Targeting Cancer Stem Cells and Peritumoral Stromal Response in Liver Cancer with Nanoparticles and Hyaluronidase
VerifiedAdded on 2023/06/14
|16
|8005
|405
AI Summary
This proposal aims to address the problems of pancreatic and hepatocellular carcinoma by targeting cancer stem cells and peritumoral stromal response. The use of targeted nanoparticles combined with another approach to eradicate the peritumoral stroma will be used to enhance exposure to the nanoparticle. The surface of the nanoparticles will include monoclonal antibodies to EpCAM to address them to the cancer stem cells and tomato red fluorescent protein to evaluate the effectiveness of targeting using the IVIS animal imaging system. The payload of the particles will include metformin and the smoothened inhibitor, vismodegib, both of which will target the CSCs. Pegylated hyaluronidase, PEGPH20 will be used to destroy and prevent regrowth of the stroma.
Contribute Materials
Your contribution can guide someone’s learning journey. Share your
documents today.
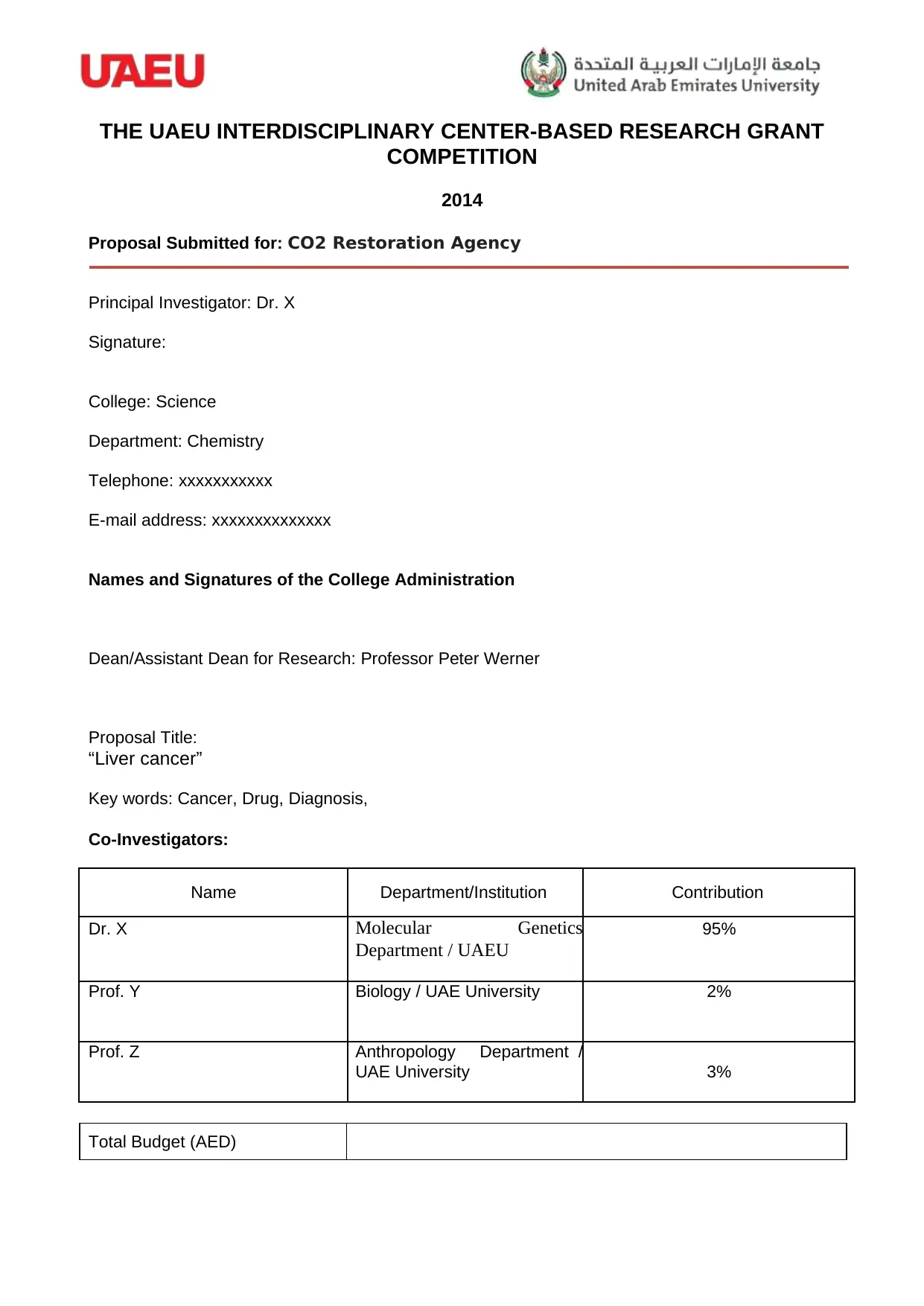
THE UAEU INTERDISCIPLINARY CENTER-BASED RESEARCH GRANT
COMPETITION
2014
Proposal Submitted for: CO2 Restoration Agency
Principal Investigator: Dr. X
Signature:
College: Science
Department: Chemistry
Telephone: xxxxxxxxxxx
E-mail address: xxxxxxxxxxxxxx
Names and Signatures of the College Administration
Dean/Assistant Dean for Research: Professor Peter Werner
Proposal Title:
“Liver cancer”
Key words: Cancer, Drug, Diagnosis,
Co-Investigators:
Name Department/Institution Contribution
Dr. X Molecular Genetics 95%
Department / UAEU
Prof. Y Biology / UAE University 2%
Prof. Z Anthropology Department /
UAE University 3%
Total Budget (AED)
COMPETITION
2014
Proposal Submitted for: CO2 Restoration Agency
Principal Investigator: Dr. X
Signature:
College: Science
Department: Chemistry
Telephone: xxxxxxxxxxx
E-mail address: xxxxxxxxxxxxxx
Names and Signatures of the College Administration
Dean/Assistant Dean for Research: Professor Peter Werner
Proposal Title:
“Liver cancer”
Key words: Cancer, Drug, Diagnosis,
Co-Investigators:
Name Department/Institution Contribution
Dr. X Molecular Genetics 95%
Department / UAEU
Prof. Y Biology / UAE University 2%
Prof. Z Anthropology Department /
UAE University 3%
Total Budget (AED)
Secure Best Marks with AI Grader
Need help grading? Try our AI Grader for instant feedback on your assignments.
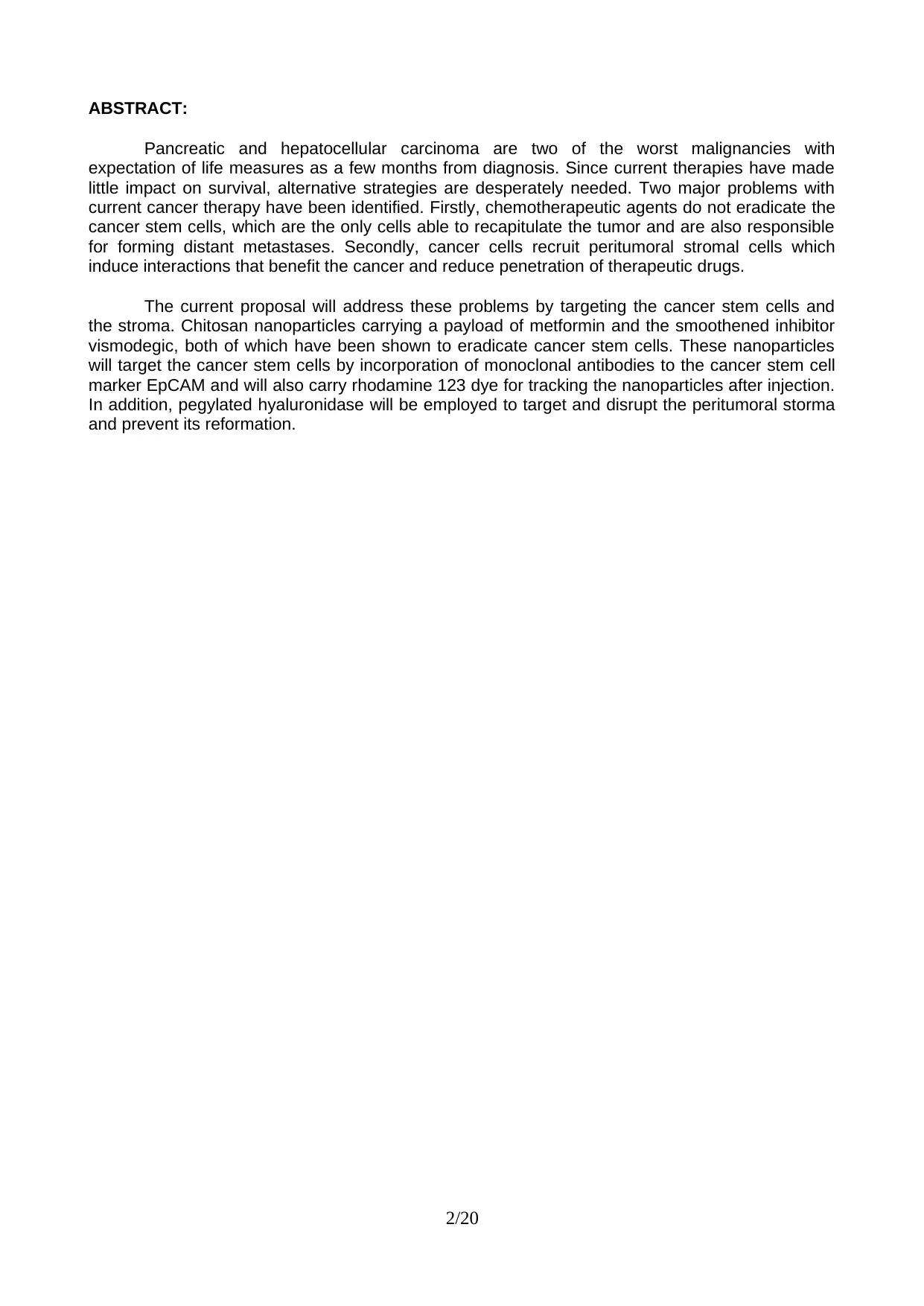
ABSTRACT:
Pancreatic and hepatocellular carcinoma are two of the worst malignancies with
expectation of life measures as a few months from diagnosis. Since current therapies have made
little impact on survival, alternative strategies are desperately needed. Two major problems with
current cancer therapy have been identified. Firstly, chemotherapeutic agents do not eradicate the
cancer stem cells, which are the only cells able to recapitulate the tumor and are also responsible
for forming distant metastases. Secondly, cancer cells recruit peritumoral stromal cells which
induce interactions that benefit the cancer and reduce penetration of therapeutic drugs.
The current proposal will address these problems by targeting the cancer stem cells and
the stroma. Chitosan nanoparticles carrying a payload of metformin and the smoothened inhibitor
vismodegic, both of which have been shown to eradicate cancer stem cells. These nanoparticles
will target the cancer stem cells by incorporation of monoclonal antibodies to the cancer stem cell
marker EpCAM and will also carry rhodamine 123 dye for tracking the nanoparticles after injection.
In addition, pegylated hyaluronidase will be employed to target and disrupt the peritumoral storma
and prevent its reformation.
2/20
Pancreatic and hepatocellular carcinoma are two of the worst malignancies with
expectation of life measures as a few months from diagnosis. Since current therapies have made
little impact on survival, alternative strategies are desperately needed. Two major problems with
current cancer therapy have been identified. Firstly, chemotherapeutic agents do not eradicate the
cancer stem cells, which are the only cells able to recapitulate the tumor and are also responsible
for forming distant metastases. Secondly, cancer cells recruit peritumoral stromal cells which
induce interactions that benefit the cancer and reduce penetration of therapeutic drugs.
The current proposal will address these problems by targeting the cancer stem cells and
the stroma. Chitosan nanoparticles carrying a payload of metformin and the smoothened inhibitor
vismodegic, both of which have been shown to eradicate cancer stem cells. These nanoparticles
will target the cancer stem cells by incorporation of monoclonal antibodies to the cancer stem cell
marker EpCAM and will also carry rhodamine 123 dye for tracking the nanoparticles after injection.
In addition, pegylated hyaluronidase will be employed to target and disrupt the peritumoral storma
and prevent its reformation.
2/20
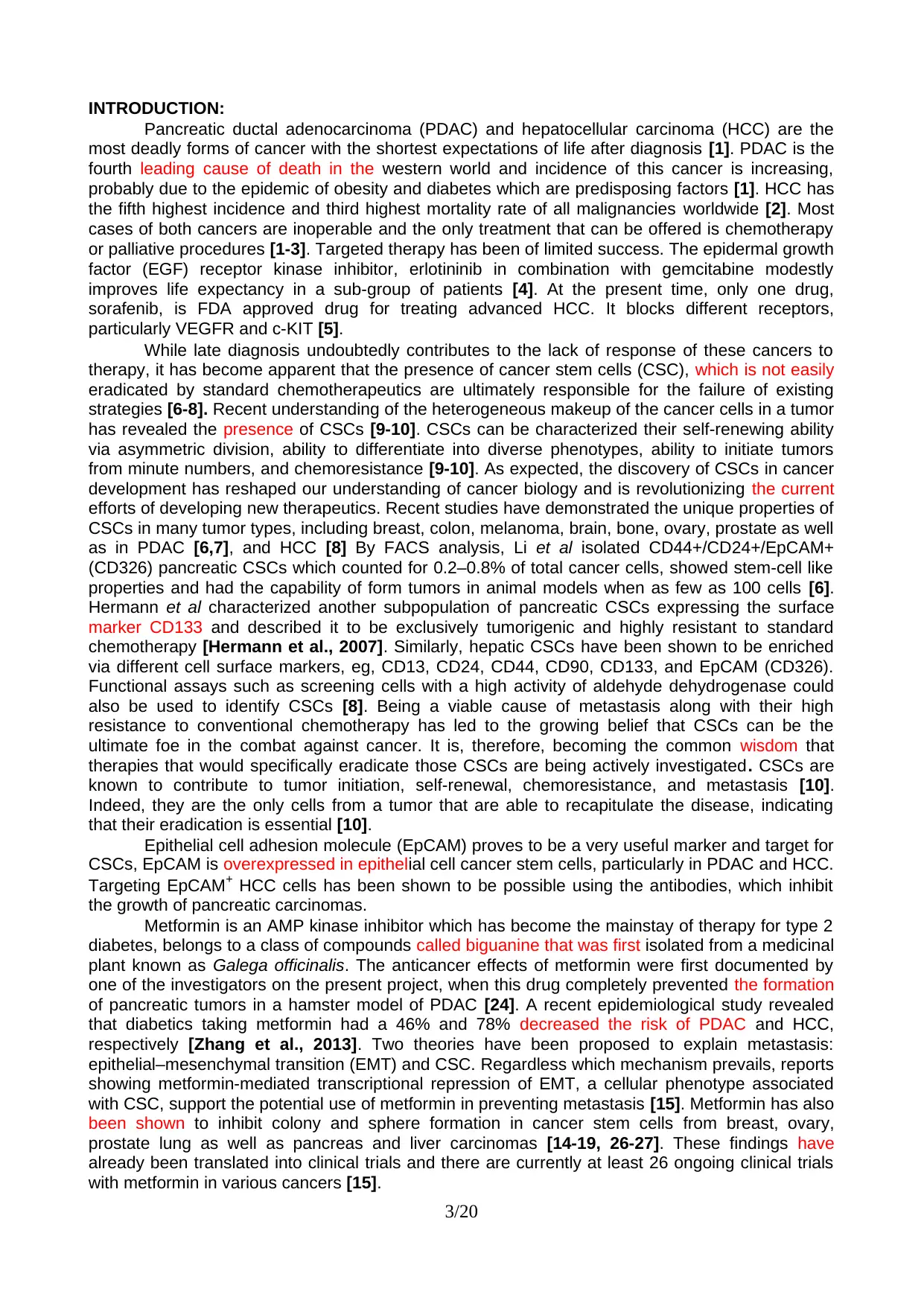
INTRODUCTION:
Pancreatic ductal adenocarcinoma (PDAC) and hepatocellular carcinoma (HCC) are the
most deadly forms of cancer with the shortest expectations of life after diagnosis [1]. PDAC is the
fourth leading cause of death in the western world and incidence of this cancer is increasing,
probably due to the epidemic of obesity and diabetes which are predisposing factors [1]. HCC has
the fifth highest incidence and third highest mortality rate of all malignancies worldwide [2]. Most
cases of both cancers are inoperable and the only treatment that can be offered is chemotherapy
or palliative procedures [1-3]. Targeted therapy has been of limited success. The epidermal growth
factor (EGF) receptor kinase inhibitor, erlotininib in combination with gemcitabine modestly
improves life expectancy in a sub-group of patients [4]. At the present time, only one drug,
sorafenib, is FDA approved drug for treating advanced HCC. It blocks different receptors,
particularly VEGFR and c-KIT [5].
While late diagnosis undoubtedly contributes to the lack of response of these cancers to
therapy, it has become apparent that the presence of cancer stem cells (CSC), which is not easily
eradicated by standard chemotherapeutics are ultimately responsible for the failure of existing
strategies [6-8]. Recent understanding of the heterogeneous makeup of the cancer cells in a tumor
has revealed the presence of CSCs [9-10]. CSCs can be characterized their self-renewing ability
via asymmetric division, ability to differentiate into diverse phenotypes, ability to initiate tumors
from minute numbers, and chemoresistance [9-10]. As expected, the discovery of CSCs in cancer
development has reshaped our understanding of cancer biology and is revolutionizing the current
efforts of developing new therapeutics. Recent studies have demonstrated the unique properties of
CSCs in many tumor types, including breast, colon, melanoma, brain, bone, ovary, prostate as well
as in PDAC [6,7], and HCC [8] By FACS analysis, Li et al isolated CD44+/CD24+/EpCAM+
(CD326) pancreatic CSCs which counted for 0.2–0.8% of total cancer cells, showed stem-cell like
properties and had the capability of form tumors in animal models when as few as 100 cells [6].
Hermann et al characterized another subpopulation of pancreatic CSCs expressing the surface
marker CD133 and described it to be exclusively tumorigenic and highly resistant to standard
chemotherapy [Hermann et al., 2007]. Similarly, hepatic CSCs have been shown to be enriched
via different cell surface markers, eg, CD13, CD24, CD44, CD90, CD133, and EpCAM (CD326).
Functional assays such as screening cells with a high activity of aldehyde dehydrogenase could
also be used to identify CSCs [8]. Being a viable cause of metastasis along with their high
resistance to conventional chemotherapy has led to the growing belief that CSCs can be the
ultimate foe in the combat against cancer. It is, therefore, becoming the common wisdom that
therapies that would specifically eradicate those CSCs are being actively investigated. CSCs are
known to contribute to tumor initiation, self-renewal, chemoresistance, and metastasis [10].
Indeed, they are the only cells from a tumor that are able to recapitulate the disease, indicating
that their eradication is essential [10].
Epithelial cell adhesion molecule (EpCAM) proves to be a very useful marker and target for
CSCs, EpCAM is overexpressed in epithelial cell cancer stem cells, particularly in PDAC and HCC.
Targeting EpCAM+ HCC cells has been shown to be possible using the antibodies, which inhibit
the growth of pancreatic carcinomas.
Metformin is an AMP kinase inhibitor which has become the mainstay of therapy for type 2
diabetes, belongs to a class of compounds called biguanine that was first isolated from a medicinal
plant known as Galega officinalis. The anticancer effects of metformin were first documented by
one of the investigators on the present project, when this drug completely prevented the formation
of pancreatic tumors in a hamster model of PDAC [24]. A recent epidemiological study revealed
that diabetics taking metformin had a 46% and 78% decreased the risk of PDAC and HCC,
respectively [Zhang et al., 2013]. Two theories have been proposed to explain metastasis:
epithelial–mesenchymal transition (EMT) and CSC. Regardless which mechanism prevails, reports
showing metformin-mediated transcriptional repression of EMT, a cellular phenotype associated
with CSC, support the potential use of metformin in preventing metastasis [15]. Metformin has also
been shown to inhibit colony and sphere formation in cancer stem cells from breast, ovary,
prostate lung as well as pancreas and liver carcinomas [14-19, 26-27]. These findings have
already been translated into clinical trials and there are currently at least 26 ongoing clinical trials
with metformin in various cancers [15].
3/20
Pancreatic ductal adenocarcinoma (PDAC) and hepatocellular carcinoma (HCC) are the
most deadly forms of cancer with the shortest expectations of life after diagnosis [1]. PDAC is the
fourth leading cause of death in the western world and incidence of this cancer is increasing,
probably due to the epidemic of obesity and diabetes which are predisposing factors [1]. HCC has
the fifth highest incidence and third highest mortality rate of all malignancies worldwide [2]. Most
cases of both cancers are inoperable and the only treatment that can be offered is chemotherapy
or palliative procedures [1-3]. Targeted therapy has been of limited success. The epidermal growth
factor (EGF) receptor kinase inhibitor, erlotininib in combination with gemcitabine modestly
improves life expectancy in a sub-group of patients [4]. At the present time, only one drug,
sorafenib, is FDA approved drug for treating advanced HCC. It blocks different receptors,
particularly VEGFR and c-KIT [5].
While late diagnosis undoubtedly contributes to the lack of response of these cancers to
therapy, it has become apparent that the presence of cancer stem cells (CSC), which is not easily
eradicated by standard chemotherapeutics are ultimately responsible for the failure of existing
strategies [6-8]. Recent understanding of the heterogeneous makeup of the cancer cells in a tumor
has revealed the presence of CSCs [9-10]. CSCs can be characterized their self-renewing ability
via asymmetric division, ability to differentiate into diverse phenotypes, ability to initiate tumors
from minute numbers, and chemoresistance [9-10]. As expected, the discovery of CSCs in cancer
development has reshaped our understanding of cancer biology and is revolutionizing the current
efforts of developing new therapeutics. Recent studies have demonstrated the unique properties of
CSCs in many tumor types, including breast, colon, melanoma, brain, bone, ovary, prostate as well
as in PDAC [6,7], and HCC [8] By FACS analysis, Li et al isolated CD44+/CD24+/EpCAM+
(CD326) pancreatic CSCs which counted for 0.2–0.8% of total cancer cells, showed stem-cell like
properties and had the capability of form tumors in animal models when as few as 100 cells [6].
Hermann et al characterized another subpopulation of pancreatic CSCs expressing the surface
marker CD133 and described it to be exclusively tumorigenic and highly resistant to standard
chemotherapy [Hermann et al., 2007]. Similarly, hepatic CSCs have been shown to be enriched
via different cell surface markers, eg, CD13, CD24, CD44, CD90, CD133, and EpCAM (CD326).
Functional assays such as screening cells with a high activity of aldehyde dehydrogenase could
also be used to identify CSCs [8]. Being a viable cause of metastasis along with their high
resistance to conventional chemotherapy has led to the growing belief that CSCs can be the
ultimate foe in the combat against cancer. It is, therefore, becoming the common wisdom that
therapies that would specifically eradicate those CSCs are being actively investigated. CSCs are
known to contribute to tumor initiation, self-renewal, chemoresistance, and metastasis [10].
Indeed, they are the only cells from a tumor that are able to recapitulate the disease, indicating
that their eradication is essential [10].
Epithelial cell adhesion molecule (EpCAM) proves to be a very useful marker and target for
CSCs, EpCAM is overexpressed in epithelial cell cancer stem cells, particularly in PDAC and HCC.
Targeting EpCAM+ HCC cells has been shown to be possible using the antibodies, which inhibit
the growth of pancreatic carcinomas.
Metformin is an AMP kinase inhibitor which has become the mainstay of therapy for type 2
diabetes, belongs to a class of compounds called biguanine that was first isolated from a medicinal
plant known as Galega officinalis. The anticancer effects of metformin were first documented by
one of the investigators on the present project, when this drug completely prevented the formation
of pancreatic tumors in a hamster model of PDAC [24]. A recent epidemiological study revealed
that diabetics taking metformin had a 46% and 78% decreased the risk of PDAC and HCC,
respectively [Zhang et al., 2013]. Two theories have been proposed to explain metastasis:
epithelial–mesenchymal transition (EMT) and CSC. Regardless which mechanism prevails, reports
showing metformin-mediated transcriptional repression of EMT, a cellular phenotype associated
with CSC, support the potential use of metformin in preventing metastasis [15]. Metformin has also
been shown to inhibit colony and sphere formation in cancer stem cells from breast, ovary,
prostate lung as well as pancreas and liver carcinomas [14-19, 26-27]. These findings have
already been translated into clinical trials and there are currently at least 26 ongoing clinical trials
with metformin in various cancers [15].
3/20
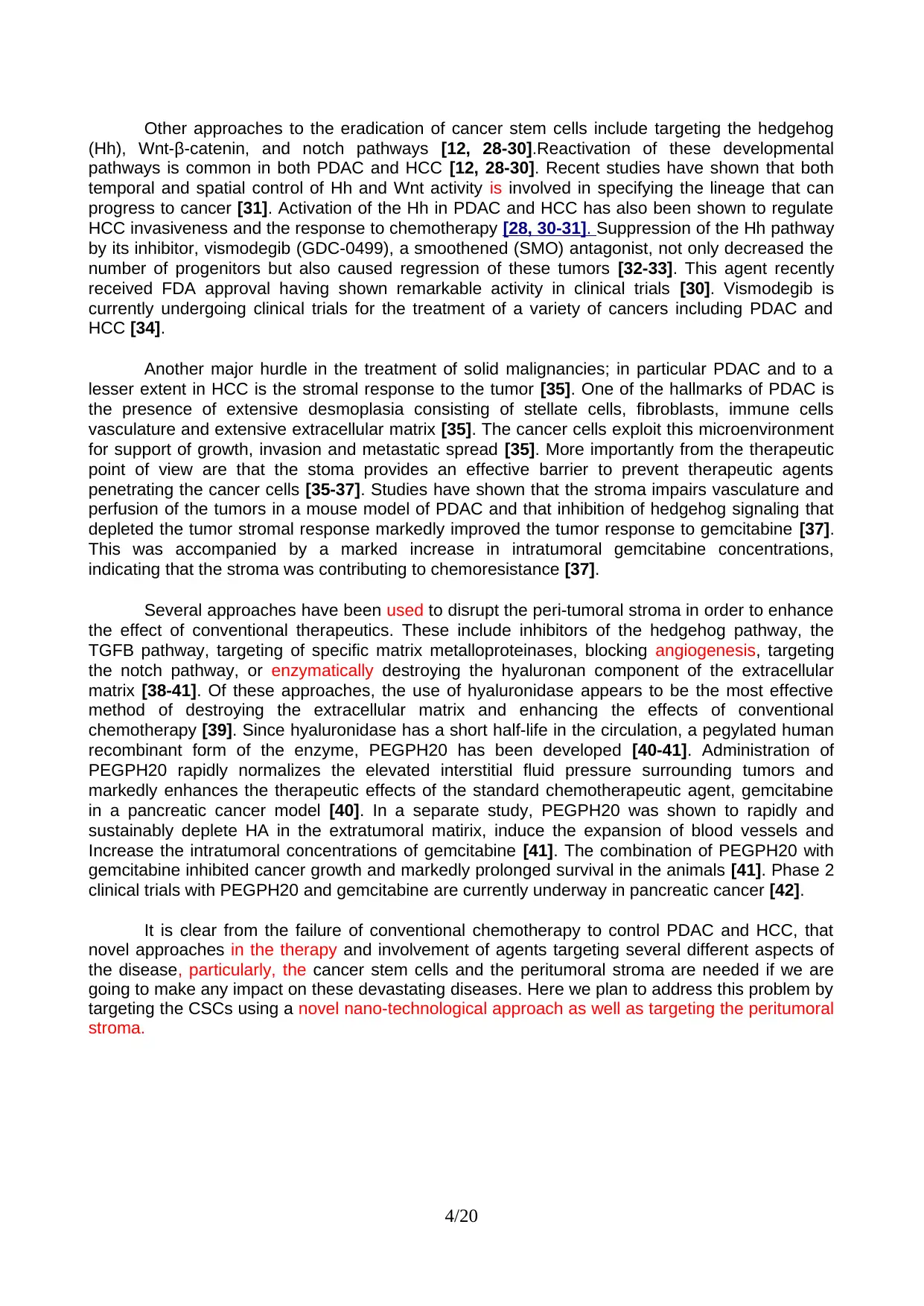
Other approaches to the eradication of cancer stem cells include targeting the hedgehog
(Hh), Wnt-β-catenin, and notch pathways [12, 28-30].Reactivation of these developmental
pathways is common in both PDAC and HCC [12, 28-30]. Recent studies have shown that both
temporal and spatial control of Hh and Wnt activity is involved in specifying the lineage that can
progress to cancer [31]. Activation of the Hh in PDAC and HCC has also been shown to regulate
HCC invasiveness and the response to chemotherapy [28, 30-31]. Suppression of the Hh pathway
by its inhibitor, vismodegib (GDC-0499), a smoothened (SMO) antagonist, not only decreased the
number of progenitors but also caused regression of these tumors [32-33]. This agent recently
received FDA approval having shown remarkable activity in clinical trials [30]. Vismodegib is
currently undergoing clinical trials for the treatment of a variety of cancers including PDAC and
HCC [34].
Another major hurdle in the treatment of solid malignancies; in particular PDAC and to a
lesser extent in HCC is the stromal response to the tumor [35]. One of the hallmarks of PDAC is
the presence of extensive desmoplasia consisting of stellate cells, fibroblasts, immune cells
vasculature and extensive extracellular matrix [35]. The cancer cells exploit this microenvironment
for support of growth, invasion and metastatic spread [35]. More importantly from the therapeutic
point of view are that the stoma provides an effective barrier to prevent therapeutic agents
penetrating the cancer cells [35-37]. Studies have shown that the stroma impairs vasculature and
perfusion of the tumors in a mouse model of PDAC and that inhibition of hedgehog signaling that
depleted the tumor stromal response markedly improved the tumor response to gemcitabine [37].
This was accompanied by a marked increase in intratumoral gemcitabine concentrations,
indicating that the stroma was contributing to chemoresistance [37].
Several approaches have been used to disrupt the peri-tumoral stroma in order to enhance
the effect of conventional therapeutics. These include inhibitors of the hedgehog pathway, the
TGFB pathway, targeting of specific matrix metalloproteinases, blocking angiogenesis, targeting
the notch pathway, or enzymatically destroying the hyaluronan component of the extracellular
matrix [38-41]. Of these approaches, the use of hyaluronidase appears to be the most effective
method of destroying the extracellular matrix and enhancing the effects of conventional
chemotherapy [39]. Since hyaluronidase has a short half-life in the circulation, a pegylated human
recombinant form of the enzyme, PEGPH20 has been developed [40-41]. Administration of
PEGPH20 rapidly normalizes the elevated interstitial fluid pressure surrounding tumors and
markedly enhances the therapeutic effects of the standard chemotherapeutic agent, gemcitabine
in a pancreatic cancer model [40]. In a separate study, PEGPH20 was shown to rapidly and
sustainably deplete HA in the extratumoral matirix, induce the expansion of blood vessels and
Increase the intratumoral concentrations of gemcitabine [41]. The combination of PEGPH20 with
gemcitabine inhibited cancer growth and markedly prolonged survival in the animals [41]. Phase 2
clinical trials with PEGPH20 and gemcitabine are currently underway in pancreatic cancer [42].
It is clear from the failure of conventional chemotherapy to control PDAC and HCC, that
novel approaches in the therapy and involvement of agents targeting several different aspects of
the disease, particularly, the cancer stem cells and the peritumoral stroma are needed if we are
going to make any impact on these devastating diseases. Here we plan to address this problem by
targeting the CSCs using a novel nano-technological approach as well as targeting the peritumoral
stroma.
4/20
(Hh), Wnt-β-catenin, and notch pathways [12, 28-30].Reactivation of these developmental
pathways is common in both PDAC and HCC [12, 28-30]. Recent studies have shown that both
temporal and spatial control of Hh and Wnt activity is involved in specifying the lineage that can
progress to cancer [31]. Activation of the Hh in PDAC and HCC has also been shown to regulate
HCC invasiveness and the response to chemotherapy [28, 30-31]. Suppression of the Hh pathway
by its inhibitor, vismodegib (GDC-0499), a smoothened (SMO) antagonist, not only decreased the
number of progenitors but also caused regression of these tumors [32-33]. This agent recently
received FDA approval having shown remarkable activity in clinical trials [30]. Vismodegib is
currently undergoing clinical trials for the treatment of a variety of cancers including PDAC and
HCC [34].
Another major hurdle in the treatment of solid malignancies; in particular PDAC and to a
lesser extent in HCC is the stromal response to the tumor [35]. One of the hallmarks of PDAC is
the presence of extensive desmoplasia consisting of stellate cells, fibroblasts, immune cells
vasculature and extensive extracellular matrix [35]. The cancer cells exploit this microenvironment
for support of growth, invasion and metastatic spread [35]. More importantly from the therapeutic
point of view are that the stoma provides an effective barrier to prevent therapeutic agents
penetrating the cancer cells [35-37]. Studies have shown that the stroma impairs vasculature and
perfusion of the tumors in a mouse model of PDAC and that inhibition of hedgehog signaling that
depleted the tumor stromal response markedly improved the tumor response to gemcitabine [37].
This was accompanied by a marked increase in intratumoral gemcitabine concentrations,
indicating that the stroma was contributing to chemoresistance [37].
Several approaches have been used to disrupt the peri-tumoral stroma in order to enhance
the effect of conventional therapeutics. These include inhibitors of the hedgehog pathway, the
TGFB pathway, targeting of specific matrix metalloproteinases, blocking angiogenesis, targeting
the notch pathway, or enzymatically destroying the hyaluronan component of the extracellular
matrix [38-41]. Of these approaches, the use of hyaluronidase appears to be the most effective
method of destroying the extracellular matrix and enhancing the effects of conventional
chemotherapy [39]. Since hyaluronidase has a short half-life in the circulation, a pegylated human
recombinant form of the enzyme, PEGPH20 has been developed [40-41]. Administration of
PEGPH20 rapidly normalizes the elevated interstitial fluid pressure surrounding tumors and
markedly enhances the therapeutic effects of the standard chemotherapeutic agent, gemcitabine
in a pancreatic cancer model [40]. In a separate study, PEGPH20 was shown to rapidly and
sustainably deplete HA in the extratumoral matirix, induce the expansion of blood vessels and
Increase the intratumoral concentrations of gemcitabine [41]. The combination of PEGPH20 with
gemcitabine inhibited cancer growth and markedly prolonged survival in the animals [41]. Phase 2
clinical trials with PEGPH20 and gemcitabine are currently underway in pancreatic cancer [42].
It is clear from the failure of conventional chemotherapy to control PDAC and HCC, that
novel approaches in the therapy and involvement of agents targeting several different aspects of
the disease, particularly, the cancer stem cells and the peritumoral stroma are needed if we are
going to make any impact on these devastating diseases. Here we plan to address this problem by
targeting the CSCs using a novel nano-technological approach as well as targeting the peritumoral
stroma.
4/20
Secure Best Marks with AI Grader
Need help grading? Try our AI Grader for instant feedback on your assignments.
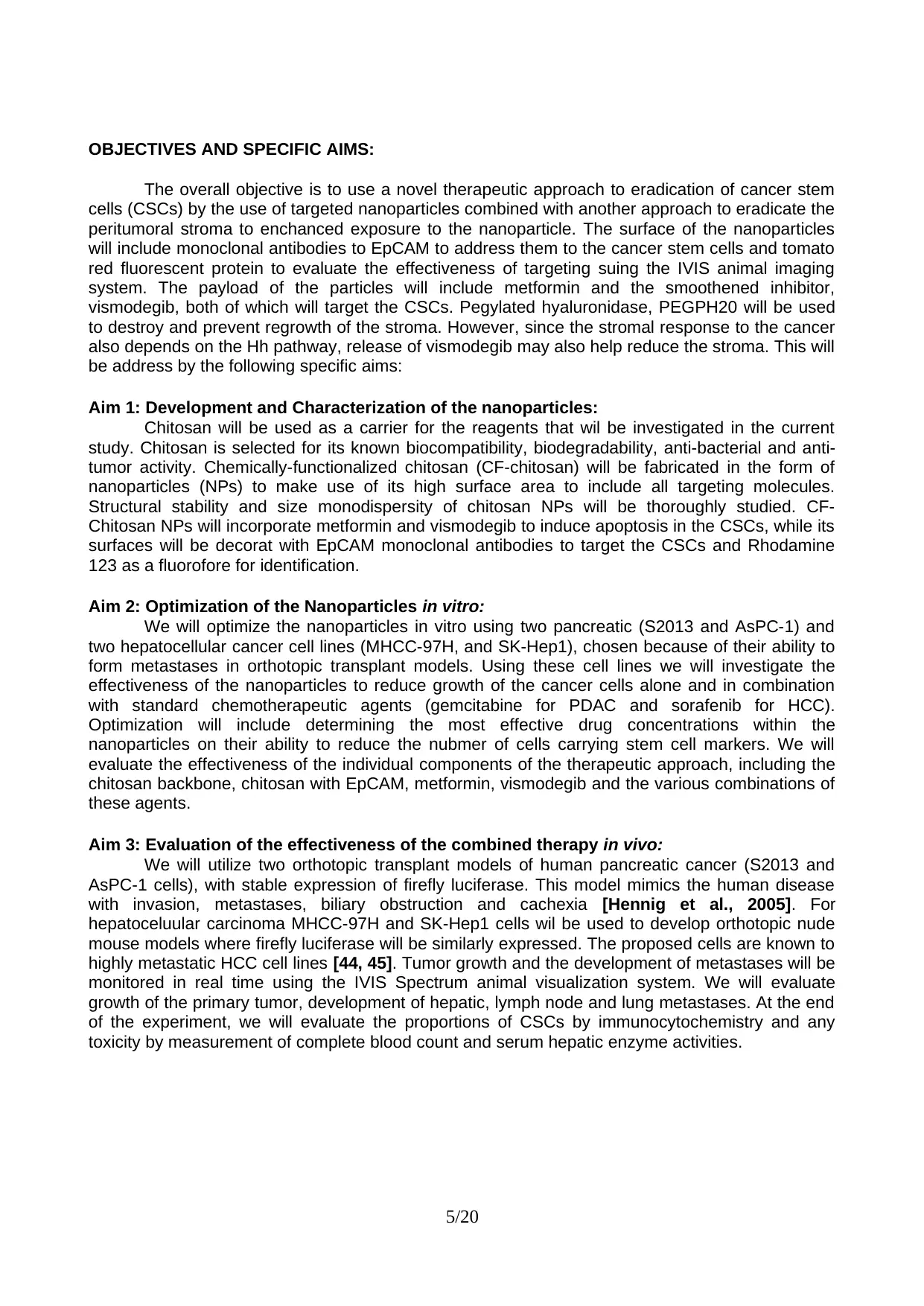
OBJECTIVES AND SPECIFIC AIMS:
The overall objective is to use a novel therapeutic approach to eradication of cancer stem
cells (CSCs) by the use of targeted nanoparticles combined with another approach to eradicate the
peritumoral stroma to enchanced exposure to the nanoparticle. The surface of the nanoparticles
will include monoclonal antibodies to EpCAM to address them to the cancer stem cells and tomato
red fluorescent protein to evaluate the effectiveness of targeting suing the IVIS animal imaging
system. The payload of the particles will include metformin and the smoothened inhibitor,
vismodegib, both of which will target the CSCs. Pegylated hyaluronidase, PEGPH20 will be used
to destroy and prevent regrowth of the stroma. However, since the stromal response to the cancer
also depends on the Hh pathway, release of vismodegib may also help reduce the stroma. This will
be address by the following specific aims:
Aim 1: Development and Characterization of the nanoparticles:
Chitosan will be used as a carrier for the reagents that wil be investigated in the current
study. Chitosan is selected for its known biocompatibility, biodegradability, anti-bacterial and anti-
tumor activity. Chemically-functionalized chitosan (CF-chitosan) will be fabricated in the form of
nanoparticles (NPs) to make use of its high surface area to include all targeting molecules.
Structural stability and size monodispersity of chitosan NPs will be thoroughly studied. CF-
Chitosan NPs will incorporate metformin and vismodegib to induce apoptosis in the CSCs, while its
surfaces will be decorat with EpCAM monoclonal antibodies to target the CSCs and Rhodamine
123 as a fluorofore for identification.
Aim 2: Optimization of the Nanoparticles in vitro:
We will optimize the nanoparticles in vitro using two pancreatic (S2013 and AsPC-1) and
two hepatocellular cancer cell lines (MHCC-97H, and SK-Hep1), chosen because of their ability to
form metastases in orthotopic transplant models. Using these cell lines we will investigate the
effectiveness of the nanoparticles to reduce growth of the cancer cells alone and in combination
with standard chemotherapeutic agents (gemcitabine for PDAC and sorafenib for HCC).
Optimization will include determining the most effective drug concentrations within the
nanoparticles on their ability to reduce the nubmer of cells carrying stem cell markers. We will
evaluate the effectiveness of the individual components of the therapeutic approach, including the
chitosan backbone, chitosan with EpCAM, metformin, vismodegib and the various combinations of
these agents.
Aim 3: Evaluation of the effectiveness of the combined therapy in vivo:
We will utilize two orthotopic transplant models of human pancreatic cancer (S2013 and
AsPC-1 cells), with stable expression of firefly luciferase. This model mimics the human disease
with invasion, metastases, biliary obstruction and cachexia [Hennig et al., 2005]. For
hepatoceluular carcinoma MHCC-97H and SK-Hep1 cells wil be used to develop orthotopic nude
mouse models where firefly luciferase will be similarly expressed. The proposed cells are known to
highly metastatic HCC cell lines [44, 45]. Tumor growth and the development of metastases will be
monitored in real time using the IVIS Spectrum animal visualization system. We will evaluate
growth of the primary tumor, development of hepatic, lymph node and lung metastases. At the end
of the experiment, we will evaluate the proportions of CSCs by immunocytochemistry and any
toxicity by measurement of complete blood count and serum hepatic enzyme activities.
5/20
The overall objective is to use a novel therapeutic approach to eradication of cancer stem
cells (CSCs) by the use of targeted nanoparticles combined with another approach to eradicate the
peritumoral stroma to enchanced exposure to the nanoparticle. The surface of the nanoparticles
will include monoclonal antibodies to EpCAM to address them to the cancer stem cells and tomato
red fluorescent protein to evaluate the effectiveness of targeting suing the IVIS animal imaging
system. The payload of the particles will include metformin and the smoothened inhibitor,
vismodegib, both of which will target the CSCs. Pegylated hyaluronidase, PEGPH20 will be used
to destroy and prevent regrowth of the stroma. However, since the stromal response to the cancer
also depends on the Hh pathway, release of vismodegib may also help reduce the stroma. This will
be address by the following specific aims:
Aim 1: Development and Characterization of the nanoparticles:
Chitosan will be used as a carrier for the reagents that wil be investigated in the current
study. Chitosan is selected for its known biocompatibility, biodegradability, anti-bacterial and anti-
tumor activity. Chemically-functionalized chitosan (CF-chitosan) will be fabricated in the form of
nanoparticles (NPs) to make use of its high surface area to include all targeting molecules.
Structural stability and size monodispersity of chitosan NPs will be thoroughly studied. CF-
Chitosan NPs will incorporate metformin and vismodegib to induce apoptosis in the CSCs, while its
surfaces will be decorat with EpCAM monoclonal antibodies to target the CSCs and Rhodamine
123 as a fluorofore for identification.
Aim 2: Optimization of the Nanoparticles in vitro:
We will optimize the nanoparticles in vitro using two pancreatic (S2013 and AsPC-1) and
two hepatocellular cancer cell lines (MHCC-97H, and SK-Hep1), chosen because of their ability to
form metastases in orthotopic transplant models. Using these cell lines we will investigate the
effectiveness of the nanoparticles to reduce growth of the cancer cells alone and in combination
with standard chemotherapeutic agents (gemcitabine for PDAC and sorafenib for HCC).
Optimization will include determining the most effective drug concentrations within the
nanoparticles on their ability to reduce the nubmer of cells carrying stem cell markers. We will
evaluate the effectiveness of the individual components of the therapeutic approach, including the
chitosan backbone, chitosan with EpCAM, metformin, vismodegib and the various combinations of
these agents.
Aim 3: Evaluation of the effectiveness of the combined therapy in vivo:
We will utilize two orthotopic transplant models of human pancreatic cancer (S2013 and
AsPC-1 cells), with stable expression of firefly luciferase. This model mimics the human disease
with invasion, metastases, biliary obstruction and cachexia [Hennig et al., 2005]. For
hepatoceluular carcinoma MHCC-97H and SK-Hep1 cells wil be used to develop orthotopic nude
mouse models where firefly luciferase will be similarly expressed. The proposed cells are known to
highly metastatic HCC cell lines [44, 45]. Tumor growth and the development of metastases will be
monitored in real time using the IVIS Spectrum animal visualization system. We will evaluate
growth of the primary tumor, development of hepatic, lymph node and lung metastases. At the end
of the experiment, we will evaluate the proportions of CSCs by immunocytochemistry and any
toxicity by measurement of complete blood count and serum hepatic enzyme activities.
5/20
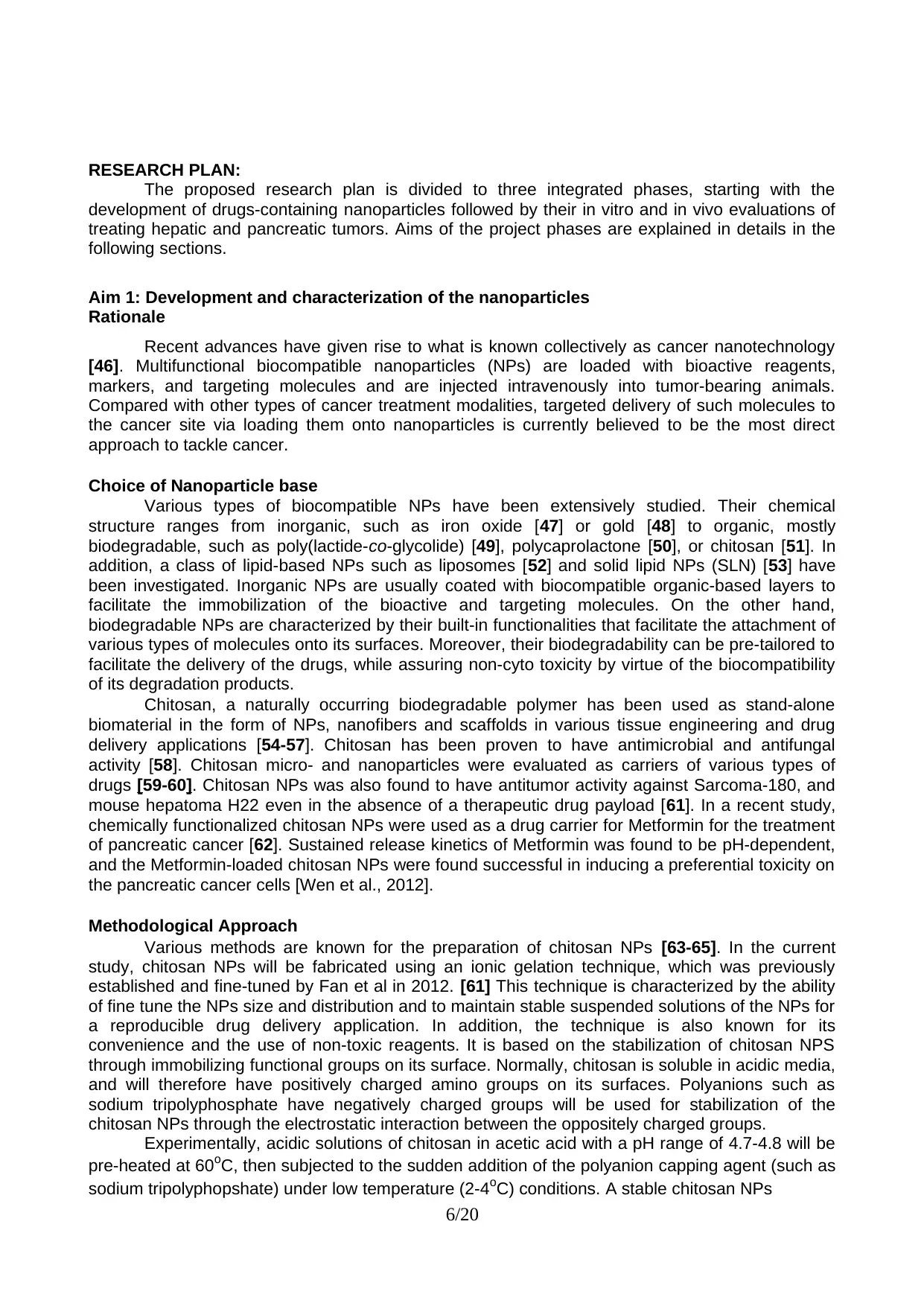
RESEARCH PLAN:
The proposed research plan is divided to three integrated phases, starting with the
development of drugs-containing nanoparticles followed by their in vitro and in vivo evaluations of
treating hepatic and pancreatic tumors. Aims of the project phases are explained in details in the
following sections.
Aim 1: Development and characterization of the nanoparticles
Rationale
Recent advances have given rise to what is known collectively as cancer nanotechnology
[46]. Multifunctional biocompatible nanoparticles (NPs) are loaded with bioactive reagents,
markers, and targeting molecules and are injected intravenously into tumor-bearing animals.
Compared with other types of cancer treatment modalities, targeted delivery of such molecules to
the cancer site via loading them onto nanoparticles is currently believed to be the most direct
approach to tackle cancer.
Choice of Nanoparticle base
Various types of biocompatible NPs have been extensively studied. Their chemical
structure ranges from inorganic, such as iron oxide [47] or gold [48] to organic, mostly
biodegradable, such as poly(lactide-co-glycolide) [49], polycaprolactone [50], or chitosan [51]. In
addition, a class of lipid-based NPs such as liposomes [52] and solid lipid NPs (SLN) [53] have
been investigated. Inorganic NPs are usually coated with biocompatible organic-based layers to
facilitate the immobilization of the bioactive and targeting molecules. On the other hand,
biodegradable NPs are characterized by their built-in functionalities that facilitate the attachment of
various types of molecules onto its surfaces. Moreover, their biodegradability can be pre-tailored to
facilitate the delivery of the drugs, while assuring non-cyto toxicity by virtue of the biocompatibility
of its degradation products.
Chitosan, a naturally occurring biodegradable polymer has been used as stand-alone
biomaterial in the form of NPs, nanofibers and scaffolds in various tissue engineering and drug
delivery applications [54-57]. Chitosan has been proven to have antimicrobial and antifungal
activity [58]. Chitosan micro- and nanoparticles were evaluated as carriers of various types of
drugs [59-60]. Chitosan NPs was also found to have antitumor activity against Sarcoma-180, and
mouse hepatoma H22 even in the absence of a therapeutic drug payload [61]. In a recent study,
chemically functionalized chitosan NPs were used as a drug carrier for Metformin for the treatment
of pancreatic cancer [62]. Sustained release kinetics of Metformin was found to be pH-dependent,
and the Metformin-loaded chitosan NPs were found successful in inducing a preferential toxicity on
the pancreatic cancer cells [Wen et al., 2012].
Methodological Approach
Various methods are known for the preparation of chitosan NPs [63-65]. In the current
study, chitosan NPs will be fabricated using an ionic gelation technique, which was previously
established and fine-tuned by Fan et al in 2012. [61] This technique is characterized by the ability
of fine tune the NPs size and distribution and to maintain stable suspended solutions of the NPs for
a reproducible drug delivery application. In addition, the technique is also known for its
convenience and the use of non-toxic reagents. It is based on the stabilization of chitosan NPS
through immobilizing functional groups on its surface. Normally, chitosan is soluble in acidic media,
and will therefore have positively charged amino groups on its surfaces. Polyanions such as
sodium tripolyphosphate have negatively charged groups will be used for stabilization of the
chitosan NPs through the electrostatic interaction between the oppositely charged groups.
Experimentally, acidic solutions of chitosan in acetic acid with a pH range of 4.7-4.8 will be
pre-heated at 60oC, then subjected to the sudden addition of the polyanion capping agent (such as
sodium tripolyphopshate) under low temperature (2-4oC) conditions. A stable chitosan NPs
6/20
The proposed research plan is divided to three integrated phases, starting with the
development of drugs-containing nanoparticles followed by their in vitro and in vivo evaluations of
treating hepatic and pancreatic tumors. Aims of the project phases are explained in details in the
following sections.
Aim 1: Development and characterization of the nanoparticles
Rationale
Recent advances have given rise to what is known collectively as cancer nanotechnology
[46]. Multifunctional biocompatible nanoparticles (NPs) are loaded with bioactive reagents,
markers, and targeting molecules and are injected intravenously into tumor-bearing animals.
Compared with other types of cancer treatment modalities, targeted delivery of such molecules to
the cancer site via loading them onto nanoparticles is currently believed to be the most direct
approach to tackle cancer.
Choice of Nanoparticle base
Various types of biocompatible NPs have been extensively studied. Their chemical
structure ranges from inorganic, such as iron oxide [47] or gold [48] to organic, mostly
biodegradable, such as poly(lactide-co-glycolide) [49], polycaprolactone [50], or chitosan [51]. In
addition, a class of lipid-based NPs such as liposomes [52] and solid lipid NPs (SLN) [53] have
been investigated. Inorganic NPs are usually coated with biocompatible organic-based layers to
facilitate the immobilization of the bioactive and targeting molecules. On the other hand,
biodegradable NPs are characterized by their built-in functionalities that facilitate the attachment of
various types of molecules onto its surfaces. Moreover, their biodegradability can be pre-tailored to
facilitate the delivery of the drugs, while assuring non-cyto toxicity by virtue of the biocompatibility
of its degradation products.
Chitosan, a naturally occurring biodegradable polymer has been used as stand-alone
biomaterial in the form of NPs, nanofibers and scaffolds in various tissue engineering and drug
delivery applications [54-57]. Chitosan has been proven to have antimicrobial and antifungal
activity [58]. Chitosan micro- and nanoparticles were evaluated as carriers of various types of
drugs [59-60]. Chitosan NPs was also found to have antitumor activity against Sarcoma-180, and
mouse hepatoma H22 even in the absence of a therapeutic drug payload [61]. In a recent study,
chemically functionalized chitosan NPs were used as a drug carrier for Metformin for the treatment
of pancreatic cancer [62]. Sustained release kinetics of Metformin was found to be pH-dependent,
and the Metformin-loaded chitosan NPs were found successful in inducing a preferential toxicity on
the pancreatic cancer cells [Wen et al., 2012].
Methodological Approach
Various methods are known for the preparation of chitosan NPs [63-65]. In the current
study, chitosan NPs will be fabricated using an ionic gelation technique, which was previously
established and fine-tuned by Fan et al in 2012. [61] This technique is characterized by the ability
of fine tune the NPs size and distribution and to maintain stable suspended solutions of the NPs for
a reproducible drug delivery application. In addition, the technique is also known for its
convenience and the use of non-toxic reagents. It is based on the stabilization of chitosan NPS
through immobilizing functional groups on its surface. Normally, chitosan is soluble in acidic media,
and will therefore have positively charged amino groups on its surfaces. Polyanions such as
sodium tripolyphosphate have negatively charged groups will be used for stabilization of the
chitosan NPs through the electrostatic interaction between the oppositely charged groups.
Experimentally, acidic solutions of chitosan in acetic acid with a pH range of 4.7-4.8 will be
pre-heated at 60oC, then subjected to the sudden addition of the polyanion capping agent (such as
sodium tripolyphopshate) under low temperature (2-4oC) conditions. A stable chitosan NPs
6/20
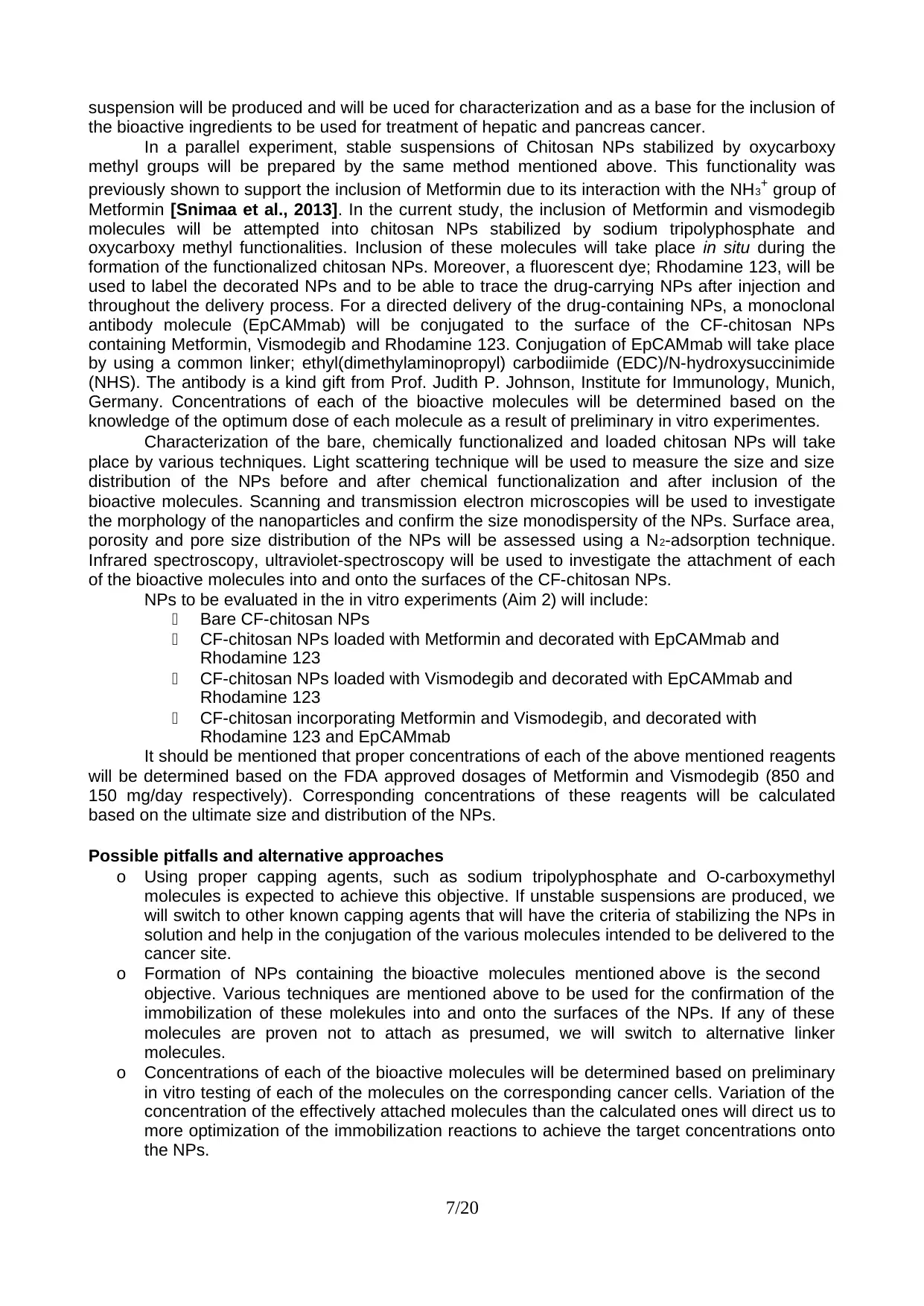
suspension will be produced and will be uced for characterization and as a base for the inclusion of
the bioactive ingredients to be used for treatment of hepatic and pancreas cancer.
In a parallel experiment, stable suspensions of Chitosan NPs stabilized by oxycarboxy
methyl groups will be prepared by the same method mentioned above. This functionality was
previously shown to support the inclusion of Metformin due to its interaction with the NH3+ group of
Metformin [Snimaa et al., 2013]. In the current study, the inclusion of Metformin and vismodegib
molecules will be attempted into chitosan NPs stabilized by sodium tripolyphosphate and
oxycarboxy methyl functionalities. Inclusion of these molecules will take place in situ during the
formation of the functionalized chitosan NPs. Moreover, a fluorescent dye; Rhodamine 123, will be
used to label the decorated NPs and to be able to trace the drug-carrying NPs after injection and
throughout the delivery process. For a directed delivery of the drug-containing NPs, a monoclonal
antibody molecule (EpCAMmab) will be conjugated to the surface of the CF-chitosan NPs
containing Metformin, Vismodegib and Rhodamine 123. Conjugation of EpCAMmab will take place
by using a common linker; ethyl(dimethylaminopropyl) carbodiimide (EDC)/N-hydroxysuccinimide
(NHS). The antibody is a kind gift from Prof. Judith P. Johnson, Institute for Immunology, Munich,
Germany. Concentrations of each of the bioactive molecules will be determined based on the
knowledge of the optimum dose of each molecule as a result of preliminary in vitro experimentes.
Characterization of the bare, chemically functionalized and loaded chitosan NPs will take
place by various techniques. Light scattering technique will be used to measure the size and size
distribution of the NPs before and after chemical functionalization and after inclusion of the
bioactive molecules. Scanning and transmission electron microscopies will be used to investigate
the morphology of the nanoparticles and confirm the size monodispersity of the NPs. Surface area,
porosity and pore size distribution of the NPs will be assessed using a N2-adsorption technique.
Infrared spectroscopy, ultraviolet-spectroscopy will be used to investigate the attachment of each
of the bioactive molecules into and onto the surfaces of the CF-chitosan NPs.
NPs to be evaluated in the in vitro experiments (Aim 2) will include:
Bare CF-chitosan NPs
CF-chitosan NPs loaded with Metformin and decorated with EpCAMmab and
Rhodamine 123
CF-chitosan NPs loaded with Vismodegib and decorated with EpCAMmab and
Rhodamine 123
CF-chitosan incorporating Metformin and Vismodegib, and decorated with
Rhodamine 123 and EpCAMmab
It should be mentioned that proper concentrations of each of the above mentioned reagents
will be determined based on the FDA approved dosages of Metformin and Vismodegib (850 and
150 mg/day respectively). Corresponding concentrations of these reagents will be calculated
based on the ultimate size and distribution of the NPs.
Possible pitfalls and alternative approaches
o Using proper capping agents, such as sodium tripolyphosphate and O-carboxymethyl
molecules is expected to achieve this objective. If unstable suspensions are produced, we
will switch to other known capping agents that will have the criteria of stabilizing the NPs in
solution and help in the conjugation of the various molecules intended to be delivered to the
cancer site.
o Formation of NPs containing the bioactive molecules mentioned above is the second
objective. Various techniques are mentioned above to be used for the confirmation of the
immobilization of these molekules into and onto the surfaces of the NPs. If any of these
molecules are proven not to attach as presumed, we will switch to alternative linker
molecules.
o Concentrations of each of the bioactive molecules will be determined based on preliminary
in vitro testing of each of the molecules on the corresponding cancer cells. Variation of the
concentration of the effectively attached molecules than the calculated ones will direct us to
more optimization of the immobilization reactions to achieve the target concentrations onto
the NPs.
7/20
the bioactive ingredients to be used for treatment of hepatic and pancreas cancer.
In a parallel experiment, stable suspensions of Chitosan NPs stabilized by oxycarboxy
methyl groups will be prepared by the same method mentioned above. This functionality was
previously shown to support the inclusion of Metformin due to its interaction with the NH3+ group of
Metformin [Snimaa et al., 2013]. In the current study, the inclusion of Metformin and vismodegib
molecules will be attempted into chitosan NPs stabilized by sodium tripolyphosphate and
oxycarboxy methyl functionalities. Inclusion of these molecules will take place in situ during the
formation of the functionalized chitosan NPs. Moreover, a fluorescent dye; Rhodamine 123, will be
used to label the decorated NPs and to be able to trace the drug-carrying NPs after injection and
throughout the delivery process. For a directed delivery of the drug-containing NPs, a monoclonal
antibody molecule (EpCAMmab) will be conjugated to the surface of the CF-chitosan NPs
containing Metformin, Vismodegib and Rhodamine 123. Conjugation of EpCAMmab will take place
by using a common linker; ethyl(dimethylaminopropyl) carbodiimide (EDC)/N-hydroxysuccinimide
(NHS). The antibody is a kind gift from Prof. Judith P. Johnson, Institute for Immunology, Munich,
Germany. Concentrations of each of the bioactive molecules will be determined based on the
knowledge of the optimum dose of each molecule as a result of preliminary in vitro experimentes.
Characterization of the bare, chemically functionalized and loaded chitosan NPs will take
place by various techniques. Light scattering technique will be used to measure the size and size
distribution of the NPs before and after chemical functionalization and after inclusion of the
bioactive molecules. Scanning and transmission electron microscopies will be used to investigate
the morphology of the nanoparticles and confirm the size monodispersity of the NPs. Surface area,
porosity and pore size distribution of the NPs will be assessed using a N2-adsorption technique.
Infrared spectroscopy, ultraviolet-spectroscopy will be used to investigate the attachment of each
of the bioactive molecules into and onto the surfaces of the CF-chitosan NPs.
NPs to be evaluated in the in vitro experiments (Aim 2) will include:
Bare CF-chitosan NPs
CF-chitosan NPs loaded with Metformin and decorated with EpCAMmab and
Rhodamine 123
CF-chitosan NPs loaded with Vismodegib and decorated with EpCAMmab and
Rhodamine 123
CF-chitosan incorporating Metformin and Vismodegib, and decorated with
Rhodamine 123 and EpCAMmab
It should be mentioned that proper concentrations of each of the above mentioned reagents
will be determined based on the FDA approved dosages of Metformin and Vismodegib (850 and
150 mg/day respectively). Corresponding concentrations of these reagents will be calculated
based on the ultimate size and distribution of the NPs.
Possible pitfalls and alternative approaches
o Using proper capping agents, such as sodium tripolyphosphate and O-carboxymethyl
molecules is expected to achieve this objective. If unstable suspensions are produced, we
will switch to other known capping agents that will have the criteria of stabilizing the NPs in
solution and help in the conjugation of the various molecules intended to be delivered to the
cancer site.
o Formation of NPs containing the bioactive molecules mentioned above is the second
objective. Various techniques are mentioned above to be used for the confirmation of the
immobilization of these molekules into and onto the surfaces of the NPs. If any of these
molecules are proven not to attach as presumed, we will switch to alternative linker
molecules.
o Concentrations of each of the bioactive molecules will be determined based on preliminary
in vitro testing of each of the molecules on the corresponding cancer cells. Variation of the
concentration of the effectively attached molecules than the calculated ones will direct us to
more optimization of the immobilization reactions to achieve the target concentrations onto
the NPs.
7/20
Paraphrase This Document
Need a fresh take? Get an instant paraphrase of this document with our AI Paraphraser
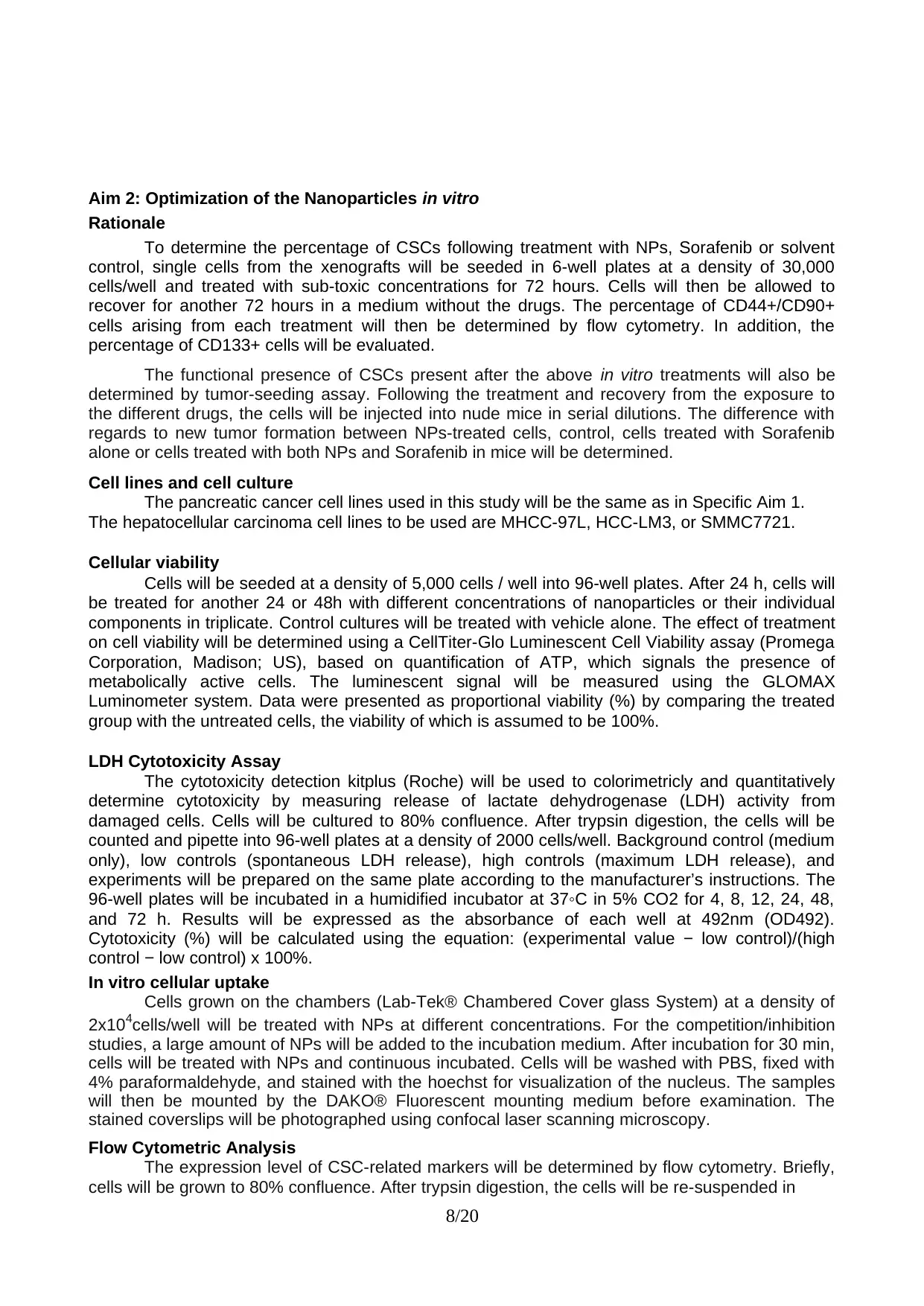
Aim 2: Optimization of the Nanoparticles in vitro
Rationale
To determine the percentage of CSCs following treatment with NPs, Sorafenib or solvent
control, single cells from the xenografts will be seeded in 6-well plates at a density of 30,000
cells/well and treated with sub-toxic concentrations for 72 hours. Cells will then be allowed to
recover for another 72 hours in a medium without the drugs. The percentage of CD44+/CD90+
cells arising from each treatment will then be determined by flow cytometry. In addition, the
percentage of CD133+ cells will be evaluated.
The functional presence of CSCs present after the above in vitro treatments will also be
determined by tumor-seeding assay. Following the treatment and recovery from the exposure to
the different drugs, the cells will be injected into nude mice in serial dilutions. The difference with
regards to new tumor formation between NPs-treated cells, control, cells treated with Sorafenib
alone or cells treated with both NPs and Sorafenib in mice will be determined.
Cell lines and cell culture
The pancreatic cancer cell lines used in this study will be the same as in Specific Aim 1.
The hepatocellular carcinoma cell lines to be used are MHCC-97L, HCC-LM3, or SMMC7721.
Cellular viability
Cells will be seeded at a density of 5,000 cells / well into 96-well plates. After 24 h, cells will
be treated for another 24 or 48h with different concentrations of nanoparticles or their individual
components in triplicate. Control cultures will be treated with vehicle alone. The effect of treatment
on cell viability will be determined using a CellTiter-Glo Luminescent Cell Viability assay (Promega
Corporation, Madison; US), based on quantification of ATP, which signals the presence of
metabolically active cells. The luminescent signal will be measured using the GLOMAX
Luminometer system. Data were presented as proportional viability (%) by comparing the treated
group with the untreated cells, the viability of which is assumed to be 100%.
LDH Cytotoxicity Assay
The cytotoxicity detection kitplus (Roche) will be used to colorimetricly and quantitatively
determine cytotoxicity by measuring release of lactate dehydrogenase (LDH) activity from
damaged cells. Cells will be cultured to 80% confluence. After trypsin digestion, the cells will be
counted and pipette into 96-well plates at a density of 2000 cells/well. Background control (medium
only), low controls (spontaneous LDH release), high controls (maximum LDH release), and
experiments will be prepared on the same plate according to the manufacturer’s instructions. The
96-well plates will be incubated in a humidified incubator at 37◦C in 5% CO2 for 4, 8, 12, 24, 48,
and 72 h. Results will be expressed as the absorbance of each well at 492nm (OD492).
Cytotoxicity (%) will be calculated using the equation: (experimental value − low control)/(high
control − low control) x 100%.
In vitro cellular uptake
Cells grown on the chambers (Lab-Tek® Chambered Cover glass System) at a density of
2x104cells/well will be treated with NPs at different concentrations. For the competition/inhibition
studies, a large amount of NPs will be added to the incubation medium. After incubation for 30 min,
cells will be treated with NPs and continuous incubated. Cells will be washed with PBS, fixed with
4% paraformaldehyde, and stained with the hoechst for visualization of the nucleus. The samples
will then be mounted by the DAKO® Fluorescent mounting medium before examination. The
stained coverslips will be photographed using confocal laser scanning microscopy.
Flow Cytometric Analysis
The expression level of CSC-related markers will be determined by flow cytometry. Briefly,
cells will be grown to 80% confluence. After trypsin digestion, the cells will be re-suspended in
8/20
Rationale
To determine the percentage of CSCs following treatment with NPs, Sorafenib or solvent
control, single cells from the xenografts will be seeded in 6-well plates at a density of 30,000
cells/well and treated with sub-toxic concentrations for 72 hours. Cells will then be allowed to
recover for another 72 hours in a medium without the drugs. The percentage of CD44+/CD90+
cells arising from each treatment will then be determined by flow cytometry. In addition, the
percentage of CD133+ cells will be evaluated.
The functional presence of CSCs present after the above in vitro treatments will also be
determined by tumor-seeding assay. Following the treatment and recovery from the exposure to
the different drugs, the cells will be injected into nude mice in serial dilutions. The difference with
regards to new tumor formation between NPs-treated cells, control, cells treated with Sorafenib
alone or cells treated with both NPs and Sorafenib in mice will be determined.
Cell lines and cell culture
The pancreatic cancer cell lines used in this study will be the same as in Specific Aim 1.
The hepatocellular carcinoma cell lines to be used are MHCC-97L, HCC-LM3, or SMMC7721.
Cellular viability
Cells will be seeded at a density of 5,000 cells / well into 96-well plates. After 24 h, cells will
be treated for another 24 or 48h with different concentrations of nanoparticles or their individual
components in triplicate. Control cultures will be treated with vehicle alone. The effect of treatment
on cell viability will be determined using a CellTiter-Glo Luminescent Cell Viability assay (Promega
Corporation, Madison; US), based on quantification of ATP, which signals the presence of
metabolically active cells. The luminescent signal will be measured using the GLOMAX
Luminometer system. Data were presented as proportional viability (%) by comparing the treated
group with the untreated cells, the viability of which is assumed to be 100%.
LDH Cytotoxicity Assay
The cytotoxicity detection kitplus (Roche) will be used to colorimetricly and quantitatively
determine cytotoxicity by measuring release of lactate dehydrogenase (LDH) activity from
damaged cells. Cells will be cultured to 80% confluence. After trypsin digestion, the cells will be
counted and pipette into 96-well plates at a density of 2000 cells/well. Background control (medium
only), low controls (spontaneous LDH release), high controls (maximum LDH release), and
experiments will be prepared on the same plate according to the manufacturer’s instructions. The
96-well plates will be incubated in a humidified incubator at 37◦C in 5% CO2 for 4, 8, 12, 24, 48,
and 72 h. Results will be expressed as the absorbance of each well at 492nm (OD492).
Cytotoxicity (%) will be calculated using the equation: (experimental value − low control)/(high
control − low control) x 100%.
In vitro cellular uptake
Cells grown on the chambers (Lab-Tek® Chambered Cover glass System) at a density of
2x104cells/well will be treated with NPs at different concentrations. For the competition/inhibition
studies, a large amount of NPs will be added to the incubation medium. After incubation for 30 min,
cells will be treated with NPs and continuous incubated. Cells will be washed with PBS, fixed with
4% paraformaldehyde, and stained with the hoechst for visualization of the nucleus. The samples
will then be mounted by the DAKO® Fluorescent mounting medium before examination. The
stained coverslips will be photographed using confocal laser scanning microscopy.
Flow Cytometric Analysis
The expression level of CSC-related markers will be determined by flow cytometry. Briefly,
cells will be grown to 80% confluence. After trypsin digestion, the cells will be re-suspended in
8/20
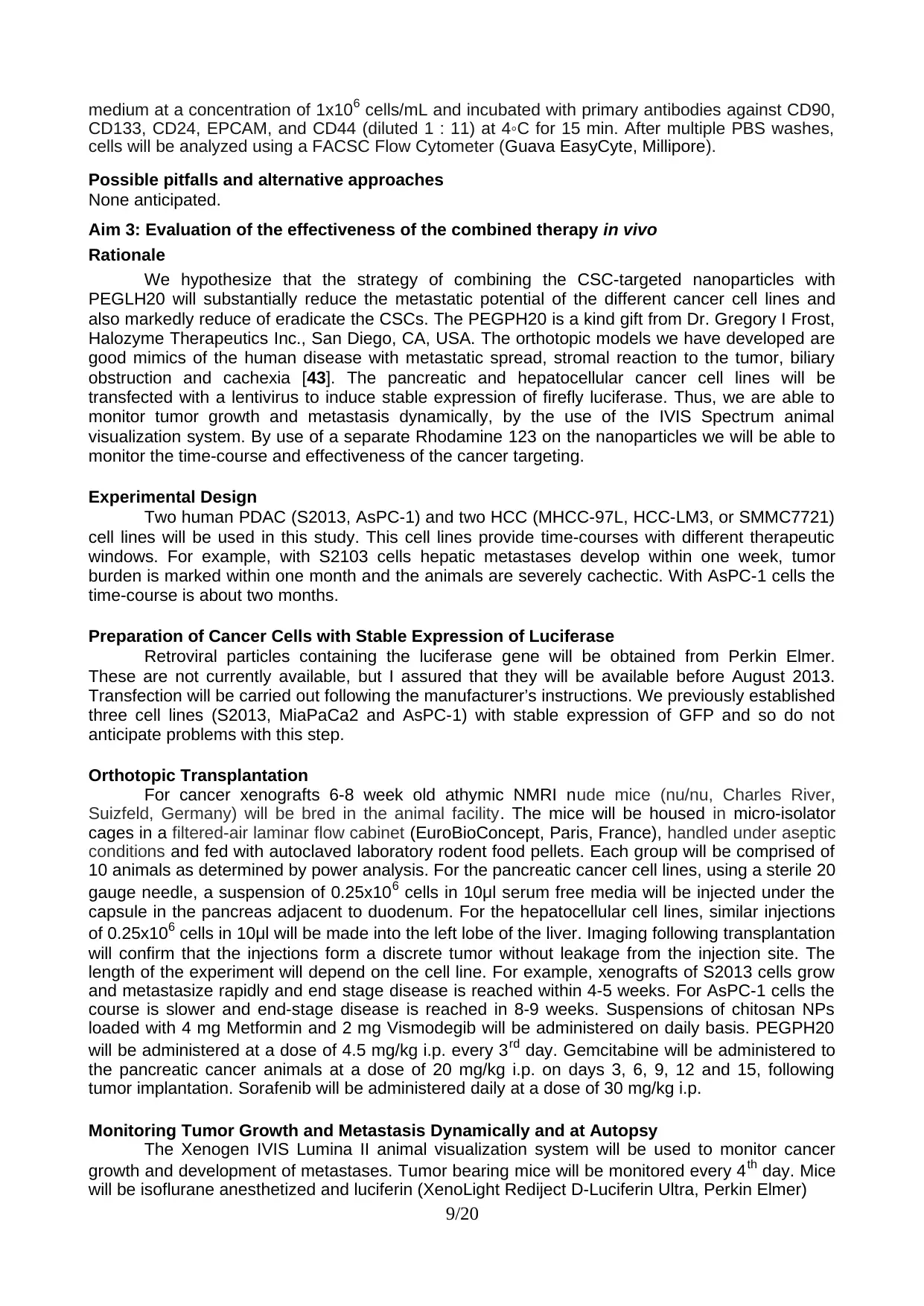
medium at a concentration of 1x106 cells/mL and incubated with primary antibodies against CD90,
CD133, CD24, EPCAM, and CD44 (diluted 1 : 11) at 4◦C for 15 min. After multiple PBS washes,
cells will be analyzed using a FACSC Flow Cytometer (Guava EasyCyte, Millipore).
Possible pitfalls and alternative approaches
None anticipated.
Aim 3: Evaluation of the effectiveness of the combined therapy in vivo
Rationale
We hypothesize that the strategy of combining the CSC-targeted nanoparticles with
PEGLH20 will substantially reduce the metastatic potential of the different cancer cell lines and
also markedly reduce of eradicate the CSCs. The PEGPH20 is a kind gift from Dr. Gregory I Frost,
Halozyme Therapeutics Inc., San Diego, CA, USA. The orthotopic models we have developed are
good mimics of the human disease with metastatic spread, stromal reaction to the tumor, biliary
obstruction and cachexia [43]. The pancreatic and hepatocellular cancer cell lines will be
transfected with a lentivirus to induce stable expression of firefly luciferase. Thus, we are able to
monitor tumor growth and metastasis dynamically, by the use of the IVIS Spectrum animal
visualization system. By use of a separate Rhodamine 123 on the nanoparticles we will be able to
monitor the time-course and effectiveness of the cancer targeting.
Experimental Design
Two human PDAC (S2013, AsPC-1) and two HCC (MHCC-97L, HCC-LM3, or SMMC7721)
cell lines will be used in this study. This cell lines provide time-courses with different therapeutic
windows. For example, with S2103 cells hepatic metastases develop within one week, tumor
burden is marked within one month and the animals are severely cachectic. With AsPC-1 cells the
time-course is about two months.
Preparation of Cancer Cells with Stable Expression of Luciferase
Retroviral particles containing the luciferase gene will be obtained from Perkin Elmer.
These are not currently available, but I assured that they will be available before August 2013.
Transfection will be carried out following the manufacturer’s instructions. We previously established
three cell lines (S2013, MiaPaCa2 and AsPC-1) with stable expression of GFP and so do not
anticipate problems with this step.
Orthotopic Transplantation
For cancer xenografts 6-8 week old athymic NMRI nude mice (nu/nu, Charles River,
Suizfeld, Germany) will be bred in the animal facility. The mice will be housed in micro-isolator
cages in a filtered-air laminar flow cabinet (EuroBioConcept, Paris, France), handled under aseptic
conditions and fed with autoclaved laboratory rodent food pellets. Each group will be comprised of
10 animals as determined by power analysis. For the pancreatic cancer cell lines, using a sterile 20
gauge needle, a suspension of 0.25x106 cells in 10μl serum free media will be injected under the
capsule in the pancreas adjacent to duodenum. For the hepatocellular cell lines, similar injections
of 0.25x106 cells in 10μl will be made into the left lobe of the liver. Imaging following transplantation
will confirm that the injections form a discrete tumor without leakage from the injection site. The
length of the experiment will depend on the cell line. For example, xenografts of S2013 cells grow
and metastasize rapidly and end stage disease is reached within 4-5 weeks. For AsPC-1 cells the
course is slower and end-stage disease is reached in 8-9 weeks. Suspensions of chitosan NPs
loaded with 4 mg Metformin and 2 mg Vismodegib will be administered on daily basis. PEGPH20
will be administered at a dose of 4.5 mg/kg i.p. every 3rd day. Gemcitabine will be administered to
the pancreatic cancer animals at a dose of 20 mg/kg i.p. on days 3, 6, 9, 12 and 15, following
tumor implantation. Sorafenib will be administered daily at a dose of 30 mg/kg i.p.
Monitoring Tumor Growth and Metastasis Dynamically and at Autopsy
The Xenogen IVIS Lumina II animal visualization system will be used to monitor cancer
growth and development of metastases. Tumor bearing mice will be monitored every 4th day. Mice
will be isoflurane anesthetized and luciferin (XenoLight Rediject D-Luciferin Ultra, Perkin Elmer)
9/20
CD133, CD24, EPCAM, and CD44 (diluted 1 : 11) at 4◦C for 15 min. After multiple PBS washes,
cells will be analyzed using a FACSC Flow Cytometer (Guava EasyCyte, Millipore).
Possible pitfalls and alternative approaches
None anticipated.
Aim 3: Evaluation of the effectiveness of the combined therapy in vivo
Rationale
We hypothesize that the strategy of combining the CSC-targeted nanoparticles with
PEGLH20 will substantially reduce the metastatic potential of the different cancer cell lines and
also markedly reduce of eradicate the CSCs. The PEGPH20 is a kind gift from Dr. Gregory I Frost,
Halozyme Therapeutics Inc., San Diego, CA, USA. The orthotopic models we have developed are
good mimics of the human disease with metastatic spread, stromal reaction to the tumor, biliary
obstruction and cachexia [43]. The pancreatic and hepatocellular cancer cell lines will be
transfected with a lentivirus to induce stable expression of firefly luciferase. Thus, we are able to
monitor tumor growth and metastasis dynamically, by the use of the IVIS Spectrum animal
visualization system. By use of a separate Rhodamine 123 on the nanoparticles we will be able to
monitor the time-course and effectiveness of the cancer targeting.
Experimental Design
Two human PDAC (S2013, AsPC-1) and two HCC (MHCC-97L, HCC-LM3, or SMMC7721)
cell lines will be used in this study. This cell lines provide time-courses with different therapeutic
windows. For example, with S2103 cells hepatic metastases develop within one week, tumor
burden is marked within one month and the animals are severely cachectic. With AsPC-1 cells the
time-course is about two months.
Preparation of Cancer Cells with Stable Expression of Luciferase
Retroviral particles containing the luciferase gene will be obtained from Perkin Elmer.
These are not currently available, but I assured that they will be available before August 2013.
Transfection will be carried out following the manufacturer’s instructions. We previously established
three cell lines (S2013, MiaPaCa2 and AsPC-1) with stable expression of GFP and so do not
anticipate problems with this step.
Orthotopic Transplantation
For cancer xenografts 6-8 week old athymic NMRI nude mice (nu/nu, Charles River,
Suizfeld, Germany) will be bred in the animal facility. The mice will be housed in micro-isolator
cages in a filtered-air laminar flow cabinet (EuroBioConcept, Paris, France), handled under aseptic
conditions and fed with autoclaved laboratory rodent food pellets. Each group will be comprised of
10 animals as determined by power analysis. For the pancreatic cancer cell lines, using a sterile 20
gauge needle, a suspension of 0.25x106 cells in 10μl serum free media will be injected under the
capsule in the pancreas adjacent to duodenum. For the hepatocellular cell lines, similar injections
of 0.25x106 cells in 10μl will be made into the left lobe of the liver. Imaging following transplantation
will confirm that the injections form a discrete tumor without leakage from the injection site. The
length of the experiment will depend on the cell line. For example, xenografts of S2013 cells grow
and metastasize rapidly and end stage disease is reached within 4-5 weeks. For AsPC-1 cells the
course is slower and end-stage disease is reached in 8-9 weeks. Suspensions of chitosan NPs
loaded with 4 mg Metformin and 2 mg Vismodegib will be administered on daily basis. PEGPH20
will be administered at a dose of 4.5 mg/kg i.p. every 3rd day. Gemcitabine will be administered to
the pancreatic cancer animals at a dose of 20 mg/kg i.p. on days 3, 6, 9, 12 and 15, following
tumor implantation. Sorafenib will be administered daily at a dose of 30 mg/kg i.p.
Monitoring Tumor Growth and Metastasis Dynamically and at Autopsy
The Xenogen IVIS Lumina II animal visualization system will be used to monitor cancer
growth and development of metastases. Tumor bearing mice will be monitored every 4th day. Mice
will be isoflurane anesthetized and luciferin (XenoLight Rediject D-Luciferin Ultra, Perkin Elmer)
9/20
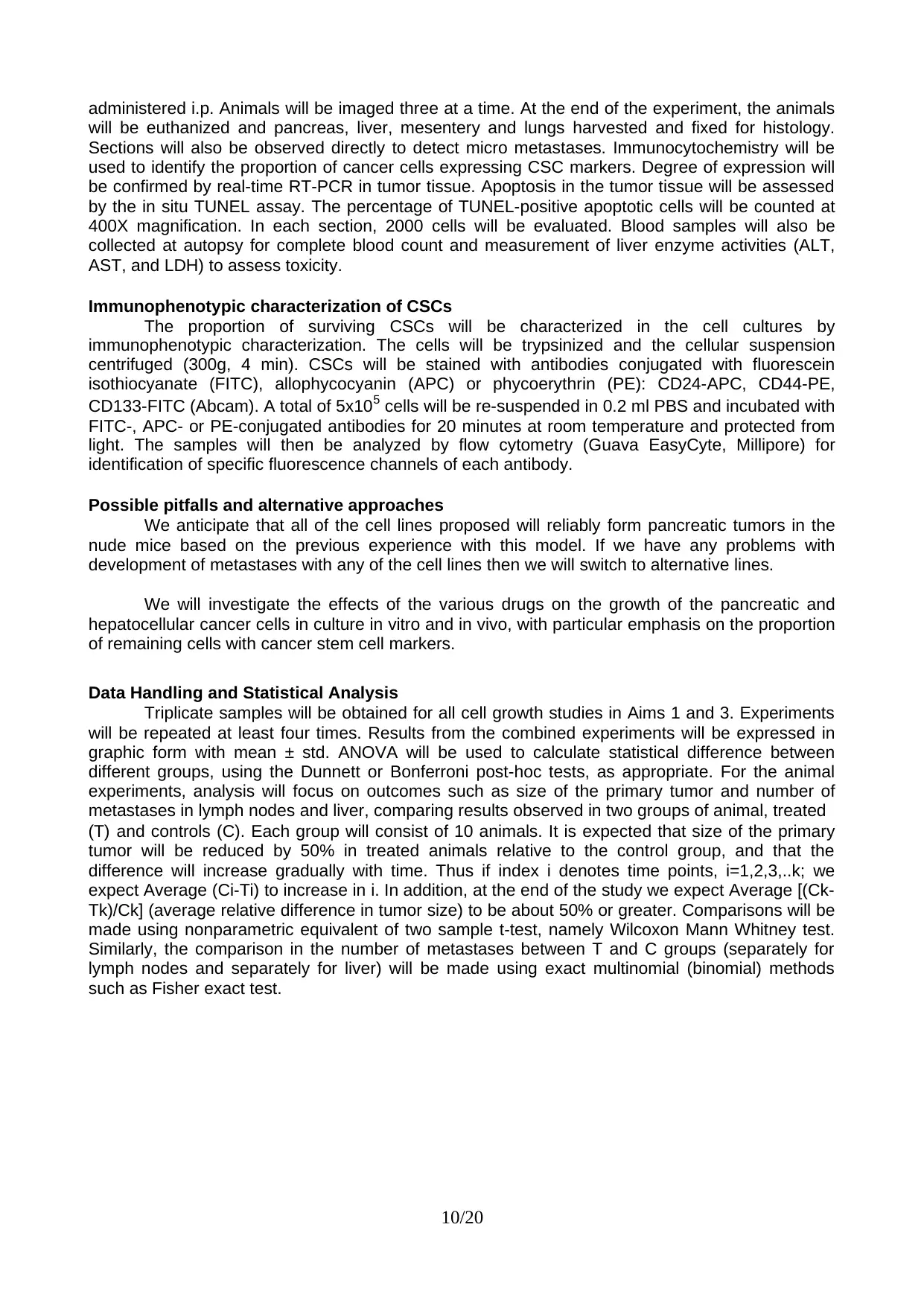
administered i.p. Animals will be imaged three at a time. At the end of the experiment, the animals
will be euthanized and pancreas, liver, mesentery and lungs harvested and fixed for histology.
Sections will also be observed directly to detect micro metastases. Immunocytochemistry will be
used to identify the proportion of cancer cells expressing CSC markers. Degree of expression will
be confirmed by real-time RT-PCR in tumor tissue. Apoptosis in the tumor tissue will be assessed
by the in situ TUNEL assay. The percentage of TUNEL-positive apoptotic cells will be counted at
400X magnification. In each section, 2000 cells will be evaluated. Blood samples will also be
collected at autopsy for complete blood count and measurement of liver enzyme activities (ALT,
AST, and LDH) to assess toxicity.
Immunophenotypic characterization of CSCs
The proportion of surviving CSCs will be characterized in the cell cultures by
immunophenotypic characterization. The cells will be trypsinized and the cellular suspension
centrifuged (300g, 4 min). CSCs will be stained with antibodies conjugated with fluorescein
isothiocyanate (FITC), allophycocyanin (APC) or phycoerythrin (PE): CD24-APC, CD44-PE,
CD133-FITC (Abcam). A total of 5x105 cells will be re-suspended in 0.2 ml PBS and incubated with
FITC-, APC- or PE-conjugated antibodies for 20 minutes at room temperature and protected from
light. The samples will then be analyzed by flow cytometry (Guava EasyCyte, Millipore) for
identification of specific fluorescence channels of each antibody.
Possible pitfalls and alternative approaches
We anticipate that all of the cell lines proposed will reliably form pancreatic tumors in the
nude mice based on the previous experience with this model. If we have any problems with
development of metastases with any of the cell lines then we will switch to alternative lines.
We will investigate the effects of the various drugs on the growth of the pancreatic and
hepatocellular cancer cells in culture in vitro and in vivo, with particular emphasis on the proportion
of remaining cells with cancer stem cell markers.
Data Handling and Statistical Analysis
Triplicate samples will be obtained for all cell growth studies in Aims 1 and 3. Experiments
will be repeated at least four times. Results from the combined experiments will be expressed in
graphic form with mean ± std. ANOVA will be used to calculate statistical difference between
different groups, using the Dunnett or Bonferroni post-hoc tests, as appropriate. For the animal
experiments, analysis will focus on outcomes such as size of the primary tumor and number of
metastases in lymph nodes and liver, comparing results observed in two groups of animal, treated
(T) and controls (C). Each group will consist of 10 animals. It is expected that size of the primary
tumor will be reduced by 50% in treated animals relative to the control group, and that the
difference will increase gradually with time. Thus if index i denotes time points, i=1,2,3,..k; we
expect Average (Ci-Ti) to increase in i. In addition, at the end of the study we expect Average [(Ck-
Tk)/Ck] (average relative difference in tumor size) to be about 50% or greater. Comparisons will be
made using nonparametric equivalent of two sample t-test, namely Wilcoxon Mann Whitney test.
Similarly, the comparison in the number of metastases between T and C groups (separately for
lymph nodes and separately for liver) will be made using exact multinomial (binomial) methods
such as Fisher exact test.
10/20
will be euthanized and pancreas, liver, mesentery and lungs harvested and fixed for histology.
Sections will also be observed directly to detect micro metastases. Immunocytochemistry will be
used to identify the proportion of cancer cells expressing CSC markers. Degree of expression will
be confirmed by real-time RT-PCR in tumor tissue. Apoptosis in the tumor tissue will be assessed
by the in situ TUNEL assay. The percentage of TUNEL-positive apoptotic cells will be counted at
400X magnification. In each section, 2000 cells will be evaluated. Blood samples will also be
collected at autopsy for complete blood count and measurement of liver enzyme activities (ALT,
AST, and LDH) to assess toxicity.
Immunophenotypic characterization of CSCs
The proportion of surviving CSCs will be characterized in the cell cultures by
immunophenotypic characterization. The cells will be trypsinized and the cellular suspension
centrifuged (300g, 4 min). CSCs will be stained with antibodies conjugated with fluorescein
isothiocyanate (FITC), allophycocyanin (APC) or phycoerythrin (PE): CD24-APC, CD44-PE,
CD133-FITC (Abcam). A total of 5x105 cells will be re-suspended in 0.2 ml PBS and incubated with
FITC-, APC- or PE-conjugated antibodies for 20 minutes at room temperature and protected from
light. The samples will then be analyzed by flow cytometry (Guava EasyCyte, Millipore) for
identification of specific fluorescence channels of each antibody.
Possible pitfalls and alternative approaches
We anticipate that all of the cell lines proposed will reliably form pancreatic tumors in the
nude mice based on the previous experience with this model. If we have any problems with
development of metastases with any of the cell lines then we will switch to alternative lines.
We will investigate the effects of the various drugs on the growth of the pancreatic and
hepatocellular cancer cells in culture in vitro and in vivo, with particular emphasis on the proportion
of remaining cells with cancer stem cell markers.
Data Handling and Statistical Analysis
Triplicate samples will be obtained for all cell growth studies in Aims 1 and 3. Experiments
will be repeated at least four times. Results from the combined experiments will be expressed in
graphic form with mean ± std. ANOVA will be used to calculate statistical difference between
different groups, using the Dunnett or Bonferroni post-hoc tests, as appropriate. For the animal
experiments, analysis will focus on outcomes such as size of the primary tumor and number of
metastases in lymph nodes and liver, comparing results observed in two groups of animal, treated
(T) and controls (C). Each group will consist of 10 animals. It is expected that size of the primary
tumor will be reduced by 50% in treated animals relative to the control group, and that the
difference will increase gradually with time. Thus if index i denotes time points, i=1,2,3,..k; we
expect Average (Ci-Ti) to increase in i. In addition, at the end of the study we expect Average [(Ck-
Tk)/Ck] (average relative difference in tumor size) to be about 50% or greater. Comparisons will be
made using nonparametric equivalent of two sample t-test, namely Wilcoxon Mann Whitney test.
Similarly, the comparison in the number of metastases between T and C groups (separately for
lymph nodes and separately for liver) will be made using exact multinomial (binomial) methods
such as Fisher exact test.
10/20
Secure Best Marks with AI Grader
Need help grading? Try our AI Grader for instant feedback on your assignments.
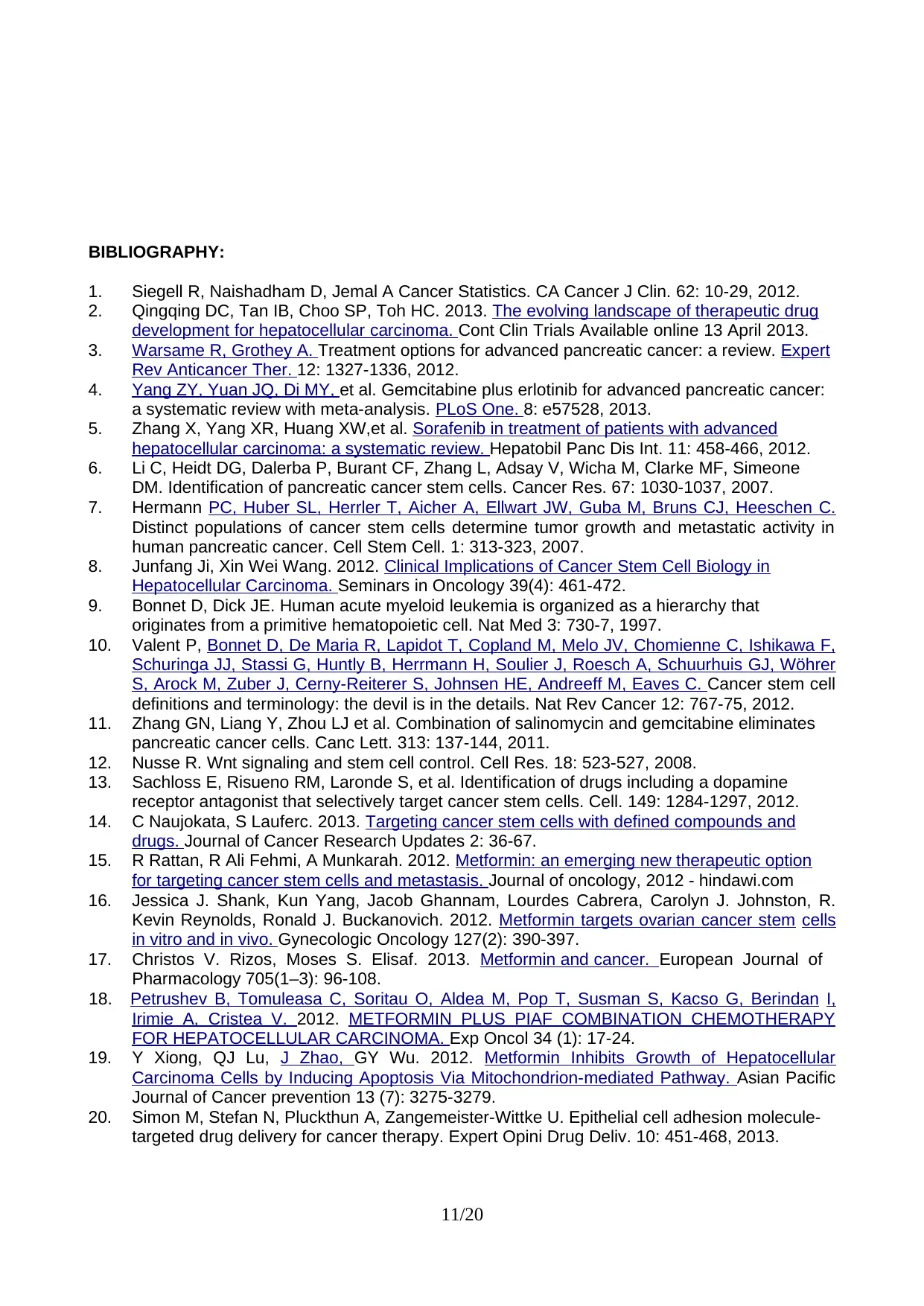
BIBLIOGRAPHY:
1. Siegell R, Naishadham D, Jemal A Cancer Statistics. CA Cancer J Clin. 62: 10-29, 2012.
2. Qingqing DC, Tan IB, Choo SP, Toh HC. 2013. The evolving landscape of therapeutic drug
development for hepatocellular carcinoma. Cont Clin Trials Available online 13 April 2013.
3. Warsame R, Grothey A. Treatment options for advanced pancreatic cancer: a review. Expert
Rev Anticancer Ther. 12: 1327-1336, 2012.
4. Yang ZY, Yuan JQ, Di MY, et al. Gemcitabine plus erlotinib for advanced pancreatic cancer:
a systematic review with meta-analysis. PLoS One. 8: e57528, 2013.
5. Zhang X, Yang XR, Huang XW,et al. Sorafenib in treatment of patients with advanced
hepatocellular carcinoma: a systematic review. Hepatobil Panc Dis Int. 11: 458-466, 2012.
6. Li C, Heidt DG, Dalerba P, Burant CF, Zhang L, Adsay V, Wicha M, Clarke MF, Simeone
DM. Identification of pancreatic cancer stem cells. Cancer Res. 67: 1030-1037, 2007.
7. Hermann PC, Huber SL, Herrler T, Aicher A, Ellwart JW, Guba M, Bruns CJ, Heeschen C.
Distinct populations of cancer stem cells determine tumor growth and metastatic activity in
human pancreatic cancer. Cell Stem Cell. 1: 313-323, 2007.
8. Junfang Ji, Xin Wei Wang. 2012. Clinical Implications of Cancer Stem Cell Biology in
Hepatocellular Carcinoma. Seminars in Oncology 39(4): 461-472.
9. Bonnet D, Dick JE. Human acute myeloid leukemia is organized as a hierarchy that
originates from a primitive hematopoietic cell. Nat Med 3: 730-7, 1997.
10. Valent P, Bonnet D, De Maria R, Lapidot T, Copland M, Melo JV, Chomienne C, Ishikawa F,
Schuringa JJ, Stassi G, Huntly B, Herrmann H, Soulier J, Roesch A, Schuurhuis GJ, Wöhrer
S, Arock M, Zuber J, Cerny-Reiterer S, Johnsen HE, Andreeff M, Eaves C. Cancer stem cell
definitions and terminology: the devil is in the details. Nat Rev Cancer 12: 767-75, 2012.
11. Zhang GN, Liang Y, Zhou LJ et al. Combination of salinomycin and gemcitabine eliminates
pancreatic cancer cells. Canc Lett. 313: 137-144, 2011.
12. Nusse R. Wnt signaling and stem cell control. Cell Res. 18: 523-527, 2008.
13. Sachloss E, Risueno RM, Laronde S, et al. Identification of drugs including a dopamine
receptor antagonist that selectively target cancer stem cells. Cell. 149: 1284-1297, 2012.
14. C Naujokata, S Lauferc. 2013. Targeting cancer stem cells with defined compounds and
drugs. Journal of Cancer Research Updates 2: 36-67.
15. R Rattan, R Ali Fehmi, A Munkarah. 2012. Metformin: an emerging new therapeutic option
for targeting cancer stem cells and metastasis. Journal of oncology, 2012 - hindawi.com
16. Jessica J. Shank, Kun Yang, Jacob Ghannam, Lourdes Cabrera, Carolyn J. Johnston, R.
Kevin Reynolds, Ronald J. Buckanovich. 2012. Metformin targets ovarian cancer stem cells
in vitro and in vivo. Gynecologic Oncology 127(2): 390-397.
17. Christos V. Rizos, Moses S. Elisaf. 2013. Metformin and cancer. European Journal of
Pharmacology 705(1–3): 96-108.
18. Petrushev B, Tomuleasa C, Soritau O, Aldea M, Pop T, Susman S, Kacso G, Berindan I,
Irimie A, Cristea V. 2012. METFORMIN PLUS PIAF COMBINATION CHEMOTHERAPY
FOR HEPATOCELLULAR CARCINOMA. Exp Oncol 34 (1): 17-24.
19. Y Xiong, QJ Lu, J Zhao, GY Wu. 2012. Metformin Inhibits Growth of Hepatocellular
Carcinoma Cells by Inducing Apoptosis Via Mitochondrion-mediated Pathway. Asian Pacific
Journal of Cancer prevention 13 (7): 3275-3279.
20. Simon M, Stefan N, Pluckthun A, Zangemeister-Wittke U. Epithelial cell adhesion molecule-
targeted drug delivery for cancer therapy. Expert Opini Drug Deliv. 10: 451-468, 2013.
11/20
1. Siegell R, Naishadham D, Jemal A Cancer Statistics. CA Cancer J Clin. 62: 10-29, 2012.
2. Qingqing DC, Tan IB, Choo SP, Toh HC. 2013. The evolving landscape of therapeutic drug
development for hepatocellular carcinoma. Cont Clin Trials Available online 13 April 2013.
3. Warsame R, Grothey A. Treatment options for advanced pancreatic cancer: a review. Expert
Rev Anticancer Ther. 12: 1327-1336, 2012.
4. Yang ZY, Yuan JQ, Di MY, et al. Gemcitabine plus erlotinib for advanced pancreatic cancer:
a systematic review with meta-analysis. PLoS One. 8: e57528, 2013.
5. Zhang X, Yang XR, Huang XW,et al. Sorafenib in treatment of patients with advanced
hepatocellular carcinoma: a systematic review. Hepatobil Panc Dis Int. 11: 458-466, 2012.
6. Li C, Heidt DG, Dalerba P, Burant CF, Zhang L, Adsay V, Wicha M, Clarke MF, Simeone
DM. Identification of pancreatic cancer stem cells. Cancer Res. 67: 1030-1037, 2007.
7. Hermann PC, Huber SL, Herrler T, Aicher A, Ellwart JW, Guba M, Bruns CJ, Heeschen C.
Distinct populations of cancer stem cells determine tumor growth and metastatic activity in
human pancreatic cancer. Cell Stem Cell. 1: 313-323, 2007.
8. Junfang Ji, Xin Wei Wang. 2012. Clinical Implications of Cancer Stem Cell Biology in
Hepatocellular Carcinoma. Seminars in Oncology 39(4): 461-472.
9. Bonnet D, Dick JE. Human acute myeloid leukemia is organized as a hierarchy that
originates from a primitive hematopoietic cell. Nat Med 3: 730-7, 1997.
10. Valent P, Bonnet D, De Maria R, Lapidot T, Copland M, Melo JV, Chomienne C, Ishikawa F,
Schuringa JJ, Stassi G, Huntly B, Herrmann H, Soulier J, Roesch A, Schuurhuis GJ, Wöhrer
S, Arock M, Zuber J, Cerny-Reiterer S, Johnsen HE, Andreeff M, Eaves C. Cancer stem cell
definitions and terminology: the devil is in the details. Nat Rev Cancer 12: 767-75, 2012.
11. Zhang GN, Liang Y, Zhou LJ et al. Combination of salinomycin and gemcitabine eliminates
pancreatic cancer cells. Canc Lett. 313: 137-144, 2011.
12. Nusse R. Wnt signaling and stem cell control. Cell Res. 18: 523-527, 2008.
13. Sachloss E, Risueno RM, Laronde S, et al. Identification of drugs including a dopamine
receptor antagonist that selectively target cancer stem cells. Cell. 149: 1284-1297, 2012.
14. C Naujokata, S Lauferc. 2013. Targeting cancer stem cells with defined compounds and
drugs. Journal of Cancer Research Updates 2: 36-67.
15. R Rattan, R Ali Fehmi, A Munkarah. 2012. Metformin: an emerging new therapeutic option
for targeting cancer stem cells and metastasis. Journal of oncology, 2012 - hindawi.com
16. Jessica J. Shank, Kun Yang, Jacob Ghannam, Lourdes Cabrera, Carolyn J. Johnston, R.
Kevin Reynolds, Ronald J. Buckanovich. 2012. Metformin targets ovarian cancer stem cells
in vitro and in vivo. Gynecologic Oncology 127(2): 390-397.
17. Christos V. Rizos, Moses S. Elisaf. 2013. Metformin and cancer. European Journal of
Pharmacology 705(1–3): 96-108.
18. Petrushev B, Tomuleasa C, Soritau O, Aldea M, Pop T, Susman S, Kacso G, Berindan I,
Irimie A, Cristea V. 2012. METFORMIN PLUS PIAF COMBINATION CHEMOTHERAPY
FOR HEPATOCELLULAR CARCINOMA. Exp Oncol 34 (1): 17-24.
19. Y Xiong, QJ Lu, J Zhao, GY Wu. 2012. Metformin Inhibits Growth of Hepatocellular
Carcinoma Cells by Inducing Apoptosis Via Mitochondrion-mediated Pathway. Asian Pacific
Journal of Cancer prevention 13 (7): 3275-3279.
20. Simon M, Stefan N, Pluckthun A, Zangemeister-Wittke U. Epithelial cell adhesion molecule-
targeted drug delivery for cancer therapy. Expert Opini Drug Deliv. 10: 451-468, 2013.
11/20
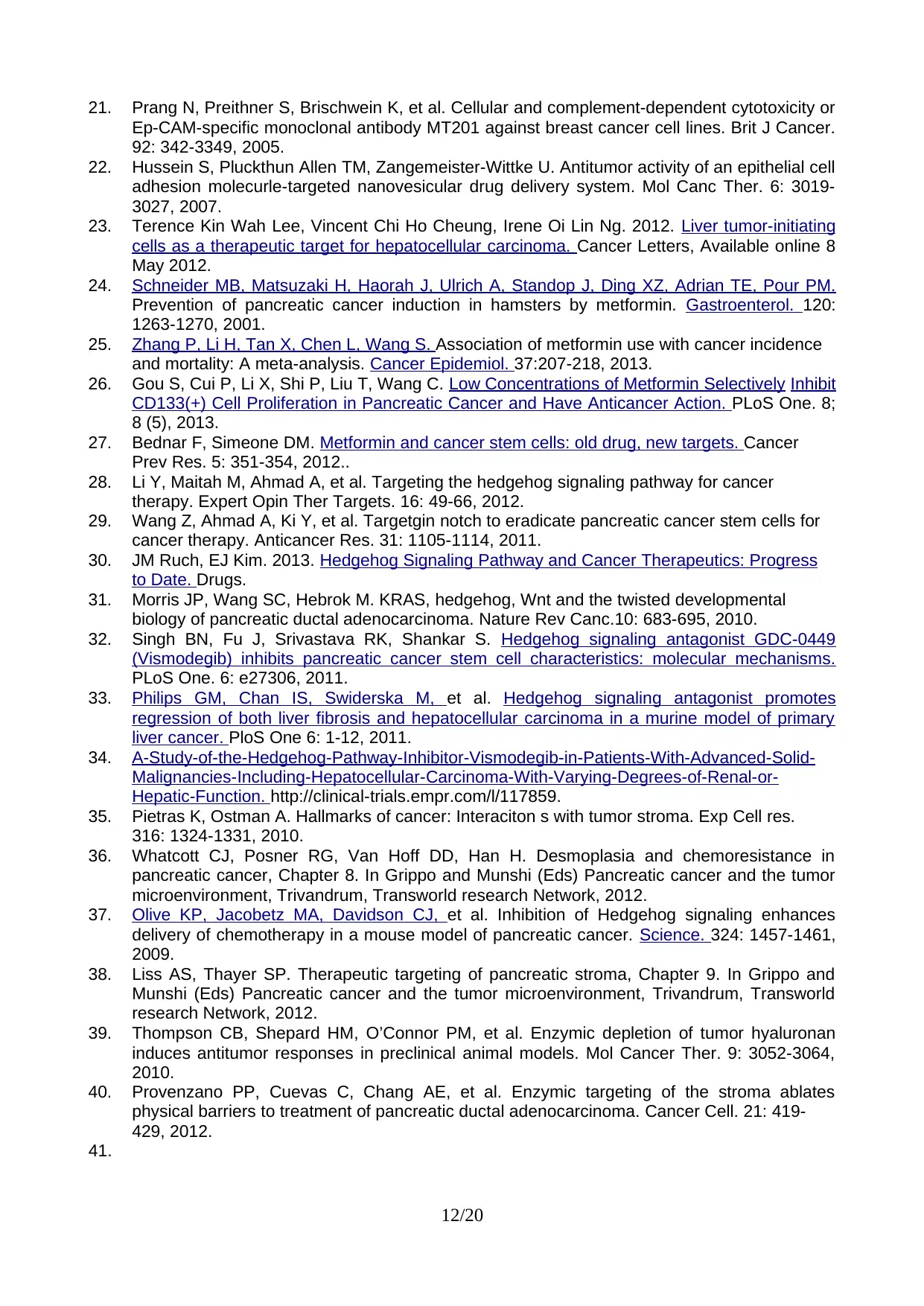
21. Prang N, Preithner S, Brischwein K, et al. Cellular and complement-dependent cytotoxicity or
Ep-CAM-specific monoclonal antibody MT201 against breast cancer cell lines. Brit J Cancer.
92: 342-3349, 2005.
22. Hussein S, Pluckthun Allen TM, Zangemeister-Wittke U. Antitumor activity of an epithelial cell
adhesion molecurle-targeted nanovesicular drug delivery system. Mol Canc Ther. 6: 3019-
3027, 2007.
23. Terence Kin Wah Lee, Vincent Chi Ho Cheung, Irene Oi Lin Ng. 2012. Liver tumor-initiating
cells as a therapeutic target for hepatocellular carcinoma. Cancer Letters, Available online 8
May 2012.
24. Schneider MB, Matsuzaki H, Haorah J, Ulrich A, Standop J, Ding XZ, Adrian TE, Pour PM.
Prevention of pancreatic cancer induction in hamsters by metformin. Gastroenterol. 120:
1263-1270, 2001.
25. Zhang P, Li H, Tan X, Chen L, Wang S. Association of metformin use with cancer incidence
and mortality: A meta-analysis. Cancer Epidemiol. 37:207-218, 2013.
26. Gou S, Cui P, Li X, Shi P, Liu T, Wang C. Low Concentrations of Metformin Selectively Inhibit
CD133(+) Cell Proliferation in Pancreatic Cancer and Have Anticancer Action. PLoS One. 8;
8 (5), 2013.
27. Bednar F, Simeone DM. Metformin and cancer stem cells: old drug, new targets. Cancer
Prev Res. 5: 351-354, 2012..
28. Li Y, Maitah M, Ahmad A, et al. Targeting the hedgehog signaling pathway for cancer
therapy. Expert Opin Ther Targets. 16: 49-66, 2012.
29. Wang Z, Ahmad A, Ki Y, et al. Targetgin notch to eradicate pancreatic cancer stem cells for
cancer therapy. Anticancer Res. 31: 1105-1114, 2011.
30. JM Ruch, EJ Kim. 2013. Hedgehog Signaling Pathway and Cancer Therapeutics: Progress
to Date. Drugs.
31. Morris JP, Wang SC, Hebrok M. KRAS, hedgehog, Wnt and the twisted developmental
biology of pancreatic ductal adenocarcinoma. Nature Rev Canc.10: 683-695, 2010.
32. Singh BN, Fu J, Srivastava RK, Shankar S. Hedgehog signaling antagonist GDC-0449
(Vismodegib) inhibits pancreatic cancer stem cell characteristics: molecular mechanisms.
PLoS One. 6: e27306, 2011.
33. Philips GM, Chan IS, Swiderska M, et al. Hedgehog signaling antagonist promotes
regression of both liver fibrosis and hepatocellular carcinoma in a murine model of primary
liver cancer. PloS One 6: 1-12, 2011.
34. A-Study-of-the-Hedgehog-Pathway-Inhibitor-Vismodegib-in-Patients-With-Advanced-Solid-
Malignancies-Including-Hepatocellular-Carcinoma-With-Varying-Degrees-of-Renal-or-
Hepatic-Function. http://clinical-trials.empr.com/l/117859.
35. Pietras K, Ostman A. Hallmarks of cancer: Interaciton s with tumor stroma. Exp Cell res.
316: 1324-1331, 2010.
36. Whatcott CJ, Posner RG, Van Hoff DD, Han H. Desmoplasia and chemoresistance in
pancreatic cancer, Chapter 8. In Grippo and Munshi (Eds) Pancreatic cancer and the tumor
microenvironment, Trivandrum, Transworld research Network, 2012.
37. Olive KP, Jacobetz MA, Davidson CJ, et al. Inhibition of Hedgehog signaling enhances
delivery of chemotherapy in a mouse model of pancreatic cancer. Science. 324: 1457-1461,
2009.
38. Liss AS, Thayer SP. Therapeutic targeting of pancreatic stroma, Chapter 9. In Grippo and
Munshi (Eds) Pancreatic cancer and the tumor microenvironment, Trivandrum, Transworld
research Network, 2012.
39. Thompson CB, Shepard HM, O’Connor PM, et al. Enzymic depletion of tumor hyaluronan
induces antitumor responses in preclinical animal models. Mol Cancer Ther. 9: 3052-3064,
2010.
40. Provenzano PP, Cuevas C, Chang AE, et al. Enzymic targeting of the stroma ablates
physical barriers to treatment of pancreatic ductal adenocarcinoma. Cancer Cell. 21: 419-
429, 2012.
41.
12/20
Ep-CAM-specific monoclonal antibody MT201 against breast cancer cell lines. Brit J Cancer.
92: 342-3349, 2005.
22. Hussein S, Pluckthun Allen TM, Zangemeister-Wittke U. Antitumor activity of an epithelial cell
adhesion molecurle-targeted nanovesicular drug delivery system. Mol Canc Ther. 6: 3019-
3027, 2007.
23. Terence Kin Wah Lee, Vincent Chi Ho Cheung, Irene Oi Lin Ng. 2012. Liver tumor-initiating
cells as a therapeutic target for hepatocellular carcinoma. Cancer Letters, Available online 8
May 2012.
24. Schneider MB, Matsuzaki H, Haorah J, Ulrich A, Standop J, Ding XZ, Adrian TE, Pour PM.
Prevention of pancreatic cancer induction in hamsters by metformin. Gastroenterol. 120:
1263-1270, 2001.
25. Zhang P, Li H, Tan X, Chen L, Wang S. Association of metformin use with cancer incidence
and mortality: A meta-analysis. Cancer Epidemiol. 37:207-218, 2013.
26. Gou S, Cui P, Li X, Shi P, Liu T, Wang C. Low Concentrations of Metformin Selectively Inhibit
CD133(+) Cell Proliferation in Pancreatic Cancer and Have Anticancer Action. PLoS One. 8;
8 (5), 2013.
27. Bednar F, Simeone DM. Metformin and cancer stem cells: old drug, new targets. Cancer
Prev Res. 5: 351-354, 2012..
28. Li Y, Maitah M, Ahmad A, et al. Targeting the hedgehog signaling pathway for cancer
therapy. Expert Opin Ther Targets. 16: 49-66, 2012.
29. Wang Z, Ahmad A, Ki Y, et al. Targetgin notch to eradicate pancreatic cancer stem cells for
cancer therapy. Anticancer Res. 31: 1105-1114, 2011.
30. JM Ruch, EJ Kim. 2013. Hedgehog Signaling Pathway and Cancer Therapeutics: Progress
to Date. Drugs.
31. Morris JP, Wang SC, Hebrok M. KRAS, hedgehog, Wnt and the twisted developmental
biology of pancreatic ductal adenocarcinoma. Nature Rev Canc.10: 683-695, 2010.
32. Singh BN, Fu J, Srivastava RK, Shankar S. Hedgehog signaling antagonist GDC-0449
(Vismodegib) inhibits pancreatic cancer stem cell characteristics: molecular mechanisms.
PLoS One. 6: e27306, 2011.
33. Philips GM, Chan IS, Swiderska M, et al. Hedgehog signaling antagonist promotes
regression of both liver fibrosis and hepatocellular carcinoma in a murine model of primary
liver cancer. PloS One 6: 1-12, 2011.
34. A-Study-of-the-Hedgehog-Pathway-Inhibitor-Vismodegib-in-Patients-With-Advanced-Solid-
Malignancies-Including-Hepatocellular-Carcinoma-With-Varying-Degrees-of-Renal-or-
Hepatic-Function. http://clinical-trials.empr.com/l/117859.
35. Pietras K, Ostman A. Hallmarks of cancer: Interaciton s with tumor stroma. Exp Cell res.
316: 1324-1331, 2010.
36. Whatcott CJ, Posner RG, Van Hoff DD, Han H. Desmoplasia and chemoresistance in
pancreatic cancer, Chapter 8. In Grippo and Munshi (Eds) Pancreatic cancer and the tumor
microenvironment, Trivandrum, Transworld research Network, 2012.
37. Olive KP, Jacobetz MA, Davidson CJ, et al. Inhibition of Hedgehog signaling enhances
delivery of chemotherapy in a mouse model of pancreatic cancer. Science. 324: 1457-1461,
2009.
38. Liss AS, Thayer SP. Therapeutic targeting of pancreatic stroma, Chapter 9. In Grippo and
Munshi (Eds) Pancreatic cancer and the tumor microenvironment, Trivandrum, Transworld
research Network, 2012.
39. Thompson CB, Shepard HM, O’Connor PM, et al. Enzymic depletion of tumor hyaluronan
induces antitumor responses in preclinical animal models. Mol Cancer Ther. 9: 3052-3064,
2010.
40. Provenzano PP, Cuevas C, Chang AE, et al. Enzymic targeting of the stroma ablates
physical barriers to treatment of pancreatic ductal adenocarcinoma. Cancer Cell. 21: 419-
429, 2012.
41.
12/20
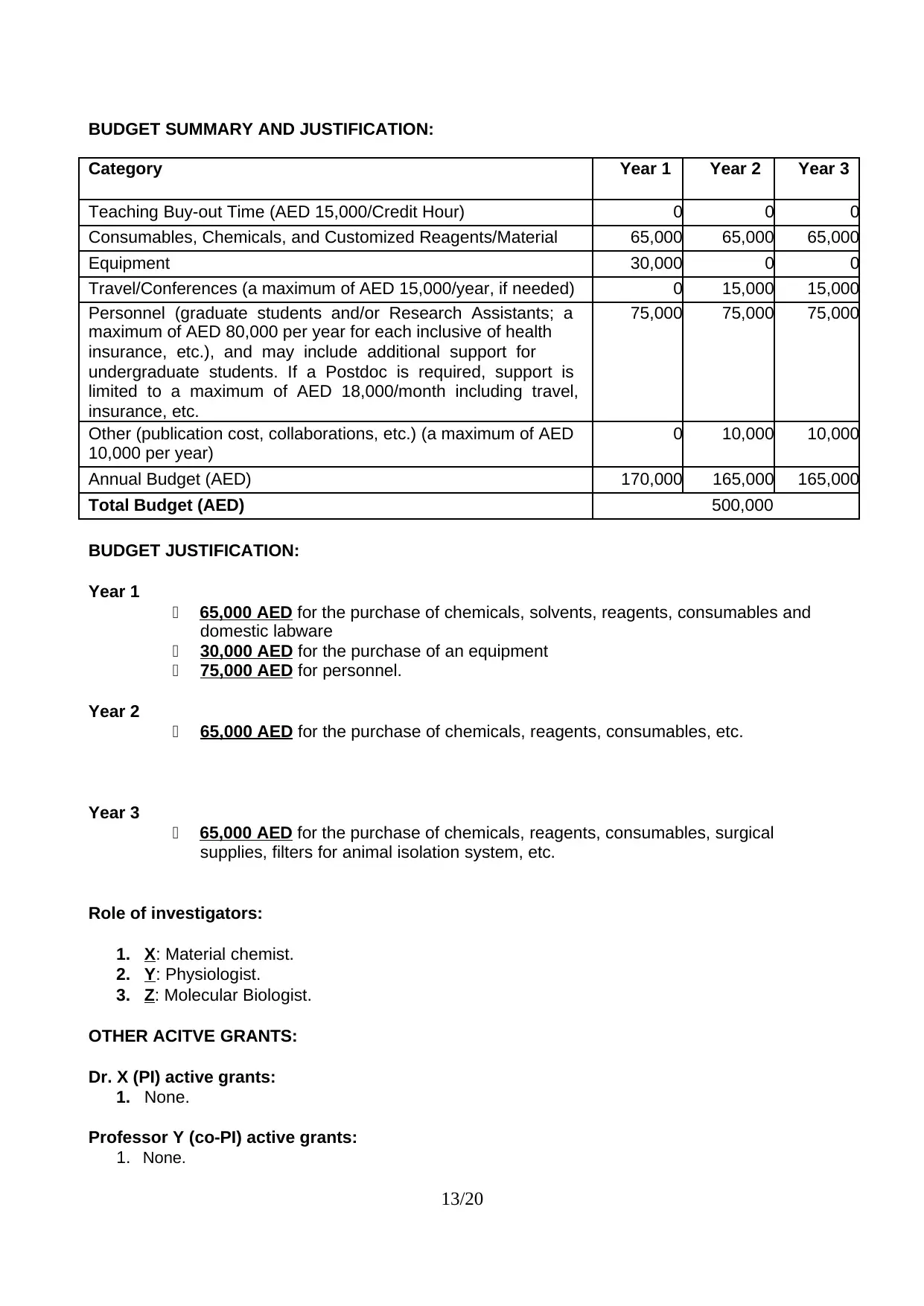
BUDGET SUMMARY AND JUSTIFICATION:
Category Year 1 Year 2 Year 3
Teaching Buy-out Time (AED 15,000/Credit Hour) 0 0 0
Consumables, Chemicals, and Customized Reagents/Material 65,000 65,000 65,000
Equipment 30,000 0 0
Travel/Conferences (a maximum of AED 15,000/year, if needed) 0 15,000 15,000
Personnel (graduate students and/or Research Assistants; a 75,000 75,000 75,000
maximum of AED 80,000 per year for each inclusive of health
insurance, etc.), and may include additional support for
undergraduate students. If a Postdoc is required, support is
limited to a maximum of AED 18,000/month including travel,
insurance, etc.
Other (publication cost, collaborations, etc.) (a maximum of AED 0 10,000 10,000
10,000 per year)
Annual Budget (AED) 170,000 165,000 165,000
Total Budget (AED) 500,000
BUDGET JUSTIFICATION:
Year 1
65,000 AED for the purchase of chemicals, solvents, reagents, consumables and
domestic labware
30,000 AED for the purchase of an equipment
75,000 AED for personnel.
Year 2
65,000 AED for the purchase of chemicals, reagents, consumables, etc.
Year 3
65,000 AED for the purchase of chemicals, reagents, consumables, surgical
supplies, filters for animal isolation system, etc.
Role of investigators:
1. X: Material chemist.
2. Y: Physiologist.
3. Z: Molecular Biologist.
OTHER ACITVE GRANTS:
Dr. X (PI) active grants:
1. None.
Professor Y (co-PI) active grants:
1. None.
13/20
Category Year 1 Year 2 Year 3
Teaching Buy-out Time (AED 15,000/Credit Hour) 0 0 0
Consumables, Chemicals, and Customized Reagents/Material 65,000 65,000 65,000
Equipment 30,000 0 0
Travel/Conferences (a maximum of AED 15,000/year, if needed) 0 15,000 15,000
Personnel (graduate students and/or Research Assistants; a 75,000 75,000 75,000
maximum of AED 80,000 per year for each inclusive of health
insurance, etc.), and may include additional support for
undergraduate students. If a Postdoc is required, support is
limited to a maximum of AED 18,000/month including travel,
insurance, etc.
Other (publication cost, collaborations, etc.) (a maximum of AED 0 10,000 10,000
10,000 per year)
Annual Budget (AED) 170,000 165,000 165,000
Total Budget (AED) 500,000
BUDGET JUSTIFICATION:
Year 1
65,000 AED for the purchase of chemicals, solvents, reagents, consumables and
domestic labware
30,000 AED for the purchase of an equipment
75,000 AED for personnel.
Year 2
65,000 AED for the purchase of chemicals, reagents, consumables, etc.
Year 3
65,000 AED for the purchase of chemicals, reagents, consumables, surgical
supplies, filters for animal isolation system, etc.
Role of investigators:
1. X: Material chemist.
2. Y: Physiologist.
3. Z: Molecular Biologist.
OTHER ACITVE GRANTS:
Dr. X (PI) active grants:
1. None.
Professor Y (co-PI) active grants:
1. None.
13/20
Paraphrase This Document
Need a fresh take? Get an instant paraphrase of this document with our AI Paraphraser
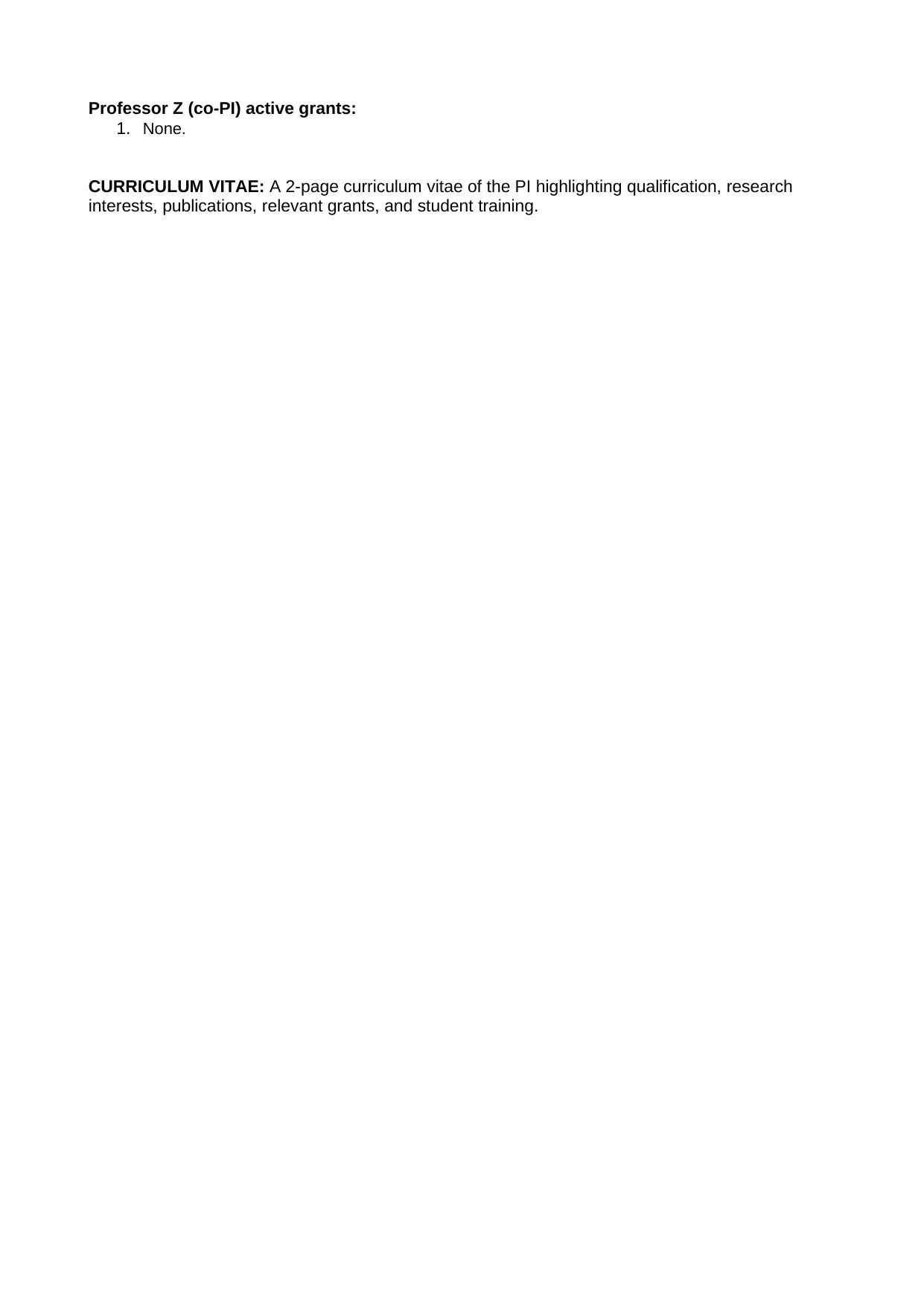
Professor Z (co-PI) active grants:
1. None.
CURRICULUM VITAE: A 2-page curriculum vitae of the PI highlighting qualification, research
interests, publications, relevant grants, and student training.
1. None.
CURRICULUM VITAE: A 2-page curriculum vitae of the PI highlighting qualification, research
interests, publications, relevant grants, and student training.
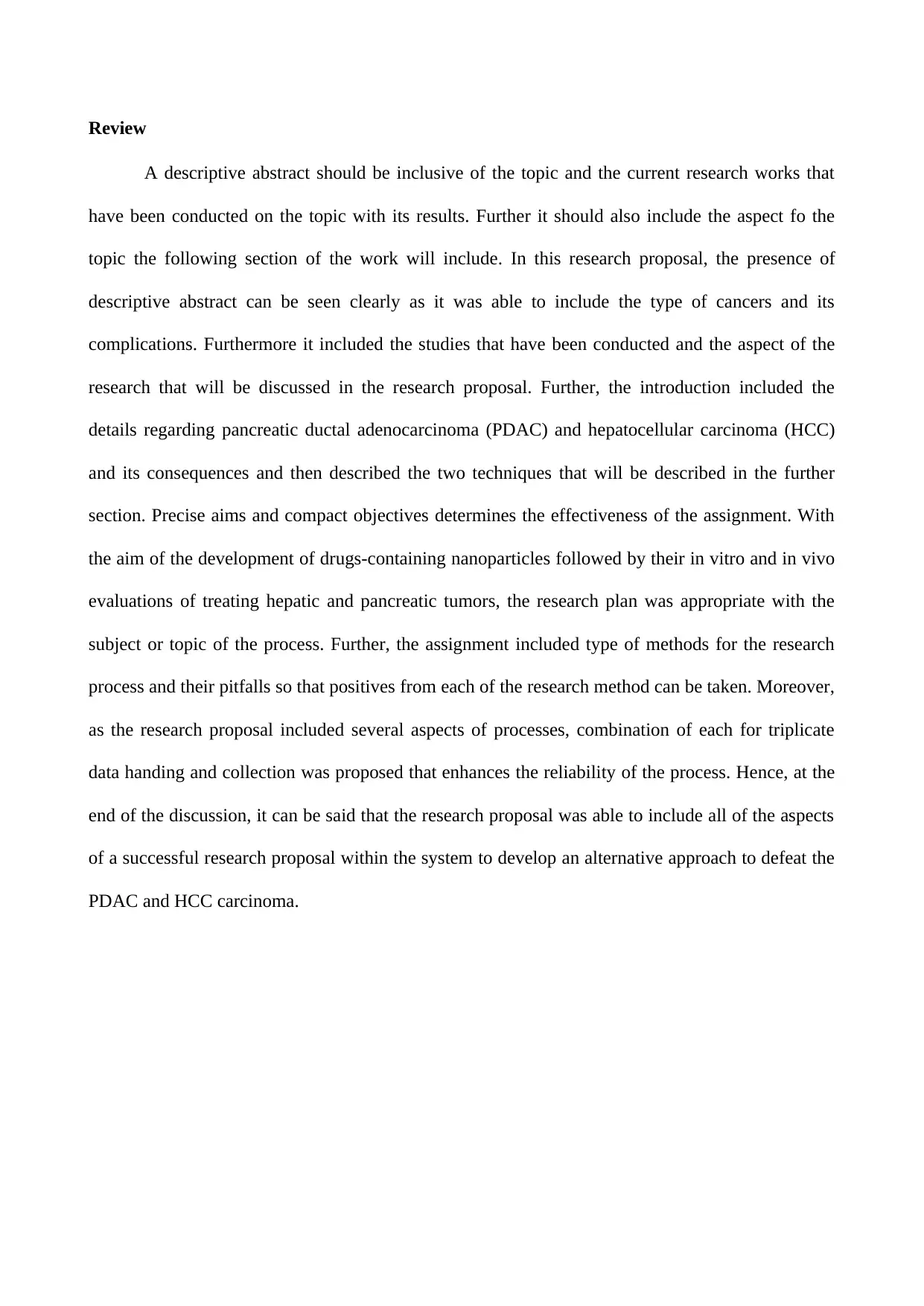
Review
A descriptive abstract should be inclusive of the topic and the current research works that
have been conducted on the topic with its results. Further it should also include the aspect fo the
topic the following section of the work will include. In this research proposal, the presence of
descriptive abstract can be seen clearly as it was able to include the type of cancers and its
complications. Furthermore it included the studies that have been conducted and the aspect of the
research that will be discussed in the research proposal. Further, the introduction included the
details regarding pancreatic ductal adenocarcinoma (PDAC) and hepatocellular carcinoma (HCC)
and its consequences and then described the two techniques that will be described in the further
section. Precise aims and compact objectives determines the effectiveness of the assignment. With
the aim of the development of drugs-containing nanoparticles followed by their in vitro and in vivo
evaluations of treating hepatic and pancreatic tumors, the research plan was appropriate with the
subject or topic of the process. Further, the assignment included type of methods for the research
process and their pitfalls so that positives from each of the research method can be taken. Moreover,
as the research proposal included several aspects of processes, combination of each for triplicate
data handing and collection was proposed that enhances the reliability of the process. Hence, at the
end of the discussion, it can be said that the research proposal was able to include all of the aspects
of a successful research proposal within the system to develop an alternative approach to defeat the
PDAC and HCC carcinoma.
A descriptive abstract should be inclusive of the topic and the current research works that
have been conducted on the topic with its results. Further it should also include the aspect fo the
topic the following section of the work will include. In this research proposal, the presence of
descriptive abstract can be seen clearly as it was able to include the type of cancers and its
complications. Furthermore it included the studies that have been conducted and the aspect of the
research that will be discussed in the research proposal. Further, the introduction included the
details regarding pancreatic ductal adenocarcinoma (PDAC) and hepatocellular carcinoma (HCC)
and its consequences and then described the two techniques that will be described in the further
section. Precise aims and compact objectives determines the effectiveness of the assignment. With
the aim of the development of drugs-containing nanoparticles followed by their in vitro and in vivo
evaluations of treating hepatic and pancreatic tumors, the research plan was appropriate with the
subject or topic of the process. Further, the assignment included type of methods for the research
process and their pitfalls so that positives from each of the research method can be taken. Moreover,
as the research proposal included several aspects of processes, combination of each for triplicate
data handing and collection was proposed that enhances the reliability of the process. Hence, at the
end of the discussion, it can be said that the research proposal was able to include all of the aspects
of a successful research proposal within the system to develop an alternative approach to defeat the
PDAC and HCC carcinoma.
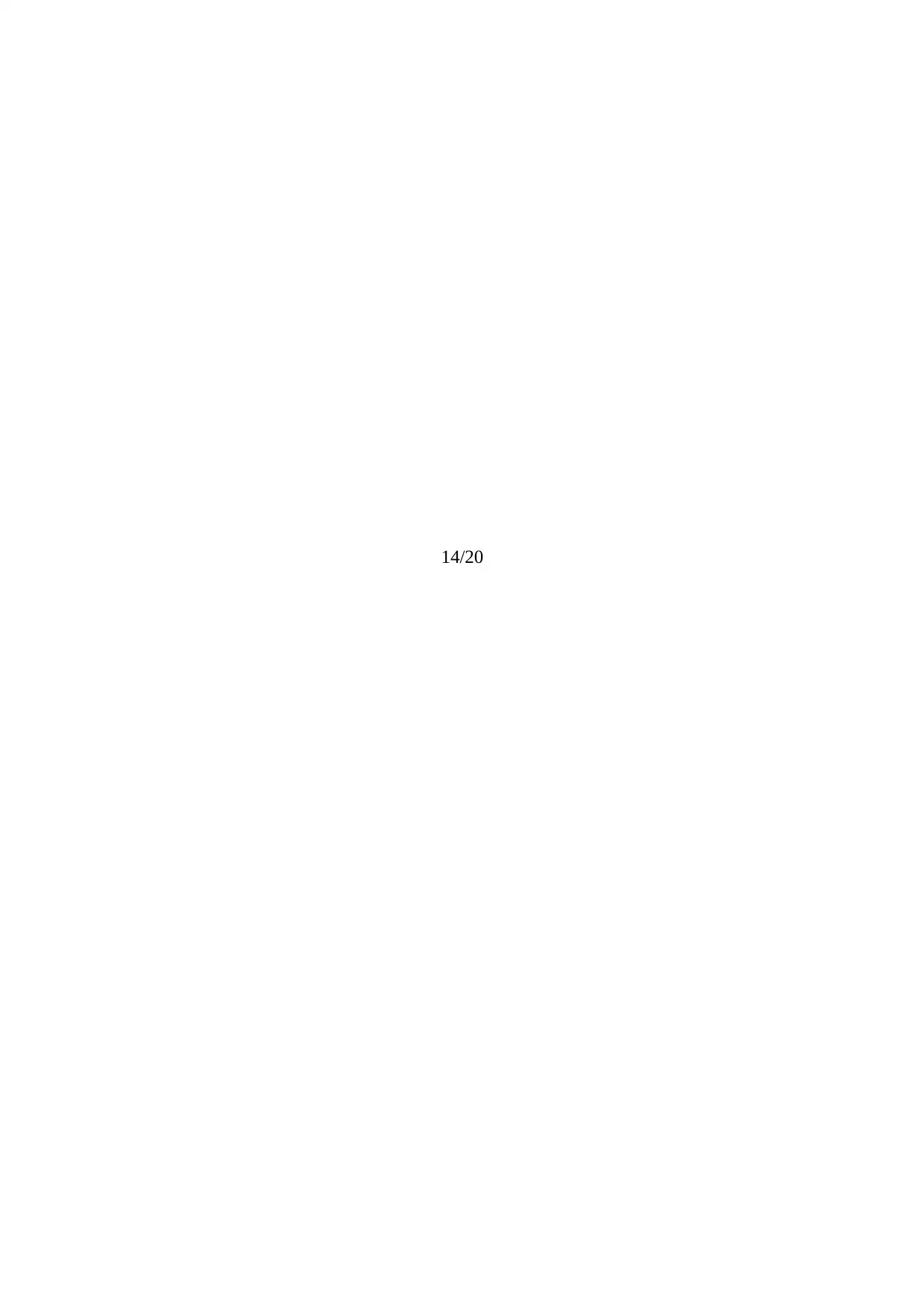
14/20
1 out of 16
![[object Object]](/_next/image/?url=%2F_next%2Fstatic%2Fmedia%2Flogo.6d15ce61.png&w=640&q=75)
Your All-in-One AI-Powered Toolkit for Academic Success.
+13062052269
info@desklib.com
Available 24*7 on WhatsApp / Email
Unlock your academic potential
© 2024 | Zucol Services PVT LTD | All rights reserved.