
However, in most cases it is not practical to reduce the content of moisture lower than the
80% relative humidity which is the critical threshold value. But, any effort to reduce the
available moisture using any right method would improve the concrete durability.
3. Chemical reaction of Alkali Silica
The chemical reactions that occurs between the alkalis present in cement (potassium and
sodium) as well as the reactive silica in the aggregates is commonly divided into two
disticnt phases. (Blight & Alexander, 2011)
[ xsi o2 ] + [ yNa ( K ) OH ] →¿
Alkali+ Silica gel+water → alkaliexpansion−silica gel
¿
Alkali+Silica+Water → Alakali−silica gel ( Reaction product ) …… … …Equation 1
2 NaOH +Si O2+ H 2 O → Na2 SiO3 +2 H2 O ...........................................Equation 2
2 KOH +Si O2+ H2 O → K2 Si O3+ 2 H2 O ................................................Equation 3
Alakali−silica gel+Moisture → Expansionof the concrete...... ...Equation 4
The improper crystals of silica (SiO2) are highly reactive and thus reacts strongly with
the hydroxide ion (OH-) present in the alkali ( NaOH and KOH) solution in the concrete. The
silica molecules are connected to each other by siloxene bridges which means that each silica
atom is bonded to each other through a bridging oxygen atom (Si....O....Si). These bridging
bonds get disintegrated in presence of alkali, due to an acid base type of reaction, to form
weak silanol bonds (Si-OH) which are not very stable. The intermediate compound
10
conditions of moisture, the assessment of the concrete prisms expansion done. From the
above experiment, concrete maintained at condition with less than 80% relative humidity
recorded minimal expansion of less than .004% after two years. Limiting the moisture
availability in the field structures can effectively minimize ASR-induced deterioration.
However, in most cases it is not practical to reduce the content of moisture lower than the
80% relative humidity which is the critical threshold value. But, any effort to reduce the
available moisture using any right method would improve the concrete durability.
3. Chemical reaction of Alkali Silica
The chemical reactions that occurs between the alkalis present in cement (potassium and
sodium) as well as the reactive silica in the aggregates is commonly divided into two
disticnt phases. (Blight & Alexander, 2011)
[ xsi o2 ] + [ yNa ( K ) OH ] →¿
Alkali+ Silica gel+water → alkaliexpansion−silica gel
¿
Alkali+Silica+Water → Alakali−silica gel ( Reaction product ) …… … …Equation 1
2 NaOH +Si O2+ H 2 O → Na2 SiO3 +2 H2 O ...........................................Equation 2
2 KOH +Si O2+ H2 O → K2 Si O3+ 2 H2 O ................................................Equation 3
Alakali−silica gel+Moisture → Expansionof the concrete...... ...Equation 4
The improper crystals of silica (SiO2) are highly reactive and thus reacts strongly with
the hydroxide ion (OH-) present in the alkali ( NaOH and KOH) solution in the concrete. The
silica molecules are connected to each other by siloxene bridges which means that each silica
atom is bonded to each other through a bridging oxygen atom (Si....O....Si). These bridging
bonds get disintegrated in presence of alkali, due to an acid base type of reaction, to form
weak silanol bonds (Si-OH) which are not very stable. The intermediate compound
10
Secure Best Marks with AI Grader
Need help grading? Try our AI Grader for instant feedback on your assignments.
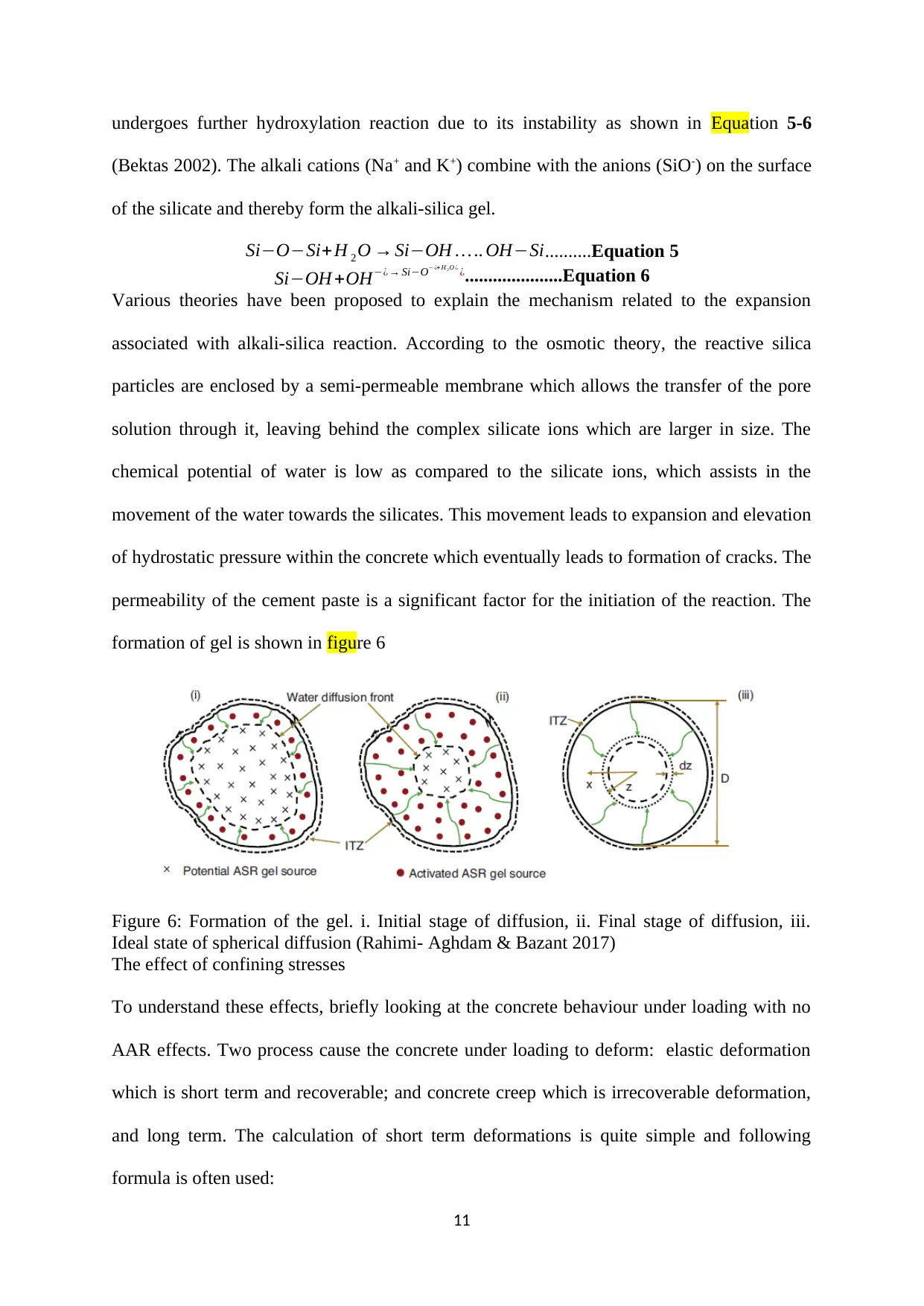
undergoes further hydroxylation reaction due to its instability as shown in Equation 5-6
(Bektas 2002). The alkali cations (Na+ and K+) combine with the anions (SiO-) on the surface
of the silicate and thereby form the alkali-silica gel.
Si−O−Si+H 2 O → Si−OH ….. OH−Si..........Equation 5
Si−OH +OH−¿→ Si−O−¿+ H 2O ¿ ¿.....................Equation 6
Various theories have been proposed to explain the mechanism related to the expansion
associated with alkali-silica reaction. According to the osmotic theory, the reactive silica
particles are enclosed by a semi-permeable membrane which allows the transfer of the pore
solution through it, leaving behind the complex silicate ions which are larger in size. The
chemical potential of water is low as compared to the silicate ions, which assists in the
movement of the water towards the silicates. This movement leads to expansion and elevation
of hydrostatic pressure within the concrete which eventually leads to formation of cracks. The
permeability of the cement paste is a significant factor for the initiation of the reaction. The
formation of gel is shown in figure 6
Figure 6: Formation of the gel. i. Initial stage of diffusion, ii. Final stage of diffusion, iii.
Ideal state of spherical diffusion (Rahimi- Aghdam & Bazant 2017)
The effect of confining stresses
To understand these effects, briefly looking at the concrete behaviour under loading with no
AAR effects. Two process cause the concrete under loading to deform: elastic deformation
which is short term and recoverable; and concrete creep which is irrecoverable deformation,
and long term. The calculation of short term deformations is quite simple and following
formula is often used:
11
(Bektas 2002). The alkali cations (Na+ and K+) combine with the anions (SiO-) on the surface
of the silicate and thereby form the alkali-silica gel.
Si−O−Si+H 2 O → Si−OH ….. OH−Si..........Equation 5
Si−OH +OH−¿→ Si−O−¿+ H 2O ¿ ¿.....................Equation 6
Various theories have been proposed to explain the mechanism related to the expansion
associated with alkali-silica reaction. According to the osmotic theory, the reactive silica
particles are enclosed by a semi-permeable membrane which allows the transfer of the pore
solution through it, leaving behind the complex silicate ions which are larger in size. The
chemical potential of water is low as compared to the silicate ions, which assists in the
movement of the water towards the silicates. This movement leads to expansion and elevation
of hydrostatic pressure within the concrete which eventually leads to formation of cracks. The
permeability of the cement paste is a significant factor for the initiation of the reaction. The
formation of gel is shown in figure 6
Figure 6: Formation of the gel. i. Initial stage of diffusion, ii. Final stage of diffusion, iii.
Ideal state of spherical diffusion (Rahimi- Aghdam & Bazant 2017)
The effect of confining stresses
To understand these effects, briefly looking at the concrete behaviour under loading with no
AAR effects. Two process cause the concrete under loading to deform: elastic deformation
which is short term and recoverable; and concrete creep which is irrecoverable deformation,
and long term. The calculation of short term deformations is quite simple and following
formula is often used:
11
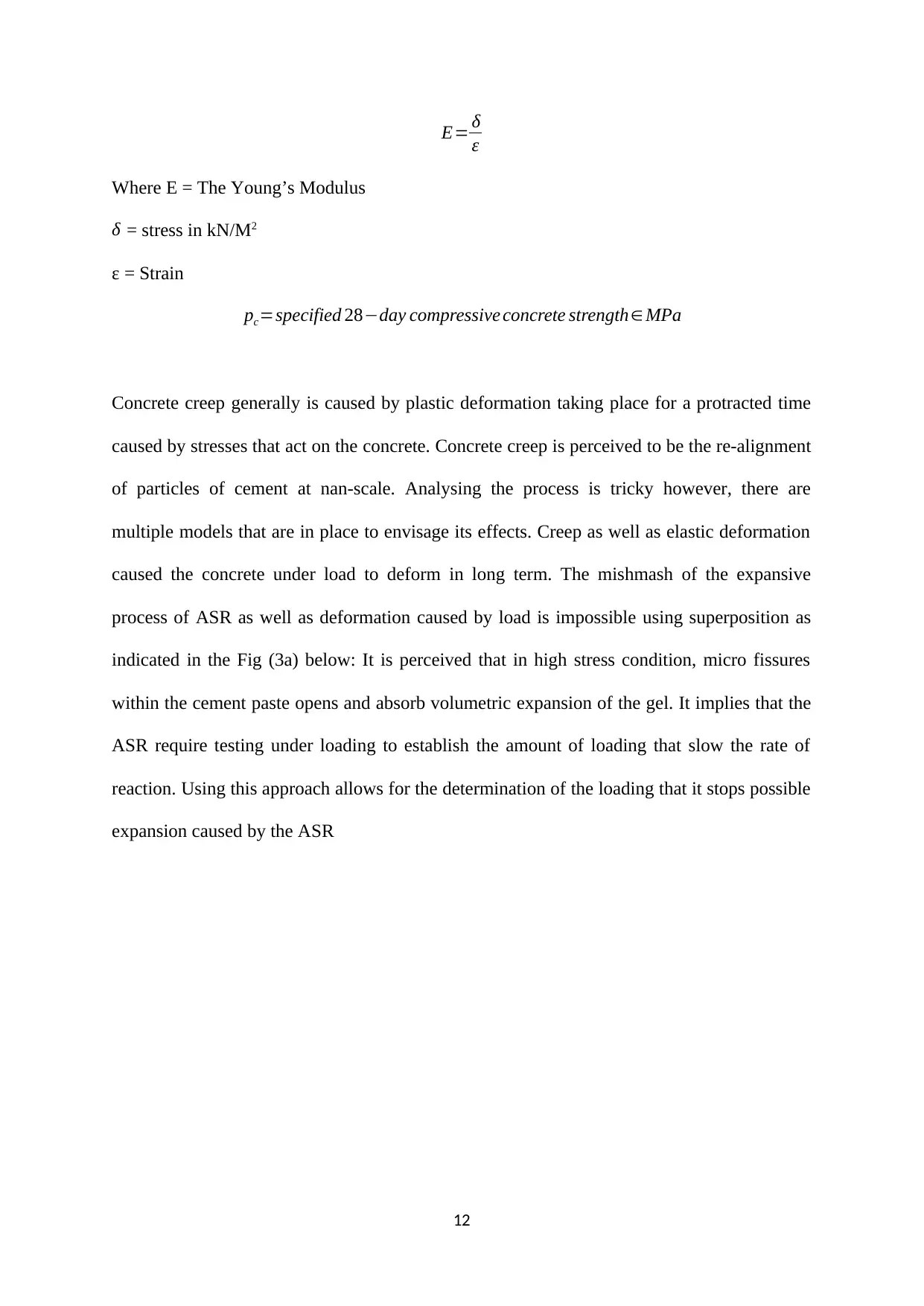
E= δ
ε
Where E = The Young’s Modulus
δ = stress in kN/M2
ε = Strain
pc=specified 28−day compressive concrete strength∈MPa
Concrete creep generally is caused by plastic deformation taking place for a protracted time
caused by stresses that act on the concrete. Concrete creep is perceived to be the re-alignment
of particles of cement at nan-scale. Analysing the process is tricky however, there are
multiple models that are in place to envisage its effects. Creep as well as elastic deformation
caused the concrete under load to deform in long term. The mishmash of the expansive
process of ASR as well as deformation caused by load is impossible using superposition as
indicated in the Fig (3a) below: It is perceived that in high stress condition, micro fissures
within the cement paste opens and absorb volumetric expansion of the gel. It implies that the
ASR require testing under loading to establish the amount of loading that slow the rate of
reaction. Using this approach allows for the determination of the loading that it stops possible
expansion caused by the ASR
12
ε
Where E = The Young’s Modulus
δ = stress in kN/M2
ε = Strain
pc=specified 28−day compressive concrete strength∈MPa
Concrete creep generally is caused by plastic deformation taking place for a protracted time
caused by stresses that act on the concrete. Concrete creep is perceived to be the re-alignment
of particles of cement at nan-scale. Analysing the process is tricky however, there are
multiple models that are in place to envisage its effects. Creep as well as elastic deformation
caused the concrete under load to deform in long term. The mishmash of the expansive
process of ASR as well as deformation caused by load is impossible using superposition as
indicated in the Fig (3a) below: It is perceived that in high stress condition, micro fissures
within the cement paste opens and absorb volumetric expansion of the gel. It implies that the
ASR require testing under loading to establish the amount of loading that slow the rate of
reaction. Using this approach allows for the determination of the loading that it stops possible
expansion caused by the ASR
12
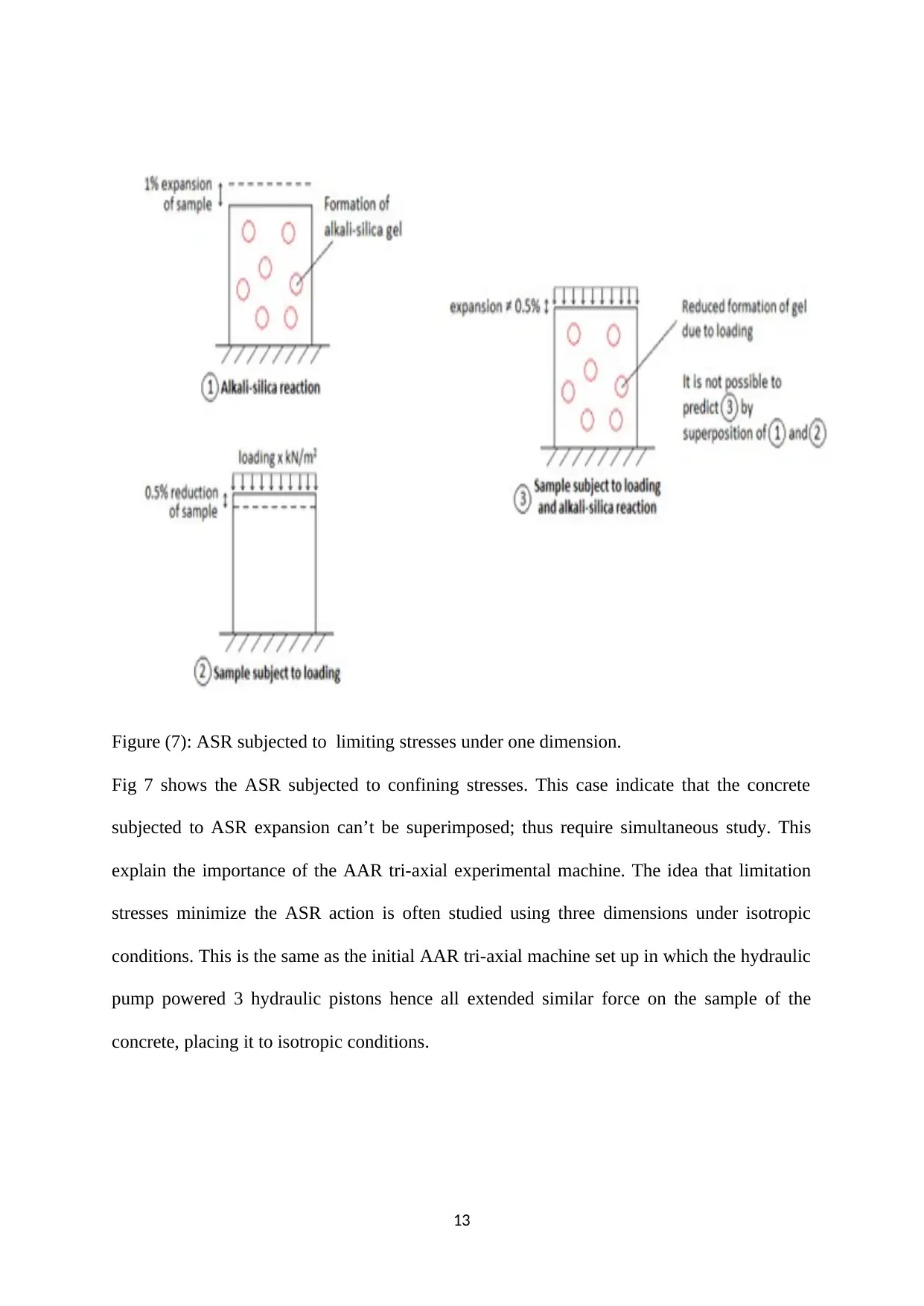
Figure (7): ASR subjected to limiting stresses under one dimension.
Fig 7 shows the ASR subjected to confining stresses. This case indicate that the concrete
subjected to ASR expansion can’t be superimposed; thus require simultaneous study. This
explain the importance of the AAR tri-axial experimental machine. The idea that limitation
stresses minimize the ASR action is often studied using three dimensions under isotropic
conditions. This is the same as the initial AAR tri-axial machine set up in which the hydraulic
pump powered 3 hydraulic pistons hence all extended similar force on the sample of the
concrete, placing it to isotropic conditions.
13
Fig 7 shows the ASR subjected to confining stresses. This case indicate that the concrete
subjected to ASR expansion can’t be superimposed; thus require simultaneous study. This
explain the importance of the AAR tri-axial experimental machine. The idea that limitation
stresses minimize the ASR action is often studied using three dimensions under isotropic
conditions. This is the same as the initial AAR tri-axial machine set up in which the hydraulic
pump powered 3 hydraulic pistons hence all extended similar force on the sample of the
concrete, placing it to isotropic conditions.
13
Paraphrase This Document
Need a fresh take? Get an instant paraphrase of this document with our AI Paraphraser
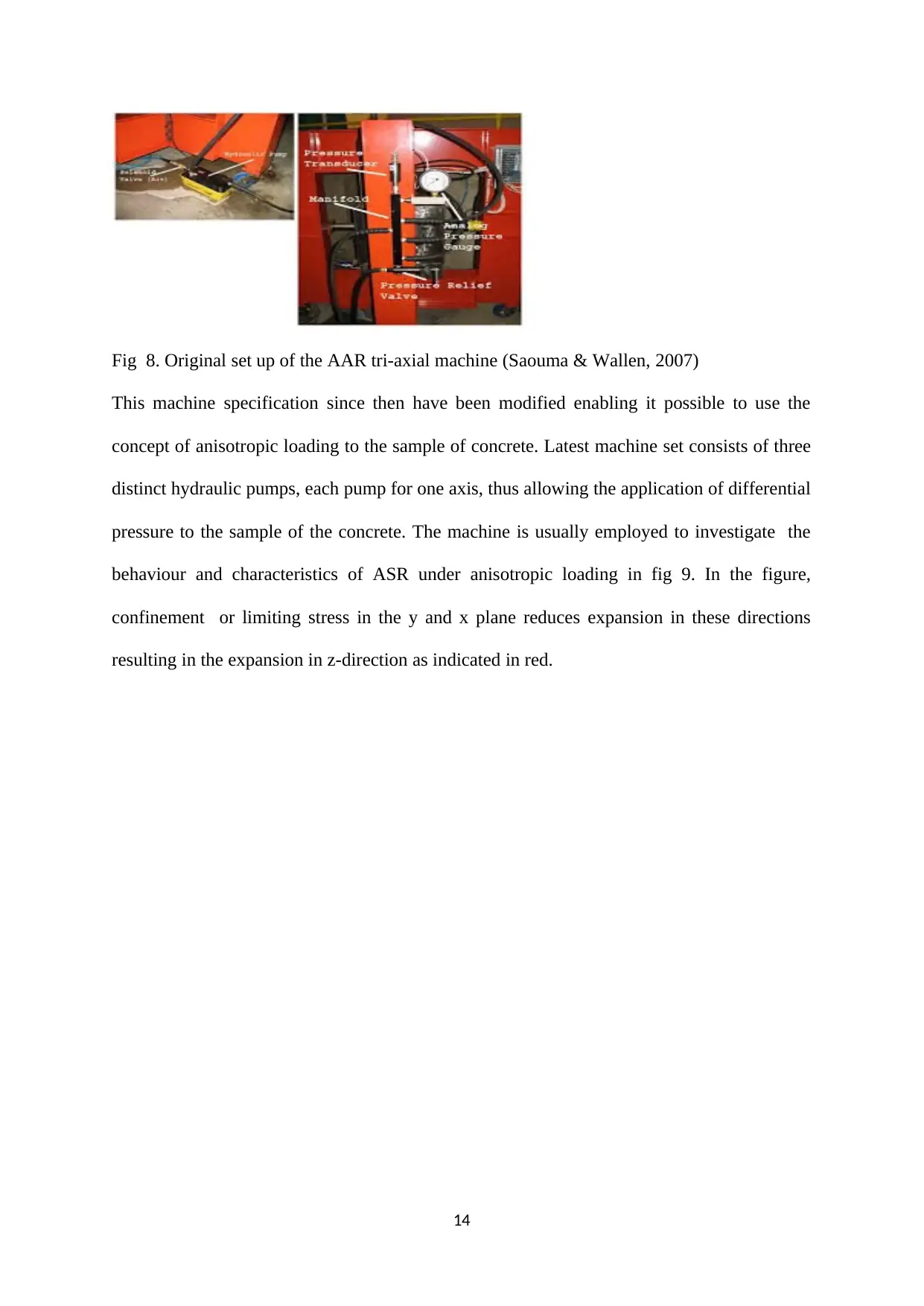
Fig 8. Original set up of the AAR tri-axial machine (Saouma & Wallen, 2007)
This machine specification since then have been modified enabling it possible to use the
concept of anisotropic loading to the sample of concrete. Latest machine set consists of three
distinct hydraulic pumps, each pump for one axis, thus allowing the application of differential
pressure to the sample of the concrete. The machine is usually employed to investigate the
behaviour and characteristics of ASR under anisotropic loading in fig 9. In the figure,
confinement or limiting stress in the y and x plane reduces expansion in these directions
resulting in the expansion in z-direction as indicated in red.
14
This machine specification since then have been modified enabling it possible to use the
concept of anisotropic loading to the sample of concrete. Latest machine set consists of three
distinct hydraulic pumps, each pump for one axis, thus allowing the application of differential
pressure to the sample of the concrete. The machine is usually employed to investigate the
behaviour and characteristics of ASR under anisotropic loading in fig 9. In the figure,
confinement or limiting stress in the y and x plane reduces expansion in these directions
resulting in the expansion in z-direction as indicated in red.
14
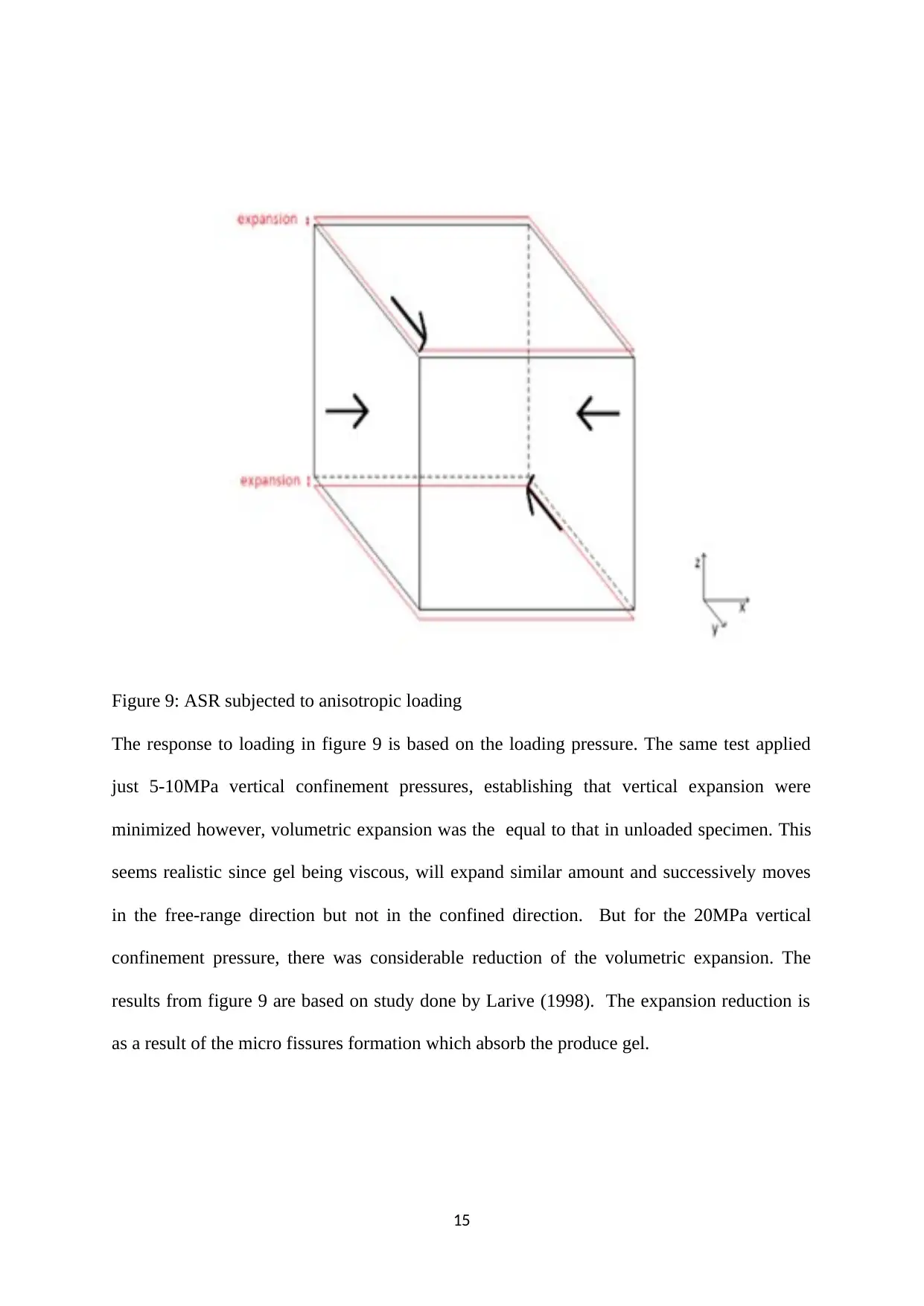
Figure 9: ASR subjected to anisotropic loading
The response to loading in figure 9 is based on the loading pressure. The same test applied
just 5-10MPa vertical confinement pressures, establishing that vertical expansion were
minimized however, volumetric expansion was the equal to that in unloaded specimen. This
seems realistic since gel being viscous, will expand similar amount and successively moves
in the free-range direction but not in the confined direction. But for the 20MPa vertical
confinement pressure, there was considerable reduction of the volumetric expansion. The
results from figure 9 are based on study done by Larive (1998). The expansion reduction is
as a result of the micro fissures formation which absorb the produce gel.
15
The response to loading in figure 9 is based on the loading pressure. The same test applied
just 5-10MPa vertical confinement pressures, establishing that vertical expansion were
minimized however, volumetric expansion was the equal to that in unloaded specimen. This
seems realistic since gel being viscous, will expand similar amount and successively moves
in the free-range direction but not in the confined direction. But for the 20MPa vertical
confinement pressure, there was considerable reduction of the volumetric expansion. The
results from figure 9 are based on study done by Larive (1998). The expansion reduction is
as a result of the micro fissures formation which absorb the produce gel.
15
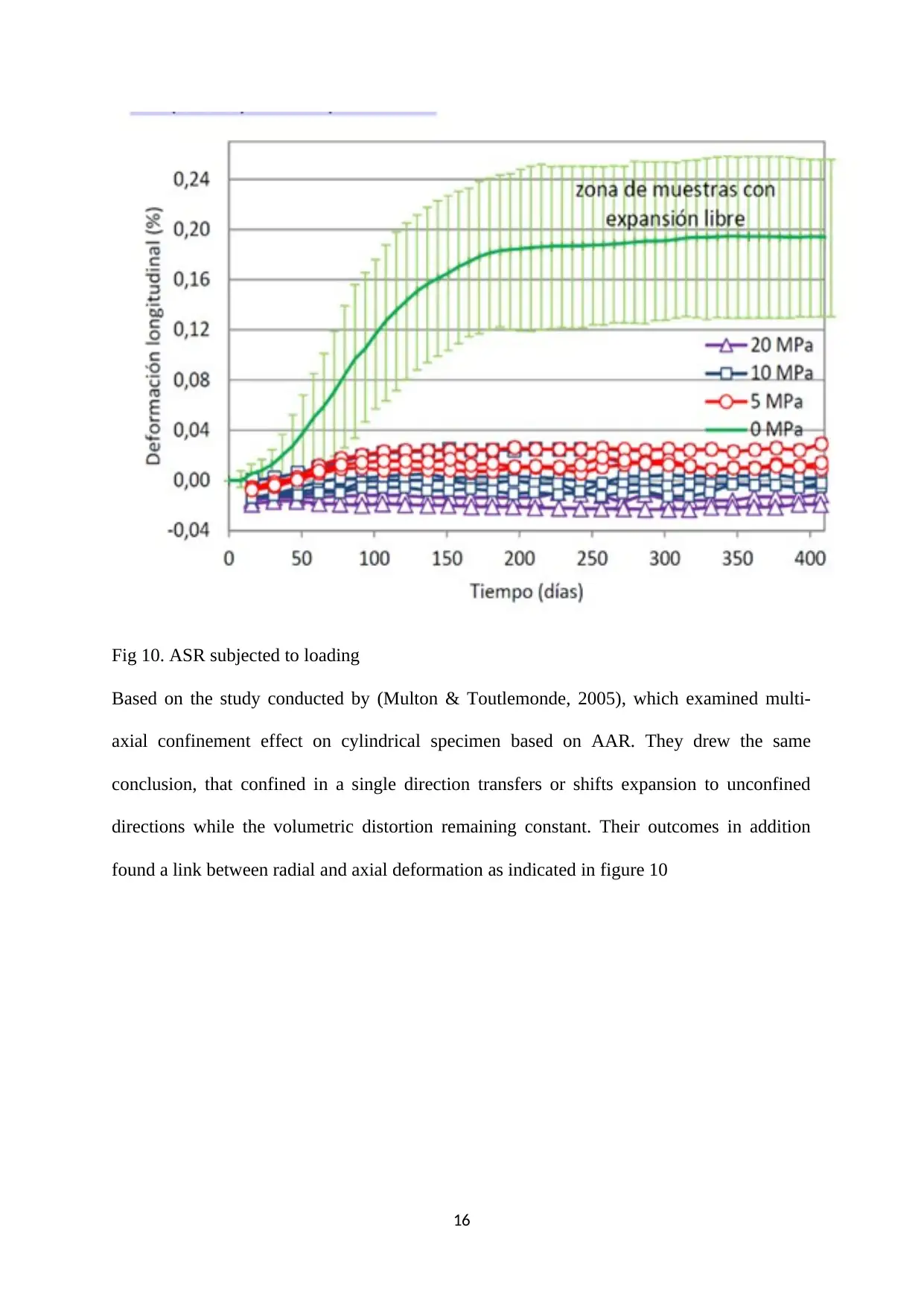
Fig 10. ASR subjected to loading
Based on the study conducted by (Multon & Toutlemonde, 2005), which examined multi-
axial confinement effect on cylindrical specimen based on AAR. They drew the same
conclusion, that confined in a single direction transfers or shifts expansion to unconfined
directions while the volumetric distortion remaining constant. Their outcomes in addition
found a link between radial and axial deformation as indicated in figure 10
16
Based on the study conducted by (Multon & Toutlemonde, 2005), which examined multi-
axial confinement effect on cylindrical specimen based on AAR. They drew the same
conclusion, that confined in a single direction transfers or shifts expansion to unconfined
directions while the volumetric distortion remaining constant. Their outcomes in addition
found a link between radial and axial deformation as indicated in figure 10
16
Secure Best Marks with AI Grader
Need help grading? Try our AI Grader for instant feedback on your assignments.
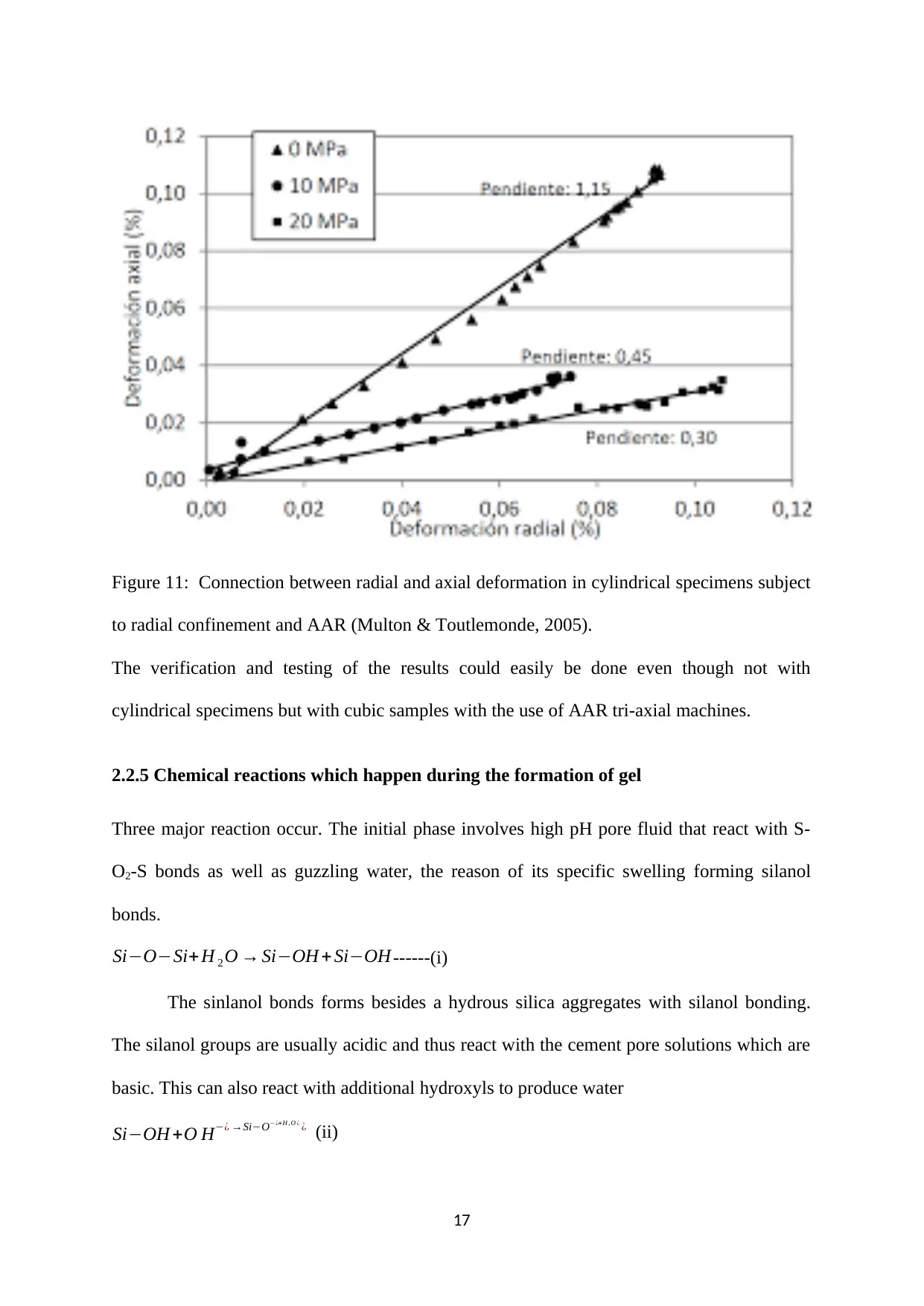
Figure 11: Connection between radial and axial deformation in cylindrical specimens subject
to radial confinement and AAR (Multon & Toutlemonde, 2005).
The verification and testing of the results could easily be done even though not with
cylindrical specimens but with cubic samples with the use of AAR tri-axial machines.
2.2.5 Chemical reactions which happen during the formation of gel
Three major reaction occur. The initial phase involves high pH pore fluid that react with S-
O2-S bonds as well as guzzling water, the reason of its specific swelling forming silanol
bonds.
Si−O−Si+ H 2 O → Si−OH + Si−OH ------(i)
The sinlanol bonds forms besides a hydrous silica aggregates with silanol bonding.
The silanol groups are usually acidic and thus react with the cement pore solutions which are
basic. This can also react with additional hydroxyls to produce water
Si−OH +O H−¿ →Si−O−¿+H s O ¿ ¿ (ii)
17
to radial confinement and AAR (Multon & Toutlemonde, 2005).
The verification and testing of the results could easily be done even though not with
cylindrical specimens but with cubic samples with the use of AAR tri-axial machines.
2.2.5 Chemical reactions which happen during the formation of gel
Three major reaction occur. The initial phase involves high pH pore fluid that react with S-
O2-S bonds as well as guzzling water, the reason of its specific swelling forming silanol
bonds.
Si−O−Si+ H 2 O → Si−OH + Si−OH ------(i)
The sinlanol bonds forms besides a hydrous silica aggregates with silanol bonding.
The silanol groups are usually acidic and thus react with the cement pore solutions which are
basic. This can also react with additional hydroxyls to produce water
Si−OH +O H−¿ →Si−O−¿+H s O ¿ ¿ (ii)
17
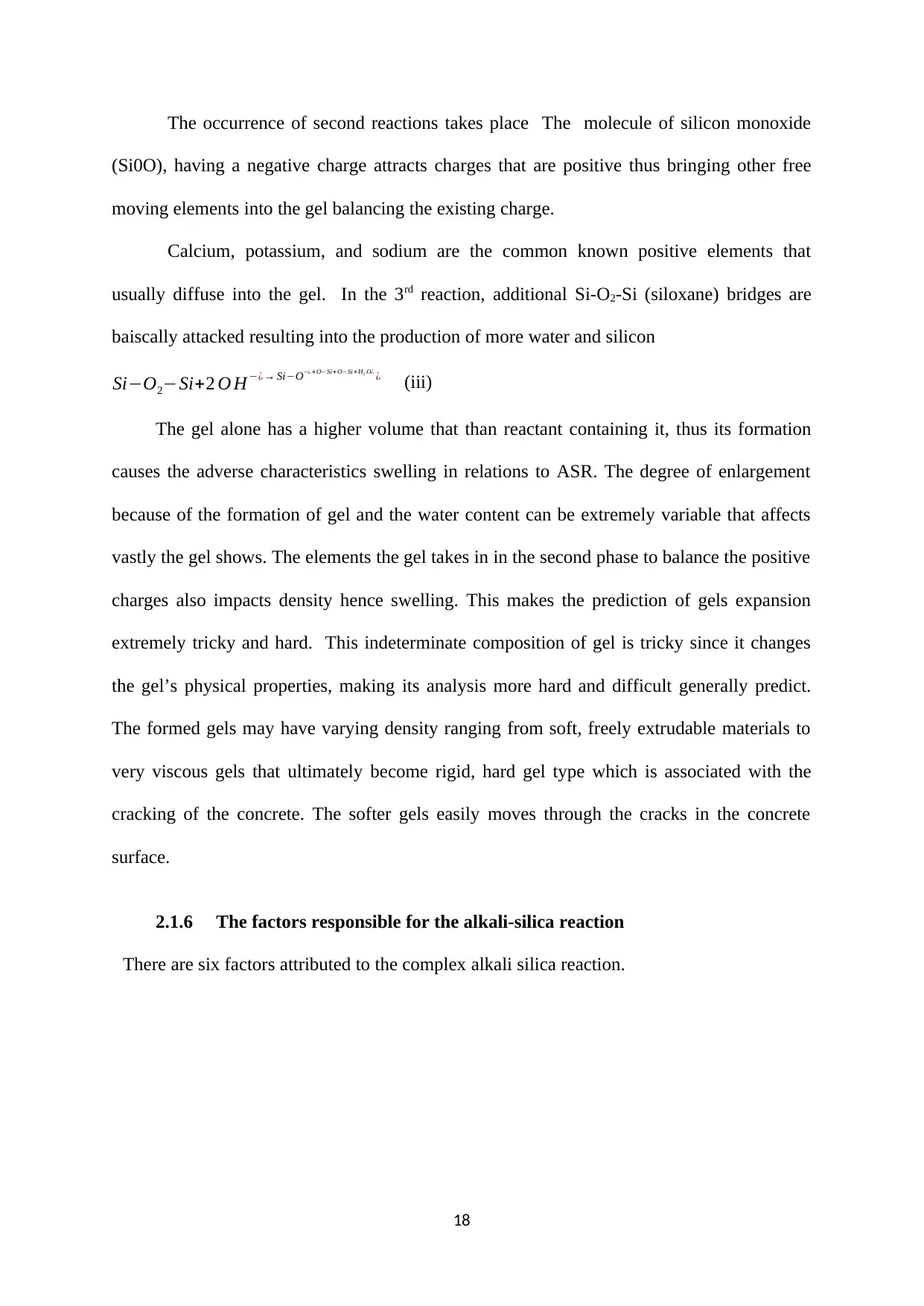
The occurrence of second reactions takes place The molecule of silicon monoxide
(Si0O), having a negative charge attracts charges that are positive thus bringing other free
moving elements into the gel balancing the existing charge.
Calcium, potassium, and sodium are the common known positive elements that
usually diffuse into the gel. In the 3rd reaction, additional Si-O2-Si (siloxane) bridges are
baiscally attacked resulting into the production of more water and silicon
Si−O2−Si+2 O H−¿→ Si−O−¿+ O−Si+ O−Si + H2 O¿ ¿ (iii)
The gel alone has a higher volume that than reactant containing it, thus its formation
causes the adverse characteristics swelling in relations to ASR. The degree of enlargement
because of the formation of gel and the water content can be extremely variable that affects
vastly the gel shows. The elements the gel takes in in the second phase to balance the positive
charges also impacts density hence swelling. This makes the prediction of gels expansion
extremely tricky and hard. This indeterminate composition of gel is tricky since it changes
the gel’s physical properties, making its analysis more hard and difficult generally predict.
The formed gels may have varying density ranging from soft, freely extrudable materials to
very viscous gels that ultimately become rigid, hard gel type which is associated with the
cracking of the concrete. The softer gels easily moves through the cracks in the concrete
surface.
2.1.6 The factors responsible for the alkali-silica reaction
There are six factors attributed to the complex alkali silica reaction.
18
(Si0O), having a negative charge attracts charges that are positive thus bringing other free
moving elements into the gel balancing the existing charge.
Calcium, potassium, and sodium are the common known positive elements that
usually diffuse into the gel. In the 3rd reaction, additional Si-O2-Si (siloxane) bridges are
baiscally attacked resulting into the production of more water and silicon
Si−O2−Si+2 O H−¿→ Si−O−¿+ O−Si+ O−Si + H2 O¿ ¿ (iii)
The gel alone has a higher volume that than reactant containing it, thus its formation
causes the adverse characteristics swelling in relations to ASR. The degree of enlargement
because of the formation of gel and the water content can be extremely variable that affects
vastly the gel shows. The elements the gel takes in in the second phase to balance the positive
charges also impacts density hence swelling. This makes the prediction of gels expansion
extremely tricky and hard. This indeterminate composition of gel is tricky since it changes
the gel’s physical properties, making its analysis more hard and difficult generally predict.
The formed gels may have varying density ranging from soft, freely extrudable materials to
very viscous gels that ultimately become rigid, hard gel type which is associated with the
cracking of the concrete. The softer gels easily moves through the cracks in the concrete
surface.
2.1.6 The factors responsible for the alkali-silica reaction
There are six factors attributed to the complex alkali silica reaction.
18
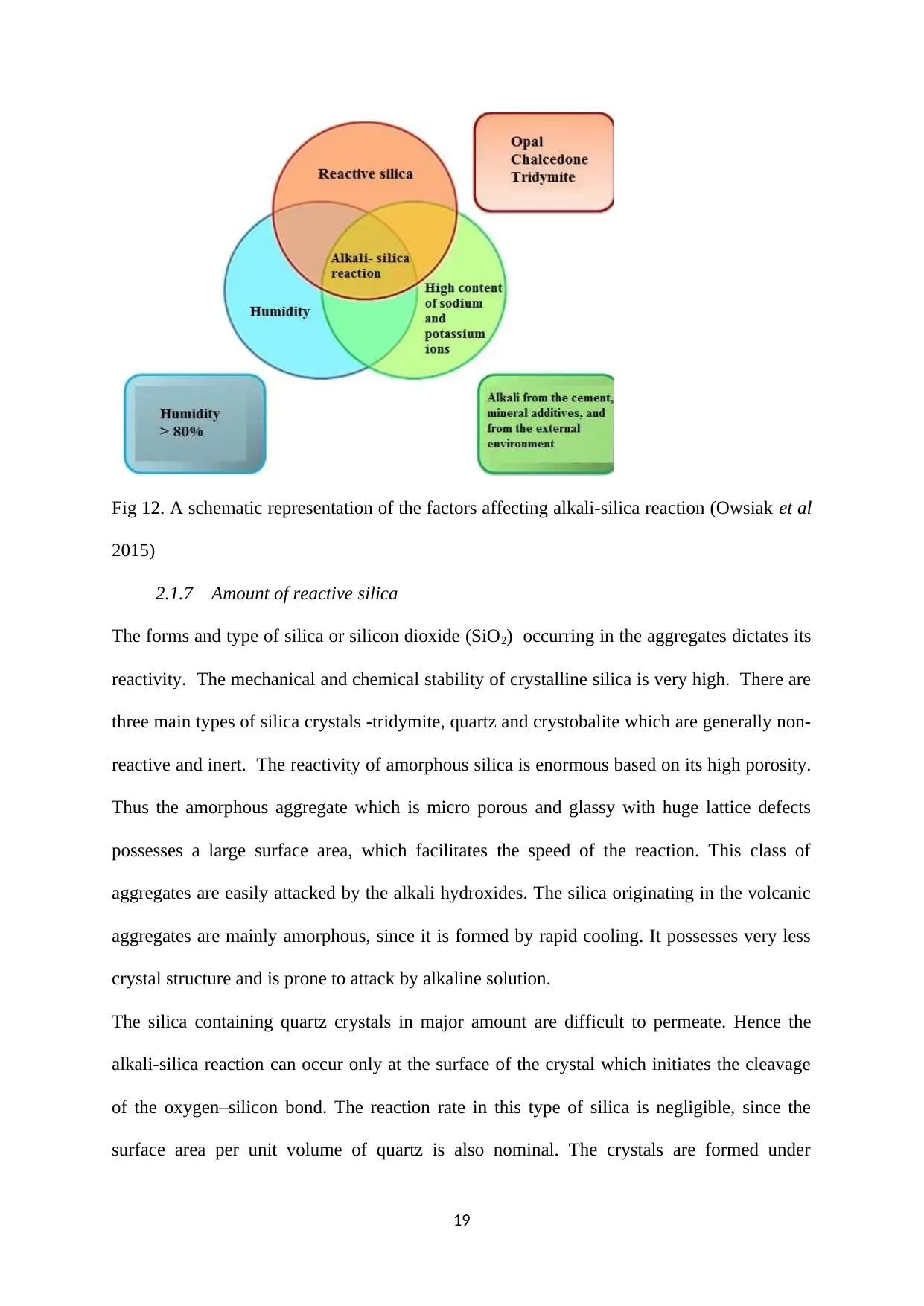
Fig 12. A schematic representation of the factors affecting alkali-silica reaction (Owsiak et al
2015)
2.1.7 Amount of reactive silica
The forms and type of silica or silicon dioxide (SiO2) occurring in the aggregates dictates its
reactivity. The mechanical and chemical stability of crystalline silica is very high. There are
three main types of silica crystals -tridymite, quartz and crystobalite which are generally non-
reactive and inert. The reactivity of amorphous silica is enormous based on its high porosity.
Thus the amorphous aggregate which is micro porous and glassy with huge lattice defects
possesses a large surface area, which facilitates the speed of the reaction. This class of
aggregates are easily attacked by the alkali hydroxides. The silica originating in the volcanic
aggregates are mainly amorphous, since it is formed by rapid cooling. It possesses very less
crystal structure and is prone to attack by alkaline solution.
The silica containing quartz crystals in major amount are difficult to permeate. Hence the
alkali-silica reaction can occur only at the surface of the crystal which initiates the cleavage
of the oxygen–silicon bond. The reaction rate in this type of silica is negligible, since the
surface area per unit volume of quartz is also nominal. The crystals are formed under
19
2015)
2.1.7 Amount of reactive silica
The forms and type of silica or silicon dioxide (SiO2) occurring in the aggregates dictates its
reactivity. The mechanical and chemical stability of crystalline silica is very high. There are
three main types of silica crystals -tridymite, quartz and crystobalite which are generally non-
reactive and inert. The reactivity of amorphous silica is enormous based on its high porosity.
Thus the amorphous aggregate which is micro porous and glassy with huge lattice defects
possesses a large surface area, which facilitates the speed of the reaction. This class of
aggregates are easily attacked by the alkali hydroxides. The silica originating in the volcanic
aggregates are mainly amorphous, since it is formed by rapid cooling. It possesses very less
crystal structure and is prone to attack by alkaline solution.
The silica containing quartz crystals in major amount are difficult to permeate. Hence the
alkali-silica reaction can occur only at the surface of the crystal which initiates the cleavage
of the oxygen–silicon bond. The reaction rate in this type of silica is negligible, since the
surface area per unit volume of quartz is also nominal. The crystals are formed under
19
Paraphrase This Document
Need a fresh take? Get an instant paraphrase of this document with our AI Paraphraser
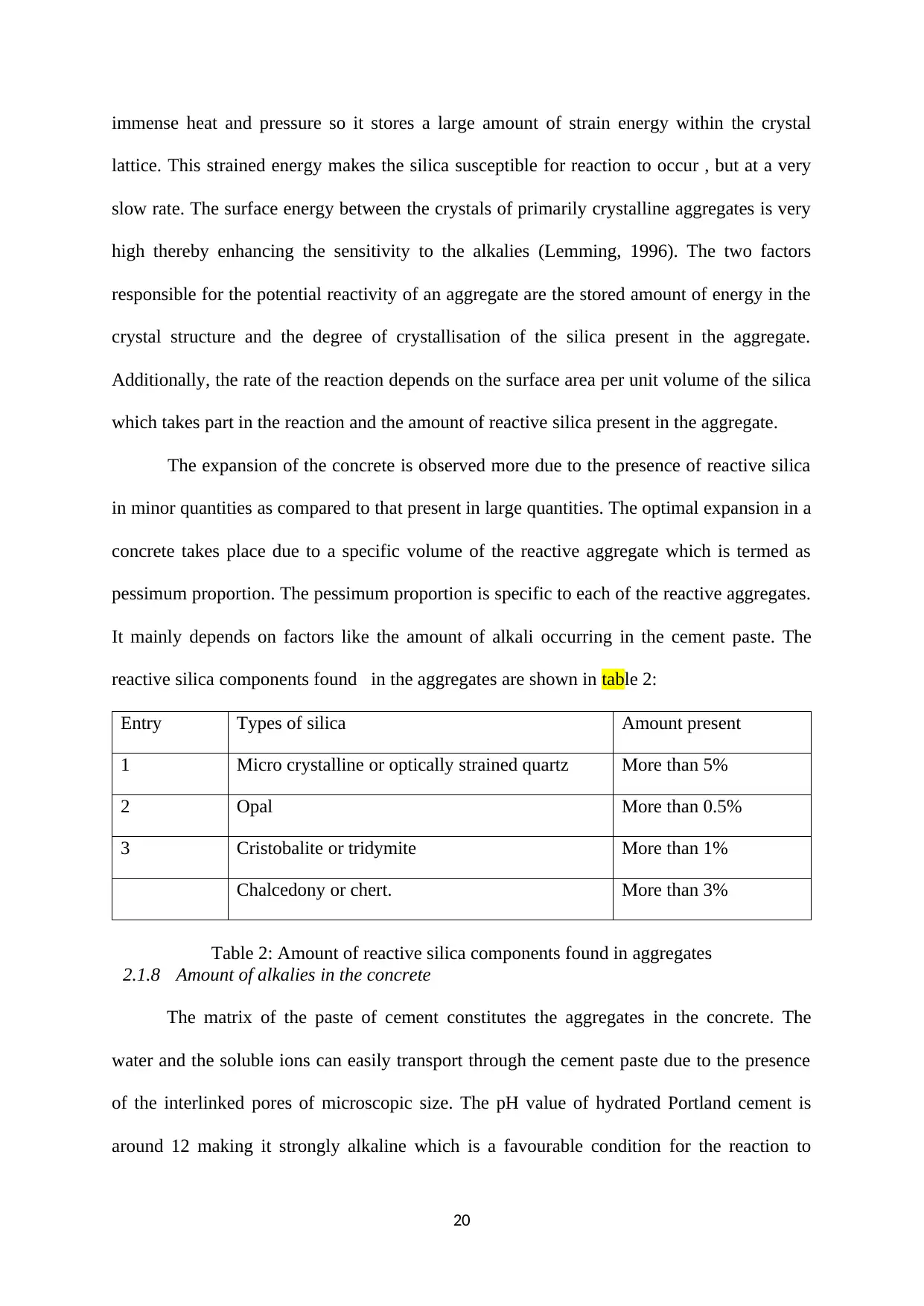
immense heat and pressure so it stores a large amount of strain energy within the crystal
lattice. This strained energy makes the silica susceptible for reaction to occur , but at a very
slow rate. The surface energy between the crystals of primarily crystalline aggregates is very
high thereby enhancing the sensitivity to the alkalies (Lemming, 1996). The two factors
responsible for the potential reactivity of an aggregate are the stored amount of energy in the
crystal structure and the degree of crystallisation of the silica present in the aggregate.
Additionally, the rate of the reaction depends on the surface area per unit volume of the silica
which takes part in the reaction and the amount of reactive silica present in the aggregate.
The expansion of the concrete is observed more due to the presence of reactive silica
in minor quantities as compared to that present in large quantities. The optimal expansion in a
concrete takes place due to a specific volume of the reactive aggregate which is termed as
pessimum proportion. The pessimum proportion is specific to each of the reactive aggregates.
It mainly depends on factors like the amount of alkali occurring in the cement paste. The
reactive silica components found in the aggregates are shown in table 2:
Entry Types of silica Amount present
1 Micro crystalline or optically strained quartz More than 5%
2 Opal More than 0.5%
3 Cristobalite or tridymite More than 1%
Chalcedony or chert. More than 3%
Table 2: Amount of reactive silica components found in aggregates
2.1.8 Amount of alkalies in the concrete
The matrix of the paste of cement constitutes the aggregates in the concrete. The
water and the soluble ions can easily transport through the cement paste due to the presence
of the interlinked pores of microscopic size. The pH value of hydrated Portland cement is
around 12 making it strongly alkaline which is a favourable condition for the reaction to
20
lattice. This strained energy makes the silica susceptible for reaction to occur , but at a very
slow rate. The surface energy between the crystals of primarily crystalline aggregates is very
high thereby enhancing the sensitivity to the alkalies (Lemming, 1996). The two factors
responsible for the potential reactivity of an aggregate are the stored amount of energy in the
crystal structure and the degree of crystallisation of the silica present in the aggregate.
Additionally, the rate of the reaction depends on the surface area per unit volume of the silica
which takes part in the reaction and the amount of reactive silica present in the aggregate.
The expansion of the concrete is observed more due to the presence of reactive silica
in minor quantities as compared to that present in large quantities. The optimal expansion in a
concrete takes place due to a specific volume of the reactive aggregate which is termed as
pessimum proportion. The pessimum proportion is specific to each of the reactive aggregates.
It mainly depends on factors like the amount of alkali occurring in the cement paste. The
reactive silica components found in the aggregates are shown in table 2:
Entry Types of silica Amount present
1 Micro crystalline or optically strained quartz More than 5%
2 Opal More than 0.5%
3 Cristobalite or tridymite More than 1%
Chalcedony or chert. More than 3%
Table 2: Amount of reactive silica components found in aggregates
2.1.8 Amount of alkalies in the concrete
The matrix of the paste of cement constitutes the aggregates in the concrete. The
water and the soluble ions can easily transport through the cement paste due to the presence
of the interlinked pores of microscopic size. The pH value of hydrated Portland cement is
around 12 making it strongly alkaline which is a favourable condition for the reaction to
20
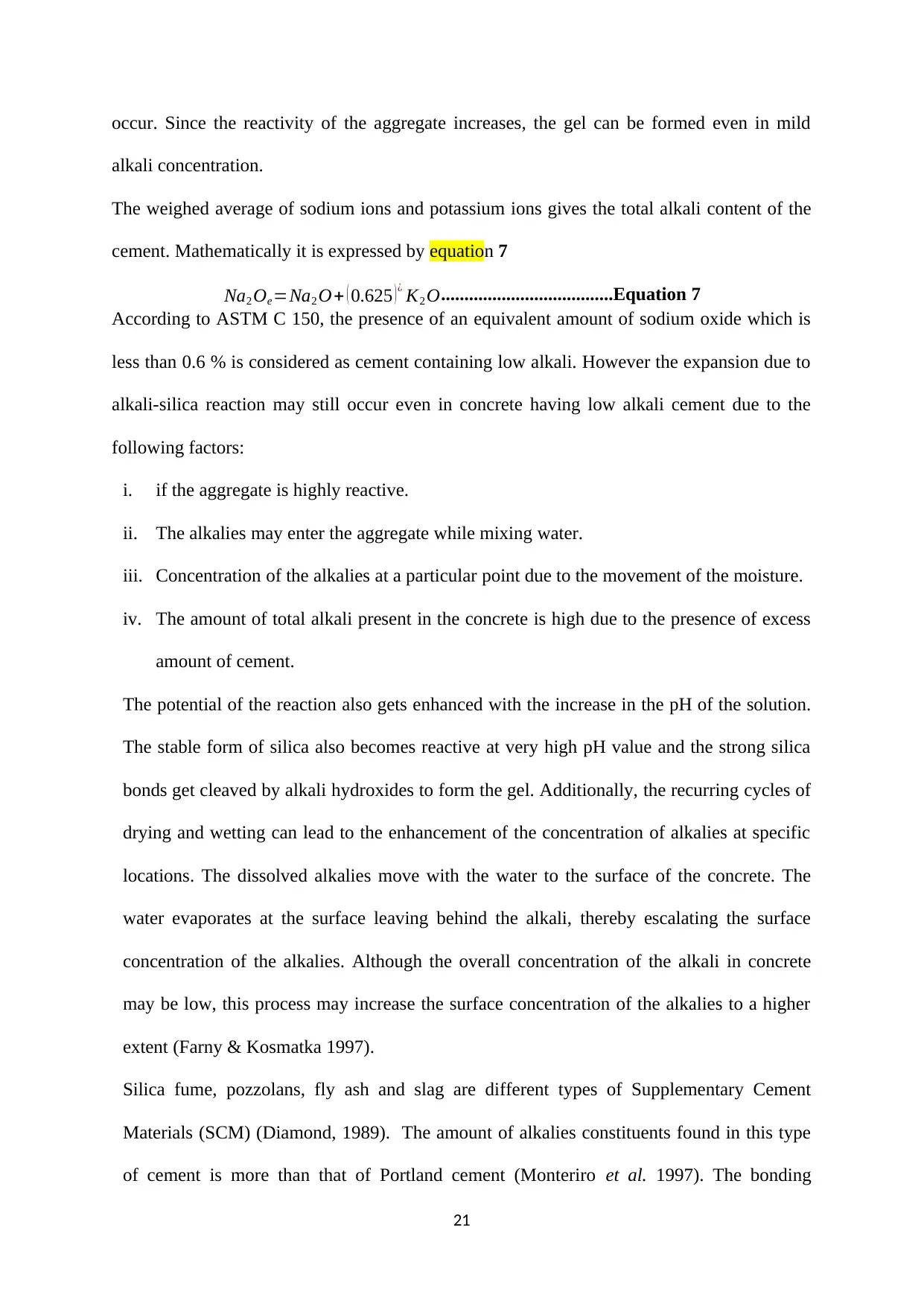
occur. Since the reactivity of the aggregate increases, the gel can be formed even in mild
alkali concentration.
The weighed average of sodium ions and potassium ions gives the total alkali content of the
cement. Mathematically it is expressed by equation 7
Na2 Oe=Na2 O+ ( 0.625 )¿ K2 O.....................................Equation 7
According to ASTM C 150, the presence of an equivalent amount of sodium oxide which is
less than 0.6 % is considered as cement containing low alkali. However the expansion due to
alkali-silica reaction may still occur even in concrete having low alkali cement due to the
following factors:
i. if the aggregate is highly reactive.
ii. The alkalies may enter the aggregate while mixing water.
iii. Concentration of the alkalies at a particular point due to the movement of the moisture.
iv. The amount of total alkali present in the concrete is high due to the presence of excess
amount of cement.
The potential of the reaction also gets enhanced with the increase in the pH of the solution.
The stable form of silica also becomes reactive at very high pH value and the strong silica
bonds get cleaved by alkali hydroxides to form the gel. Additionally, the recurring cycles of
drying and wetting can lead to the enhancement of the concentration of alkalies at specific
locations. The dissolved alkalies move with the water to the surface of the concrete. The
water evaporates at the surface leaving behind the alkali, thereby escalating the surface
concentration of the alkalies. Although the overall concentration of the alkali in concrete
may be low, this process may increase the surface concentration of the alkalies to a higher
extent (Farny & Kosmatka 1997).
Silica fume, pozzolans, fly ash and slag are different types of Supplementary Cement
Materials (SCM) (Diamond, 1989). The amount of alkalies constituents found in this type
of cement is more than that of Portland cement (Monteriro et al. 1997). The bonding
21
alkali concentration.
The weighed average of sodium ions and potassium ions gives the total alkali content of the
cement. Mathematically it is expressed by equation 7
Na2 Oe=Na2 O+ ( 0.625 )¿ K2 O.....................................Equation 7
According to ASTM C 150, the presence of an equivalent amount of sodium oxide which is
less than 0.6 % is considered as cement containing low alkali. However the expansion due to
alkali-silica reaction may still occur even in concrete having low alkali cement due to the
following factors:
i. if the aggregate is highly reactive.
ii. The alkalies may enter the aggregate while mixing water.
iii. Concentration of the alkalies at a particular point due to the movement of the moisture.
iv. The amount of total alkali present in the concrete is high due to the presence of excess
amount of cement.
The potential of the reaction also gets enhanced with the increase in the pH of the solution.
The stable form of silica also becomes reactive at very high pH value and the strong silica
bonds get cleaved by alkali hydroxides to form the gel. Additionally, the recurring cycles of
drying and wetting can lead to the enhancement of the concentration of alkalies at specific
locations. The dissolved alkalies move with the water to the surface of the concrete. The
water evaporates at the surface leaving behind the alkali, thereby escalating the surface
concentration of the alkalies. Although the overall concentration of the alkali in concrete
may be low, this process may increase the surface concentration of the alkalies to a higher
extent (Farny & Kosmatka 1997).
Silica fume, pozzolans, fly ash and slag are different types of Supplementary Cement
Materials (SCM) (Diamond, 1989). The amount of alkalies constituents found in this type
of cement is more than that of Portland cement (Monteriro et al. 1997). The bonding
21
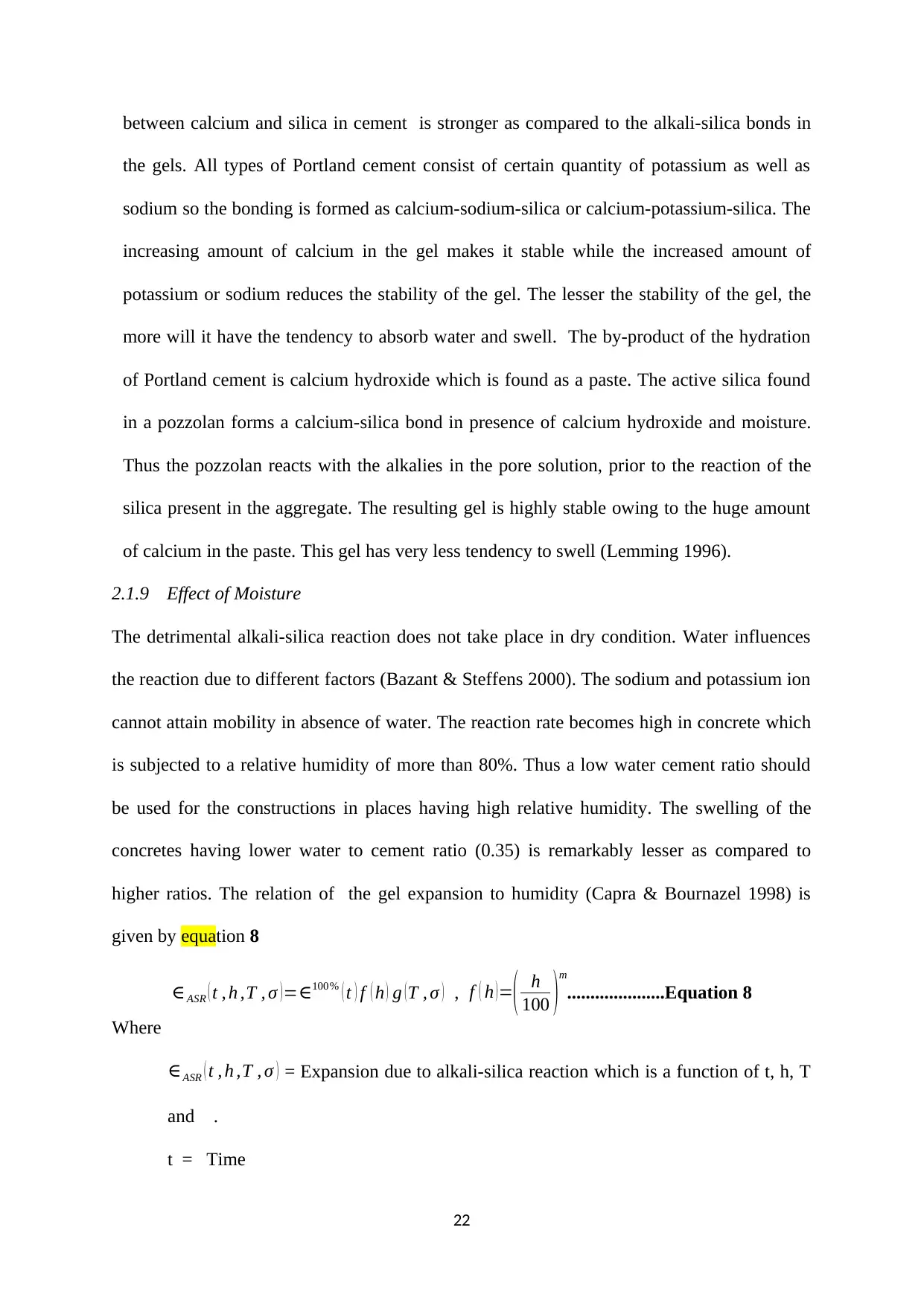
between calcium and silica in cement is stronger as compared to the alkali-silica bonds in
the gels. All types of Portland cement consist of certain quantity of potassium as well as
sodium so the bonding is formed as calcium-sodium-silica or calcium-potassium-silica. The
increasing amount of calcium in the gel makes it stable while the increased amount of
potassium or sodium reduces the stability of the gel. The lesser the stability of the gel, the
more will it have the tendency to absorb water and swell. The by-product of the hydration
of Portland cement is calcium hydroxide which is found as a paste. The active silica found
in a pozzolan forms a calcium-silica bond in presence of calcium hydroxide and moisture.
Thus the pozzolan reacts with the alkalies in the pore solution, prior to the reaction of the
silica present in the aggregate. The resulting gel is highly stable owing to the huge amount
of calcium in the paste. This gel has very less tendency to swell (Lemming 1996).
2.1.9 Effect of Moisture
The detrimental alkali-silica reaction does not take place in dry condition. Water influences
the reaction due to different factors (Bazant & Steffens 2000). The sodium and potassium ion
cannot attain mobility in absence of water. The reaction rate becomes high in concrete which
is subjected to a relative humidity of more than 80%. Thus a low water cement ratio should
be used for the constructions in places having high relative humidity. The swelling of the
concretes having lower water to cement ratio (0.35) is remarkably lesser as compared to
higher ratios. The relation of the gel expansion to humidity (Capra & Bournazel 1998) is
given by equation 8
∈ASR ( t , h ,T , σ ) =∈100% ( t ) f ( h ) g ( T , σ ) , f ( h ) =( h
100 )
m
.....................Equation 8
Where
∈ASR ( t , h ,T , σ ) = Expansion due to alkali-silica reaction which is a function of t, h, T
and .
t = Time
22
the gels. All types of Portland cement consist of certain quantity of potassium as well as
sodium so the bonding is formed as calcium-sodium-silica or calcium-potassium-silica. The
increasing amount of calcium in the gel makes it stable while the increased amount of
potassium or sodium reduces the stability of the gel. The lesser the stability of the gel, the
more will it have the tendency to absorb water and swell. The by-product of the hydration
of Portland cement is calcium hydroxide which is found as a paste. The active silica found
in a pozzolan forms a calcium-silica bond in presence of calcium hydroxide and moisture.
Thus the pozzolan reacts with the alkalies in the pore solution, prior to the reaction of the
silica present in the aggregate. The resulting gel is highly stable owing to the huge amount
of calcium in the paste. This gel has very less tendency to swell (Lemming 1996).
2.1.9 Effect of Moisture
The detrimental alkali-silica reaction does not take place in dry condition. Water influences
the reaction due to different factors (Bazant & Steffens 2000). The sodium and potassium ion
cannot attain mobility in absence of water. The reaction rate becomes high in concrete which
is subjected to a relative humidity of more than 80%. Thus a low water cement ratio should
be used for the constructions in places having high relative humidity. The swelling of the
concretes having lower water to cement ratio (0.35) is remarkably lesser as compared to
higher ratios. The relation of the gel expansion to humidity (Capra & Bournazel 1998) is
given by equation 8
∈ASR ( t , h ,T , σ ) =∈100% ( t ) f ( h ) g ( T , σ ) , f ( h ) =( h
100 )
m
.....................Equation 8
Where
∈ASR ( t , h ,T , σ ) = Expansion due to alkali-silica reaction which is a function of t, h, T
and .
t = Time
22
Secure Best Marks with AI Grader
Need help grading? Try our AI Grader for instant feedback on your assignments.
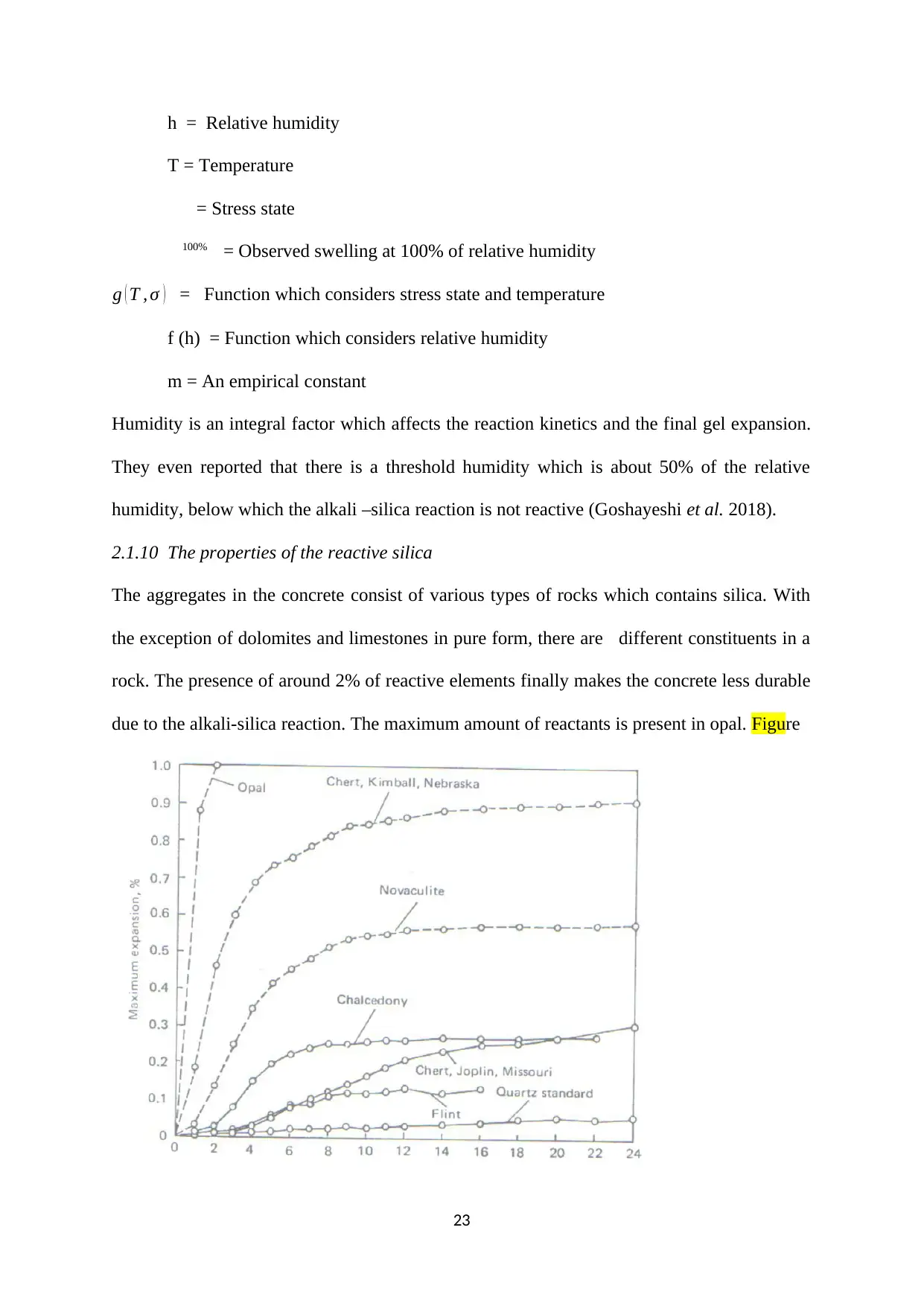
h = Relative humidity
T = Temperature
= Stress state
100% = Observed swelling at 100% of relative humidity
g ( T , σ ) = Function which considers stress state and temperature
f (h) = Function which considers relative humidity
m = An empirical constant
Humidity is an integral factor which affects the reaction kinetics and the final gel expansion.
They even reported that there is a threshold humidity which is about 50% of the relative
humidity, below which the alkali –silica reaction is not reactive (Goshayeshi et al. 2018).
2.1.10 The properties of the reactive silica
The aggregates in the concrete consist of various types of rocks which contains silica. With
the exception of dolomites and limestones in pure form, there are different constituents in a
rock. The presence of around 2% of reactive elements finally makes the concrete less durable
due to the alkali-silica reaction. The maximum amount of reactants is present in opal. Figure
23
T = Temperature
= Stress state
100% = Observed swelling at 100% of relative humidity
g ( T , σ ) = Function which considers stress state and temperature
f (h) = Function which considers relative humidity
m = An empirical constant
Humidity is an integral factor which affects the reaction kinetics and the final gel expansion.
They even reported that there is a threshold humidity which is about 50% of the relative
humidity, below which the alkali –silica reaction is not reactive (Goshayeshi et al. 2018).
2.1.10 The properties of the reactive silica
The aggregates in the concrete consist of various types of rocks which contains silica. With
the exception of dolomites and limestones in pure form, there are different constituents in a
rock. The presence of around 2% of reactive elements finally makes the concrete less durable
due to the alkali-silica reaction. The maximum amount of reactants is present in opal. Figure
23
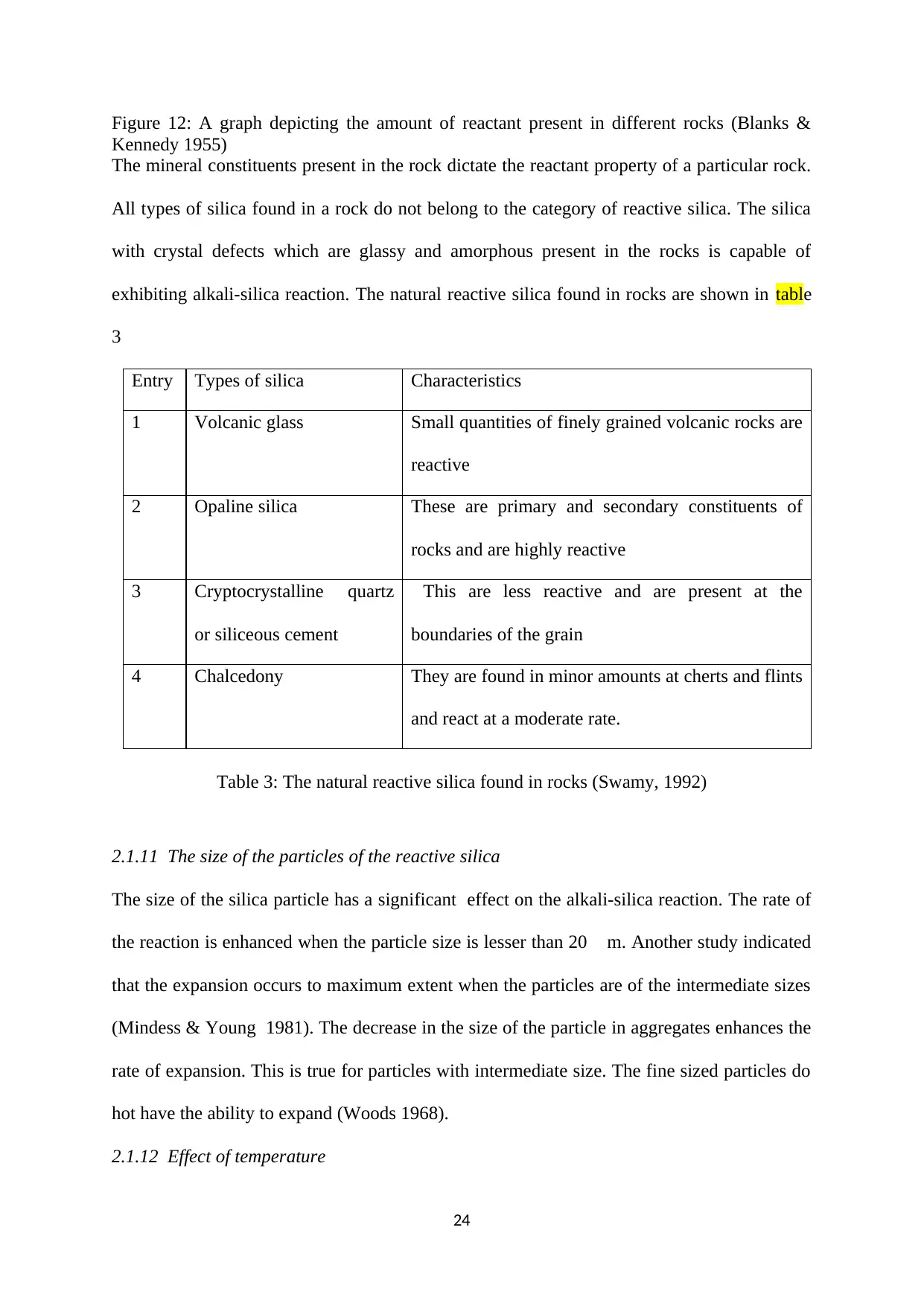
Figure 12: A graph depicting the amount of reactant present in different rocks (Blanks &
Kennedy 1955)
The mineral constituents present in the rock dictate the reactant property of a particular rock.
All types of silica found in a rock do not belong to the category of reactive silica. The silica
with crystal defects which are glassy and amorphous present in the rocks is capable of
exhibiting alkali-silica reaction. The natural reactive silica found in rocks are shown in table
3
Entry Types of silica Characteristics
1 Volcanic glass Small quantities of finely grained volcanic rocks are
reactive
2 Opaline silica These are primary and secondary constituents of
rocks and are highly reactive
3 Cryptocrystalline quartz
or siliceous cement
This are less reactive and are present at the
boundaries of the grain
4 Chalcedony They are found in minor amounts at cherts and flints
and react at a moderate rate.
Table 3: The natural reactive silica found in rocks (Swamy, 1992)
2.1.11 The size of the particles of the reactive silica
The size of the silica particle has a significant effect on the alkali-silica reaction. The rate of
the reaction is enhanced when the particle size is lesser than 20 m. Another study indicated
that the expansion occurs to maximum extent when the particles are of the intermediate sizes
(Mindess & Young 1981). The decrease in the size of the particle in aggregates enhances the
rate of expansion. This is true for particles with intermediate size. The fine sized particles do
hot have the ability to expand (Woods 1968).
2.1.12 Effect of temperature
24
Kennedy 1955)
The mineral constituents present in the rock dictate the reactant property of a particular rock.
All types of silica found in a rock do not belong to the category of reactive silica. The silica
with crystal defects which are glassy and amorphous present in the rocks is capable of
exhibiting alkali-silica reaction. The natural reactive silica found in rocks are shown in table
3
Entry Types of silica Characteristics
1 Volcanic glass Small quantities of finely grained volcanic rocks are
reactive
2 Opaline silica These are primary and secondary constituents of
rocks and are highly reactive
3 Cryptocrystalline quartz
or siliceous cement
This are less reactive and are present at the
boundaries of the grain
4 Chalcedony They are found in minor amounts at cherts and flints
and react at a moderate rate.
Table 3: The natural reactive silica found in rocks (Swamy, 1992)
2.1.11 The size of the particles of the reactive silica
The size of the silica particle has a significant effect on the alkali-silica reaction. The rate of
the reaction is enhanced when the particle size is lesser than 20 m. Another study indicated
that the expansion occurs to maximum extent when the particles are of the intermediate sizes
(Mindess & Young 1981). The decrease in the size of the particle in aggregates enhances the
rate of expansion. This is true for particles with intermediate size. The fine sized particles do
hot have the ability to expand (Woods 1968).
2.1.12 Effect of temperature
24
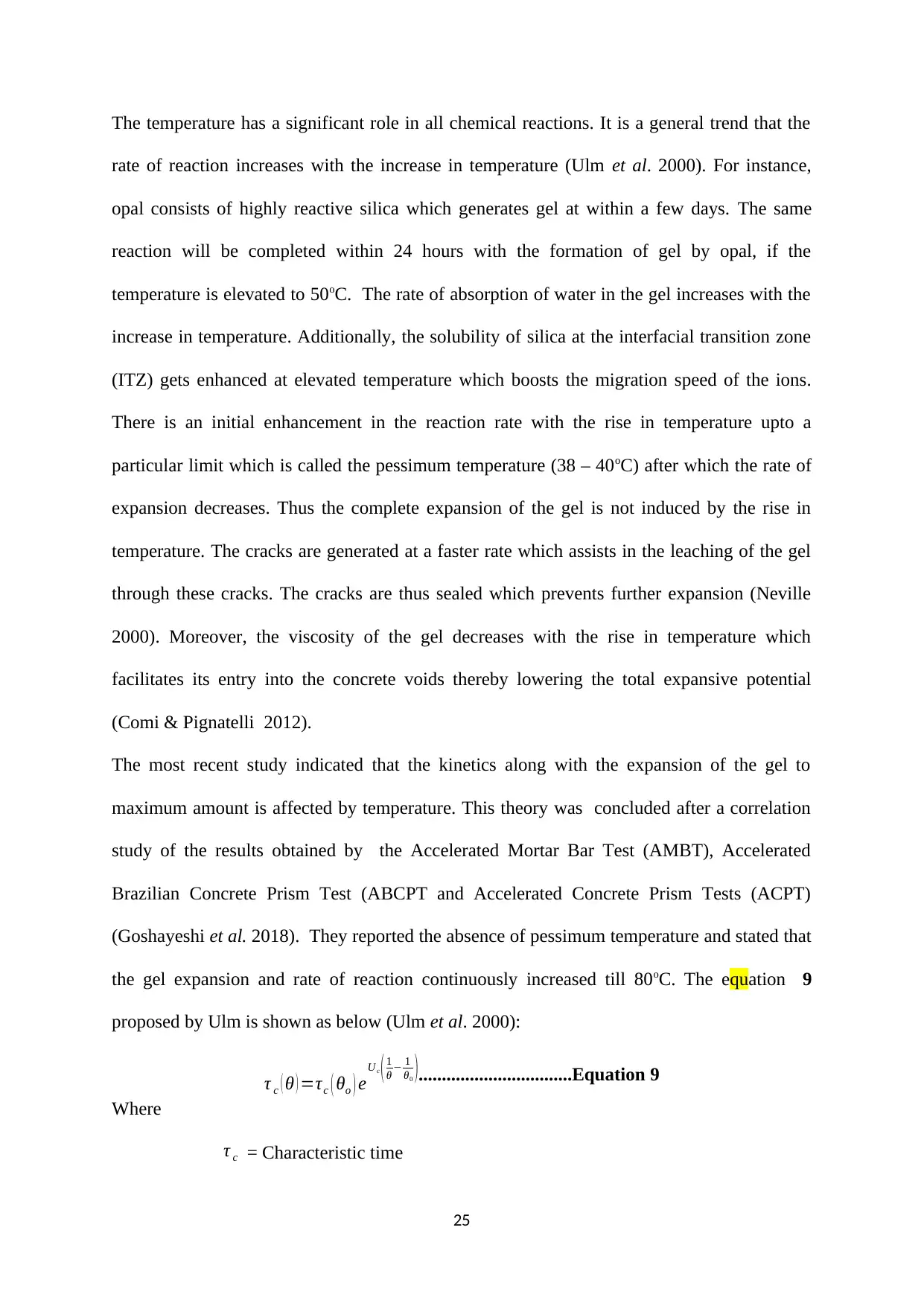
The temperature has a significant role in all chemical reactions. It is a general trend that the
rate of reaction increases with the increase in temperature (Ulm et al. 2000). For instance,
opal consists of highly reactive silica which generates gel at within a few days. The same
reaction will be completed within 24 hours with the formation of gel by opal, if the
temperature is elevated to 50oC. The rate of absorption of water in the gel increases with the
increase in temperature. Additionally, the solubility of silica at the interfacial transition zone
(ITZ) gets enhanced at elevated temperature which boosts the migration speed of the ions.
There is an initial enhancement in the reaction rate with the rise in temperature upto a
particular limit which is called the pessimum temperature (38 – 40oC) after which the rate of
expansion decreases. Thus the complete expansion of the gel is not induced by the rise in
temperature. The cracks are generated at a faster rate which assists in the leaching of the gel
through these cracks. The cracks are thus sealed which prevents further expansion (Neville
2000). Moreover, the viscosity of the gel decreases with the rise in temperature which
facilitates its entry into the concrete voids thereby lowering the total expansive potential
(Comi & Pignatelli 2012).
The most recent study indicated that the kinetics along with the expansion of the gel to
maximum amount is affected by temperature. This theory was concluded after a correlation
study of the results obtained by the Accelerated Mortar Bar Test (AMBT), Accelerated
Brazilian Concrete Prism Test (ABCPT and Accelerated Concrete Prism Tests (ACPT)
(Goshayeshi et al. 2018). They reported the absence of pessimum temperature and stated that
the gel expansion and rate of reaction continuously increased till 80oC. The equation 9
proposed by Ulm is shown as below (Ulm et al. 2000):
τ c ( θ ) =τc ( θo ) eU c ( 1
θ − 1
θ0 ).................................Equation 9
Where
τ c = Characteristic time
25
rate of reaction increases with the increase in temperature (Ulm et al. 2000). For instance,
opal consists of highly reactive silica which generates gel at within a few days. The same
reaction will be completed within 24 hours with the formation of gel by opal, if the
temperature is elevated to 50oC. The rate of absorption of water in the gel increases with the
increase in temperature. Additionally, the solubility of silica at the interfacial transition zone
(ITZ) gets enhanced at elevated temperature which boosts the migration speed of the ions.
There is an initial enhancement in the reaction rate with the rise in temperature upto a
particular limit which is called the pessimum temperature (38 – 40oC) after which the rate of
expansion decreases. Thus the complete expansion of the gel is not induced by the rise in
temperature. The cracks are generated at a faster rate which assists in the leaching of the gel
through these cracks. The cracks are thus sealed which prevents further expansion (Neville
2000). Moreover, the viscosity of the gel decreases with the rise in temperature which
facilitates its entry into the concrete voids thereby lowering the total expansive potential
(Comi & Pignatelli 2012).
The most recent study indicated that the kinetics along with the expansion of the gel to
maximum amount is affected by temperature. This theory was concluded after a correlation
study of the results obtained by the Accelerated Mortar Bar Test (AMBT), Accelerated
Brazilian Concrete Prism Test (ABCPT and Accelerated Concrete Prism Tests (ACPT)
(Goshayeshi et al. 2018). They reported the absence of pessimum temperature and stated that
the gel expansion and rate of reaction continuously increased till 80oC. The equation 9
proposed by Ulm is shown as below (Ulm et al. 2000):
τ c ( θ ) =τc ( θo ) eU c ( 1
θ − 1
θ0 ).................................Equation 9
Where
τ c = Characteristic time
25
Paraphrase This Document
Need a fresh take? Get an instant paraphrase of this document with our AI Paraphraser
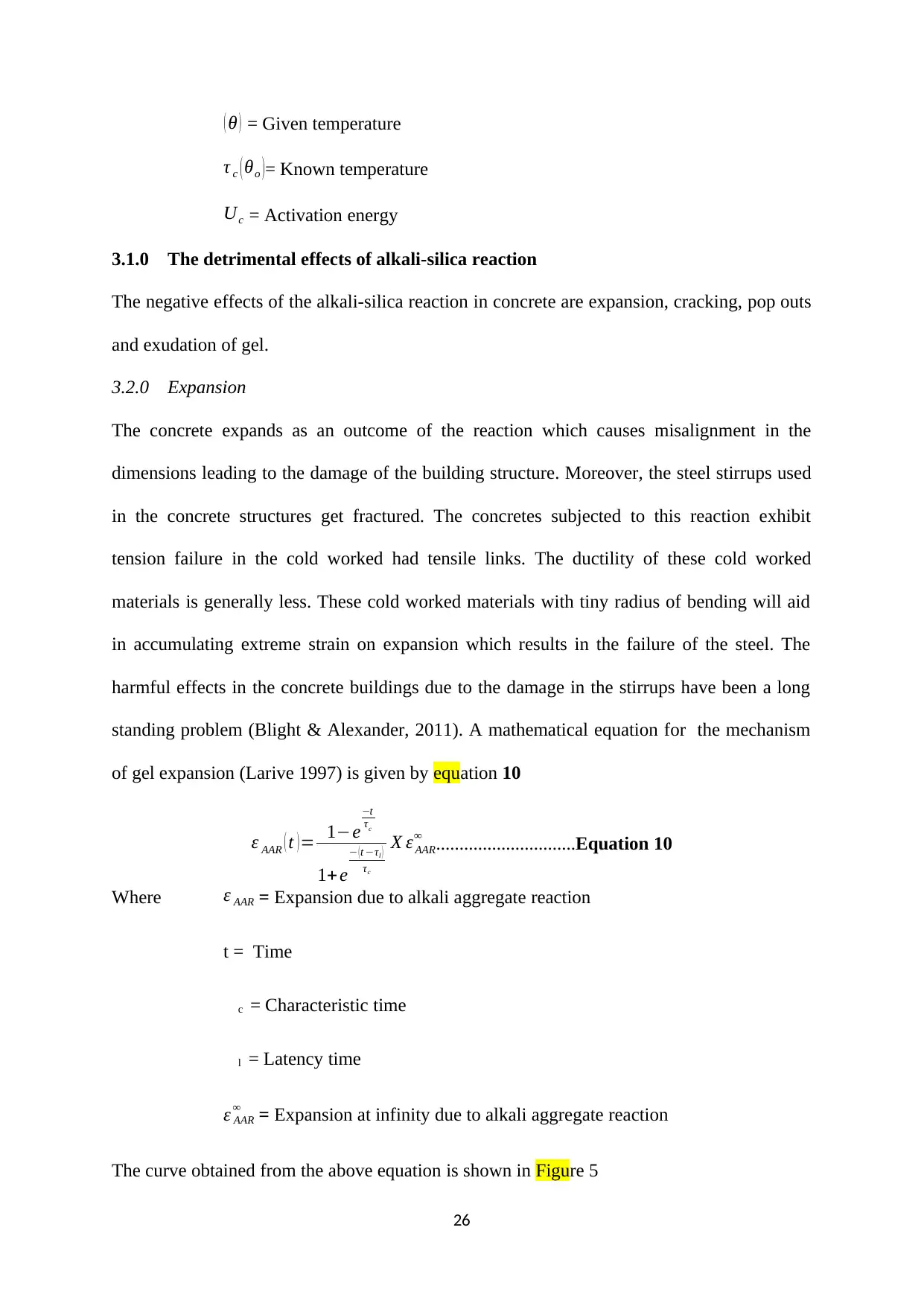
( θ ) = Given temperature
τ c ( θo )= Known temperature
Uc = Activation energy
3.1.0 The detrimental effects of alkali-silica reaction
The negative effects of the alkali-silica reaction in concrete are expansion, cracking, pop outs
and exudation of gel.
3.2.0 Expansion
The concrete expands as an outcome of the reaction which causes misalignment in the
dimensions leading to the damage of the building structure. Moreover, the steel stirrups used
in the concrete structures get fractured. The concretes subjected to this reaction exhibit
tension failure in the cold worked had tensile links. The ductility of these cold worked
materials is generally less. These cold worked materials with tiny radius of bending will aid
in accumulating extreme strain on expansion which results in the failure of the steel. The
harmful effects in the concrete buildings due to the damage in the stirrups have been a long
standing problem (Blight & Alexander, 2011). A mathematical equation for the mechanism
of gel expansion (Larive 1997) is given by equation 10
ε AAR ( t ) = 1−e
−t
τc
1+ e
− ( t −τl )
τc
X ε AAR
∞ ..............................Equation 10
Where ε AAR = Expansion due to alkali aggregate reaction
t = Time
c = Characteristic time
l = Latency time
ε AAR
∞ = Expansion at infinity due to alkali aggregate reaction
The curve obtained from the above equation is shown in Figure 5
26
τ c ( θo )= Known temperature
Uc = Activation energy
3.1.0 The detrimental effects of alkali-silica reaction
The negative effects of the alkali-silica reaction in concrete are expansion, cracking, pop outs
and exudation of gel.
3.2.0 Expansion
The concrete expands as an outcome of the reaction which causes misalignment in the
dimensions leading to the damage of the building structure. Moreover, the steel stirrups used
in the concrete structures get fractured. The concretes subjected to this reaction exhibit
tension failure in the cold worked had tensile links. The ductility of these cold worked
materials is generally less. These cold worked materials with tiny radius of bending will aid
in accumulating extreme strain on expansion which results in the failure of the steel. The
harmful effects in the concrete buildings due to the damage in the stirrups have been a long
standing problem (Blight & Alexander, 2011). A mathematical equation for the mechanism
of gel expansion (Larive 1997) is given by equation 10
ε AAR ( t ) = 1−e
−t
τc
1+ e
− ( t −τl )
τc
X ε AAR
∞ ..............................Equation 10
Where ε AAR = Expansion due to alkali aggregate reaction
t = Time
c = Characteristic time
l = Latency time
ε AAR
∞ = Expansion at infinity due to alkali aggregate reaction
The curve obtained from the above equation is shown in Figure 5
26
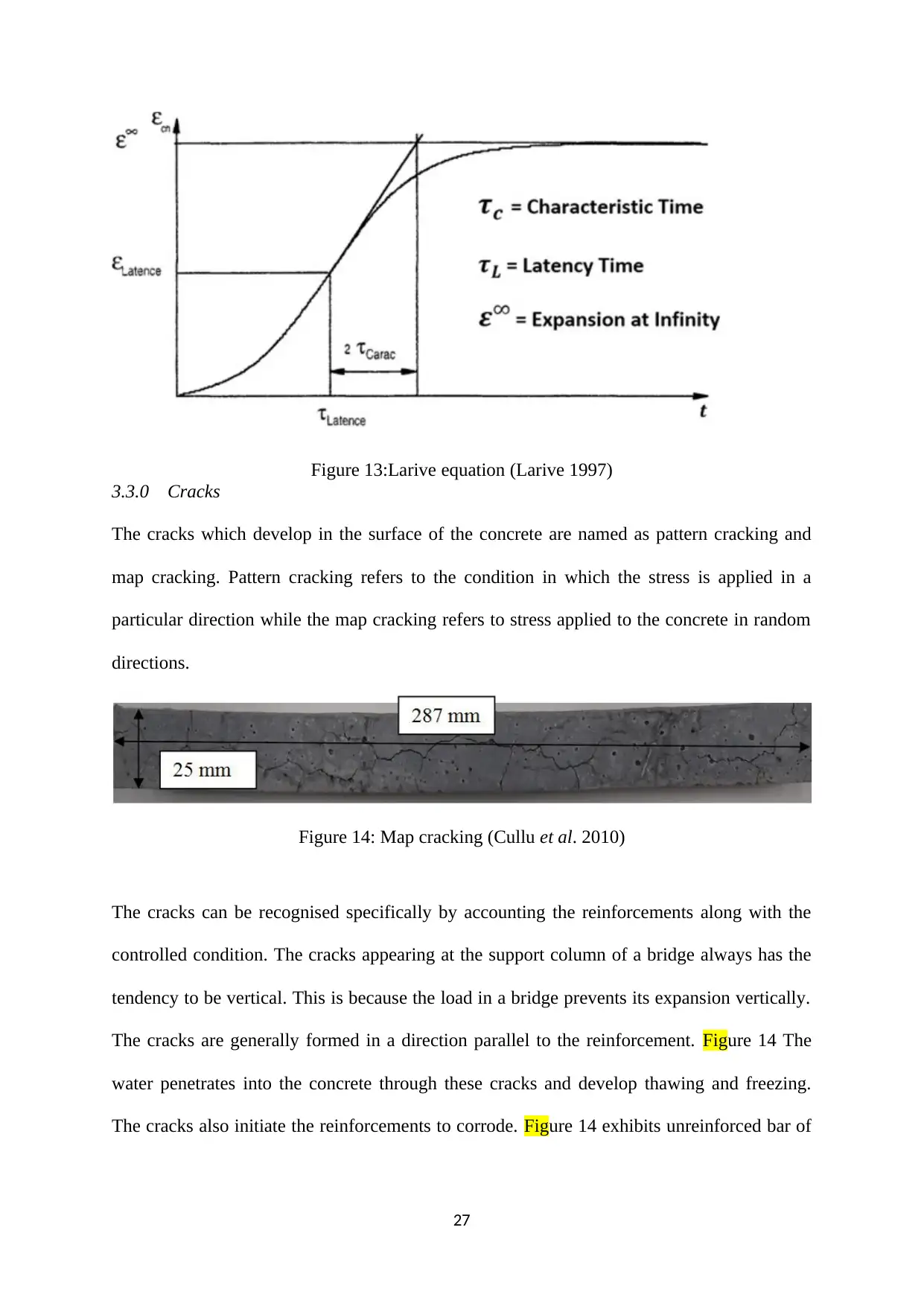
Figure 13:Larive equation (Larive 1997)
3.3.0 Cracks
The cracks which develop in the surface of the concrete are named as pattern cracking and
map cracking. Pattern cracking refers to the condition in which the stress is applied in a
particular direction while the map cracking refers to stress applied to the concrete in random
directions.
Figure 14: Map cracking (Cullu et al. 2010)
The cracks can be recognised specifically by accounting the reinforcements along with the
controlled condition. The cracks appearing at the support column of a bridge always has the
tendency to be vertical. This is because the load in a bridge prevents its expansion vertically.
The cracks are generally formed in a direction parallel to the reinforcement. Figure 14 The
water penetrates into the concrete through these cracks and develop thawing and freezing.
The cracks also initiate the reinforcements to corrode. Figure 14 exhibits unreinforced bar of
27
3.3.0 Cracks
The cracks which develop in the surface of the concrete are named as pattern cracking and
map cracking. Pattern cracking refers to the condition in which the stress is applied in a
particular direction while the map cracking refers to stress applied to the concrete in random
directions.
Figure 14: Map cracking (Cullu et al. 2010)
The cracks can be recognised specifically by accounting the reinforcements along with the
controlled condition. The cracks appearing at the support column of a bridge always has the
tendency to be vertical. This is because the load in a bridge prevents its expansion vertically.
The cracks are generally formed in a direction parallel to the reinforcement. Figure 14 The
water penetrates into the concrete through these cracks and develop thawing and freezing.
The cracks also initiate the reinforcements to corrode. Figure 14 exhibits unreinforced bar of
27
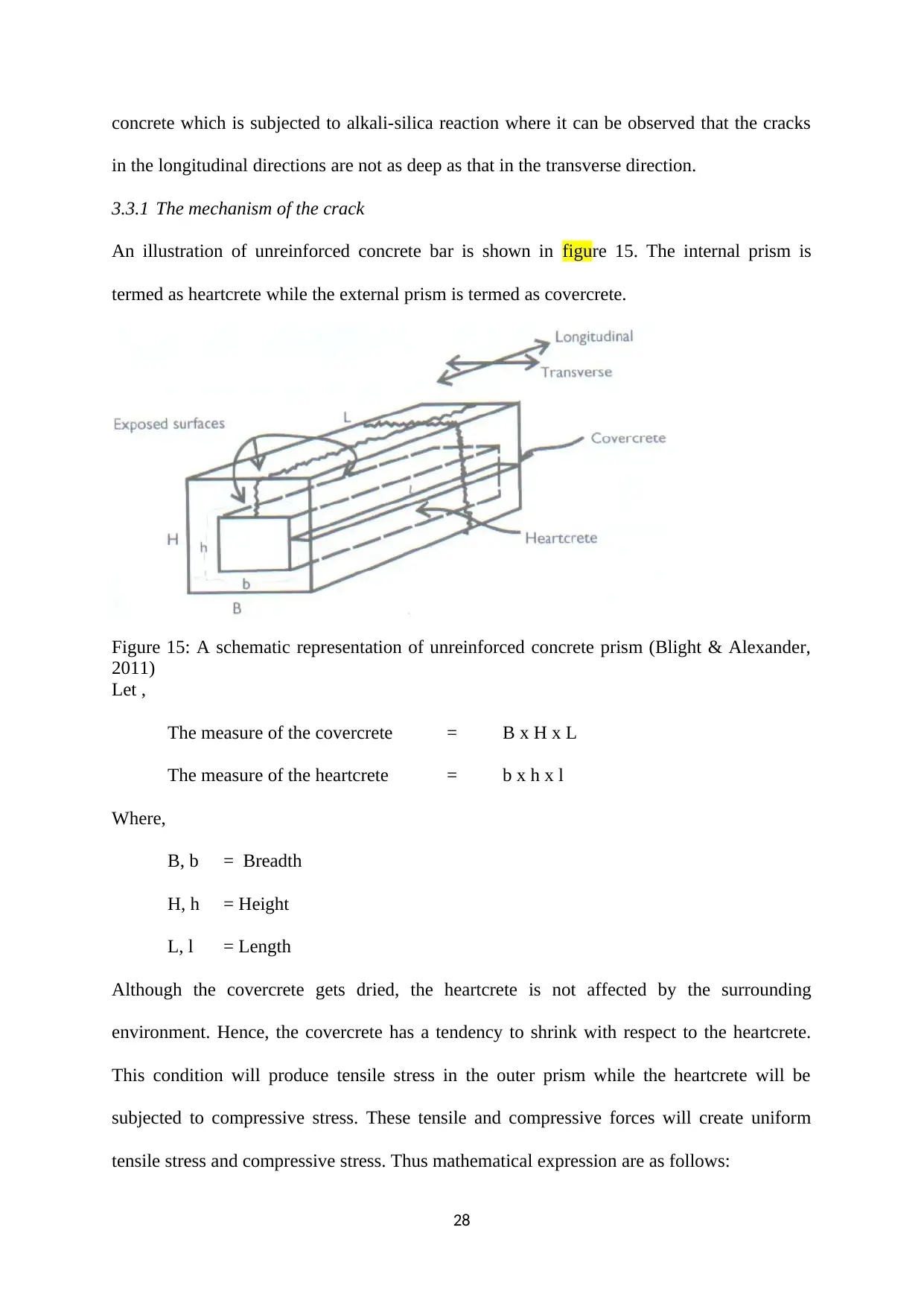
concrete which is subjected to alkali-silica reaction where it can be observed that the cracks
in the longitudinal directions are not as deep as that in the transverse direction.
3.3.1 The mechanism of the crack
An illustration of unreinforced concrete bar is shown in figure 15. The internal prism is
termed as heartcrete while the external prism is termed as covercrete.
Figure 15: A schematic representation of unreinforced concrete prism (Blight & Alexander,
2011)
Let ,
The measure of the covercrete = B x H x L
The measure of the heartcrete = b x h x l
Where,
B, b = Breadth
H, h = Height
L, l = Length
Although the covercrete gets dried, the heartcrete is not affected by the surrounding
environment. Hence, the covercrete has a tendency to shrink with respect to the heartcrete.
This condition will produce tensile stress in the outer prism while the heartcrete will be
subjected to compressive stress. These tensile and compressive forces will create uniform
tensile stress and compressive stress. Thus mathematical expression are as follows:
28
in the longitudinal directions are not as deep as that in the transverse direction.
3.3.1 The mechanism of the crack
An illustration of unreinforced concrete bar is shown in figure 15. The internal prism is
termed as heartcrete while the external prism is termed as covercrete.
Figure 15: A schematic representation of unreinforced concrete prism (Blight & Alexander,
2011)
Let ,
The measure of the covercrete = B x H x L
The measure of the heartcrete = b x h x l
Where,
B, b = Breadth
H, h = Height
L, l = Length
Although the covercrete gets dried, the heartcrete is not affected by the surrounding
environment. Hence, the covercrete has a tendency to shrink with respect to the heartcrete.
This condition will produce tensile stress in the outer prism while the heartcrete will be
subjected to compressive stress. These tensile and compressive forces will create uniform
tensile stress and compressive stress. Thus mathematical expression are as follows:
28
Secure Best Marks with AI Grader
Need help grading? Try our AI Grader for instant feedback on your assignments.
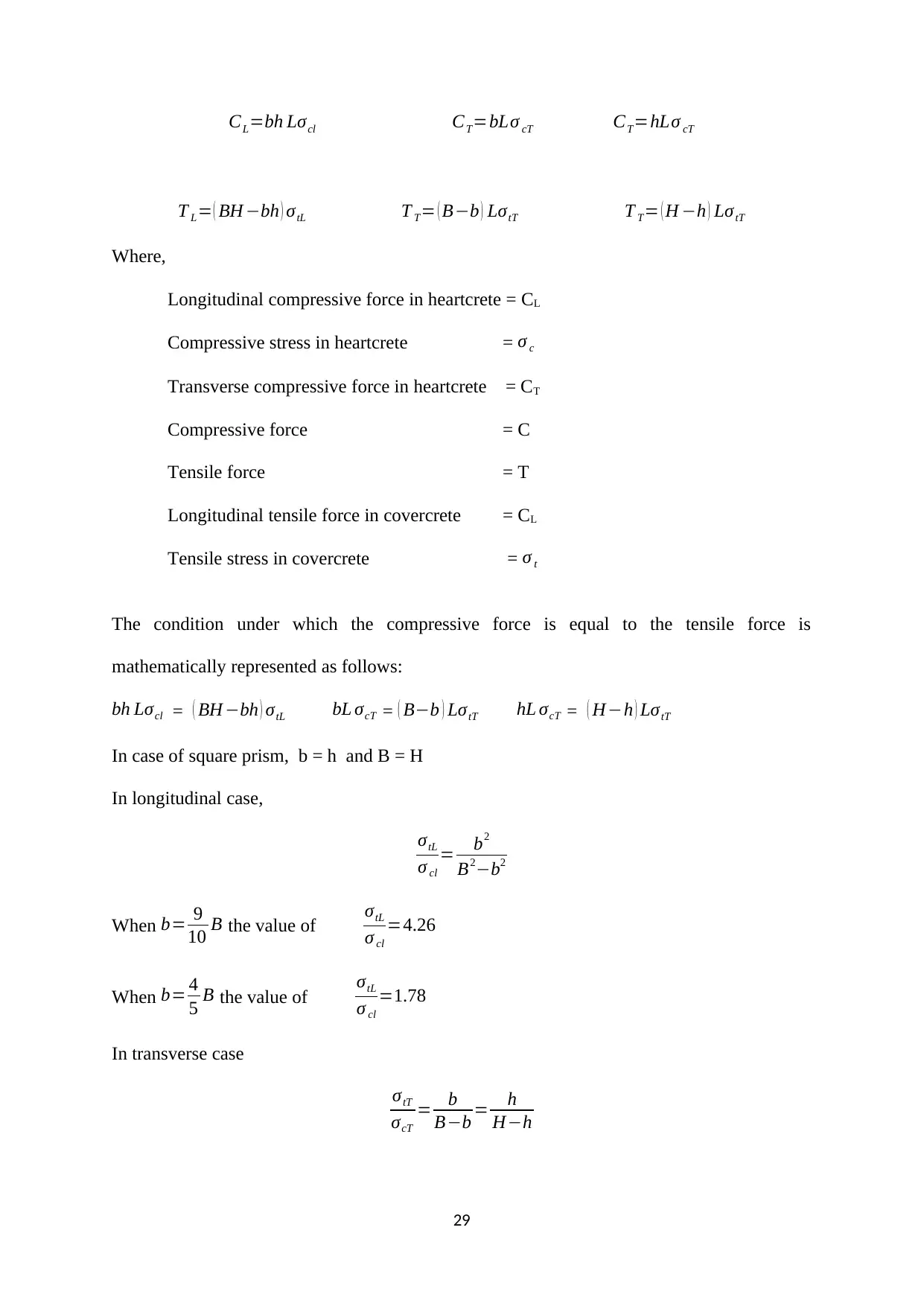
CL=bh Lσcl CT=bLσ cT CT=hLσ cT
T L= ( BH −bh ) σtL T T= ( B−b ) LσtT T T= ( H −h ) LσtT
Where,
Longitudinal compressive force in heartcrete = CL
Compressive stress in heartcrete = σ c
Transverse compressive force in heartcrete = CT
Compressive force = C
Tensile force = T
Longitudinal tensile force in covercrete = CL
Tensile stress in covercrete = σ t
The condition under which the compressive force is equal to the tensile force is
mathematically represented as follows:
bh Lσcl = ( BH −bh ) σtL bL σcT = ( B−b ) LσtT hL σcT = ( H−h ) LσtT
In case of square prism, b = h and B = H
In longitudinal case,
σtL
σ cl
= b2
B2−b2
When b= 9
10 B the value of σtL
σ cl
=4.26
When b= 4
5 B the value of σtL
σ cl
=1.78
In transverse case
σtT
σcT
= b
B−b = h
H−h
29
T L= ( BH −bh ) σtL T T= ( B−b ) LσtT T T= ( H −h ) LσtT
Where,
Longitudinal compressive force in heartcrete = CL
Compressive stress in heartcrete = σ c
Transverse compressive force in heartcrete = CT
Compressive force = C
Tensile force = T
Longitudinal tensile force in covercrete = CL
Tensile stress in covercrete = σ t
The condition under which the compressive force is equal to the tensile force is
mathematically represented as follows:
bh Lσcl = ( BH −bh ) σtL bL σcT = ( B−b ) LσtT hL σcT = ( H−h ) LσtT
In case of square prism, b = h and B = H
In longitudinal case,
σtL
σ cl
= b2
B2−b2
When b= 9
10 B the value of σtL
σ cl
=4.26
When b= 4
5 B the value of σtL
σ cl
=1.78
In transverse case
σtT
σcT
= b
B−b = h
H−h
29
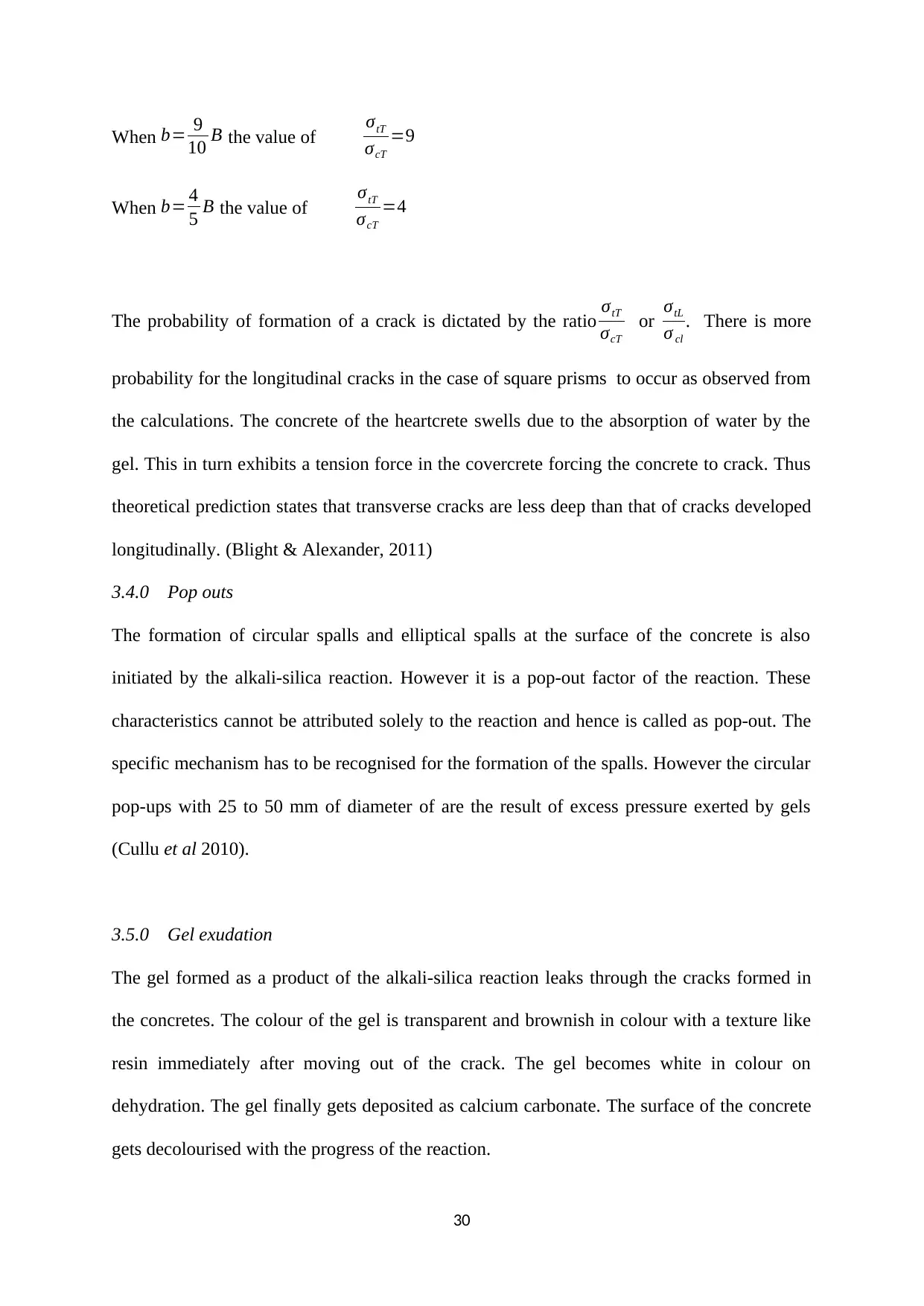
When b= 9
10 B the value of σtT
σcT
=9
When b= 4
5 B the value of σtT
σcT
=4
The probability of formation of a crack is dictated by the ratio σtT
σcT
or σtL
σ cl
. There is more
probability for the longitudinal cracks in the case of square prisms to occur as observed from
the calculations. The concrete of the heartcrete swells due to the absorption of water by the
gel. This in turn exhibits a tension force in the covercrete forcing the concrete to crack. Thus
theoretical prediction states that transverse cracks are less deep than that of cracks developed
longitudinally. (Blight & Alexander, 2011)
3.4.0 Pop outs
The formation of circular spalls and elliptical spalls at the surface of the concrete is also
initiated by the alkali-silica reaction. However it is a pop-out factor of the reaction. These
characteristics cannot be attributed solely to the reaction and hence is called as pop-out. The
specific mechanism has to be recognised for the formation of the spalls. However the circular
pop-ups with 25 to 50 mm of diameter of are the result of excess pressure exerted by gels
(Cullu et al 2010).
3.5.0 Gel exudation
The gel formed as a product of the alkali-silica reaction leaks through the cracks formed in
the concretes. The colour of the gel is transparent and brownish in colour with a texture like
resin immediately after moving out of the crack. The gel becomes white in colour on
dehydration. The gel finally gets deposited as calcium carbonate. The surface of the concrete
gets decolourised with the progress of the reaction.
30
10 B the value of σtT
σcT
=9
When b= 4
5 B the value of σtT
σcT
=4
The probability of formation of a crack is dictated by the ratio σtT
σcT
or σtL
σ cl
. There is more
probability for the longitudinal cracks in the case of square prisms to occur as observed from
the calculations. The concrete of the heartcrete swells due to the absorption of water by the
gel. This in turn exhibits a tension force in the covercrete forcing the concrete to crack. Thus
theoretical prediction states that transverse cracks are less deep than that of cracks developed
longitudinally. (Blight & Alexander, 2011)
3.4.0 Pop outs
The formation of circular spalls and elliptical spalls at the surface of the concrete is also
initiated by the alkali-silica reaction. However it is a pop-out factor of the reaction. These
characteristics cannot be attributed solely to the reaction and hence is called as pop-out. The
specific mechanism has to be recognised for the formation of the spalls. However the circular
pop-ups with 25 to 50 mm of diameter of are the result of excess pressure exerted by gels
(Cullu et al 2010).
3.5.0 Gel exudation
The gel formed as a product of the alkali-silica reaction leaks through the cracks formed in
the concretes. The colour of the gel is transparent and brownish in colour with a texture like
resin immediately after moving out of the crack. The gel becomes white in colour on
dehydration. The gel finally gets deposited as calcium carbonate. The surface of the concrete
gets decolourised with the progress of the reaction.
30
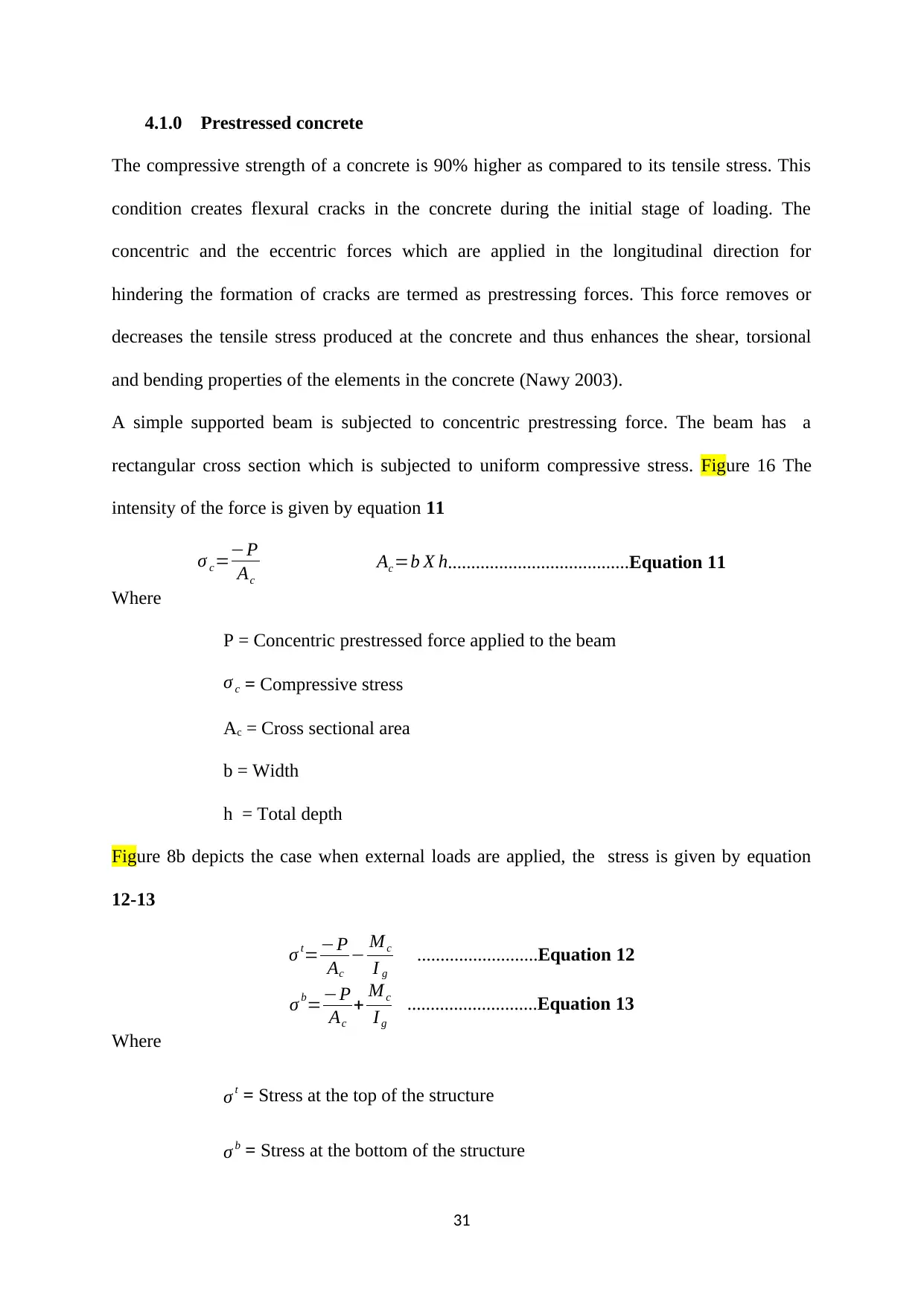
4.1.0 Prestressed concrete
The compressive strength of a concrete is 90% higher as compared to its tensile stress. This
condition creates flexural cracks in the concrete during the initial stage of loading. The
concentric and the eccentric forces which are applied in the longitudinal direction for
hindering the formation of cracks are termed as prestressing forces. This force removes or
decreases the tensile stress produced at the concrete and thus enhances the shear, torsional
and bending properties of the elements in the concrete (Nawy 2003).
A simple supported beam is subjected to concentric prestressing force. The beam has a
rectangular cross section which is subjected to uniform compressive stress. Figure 16 The
intensity of the force is given by equation 11
σ c=−P
Ac
Ac=b X h.......................................Equation 11
Where
P = Concentric prestressed force applied to the beam
σ c = Compressive stress
Ac = Cross sectional area
b = Width
h = Total depth
Figure 8b depicts the case when external loads are applied, the stress is given by equation
12-13
σ t=−P
Ac
− M c
I g
..........................Equation 12
σ b=−P
Ac
+ M c
I g
............................Equation 13
Where
σ t = Stress at the top of the structure
σ b = Stress at the bottom of the structure
31
The compressive strength of a concrete is 90% higher as compared to its tensile stress. This
condition creates flexural cracks in the concrete during the initial stage of loading. The
concentric and the eccentric forces which are applied in the longitudinal direction for
hindering the formation of cracks are termed as prestressing forces. This force removes or
decreases the tensile stress produced at the concrete and thus enhances the shear, torsional
and bending properties of the elements in the concrete (Nawy 2003).
A simple supported beam is subjected to concentric prestressing force. The beam has a
rectangular cross section which is subjected to uniform compressive stress. Figure 16 The
intensity of the force is given by equation 11
σ c=−P
Ac
Ac=b X h.......................................Equation 11
Where
P = Concentric prestressed force applied to the beam
σ c = Compressive stress
Ac = Cross sectional area
b = Width
h = Total depth
Figure 8b depicts the case when external loads are applied, the stress is given by equation
12-13
σ t=−P
Ac
− M c
I g
..........................Equation 12
σ b=−P
Ac
+ M c
I g
............................Equation 13
Where
σ t = Stress at the top of the structure
σ b = Stress at the bottom of the structure
31
Paraphrase This Document
Need a fresh take? Get an instant paraphrase of this document with our AI Paraphraser
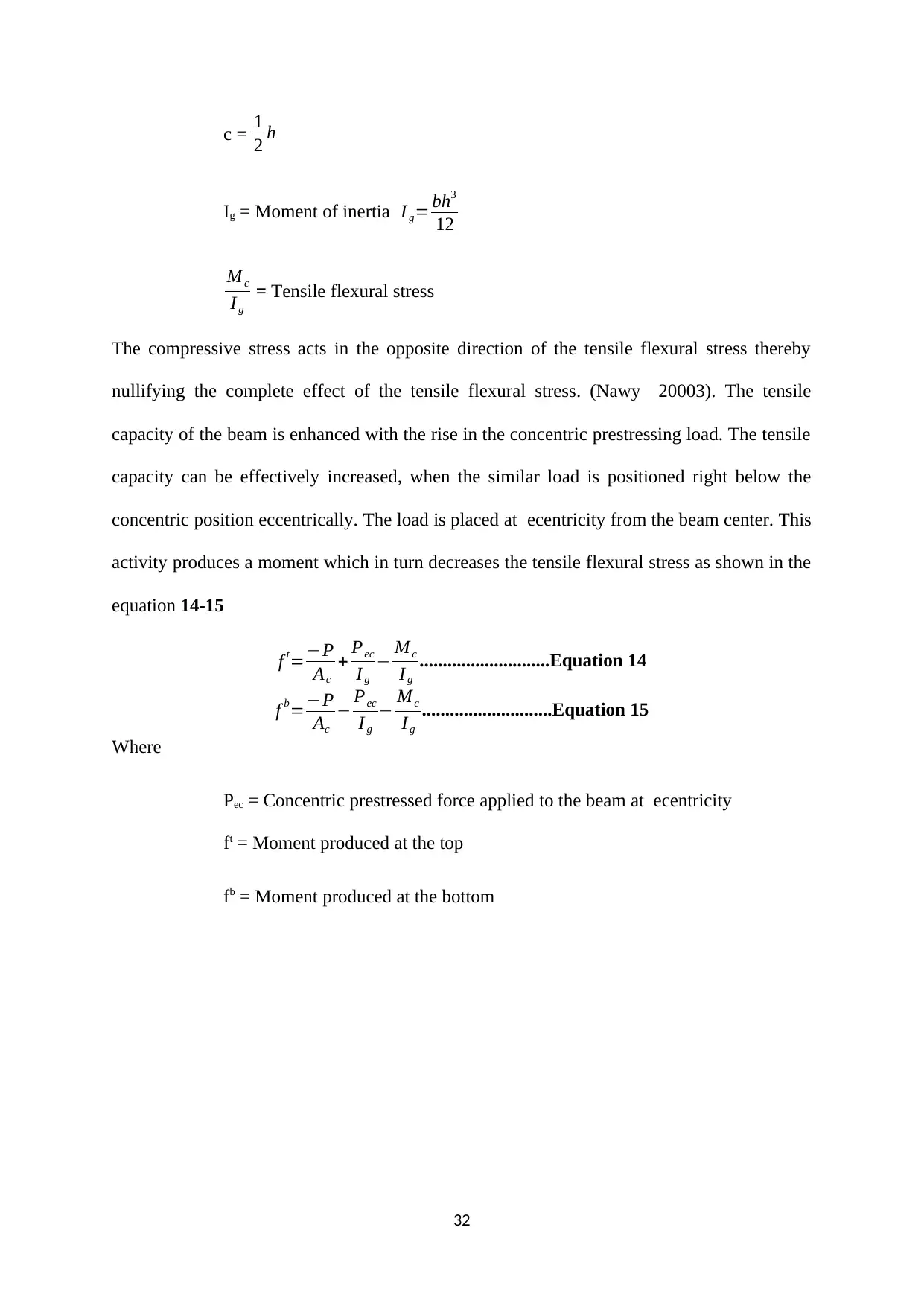
c = 1
2 h
Ig = Moment of inertia I g= bh3
12
M c
I g
= Tensile flexural stress
The compressive stress acts in the opposite direction of the tensile flexural stress thereby
nullifying the complete effect of the tensile flexural stress. (Nawy 20003). The tensile
capacity of the beam is enhanced with the rise in the concentric prestressing load. The tensile
capacity can be effectively increased, when the similar load is positioned right below the
concentric position eccentrically. The load is placed at ecentricity from the beam center. This
activity produces a moment which in turn decreases the tensile flexural stress as shown in the
equation 14-15
f t=−P
Ac
+ Pec
I g
− M c
I g
............................Equation 14
f b=−P
Ac
− Pec
I g
− Mc
Ig
............................Equation 15
Where
Pec = Concentric prestressed force applied to the beam at ecentricity
ft = Moment produced at the top
fb = Moment produced at the bottom
32
2 h
Ig = Moment of inertia I g= bh3
12
M c
I g
= Tensile flexural stress
The compressive stress acts in the opposite direction of the tensile flexural stress thereby
nullifying the complete effect of the tensile flexural stress. (Nawy 20003). The tensile
capacity of the beam is enhanced with the rise in the concentric prestressing load. The tensile
capacity can be effectively increased, when the similar load is positioned right below the
concentric position eccentrically. The load is placed at ecentricity from the beam center. This
activity produces a moment which in turn decreases the tensile flexural stress as shown in the
equation 14-15
f t=−P
Ac
+ Pec
I g
− M c
I g
............................Equation 14
f b=−P
Ac
− Pec
I g
− Mc
Ig
............................Equation 15
Where
Pec = Concentric prestressed force applied to the beam at ecentricity
ft = Moment produced at the top
fb = Moment produced at the bottom
32
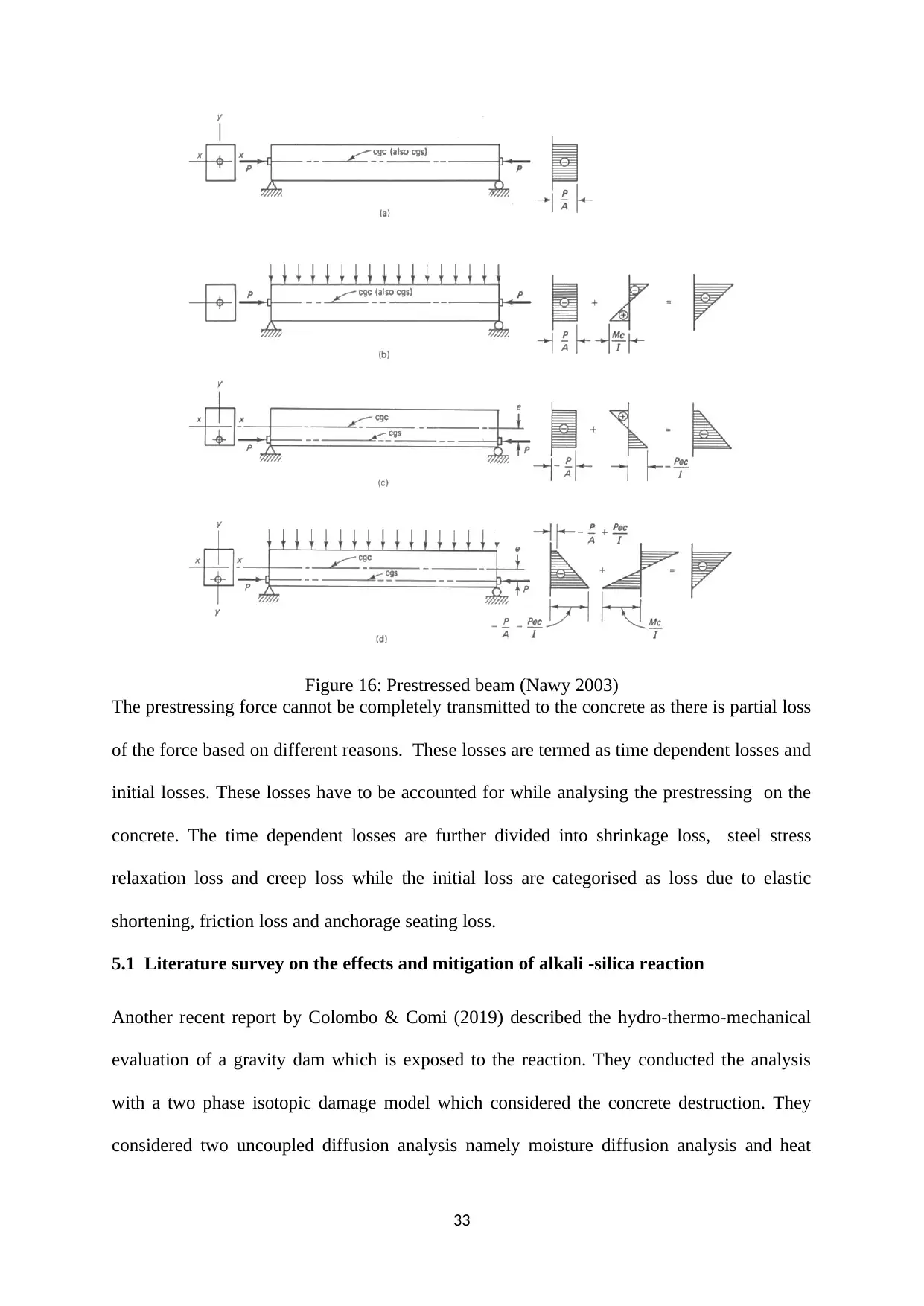
Figure 16: Prestressed beam (Nawy 2003)
The prestressing force cannot be completely transmitted to the concrete as there is partial loss
of the force based on different reasons. These losses are termed as time dependent losses and
initial losses. These losses have to be accounted for while analysing the prestressing on the
concrete. The time dependent losses are further divided into shrinkage loss, steel stress
relaxation loss and creep loss while the initial loss are categorised as loss due to elastic
shortening, friction loss and anchorage seating loss.
5.1 Literature survey on the effects and mitigation of alkali -silica reaction
Another recent report by Colombo & Comi (2019) described the hydro-thermo-mechanical
evaluation of a gravity dam which is exposed to the reaction. They conducted the analysis
with a two phase isotopic damage model which considered the concrete destruction. They
considered two uncoupled diffusion analysis namely moisture diffusion analysis and heat
33
The prestressing force cannot be completely transmitted to the concrete as there is partial loss
of the force based on different reasons. These losses are termed as time dependent losses and
initial losses. These losses have to be accounted for while analysing the prestressing on the
concrete. The time dependent losses are further divided into shrinkage loss, steel stress
relaxation loss and creep loss while the initial loss are categorised as loss due to elastic
shortening, friction loss and anchorage seating loss.
5.1 Literature survey on the effects and mitigation of alkali -silica reaction
Another recent report by Colombo & Comi (2019) described the hydro-thermo-mechanical
evaluation of a gravity dam which is exposed to the reaction. They conducted the analysis
with a two phase isotopic damage model which considered the concrete destruction. They
considered two uncoupled diffusion analysis namely moisture diffusion analysis and heat
33
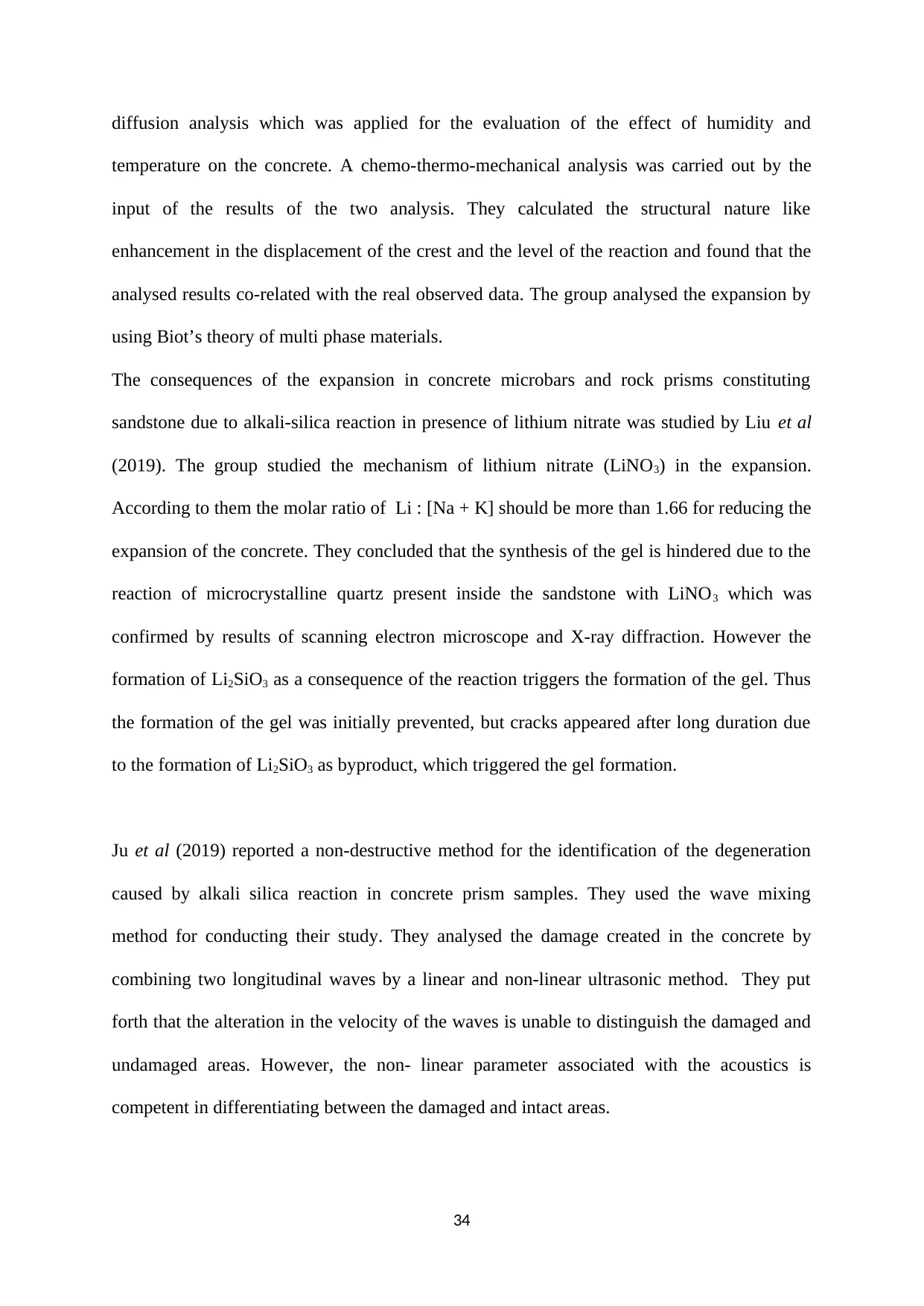
diffusion analysis which was applied for the evaluation of the effect of humidity and
temperature on the concrete. A chemo-thermo-mechanical analysis was carried out by the
input of the results of the two analysis. They calculated the structural nature like
enhancement in the displacement of the crest and the level of the reaction and found that the
analysed results co-related with the real observed data. The group analysed the expansion by
using Biot’s theory of multi phase materials.
The consequences of the expansion in concrete microbars and rock prisms constituting
sandstone due to alkali-silica reaction in presence of lithium nitrate was studied by Liu et al
(2019). The group studied the mechanism of lithium nitrate (LiNO3) in the expansion.
According to them the molar ratio of Li : [Na + K] should be more than 1.66 for reducing the
expansion of the concrete. They concluded that the synthesis of the gel is hindered due to the
reaction of microcrystalline quartz present inside the sandstone with LiNO3 which was
confirmed by results of scanning electron microscope and X-ray diffraction. However the
formation of Li2SiO3 as a consequence of the reaction triggers the formation of the gel. Thus
the formation of the gel was initially prevented, but cracks appeared after long duration due
to the formation of Li2SiO3 as byproduct, which triggered the gel formation.
Ju et al (2019) reported a non-destructive method for the identification of the degeneration
caused by alkali silica reaction in concrete prism samples. They used the wave mixing
method for conducting their study. They analysed the damage created in the concrete by
combining two longitudinal waves by a linear and non-linear ultrasonic method. They put
forth that the alteration in the velocity of the waves is unable to distinguish the damaged and
undamaged areas. However, the non- linear parameter associated with the acoustics is
competent in differentiating between the damaged and intact areas.
34
temperature on the concrete. A chemo-thermo-mechanical analysis was carried out by the
input of the results of the two analysis. They calculated the structural nature like
enhancement in the displacement of the crest and the level of the reaction and found that the
analysed results co-related with the real observed data. The group analysed the expansion by
using Biot’s theory of multi phase materials.
The consequences of the expansion in concrete microbars and rock prisms constituting
sandstone due to alkali-silica reaction in presence of lithium nitrate was studied by Liu et al
(2019). The group studied the mechanism of lithium nitrate (LiNO3) in the expansion.
According to them the molar ratio of Li : [Na + K] should be more than 1.66 for reducing the
expansion of the concrete. They concluded that the synthesis of the gel is hindered due to the
reaction of microcrystalline quartz present inside the sandstone with LiNO3 which was
confirmed by results of scanning electron microscope and X-ray diffraction. However the
formation of Li2SiO3 as a consequence of the reaction triggers the formation of the gel. Thus
the formation of the gel was initially prevented, but cracks appeared after long duration due
to the formation of Li2SiO3 as byproduct, which triggered the gel formation.
Ju et al (2019) reported a non-destructive method for the identification of the degeneration
caused by alkali silica reaction in concrete prism samples. They used the wave mixing
method for conducting their study. They analysed the damage created in the concrete by
combining two longitudinal waves by a linear and non-linear ultrasonic method. They put
forth that the alteration in the velocity of the waves is unable to distinguish the damaged and
undamaged areas. However, the non- linear parameter associated with the acoustics is
competent in differentiating between the damaged and intact areas.
34
Secure Best Marks with AI Grader
Need help grading? Try our AI Grader for instant feedback on your assignments.
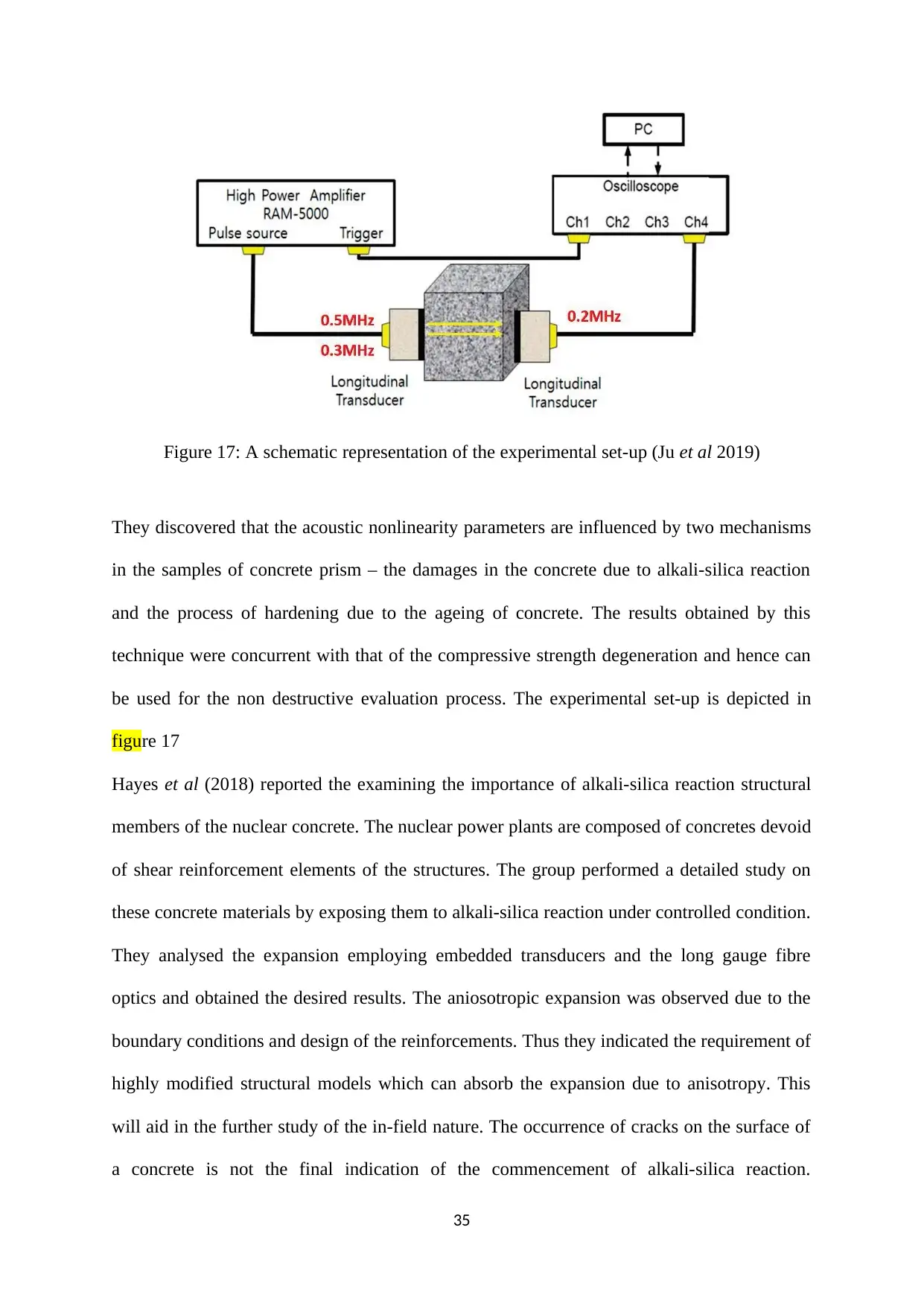
Figure 17: A schematic representation of the experimental set-up (Ju et al 2019)
They discovered that the acoustic nonlinearity parameters are influenced by two mechanisms
in the samples of concrete prism – the damages in the concrete due to alkali-silica reaction
and the process of hardening due to the ageing of concrete. The results obtained by this
technique were concurrent with that of the compressive strength degeneration and hence can
be used for the non destructive evaluation process. The experimental set-up is depicted in
figure 17
Hayes et al (2018) reported the examining the importance of alkali-silica reaction structural
members of the nuclear concrete. The nuclear power plants are composed of concretes devoid
of shear reinforcement elements of the structures. The group performed a detailed study on
these concrete materials by exposing them to alkali-silica reaction under controlled condition.
They analysed the expansion employing embedded transducers and the long gauge fibre
optics and obtained the desired results. The aniosotropic expansion was observed due to the
boundary conditions and design of the reinforcements. Thus they indicated the requirement of
highly modified structural models which can absorb the expansion due to anisotropy. This
will aid in the further study of the in-field nature. The occurrence of cracks on the surface of
a concrete is not the final indication of the commencement of alkali-silica reaction.
35
They discovered that the acoustic nonlinearity parameters are influenced by two mechanisms
in the samples of concrete prism – the damages in the concrete due to alkali-silica reaction
and the process of hardening due to the ageing of concrete. The results obtained by this
technique were concurrent with that of the compressive strength degeneration and hence can
be used for the non destructive evaluation process. The experimental set-up is depicted in
figure 17
Hayes et al (2018) reported the examining the importance of alkali-silica reaction structural
members of the nuclear concrete. The nuclear power plants are composed of concretes devoid
of shear reinforcement elements of the structures. The group performed a detailed study on
these concrete materials by exposing them to alkali-silica reaction under controlled condition.
They analysed the expansion employing embedded transducers and the long gauge fibre
optics and obtained the desired results. The aniosotropic expansion was observed due to the
boundary conditions and design of the reinforcements. Thus they indicated the requirement of
highly modified structural models which can absorb the expansion due to anisotropy. This
will aid in the further study of the in-field nature. The occurrence of cracks on the surface of
a concrete is not the final indication of the commencement of alkali-silica reaction.
35
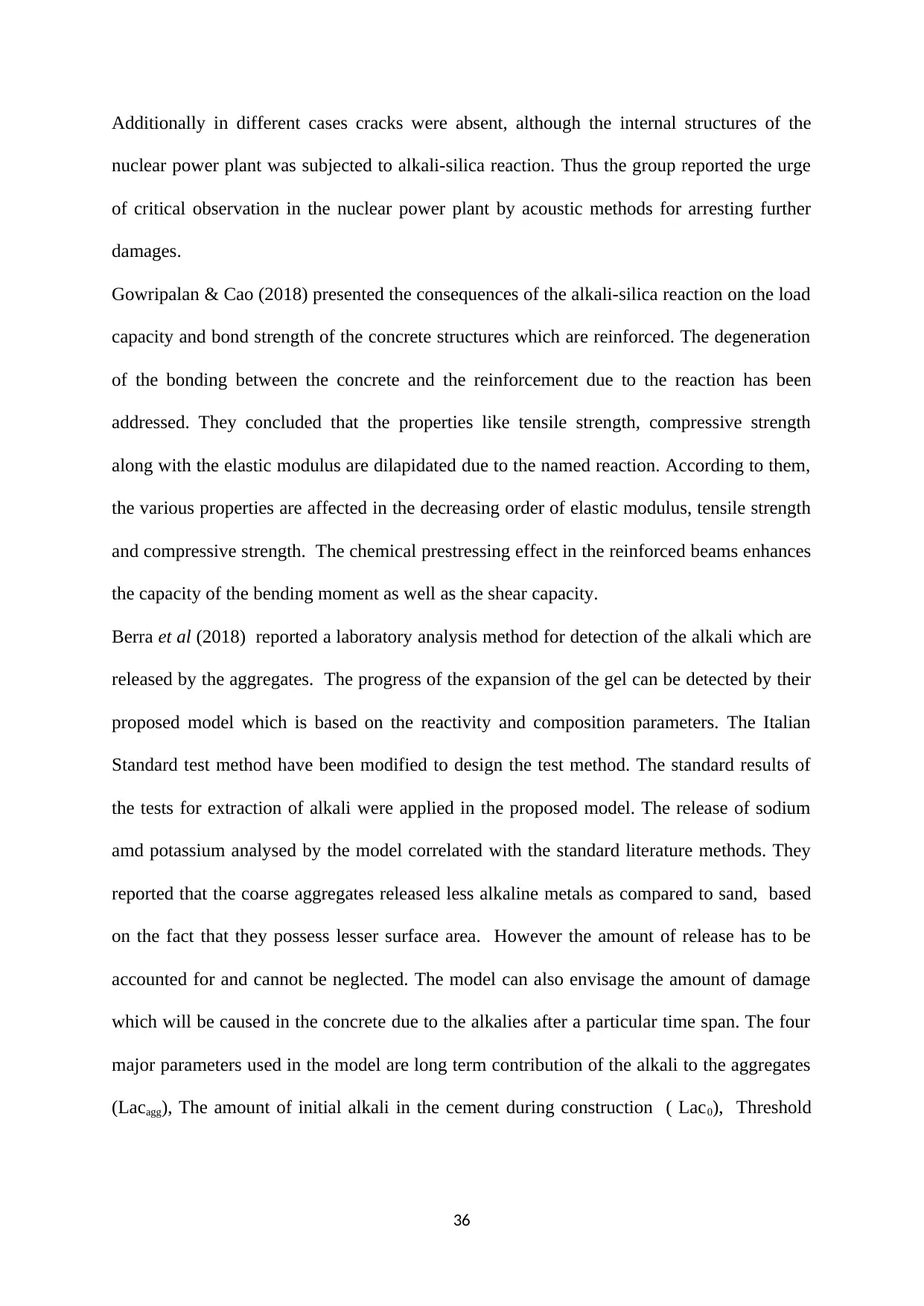
Additionally in different cases cracks were absent, although the internal structures of the
nuclear power plant was subjected to alkali-silica reaction. Thus the group reported the urge
of critical observation in the nuclear power plant by acoustic methods for arresting further
damages.
Gowripalan & Cao (2018) presented the consequences of the alkali-silica reaction on the load
capacity and bond strength of the concrete structures which are reinforced. The degeneration
of the bonding between the concrete and the reinforcement due to the reaction has been
addressed. They concluded that the properties like tensile strength, compressive strength
along with the elastic modulus are dilapidated due to the named reaction. According to them,
the various properties are affected in the decreasing order of elastic modulus, tensile strength
and compressive strength. The chemical prestressing effect in the reinforced beams enhances
the capacity of the bending moment as well as the shear capacity.
Berra et al (2018) reported a laboratory analysis method for detection of the alkali which are
released by the aggregates. The progress of the expansion of the gel can be detected by their
proposed model which is based on the reactivity and composition parameters. The Italian
Standard test method have been modified to design the test method. The standard results of
the tests for extraction of alkali were applied in the proposed model. The release of sodium
amd potassium analysed by the model correlated with the standard literature methods. They
reported that the coarse aggregates released less alkaline metals as compared to sand, based
on the fact that they possess lesser surface area. However the amount of release has to be
accounted for and cannot be neglected. The model can also envisage the amount of damage
which will be caused in the concrete due to the alkalies after a particular time span. The four
major parameters used in the model are long term contribution of the alkali to the aggregates
(Lacagg), The amount of initial alkali in the cement during construction ( Lac0), Threshold
36
nuclear power plant was subjected to alkali-silica reaction. Thus the group reported the urge
of critical observation in the nuclear power plant by acoustic methods for arresting further
damages.
Gowripalan & Cao (2018) presented the consequences of the alkali-silica reaction on the load
capacity and bond strength of the concrete structures which are reinforced. The degeneration
of the bonding between the concrete and the reinforcement due to the reaction has been
addressed. They concluded that the properties like tensile strength, compressive strength
along with the elastic modulus are dilapidated due to the named reaction. According to them,
the various properties are affected in the decreasing order of elastic modulus, tensile strength
and compressive strength. The chemical prestressing effect in the reinforced beams enhances
the capacity of the bending moment as well as the shear capacity.
Berra et al (2018) reported a laboratory analysis method for detection of the alkali which are
released by the aggregates. The progress of the expansion of the gel can be detected by their
proposed model which is based on the reactivity and composition parameters. The Italian
Standard test method have been modified to design the test method. The standard results of
the tests for extraction of alkali were applied in the proposed model. The release of sodium
amd potassium analysed by the model correlated with the standard literature methods. They
reported that the coarse aggregates released less alkaline metals as compared to sand, based
on the fact that they possess lesser surface area. However the amount of release has to be
accounted for and cannot be neglected. The model can also envisage the amount of damage
which will be caused in the concrete due to the alkalies after a particular time span. The four
major parameters used in the model are long term contribution of the alkali to the aggregates
(Lacagg), The amount of initial alkali in the cement during construction ( Lac0), Threshold
36
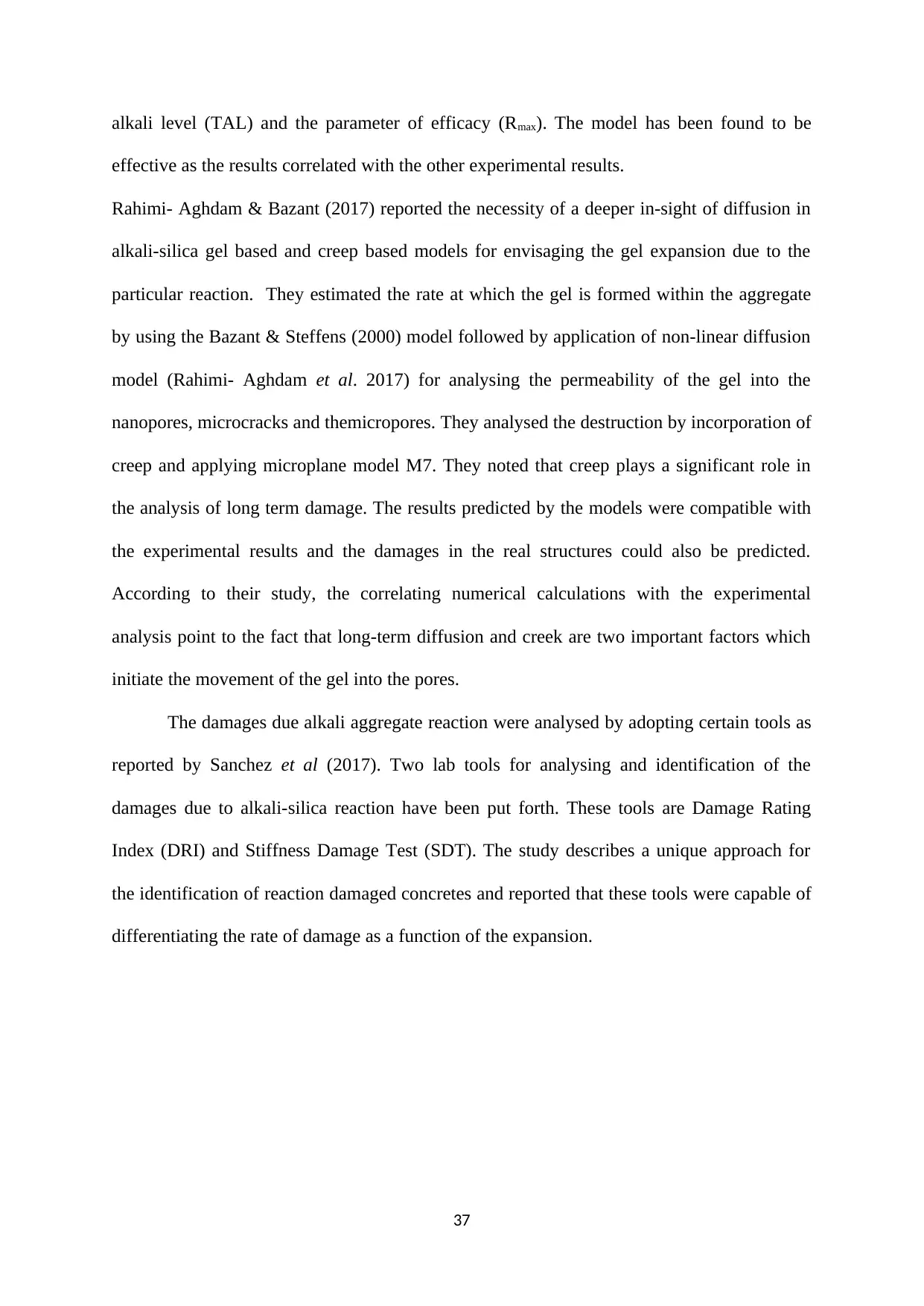
alkali level (TAL) and the parameter of efficacy (Rmax). The model has been found to be
effective as the results correlated with the other experimental results.
Rahimi- Aghdam & Bazant (2017) reported the necessity of a deeper in-sight of diffusion in
alkali-silica gel based and creep based models for envisaging the gel expansion due to the
particular reaction. They estimated the rate at which the gel is formed within the aggregate
by using the Bazant & Steffens (2000) model followed by application of non-linear diffusion
model (Rahimi- Aghdam et al. 2017) for analysing the permeability of the gel into the
nanopores, microcracks and themicropores. They analysed the destruction by incorporation of
creep and applying microplane model M7. They noted that creep plays a significant role in
the analysis of long term damage. The results predicted by the models were compatible with
the experimental results and the damages in the real structures could also be predicted.
According to their study, the correlating numerical calculations with the experimental
analysis point to the fact that long-term diffusion and creek are two important factors which
initiate the movement of the gel into the pores.
The damages due alkali aggregate reaction were analysed by adopting certain tools as
reported by Sanchez et al (2017). Two lab tools for analysing and identification of the
damages due to alkali-silica reaction have been put forth. These tools are Damage Rating
Index (DRI) and Stiffness Damage Test (SDT). The study describes a unique approach for
the identification of reaction damaged concretes and reported that these tools were capable of
differentiating the rate of damage as a function of the expansion.
37
effective as the results correlated with the other experimental results.
Rahimi- Aghdam & Bazant (2017) reported the necessity of a deeper in-sight of diffusion in
alkali-silica gel based and creep based models for envisaging the gel expansion due to the
particular reaction. They estimated the rate at which the gel is formed within the aggregate
by using the Bazant & Steffens (2000) model followed by application of non-linear diffusion
model (Rahimi- Aghdam et al. 2017) for analysing the permeability of the gel into the
nanopores, microcracks and themicropores. They analysed the destruction by incorporation of
creep and applying microplane model M7. They noted that creep plays a significant role in
the analysis of long term damage. The results predicted by the models were compatible with
the experimental results and the damages in the real structures could also be predicted.
According to their study, the correlating numerical calculations with the experimental
analysis point to the fact that long-term diffusion and creek are two important factors which
initiate the movement of the gel into the pores.
The damages due alkali aggregate reaction were analysed by adopting certain tools as
reported by Sanchez et al (2017). Two lab tools for analysing and identification of the
damages due to alkali-silica reaction have been put forth. These tools are Damage Rating
Index (DRI) and Stiffness Damage Test (SDT). The study describes a unique approach for
the identification of reaction damaged concretes and reported that these tools were capable of
differentiating the rate of damage as a function of the expansion.
37
Paraphrase This Document
Need a fresh take? Get an instant paraphrase of this document with our AI Paraphraser
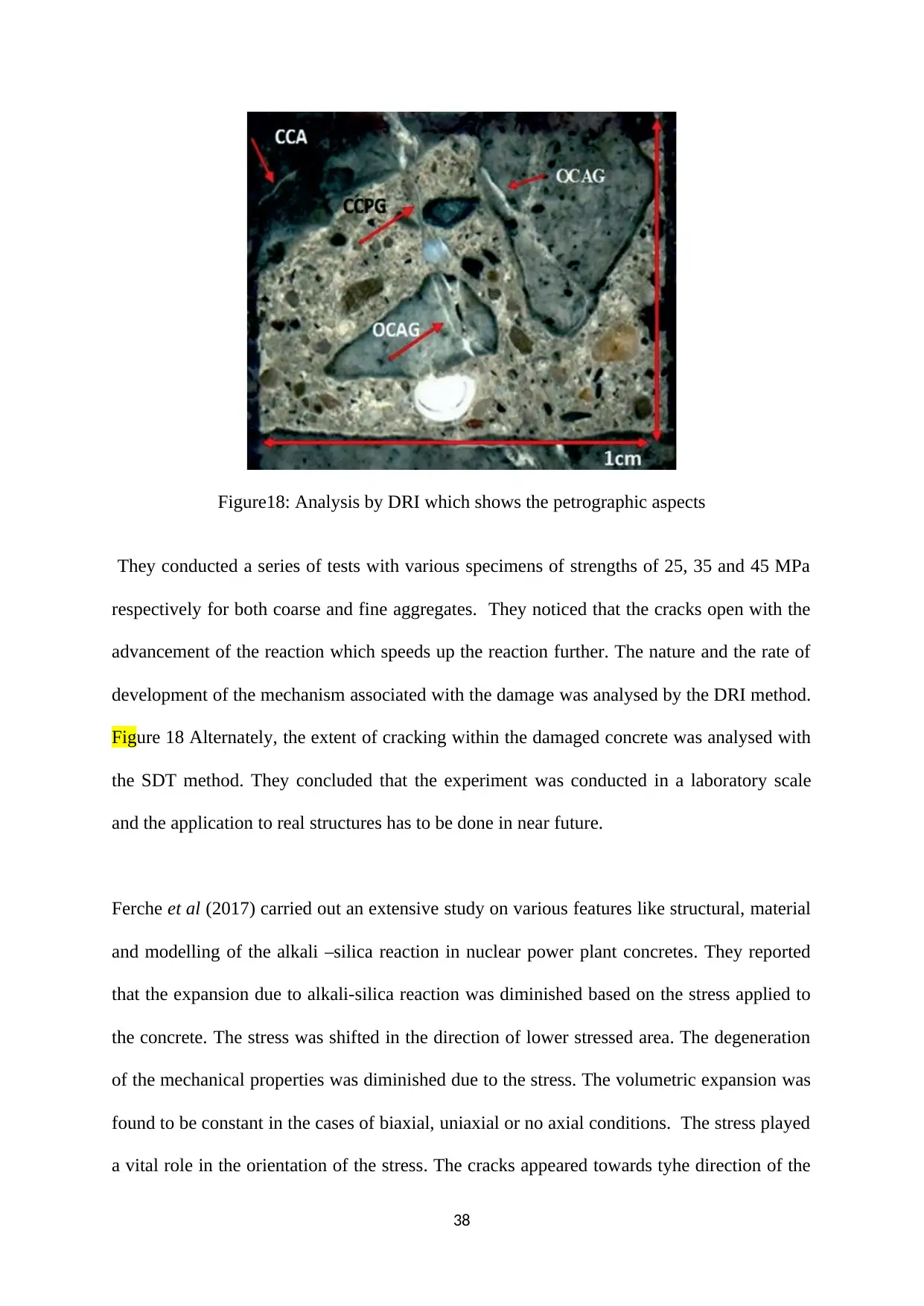
Figure18: Analysis by DRI which shows the petrographic aspects
They conducted a series of tests with various specimens of strengths of 25, 35 and 45 MPa
respectively for both coarse and fine aggregates. They noticed that the cracks open with the
advancement of the reaction which speeds up the reaction further. The nature and the rate of
development of the mechanism associated with the damage was analysed by the DRI method.
Figure 18 Alternately, the extent of cracking within the damaged concrete was analysed with
the SDT method. They concluded that the experiment was conducted in a laboratory scale
and the application to real structures has to be done in near future.
Ferche et al (2017) carried out an extensive study on various features like structural, material
and modelling of the alkali –silica reaction in nuclear power plant concretes. They reported
that the expansion due to alkali-silica reaction was diminished based on the stress applied to
the concrete. The stress was shifted in the direction of lower stressed area. The degeneration
of the mechanical properties was diminished due to the stress. The volumetric expansion was
found to be constant in the cases of biaxial, uniaxial or no axial conditions. The stress played
a vital role in the orientation of the stress. The cracks appeared towards tyhe direction of the
38
They conducted a series of tests with various specimens of strengths of 25, 35 and 45 MPa
respectively for both coarse and fine aggregates. They noticed that the cracks open with the
advancement of the reaction which speeds up the reaction further. The nature and the rate of
development of the mechanism associated with the damage was analysed by the DRI method.
Figure 18 Alternately, the extent of cracking within the damaged concrete was analysed with
the SDT method. They concluded that the experiment was conducted in a laboratory scale
and the application to real structures has to be done in near future.
Ferche et al (2017) carried out an extensive study on various features like structural, material
and modelling of the alkali –silica reaction in nuclear power plant concretes. They reported
that the expansion due to alkali-silica reaction was diminished based on the stress applied to
the concrete. The stress was shifted in the direction of lower stressed area. The degeneration
of the mechanical properties was diminished due to the stress. The volumetric expansion was
found to be constant in the cases of biaxial, uniaxial or no axial conditions. The stress played
a vital role in the orientation of the stress. The cracks appeared towards tyhe direction of the
38
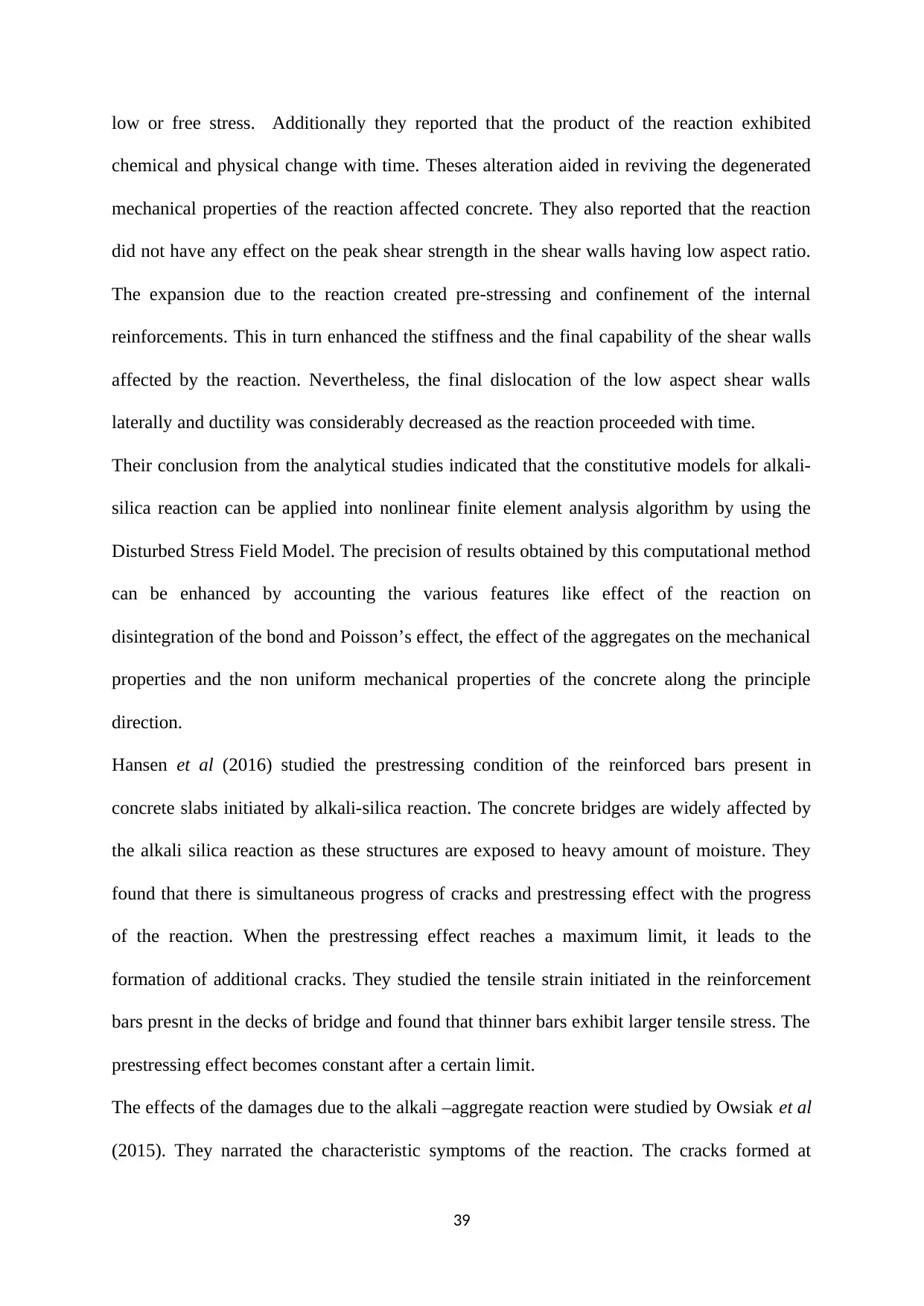
low or free stress. Additionally they reported that the product of the reaction exhibited
chemical and physical change with time. Theses alteration aided in reviving the degenerated
mechanical properties of the reaction affected concrete. They also reported that the reaction
did not have any effect on the peak shear strength in the shear walls having low aspect ratio.
The expansion due to the reaction created pre-stressing and confinement of the internal
reinforcements. This in turn enhanced the stiffness and the final capability of the shear walls
affected by the reaction. Nevertheless, the final dislocation of the low aspect shear walls
laterally and ductility was considerably decreased as the reaction proceeded with time.
Their conclusion from the analytical studies indicated that the constitutive models for alkali-
silica reaction can be applied into nonlinear finite element analysis algorithm by using the
Disturbed Stress Field Model. The precision of results obtained by this computational method
can be enhanced by accounting the various features like effect of the reaction on
disintegration of the bond and Poisson’s effect, the effect of the aggregates on the mechanical
properties and the non uniform mechanical properties of the concrete along the principle
direction.
Hansen et al (2016) studied the prestressing condition of the reinforced bars present in
concrete slabs initiated by alkali-silica reaction. The concrete bridges are widely affected by
the alkali silica reaction as these structures are exposed to heavy amount of moisture. They
found that there is simultaneous progress of cracks and prestressing effect with the progress
of the reaction. When the prestressing effect reaches a maximum limit, it leads to the
formation of additional cracks. They studied the tensile strain initiated in the reinforcement
bars presnt in the decks of bridge and found that thinner bars exhibit larger tensile stress. The
prestressing effect becomes constant after a certain limit.
The effects of the damages due to the alkali –aggregate reaction were studied by Owsiak et al
(2015). They narrated the characteristic symptoms of the reaction. The cracks formed at
39
chemical and physical change with time. Theses alteration aided in reviving the degenerated
mechanical properties of the reaction affected concrete. They also reported that the reaction
did not have any effect on the peak shear strength in the shear walls having low aspect ratio.
The expansion due to the reaction created pre-stressing and confinement of the internal
reinforcements. This in turn enhanced the stiffness and the final capability of the shear walls
affected by the reaction. Nevertheless, the final dislocation of the low aspect shear walls
laterally and ductility was considerably decreased as the reaction proceeded with time.
Their conclusion from the analytical studies indicated that the constitutive models for alkali-
silica reaction can be applied into nonlinear finite element analysis algorithm by using the
Disturbed Stress Field Model. The precision of results obtained by this computational method
can be enhanced by accounting the various features like effect of the reaction on
disintegration of the bond and Poisson’s effect, the effect of the aggregates on the mechanical
properties and the non uniform mechanical properties of the concrete along the principle
direction.
Hansen et al (2016) studied the prestressing condition of the reinforced bars present in
concrete slabs initiated by alkali-silica reaction. The concrete bridges are widely affected by
the alkali silica reaction as these structures are exposed to heavy amount of moisture. They
found that there is simultaneous progress of cracks and prestressing effect with the progress
of the reaction. When the prestressing effect reaches a maximum limit, it leads to the
formation of additional cracks. They studied the tensile strain initiated in the reinforcement
bars presnt in the decks of bridge and found that thinner bars exhibit larger tensile stress. The
prestressing effect becomes constant after a certain limit.
The effects of the damages due to the alkali –aggregate reaction were studied by Owsiak et al
(2015). They narrated the characteristic symptoms of the reaction. The cracks formed at
39
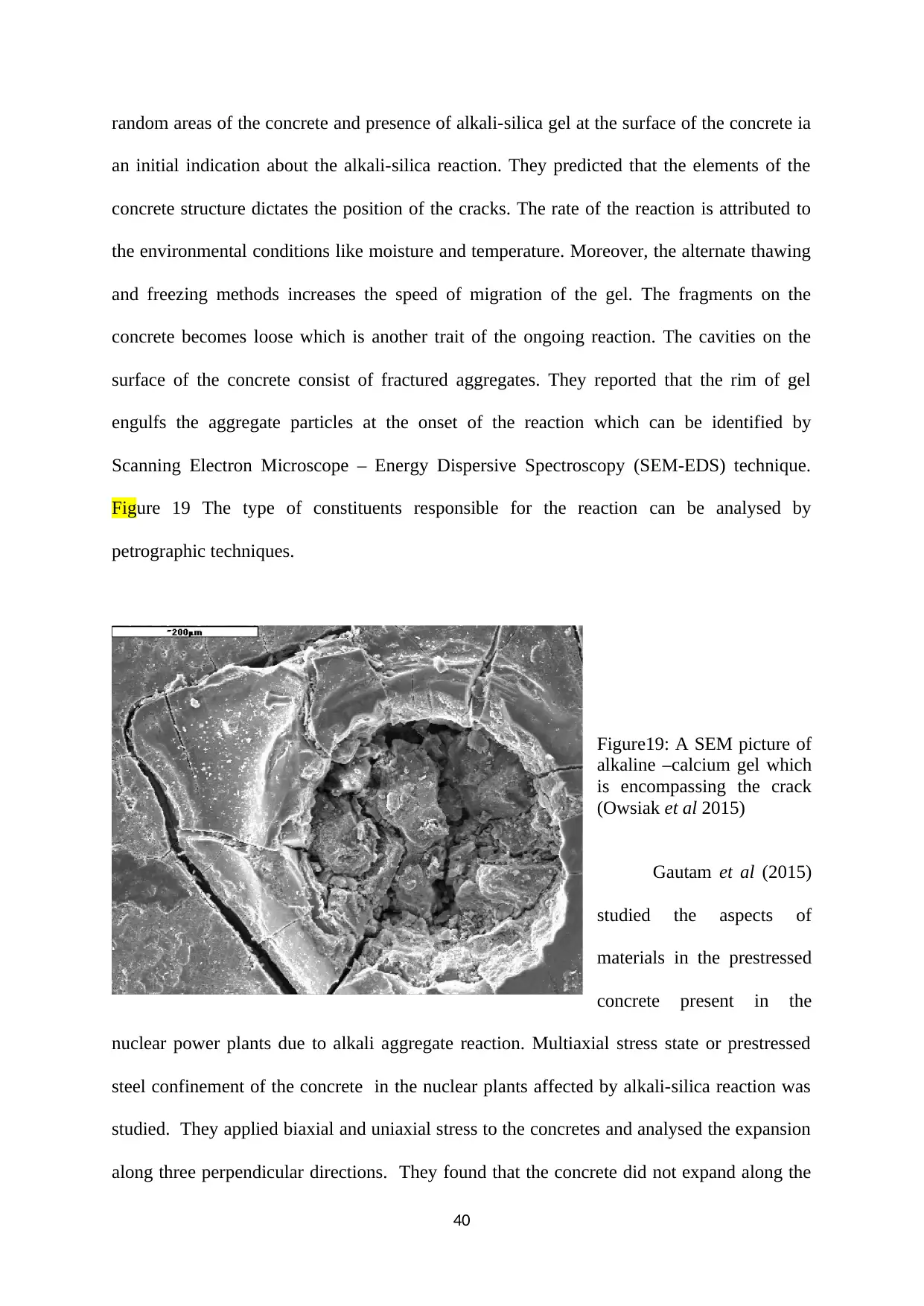
random areas of the concrete and presence of alkali-silica gel at the surface of the concrete ia
an initial indication about the alkali-silica reaction. They predicted that the elements of the
concrete structure dictates the position of the cracks. The rate of the reaction is attributed to
the environmental conditions like moisture and temperature. Moreover, the alternate thawing
and freezing methods increases the speed of migration of the gel. The fragments on the
concrete becomes loose which is another trait of the ongoing reaction. The cavities on the
surface of the concrete consist of fractured aggregates. They reported that the rim of gel
engulfs the aggregate particles at the onset of the reaction which can be identified by
Scanning Electron Microscope – Energy Dispersive Spectroscopy (SEM-EDS) technique.
Figure 19 The type of constituents responsible for the reaction can be analysed by
petrographic techniques.
Figure19: A SEM picture of
alkaline –calcium gel which
is encompassing the crack
(Owsiak et al 2015)
Gautam et al (2015)
studied the aspects of
materials in the prestressed
concrete present in the
nuclear power plants due to alkali aggregate reaction. Multiaxial stress state or prestressed
steel confinement of the concrete in the nuclear plants affected by alkali-silica reaction was
studied. They applied biaxial and uniaxial stress to the concretes and analysed the expansion
along three perpendicular directions. They found that the concrete did not expand along the
40
an initial indication about the alkali-silica reaction. They predicted that the elements of the
concrete structure dictates the position of the cracks. The rate of the reaction is attributed to
the environmental conditions like moisture and temperature. Moreover, the alternate thawing
and freezing methods increases the speed of migration of the gel. The fragments on the
concrete becomes loose which is another trait of the ongoing reaction. The cavities on the
surface of the concrete consist of fractured aggregates. They reported that the rim of gel
engulfs the aggregate particles at the onset of the reaction which can be identified by
Scanning Electron Microscope – Energy Dispersive Spectroscopy (SEM-EDS) technique.
Figure 19 The type of constituents responsible for the reaction can be analysed by
petrographic techniques.
Figure19: A SEM picture of
alkaline –calcium gel which
is encompassing the crack
(Owsiak et al 2015)
Gautam et al (2015)
studied the aspects of
materials in the prestressed
concrete present in the
nuclear power plants due to alkali aggregate reaction. Multiaxial stress state or prestressed
steel confinement of the concrete in the nuclear plants affected by alkali-silica reaction was
studied. They applied biaxial and uniaxial stress to the concretes and analysed the expansion
along three perpendicular directions. They found that the concrete did not expand along the
40
Secure Best Marks with AI Grader
Need help grading? Try our AI Grader for instant feedback on your assignments.
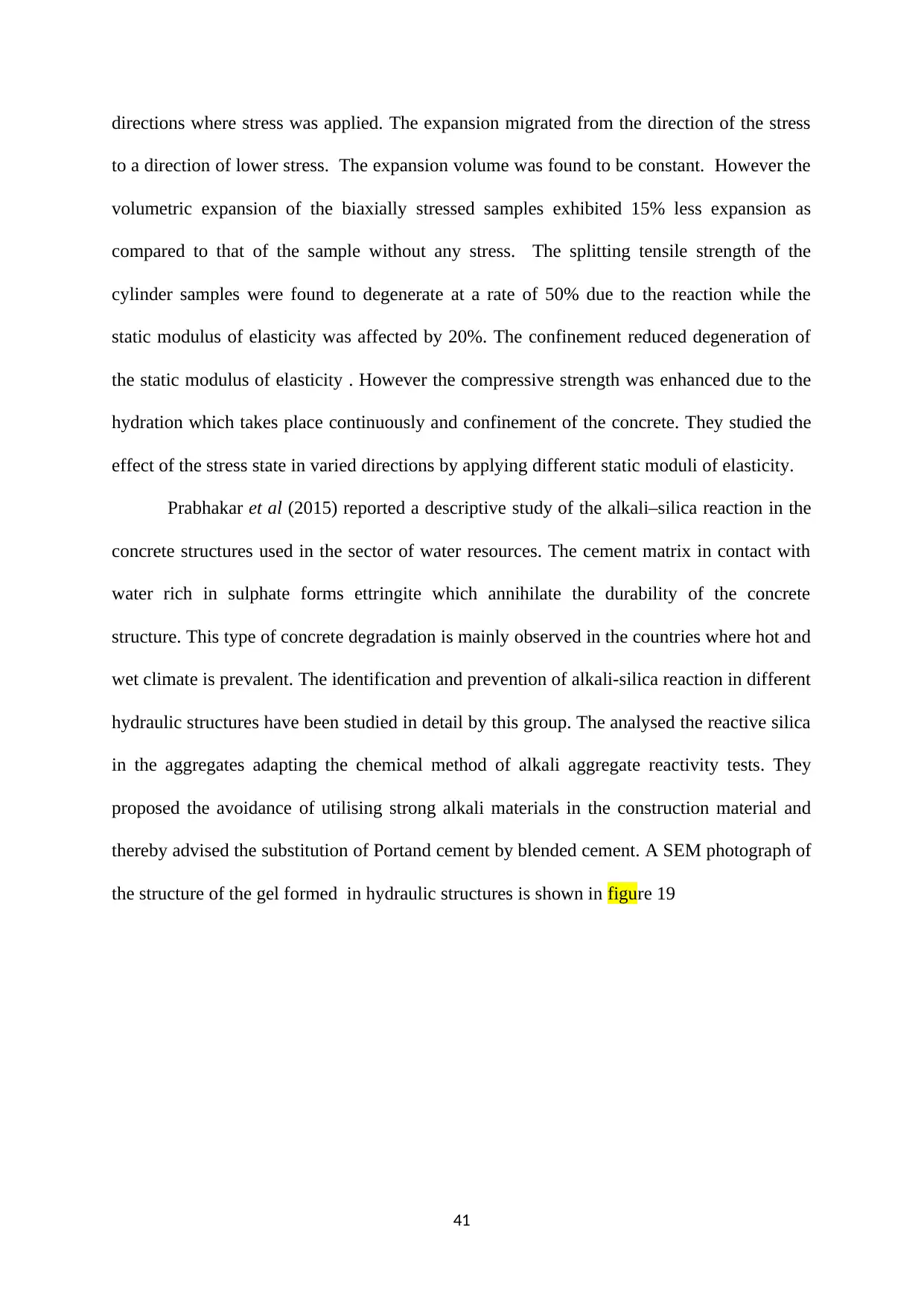
directions where stress was applied. The expansion migrated from the direction of the stress
to a direction of lower stress. The expansion volume was found to be constant. However the
volumetric expansion of the biaxially stressed samples exhibited 15% less expansion as
compared to that of the sample without any stress. The splitting tensile strength of the
cylinder samples were found to degenerate at a rate of 50% due to the reaction while the
static modulus of elasticity was affected by 20%. The confinement reduced degeneration of
the static modulus of elasticity . However the compressive strength was enhanced due to the
hydration which takes place continuously and confinement of the concrete. They studied the
effect of the stress state in varied directions by applying different static moduli of elasticity.
Prabhakar et al (2015) reported a descriptive study of the alkali–silica reaction in the
concrete structures used in the sector of water resources. The cement matrix in contact with
water rich in sulphate forms ettringite which annihilate the durability of the concrete
structure. This type of concrete degradation is mainly observed in the countries where hot and
wet climate is prevalent. The identification and prevention of alkali-silica reaction in different
hydraulic structures have been studied in detail by this group. The analysed the reactive silica
in the aggregates adapting the chemical method of alkali aggregate reactivity tests. They
proposed the avoidance of utilising strong alkali materials in the construction material and
thereby advised the substitution of Portand cement by blended cement. A SEM photograph of
the structure of the gel formed in hydraulic structures is shown in figure 19
41
to a direction of lower stress. The expansion volume was found to be constant. However the
volumetric expansion of the biaxially stressed samples exhibited 15% less expansion as
compared to that of the sample without any stress. The splitting tensile strength of the
cylinder samples were found to degenerate at a rate of 50% due to the reaction while the
static modulus of elasticity was affected by 20%. The confinement reduced degeneration of
the static modulus of elasticity . However the compressive strength was enhanced due to the
hydration which takes place continuously and confinement of the concrete. They studied the
effect of the stress state in varied directions by applying different static moduli of elasticity.
Prabhakar et al (2015) reported a descriptive study of the alkali–silica reaction in the
concrete structures used in the sector of water resources. The cement matrix in contact with
water rich in sulphate forms ettringite which annihilate the durability of the concrete
structure. This type of concrete degradation is mainly observed in the countries where hot and
wet climate is prevalent. The identification and prevention of alkali-silica reaction in different
hydraulic structures have been studied in detail by this group. The analysed the reactive silica
in the aggregates adapting the chemical method of alkali aggregate reactivity tests. They
proposed the avoidance of utilising strong alkali materials in the construction material and
thereby advised the substitution of Portand cement by blended cement. A SEM photograph of
the structure of the gel formed in hydraulic structures is shown in figure 19
41
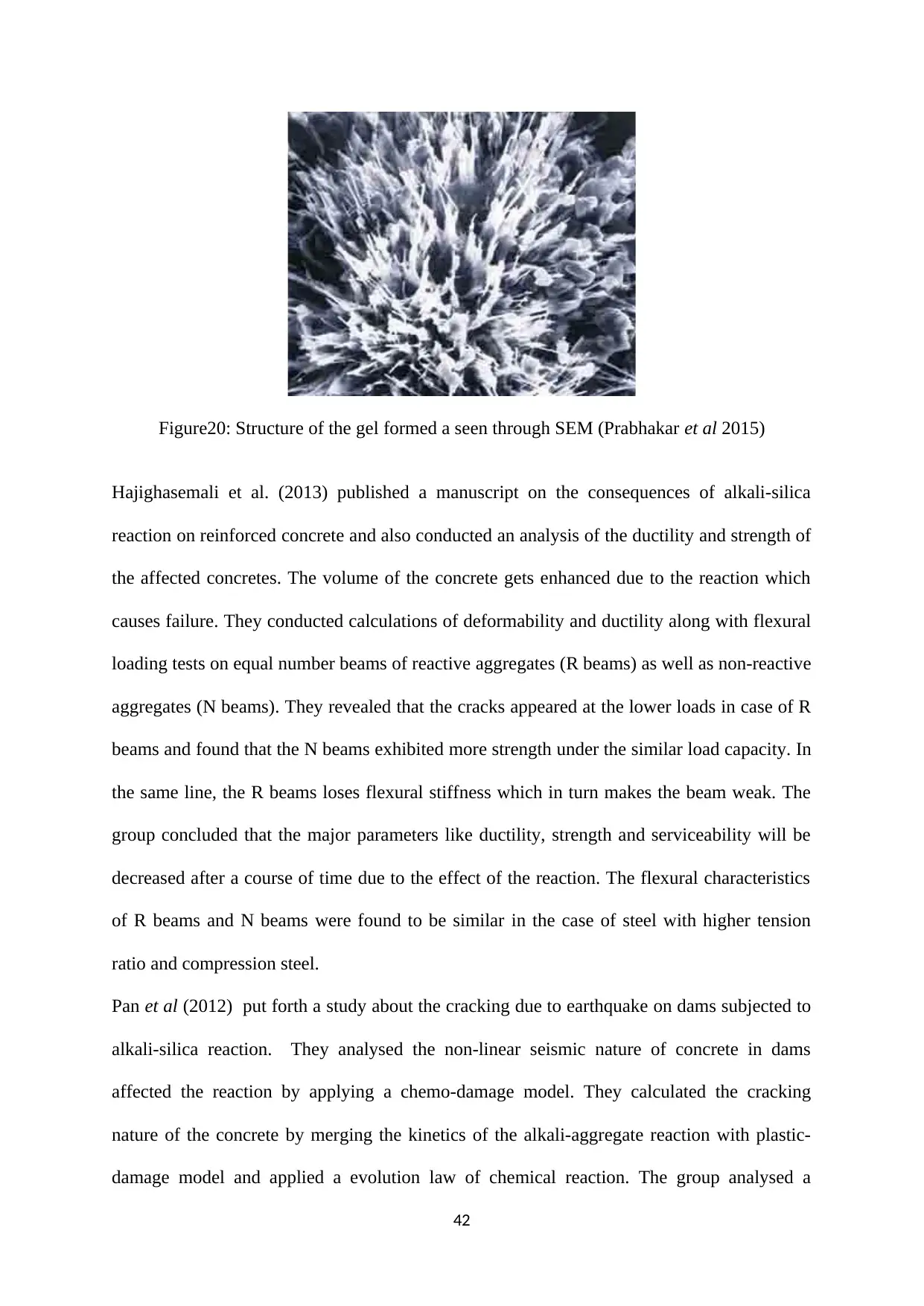
Figure20: Structure of the gel formed a seen through SEM (Prabhakar et al 2015)
Hajighasemali et al. (2013) published a manuscript on the consequences of alkali-silica
reaction on reinforced concrete and also conducted an analysis of the ductility and strength of
the affected concretes. The volume of the concrete gets enhanced due to the reaction which
causes failure. They conducted calculations of deformability and ductility along with flexural
loading tests on equal number beams of reactive aggregates (R beams) as well as non-reactive
aggregates (N beams). They revealed that the cracks appeared at the lower loads in case of R
beams and found that the N beams exhibited more strength under the similar load capacity. In
the same line, the R beams loses flexural stiffness which in turn makes the beam weak. The
group concluded that the major parameters like ductility, strength and serviceability will be
decreased after a course of time due to the effect of the reaction. The flexural characteristics
of R beams and N beams were found to be similar in the case of steel with higher tension
ratio and compression steel.
Pan et al (2012) put forth a study about the cracking due to earthquake on dams subjected to
alkali-silica reaction. They analysed the non-linear seismic nature of concrete in dams
affected the reaction by applying a chemo-damage model. They calculated the cracking
nature of the concrete by merging the kinetics of the alkali-aggregate reaction with plastic-
damage model and applied a evolution law of chemical reaction. The group analysed a
42
Hajighasemali et al. (2013) published a manuscript on the consequences of alkali-silica
reaction on reinforced concrete and also conducted an analysis of the ductility and strength of
the affected concretes. The volume of the concrete gets enhanced due to the reaction which
causes failure. They conducted calculations of deformability and ductility along with flexural
loading tests on equal number beams of reactive aggregates (R beams) as well as non-reactive
aggregates (N beams). They revealed that the cracks appeared at the lower loads in case of R
beams and found that the N beams exhibited more strength under the similar load capacity. In
the same line, the R beams loses flexural stiffness which in turn makes the beam weak. The
group concluded that the major parameters like ductility, strength and serviceability will be
decreased after a course of time due to the effect of the reaction. The flexural characteristics
of R beams and N beams were found to be similar in the case of steel with higher tension
ratio and compression steel.
Pan et al (2012) put forth a study about the cracking due to earthquake on dams subjected to
alkali-silica reaction. They analysed the non-linear seismic nature of concrete in dams
affected the reaction by applying a chemo-damage model. They calculated the cracking
nature of the concrete by merging the kinetics of the alkali-aggregate reaction with plastic-
damage model and applied a evolution law of chemical reaction. The group analysed a
42
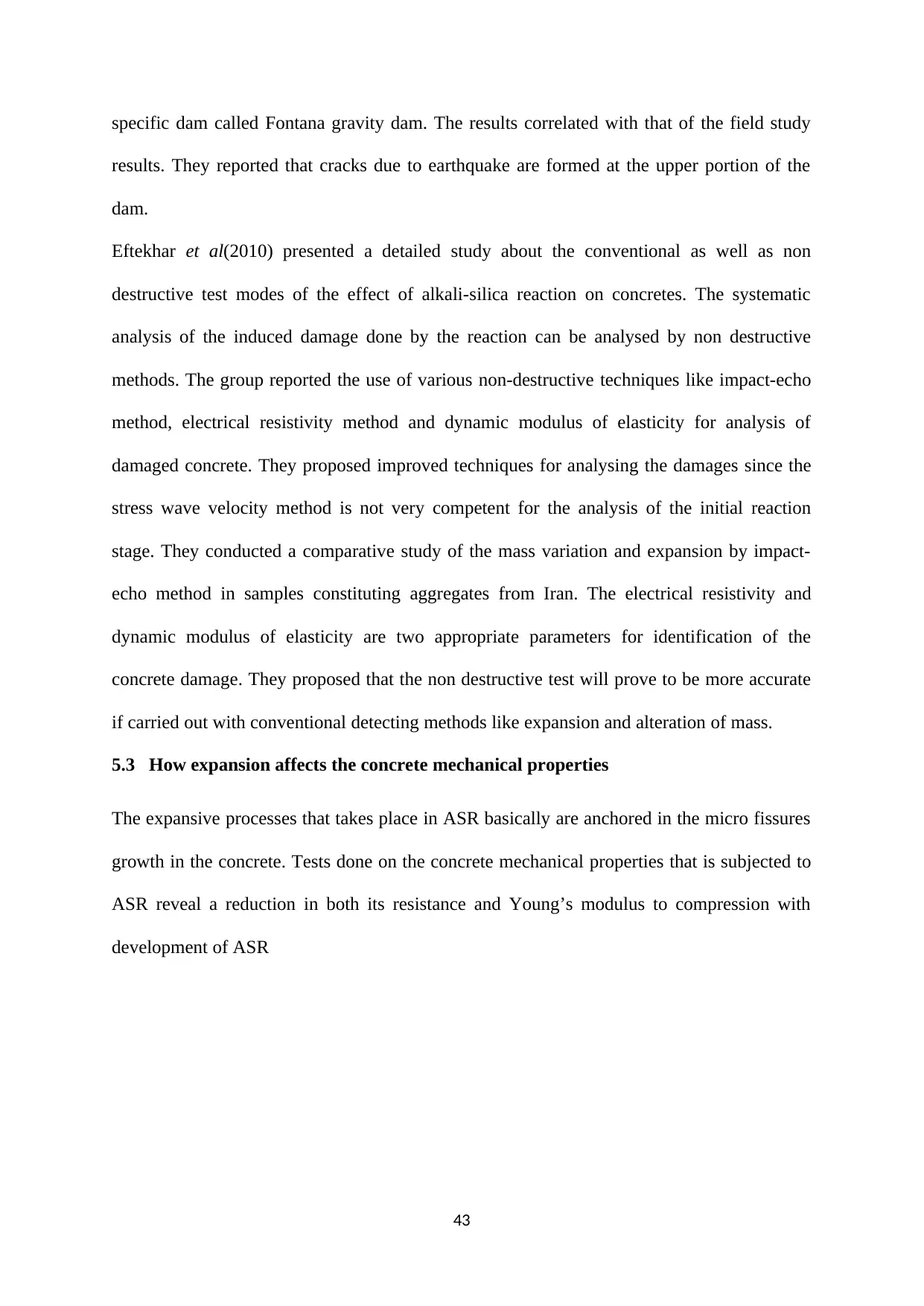
specific dam called Fontana gravity dam. The results correlated with that of the field study
results. They reported that cracks due to earthquake are formed at the upper portion of the
dam.
Eftekhar et al(2010) presented a detailed study about the conventional as well as non
destructive test modes of the effect of alkali-silica reaction on concretes. The systematic
analysis of the induced damage done by the reaction can be analysed by non destructive
methods. The group reported the use of various non-destructive techniques like impact-echo
method, electrical resistivity method and dynamic modulus of elasticity for analysis of
damaged concrete. They proposed improved techniques for analysing the damages since the
stress wave velocity method is not very competent for the analysis of the initial reaction
stage. They conducted a comparative study of the mass variation and expansion by impact-
echo method in samples constituting aggregates from Iran. The electrical resistivity and
dynamic modulus of elasticity are two appropriate parameters for identification of the
concrete damage. They proposed that the non destructive test will prove to be more accurate
if carried out with conventional detecting methods like expansion and alteration of mass.
5.3 How expansion affects the concrete mechanical properties
The expansive processes that takes place in ASR basically are anchored in the micro fissures
growth in the concrete. Tests done on the concrete mechanical properties that is subjected to
ASR reveal a reduction in both its resistance and Young’s modulus to compression with
development of ASR
43
results. They reported that cracks due to earthquake are formed at the upper portion of the
dam.
Eftekhar et al(2010) presented a detailed study about the conventional as well as non
destructive test modes of the effect of alkali-silica reaction on concretes. The systematic
analysis of the induced damage done by the reaction can be analysed by non destructive
methods. The group reported the use of various non-destructive techniques like impact-echo
method, electrical resistivity method and dynamic modulus of elasticity for analysis of
damaged concrete. They proposed improved techniques for analysing the damages since the
stress wave velocity method is not very competent for the analysis of the initial reaction
stage. They conducted a comparative study of the mass variation and expansion by impact-
echo method in samples constituting aggregates from Iran. The electrical resistivity and
dynamic modulus of elasticity are two appropriate parameters for identification of the
concrete damage. They proposed that the non destructive test will prove to be more accurate
if carried out with conventional detecting methods like expansion and alteration of mass.
5.3 How expansion affects the concrete mechanical properties
The expansive processes that takes place in ASR basically are anchored in the micro fissures
growth in the concrete. Tests done on the concrete mechanical properties that is subjected to
ASR reveal a reduction in both its resistance and Young’s modulus to compression with
development of ASR
43
Paraphrase This Document
Need a fresh take? Get an instant paraphrase of this document with our AI Paraphraser
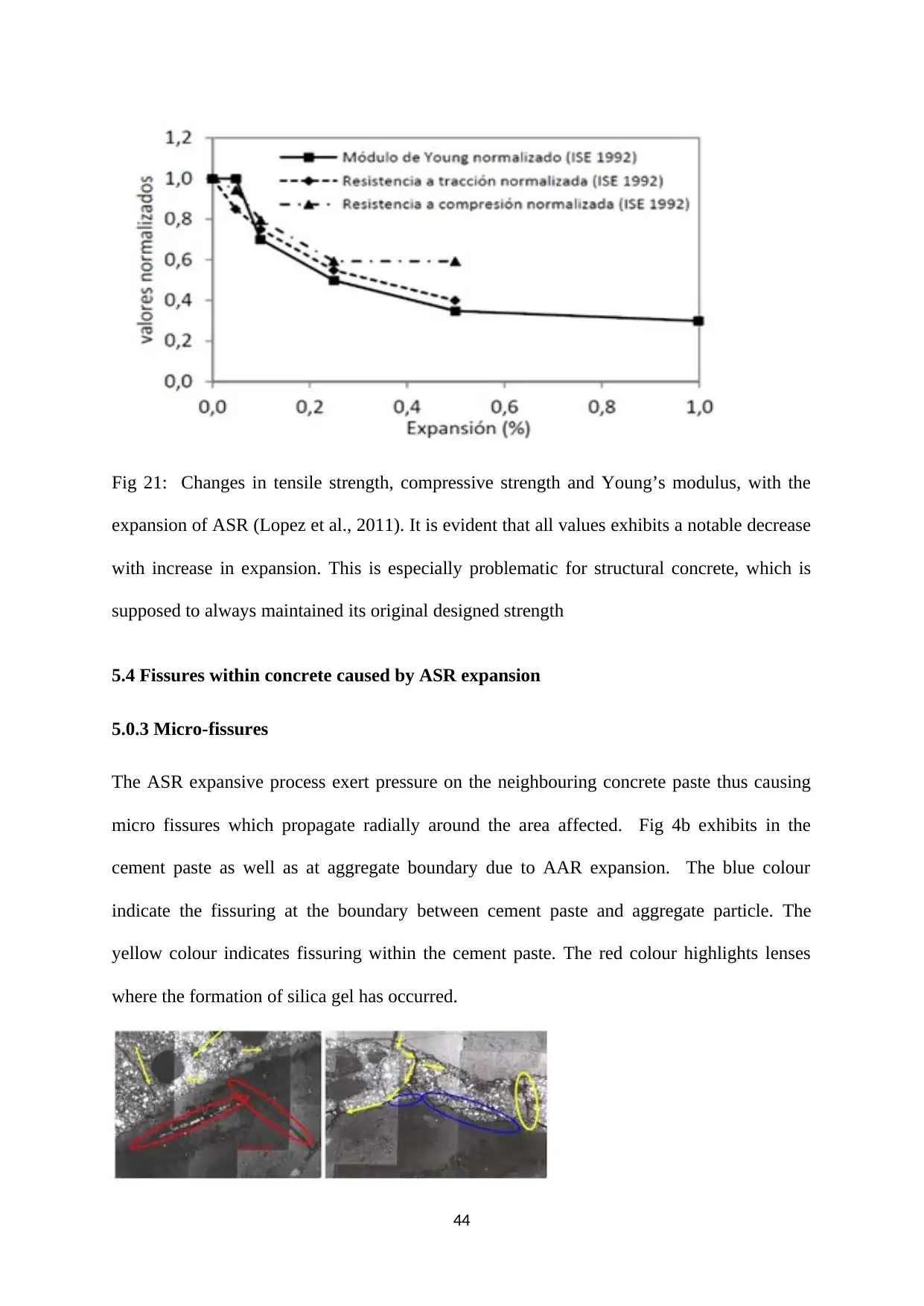
Fig 21: Changes in tensile strength, compressive strength and Young’s modulus, with the
expansion of ASR (Lopez et al., 2011). It is evident that all values exhibits a notable decrease
with increase in expansion. This is especially problematic for structural concrete, which is
supposed to always maintained its original designed strength
5.4 Fissures within concrete caused by ASR expansion
5.0.3 Micro-fissures
The ASR expansive process exert pressure on the neighbouring concrete paste thus causing
micro fissures which propagate radially around the area affected. Fig 4b exhibits in the
cement paste as well as at aggregate boundary due to AAR expansion. The blue colour
indicate the fissuring at the boundary between cement paste and aggregate particle. The
yellow colour indicates fissuring within the cement paste. The red colour highlights lenses
where the formation of silica gel has occurred.
44
expansion of ASR (Lopez et al., 2011). It is evident that all values exhibits a notable decrease
with increase in expansion. This is especially problematic for structural concrete, which is
supposed to always maintained its original designed strength
5.4 Fissures within concrete caused by ASR expansion
5.0.3 Micro-fissures
The ASR expansive process exert pressure on the neighbouring concrete paste thus causing
micro fissures which propagate radially around the area affected. Fig 4b exhibits in the
cement paste as well as at aggregate boundary due to AAR expansion. The blue colour
indicate the fissuring at the boundary between cement paste and aggregate particle. The
yellow colour indicates fissuring within the cement paste. The red colour highlights lenses
where the formation of silica gel has occurred.
44
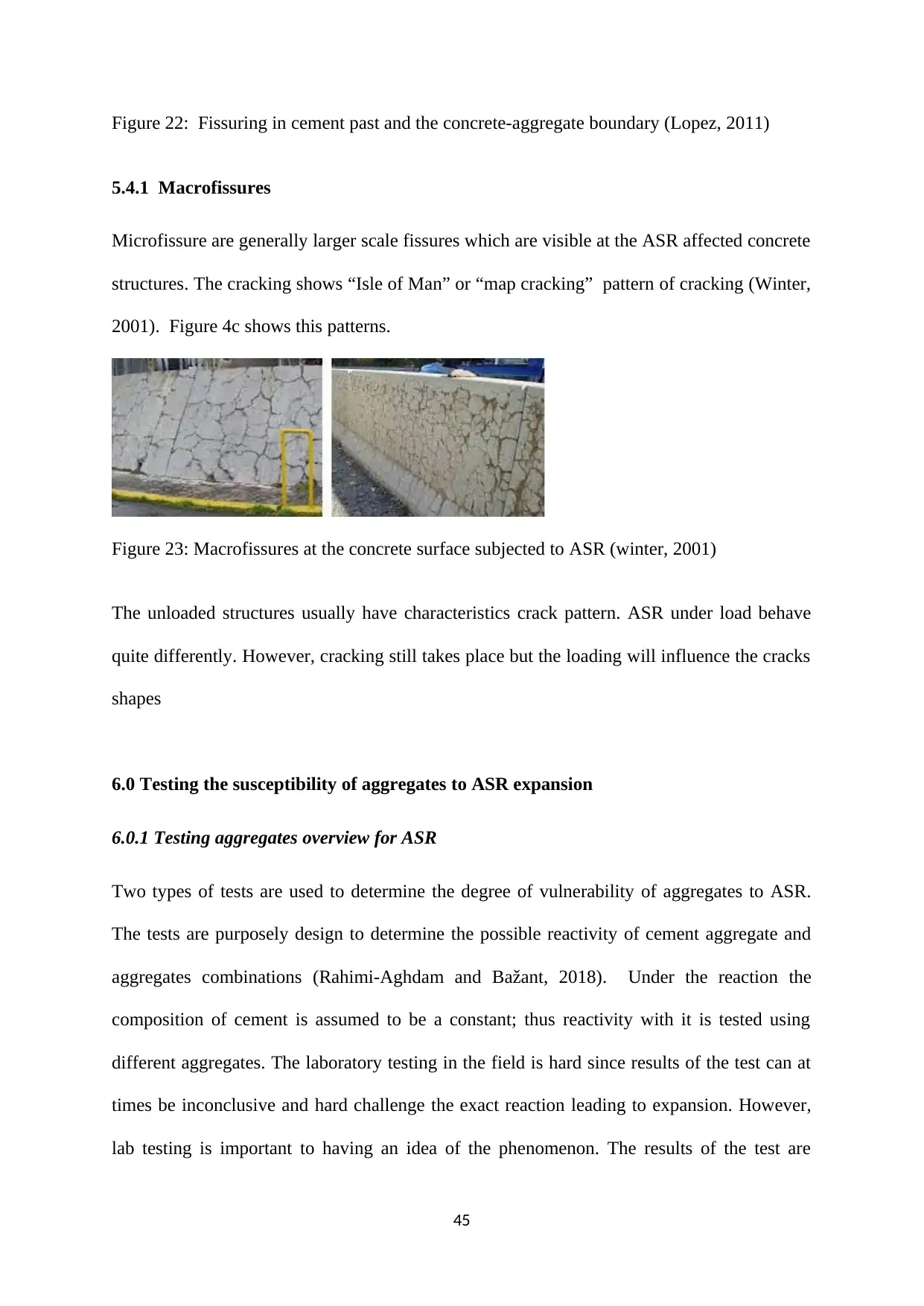
Figure 22: Fissuring in cement past and the concrete-aggregate boundary (Lopez, 2011)
5.4.1 Macrofissures
Microfissure are generally larger scale fissures which are visible at the ASR affected concrete
structures. The cracking shows “Isle of Man” or “map cracking” pattern of cracking (Winter,
2001). Figure 4c shows this patterns.
Figure 23: Macrofissures at the concrete surface subjected to ASR (winter, 2001)
The unloaded structures usually have characteristics crack pattern. ASR under load behave
quite differently. However, cracking still takes place but the loading will influence the cracks
shapes
6.0 Testing the susceptibility of aggregates to ASR expansion
6.0.1 Testing aggregates overview for ASR
Two types of tests are used to determine the degree of vulnerability of aggregates to ASR.
The tests are purposely design to determine the possible reactivity of cement aggregate and
aggregates combinations (Rahimi-Aghdam and Bažant, 2018). Under the reaction the
composition of cement is assumed to be a constant; thus reactivity with it is tested using
different aggregates. The laboratory testing in the field is hard since results of the test can at
times be inconclusive and hard challenge the exact reaction leading to expansion. However,
lab testing is important to having an idea of the phenomenon. The results of the test are
45
5.4.1 Macrofissures
Microfissure are generally larger scale fissures which are visible at the ASR affected concrete
structures. The cracking shows “Isle of Man” or “map cracking” pattern of cracking (Winter,
2001). Figure 4c shows this patterns.
Figure 23: Macrofissures at the concrete surface subjected to ASR (winter, 2001)
The unloaded structures usually have characteristics crack pattern. ASR under load behave
quite differently. However, cracking still takes place but the loading will influence the cracks
shapes
6.0 Testing the susceptibility of aggregates to ASR expansion
6.0.1 Testing aggregates overview for ASR
Two types of tests are used to determine the degree of vulnerability of aggregates to ASR.
The tests are purposely design to determine the possible reactivity of cement aggregate and
aggregates combinations (Rahimi-Aghdam and Bažant, 2018). Under the reaction the
composition of cement is assumed to be a constant; thus reactivity with it is tested using
different aggregates. The laboratory testing in the field is hard since results of the test can at
times be inconclusive and hard challenge the exact reaction leading to expansion. However,
lab testing is important to having an idea of the phenomenon. The results of the test are
45
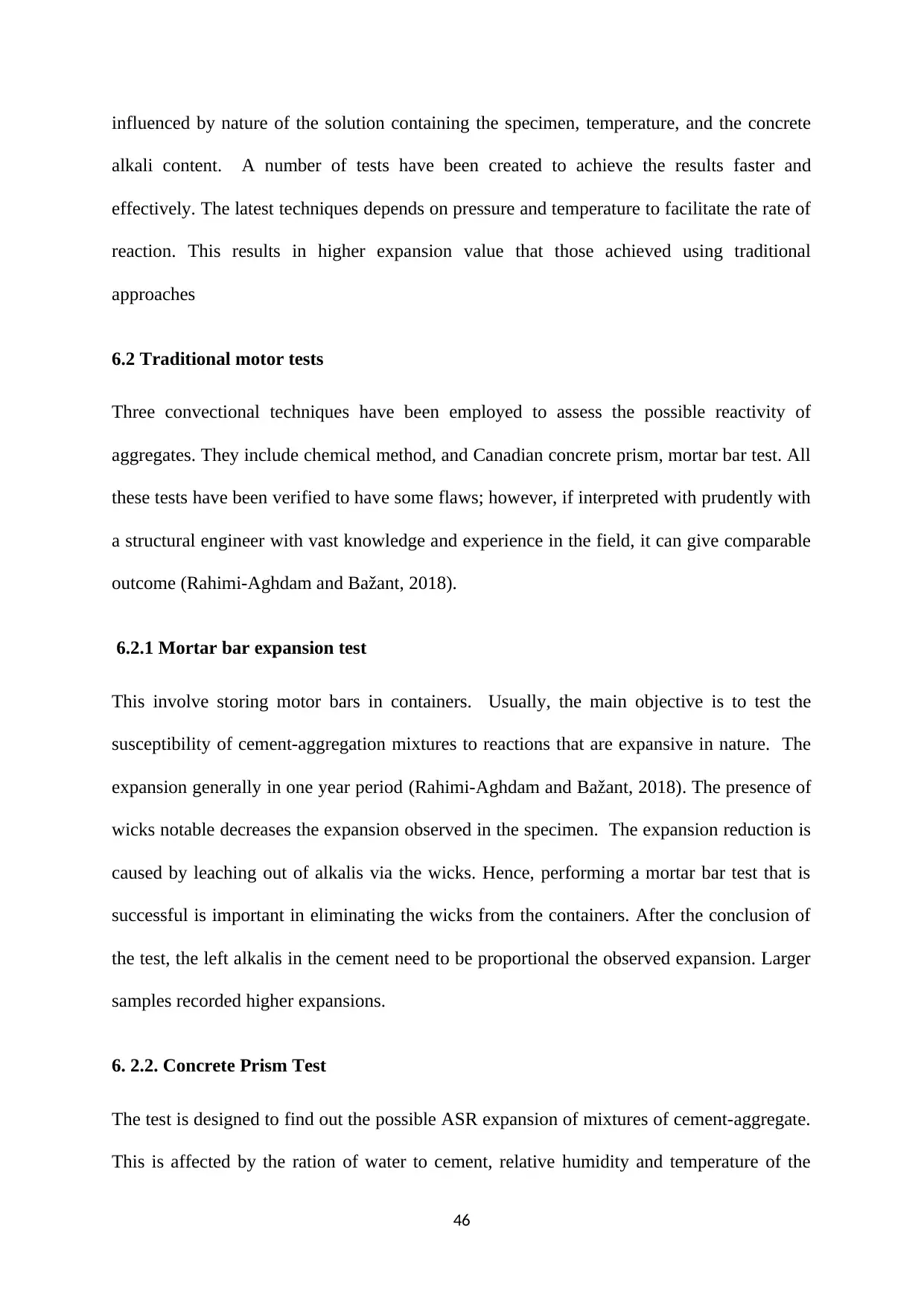
influenced by nature of the solution containing the specimen, temperature, and the concrete
alkali content. A number of tests have been created to achieve the results faster and
effectively. The latest techniques depends on pressure and temperature to facilitate the rate of
reaction. This results in higher expansion value that those achieved using traditional
approaches
6.2 Traditional motor tests
Three convectional techniques have been employed to assess the possible reactivity of
aggregates. They include chemical method, and Canadian concrete prism, mortar bar test. All
these tests have been verified to have some flaws; however, if interpreted with prudently with
a structural engineer with vast knowledge and experience in the field, it can give comparable
outcome (Rahimi-Aghdam and Bažant, 2018).
6.2.1 Mortar bar expansion test
This involve storing motor bars in containers. Usually, the main objective is to test the
susceptibility of cement-aggregation mixtures to reactions that are expansive in nature. The
expansion generally in one year period (Rahimi-Aghdam and Bažant, 2018). The presence of
wicks notable decreases the expansion observed in the specimen. The expansion reduction is
caused by leaching out of alkalis via the wicks. Hence, performing a mortar bar test that is
successful is important in eliminating the wicks from the containers. After the conclusion of
the test, the left alkalis in the cement need to be proportional the observed expansion. Larger
samples recorded higher expansions.
6. 2.2. Concrete Prism Test
The test is designed to find out the possible ASR expansion of mixtures of cement-aggregate.
This is affected by the ration of water to cement, relative humidity and temperature of the
46
alkali content. A number of tests have been created to achieve the results faster and
effectively. The latest techniques depends on pressure and temperature to facilitate the rate of
reaction. This results in higher expansion value that those achieved using traditional
approaches
6.2 Traditional motor tests
Three convectional techniques have been employed to assess the possible reactivity of
aggregates. They include chemical method, and Canadian concrete prism, mortar bar test. All
these tests have been verified to have some flaws; however, if interpreted with prudently with
a structural engineer with vast knowledge and experience in the field, it can give comparable
outcome (Rahimi-Aghdam and Bažant, 2018).
6.2.1 Mortar bar expansion test
This involve storing motor bars in containers. Usually, the main objective is to test the
susceptibility of cement-aggregation mixtures to reactions that are expansive in nature. The
expansion generally in one year period (Rahimi-Aghdam and Bažant, 2018). The presence of
wicks notable decreases the expansion observed in the specimen. The expansion reduction is
caused by leaching out of alkalis via the wicks. Hence, performing a mortar bar test that is
successful is important in eliminating the wicks from the containers. After the conclusion of
the test, the left alkalis in the cement need to be proportional the observed expansion. Larger
samples recorded higher expansions.
6. 2.2. Concrete Prism Test
The test is designed to find out the possible ASR expansion of mixtures of cement-aggregate.
This is affected by the ration of water to cement, relative humidity and temperature of the
46
Secure Best Marks with AI Grader
Need help grading? Try our AI Grader for instant feedback on your assignments.
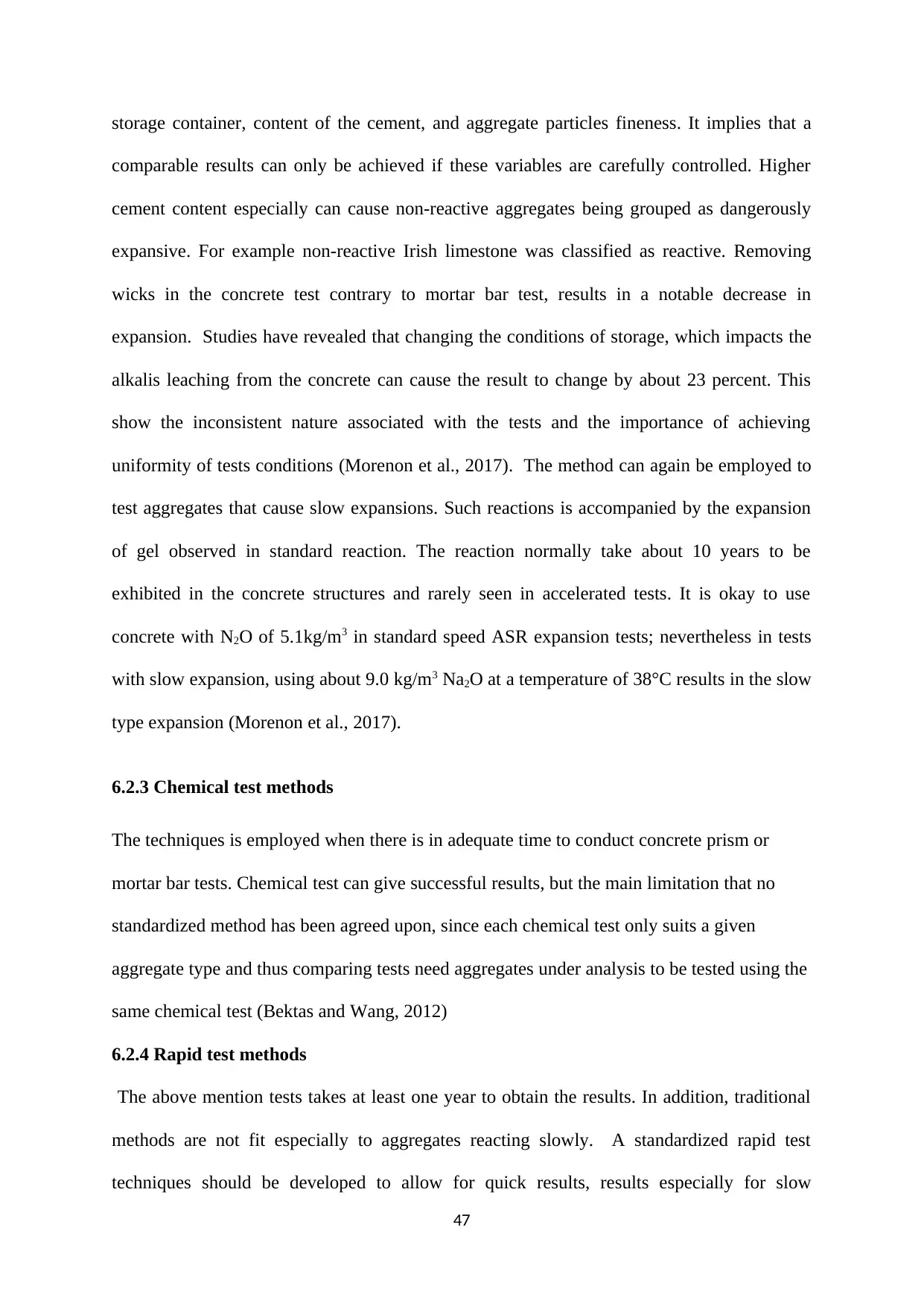
storage container, content of the cement, and aggregate particles fineness. It implies that a
comparable results can only be achieved if these variables are carefully controlled. Higher
cement content especially can cause non-reactive aggregates being grouped as dangerously
expansive. For example non-reactive Irish limestone was classified as reactive. Removing
wicks in the concrete test contrary to mortar bar test, results in a notable decrease in
expansion. Studies have revealed that changing the conditions of storage, which impacts the
alkalis leaching from the concrete can cause the result to change by about 23 percent. This
show the inconsistent nature associated with the tests and the importance of achieving
uniformity of tests conditions (Morenon et al., 2017). The method can again be employed to
test aggregates that cause slow expansions. Such reactions is accompanied by the expansion
of gel observed in standard reaction. The reaction normally take about 10 years to be
exhibited in the concrete structures and rarely seen in accelerated tests. It is okay to use
concrete with N2O of 5.1kg/m3 in standard speed ASR expansion tests; nevertheless in tests
with slow expansion, using about 9.0 kg/m3 Na2O at a temperature of 38°C results in the slow
type expansion (Morenon et al., 2017).
6.2.3 Chemical test methods
The techniques is employed when there is in adequate time to conduct concrete prism or
mortar bar tests. Chemical test can give successful results, but the main limitation that no
standardized method has been agreed upon, since each chemical test only suits a given
aggregate type and thus comparing tests need aggregates under analysis to be tested using the
same chemical test (Bektas and Wang, 2012)
6.2.4 Rapid test methods
The above mention tests takes at least one year to obtain the results. In addition, traditional
methods are not fit especially to aggregates reacting slowly. A standardized rapid test
techniques should be developed to allow for quick results, results especially for slow
47
comparable results can only be achieved if these variables are carefully controlled. Higher
cement content especially can cause non-reactive aggregates being grouped as dangerously
expansive. For example non-reactive Irish limestone was classified as reactive. Removing
wicks in the concrete test contrary to mortar bar test, results in a notable decrease in
expansion. Studies have revealed that changing the conditions of storage, which impacts the
alkalis leaching from the concrete can cause the result to change by about 23 percent. This
show the inconsistent nature associated with the tests and the importance of achieving
uniformity of tests conditions (Morenon et al., 2017). The method can again be employed to
test aggregates that cause slow expansions. Such reactions is accompanied by the expansion
of gel observed in standard reaction. The reaction normally take about 10 years to be
exhibited in the concrete structures and rarely seen in accelerated tests. It is okay to use
concrete with N2O of 5.1kg/m3 in standard speed ASR expansion tests; nevertheless in tests
with slow expansion, using about 9.0 kg/m3 Na2O at a temperature of 38°C results in the slow
type expansion (Morenon et al., 2017).
6.2.3 Chemical test methods
The techniques is employed when there is in adequate time to conduct concrete prism or
mortar bar tests. Chemical test can give successful results, but the main limitation that no
standardized method has been agreed upon, since each chemical test only suits a given
aggregate type and thus comparing tests need aggregates under analysis to be tested using the
same chemical test (Bektas and Wang, 2012)
6.2.4 Rapid test methods
The above mention tests takes at least one year to obtain the results. In addition, traditional
methods are not fit especially to aggregates reacting slowly. A standardized rapid test
techniques should be developed to allow for quick results, results especially for slow
47
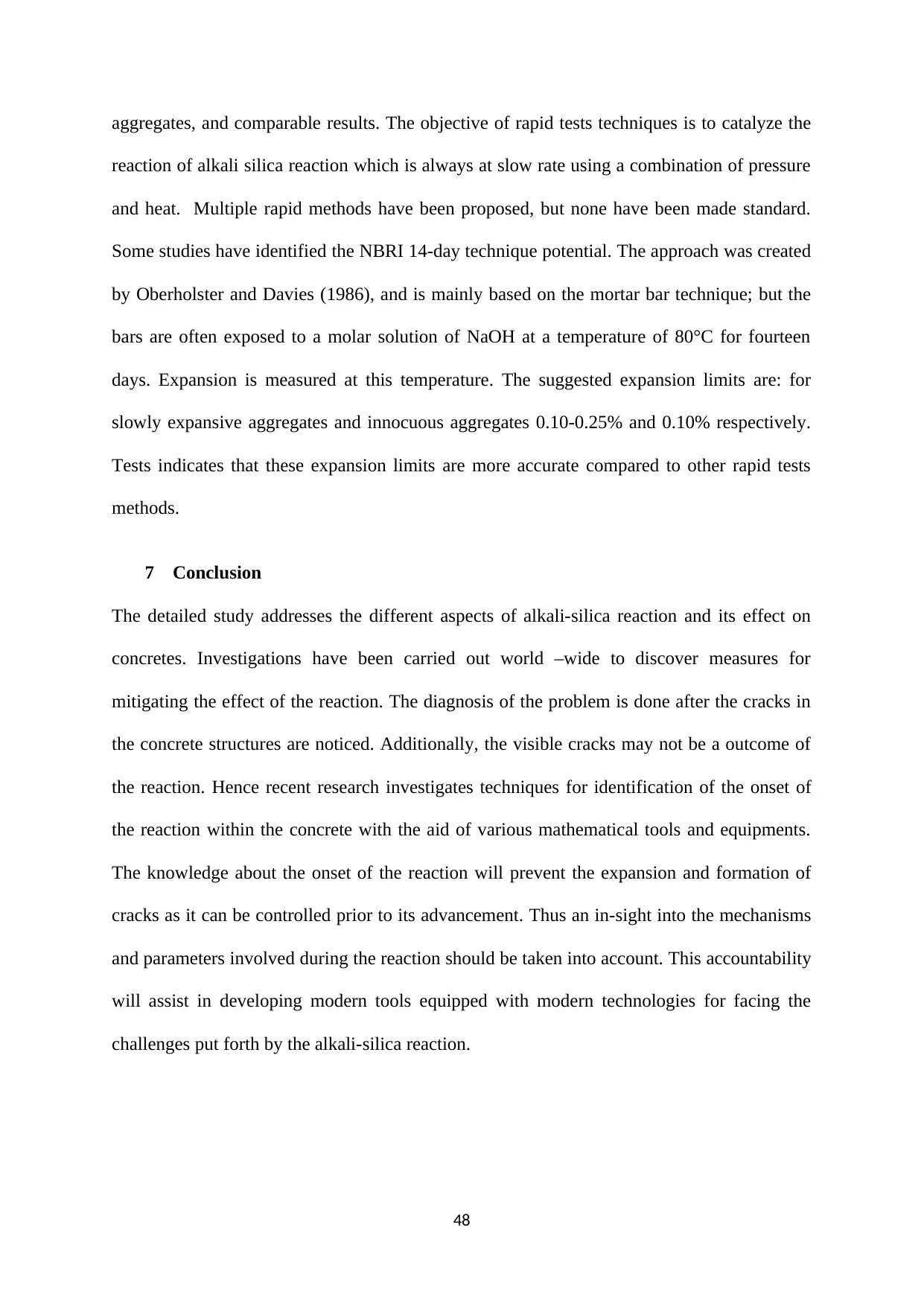
aggregates, and comparable results. The objective of rapid tests techniques is to catalyze the
reaction of alkali silica reaction which is always at slow rate using a combination of pressure
and heat. Multiple rapid methods have been proposed, but none have been made standard.
Some studies have identified the NBRI 14-day technique potential. The approach was created
by Oberholster and Davies (1986), and is mainly based on the mortar bar technique; but the
bars are often exposed to a molar solution of NaOH at a temperature of 80°C for fourteen
days. Expansion is measured at this temperature. The suggested expansion limits are: for
slowly expansive aggregates and innocuous aggregates 0.10-0.25% and 0.10% respectively.
Tests indicates that these expansion limits are more accurate compared to other rapid tests
methods.
7 Conclusion
The detailed study addresses the different aspects of alkali-silica reaction and its effect on
concretes. Investigations have been carried out world –wide to discover measures for
mitigating the effect of the reaction. The diagnosis of the problem is done after the cracks in
the concrete structures are noticed. Additionally, the visible cracks may not be a outcome of
the reaction. Hence recent research investigates techniques for identification of the onset of
the reaction within the concrete with the aid of various mathematical tools and equipments.
The knowledge about the onset of the reaction will prevent the expansion and formation of
cracks as it can be controlled prior to its advancement. Thus an in-sight into the mechanisms
and parameters involved during the reaction should be taken into account. This accountability
will assist in developing modern tools equipped with modern technologies for facing the
challenges put forth by the alkali-silica reaction.
48
reaction of alkali silica reaction which is always at slow rate using a combination of pressure
and heat. Multiple rapid methods have been proposed, but none have been made standard.
Some studies have identified the NBRI 14-day technique potential. The approach was created
by Oberholster and Davies (1986), and is mainly based on the mortar bar technique; but the
bars are often exposed to a molar solution of NaOH at a temperature of 80°C for fourteen
days. Expansion is measured at this temperature. The suggested expansion limits are: for
slowly expansive aggregates and innocuous aggregates 0.10-0.25% and 0.10% respectively.
Tests indicates that these expansion limits are more accurate compared to other rapid tests
methods.
7 Conclusion
The detailed study addresses the different aspects of alkali-silica reaction and its effect on
concretes. Investigations have been carried out world –wide to discover measures for
mitigating the effect of the reaction. The diagnosis of the problem is done after the cracks in
the concrete structures are noticed. Additionally, the visible cracks may not be a outcome of
the reaction. Hence recent research investigates techniques for identification of the onset of
the reaction within the concrete with the aid of various mathematical tools and equipments.
The knowledge about the onset of the reaction will prevent the expansion and formation of
cracks as it can be controlled prior to its advancement. Thus an in-sight into the mechanisms
and parameters involved during the reaction should be taken into account. This accountability
will assist in developing modern tools equipped with modern technologies for facing the
challenges put forth by the alkali-silica reaction.
48
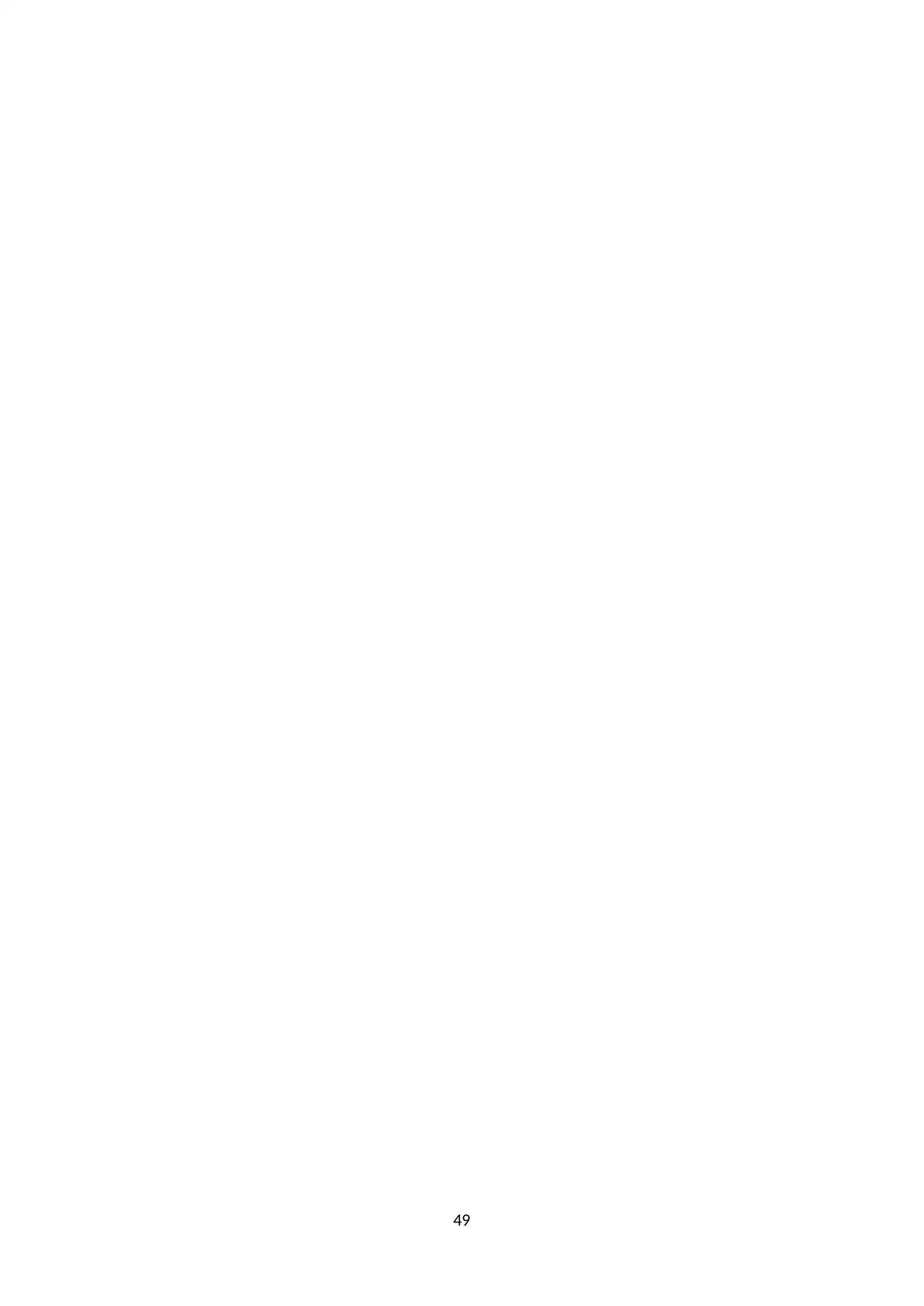
49
Paraphrase This Document
Need a fresh take? Get an instant paraphrase of this document with our AI Paraphraser
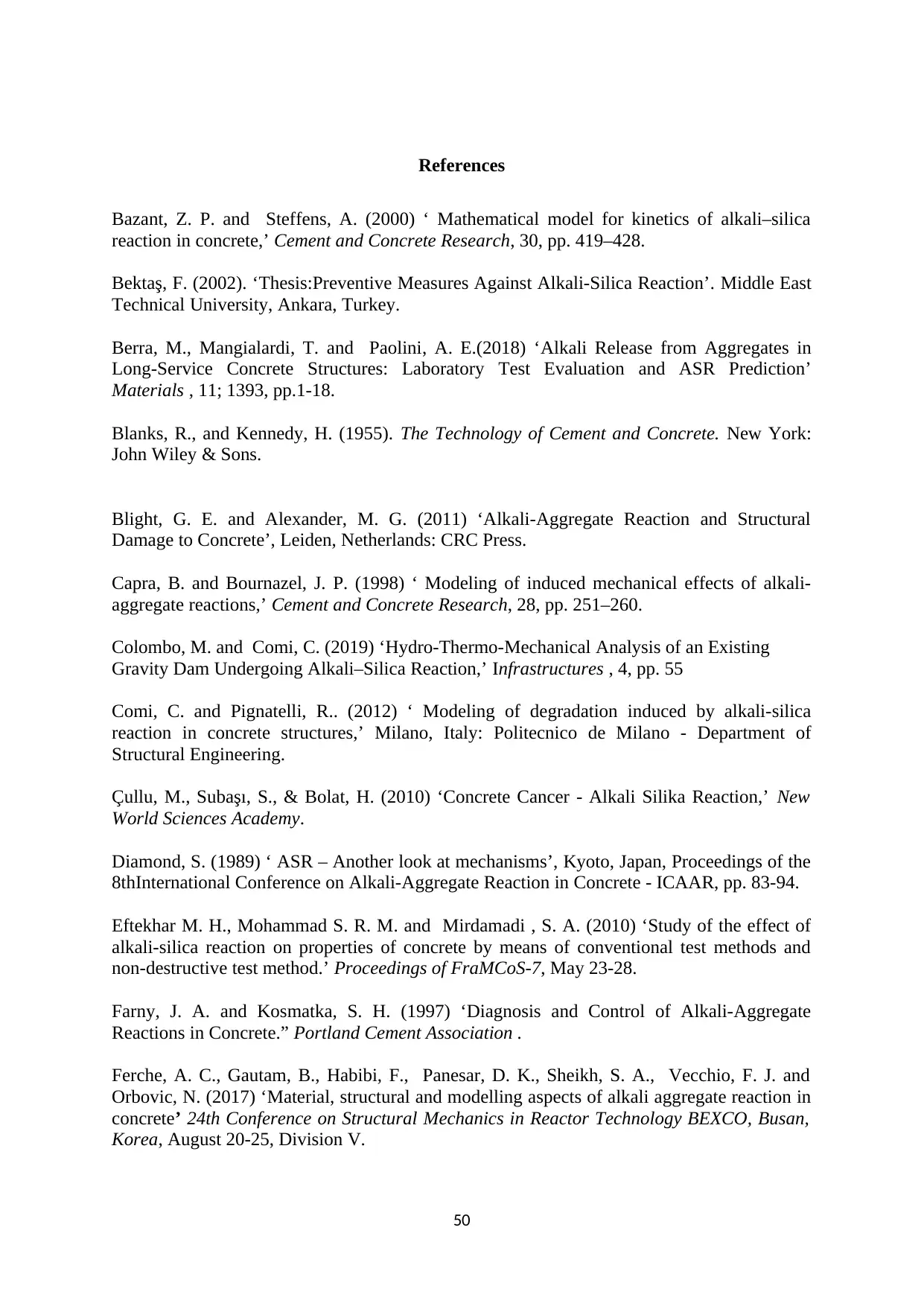
References
Bazant, Z. P. and Steffens, A. (2000) ‘ Mathematical model for kinetics of alkali–silica
reaction in concrete,’ Cement and Concrete Research, 30, pp. 419–428.
Bektaş, F. (2002). ‘Thesis:Preventive Measures Against Alkali-Silica Reaction’. Middle East
Technical University, Ankara, Turkey.
Berra, M., Mangialardi, T. and Paolini, A. E.(2018) ‘Alkali Release from Aggregates in
Long-Service Concrete Structures: Laboratory Test Evaluation and ASR Prediction’
Materials , 11; 1393, pp.1-18.
Blanks, R., and Kennedy, H. (1955). The Technology of Cement and Concrete. New York:
John Wiley & Sons.
Blight, G. E. and Alexander, M. G. (2011) ‘Alkali-Aggregate Reaction and Structural
Damage to Concrete’, Leiden, Netherlands: CRC Press.
Capra, B. and Bournazel, J. P. (1998) ‘ Modeling of induced mechanical effects of alkali-
aggregate reactions,’ Cement and Concrete Research, 28, pp. 251–260.
Colombo, M. and Comi, C. (2019) ‘Hydro-Thermo-Mechanical Analysis of an Existing
Gravity Dam Undergoing Alkali–Silica Reaction,’ Infrastructures , 4, pp. 55
Comi, C. and Pignatelli, R.. (2012) ‘ Modeling of degradation induced by alkali-silica
reaction in concrete structures,’ Milano, Italy: Politecnico de Milano - Department of
Structural Engineering.
Çullu, M., Subaşı, S., & Bolat, H. (2010) ‘Concrete Cancer - Alkali Silika Reaction,’ New
World Sciences Academy.
Diamond, S. (1989) ‘ ASR – Another look at mechanisms’, Kyoto, Japan, Proceedings of the
8thInternational Conference on Alkali-Aggregate Reaction in Concrete - ICAAR, pp. 83-94.
Eftekhar M. H., Mohammad S. R. M. and Mirdamadi , S. A. (2010) ‘Study of the effect of
alkali-silica reaction on properties of concrete by means of conventional test methods and
non-destructive test method.’ Proceedings of FraMCoS-7, May 23-28.
Farny, J. A. and Kosmatka, S. H. (1997) ‘Diagnosis and Control of Alkali-Aggregate
Reactions in Concrete.” Portland Cement Association .
Ferche, A. C., Gautam, B., Habibi, F., Panesar, D. K., Sheikh, S. A., Vecchio, F. J. and
Orbovic, N. (2017) ‘Material, structural and modelling aspects of alkali aggregate reaction in
concrete’ 24th Conference on Structural Mechanics in Reactor Technology BEXCO, Busan,
Korea, August 20-25, Division V.
50
Bazant, Z. P. and Steffens, A. (2000) ‘ Mathematical model for kinetics of alkali–silica
reaction in concrete,’ Cement and Concrete Research, 30, pp. 419–428.
Bektaş, F. (2002). ‘Thesis:Preventive Measures Against Alkali-Silica Reaction’. Middle East
Technical University, Ankara, Turkey.
Berra, M., Mangialardi, T. and Paolini, A. E.(2018) ‘Alkali Release from Aggregates in
Long-Service Concrete Structures: Laboratory Test Evaluation and ASR Prediction’
Materials , 11; 1393, pp.1-18.
Blanks, R., and Kennedy, H. (1955). The Technology of Cement and Concrete. New York:
John Wiley & Sons.
Blight, G. E. and Alexander, M. G. (2011) ‘Alkali-Aggregate Reaction and Structural
Damage to Concrete’, Leiden, Netherlands: CRC Press.
Capra, B. and Bournazel, J. P. (1998) ‘ Modeling of induced mechanical effects of alkali-
aggregate reactions,’ Cement and Concrete Research, 28, pp. 251–260.
Colombo, M. and Comi, C. (2019) ‘Hydro-Thermo-Mechanical Analysis of an Existing
Gravity Dam Undergoing Alkali–Silica Reaction,’ Infrastructures , 4, pp. 55
Comi, C. and Pignatelli, R.. (2012) ‘ Modeling of degradation induced by alkali-silica
reaction in concrete structures,’ Milano, Italy: Politecnico de Milano - Department of
Structural Engineering.
Çullu, M., Subaşı, S., & Bolat, H. (2010) ‘Concrete Cancer - Alkali Silika Reaction,’ New
World Sciences Academy.
Diamond, S. (1989) ‘ ASR – Another look at mechanisms’, Kyoto, Japan, Proceedings of the
8thInternational Conference on Alkali-Aggregate Reaction in Concrete - ICAAR, pp. 83-94.
Eftekhar M. H., Mohammad S. R. M. and Mirdamadi , S. A. (2010) ‘Study of the effect of
alkali-silica reaction on properties of concrete by means of conventional test methods and
non-destructive test method.’ Proceedings of FraMCoS-7, May 23-28.
Farny, J. A. and Kosmatka, S. H. (1997) ‘Diagnosis and Control of Alkali-Aggregate
Reactions in Concrete.” Portland Cement Association .
Ferche, A. C., Gautam, B., Habibi, F., Panesar, D. K., Sheikh, S. A., Vecchio, F. J. and
Orbovic, N. (2017) ‘Material, structural and modelling aspects of alkali aggregate reaction in
concrete’ 24th Conference on Structural Mechanics in Reactor Technology BEXCO, Busan,
Korea, August 20-25, Division V.
50
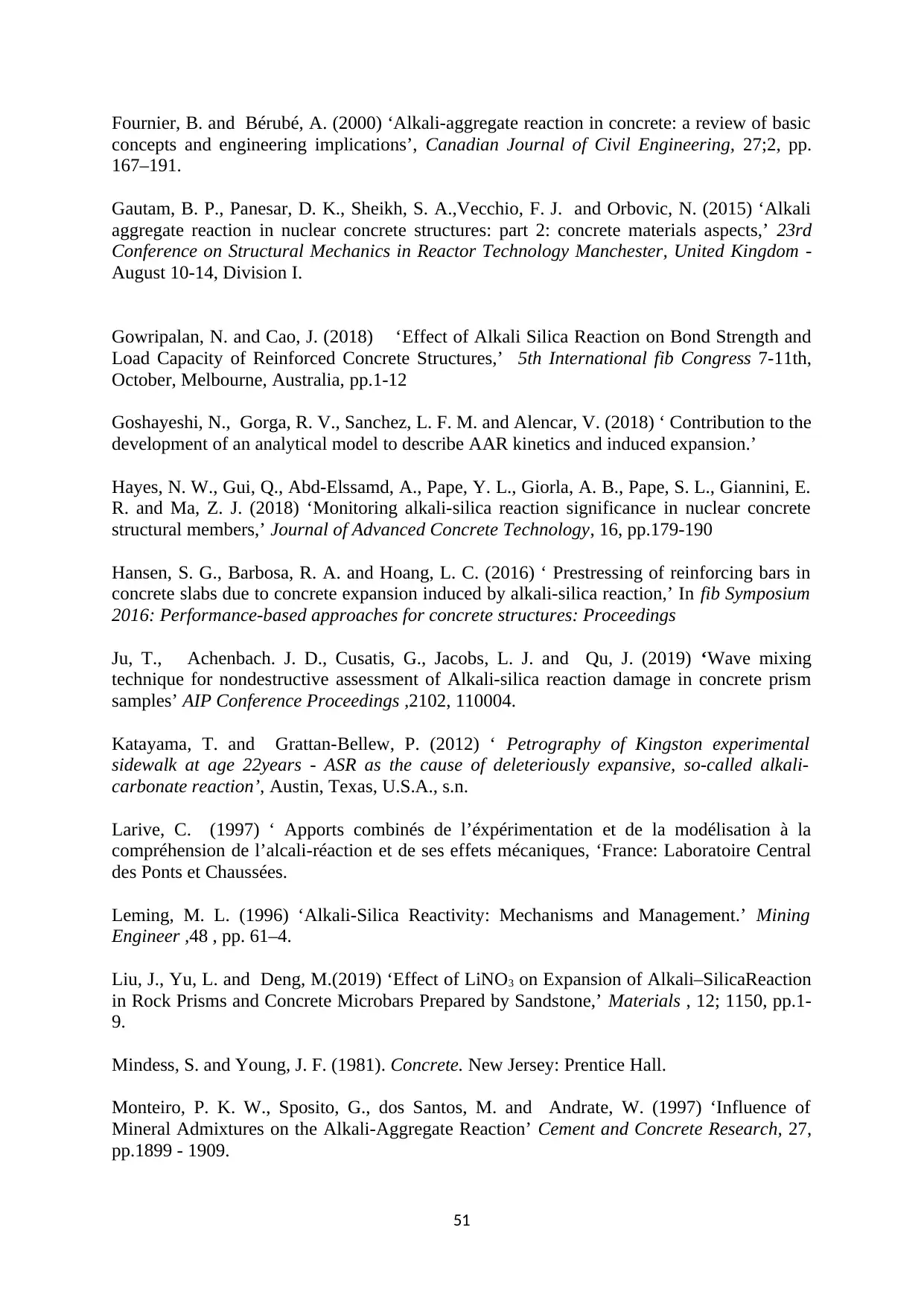
Fournier, B. and Bérubé, A. (2000) ‘Alkali-aggregate reaction in concrete: a review of basic
concepts and engineering implications’, Canadian Journal of Civil Engineering, 27;2, pp.
167–191.
Gautam, B. P., Panesar, D. K., Sheikh, S. A.,Vecchio, F. J. and Orbovic, N. (2015) ‘Alkali
aggregate reaction in nuclear concrete structures: part 2: concrete materials aspects,’ 23rd
Conference on Structural Mechanics in Reactor Technology Manchester, United Kingdom -
August 10-14, Division I.
Gowripalan, N. and Cao, J. (2018) ‘Effect of Alkali Silica Reaction on Bond Strength and
Load Capacity of Reinforced Concrete Structures,’ 5th International fib Congress 7-11th,
October, Melbourne, Australia, pp.1-12
Goshayeshi, N., Gorga, R. V., Sanchez, L. F. M. and Alencar, V. (2018) ‘ Contribution to the
development of an analytical model to describe AAR kinetics and induced expansion.’
Hayes, N. W., Gui, Q., Abd-Elssamd, A., Pape, Y. L., Giorla, A. B., Pape, S. L., Giannini, E.
R. and Ma, Z. J. (2018) ‘Monitoring alkali-silica reaction significance in nuclear concrete
structural members,’ Journal of Advanced Concrete Technology, 16, pp.179-190
Hansen, S. G., Barbosa, R. A. and Hoang, L. C. (2016) ‘ Prestressing of reinforcing bars in
concrete slabs due to concrete expansion induced by alkali-silica reaction,’ In fib Symposium
2016: Performance-based approaches for concrete structures: Proceedings
Ju, T., Achenbach. J. D., Cusatis, G., Jacobs, L. J. and Qu, J. (2019) ‘Wave mixing
technique for nondestructive assessment of Alkali-silica reaction damage in concrete prism
samples’ AIP Conference Proceedings ,2102, 110004.
Katayama, T. and Grattan-Bellew, P. (2012) ‘ Petrography of Kingston experimental
sidewalk at age 22years - ASR as the cause of deleteriously expansive, so-called alkali-
carbonate reaction’, Austin, Texas, U.S.A., s.n.
Larive, C. (1997) ‘ Apports combinés de l’éxpérimentation et de la modélisation à la
compréhension de l’alcali-réaction et de ses effets mécaniques, ‘France: Laboratoire Central
des Ponts et Chaussées.
Leming, M. L. (1996) ‘Alkali-Silica Reactivity: Mechanisms and Management.’ Mining
Engineer ,48 , pp. 61–4.
Liu, J., Yu, L. and Deng, M.(2019) ‘Effect of LiNO3 on Expansion of Alkali–SilicaReaction
in Rock Prisms and Concrete Microbars Prepared by Sandstone,’ Materials , 12; 1150, pp.1-
9.
Mindess, S. and Young, J. F. (1981). Concrete. New Jersey: Prentice Hall.
Monteiro, P. K. W., Sposito, G., dos Santos, M. and Andrate, W. (1997) ‘Influence of
Mineral Admixtures on the Alkali-Aggregate Reaction’ Cement and Concrete Research, 27,
pp.1899 - 1909.
51
concepts and engineering implications’, Canadian Journal of Civil Engineering, 27;2, pp.
167–191.
Gautam, B. P., Panesar, D. K., Sheikh, S. A.,Vecchio, F. J. and Orbovic, N. (2015) ‘Alkali
aggregate reaction in nuclear concrete structures: part 2: concrete materials aspects,’ 23rd
Conference on Structural Mechanics in Reactor Technology Manchester, United Kingdom -
August 10-14, Division I.
Gowripalan, N. and Cao, J. (2018) ‘Effect of Alkali Silica Reaction on Bond Strength and
Load Capacity of Reinforced Concrete Structures,’ 5th International fib Congress 7-11th,
October, Melbourne, Australia, pp.1-12
Goshayeshi, N., Gorga, R. V., Sanchez, L. F. M. and Alencar, V. (2018) ‘ Contribution to the
development of an analytical model to describe AAR kinetics and induced expansion.’
Hayes, N. W., Gui, Q., Abd-Elssamd, A., Pape, Y. L., Giorla, A. B., Pape, S. L., Giannini, E.
R. and Ma, Z. J. (2018) ‘Monitoring alkali-silica reaction significance in nuclear concrete
structural members,’ Journal of Advanced Concrete Technology, 16, pp.179-190
Hansen, S. G., Barbosa, R. A. and Hoang, L. C. (2016) ‘ Prestressing of reinforcing bars in
concrete slabs due to concrete expansion induced by alkali-silica reaction,’ In fib Symposium
2016: Performance-based approaches for concrete structures: Proceedings
Ju, T., Achenbach. J. D., Cusatis, G., Jacobs, L. J. and Qu, J. (2019) ‘Wave mixing
technique for nondestructive assessment of Alkali-silica reaction damage in concrete prism
samples’ AIP Conference Proceedings ,2102, 110004.
Katayama, T. and Grattan-Bellew, P. (2012) ‘ Petrography of Kingston experimental
sidewalk at age 22years - ASR as the cause of deleteriously expansive, so-called alkali-
carbonate reaction’, Austin, Texas, U.S.A., s.n.
Larive, C. (1997) ‘ Apports combinés de l’éxpérimentation et de la modélisation à la
compréhension de l’alcali-réaction et de ses effets mécaniques, ‘France: Laboratoire Central
des Ponts et Chaussées.
Leming, M. L. (1996) ‘Alkali-Silica Reactivity: Mechanisms and Management.’ Mining
Engineer ,48 , pp. 61–4.
Liu, J., Yu, L. and Deng, M.(2019) ‘Effect of LiNO3 on Expansion of Alkali–SilicaReaction
in Rock Prisms and Concrete Microbars Prepared by Sandstone,’ Materials , 12; 1150, pp.1-
9.
Mindess, S. and Young, J. F. (1981). Concrete. New Jersey: Prentice Hall.
Monteiro, P. K. W., Sposito, G., dos Santos, M. and Andrate, W. (1997) ‘Influence of
Mineral Admixtures on the Alkali-Aggregate Reaction’ Cement and Concrete Research, 27,
pp.1899 - 1909.
51
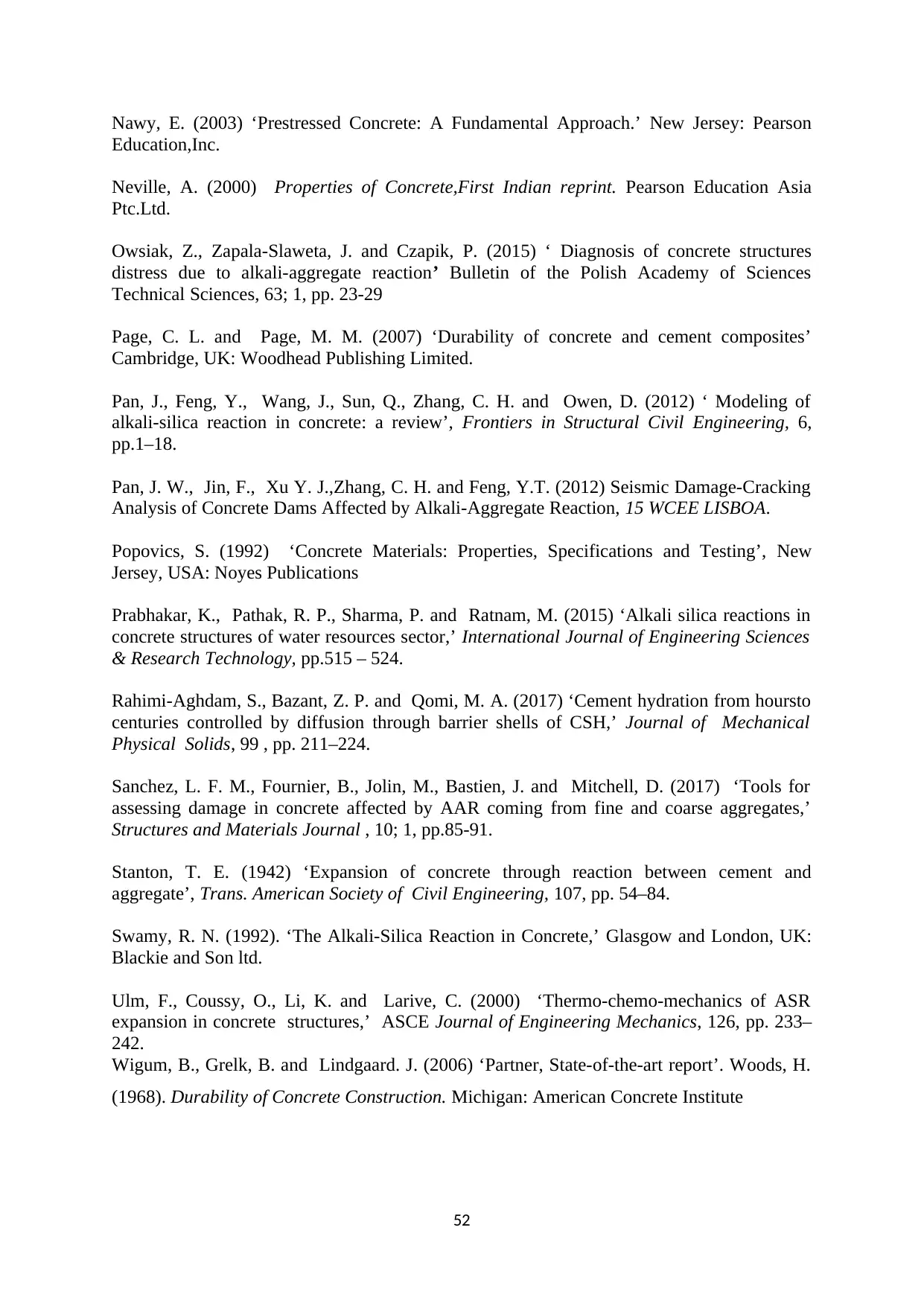
Nawy, E. (2003) ‘Prestressed Concrete: A Fundamental Approach.’ New Jersey: Pearson
Education,Inc.
Neville, A. (2000) Properties of Concrete,First Indian reprint. Pearson Education Asia
Ptc.Ltd.
Owsiak, Z., Zapala-Slaweta, J. and Czapik, P. (2015) ‘ Diagnosis of concrete structures
distress due to alkali-aggregate reaction’ Bulletin of the Polish Academy of Sciences
Technical Sciences, 63; 1, pp. 23-29
Page, C. L. and Page, M. M. (2007) ‘Durability of concrete and cement composites’
Cambridge, UK: Woodhead Publishing Limited.
Pan, J., Feng, Y., Wang, J., Sun, Q., Zhang, C. H. and Owen, D. (2012) ‘ Modeling of
alkali-silica reaction in concrete: a review’, Frontiers in Structural Civil Engineering, 6,
pp.1–18.
Pan, J. W., Jin, F., Xu Y. J.,Zhang, C. H. and Feng, Y.T. (2012) Seismic Damage-Cracking
Analysis of Concrete Dams Affected by Alkali-Aggregate Reaction, 15 WCEE LISBOA.
Popovics, S. (1992) ‘Concrete Materials: Properties, Specifications and Testing’, New
Jersey, USA: Noyes Publications
Prabhakar, K., Pathak, R. P., Sharma, P. and Ratnam, M. (2015) ‘Alkali silica reactions in
concrete structures of water resources sector,’ International Journal of Engineering Sciences
& Research Technology, pp.515 – 524.
Rahimi-Aghdam, S., Bazant, Z. P. and Qomi, M. A. (2017) ‘Cement hydration from hoursto
centuries controlled by diffusion through barrier shells of CSH,’ Journal of Mechanical
Physical Solids, 99 , pp. 211–224.
Sanchez, L. F. M., Fournier, B., Jolin, M., Bastien, J. and Mitchell, D. (2017) ‘Tools for
assessing damage in concrete affected by AAR coming from fine and coarse aggregates,’
Structures and Materials Journal , 10; 1, pp.85-91.
Stanton, T. E. (1942) ‘Expansion of concrete through reaction between cement and
aggregate’, Trans. American Society of Civil Engineering, 107, pp. 54–84.
Swamy, R. N. (1992). ‘The Alkali-Silica Reaction in Concrete,’ Glasgow and London, UK:
Blackie and Son ltd.
Ulm, F., Coussy, O., Li, K. and Larive, C. (2000) ‘Thermo-chemo-mechanics of ASR
expansion in concrete structures,’ ASCE Journal of Engineering Mechanics, 126, pp. 233–
242.
Wigum, B., Grelk, B. and Lindgaard. J. (2006) ‘Partner, State-of-the-art report’. Woods, H.
(1968). Durability of Concrete Construction. Michigan: American Concrete Institute
52
Education,Inc.
Neville, A. (2000) Properties of Concrete,First Indian reprint. Pearson Education Asia
Ptc.Ltd.
Owsiak, Z., Zapala-Slaweta, J. and Czapik, P. (2015) ‘ Diagnosis of concrete structures
distress due to alkali-aggregate reaction’ Bulletin of the Polish Academy of Sciences
Technical Sciences, 63; 1, pp. 23-29
Page, C. L. and Page, M. M. (2007) ‘Durability of concrete and cement composites’
Cambridge, UK: Woodhead Publishing Limited.
Pan, J., Feng, Y., Wang, J., Sun, Q., Zhang, C. H. and Owen, D. (2012) ‘ Modeling of
alkali-silica reaction in concrete: a review’, Frontiers in Structural Civil Engineering, 6,
pp.1–18.
Pan, J. W., Jin, F., Xu Y. J.,Zhang, C. H. and Feng, Y.T. (2012) Seismic Damage-Cracking
Analysis of Concrete Dams Affected by Alkali-Aggregate Reaction, 15 WCEE LISBOA.
Popovics, S. (1992) ‘Concrete Materials: Properties, Specifications and Testing’, New
Jersey, USA: Noyes Publications
Prabhakar, K., Pathak, R. P., Sharma, P. and Ratnam, M. (2015) ‘Alkali silica reactions in
concrete structures of water resources sector,’ International Journal of Engineering Sciences
& Research Technology, pp.515 – 524.
Rahimi-Aghdam, S., Bazant, Z. P. and Qomi, M. A. (2017) ‘Cement hydration from hoursto
centuries controlled by diffusion through barrier shells of CSH,’ Journal of Mechanical
Physical Solids, 99 , pp. 211–224.
Sanchez, L. F. M., Fournier, B., Jolin, M., Bastien, J. and Mitchell, D. (2017) ‘Tools for
assessing damage in concrete affected by AAR coming from fine and coarse aggregates,’
Structures and Materials Journal , 10; 1, pp.85-91.
Stanton, T. E. (1942) ‘Expansion of concrete through reaction between cement and
aggregate’, Trans. American Society of Civil Engineering, 107, pp. 54–84.
Swamy, R. N. (1992). ‘The Alkali-Silica Reaction in Concrete,’ Glasgow and London, UK:
Blackie and Son ltd.
Ulm, F., Coussy, O., Li, K. and Larive, C. (2000) ‘Thermo-chemo-mechanics of ASR
expansion in concrete structures,’ ASCE Journal of Engineering Mechanics, 126, pp. 233–
242.
Wigum, B., Grelk, B. and Lindgaard. J. (2006) ‘Partner, State-of-the-art report’. Woods, H.
(1968). Durability of Concrete Construction. Michigan: American Concrete Institute
52
Secure Best Marks with AI Grader
Need help grading? Try our AI Grader for instant feedback on your assignments.
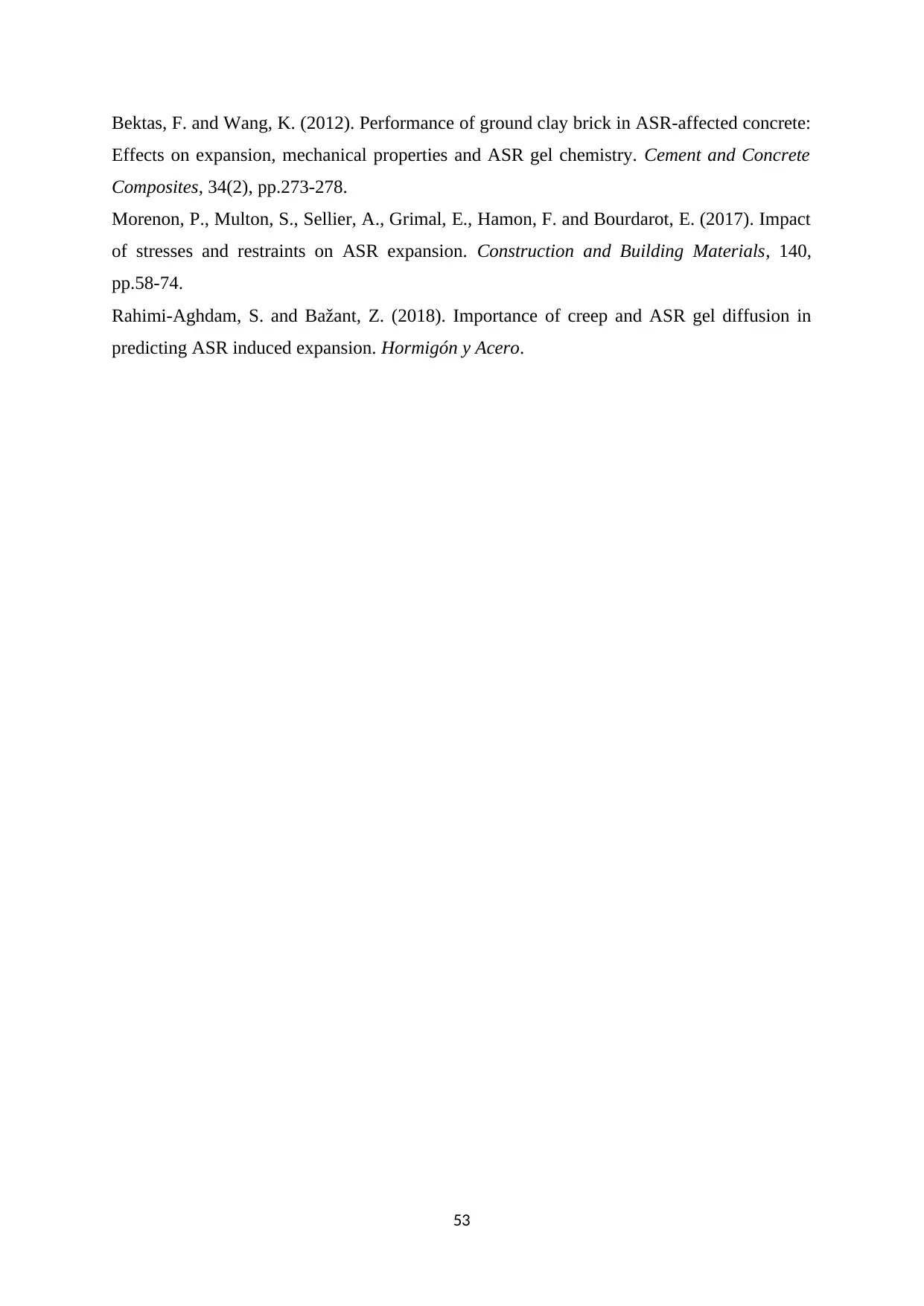
Bektas, F. and Wang, K. (2012). Performance of ground clay brick in ASR-affected concrete:
Effects on expansion, mechanical properties and ASR gel chemistry. Cement and Concrete
Composites, 34(2), pp.273-278.
Morenon, P., Multon, S., Sellier, A., Grimal, E., Hamon, F. and Bourdarot, E. (2017). Impact
of stresses and restraints on ASR expansion. Construction and Building Materials, 140,
pp.58-74.
Rahimi-Aghdam, S. and Bažant, Z. (2018). Importance of creep and ASR gel diffusion in
predicting ASR induced expansion. Hormigón y Acero.
53
Effects on expansion, mechanical properties and ASR gel chemistry. Cement and Concrete
Composites, 34(2), pp.273-278.
Morenon, P., Multon, S., Sellier, A., Grimal, E., Hamon, F. and Bourdarot, E. (2017). Impact
of stresses and restraints on ASR expansion. Construction and Building Materials, 140,
pp.58-74.
Rahimi-Aghdam, S. and Bažant, Z. (2018). Importance of creep and ASR gel diffusion in
predicting ASR induced expansion. Hormigón y Acero.
53
1 out of 53
![[object Object]](/_next/image/?url=%2F_next%2Fstatic%2Fmedia%2Flogo.6d15ce61.png&w=640&q=75)
Your All-in-One AI-Powered Toolkit for Academic Success.
+13062052269
info@desklib.com
Available 24*7 on WhatsApp / Email
Unlock your academic potential
© 2024 | Zucol Services PVT LTD | All rights reserved.