Mechanical and Thermal Properties of Hydrogel Nanocomposites
VerifiedAdded on 2019/11/26
|36
|8875
|256
Report
AI Summary
The provided content includes a collection of research articles and studies on the topic of nanoparticle solutions as adhesives for gels and biological tissues, as well as the mechanical and thermal properties of hydrogel nanocomposites. The papers explore the interactions between nanoparticles and polymers, investigating the effects of particle size, shape, and surface chemistry on the properties of the resulting composites. The studies cover a range of topics, including the reinforcement mechanism of nanofilled polymer melts, the structural and mechanical properties of polymer nanocomposites, and the development of novel hydrogel-based materials for biomedical applications.
Contribute Materials
Your contribution can guide someone’s learning journey. Share your
documents today.
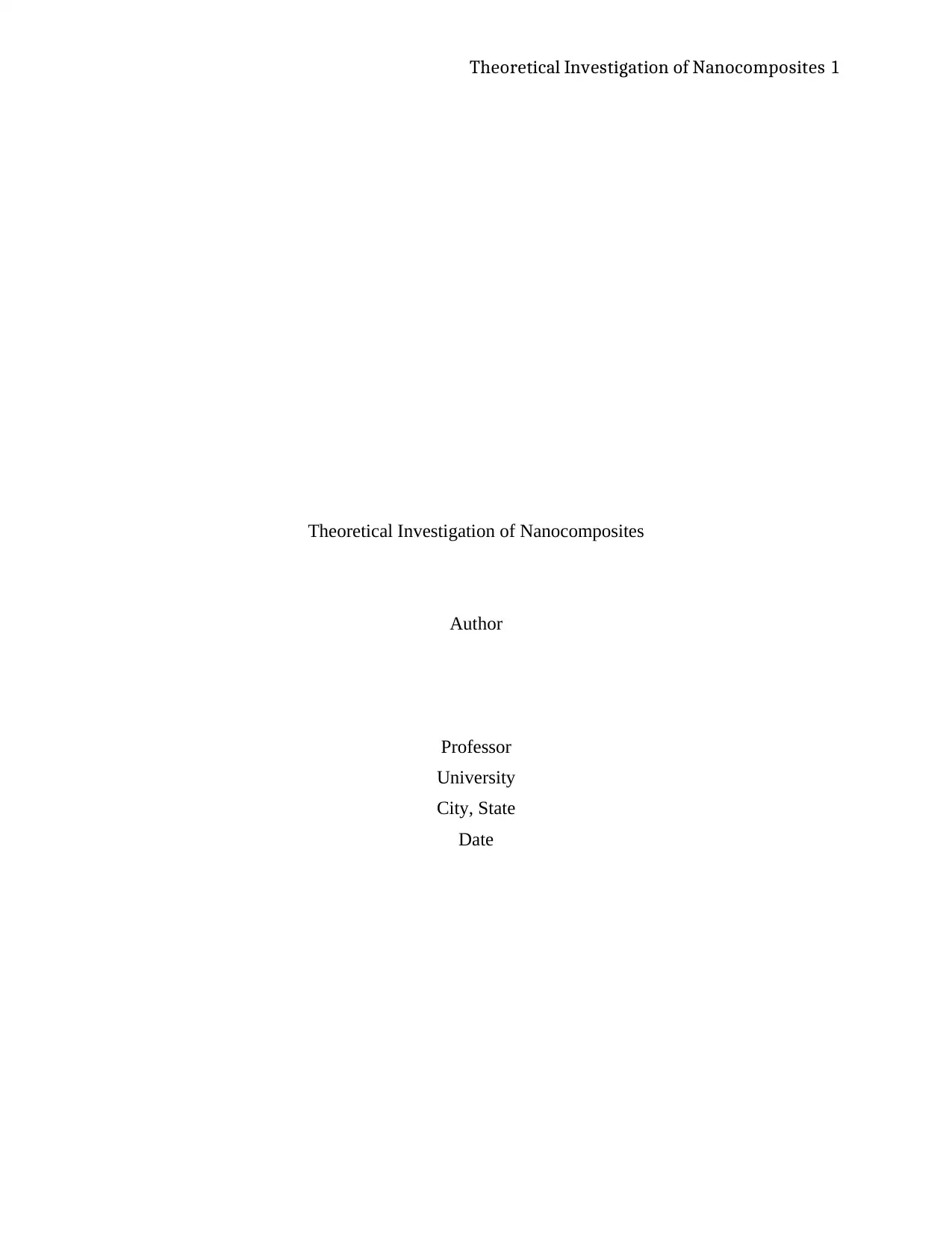
Theoretical Investigation of Nanocomposites 1
Theoretical Investigation of Nanocomposites
Author
Professor
University
City, State
Date
Theoretical Investigation of Nanocomposites
Author
Professor
University
City, State
Date
Secure Best Marks with AI Grader
Need help grading? Try our AI Grader for instant feedback on your assignments.
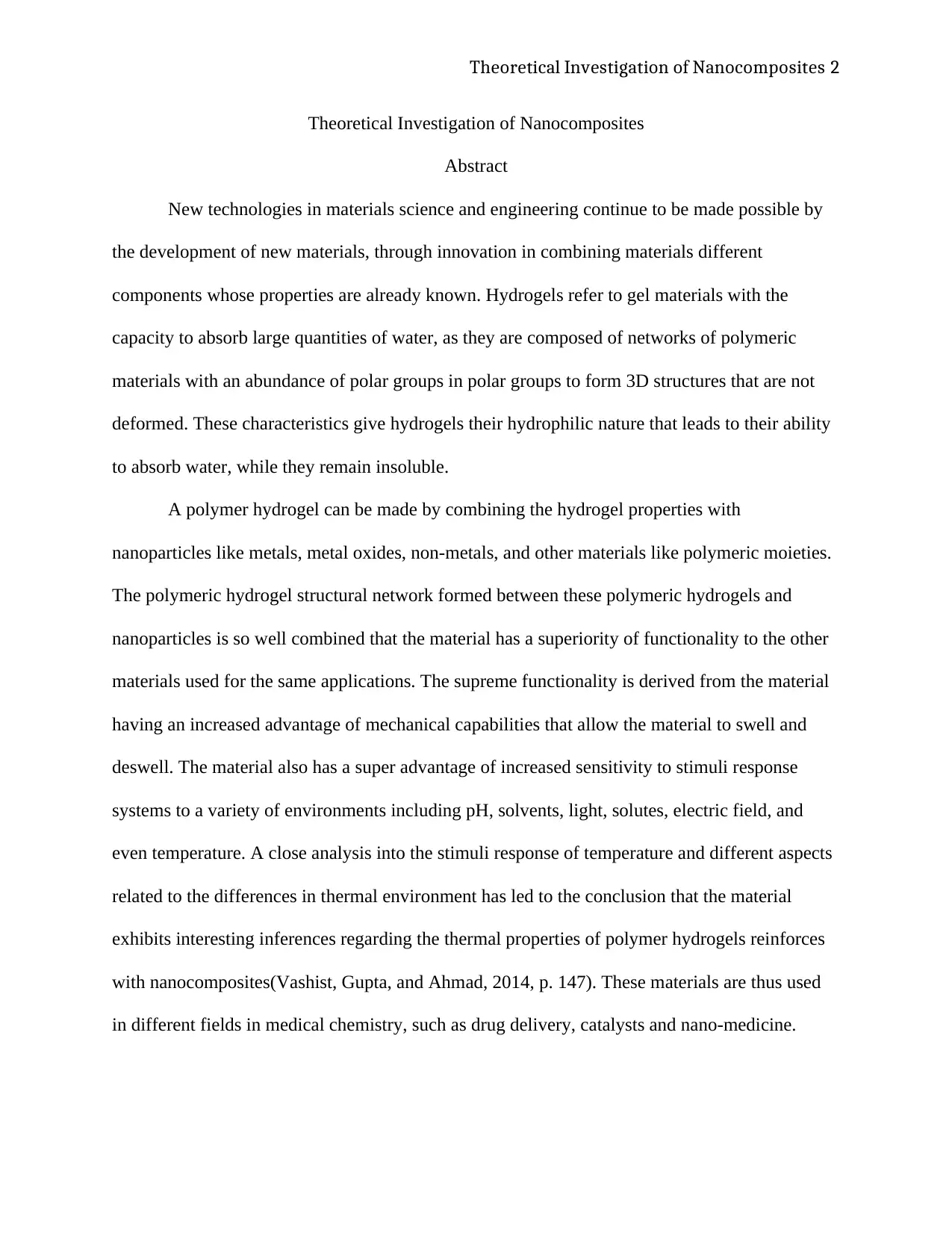
Theoretical Investigation of Nanocomposites 2
Theoretical Investigation of Nanocomposites
Abstract
New technologies in materials science and engineering continue to be made possible by
the development of new materials, through innovation in combining materials different
components whose properties are already known. Hydrogels refer to gel materials with the
capacity to absorb large quantities of water, as they are composed of networks of polymeric
materials with an abundance of polar groups in polar groups to form 3D structures that are not
deformed. These characteristics give hydrogels their hydrophilic nature that leads to their ability
to absorb water, while they remain insoluble.
A polymer hydrogel can be made by combining the hydrogel properties with
nanoparticles like metals, metal oxides, non-metals, and other materials like polymeric moieties.
The polymeric hydrogel structural network formed between these polymeric hydrogels and
nanoparticles is so well combined that the material has a superiority of functionality to the other
materials used for the same applications. The supreme functionality is derived from the material
having an increased advantage of mechanical capabilities that allow the material to swell and
deswell. The material also has a super advantage of increased sensitivity to stimuli response
systems to a variety of environments including pH, solvents, light, solutes, electric field, and
even temperature. A close analysis into the stimuli response of temperature and different aspects
related to the differences in thermal environment has led to the conclusion that the material
exhibits interesting inferences regarding the thermal properties of polymer hydrogels reinforces
with nanocomposites(Vashist, Gupta, and Ahmad, 2014, p. 147). These materials are thus used
in different fields in medical chemistry, such as drug delivery, catalysts and nano-medicine.
Theoretical Investigation of Nanocomposites
Abstract
New technologies in materials science and engineering continue to be made possible by
the development of new materials, through innovation in combining materials different
components whose properties are already known. Hydrogels refer to gel materials with the
capacity to absorb large quantities of water, as they are composed of networks of polymeric
materials with an abundance of polar groups in polar groups to form 3D structures that are not
deformed. These characteristics give hydrogels their hydrophilic nature that leads to their ability
to absorb water, while they remain insoluble.
A polymer hydrogel can be made by combining the hydrogel properties with
nanoparticles like metals, metal oxides, non-metals, and other materials like polymeric moieties.
The polymeric hydrogel structural network formed between these polymeric hydrogels and
nanoparticles is so well combined that the material has a superiority of functionality to the other
materials used for the same applications. The supreme functionality is derived from the material
having an increased advantage of mechanical capabilities that allow the material to swell and
deswell. The material also has a super advantage of increased sensitivity to stimuli response
systems to a variety of environments including pH, solvents, light, solutes, electric field, and
even temperature. A close analysis into the stimuli response of temperature and different aspects
related to the differences in thermal environment has led to the conclusion that the material
exhibits interesting inferences regarding the thermal properties of polymer hydrogels reinforces
with nanocomposites(Vashist, Gupta, and Ahmad, 2014, p. 147). These materials are thus used
in different fields in medical chemistry, such as drug delivery, catalysts and nano-medicine.
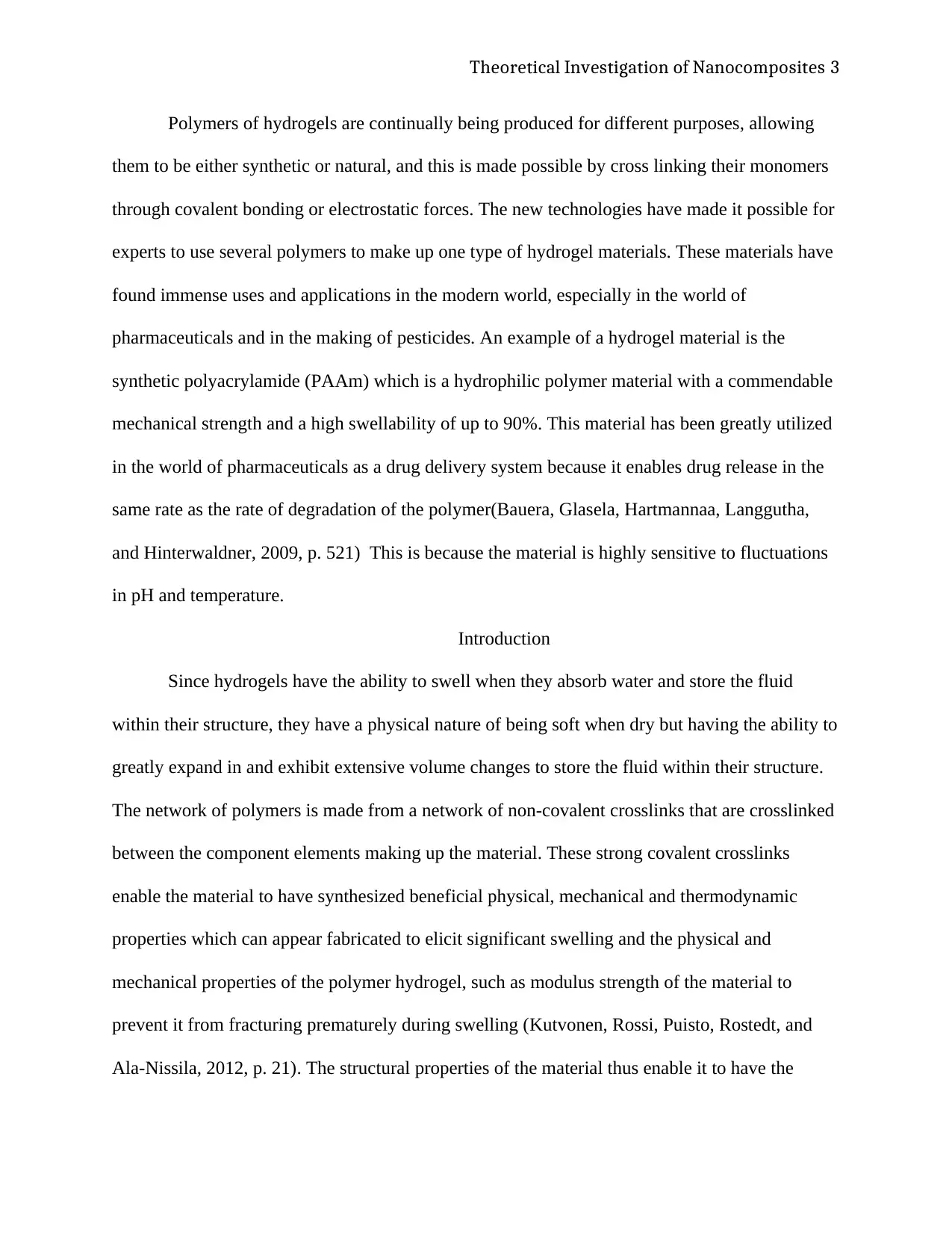
Theoretical Investigation of Nanocomposites 3
Polymers of hydrogels are continually being produced for different purposes, allowing
them to be either synthetic or natural, and this is made possible by cross linking their monomers
through covalent bonding or electrostatic forces. The new technologies have made it possible for
experts to use several polymers to make up one type of hydrogel materials. These materials have
found immense uses and applications in the modern world, especially in the world of
pharmaceuticals and in the making of pesticides. An example of a hydrogel material is the
synthetic polyacrylamide (PAAm) which is a hydrophilic polymer material with a commendable
mechanical strength and a high swellability of up to 90%. This material has been greatly utilized
in the world of pharmaceuticals as a drug delivery system because it enables drug release in the
same rate as the rate of degradation of the polymer(Bauera, Glasela, Hartmannaa, Langgutha,
and Hinterwaldner, 2009, p. 521) This is because the material is highly sensitive to fluctuations
in pH and temperature.
Introduction
Since hydrogels have the ability to swell when they absorb water and store the fluid
within their structure, they have a physical nature of being soft when dry but having the ability to
greatly expand in and exhibit extensive volume changes to store the fluid within their structure.
The network of polymers is made from a network of non-covalent crosslinks that are crosslinked
between the component elements making up the material. These strong covalent crosslinks
enable the material to have synthesized beneficial physical, mechanical and thermodynamic
properties which can appear fabricated to elicit significant swelling and the physical and
mechanical properties of the polymer hydrogel, such as modulus strength of the material to
prevent it from fracturing prematurely during swelling (Kutvonen, Rossi, Puisto, Rostedt, and
Ala-Nissila, 2012, p. 21). The structural properties of the material thus enable it to have the
Polymers of hydrogels are continually being produced for different purposes, allowing
them to be either synthetic or natural, and this is made possible by cross linking their monomers
through covalent bonding or electrostatic forces. The new technologies have made it possible for
experts to use several polymers to make up one type of hydrogel materials. These materials have
found immense uses and applications in the modern world, especially in the world of
pharmaceuticals and in the making of pesticides. An example of a hydrogel material is the
synthetic polyacrylamide (PAAm) which is a hydrophilic polymer material with a commendable
mechanical strength and a high swellability of up to 90%. This material has been greatly utilized
in the world of pharmaceuticals as a drug delivery system because it enables drug release in the
same rate as the rate of degradation of the polymer(Bauera, Glasela, Hartmannaa, Langgutha,
and Hinterwaldner, 2009, p. 521) This is because the material is highly sensitive to fluctuations
in pH and temperature.
Introduction
Since hydrogels have the ability to swell when they absorb water and store the fluid
within their structure, they have a physical nature of being soft when dry but having the ability to
greatly expand in and exhibit extensive volume changes to store the fluid within their structure.
The network of polymers is made from a network of non-covalent crosslinks that are crosslinked
between the component elements making up the material. These strong covalent crosslinks
enable the material to have synthesized beneficial physical, mechanical and thermodynamic
properties which can appear fabricated to elicit significant swelling and the physical and
mechanical properties of the polymer hydrogel, such as modulus strength of the material to
prevent it from fracturing prematurely during swelling (Kutvonen, Rossi, Puisto, Rostedt, and
Ala-Nissila, 2012, p. 21). The structural properties of the material thus enable it to have the
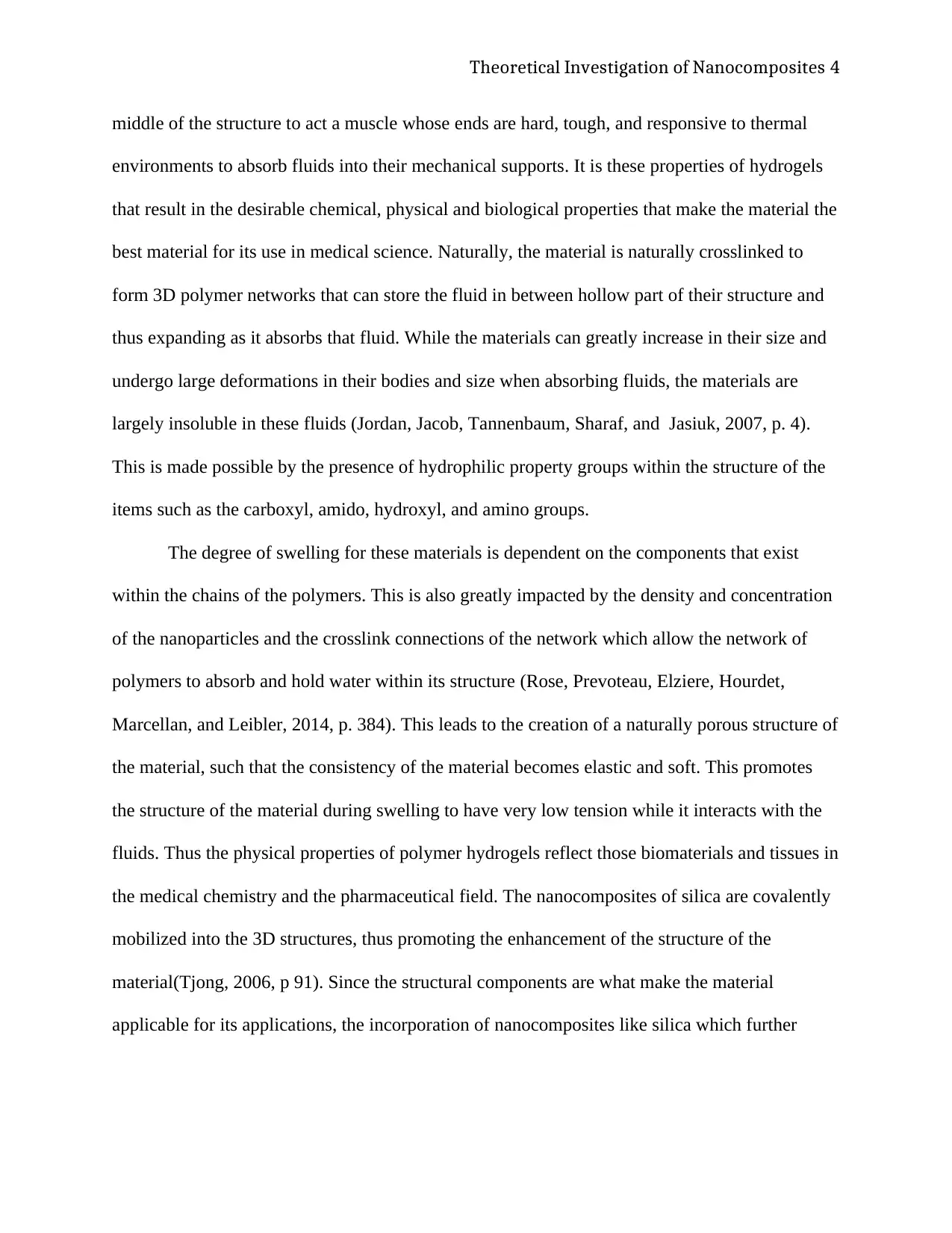
Theoretical Investigation of Nanocomposites 4
middle of the structure to act a muscle whose ends are hard, tough, and responsive to thermal
environments to absorb fluids into their mechanical supports. It is these properties of hydrogels
that result in the desirable chemical, physical and biological properties that make the material the
best material for its use in medical science. Naturally, the material is naturally crosslinked to
form 3D polymer networks that can store the fluid in between hollow part of their structure and
thus expanding as it absorbs that fluid. While the materials can greatly increase in their size and
undergo large deformations in their bodies and size when absorbing fluids, the materials are
largely insoluble in these fluids (Jordan, Jacob, Tannenbaum, Sharaf, and Jasiuk, 2007, p. 4).
This is made possible by the presence of hydrophilic property groups within the structure of the
items such as the carboxyl, amido, hydroxyl, and amino groups.
The degree of swelling for these materials is dependent on the components that exist
within the chains of the polymers. This is also greatly impacted by the density and concentration
of the nanoparticles and the crosslink connections of the network which allow the network of
polymers to absorb and hold water within its structure (Rose, Prevoteau, Elziere, Hourdet,
Marcellan, and Leibler, 2014, p. 384). This leads to the creation of a naturally porous structure of
the material, such that the consistency of the material becomes elastic and soft. This promotes
the structure of the material during swelling to have very low tension while it interacts with the
fluids. Thus the physical properties of polymer hydrogels reflect those biomaterials and tissues in
the medical chemistry and the pharmaceutical field. The nanocomposites of silica are covalently
mobilized into the 3D structures, thus promoting the enhancement of the structure of the
material(Tjong, 2006, p 91). Since the structural components are what make the material
applicable for its applications, the incorporation of nanocomposites like silica which further
middle of the structure to act a muscle whose ends are hard, tough, and responsive to thermal
environments to absorb fluids into their mechanical supports. It is these properties of hydrogels
that result in the desirable chemical, physical and biological properties that make the material the
best material for its use in medical science. Naturally, the material is naturally crosslinked to
form 3D polymer networks that can store the fluid in between hollow part of their structure and
thus expanding as it absorbs that fluid. While the materials can greatly increase in their size and
undergo large deformations in their bodies and size when absorbing fluids, the materials are
largely insoluble in these fluids (Jordan, Jacob, Tannenbaum, Sharaf, and Jasiuk, 2007, p. 4).
This is made possible by the presence of hydrophilic property groups within the structure of the
items such as the carboxyl, amido, hydroxyl, and amino groups.
The degree of swelling for these materials is dependent on the components that exist
within the chains of the polymers. This is also greatly impacted by the density and concentration
of the nanoparticles and the crosslink connections of the network which allow the network of
polymers to absorb and hold water within its structure (Rose, Prevoteau, Elziere, Hourdet,
Marcellan, and Leibler, 2014, p. 384). This leads to the creation of a naturally porous structure of
the material, such that the consistency of the material becomes elastic and soft. This promotes
the structure of the material during swelling to have very low tension while it interacts with the
fluids. Thus the physical properties of polymer hydrogels reflect those biomaterials and tissues in
the medical chemistry and the pharmaceutical field. The nanocomposites of silica are covalently
mobilized into the 3D structures, thus promoting the enhancement of the structure of the
material(Tjong, 2006, p 91). Since the structural components are what make the material
applicable for its applications, the incorporation of nanocomposites like silica which further
Secure Best Marks with AI Grader
Need help grading? Try our AI Grader for instant feedback on your assignments.
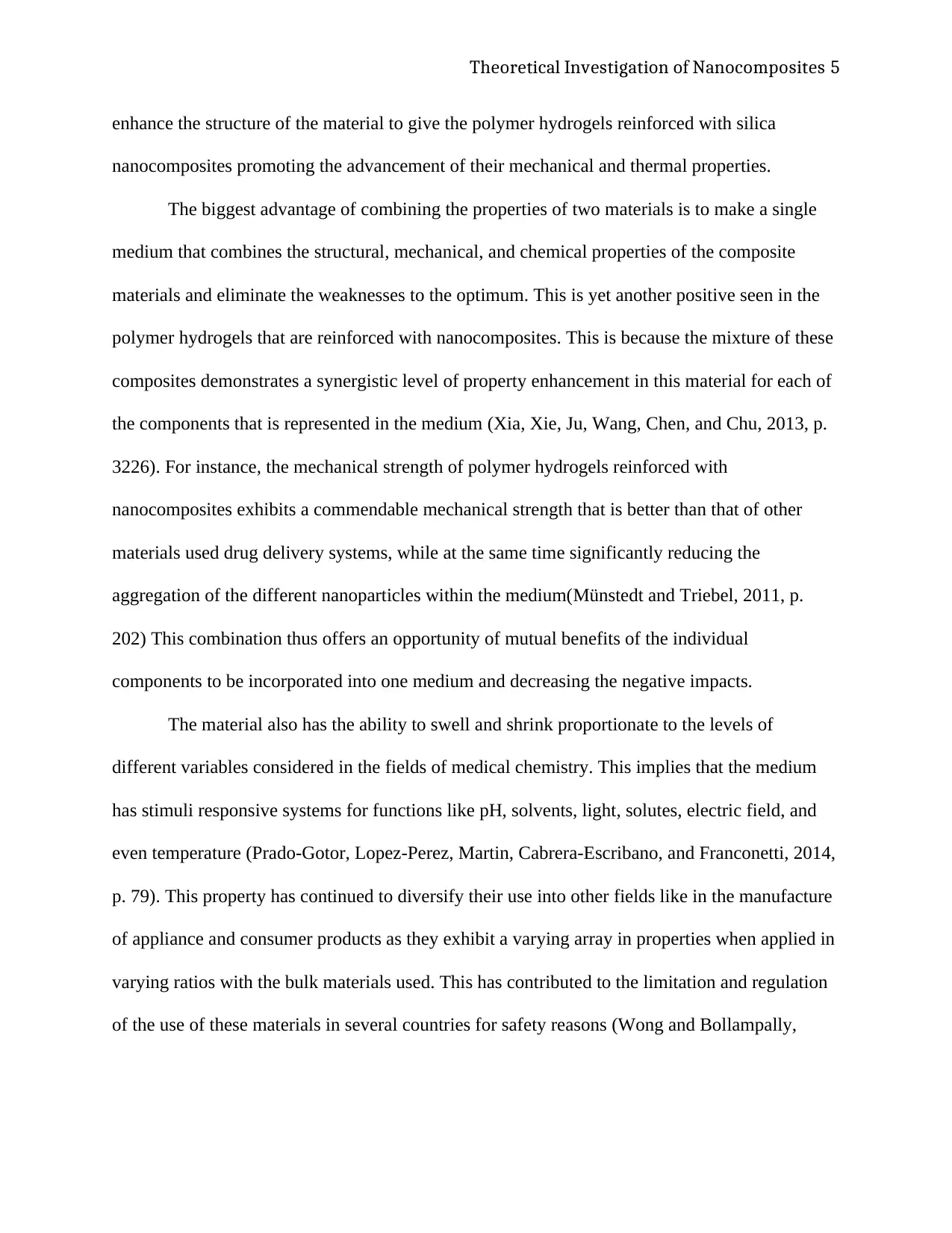
Theoretical Investigation of Nanocomposites 5
enhance the structure of the material to give the polymer hydrogels reinforced with silica
nanocomposites promoting the advancement of their mechanical and thermal properties.
The biggest advantage of combining the properties of two materials is to make a single
medium that combines the structural, mechanical, and chemical properties of the composite
materials and eliminate the weaknesses to the optimum. This is yet another positive seen in the
polymer hydrogels that are reinforced with nanocomposites. This is because the mixture of these
composites demonstrates a synergistic level of property enhancement in this material for each of
the components that is represented in the medium (Xia, Xie, Ju, Wang, Chen, and Chu, 2013, p.
3226). For instance, the mechanical strength of polymer hydrogels reinforced with
nanocomposites exhibits a commendable mechanical strength that is better than that of other
materials used drug delivery systems, while at the same time significantly reducing the
aggregation of the different nanoparticles within the medium(Münstedt and Triebel, 2011, p.
202) This combination thus offers an opportunity of mutual benefits of the individual
components to be incorporated into one medium and decreasing the negative impacts.
The material also has the ability to swell and shrink proportionate to the levels of
different variables considered in the fields of medical chemistry. This implies that the medium
has stimuli responsive systems for functions like pH, solvents, light, solutes, electric field, and
even temperature (Prado-Gotor, Lopez-Perez, Martin, Cabrera-Escribano, and Franconetti, 2014,
p. 79). This property has continued to diversify their use into other fields like in the manufacture
of appliance and consumer products as they exhibit a varying array in properties when applied in
varying ratios with the bulk materials used. This has contributed to the limitation and regulation
of the use of these materials in several countries for safety reasons (Wong and Bollampally,
enhance the structure of the material to give the polymer hydrogels reinforced with silica
nanocomposites promoting the advancement of their mechanical and thermal properties.
The biggest advantage of combining the properties of two materials is to make a single
medium that combines the structural, mechanical, and chemical properties of the composite
materials and eliminate the weaknesses to the optimum. This is yet another positive seen in the
polymer hydrogels that are reinforced with nanocomposites. This is because the mixture of these
composites demonstrates a synergistic level of property enhancement in this material for each of
the components that is represented in the medium (Xia, Xie, Ju, Wang, Chen, and Chu, 2013, p.
3226). For instance, the mechanical strength of polymer hydrogels reinforced with
nanocomposites exhibits a commendable mechanical strength that is better than that of other
materials used drug delivery systems, while at the same time significantly reducing the
aggregation of the different nanoparticles within the medium(Münstedt and Triebel, 2011, p.
202) This combination thus offers an opportunity of mutual benefits of the individual
components to be incorporated into one medium and decreasing the negative impacts.
The material also has the ability to swell and shrink proportionate to the levels of
different variables considered in the fields of medical chemistry. This implies that the medium
has stimuli responsive systems for functions like pH, solvents, light, solutes, electric field, and
even temperature (Prado-Gotor, Lopez-Perez, Martin, Cabrera-Escribano, and Franconetti, 2014,
p. 79). This property has continued to diversify their use into other fields like in the manufacture
of appliance and consumer products as they exhibit a varying array in properties when applied in
varying ratios with the bulk materials used. This has contributed to the limitation and regulation
of the use of these materials in several countries for safety reasons (Wong and Bollampally,
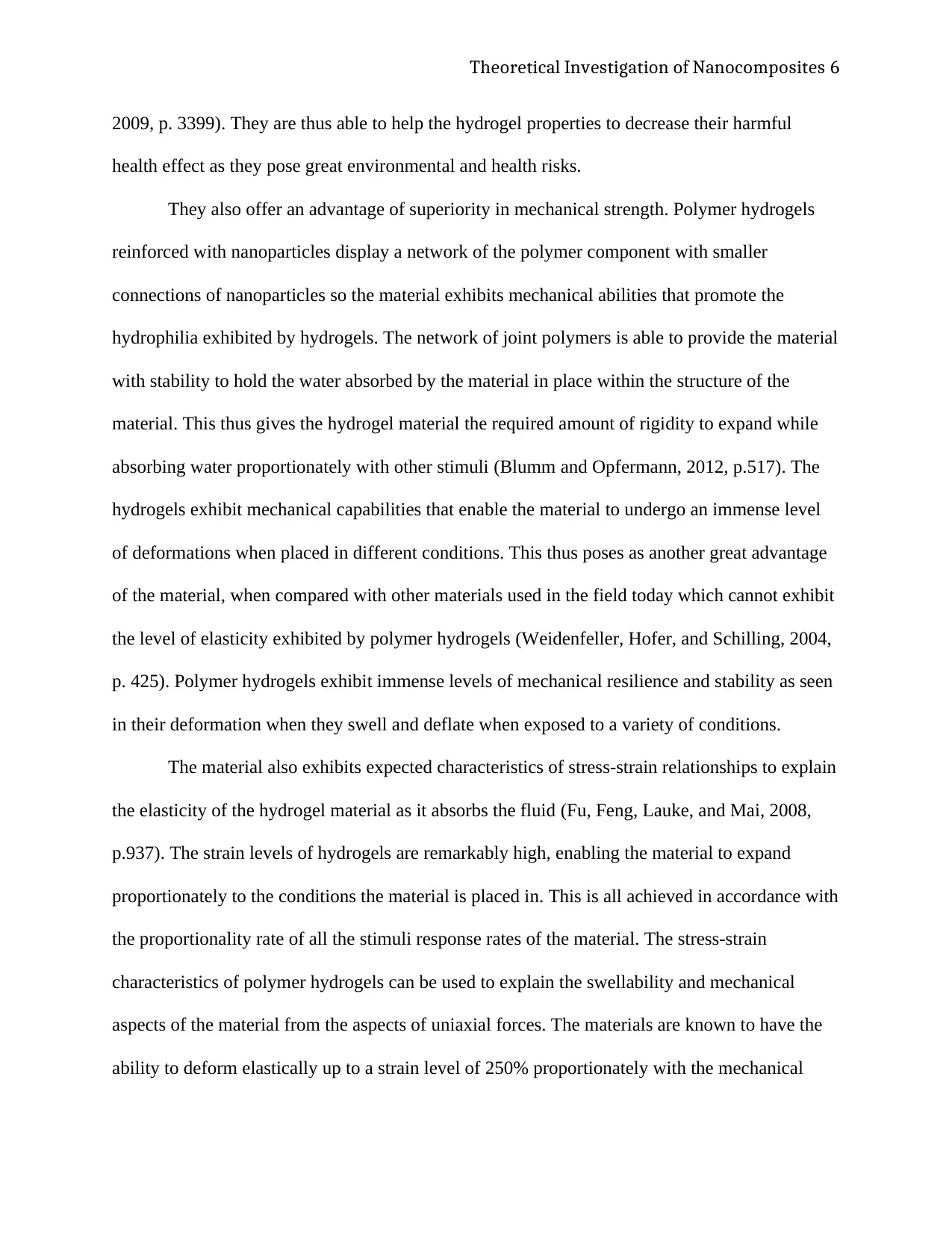
Theoretical Investigation of Nanocomposites 6
2009, p. 3399). They are thus able to help the hydrogel properties to decrease their harmful
health effect as they pose great environmental and health risks.
They also offer an advantage of superiority in mechanical strength. Polymer hydrogels
reinforced with nanoparticles display a network of the polymer component with smaller
connections of nanoparticles so the material exhibits mechanical abilities that promote the
hydrophilia exhibited by hydrogels. The network of joint polymers is able to provide the material
with stability to hold the water absorbed by the material in place within the structure of the
material. This thus gives the hydrogel material the required amount of rigidity to expand while
absorbing water proportionately with other stimuli (Blumm and Opfermann, 2012, p.517). The
hydrogels exhibit mechanical capabilities that enable the material to undergo an immense level
of deformations when placed in different conditions. This thus poses as another great advantage
of the material, when compared with other materials used in the field today which cannot exhibit
the level of elasticity exhibited by polymer hydrogels (Weidenfeller, Hofer, and Schilling, 2004,
p. 425). Polymer hydrogels exhibit immense levels of mechanical resilience and stability as seen
in their deformation when they swell and deflate when exposed to a variety of conditions.
The material also exhibits expected characteristics of stress-strain relationships to explain
the elasticity of the hydrogel material as it absorbs the fluid (Fu, Feng, Lauke, and Mai, 2008,
p.937). The strain levels of hydrogels are remarkably high, enabling the material to expand
proportionately to the conditions the material is placed in. This is all achieved in accordance with
the proportionality rate of all the stimuli response rates of the material. The stress-strain
characteristics of polymer hydrogels can be used to explain the swellability and mechanical
aspects of the material from the aspects of uniaxial forces. The materials are known to have the
ability to deform elastically up to a strain level of 250% proportionately with the mechanical
2009, p. 3399). They are thus able to help the hydrogel properties to decrease their harmful
health effect as they pose great environmental and health risks.
They also offer an advantage of superiority in mechanical strength. Polymer hydrogels
reinforced with nanoparticles display a network of the polymer component with smaller
connections of nanoparticles so the material exhibits mechanical abilities that promote the
hydrophilia exhibited by hydrogels. The network of joint polymers is able to provide the material
with stability to hold the water absorbed by the material in place within the structure of the
material. This thus gives the hydrogel material the required amount of rigidity to expand while
absorbing water proportionately with other stimuli (Blumm and Opfermann, 2012, p.517). The
hydrogels exhibit mechanical capabilities that enable the material to undergo an immense level
of deformations when placed in different conditions. This thus poses as another great advantage
of the material, when compared with other materials used in the field today which cannot exhibit
the level of elasticity exhibited by polymer hydrogels (Weidenfeller, Hofer, and Schilling, 2004,
p. 425). Polymer hydrogels exhibit immense levels of mechanical resilience and stability as seen
in their deformation when they swell and deflate when exposed to a variety of conditions.
The material also exhibits expected characteristics of stress-strain relationships to explain
the elasticity of the hydrogel material as it absorbs the fluid (Fu, Feng, Lauke, and Mai, 2008,
p.937). The strain levels of hydrogels are remarkably high, enabling the material to expand
proportionately to the conditions the material is placed in. This is all achieved in accordance with
the proportionality rate of all the stimuli response rates of the material. The stress-strain
characteristics of polymer hydrogels can be used to explain the swellability and mechanical
aspects of the material from the aspects of uniaxial forces. The materials are known to have the
ability to deform elastically up to a strain level of 250% proportionately with the mechanical
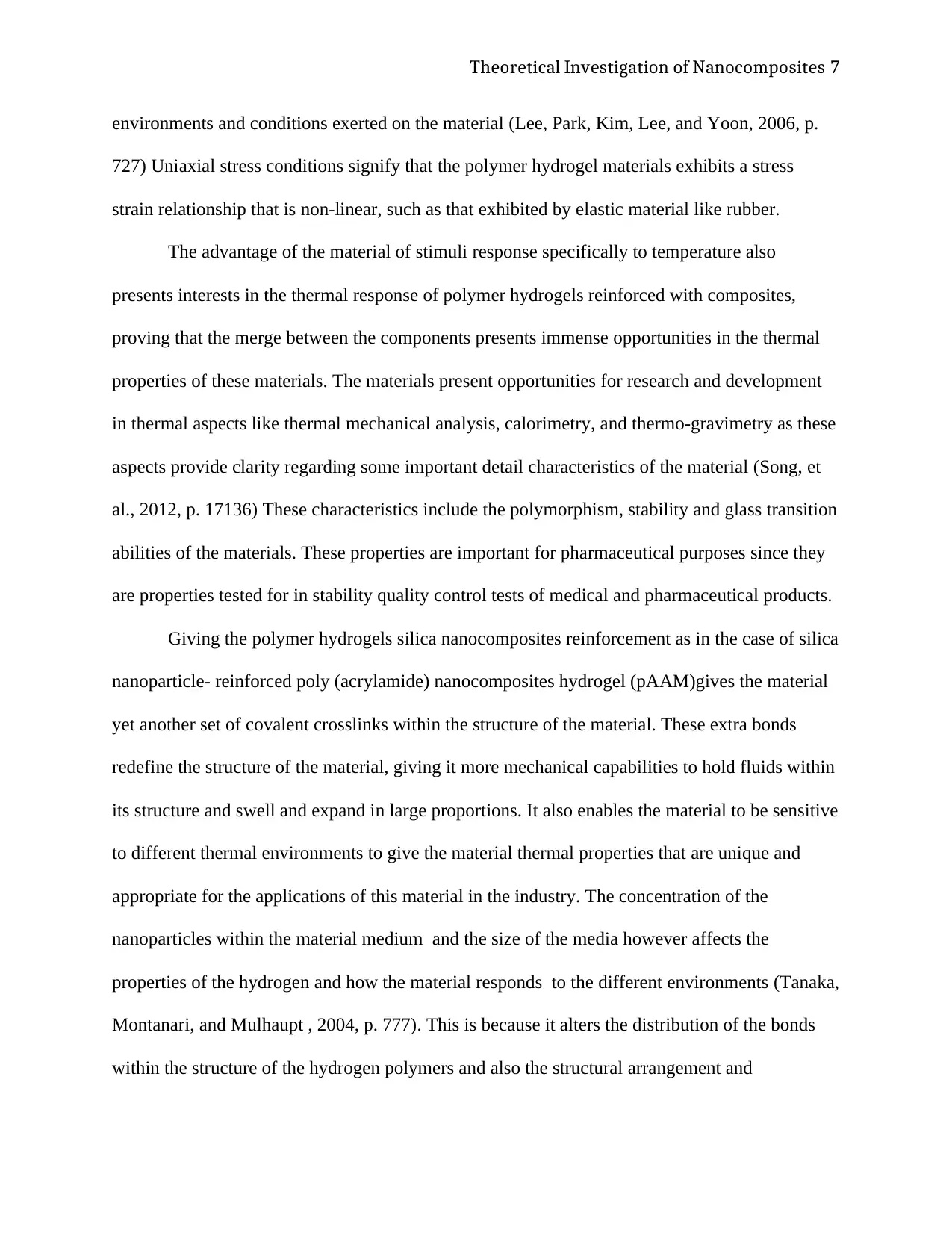
Theoretical Investigation of Nanocomposites 7
environments and conditions exerted on the material (Lee, Park, Kim, Lee, and Yoon, 2006, p.
727) Uniaxial stress conditions signify that the polymer hydrogel materials exhibits a stress
strain relationship that is non-linear, such as that exhibited by elastic material like rubber.
The advantage of the material of stimuli response specifically to temperature also
presents interests in the thermal response of polymer hydrogels reinforced with composites,
proving that the merge between the components presents immense opportunities in the thermal
properties of these materials. The materials present opportunities for research and development
in thermal aspects like thermal mechanical analysis, calorimetry, and thermo-gravimetry as these
aspects provide clarity regarding some important detail characteristics of the material (Song, et
al., 2012, p. 17136) These characteristics include the polymorphism, stability and glass transition
abilities of the materials. These properties are important for pharmaceutical purposes since they
are properties tested for in stability quality control tests of medical and pharmaceutical products.
Giving the polymer hydrogels silica nanocomposites reinforcement as in the case of silica
nanoparticle- reinforced poly (acrylamide) nanocomposites hydrogel (pAAM)gives the material
yet another set of covalent crosslinks within the structure of the material. These extra bonds
redefine the structure of the material, giving it more mechanical capabilities to hold fluids within
its structure and swell and expand in large proportions. It also enables the material to be sensitive
to different thermal environments to give the material thermal properties that are unique and
appropriate for the applications of this material in the industry. The concentration of the
nanoparticles within the material medium and the size of the media however affects the
properties of the hydrogen and how the material responds to the different environments (Tanaka,
Montanari, and Mulhaupt , 2004, p. 777). This is because it alters the distribution of the bonds
within the structure of the hydrogen polymers and also the structural arrangement and
environments and conditions exerted on the material (Lee, Park, Kim, Lee, and Yoon, 2006, p.
727) Uniaxial stress conditions signify that the polymer hydrogel materials exhibits a stress
strain relationship that is non-linear, such as that exhibited by elastic material like rubber.
The advantage of the material of stimuli response specifically to temperature also
presents interests in the thermal response of polymer hydrogels reinforced with composites,
proving that the merge between the components presents immense opportunities in the thermal
properties of these materials. The materials present opportunities for research and development
in thermal aspects like thermal mechanical analysis, calorimetry, and thermo-gravimetry as these
aspects provide clarity regarding some important detail characteristics of the material (Song, et
al., 2012, p. 17136) These characteristics include the polymorphism, stability and glass transition
abilities of the materials. These properties are important for pharmaceutical purposes since they
are properties tested for in stability quality control tests of medical and pharmaceutical products.
Giving the polymer hydrogels silica nanocomposites reinforcement as in the case of silica
nanoparticle- reinforced poly (acrylamide) nanocomposites hydrogel (pAAM)gives the material
yet another set of covalent crosslinks within the structure of the material. These extra bonds
redefine the structure of the material, giving it more mechanical capabilities to hold fluids within
its structure and swell and expand in large proportions. It also enables the material to be sensitive
to different thermal environments to give the material thermal properties that are unique and
appropriate for the applications of this material in the industry. The concentration of the
nanoparticles within the material medium and the size of the media however affects the
properties of the hydrogen and how the material responds to the different environments (Tanaka,
Montanari, and Mulhaupt , 2004, p. 777). This is because it alters the distribution of the bonds
within the structure of the hydrogen polymers and also the structural arrangement and
Paraphrase This Document
Need a fresh take? Get an instant paraphrase of this document with our AI Paraphraser
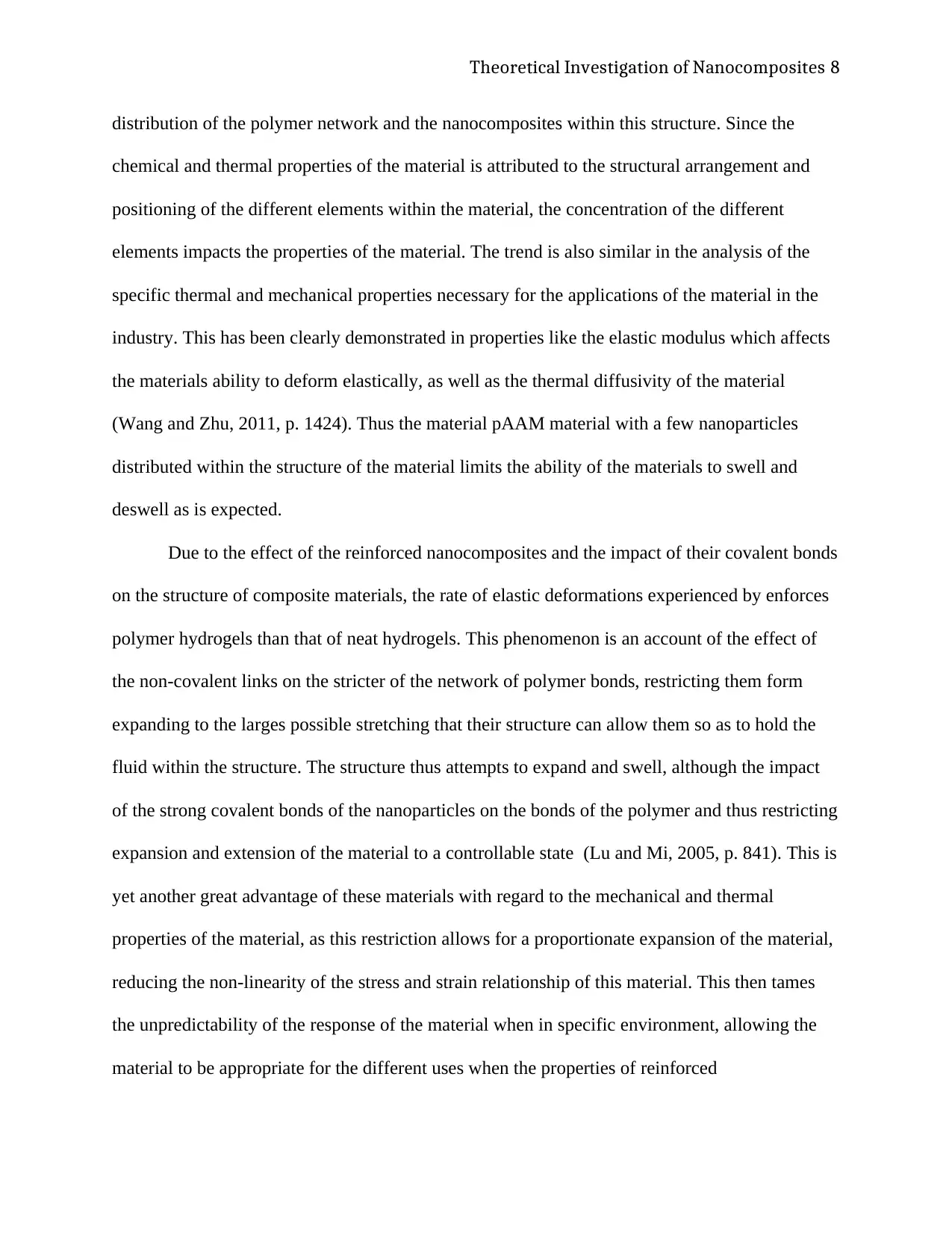
Theoretical Investigation of Nanocomposites 8
distribution of the polymer network and the nanocomposites within this structure. Since the
chemical and thermal properties of the material is attributed to the structural arrangement and
positioning of the different elements within the material, the concentration of the different
elements impacts the properties of the material. The trend is also similar in the analysis of the
specific thermal and mechanical properties necessary for the applications of the material in the
industry. This has been clearly demonstrated in properties like the elastic modulus which affects
the materials ability to deform elastically, as well as the thermal diffusivity of the material
(Wang and Zhu, 2011, p. 1424). Thus the material pAAM material with a few nanoparticles
distributed within the structure of the material limits the ability of the materials to swell and
deswell as is expected.
Due to the effect of the reinforced nanocomposites and the impact of their covalent bonds
on the structure of composite materials, the rate of elastic deformations experienced by enforces
polymer hydrogels than that of neat hydrogels. This phenomenon is an account of the effect of
the non-covalent links on the stricter of the network of polymer bonds, restricting them form
expanding to the larges possible stretching that their structure can allow them so as to hold the
fluid within the structure. The structure thus attempts to expand and swell, although the impact
of the strong covalent bonds of the nanoparticles on the bonds of the polymer and thus restricting
expansion and extension of the material to a controllable state (Lu and Mi, 2005, p. 841). This is
yet another great advantage of these materials with regard to the mechanical and thermal
properties of the material, as this restriction allows for a proportionate expansion of the material,
reducing the non-linearity of the stress and strain relationship of this material. This then tames
the unpredictability of the response of the material when in specific environment, allowing the
material to be appropriate for the different uses when the properties of reinforced
distribution of the polymer network and the nanocomposites within this structure. Since the
chemical and thermal properties of the material is attributed to the structural arrangement and
positioning of the different elements within the material, the concentration of the different
elements impacts the properties of the material. The trend is also similar in the analysis of the
specific thermal and mechanical properties necessary for the applications of the material in the
industry. This has been clearly demonstrated in properties like the elastic modulus which affects
the materials ability to deform elastically, as well as the thermal diffusivity of the material
(Wang and Zhu, 2011, p. 1424). Thus the material pAAM material with a few nanoparticles
distributed within the structure of the material limits the ability of the materials to swell and
deswell as is expected.
Due to the effect of the reinforced nanocomposites and the impact of their covalent bonds
on the structure of composite materials, the rate of elastic deformations experienced by enforces
polymer hydrogels than that of neat hydrogels. This phenomenon is an account of the effect of
the non-covalent links on the stricter of the network of polymer bonds, restricting them form
expanding to the larges possible stretching that their structure can allow them so as to hold the
fluid within the structure. The structure thus attempts to expand and swell, although the impact
of the strong covalent bonds of the nanoparticles on the bonds of the polymer and thus restricting
expansion and extension of the material to a controllable state (Lu and Mi, 2005, p. 841). This is
yet another great advantage of these materials with regard to the mechanical and thermal
properties of the material, as this restriction allows for a proportionate expansion of the material,
reducing the non-linearity of the stress and strain relationship of this material. This then tames
the unpredictability of the response of the material when in specific environment, allowing the
material to be appropriate for the different uses when the properties of reinforced
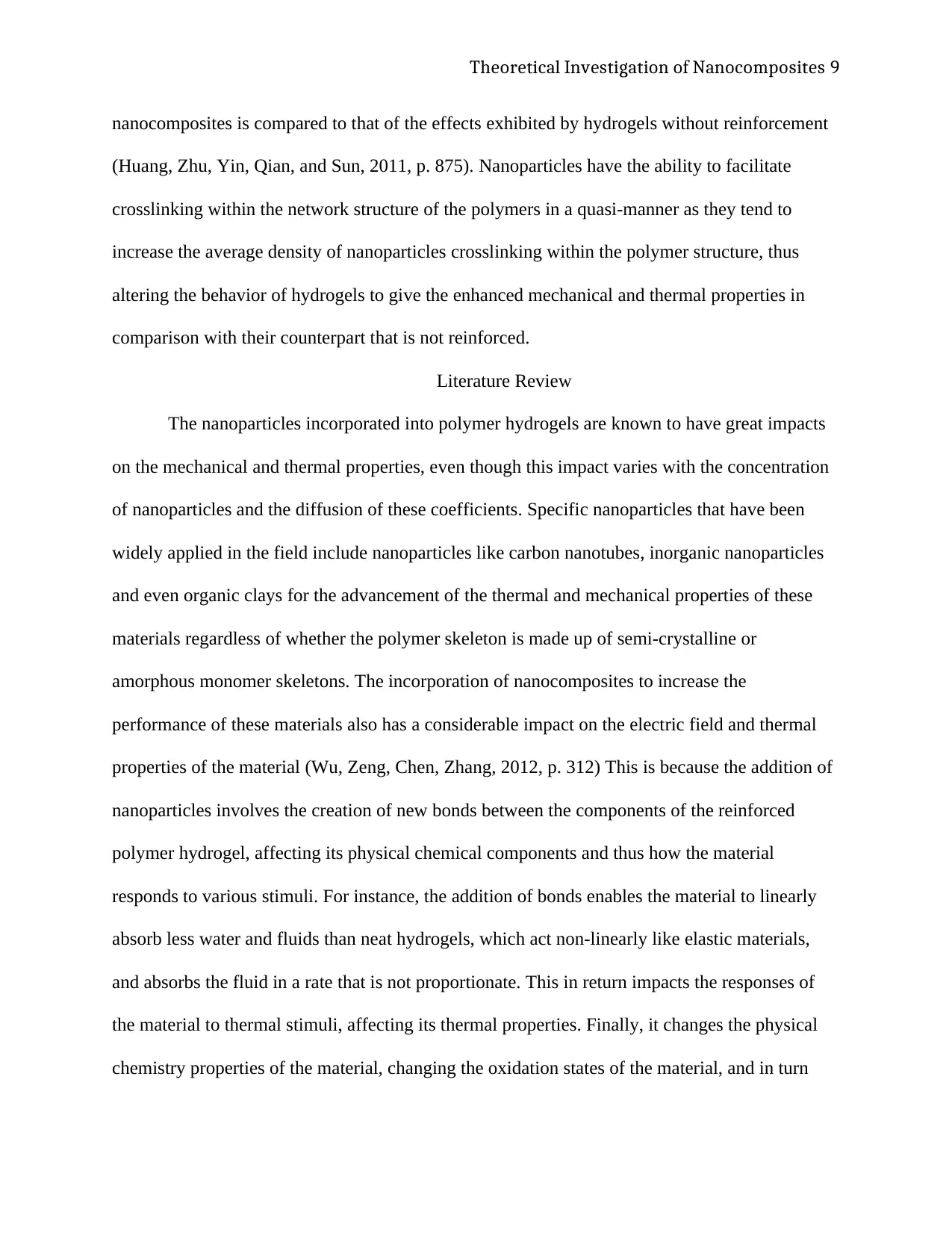
Theoretical Investigation of Nanocomposites 9
nanocomposites is compared to that of the effects exhibited by hydrogels without reinforcement
(Huang, Zhu, Yin, Qian, and Sun, 2011, p. 875). Nanoparticles have the ability to facilitate
crosslinking within the network structure of the polymers in a quasi-manner as they tend to
increase the average density of nanoparticles crosslinking within the polymer structure, thus
altering the behavior of hydrogels to give the enhanced mechanical and thermal properties in
comparison with their counterpart that is not reinforced.
Literature Review
The nanoparticles incorporated into polymer hydrogels are known to have great impacts
on the mechanical and thermal properties, even though this impact varies with the concentration
of nanoparticles and the diffusion of these coefficients. Specific nanoparticles that have been
widely applied in the field include nanoparticles like carbon nanotubes, inorganic nanoparticles
and even organic clays for the advancement of the thermal and mechanical properties of these
materials regardless of whether the polymer skeleton is made up of semi-crystalline or
amorphous monomer skeletons. The incorporation of nanocomposites to increase the
performance of these materials also has a considerable impact on the electric field and thermal
properties of the material (Wu, Zeng, Chen, Zhang, 2012, p. 312) This is because the addition of
nanoparticles involves the creation of new bonds between the components of the reinforced
polymer hydrogel, affecting its physical chemical components and thus how the material
responds to various stimuli. For instance, the addition of bonds enables the material to linearly
absorb less water and fluids than neat hydrogels, which act non-linearly like elastic materials,
and absorbs the fluid in a rate that is not proportionate. This in return impacts the responses of
the material to thermal stimuli, affecting its thermal properties. Finally, it changes the physical
chemistry properties of the material, changing the oxidation states of the material, and in turn
nanocomposites is compared to that of the effects exhibited by hydrogels without reinforcement
(Huang, Zhu, Yin, Qian, and Sun, 2011, p. 875). Nanoparticles have the ability to facilitate
crosslinking within the network structure of the polymers in a quasi-manner as they tend to
increase the average density of nanoparticles crosslinking within the polymer structure, thus
altering the behavior of hydrogels to give the enhanced mechanical and thermal properties in
comparison with their counterpart that is not reinforced.
Literature Review
The nanoparticles incorporated into polymer hydrogels are known to have great impacts
on the mechanical and thermal properties, even though this impact varies with the concentration
of nanoparticles and the diffusion of these coefficients. Specific nanoparticles that have been
widely applied in the field include nanoparticles like carbon nanotubes, inorganic nanoparticles
and even organic clays for the advancement of the thermal and mechanical properties of these
materials regardless of whether the polymer skeleton is made up of semi-crystalline or
amorphous monomer skeletons. The incorporation of nanocomposites to increase the
performance of these materials also has a considerable impact on the electric field and thermal
properties of the material (Wu, Zeng, Chen, Zhang, 2012, p. 312) This is because the addition of
nanoparticles involves the creation of new bonds between the components of the reinforced
polymer hydrogel, affecting its physical chemical components and thus how the material
responds to various stimuli. For instance, the addition of bonds enables the material to linearly
absorb less water and fluids than neat hydrogels, which act non-linearly like elastic materials,
and absorbs the fluid in a rate that is not proportionate. This in return impacts the responses of
the material to thermal stimuli, affecting its thermal properties. Finally, it changes the physical
chemistry properties of the material, changing the oxidation states of the material, and in turn
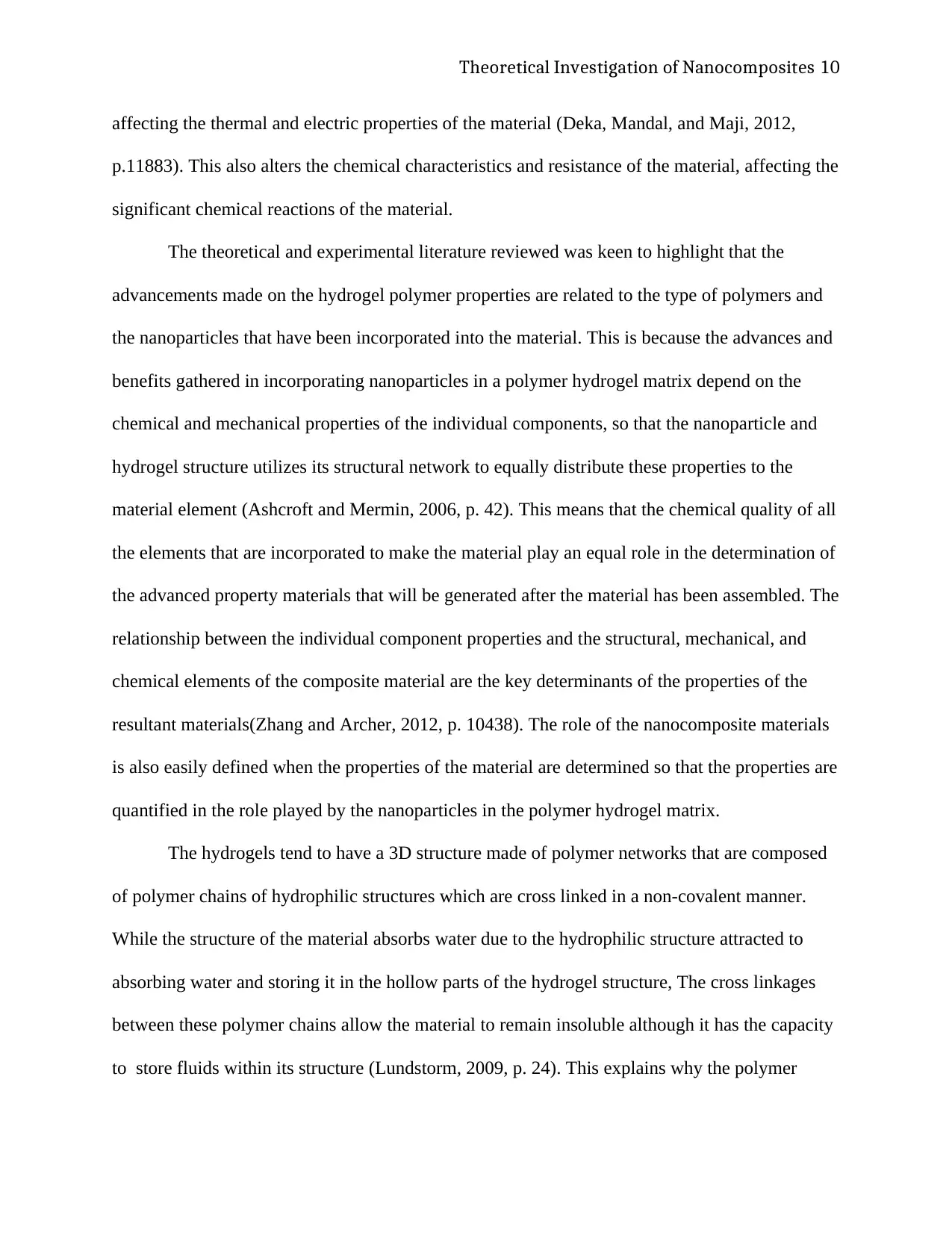
Theoretical Investigation of Nanocomposites 10
affecting the thermal and electric properties of the material (Deka, Mandal, and Maji, 2012,
p.11883). This also alters the chemical characteristics and resistance of the material, affecting the
significant chemical reactions of the material.
The theoretical and experimental literature reviewed was keen to highlight that the
advancements made on the hydrogel polymer properties are related to the type of polymers and
the nanoparticles that have been incorporated into the material. This is because the advances and
benefits gathered in incorporating nanoparticles in a polymer hydrogel matrix depend on the
chemical and mechanical properties of the individual components, so that the nanoparticle and
hydrogel structure utilizes its structural network to equally distribute these properties to the
material element (Ashcroft and Mermin, 2006, p. 42). This means that the chemical quality of all
the elements that are incorporated to make the material play an equal role in the determination of
the advanced property materials that will be generated after the material has been assembled. The
relationship between the individual component properties and the structural, mechanical, and
chemical elements of the composite material are the key determinants of the properties of the
resultant materials(Zhang and Archer, 2012, p. 10438). The role of the nanocomposite materials
is also easily defined when the properties of the material are determined so that the properties are
quantified in the role played by the nanoparticles in the polymer hydrogel matrix.
The hydrogels tend to have a 3D structure made of polymer networks that are composed
of polymer chains of hydrophilic structures which are cross linked in a non-covalent manner.
While the structure of the material absorbs water due to the hydrophilic structure attracted to
absorbing water and storing it in the hollow parts of the hydrogel structure, The cross linkages
between these polymer chains allow the material to remain insoluble although it has the capacity
to store fluids within its structure (Lundstorm, 2009, p. 24). This explains why the polymer
affecting the thermal and electric properties of the material (Deka, Mandal, and Maji, 2012,
p.11883). This also alters the chemical characteristics and resistance of the material, affecting the
significant chemical reactions of the material.
The theoretical and experimental literature reviewed was keen to highlight that the
advancements made on the hydrogel polymer properties are related to the type of polymers and
the nanoparticles that have been incorporated into the material. This is because the advances and
benefits gathered in incorporating nanoparticles in a polymer hydrogel matrix depend on the
chemical and mechanical properties of the individual components, so that the nanoparticle and
hydrogel structure utilizes its structural network to equally distribute these properties to the
material element (Ashcroft and Mermin, 2006, p. 42). This means that the chemical quality of all
the elements that are incorporated to make the material play an equal role in the determination of
the advanced property materials that will be generated after the material has been assembled. The
relationship between the individual component properties and the structural, mechanical, and
chemical elements of the composite material are the key determinants of the properties of the
resultant materials(Zhang and Archer, 2012, p. 10438). The role of the nanocomposite materials
is also easily defined when the properties of the material are determined so that the properties are
quantified in the role played by the nanoparticles in the polymer hydrogel matrix.
The hydrogels tend to have a 3D structure made of polymer networks that are composed
of polymer chains of hydrophilic structures which are cross linked in a non-covalent manner.
While the structure of the material absorbs water due to the hydrophilic structure attracted to
absorbing water and storing it in the hollow parts of the hydrogel structure, The cross linkages
between these polymer chains allow the material to remain insoluble although it has the capacity
to store fluids within its structure (Lundstorm, 2009, p. 24). This explains why the polymer
Secure Best Marks with AI Grader
Need help grading? Try our AI Grader for instant feedback on your assignments.
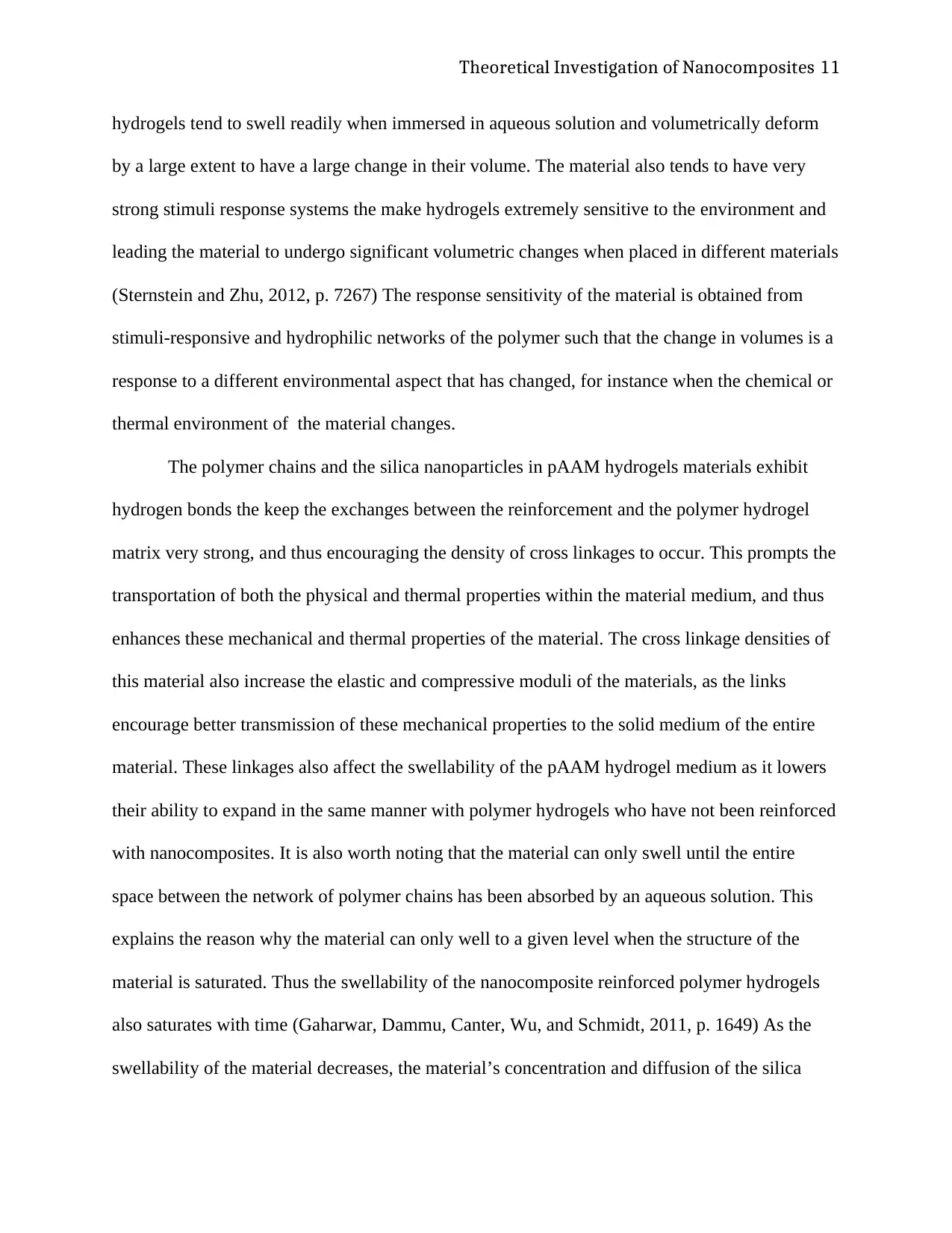
Theoretical Investigation of Nanocomposites 11
hydrogels tend to swell readily when immersed in aqueous solution and volumetrically deform
by a large extent to have a large change in their volume. The material also tends to have very
strong stimuli response systems the make hydrogels extremely sensitive to the environment and
leading the material to undergo significant volumetric changes when placed in different materials
(Sternstein and Zhu, 2012, p. 7267) The response sensitivity of the material is obtained from
stimuli-responsive and hydrophilic networks of the polymer such that the change in volumes is a
response to a different environmental aspect that has changed, for instance when the chemical or
thermal environment of the material changes.
The polymer chains and the silica nanoparticles in pAAM hydrogels materials exhibit
hydrogen bonds the keep the exchanges between the reinforcement and the polymer hydrogel
matrix very strong, and thus encouraging the density of cross linkages to occur. This prompts the
transportation of both the physical and thermal properties within the material medium, and thus
enhances these mechanical and thermal properties of the material. The cross linkage densities of
this material also increase the elastic and compressive moduli of the materials, as the links
encourage better transmission of these mechanical properties to the solid medium of the entire
material. These linkages also affect the swellability of the pAAM hydrogel medium as it lowers
their ability to expand in the same manner with polymer hydrogels who have not been reinforced
with nanocomposites. It is also worth noting that the material can only swell until the entire
space between the network of polymer chains has been absorbed by an aqueous solution. This
explains the reason why the material can only well to a given level when the structure of the
material is saturated. Thus the swellability of the nanocomposite reinforced polymer hydrogels
also saturates with time (Gaharwar, Dammu, Canter, Wu, and Schmidt, 2011, p. 1649) As the
swellability of the material decreases, the material’s concentration and diffusion of the silica
hydrogels tend to swell readily when immersed in aqueous solution and volumetrically deform
by a large extent to have a large change in their volume. The material also tends to have very
strong stimuli response systems the make hydrogels extremely sensitive to the environment and
leading the material to undergo significant volumetric changes when placed in different materials
(Sternstein and Zhu, 2012, p. 7267) The response sensitivity of the material is obtained from
stimuli-responsive and hydrophilic networks of the polymer such that the change in volumes is a
response to a different environmental aspect that has changed, for instance when the chemical or
thermal environment of the material changes.
The polymer chains and the silica nanoparticles in pAAM hydrogels materials exhibit
hydrogen bonds the keep the exchanges between the reinforcement and the polymer hydrogel
matrix very strong, and thus encouraging the density of cross linkages to occur. This prompts the
transportation of both the physical and thermal properties within the material medium, and thus
enhances these mechanical and thermal properties of the material. The cross linkage densities of
this material also increase the elastic and compressive moduli of the materials, as the links
encourage better transmission of these mechanical properties to the solid medium of the entire
material. These linkages also affect the swellability of the pAAM hydrogel medium as it lowers
their ability to expand in the same manner with polymer hydrogels who have not been reinforced
with nanocomposites. It is also worth noting that the material can only swell until the entire
space between the network of polymer chains has been absorbed by an aqueous solution. This
explains the reason why the material can only well to a given level when the structure of the
material is saturated. Thus the swellability of the nanocomposite reinforced polymer hydrogels
also saturates with time (Gaharwar, Dammu, Canter, Wu, and Schmidt, 2011, p. 1649) As the
swellability of the material decreases, the material’s concentration and diffusion of the silica
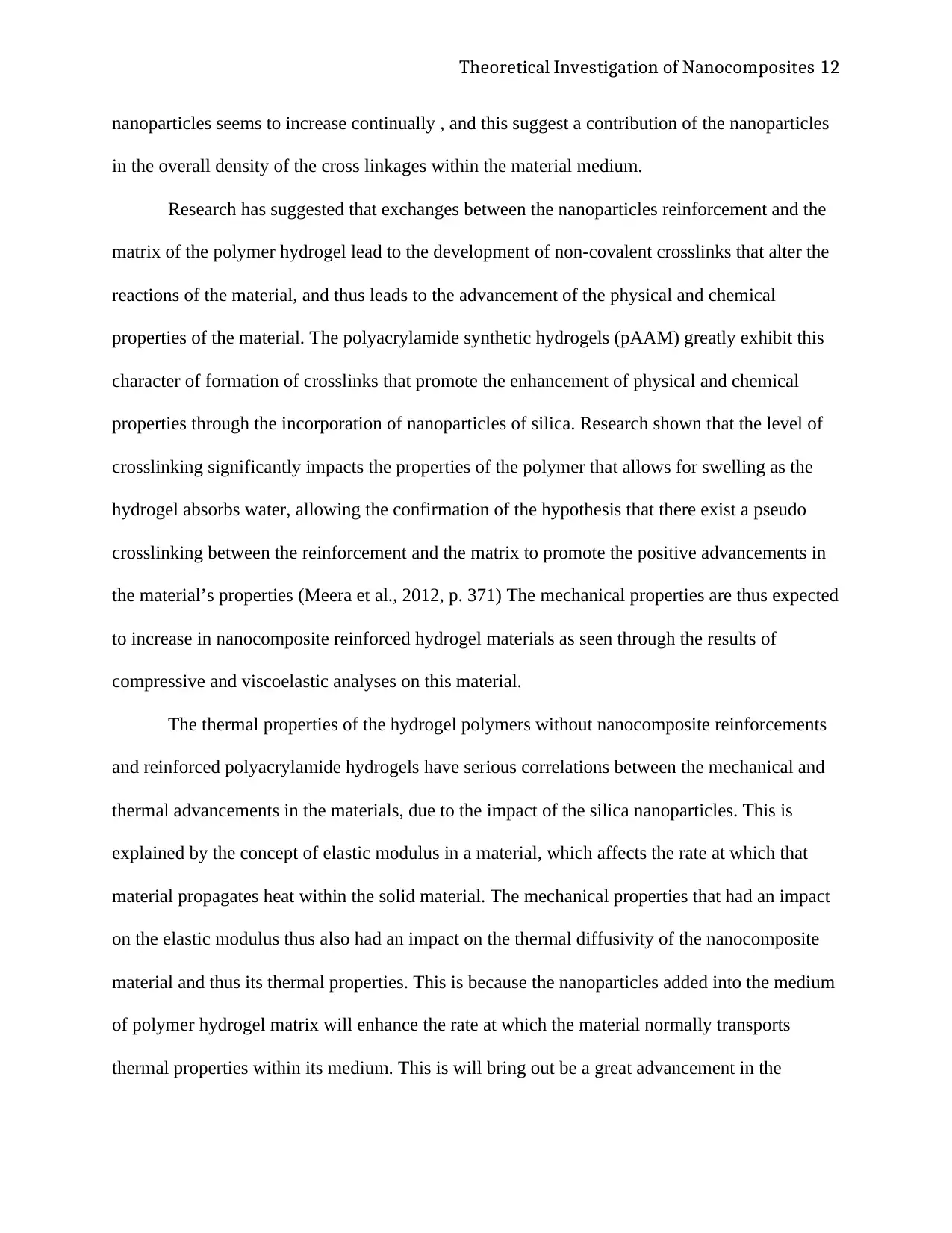
Theoretical Investigation of Nanocomposites 12
nanoparticles seems to increase continually , and this suggest a contribution of the nanoparticles
in the overall density of the cross linkages within the material medium.
Research has suggested that exchanges between the nanoparticles reinforcement and the
matrix of the polymer hydrogel lead to the development of non-covalent crosslinks that alter the
reactions of the material, and thus leads to the advancement of the physical and chemical
properties of the material. The polyacrylamide synthetic hydrogels (pAAM) greatly exhibit this
character of formation of crosslinks that promote the enhancement of physical and chemical
properties through the incorporation of nanoparticles of silica. Research shown that the level of
crosslinking significantly impacts the properties of the polymer that allows for swelling as the
hydrogel absorbs water, allowing the confirmation of the hypothesis that there exist a pseudo
crosslinking between the reinforcement and the matrix to promote the positive advancements in
the material’s properties (Meera et al., 2012, p. 371) The mechanical properties are thus expected
to increase in nanocomposite reinforced hydrogel materials as seen through the results of
compressive and viscoelastic analyses on this material.
The thermal properties of the hydrogel polymers without nanocomposite reinforcements
and reinforced polyacrylamide hydrogels have serious correlations between the mechanical and
thermal advancements in the materials, due to the impact of the silica nanoparticles. This is
explained by the concept of elastic modulus in a material, which affects the rate at which that
material propagates heat within the solid material. The mechanical properties that had an impact
on the elastic modulus thus also had an impact on the thermal diffusivity of the nanocomposite
material and thus its thermal properties. This is because the nanoparticles added into the medium
of polymer hydrogel matrix will enhance the rate at which the material normally transports
thermal properties within its medium. This is will bring out be a great advancement in the
nanoparticles seems to increase continually , and this suggest a contribution of the nanoparticles
in the overall density of the cross linkages within the material medium.
Research has suggested that exchanges between the nanoparticles reinforcement and the
matrix of the polymer hydrogel lead to the development of non-covalent crosslinks that alter the
reactions of the material, and thus leads to the advancement of the physical and chemical
properties of the material. The polyacrylamide synthetic hydrogels (pAAM) greatly exhibit this
character of formation of crosslinks that promote the enhancement of physical and chemical
properties through the incorporation of nanoparticles of silica. Research shown that the level of
crosslinking significantly impacts the properties of the polymer that allows for swelling as the
hydrogel absorbs water, allowing the confirmation of the hypothesis that there exist a pseudo
crosslinking between the reinforcement and the matrix to promote the positive advancements in
the material’s properties (Meera et al., 2012, p. 371) The mechanical properties are thus expected
to increase in nanocomposite reinforced hydrogel materials as seen through the results of
compressive and viscoelastic analyses on this material.
The thermal properties of the hydrogel polymers without nanocomposite reinforcements
and reinforced polyacrylamide hydrogels have serious correlations between the mechanical and
thermal advancements in the materials, due to the impact of the silica nanoparticles. This is
explained by the concept of elastic modulus in a material, which affects the rate at which that
material propagates heat within the solid material. The mechanical properties that had an impact
on the elastic modulus thus also had an impact on the thermal diffusivity of the nanocomposite
material and thus its thermal properties. This is because the nanoparticles added into the medium
of polymer hydrogel matrix will enhance the rate at which the material normally transports
thermal properties within its medium. This is will bring out be a great advancement in the
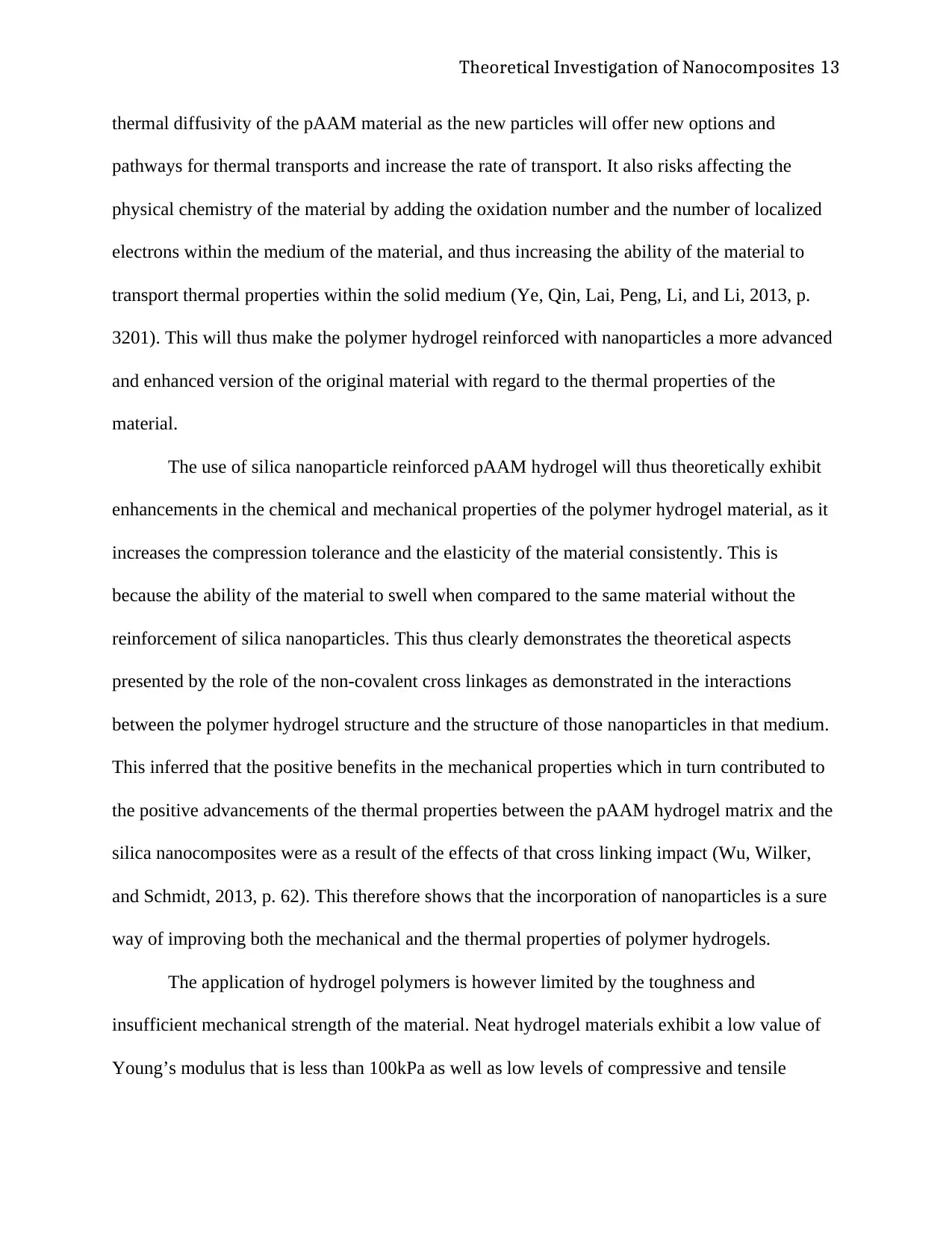
Theoretical Investigation of Nanocomposites 13
thermal diffusivity of the pAAM material as the new particles will offer new options and
pathways for thermal transports and increase the rate of transport. It also risks affecting the
physical chemistry of the material by adding the oxidation number and the number of localized
electrons within the medium of the material, and thus increasing the ability of the material to
transport thermal properties within the solid medium (Ye, Qin, Lai, Peng, Li, and Li, 2013, p.
3201). This will thus make the polymer hydrogel reinforced with nanoparticles a more advanced
and enhanced version of the original material with regard to the thermal properties of the
material.
The use of silica nanoparticle reinforced pAAM hydrogel will thus theoretically exhibit
enhancements in the chemical and mechanical properties of the polymer hydrogel material, as it
increases the compression tolerance and the elasticity of the material consistently. This is
because the ability of the material to swell when compared to the same material without the
reinforcement of silica nanoparticles. This thus clearly demonstrates the theoretical aspects
presented by the role of the non-covalent cross linkages as demonstrated in the interactions
between the polymer hydrogel structure and the structure of those nanoparticles in that medium.
This inferred that the positive benefits in the mechanical properties which in turn contributed to
the positive advancements of the thermal properties between the pAAM hydrogel matrix and the
silica nanocomposites were as a result of the effects of that cross linking impact (Wu, Wilker,
and Schmidt, 2013, p. 62). This therefore shows that the incorporation of nanoparticles is a sure
way of improving both the mechanical and the thermal properties of polymer hydrogels.
The application of hydrogel polymers is however limited by the toughness and
insufficient mechanical strength of the material. Neat hydrogel materials exhibit a low value of
Young’s modulus that is less than 100kPa as well as low levels of compressive and tensile
thermal diffusivity of the pAAM material as the new particles will offer new options and
pathways for thermal transports and increase the rate of transport. It also risks affecting the
physical chemistry of the material by adding the oxidation number and the number of localized
electrons within the medium of the material, and thus increasing the ability of the material to
transport thermal properties within the solid medium (Ye, Qin, Lai, Peng, Li, and Li, 2013, p.
3201). This will thus make the polymer hydrogel reinforced with nanoparticles a more advanced
and enhanced version of the original material with regard to the thermal properties of the
material.
The use of silica nanoparticle reinforced pAAM hydrogel will thus theoretically exhibit
enhancements in the chemical and mechanical properties of the polymer hydrogel material, as it
increases the compression tolerance and the elasticity of the material consistently. This is
because the ability of the material to swell when compared to the same material without the
reinforcement of silica nanoparticles. This thus clearly demonstrates the theoretical aspects
presented by the role of the non-covalent cross linkages as demonstrated in the interactions
between the polymer hydrogel structure and the structure of those nanoparticles in that medium.
This inferred that the positive benefits in the mechanical properties which in turn contributed to
the positive advancements of the thermal properties between the pAAM hydrogel matrix and the
silica nanocomposites were as a result of the effects of that cross linking impact (Wu, Wilker,
and Schmidt, 2013, p. 62). This therefore shows that the incorporation of nanoparticles is a sure
way of improving both the mechanical and the thermal properties of polymer hydrogels.
The application of hydrogel polymers is however limited by the toughness and
insufficient mechanical strength of the material. Neat hydrogel materials exhibit a low value of
Young’s modulus that is less than 100kPa as well as low levels of compressive and tensile
Paraphrase This Document
Need a fresh take? Get an instant paraphrase of this document with our AI Paraphraser
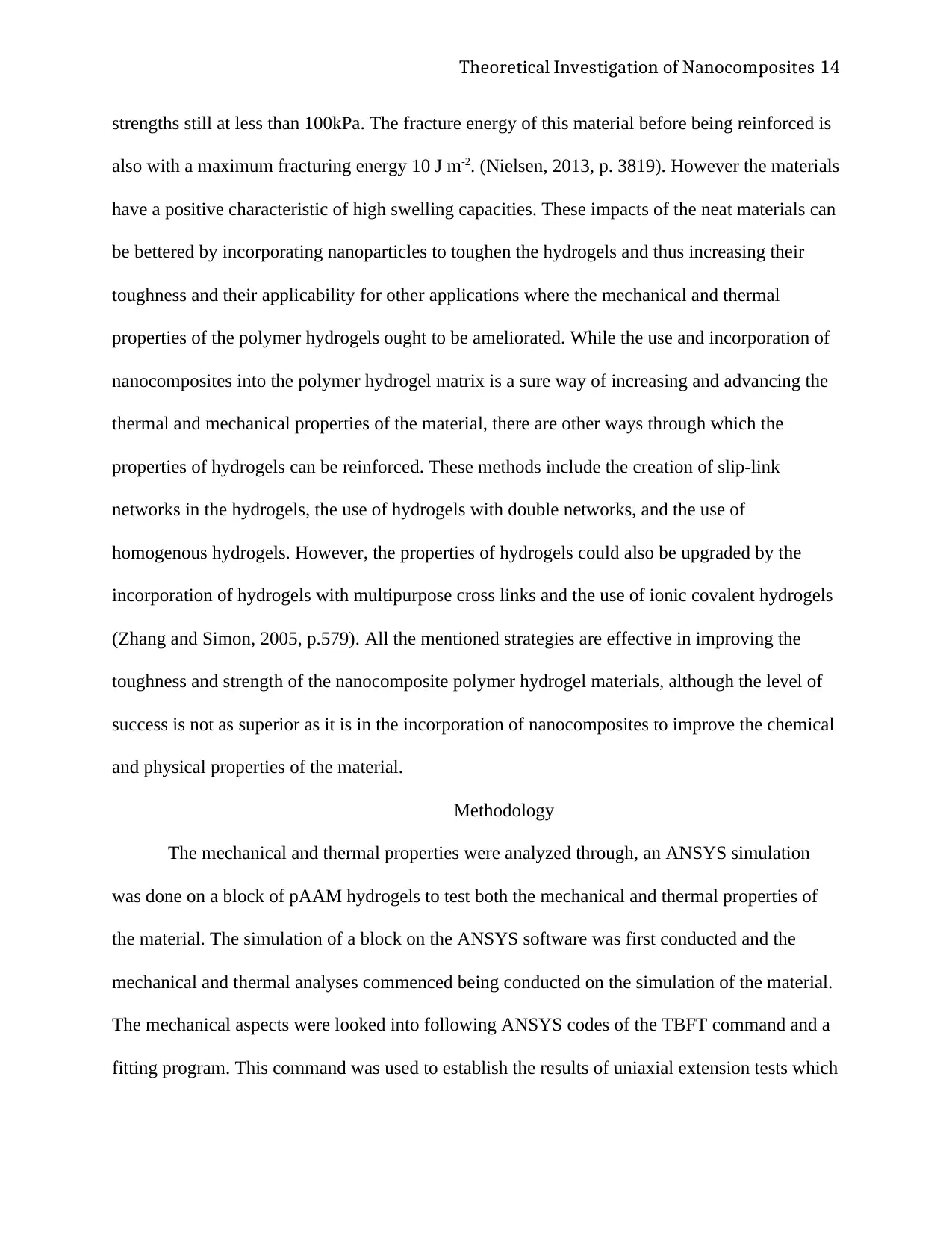
Theoretical Investigation of Nanocomposites 14
strengths still at less than 100kPa. The fracture energy of this material before being reinforced is
also with a maximum fracturing energy 10 J m-2. (Nielsen, 2013, p. 3819). However the materials
have a positive characteristic of high swelling capacities. These impacts of the neat materials can
be bettered by incorporating nanoparticles to toughen the hydrogels and thus increasing their
toughness and their applicability for other applications where the mechanical and thermal
properties of the polymer hydrogels ought to be ameliorated. While the use and incorporation of
nanocomposites into the polymer hydrogel matrix is a sure way of increasing and advancing the
thermal and mechanical properties of the material, there are other ways through which the
properties of hydrogels can be reinforced. These methods include the creation of slip-link
networks in the hydrogels, the use of hydrogels with double networks, and the use of
homogenous hydrogels. However, the properties of hydrogels could also be upgraded by the
incorporation of hydrogels with multipurpose cross links and the use of ionic covalent hydrogels
(Zhang and Simon, 2005, p.579). All the mentioned strategies are effective in improving the
toughness and strength of the nanocomposite polymer hydrogel materials, although the level of
success is not as superior as it is in the incorporation of nanocomposites to improve the chemical
and physical properties of the material.
Methodology
The mechanical and thermal properties were analyzed through, an ANSYS simulation
was done on a block of pAAM hydrogels to test both the mechanical and thermal properties of
the material. The simulation of a block on the ANSYS software was first conducted and the
mechanical and thermal analyses commenced being conducted on the simulation of the material.
The mechanical aspects were looked into following ANSYS codes of the TBFT command and a
fitting program. This command was used to establish the results of uniaxial extension tests which
strengths still at less than 100kPa. The fracture energy of this material before being reinforced is
also with a maximum fracturing energy 10 J m-2. (Nielsen, 2013, p. 3819). However the materials
have a positive characteristic of high swelling capacities. These impacts of the neat materials can
be bettered by incorporating nanoparticles to toughen the hydrogels and thus increasing their
toughness and their applicability for other applications where the mechanical and thermal
properties of the polymer hydrogels ought to be ameliorated. While the use and incorporation of
nanocomposites into the polymer hydrogel matrix is a sure way of increasing and advancing the
thermal and mechanical properties of the material, there are other ways through which the
properties of hydrogels can be reinforced. These methods include the creation of slip-link
networks in the hydrogels, the use of hydrogels with double networks, and the use of
homogenous hydrogels. However, the properties of hydrogels could also be upgraded by the
incorporation of hydrogels with multipurpose cross links and the use of ionic covalent hydrogels
(Zhang and Simon, 2005, p.579). All the mentioned strategies are effective in improving the
toughness and strength of the nanocomposite polymer hydrogel materials, although the level of
success is not as superior as it is in the incorporation of nanocomposites to improve the chemical
and physical properties of the material.
Methodology
The mechanical and thermal properties were analyzed through, an ANSYS simulation
was done on a block of pAAM hydrogels to test both the mechanical and thermal properties of
the material. The simulation of a block on the ANSYS software was first conducted and the
mechanical and thermal analyses commenced being conducted on the simulation of the material.
The mechanical aspects were looked into following ANSYS codes of the TBFT command and a
fitting program. This command was used to establish the results of uniaxial extension tests which
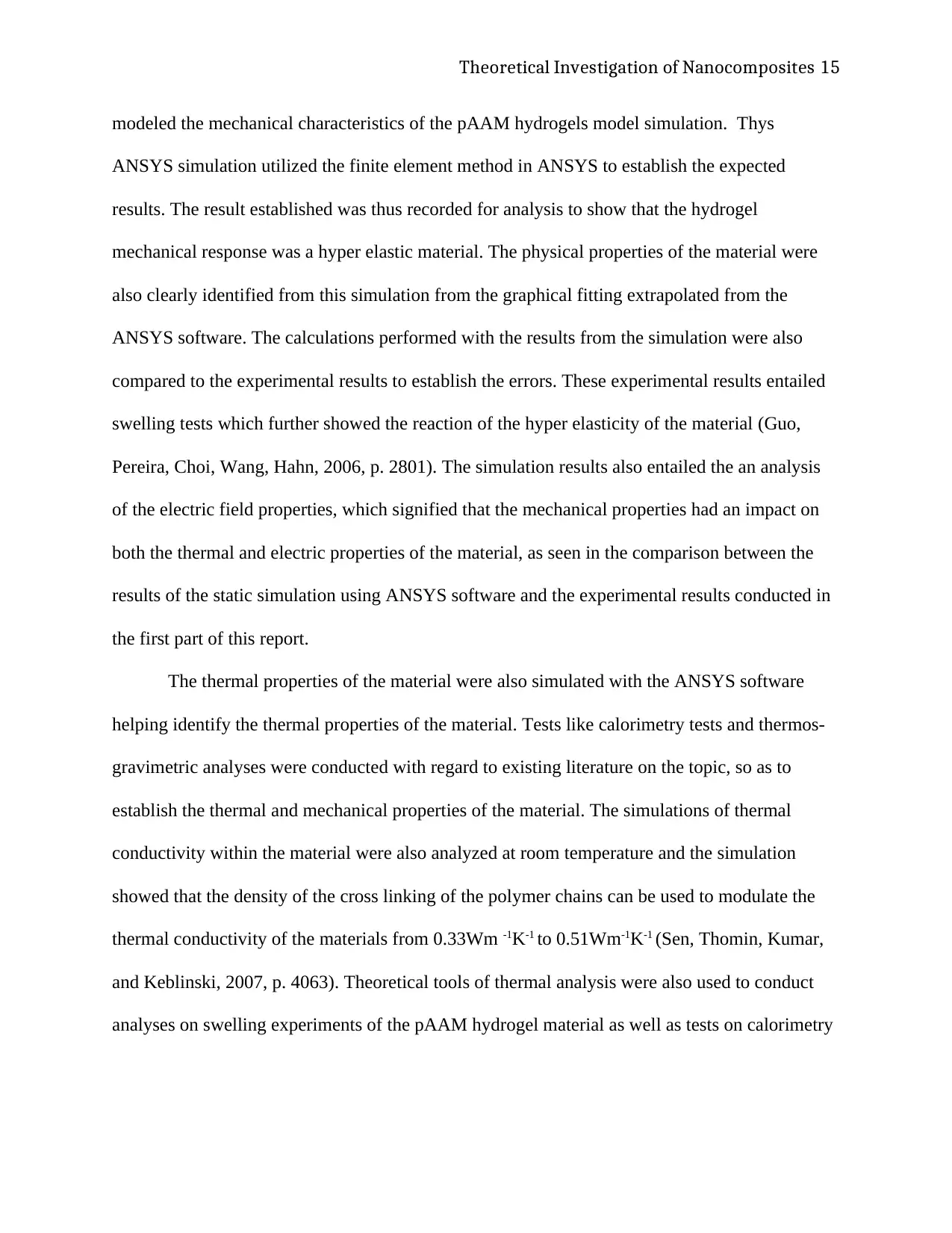
Theoretical Investigation of Nanocomposites 15
modeled the mechanical characteristics of the pAAM hydrogels model simulation. Thys
ANSYS simulation utilized the finite element method in ANSYS to establish the expected
results. The result established was thus recorded for analysis to show that the hydrogel
mechanical response was a hyper elastic material. The physical properties of the material were
also clearly identified from this simulation from the graphical fitting extrapolated from the
ANSYS software. The calculations performed with the results from the simulation were also
compared to the experimental results to establish the errors. These experimental results entailed
swelling tests which further showed the reaction of the hyper elasticity of the material (Guo,
Pereira, Choi, Wang, Hahn, 2006, p. 2801). The simulation results also entailed the an analysis
of the electric field properties, which signified that the mechanical properties had an impact on
both the thermal and electric properties of the material, as seen in the comparison between the
results of the static simulation using ANSYS software and the experimental results conducted in
the first part of this report.
The thermal properties of the material were also simulated with the ANSYS software
helping identify the thermal properties of the material. Tests like calorimetry tests and thermos-
gravimetric analyses were conducted with regard to existing literature on the topic, so as to
establish the thermal and mechanical properties of the material. The simulations of thermal
conductivity within the material were also analyzed at room temperature and the simulation
showed that the density of the cross linking of the polymer chains can be used to modulate the
thermal conductivity of the materials from 0.33Wm -1K-1 to 0.51Wm-1K-1 (Sen, Thomin, Kumar,
and Keblinski, 2007, p. 4063). Theoretical tools of thermal analysis were also used to conduct
analyses on swelling experiments of the pAAM hydrogel material as well as tests on calorimetry
modeled the mechanical characteristics of the pAAM hydrogels model simulation. Thys
ANSYS simulation utilized the finite element method in ANSYS to establish the expected
results. The result established was thus recorded for analysis to show that the hydrogel
mechanical response was a hyper elastic material. The physical properties of the material were
also clearly identified from this simulation from the graphical fitting extrapolated from the
ANSYS software. The calculations performed with the results from the simulation were also
compared to the experimental results to establish the errors. These experimental results entailed
swelling tests which further showed the reaction of the hyper elasticity of the material (Guo,
Pereira, Choi, Wang, Hahn, 2006, p. 2801). The simulation results also entailed the an analysis
of the electric field properties, which signified that the mechanical properties had an impact on
both the thermal and electric properties of the material, as seen in the comparison between the
results of the static simulation using ANSYS software and the experimental results conducted in
the first part of this report.
The thermal properties of the material were also simulated with the ANSYS software
helping identify the thermal properties of the material. Tests like calorimetry tests and thermos-
gravimetric analyses were conducted with regard to existing literature on the topic, so as to
establish the thermal and mechanical properties of the material. The simulations of thermal
conductivity within the material were also analyzed at room temperature and the simulation
showed that the density of the cross linking of the polymer chains can be used to modulate the
thermal conductivity of the materials from 0.33Wm -1K-1 to 0.51Wm-1K-1 (Sen, Thomin, Kumar,
and Keblinski, 2007, p. 4063). Theoretical tools of thermal analysis were also used to conduct
analyses on swelling experiments of the pAAM hydrogel material as well as tests on calorimetry
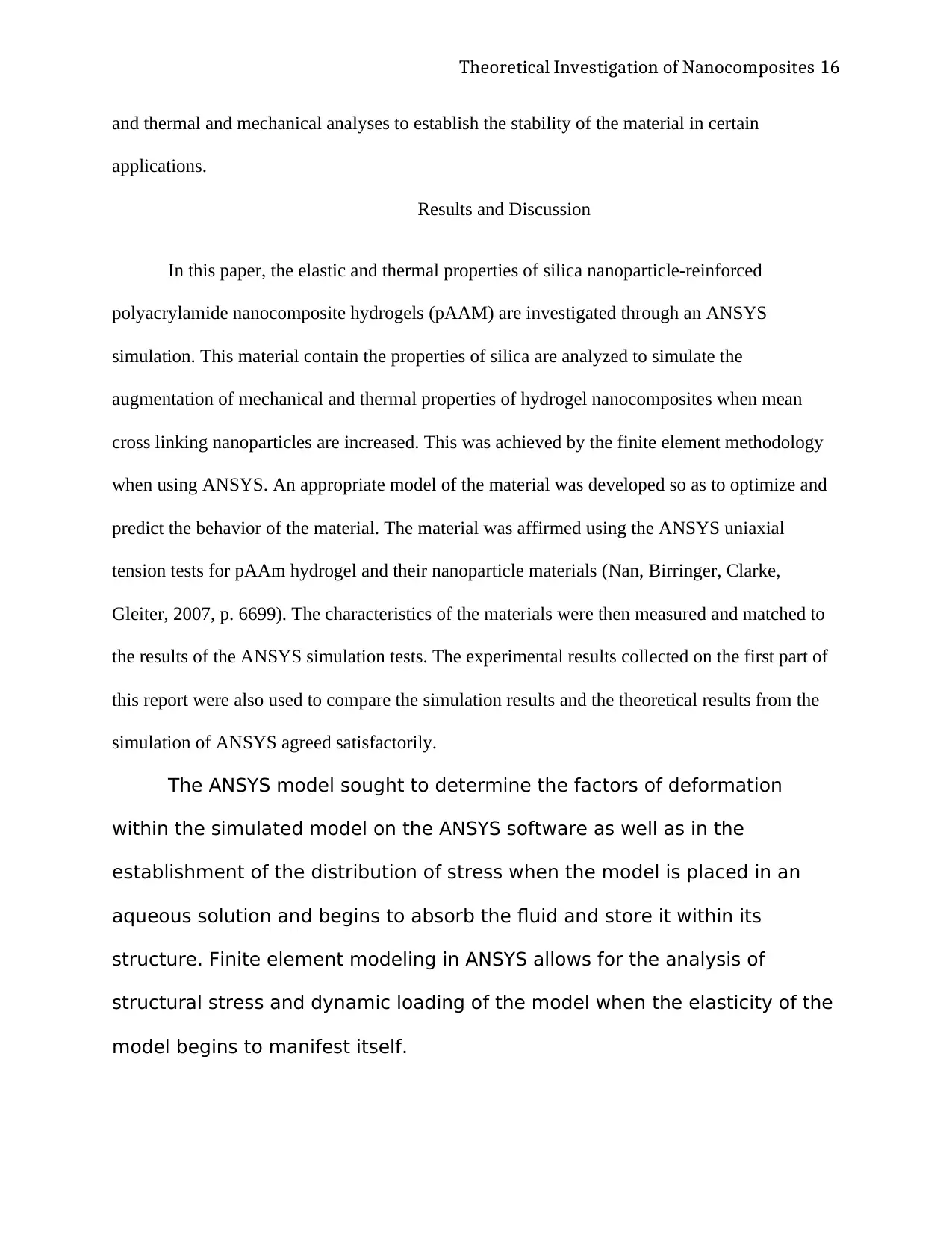
Theoretical Investigation of Nanocomposites 16
and thermal and mechanical analyses to establish the stability of the material in certain
applications.
Results and Discussion
In this paper, the elastic and thermal properties of silica nanoparticle-reinforced
polyacrylamide nanocomposite hydrogels (pAAM) are investigated through an ANSYS
simulation. This material contain the properties of silica are analyzed to simulate the
augmentation of mechanical and thermal properties of hydrogel nanocomposites when mean
cross linking nanoparticles are increased. This was achieved by the finite element methodology
when using ANSYS. An appropriate model of the material was developed so as to optimize and
predict the behavior of the material. The material was affirmed using the ANSYS uniaxial
tension tests for pAAm hydrogel and their nanoparticle materials (Nan, Birringer, Clarke,
Gleiter, 2007, p. 6699). The characteristics of the materials were then measured and matched to
the results of the ANSYS simulation tests. The experimental results collected on the first part of
this report were also used to compare the simulation results and the theoretical results from the
simulation of ANSYS agreed satisfactorily.
The ANSYS model sought to determine the factors of deformation
within the simulated model on the ANSYS software as well as in the
establishment of the distribution of stress when the model is placed in an
aqueous solution and begins to absorb the fluid and store it within its
structure. Finite element modeling in ANSYS allows for the analysis of
structural stress and dynamic loading of the model when the elasticity of the
model begins to manifest itself.
and thermal and mechanical analyses to establish the stability of the material in certain
applications.
Results and Discussion
In this paper, the elastic and thermal properties of silica nanoparticle-reinforced
polyacrylamide nanocomposite hydrogels (pAAM) are investigated through an ANSYS
simulation. This material contain the properties of silica are analyzed to simulate the
augmentation of mechanical and thermal properties of hydrogel nanocomposites when mean
cross linking nanoparticles are increased. This was achieved by the finite element methodology
when using ANSYS. An appropriate model of the material was developed so as to optimize and
predict the behavior of the material. The material was affirmed using the ANSYS uniaxial
tension tests for pAAm hydrogel and their nanoparticle materials (Nan, Birringer, Clarke,
Gleiter, 2007, p. 6699). The characteristics of the materials were then measured and matched to
the results of the ANSYS simulation tests. The experimental results collected on the first part of
this report were also used to compare the simulation results and the theoretical results from the
simulation of ANSYS agreed satisfactorily.
The ANSYS model sought to determine the factors of deformation
within the simulated model on the ANSYS software as well as in the
establishment of the distribution of stress when the model is placed in an
aqueous solution and begins to absorb the fluid and store it within its
structure. Finite element modeling in ANSYS allows for the analysis of
structural stress and dynamic loading of the model when the elasticity of the
model begins to manifest itself.
Secure Best Marks with AI Grader
Need help grading? Try our AI Grader for instant feedback on your assignments.
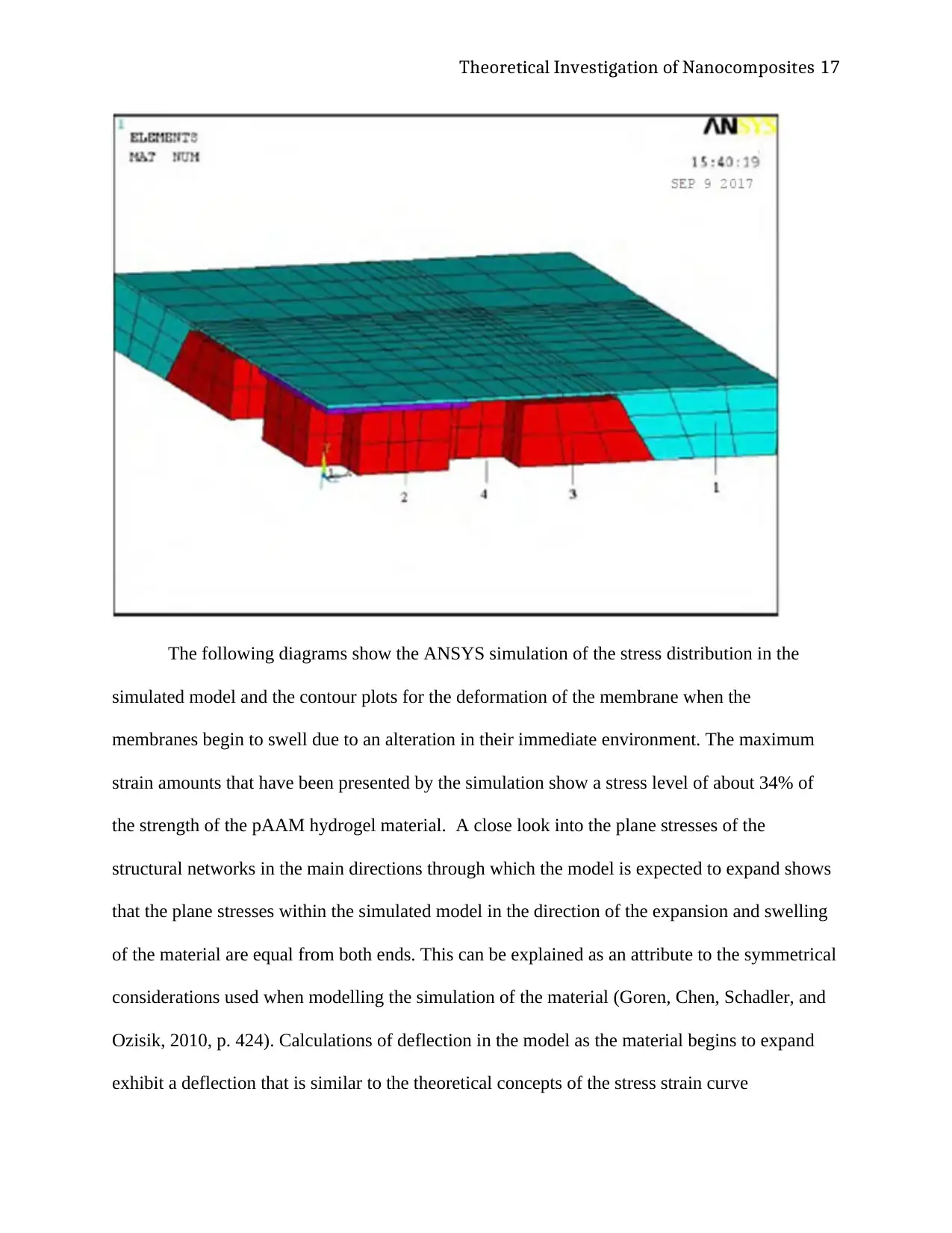
Theoretical Investigation of Nanocomposites 17
The following diagrams show the ANSYS simulation of the stress distribution in the
simulated model and the contour plots for the deformation of the membrane when the
membranes begin to swell due to an alteration in their immediate environment. The maximum
strain amounts that have been presented by the simulation show a stress level of about 34% of
the strength of the pAAM hydrogel material. A close look into the plane stresses of the
structural networks in the main directions through which the model is expected to expand shows
that the plane stresses within the simulated model in the direction of the expansion and swelling
of the material are equal from both ends. This can be explained as an attribute to the symmetrical
considerations used when modelling the simulation of the material (Goren, Chen, Schadler, and
Ozisik, 2010, p. 424). Calculations of deflection in the model as the material begins to expand
exhibit a deflection that is similar to the theoretical concepts of the stress strain curve
The following diagrams show the ANSYS simulation of the stress distribution in the
simulated model and the contour plots for the deformation of the membrane when the
membranes begin to swell due to an alteration in their immediate environment. The maximum
strain amounts that have been presented by the simulation show a stress level of about 34% of
the strength of the pAAM hydrogel material. A close look into the plane stresses of the
structural networks in the main directions through which the model is expected to expand shows
that the plane stresses within the simulated model in the direction of the expansion and swelling
of the material are equal from both ends. This can be explained as an attribute to the symmetrical
considerations used when modelling the simulation of the material (Goren, Chen, Schadler, and
Ozisik, 2010, p. 424). Calculations of deflection in the model as the material begins to expand
exhibit a deflection that is similar to the theoretical concepts of the stress strain curve
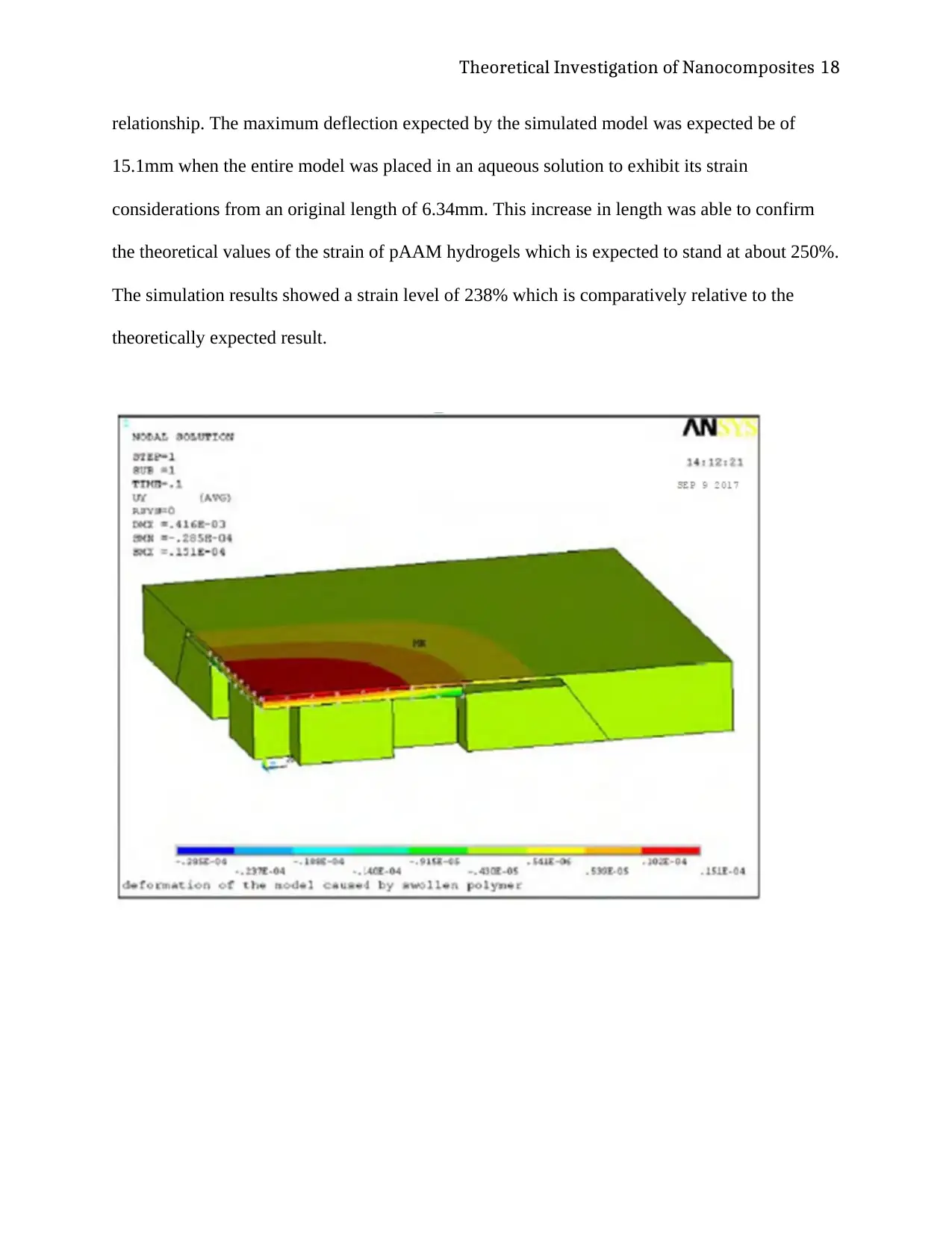
Theoretical Investigation of Nanocomposites 18
relationship. The maximum deflection expected by the simulated model was expected be of
15.1mm when the entire model was placed in an aqueous solution to exhibit its strain
considerations from an original length of 6.34mm. This increase in length was able to confirm
the theoretical values of the strain of pAAM hydrogels which is expected to stand at about 250%.
The simulation results showed a strain level of 238% which is comparatively relative to the
theoretically expected result.
relationship. The maximum deflection expected by the simulated model was expected be of
15.1mm when the entire model was placed in an aqueous solution to exhibit its strain
considerations from an original length of 6.34mm. This increase in length was able to confirm
the theoretical values of the strain of pAAM hydrogels which is expected to stand at about 250%.
The simulation results showed a strain level of 238% which is comparatively relative to the
theoretically expected result.
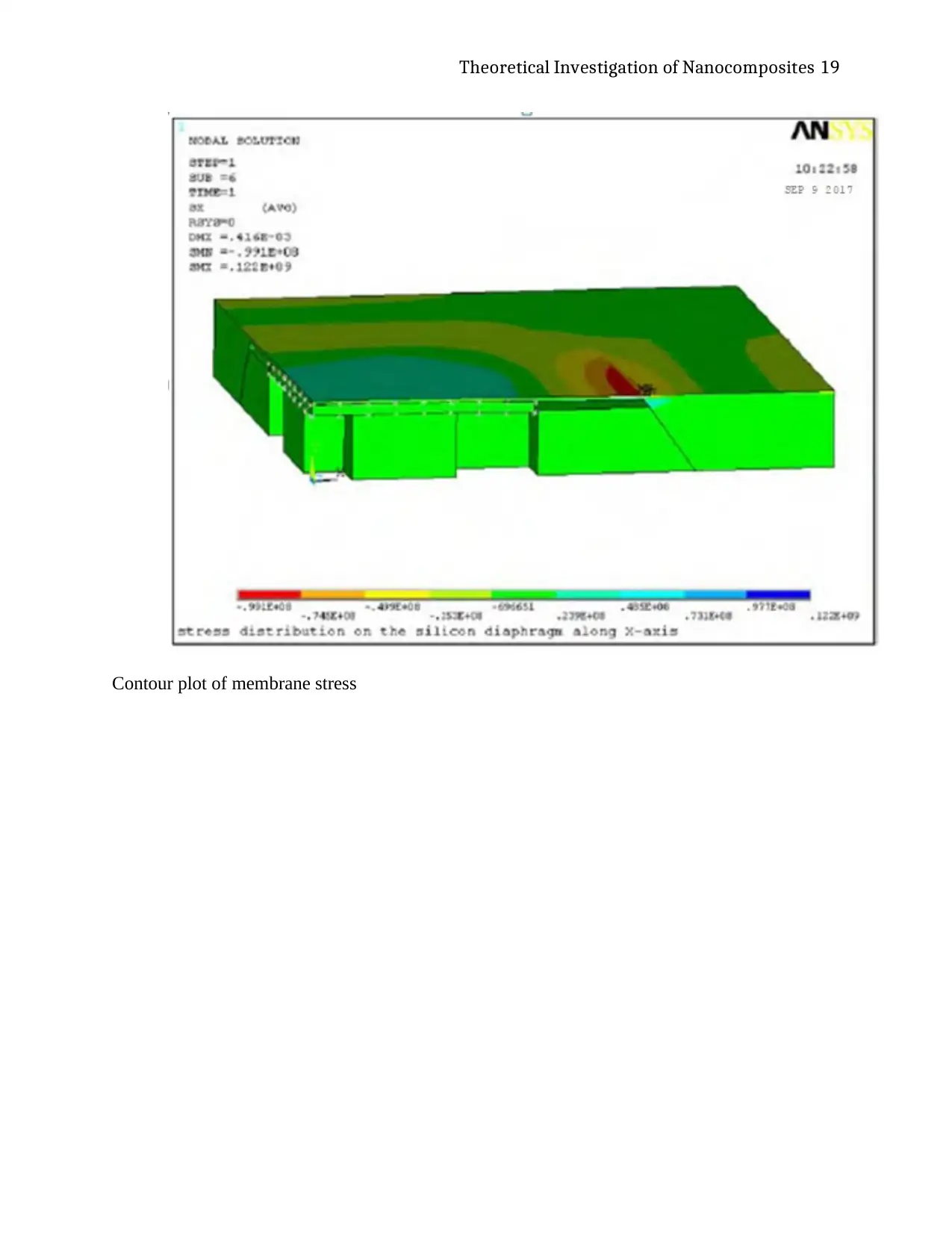
Theoretical Investigation of Nanocomposites 19
Contour plot of membrane stress
Contour plot of membrane stress
Paraphrase This Document
Need a fresh take? Get an instant paraphrase of this document with our AI Paraphraser
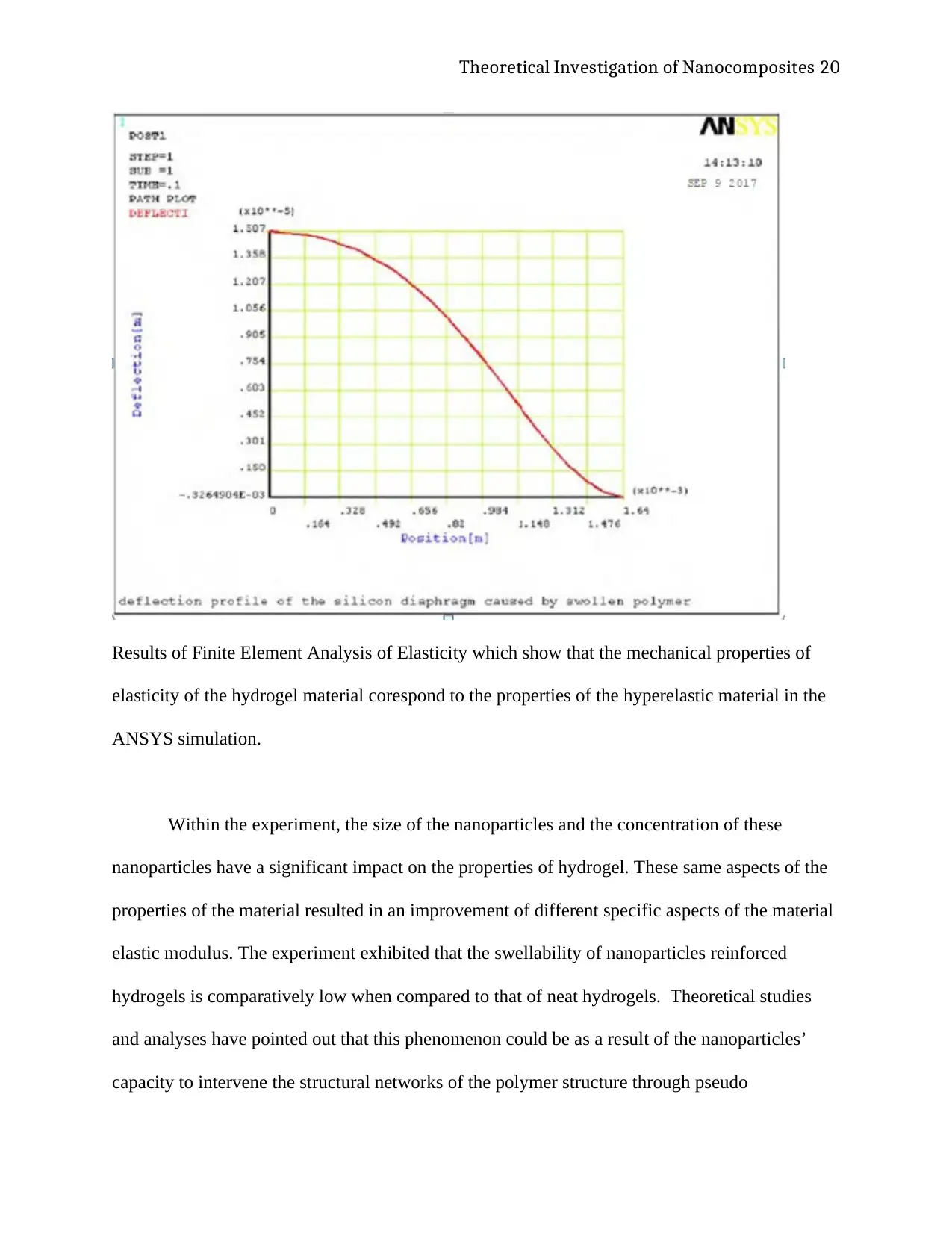
Theoretical Investigation of Nanocomposites 20
Results of Finite Element Analysis of Elasticity which show that the mechanical properties of
elasticity of the hydrogel material corespond to the properties of the hyperelastic material in the
ANSYS simulation.
Within the experiment, the size of the nanoparticles and the concentration of these
nanoparticles have a significant impact on the properties of hydrogel. These same aspects of the
properties of the material resulted in an improvement of different specific aspects of the material
elastic modulus. The experiment exhibited that the swellability of nanoparticles reinforced
hydrogels is comparatively low when compared to that of neat hydrogels. Theoretical studies
and analyses have pointed out that this phenomenon could be as a result of the nanoparticles’
capacity to intervene the structural networks of the polymer structure through pseudo
Results of Finite Element Analysis of Elasticity which show that the mechanical properties of
elasticity of the hydrogel material corespond to the properties of the hyperelastic material in the
ANSYS simulation.
Within the experiment, the size of the nanoparticles and the concentration of these
nanoparticles have a significant impact on the properties of hydrogel. These same aspects of the
properties of the material resulted in an improvement of different specific aspects of the material
elastic modulus. The experiment exhibited that the swellability of nanoparticles reinforced
hydrogels is comparatively low when compared to that of neat hydrogels. Theoretical studies
and analyses have pointed out that this phenomenon could be as a result of the nanoparticles’
capacity to intervene the structural networks of the polymer structure through pseudo
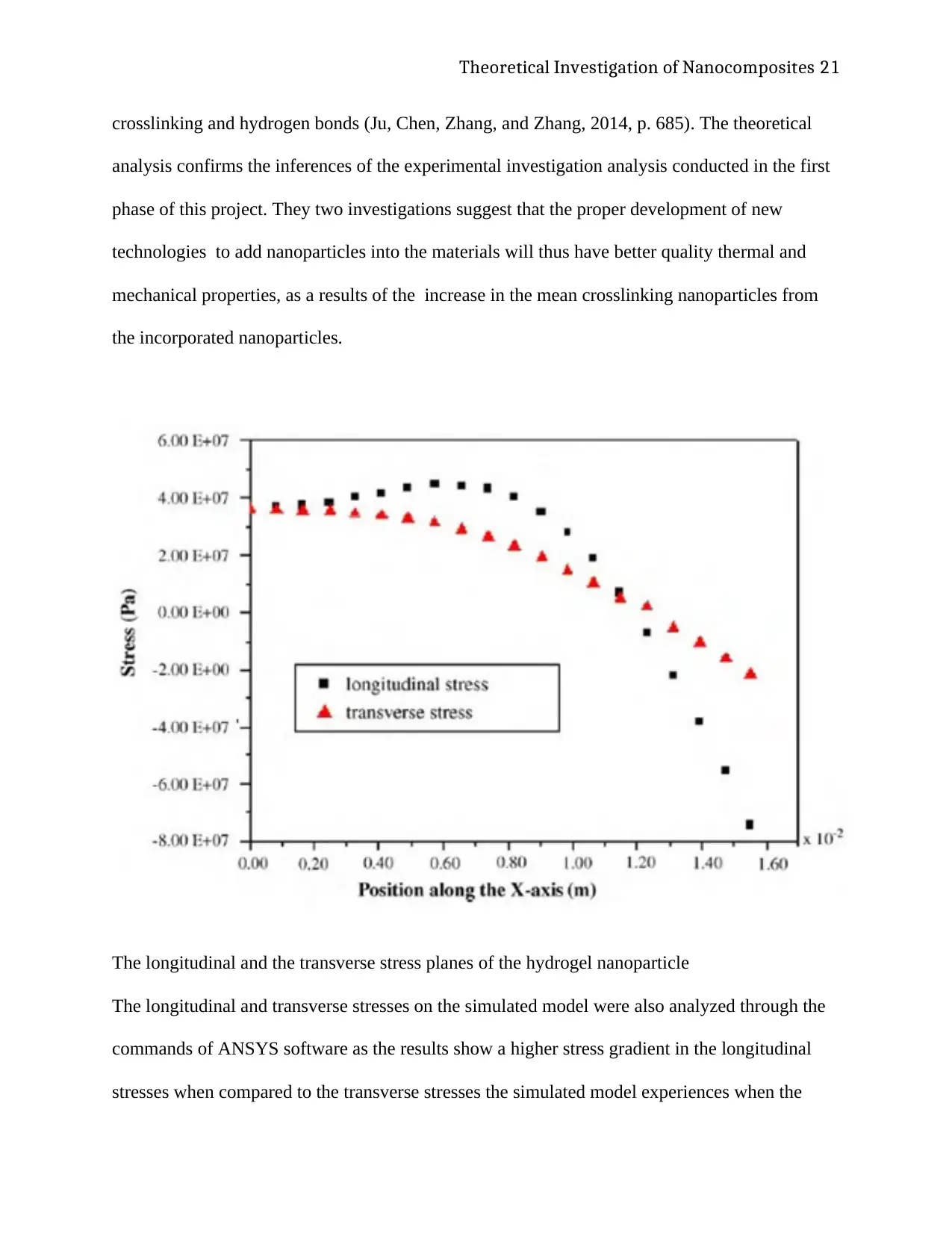
Theoretical Investigation of Nanocomposites 21
crosslinking and hydrogen bonds (Ju, Chen, Zhang, and Zhang, 2014, p. 685). The theoretical
analysis confirms the inferences of the experimental investigation analysis conducted in the first
phase of this project. They two investigations suggest that the proper development of new
technologies to add nanoparticles into the materials will thus have better quality thermal and
mechanical properties, as a results of the increase in the mean crosslinking nanoparticles from
the incorporated nanoparticles.
The longitudinal and the transverse stress planes of the hydrogel nanoparticle
The longitudinal and transverse stresses on the simulated model were also analyzed through the
commands of ANSYS software as the results show a higher stress gradient in the longitudinal
stresses when compared to the transverse stresses the simulated model experiences when the
crosslinking and hydrogen bonds (Ju, Chen, Zhang, and Zhang, 2014, p. 685). The theoretical
analysis confirms the inferences of the experimental investigation analysis conducted in the first
phase of this project. They two investigations suggest that the proper development of new
technologies to add nanoparticles into the materials will thus have better quality thermal and
mechanical properties, as a results of the increase in the mean crosslinking nanoparticles from
the incorporated nanoparticles.
The longitudinal and the transverse stress planes of the hydrogel nanoparticle
The longitudinal and transverse stresses on the simulated model were also analyzed through the
commands of ANSYS software as the results show a higher stress gradient in the longitudinal
stresses when compared to the transverse stresses the simulated model experiences when the
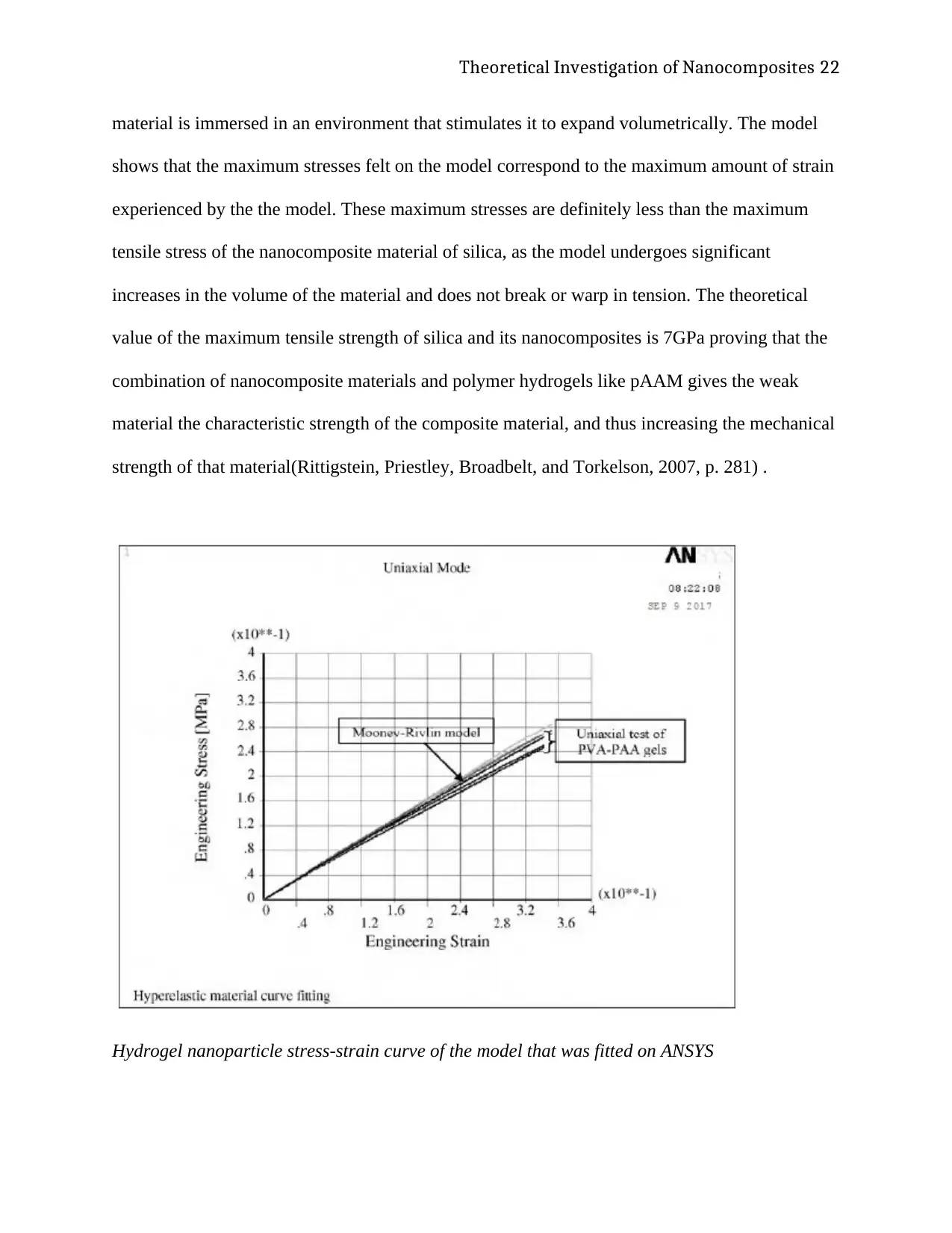
Theoretical Investigation of Nanocomposites 22
material is immersed in an environment that stimulates it to expand volumetrically. The model
shows that the maximum stresses felt on the model correspond to the maximum amount of strain
experienced by the the model. These maximum stresses are definitely less than the maximum
tensile stress of the nanocomposite material of silica, as the model undergoes significant
increases in the volume of the material and does not break or warp in tension. The theoretical
value of the maximum tensile strength of silica and its nanocomposites is 7GPa proving that the
combination of nanocomposite materials and polymer hydrogels like pAAM gives the weak
material the characteristic strength of the composite material, and thus increasing the mechanical
strength of that material(Rittigstein, Priestley, Broadbelt, and Torkelson, 2007, p. 281) .
Hydrogel nanoparticle stress-strain curve of the model that was fitted on ANSYS
material is immersed in an environment that stimulates it to expand volumetrically. The model
shows that the maximum stresses felt on the model correspond to the maximum amount of strain
experienced by the the model. These maximum stresses are definitely less than the maximum
tensile stress of the nanocomposite material of silica, as the model undergoes significant
increases in the volume of the material and does not break or warp in tension. The theoretical
value of the maximum tensile strength of silica and its nanocomposites is 7GPa proving that the
combination of nanocomposite materials and polymer hydrogels like pAAM gives the weak
material the characteristic strength of the composite material, and thus increasing the mechanical
strength of that material(Rittigstein, Priestley, Broadbelt, and Torkelson, 2007, p. 281) .
Hydrogel nanoparticle stress-strain curve of the model that was fitted on ANSYS
Secure Best Marks with AI Grader
Need help grading? Try our AI Grader for instant feedback on your assignments.
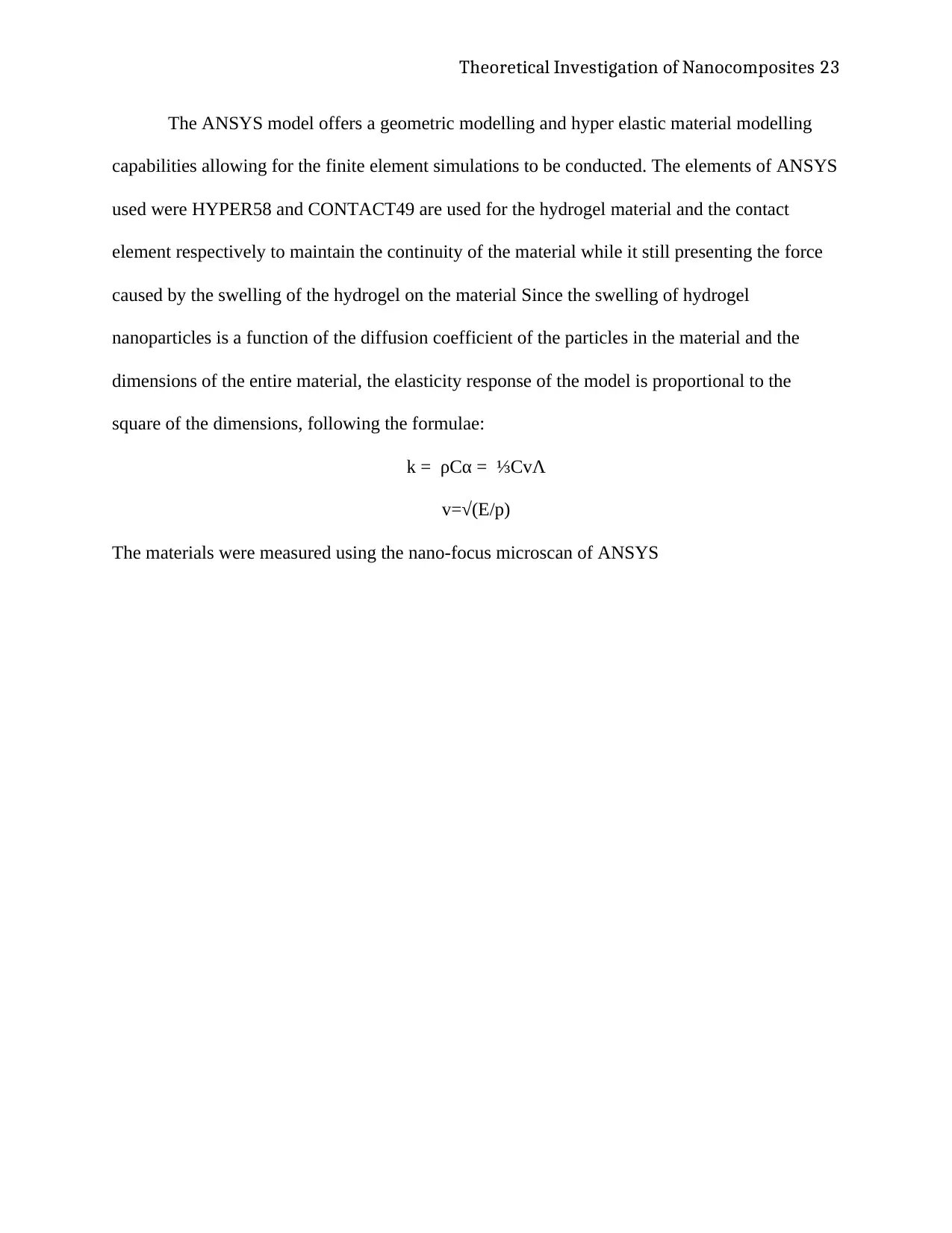
Theoretical Investigation of Nanocomposites 23
The ANSYS model offers a geometric modelling and hyper elastic material modelling
capabilities allowing for the finite element simulations to be conducted. The elements of ANSYS
used were HYPER58 and CONTACT49 are used for the hydrogel material and the contact
element respectively to maintain the continuity of the material while it still presenting the force
caused by the swelling of the hydrogel on the material Since the swelling of hydrogel
nanoparticles is a function of the diffusion coefficient of the particles in the material and the
dimensions of the entire material, the elasticity response of the model is proportional to the
square of the dimensions, following the formulae:
k = ρCα = ⅓CvΛ
v=√(E/p)
The materials were measured using the nano-focus microscan of ANSYS
The ANSYS model offers a geometric modelling and hyper elastic material modelling
capabilities allowing for the finite element simulations to be conducted. The elements of ANSYS
used were HYPER58 and CONTACT49 are used for the hydrogel material and the contact
element respectively to maintain the continuity of the material while it still presenting the force
caused by the swelling of the hydrogel on the material Since the swelling of hydrogel
nanoparticles is a function of the diffusion coefficient of the particles in the material and the
dimensions of the entire material, the elasticity response of the model is proportional to the
square of the dimensions, following the formulae:
k = ρCα = ⅓CvΛ
v=√(E/p)
The materials were measured using the nano-focus microscan of ANSYS
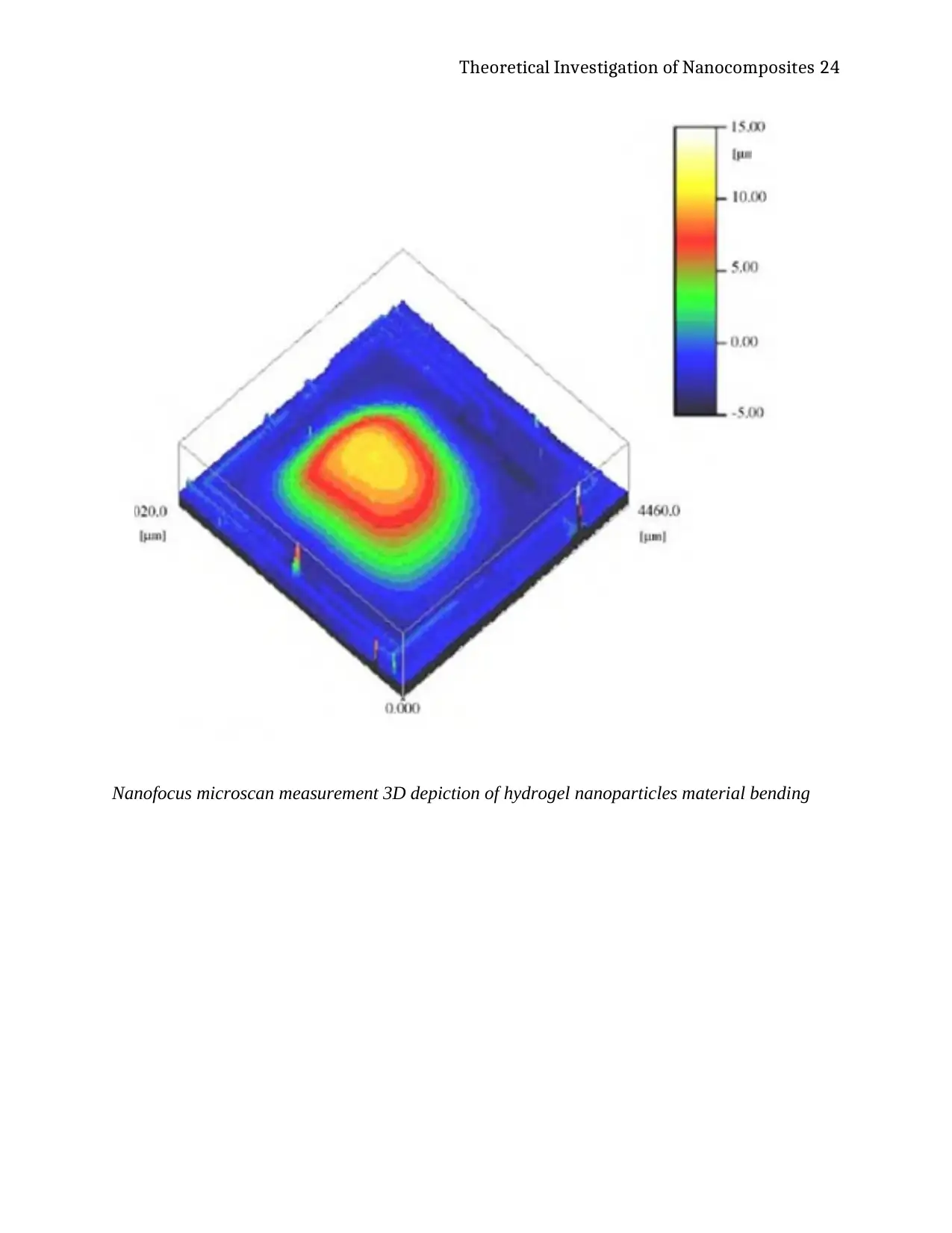
Theoretical Investigation of Nanocomposites 24
Nanofocus microscan measurement 3D depiction of hydrogel nanoparticles material bending
Nanofocus microscan measurement 3D depiction of hydrogel nanoparticles material bending
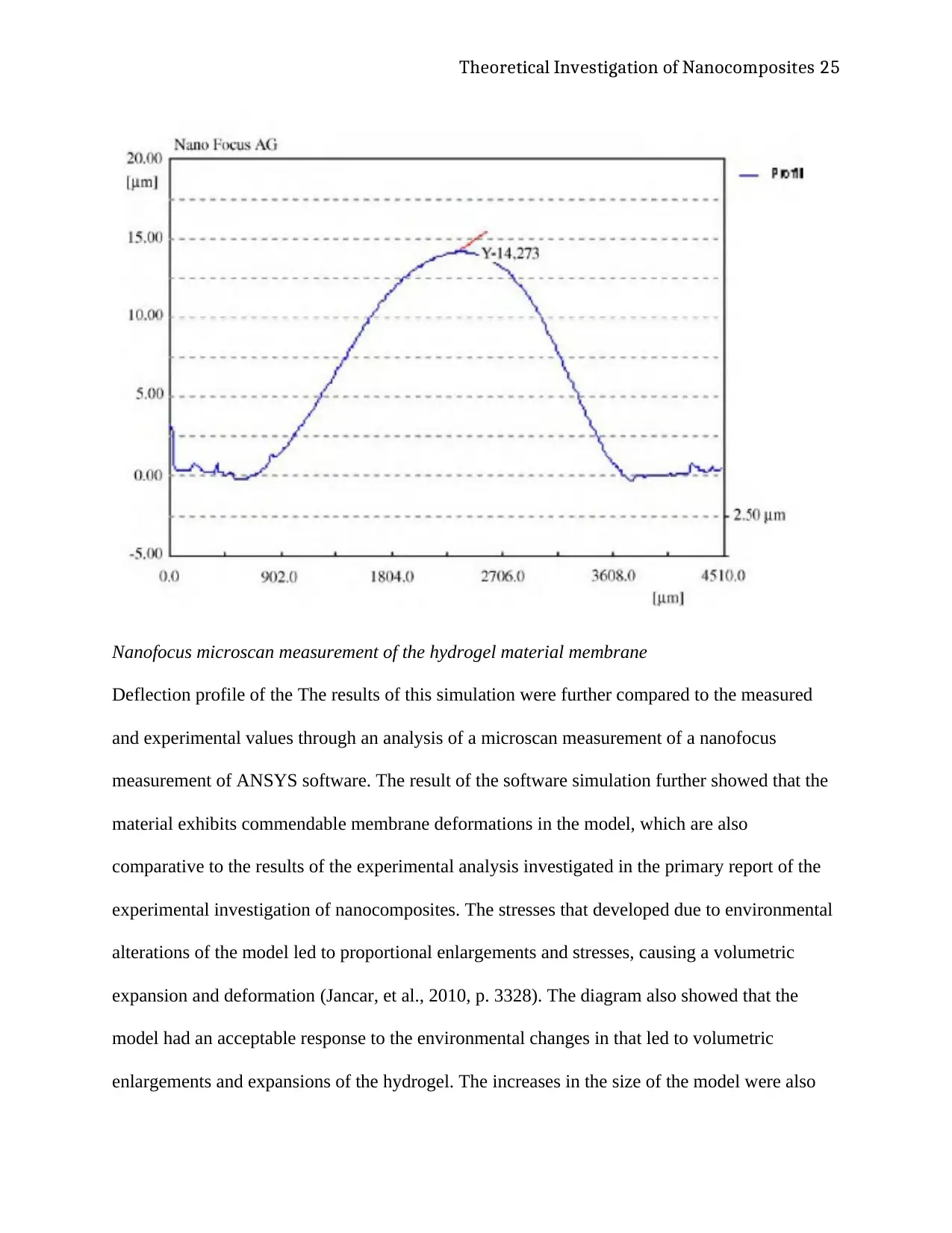
Theoretical Investigation of Nanocomposites 25
Nanofocus microscan measurement of the hydrogel material membrane
Deflection profile of the The results of this simulation were further compared to the measured
and experimental values through an analysis of a microscan measurement of a nanofocus
measurement of ANSYS software. The result of the software simulation further showed that the
material exhibits commendable membrane deformations in the model, which are also
comparative to the results of the experimental analysis investigated in the primary report of the
experimental investigation of nanocomposites. The stresses that developed due to environmental
alterations of the model led to proportional enlargements and stresses, causing a volumetric
expansion and deformation (Jancar, et al., 2010, p. 3328). The diagram also showed that the
model had an acceptable response to the environmental changes in that led to volumetric
enlargements and expansions of the hydrogel. The increases in the size of the model were also
Nanofocus microscan measurement of the hydrogel material membrane
Deflection profile of the The results of this simulation were further compared to the measured
and experimental values through an analysis of a microscan measurement of a nanofocus
measurement of ANSYS software. The result of the software simulation further showed that the
material exhibits commendable membrane deformations in the model, which are also
comparative to the results of the experimental analysis investigated in the primary report of the
experimental investigation of nanocomposites. The stresses that developed due to environmental
alterations of the model led to proportional enlargements and stresses, causing a volumetric
expansion and deformation (Jancar, et al., 2010, p. 3328). The diagram also showed that the
model had an acceptable response to the environmental changes in that led to volumetric
enlargements and expansions of the hydrogel. The increases in the size of the model were also
Paraphrase This Document
Need a fresh take? Get an instant paraphrase of this document with our AI Paraphraser
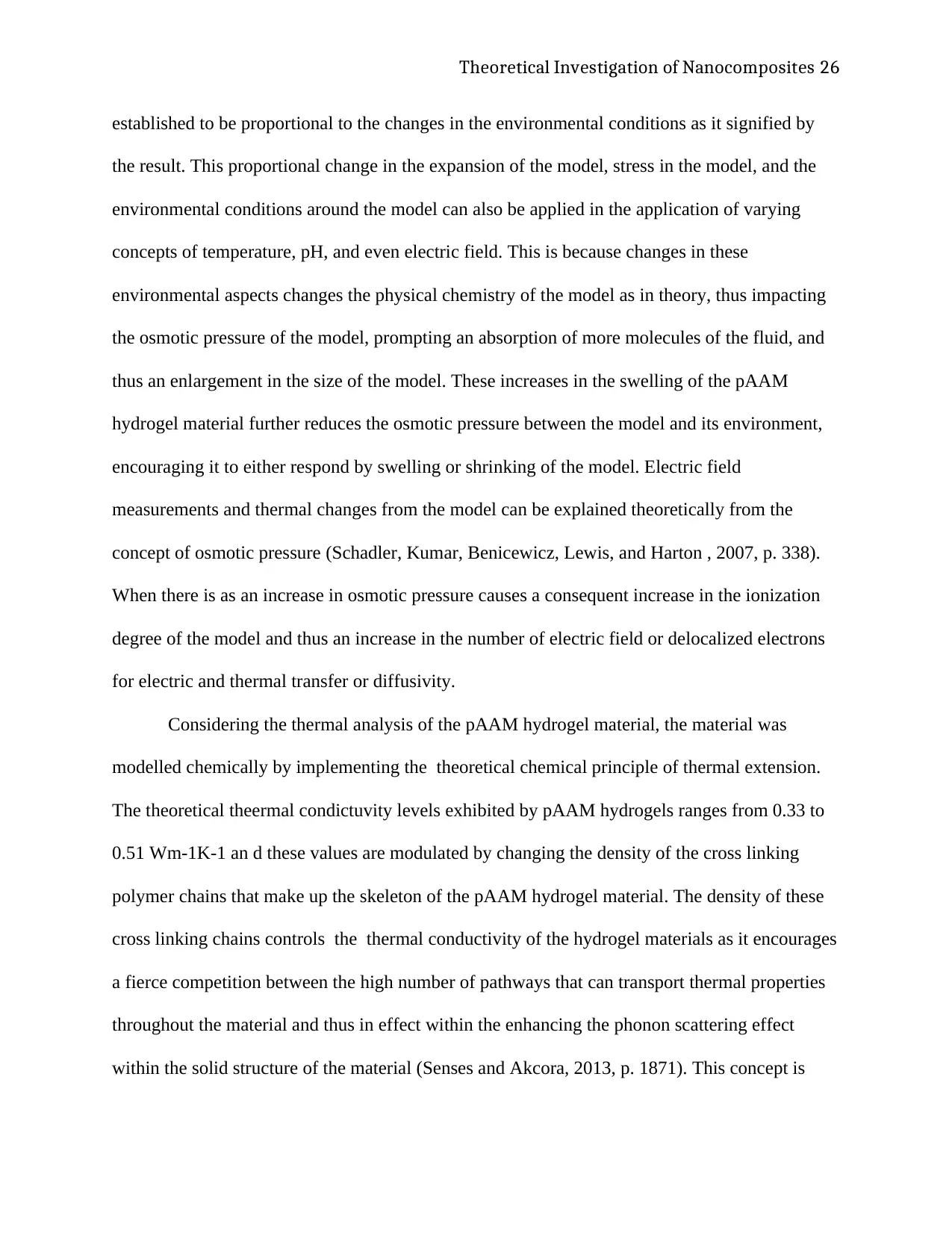
Theoretical Investigation of Nanocomposites 26
established to be proportional to the changes in the environmental conditions as it signified by
the result. This proportional change in the expansion of the model, stress in the model, and the
environmental conditions around the model can also be applied in the application of varying
concepts of temperature, pH, and even electric field. This is because changes in these
environmental aspects changes the physical chemistry of the model as in theory, thus impacting
the osmotic pressure of the model, prompting an absorption of more molecules of the fluid, and
thus an enlargement in the size of the model. These increases in the swelling of the pAAM
hydrogel material further reduces the osmotic pressure between the model and its environment,
encouraging it to either respond by swelling or shrinking of the model. Electric field
measurements and thermal changes from the model can be explained theoretically from the
concept of osmotic pressure (Schadler, Kumar, Benicewicz, Lewis, and Harton , 2007, p. 338).
When there is as an increase in osmotic pressure causes a consequent increase in the ionization
degree of the model and thus an increase in the number of electric field or delocalized electrons
for electric and thermal transfer or diffusivity.
Considering the thermal analysis of the pAAM hydrogel material, the material was
modelled chemically by implementing the theoretical chemical principle of thermal extension.
The theoretical theermal condictuvity levels exhibited by pAAM hydrogels ranges from 0.33 to
0.51 Wm-1K-1 an d these values are modulated by changing the density of the cross linking
polymer chains that make up the skeleton of the pAAM hydrogel material. The density of these
cross linking chains controls the thermal conductivity of the hydrogel materials as it encourages
a fierce competition between the high number of pathways that can transport thermal properties
throughout the material and thus in effect within the enhancing the phonon scattering effect
within the solid structure of the material (Senses and Akcora, 2013, p. 1871). This concept is
established to be proportional to the changes in the environmental conditions as it signified by
the result. This proportional change in the expansion of the model, stress in the model, and the
environmental conditions around the model can also be applied in the application of varying
concepts of temperature, pH, and even electric field. This is because changes in these
environmental aspects changes the physical chemistry of the model as in theory, thus impacting
the osmotic pressure of the model, prompting an absorption of more molecules of the fluid, and
thus an enlargement in the size of the model. These increases in the swelling of the pAAM
hydrogel material further reduces the osmotic pressure between the model and its environment,
encouraging it to either respond by swelling or shrinking of the model. Electric field
measurements and thermal changes from the model can be explained theoretically from the
concept of osmotic pressure (Schadler, Kumar, Benicewicz, Lewis, and Harton , 2007, p. 338).
When there is as an increase in osmotic pressure causes a consequent increase in the ionization
degree of the model and thus an increase in the number of electric field or delocalized electrons
for electric and thermal transfer or diffusivity.
Considering the thermal analysis of the pAAM hydrogel material, the material was
modelled chemically by implementing the theoretical chemical principle of thermal extension.
The theoretical theermal condictuvity levels exhibited by pAAM hydrogels ranges from 0.33 to
0.51 Wm-1K-1 an d these values are modulated by changing the density of the cross linking
polymer chains that make up the skeleton of the pAAM hydrogel material. The density of these
cross linking chains controls the thermal conductivity of the hydrogel materials as it encourages
a fierce competition between the high number of pathways that can transport thermal properties
throughout the material and thus in effect within the enhancing the phonon scattering effect
within the solid structure of the material (Senses and Akcora, 2013, p. 1871). This concept is
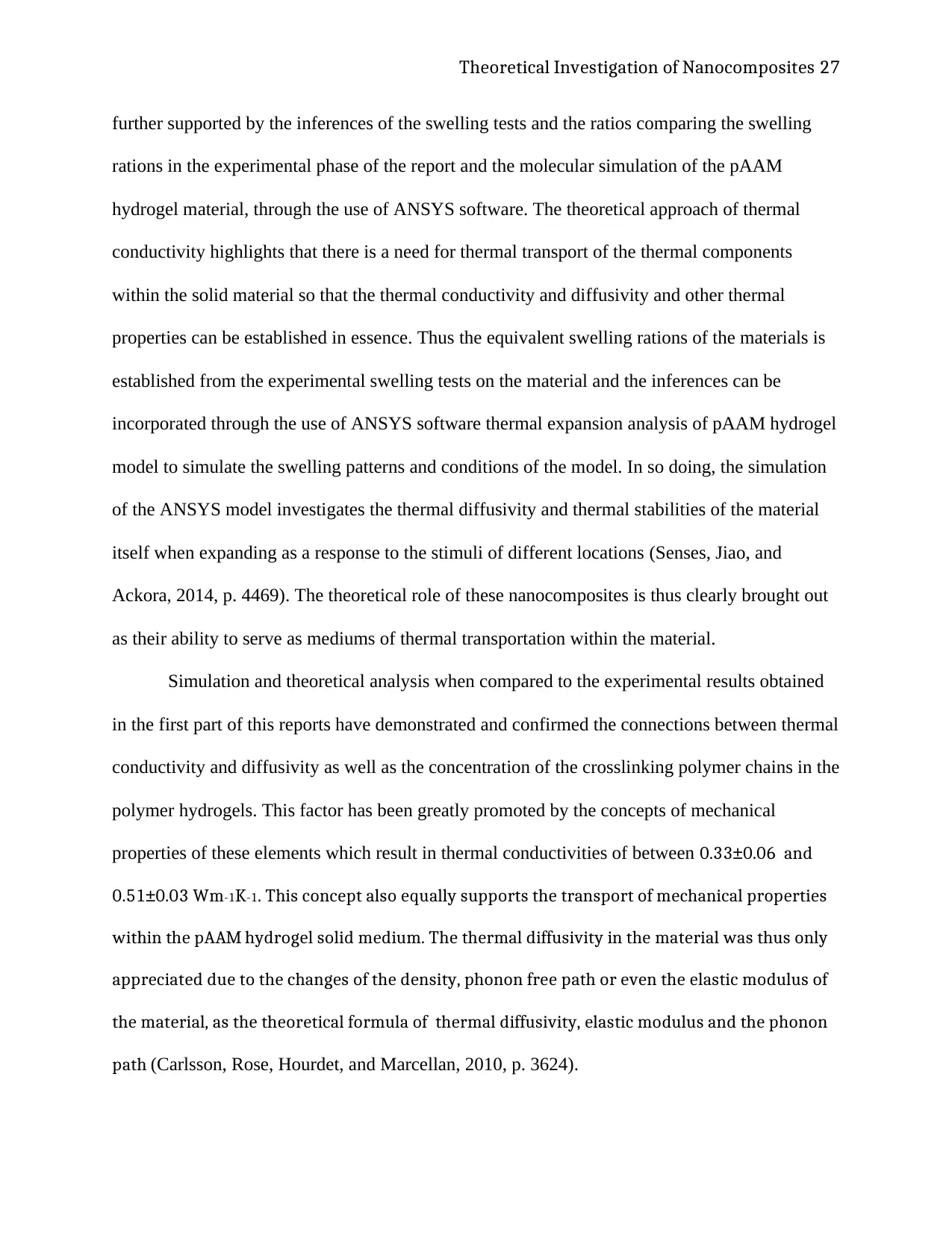
Theoretical Investigation of Nanocomposites 27
further supported by the inferences of the swelling tests and the ratios comparing the swelling
rations in the experimental phase of the report and the molecular simulation of the pAAM
hydrogel material, through the use of ANSYS software. The theoretical approach of thermal
conductivity highlights that there is a need for thermal transport of the thermal components
within the solid material so that the thermal conductivity and diffusivity and other thermal
properties can be established in essence. Thus the equivalent swelling rations of the materials is
established from the experimental swelling tests on the material and the inferences can be
incorporated through the use of ANSYS software thermal expansion analysis of pAAM hydrogel
model to simulate the swelling patterns and conditions of the model. In so doing, the simulation
of the ANSYS model investigates the thermal diffusivity and thermal stabilities of the material
itself when expanding as a response to the stimuli of different locations (Senses, Jiao, and
Ackora, 2014, p. 4469). The theoretical role of these nanocomposites is thus clearly brought out
as their ability to serve as mediums of thermal transportation within the material.
Simulation and theoretical analysis when compared to the experimental results obtained
in the first part of this reports have demonstrated and confirmed the connections between thermal
conductivity and diffusivity as well as the concentration of the crosslinking polymer chains in the
polymer hydrogels. This factor has been greatly promoted by the concepts of mechanical
properties of these elements which result in thermal conductivities of between 0.33±0.06 and
0.51±0.03 Wm-1K-1. This concept also equally supports the transport of mechanical properties
within the pAAM hydrogel solid medium. The thermal diffusivity in the material was thus only
appreciated due to the changes of the density, phonon free path or even the elastic modulus of
the material, as the theoretical formula of thermal diffusivity, elastic modulus and the phonon
path (Carlsson, Rose, Hourdet, and Marcellan, 2010, p. 3624).
further supported by the inferences of the swelling tests and the ratios comparing the swelling
rations in the experimental phase of the report and the molecular simulation of the pAAM
hydrogel material, through the use of ANSYS software. The theoretical approach of thermal
conductivity highlights that there is a need for thermal transport of the thermal components
within the solid material so that the thermal conductivity and diffusivity and other thermal
properties can be established in essence. Thus the equivalent swelling rations of the materials is
established from the experimental swelling tests on the material and the inferences can be
incorporated through the use of ANSYS software thermal expansion analysis of pAAM hydrogel
model to simulate the swelling patterns and conditions of the model. In so doing, the simulation
of the ANSYS model investigates the thermal diffusivity and thermal stabilities of the material
itself when expanding as a response to the stimuli of different locations (Senses, Jiao, and
Ackora, 2014, p. 4469). The theoretical role of these nanocomposites is thus clearly brought out
as their ability to serve as mediums of thermal transportation within the material.
Simulation and theoretical analysis when compared to the experimental results obtained
in the first part of this reports have demonstrated and confirmed the connections between thermal
conductivity and diffusivity as well as the concentration of the crosslinking polymer chains in the
polymer hydrogels. This factor has been greatly promoted by the concepts of mechanical
properties of these elements which result in thermal conductivities of between 0.33±0.06 and
0.51±0.03 Wm-1K-1. This concept also equally supports the transport of mechanical properties
within the pAAM hydrogel solid medium. The thermal diffusivity in the material was thus only
appreciated due to the changes of the density, phonon free path or even the elastic modulus of
the material, as the theoretical formula of thermal diffusivity, elastic modulus and the phonon
path (Carlsson, Rose, Hourdet, and Marcellan, 2010, p. 3624).
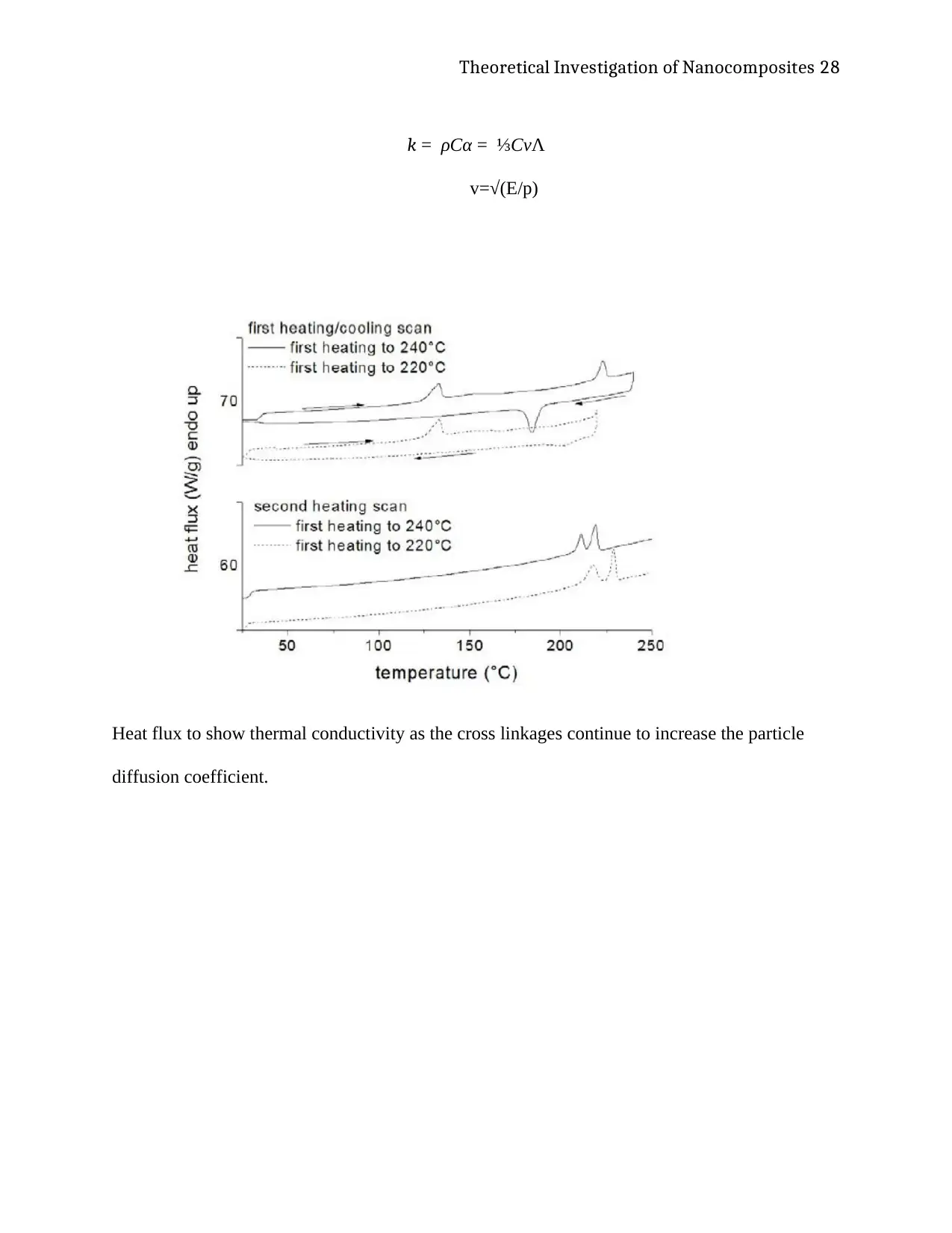
Theoretical Investigation of Nanocomposites 28
k = ρCα = ⅓CvΛ
v=√(E/p)
Heat flux to show thermal conductivity as the cross linkages continue to increase the particle
diffusion coefficient.
k = ρCα = ⅓CvΛ
v=√(E/p)
Heat flux to show thermal conductivity as the cross linkages continue to increase the particle
diffusion coefficient.
Secure Best Marks with AI Grader
Need help grading? Try our AI Grader for instant feedback on your assignments.
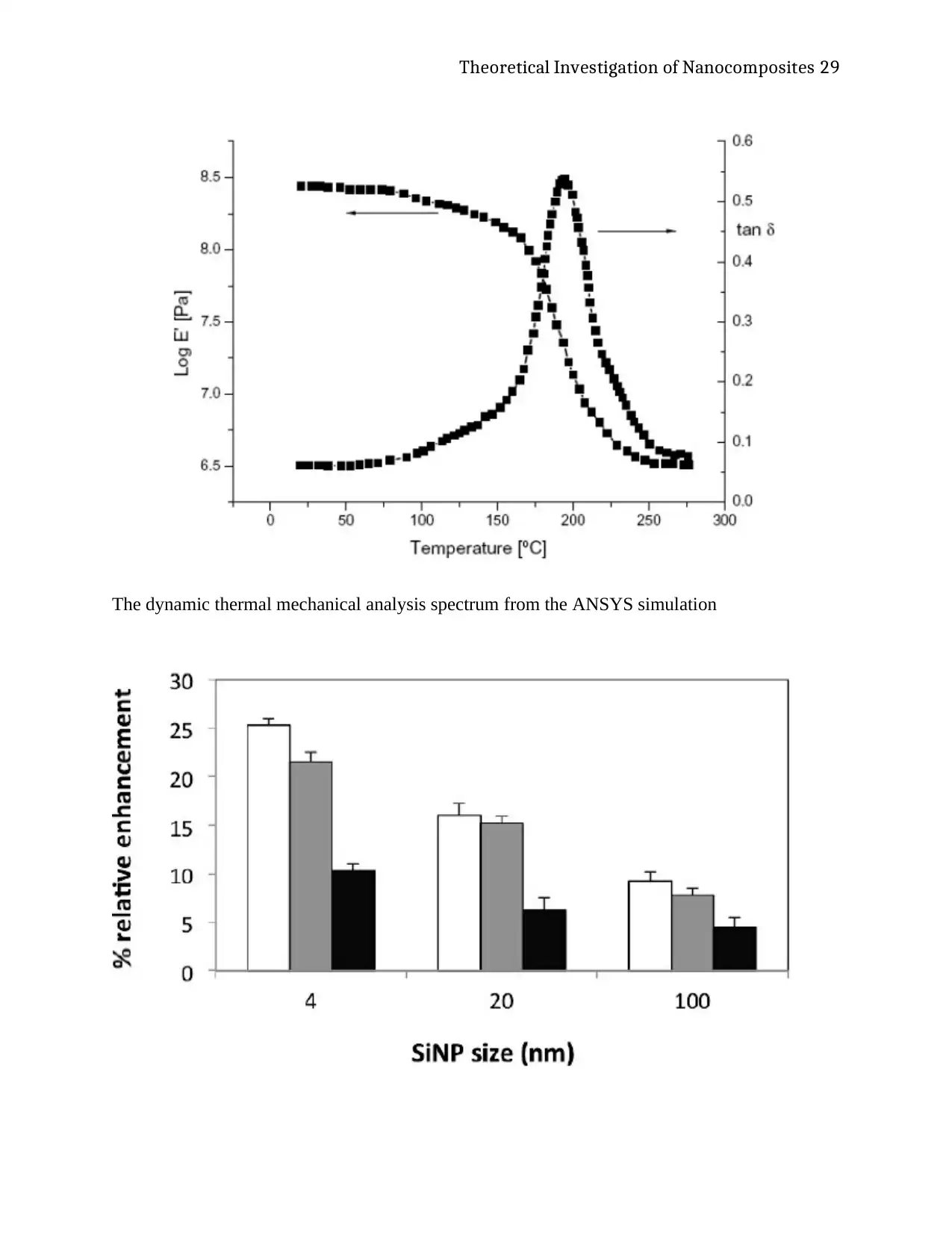
Theoretical Investigation of Nanocomposites 29
The dynamic thermal mechanical analysis spectrum from the ANSYS simulation
The dynamic thermal mechanical analysis spectrum from the ANSYS simulation
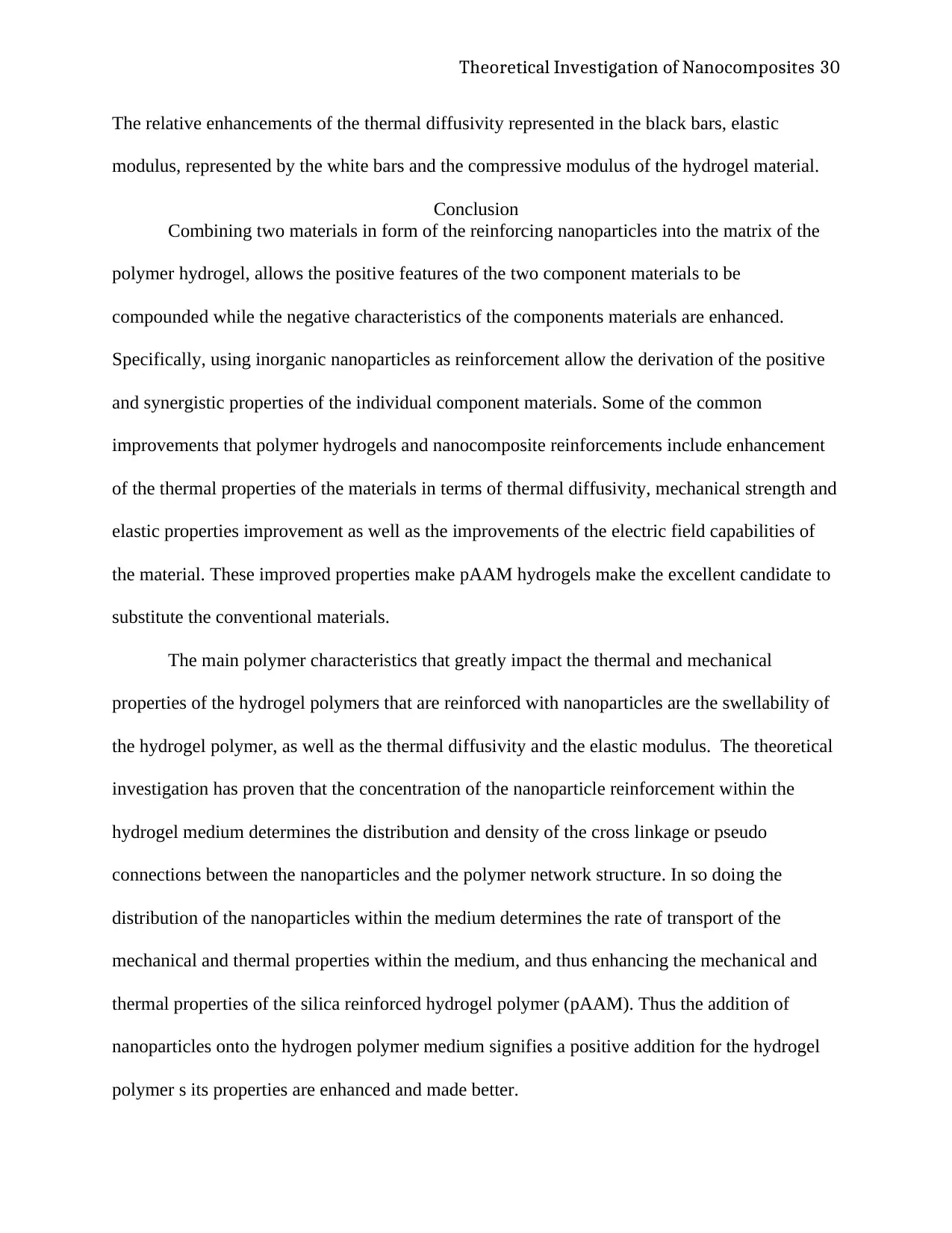
Theoretical Investigation of Nanocomposites 30
The relative enhancements of the thermal diffusivity represented in the black bars, elastic
modulus, represented by the white bars and the compressive modulus of the hydrogel material.
Conclusion
Combining two materials in form of the reinforcing nanoparticles into the matrix of the
polymer hydrogel, allows the positive features of the two component materials to be
compounded while the negative characteristics of the components materials are enhanced.
Specifically, using inorganic nanoparticles as reinforcement allow the derivation of the positive
and synergistic properties of the individual component materials. Some of the common
improvements that polymer hydrogels and nanocomposite reinforcements include enhancement
of the thermal properties of the materials in terms of thermal diffusivity, mechanical strength and
elastic properties improvement as well as the improvements of the electric field capabilities of
the material. These improved properties make pAAM hydrogels make the excellent candidate to
substitute the conventional materials.
The main polymer characteristics that greatly impact the thermal and mechanical
properties of the hydrogel polymers that are reinforced with nanoparticles are the swellability of
the hydrogel polymer, as well as the thermal diffusivity and the elastic modulus. The theoretical
investigation has proven that the concentration of the nanoparticle reinforcement within the
hydrogel medium determines the distribution and density of the cross linkage or pseudo
connections between the nanoparticles and the polymer network structure. In so doing the
distribution of the nanoparticles within the medium determines the rate of transport of the
mechanical and thermal properties within the medium, and thus enhancing the mechanical and
thermal properties of the silica reinforced hydrogel polymer (pAAM). Thus the addition of
nanoparticles onto the hydrogen polymer medium signifies a positive addition for the hydrogel
polymer s its properties are enhanced and made better.
The relative enhancements of the thermal diffusivity represented in the black bars, elastic
modulus, represented by the white bars and the compressive modulus of the hydrogel material.
Conclusion
Combining two materials in form of the reinforcing nanoparticles into the matrix of the
polymer hydrogel, allows the positive features of the two component materials to be
compounded while the negative characteristics of the components materials are enhanced.
Specifically, using inorganic nanoparticles as reinforcement allow the derivation of the positive
and synergistic properties of the individual component materials. Some of the common
improvements that polymer hydrogels and nanocomposite reinforcements include enhancement
of the thermal properties of the materials in terms of thermal diffusivity, mechanical strength and
elastic properties improvement as well as the improvements of the electric field capabilities of
the material. These improved properties make pAAM hydrogels make the excellent candidate to
substitute the conventional materials.
The main polymer characteristics that greatly impact the thermal and mechanical
properties of the hydrogel polymers that are reinforced with nanoparticles are the swellability of
the hydrogel polymer, as well as the thermal diffusivity and the elastic modulus. The theoretical
investigation has proven that the concentration of the nanoparticle reinforcement within the
hydrogel medium determines the distribution and density of the cross linkage or pseudo
connections between the nanoparticles and the polymer network structure. In so doing the
distribution of the nanoparticles within the medium determines the rate of transport of the
mechanical and thermal properties within the medium, and thus enhancing the mechanical and
thermal properties of the silica reinforced hydrogel polymer (pAAM). Thus the addition of
nanoparticles onto the hydrogen polymer medium signifies a positive addition for the hydrogel
polymer s its properties are enhanced and made better.
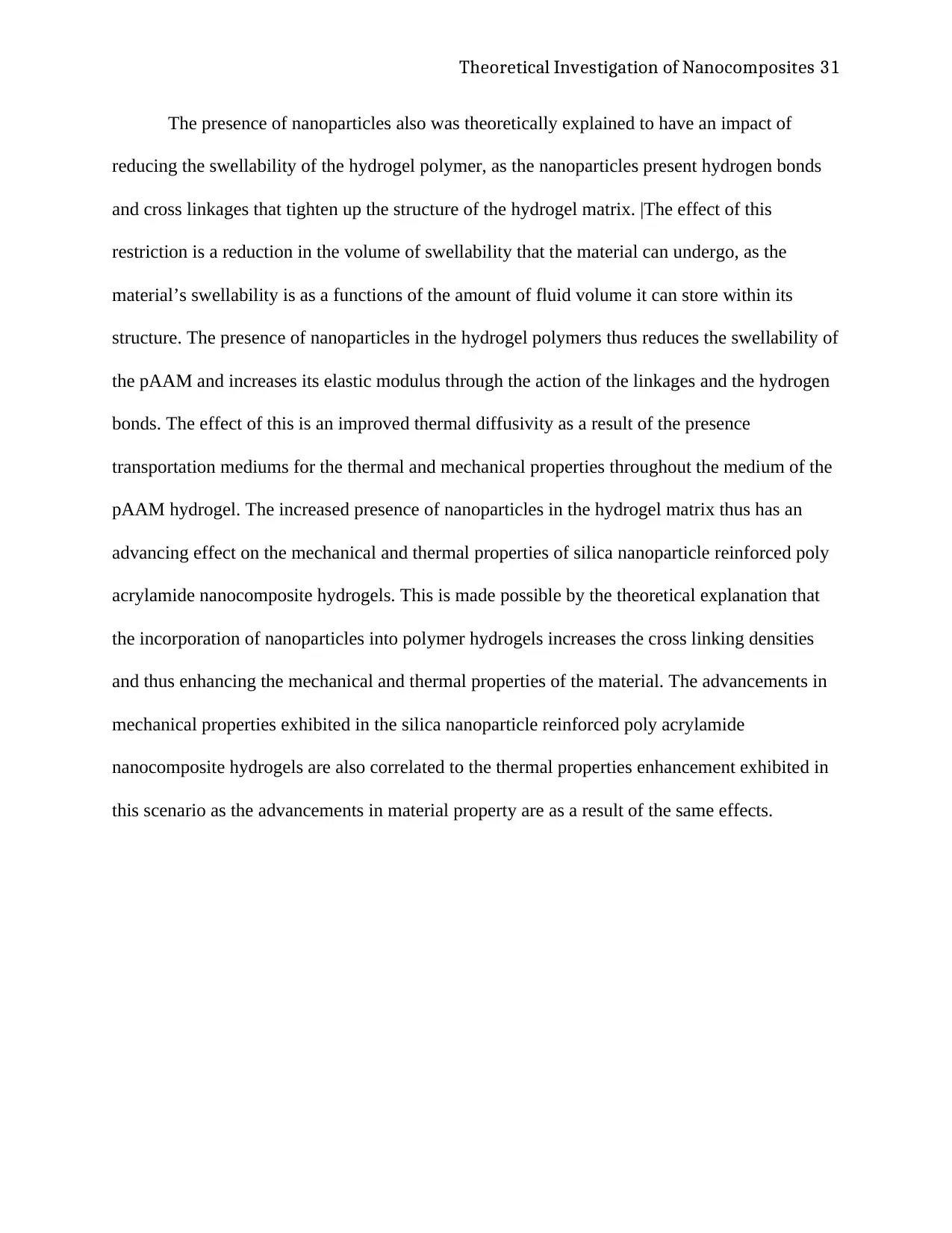
Theoretical Investigation of Nanocomposites 31
The presence of nanoparticles also was theoretically explained to have an impact of
reducing the swellability of the hydrogel polymer, as the nanoparticles present hydrogen bonds
and cross linkages that tighten up the structure of the hydrogel matrix. |The effect of this
restriction is a reduction in the volume of swellability that the material can undergo, as the
material’s swellability is as a functions of the amount of fluid volume it can store within its
structure. The presence of nanoparticles in the hydrogel polymers thus reduces the swellability of
the pAAM and increases its elastic modulus through the action of the linkages and the hydrogen
bonds. The effect of this is an improved thermal diffusivity as a result of the presence
transportation mediums for the thermal and mechanical properties throughout the medium of the
pAAM hydrogel. The increased presence of nanoparticles in the hydrogel matrix thus has an
advancing effect on the mechanical and thermal properties of silica nanoparticle reinforced poly
acrylamide nanocomposite hydrogels. This is made possible by the theoretical explanation that
the incorporation of nanoparticles into polymer hydrogels increases the cross linking densities
and thus enhancing the mechanical and thermal properties of the material. The advancements in
mechanical properties exhibited in the silica nanoparticle reinforced poly acrylamide
nanocomposite hydrogels are also correlated to the thermal properties enhancement exhibited in
this scenario as the advancements in material property are as a result of the same effects.
The presence of nanoparticles also was theoretically explained to have an impact of
reducing the swellability of the hydrogel polymer, as the nanoparticles present hydrogen bonds
and cross linkages that tighten up the structure of the hydrogel matrix. |The effect of this
restriction is a reduction in the volume of swellability that the material can undergo, as the
material’s swellability is as a functions of the amount of fluid volume it can store within its
structure. The presence of nanoparticles in the hydrogel polymers thus reduces the swellability of
the pAAM and increases its elastic modulus through the action of the linkages and the hydrogen
bonds. The effect of this is an improved thermal diffusivity as a result of the presence
transportation mediums for the thermal and mechanical properties throughout the medium of the
pAAM hydrogel. The increased presence of nanoparticles in the hydrogel matrix thus has an
advancing effect on the mechanical and thermal properties of silica nanoparticle reinforced poly
acrylamide nanocomposite hydrogels. This is made possible by the theoretical explanation that
the incorporation of nanoparticles into polymer hydrogels increases the cross linking densities
and thus enhancing the mechanical and thermal properties of the material. The advancements in
mechanical properties exhibited in the silica nanoparticle reinforced poly acrylamide
nanocomposite hydrogels are also correlated to the thermal properties enhancement exhibited in
this scenario as the advancements in material property are as a result of the same effects.
Paraphrase This Document
Need a fresh take? Get an instant paraphrase of this document with our AI Paraphraser
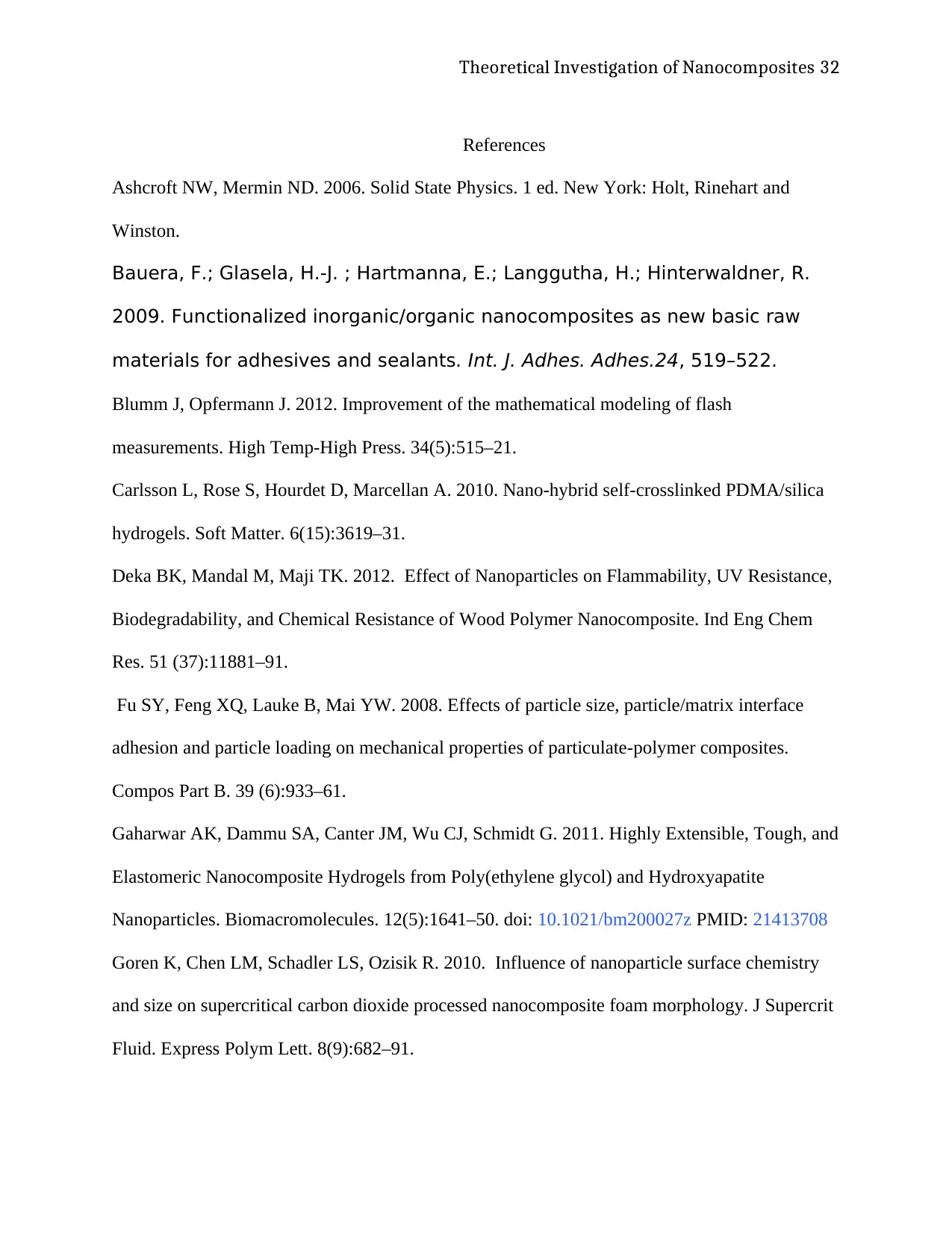
Theoretical Investigation of Nanocomposites 32
References
Ashcroft NW, Mermin ND. 2006. Solid State Physics. 1 ed. New York: Holt, Rinehart and
Winston.
Bauera, F.; Glasela, H.-J. ; Hartmanna, E.; Langgutha, H.; Hinterwaldner, R.
2009. Functionalized inorganic/organic nanocomposites as new basic raw
materials for adhesives and sealants. Int. J. Adhes. Adhes.24, 519–522.
Blumm J, Opfermann J. 2012. Improvement of the mathematical modeling of flash
measurements. High Temp-High Press. 34(5):515–21.
Carlsson L, Rose S, Hourdet D, Marcellan A. 2010. Nano-hybrid self-crosslinked PDMA/silica
hydrogels. Soft Matter. 6(15):3619–31.
Deka BK, Mandal M, Maji TK. 2012. Effect of Nanoparticles on Flammability, UV Resistance,
Biodegradability, and Chemical Resistance of Wood Polymer Nanocomposite. Ind Eng Chem
Res. 51 (37):11881–91.
Fu SY, Feng XQ, Lauke B, Mai YW. 2008. Effects of particle size, particle/matrix interface
adhesion and particle loading on mechanical properties of particulate-polymer composites.
Compos Part B. 39 (6):933–61.
Gaharwar AK, Dammu SA, Canter JM, Wu CJ, Schmidt G. 2011. Highly Extensible, Tough, and
Elastomeric Nanocomposite Hydrogels from Poly(ethylene glycol) and Hydroxyapatite
Nanoparticles. Biomacromolecules. 12(5):1641–50. doi: 10.1021/bm200027z PMID: 21413708
Goren K, Chen LM, Schadler LS, Ozisik R. 2010. Influence of nanoparticle surface chemistry
and size on supercritical carbon dioxide processed nanocomposite foam morphology. J Supercrit
Fluid. Express Polym Lett. 8(9):682–91.
References
Ashcroft NW, Mermin ND. 2006. Solid State Physics. 1 ed. New York: Holt, Rinehart and
Winston.
Bauera, F.; Glasela, H.-J. ; Hartmanna, E.; Langgutha, H.; Hinterwaldner, R.
2009. Functionalized inorganic/organic nanocomposites as new basic raw
materials for adhesives and sealants. Int. J. Adhes. Adhes.24, 519–522.
Blumm J, Opfermann J. 2012. Improvement of the mathematical modeling of flash
measurements. High Temp-High Press. 34(5):515–21.
Carlsson L, Rose S, Hourdet D, Marcellan A. 2010. Nano-hybrid self-crosslinked PDMA/silica
hydrogels. Soft Matter. 6(15):3619–31.
Deka BK, Mandal M, Maji TK. 2012. Effect of Nanoparticles on Flammability, UV Resistance,
Biodegradability, and Chemical Resistance of Wood Polymer Nanocomposite. Ind Eng Chem
Res. 51 (37):11881–91.
Fu SY, Feng XQ, Lauke B, Mai YW. 2008. Effects of particle size, particle/matrix interface
adhesion and particle loading on mechanical properties of particulate-polymer composites.
Compos Part B. 39 (6):933–61.
Gaharwar AK, Dammu SA, Canter JM, Wu CJ, Schmidt G. 2011. Highly Extensible, Tough, and
Elastomeric Nanocomposite Hydrogels from Poly(ethylene glycol) and Hydroxyapatite
Nanoparticles. Biomacromolecules. 12(5):1641–50. doi: 10.1021/bm200027z PMID: 21413708
Goren K, Chen LM, Schadler LS, Ozisik R. 2010. Influence of nanoparticle surface chemistry
and size on supercritical carbon dioxide processed nanocomposite foam morphology. J Supercrit
Fluid. Express Polym Lett. 8(9):682–91.
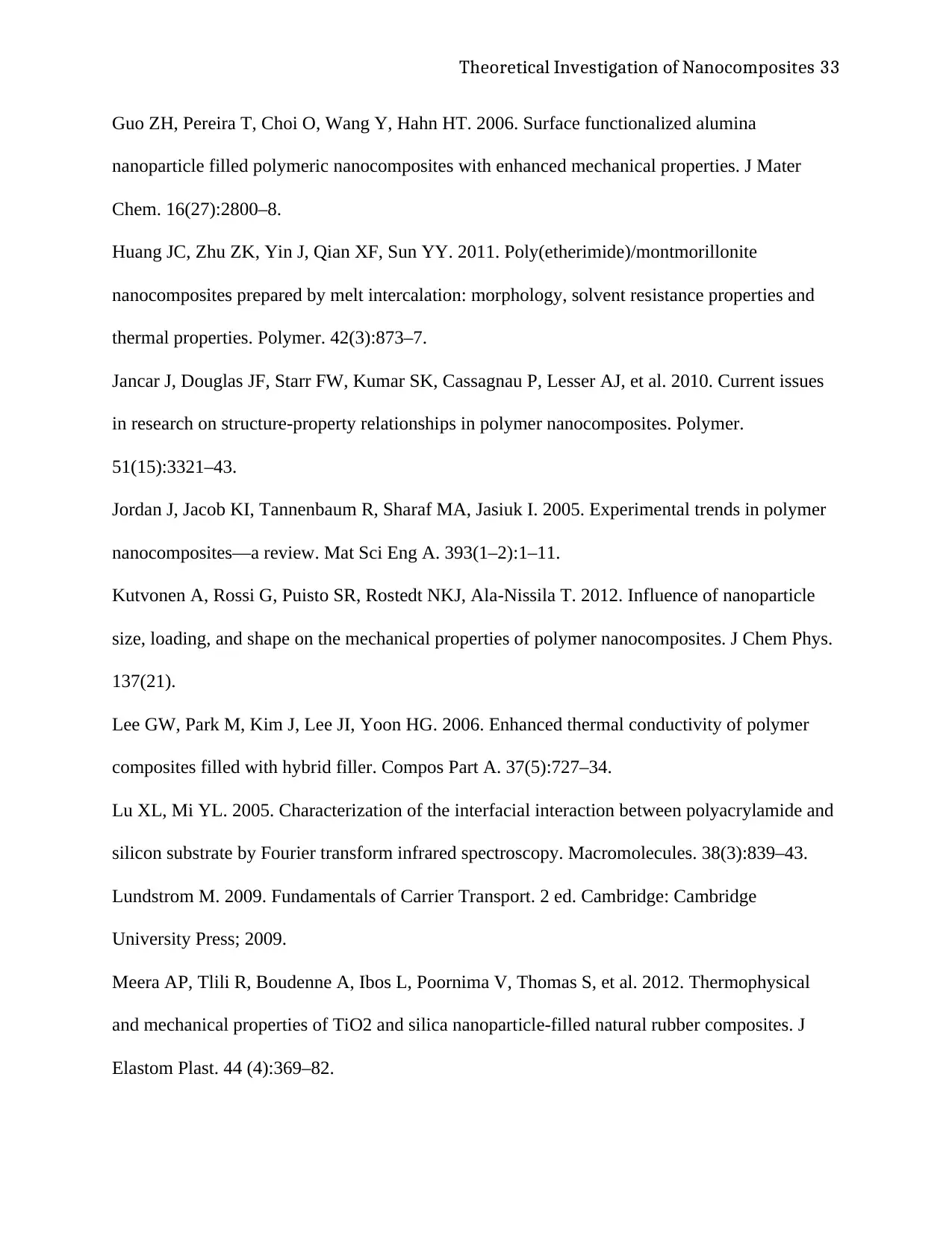
Theoretical Investigation of Nanocomposites 33
Guo ZH, Pereira T, Choi O, Wang Y, Hahn HT. 2006. Surface functionalized alumina
nanoparticle filled polymeric nanocomposites with enhanced mechanical properties. J Mater
Chem. 16(27):2800–8.
Huang JC, Zhu ZK, Yin J, Qian XF, Sun YY. 2011. Poly(etherimide)/montmorillonite
nanocomposites prepared by melt intercalation: morphology, solvent resistance properties and
thermal properties. Polymer. 42(3):873–7.
Jancar J, Douglas JF, Starr FW, Kumar SK, Cassagnau P, Lesser AJ, et al. 2010. Current issues
in research on structure-property relationships in polymer nanocomposites. Polymer.
51(15):3321–43.
Jordan J, Jacob KI, Tannenbaum R, Sharaf MA, Jasiuk I. 2005. Experimental trends in polymer
nanocomposites—a review. Mat Sci Eng A. 393(1–2):1–11.
Kutvonen A, Rossi G, Puisto SR, Rostedt NKJ, Ala-Nissila T. 2012. Influence of nanoparticle
size, loading, and shape on the mechanical properties of polymer nanocomposites. J Chem Phys.
137(21).
Lee GW, Park M, Kim J, Lee JI, Yoon HG. 2006. Enhanced thermal conductivity of polymer
composites filled with hybrid filler. Compos Part A. 37(5):727–34.
Lu XL, Mi YL. 2005. Characterization of the interfacial interaction between polyacrylamide and
silicon substrate by Fourier transform infrared spectroscopy. Macromolecules. 38(3):839–43.
Lundstrom M. 2009. Fundamentals of Carrier Transport. 2 ed. Cambridge: Cambridge
University Press; 2009.
Meera AP, Tlili R, Boudenne A, Ibos L, Poornima V, Thomas S, et al. 2012. Thermophysical
and mechanical properties of TiO2 and silica nanoparticle-filled natural rubber composites. J
Elastom Plast. 44 (4):369–82.
Guo ZH, Pereira T, Choi O, Wang Y, Hahn HT. 2006. Surface functionalized alumina
nanoparticle filled polymeric nanocomposites with enhanced mechanical properties. J Mater
Chem. 16(27):2800–8.
Huang JC, Zhu ZK, Yin J, Qian XF, Sun YY. 2011. Poly(etherimide)/montmorillonite
nanocomposites prepared by melt intercalation: morphology, solvent resistance properties and
thermal properties. Polymer. 42(3):873–7.
Jancar J, Douglas JF, Starr FW, Kumar SK, Cassagnau P, Lesser AJ, et al. 2010. Current issues
in research on structure-property relationships in polymer nanocomposites. Polymer.
51(15):3321–43.
Jordan J, Jacob KI, Tannenbaum R, Sharaf MA, Jasiuk I. 2005. Experimental trends in polymer
nanocomposites—a review. Mat Sci Eng A. 393(1–2):1–11.
Kutvonen A, Rossi G, Puisto SR, Rostedt NKJ, Ala-Nissila T. 2012. Influence of nanoparticle
size, loading, and shape on the mechanical properties of polymer nanocomposites. J Chem Phys.
137(21).
Lee GW, Park M, Kim J, Lee JI, Yoon HG. 2006. Enhanced thermal conductivity of polymer
composites filled with hybrid filler. Compos Part A. 37(5):727–34.
Lu XL, Mi YL. 2005. Characterization of the interfacial interaction between polyacrylamide and
silicon substrate by Fourier transform infrared spectroscopy. Macromolecules. 38(3):839–43.
Lundstrom M. 2009. Fundamentals of Carrier Transport. 2 ed. Cambridge: Cambridge
University Press; 2009.
Meera AP, Tlili R, Boudenne A, Ibos L, Poornima V, Thomas S, et al. 2012. Thermophysical
and mechanical properties of TiO2 and silica nanoparticle-filled natural rubber composites. J
Elastom Plast. 44 (4):369–82.
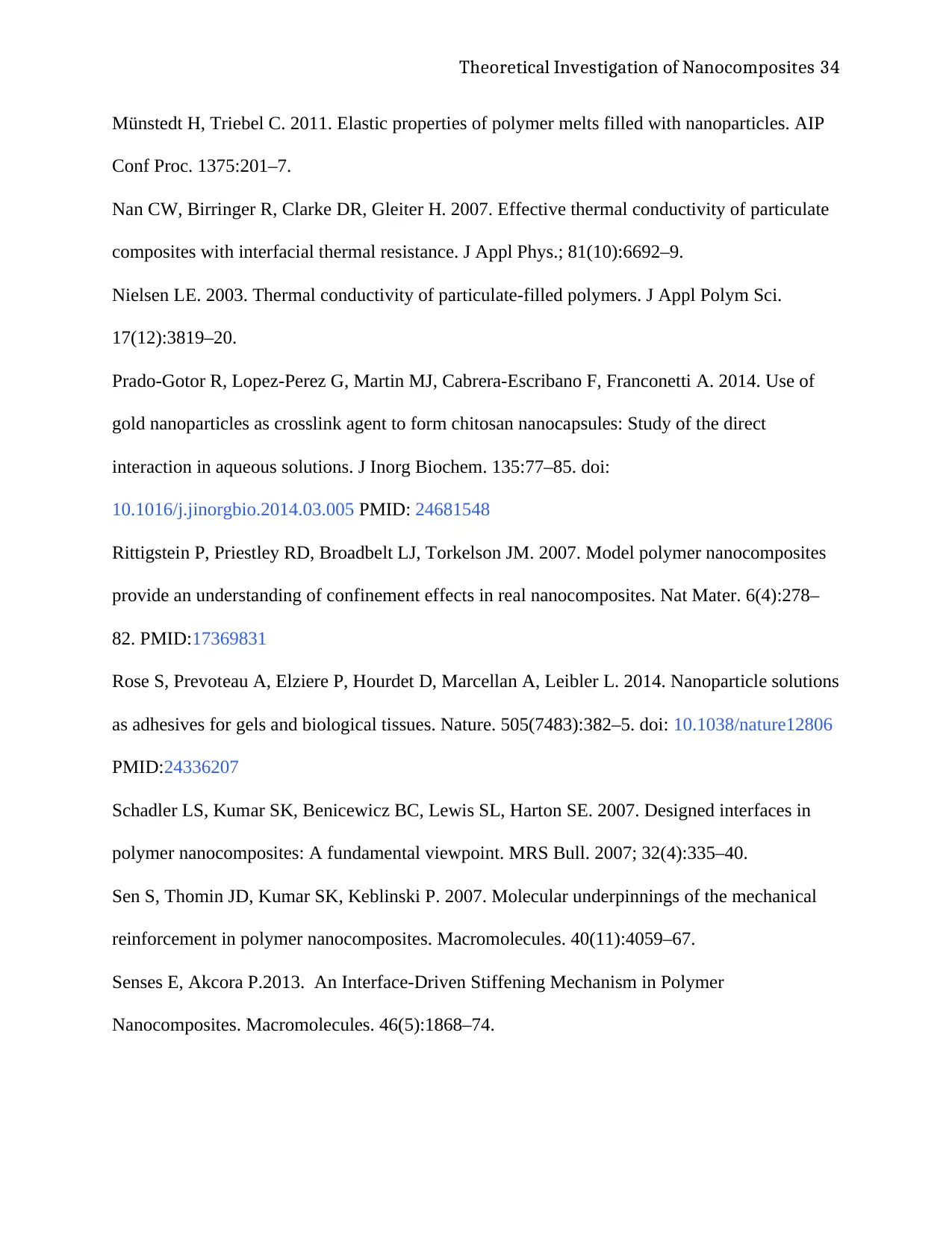
Theoretical Investigation of Nanocomposites 34
Münstedt H, Triebel C. 2011. Elastic properties of polymer melts filled with nanoparticles. AIP
Conf Proc. 1375:201–7.
Nan CW, Birringer R, Clarke DR, Gleiter H. 2007. Effective thermal conductivity of particulate
composites with interfacial thermal resistance. J Appl Phys.; 81(10):6692–9.
Nielsen LE. 2003. Thermal conductivity of particulate-filled polymers. J Appl Polym Sci.
17(12):3819–20.
Prado-Gotor R, Lopez-Perez G, Martin MJ, Cabrera-Escribano F, Franconetti A. 2014. Use of
gold nanoparticles as crosslink agent to form chitosan nanocapsules: Study of the direct
interaction in aqueous solutions. J Inorg Biochem. 135:77–85. doi:
10.1016/j.jinorgbio.2014.03.005 PMID: 24681548
Rittigstein P, Priestley RD, Broadbelt LJ, Torkelson JM. 2007. Model polymer nanocomposites
provide an understanding of confinement effects in real nanocomposites. Nat Mater. 6(4):278–
82. PMID:17369831
Rose S, Prevoteau A, Elziere P, Hourdet D, Marcellan A, Leibler L. 2014. Nanoparticle solutions
as adhesives for gels and biological tissues. Nature. 505(7483):382–5. doi: 10.1038/nature12806
PMID:24336207
Schadler LS, Kumar SK, Benicewicz BC, Lewis SL, Harton SE. 2007. Designed interfaces in
polymer nanocomposites: A fundamental viewpoint. MRS Bull. 2007; 32(4):335–40.
Sen S, Thomin JD, Kumar SK, Keblinski P. 2007. Molecular underpinnings of the mechanical
reinforcement in polymer nanocomposites. Macromolecules. 40(11):4059–67.
Senses E, Akcora P.2013. An Interface-Driven Stiffening Mechanism in Polymer
Nanocomposites. Macromolecules. 46(5):1868–74.
Münstedt H, Triebel C. 2011. Elastic properties of polymer melts filled with nanoparticles. AIP
Conf Proc. 1375:201–7.
Nan CW, Birringer R, Clarke DR, Gleiter H. 2007. Effective thermal conductivity of particulate
composites with interfacial thermal resistance. J Appl Phys.; 81(10):6692–9.
Nielsen LE. 2003. Thermal conductivity of particulate-filled polymers. J Appl Polym Sci.
17(12):3819–20.
Prado-Gotor R, Lopez-Perez G, Martin MJ, Cabrera-Escribano F, Franconetti A. 2014. Use of
gold nanoparticles as crosslink agent to form chitosan nanocapsules: Study of the direct
interaction in aqueous solutions. J Inorg Biochem. 135:77–85. doi:
10.1016/j.jinorgbio.2014.03.005 PMID: 24681548
Rittigstein P, Priestley RD, Broadbelt LJ, Torkelson JM. 2007. Model polymer nanocomposites
provide an understanding of confinement effects in real nanocomposites. Nat Mater. 6(4):278–
82. PMID:17369831
Rose S, Prevoteau A, Elziere P, Hourdet D, Marcellan A, Leibler L. 2014. Nanoparticle solutions
as adhesives for gels and biological tissues. Nature. 505(7483):382–5. doi: 10.1038/nature12806
PMID:24336207
Schadler LS, Kumar SK, Benicewicz BC, Lewis SL, Harton SE. 2007. Designed interfaces in
polymer nanocomposites: A fundamental viewpoint. MRS Bull. 2007; 32(4):335–40.
Sen S, Thomin JD, Kumar SK, Keblinski P. 2007. Molecular underpinnings of the mechanical
reinforcement in polymer nanocomposites. Macromolecules. 40(11):4059–67.
Senses E, Akcora P.2013. An Interface-Driven Stiffening Mechanism in Polymer
Nanocomposites. Macromolecules. 46(5):1868–74.
Secure Best Marks with AI Grader
Need help grading? Try our AI Grader for instant feedback on your assignments.
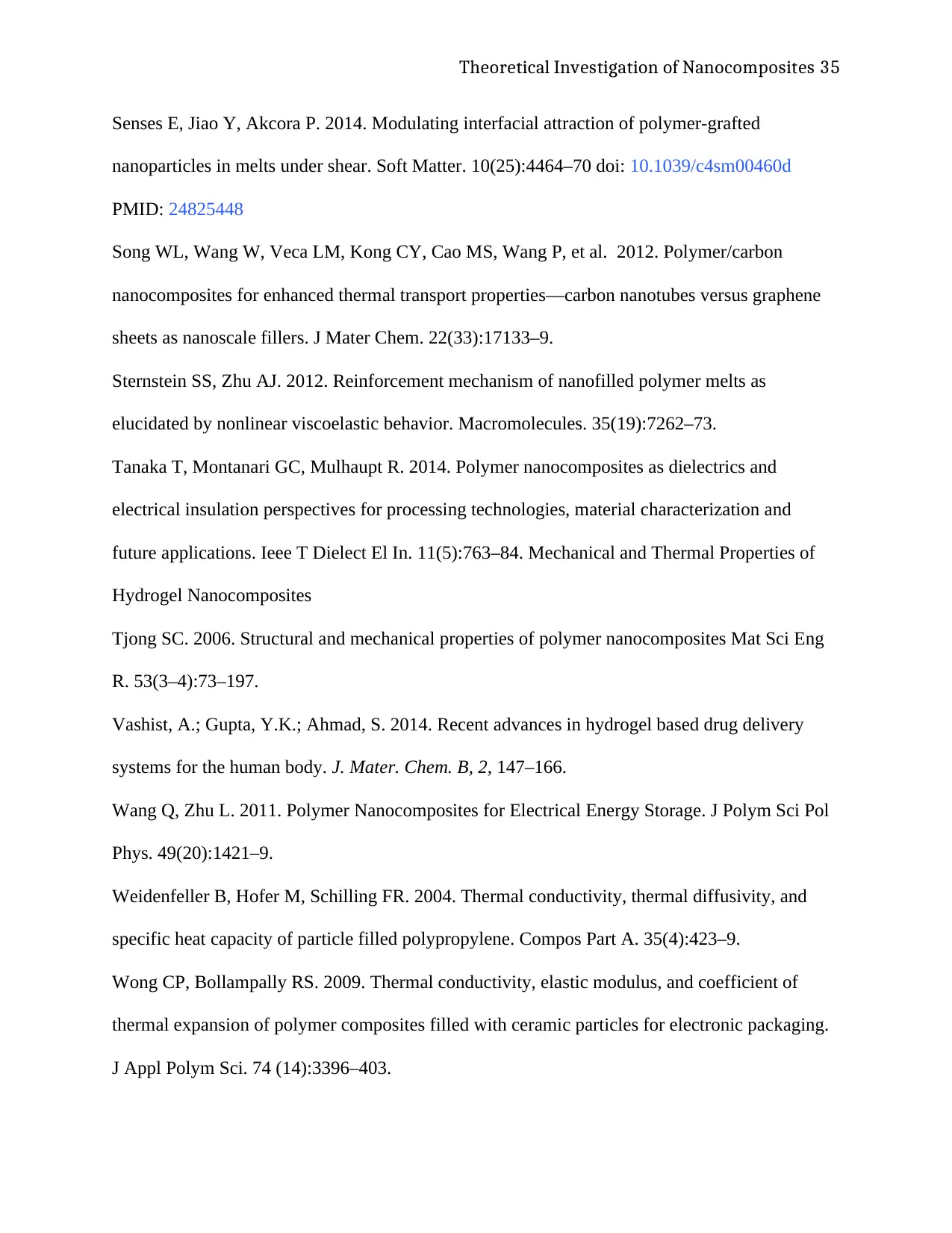
Theoretical Investigation of Nanocomposites 35
Senses E, Jiao Y, Akcora P. 2014. Modulating interfacial attraction of polymer-grafted
nanoparticles in melts under shear. Soft Matter. 10(25):4464–70 doi: 10.1039/c4sm00460d
PMID: 24825448
Song WL, Wang W, Veca LM, Kong CY, Cao MS, Wang P, et al. 2012. Polymer/carbon
nanocomposites for enhanced thermal transport properties—carbon nanotubes versus graphene
sheets as nanoscale fillers. J Mater Chem. 22(33):17133–9.
Sternstein SS, Zhu AJ. 2012. Reinforcement mechanism of nanofilled polymer melts as
elucidated by nonlinear viscoelastic behavior. Macromolecules. 35(19):7262–73.
Tanaka T, Montanari GC, Mulhaupt R. 2014. Polymer nanocomposites as dielectrics and
electrical insulation perspectives for processing technologies, material characterization and
future applications. Ieee T Dielect El In. 11(5):763–84. Mechanical and Thermal Properties of
Hydrogel Nanocomposites
Tjong SC. 2006. Structural and mechanical properties of polymer nanocomposites Mat Sci Eng
R. 53(3–4):73–197.
Vashist, A.; Gupta, Y.K.; Ahmad, S. 2014. Recent advances in hydrogel based drug delivery
systems for the human body. J. Mater. Chem. B, 2, 147–166.
Wang Q, Zhu L. 2011. Polymer Nanocomposites for Electrical Energy Storage. J Polym Sci Pol
Phys. 49(20):1421–9.
Weidenfeller B, Hofer M, Schilling FR. 2004. Thermal conductivity, thermal diffusivity, and
specific heat capacity of particle filled polypropylene. Compos Part A. 35(4):423–9.
Wong CP, Bollampally RS. 2009. Thermal conductivity, elastic modulus, and coefficient of
thermal expansion of polymer composites filled with ceramic particles for electronic packaging.
J Appl Polym Sci. 74 (14):3396–403.
Senses E, Jiao Y, Akcora P. 2014. Modulating interfacial attraction of polymer-grafted
nanoparticles in melts under shear. Soft Matter. 10(25):4464–70 doi: 10.1039/c4sm00460d
PMID: 24825448
Song WL, Wang W, Veca LM, Kong CY, Cao MS, Wang P, et al. 2012. Polymer/carbon
nanocomposites for enhanced thermal transport properties—carbon nanotubes versus graphene
sheets as nanoscale fillers. J Mater Chem. 22(33):17133–9.
Sternstein SS, Zhu AJ. 2012. Reinforcement mechanism of nanofilled polymer melts as
elucidated by nonlinear viscoelastic behavior. Macromolecules. 35(19):7262–73.
Tanaka T, Montanari GC, Mulhaupt R. 2014. Polymer nanocomposites as dielectrics and
electrical insulation perspectives for processing technologies, material characterization and
future applications. Ieee T Dielect El In. 11(5):763–84. Mechanical and Thermal Properties of
Hydrogel Nanocomposites
Tjong SC. 2006. Structural and mechanical properties of polymer nanocomposites Mat Sci Eng
R. 53(3–4):73–197.
Vashist, A.; Gupta, Y.K.; Ahmad, S. 2014. Recent advances in hydrogel based drug delivery
systems for the human body. J. Mater. Chem. B, 2, 147–166.
Wang Q, Zhu L. 2011. Polymer Nanocomposites for Electrical Energy Storage. J Polym Sci Pol
Phys. 49(20):1421–9.
Weidenfeller B, Hofer M, Schilling FR. 2004. Thermal conductivity, thermal diffusivity, and
specific heat capacity of particle filled polypropylene. Compos Part A. 35(4):423–9.
Wong CP, Bollampally RS. 2009. Thermal conductivity, elastic modulus, and coefficient of
thermal expansion of polymer composites filled with ceramic particles for electronic packaging.
J Appl Polym Sci. 74 (14):3396–403.
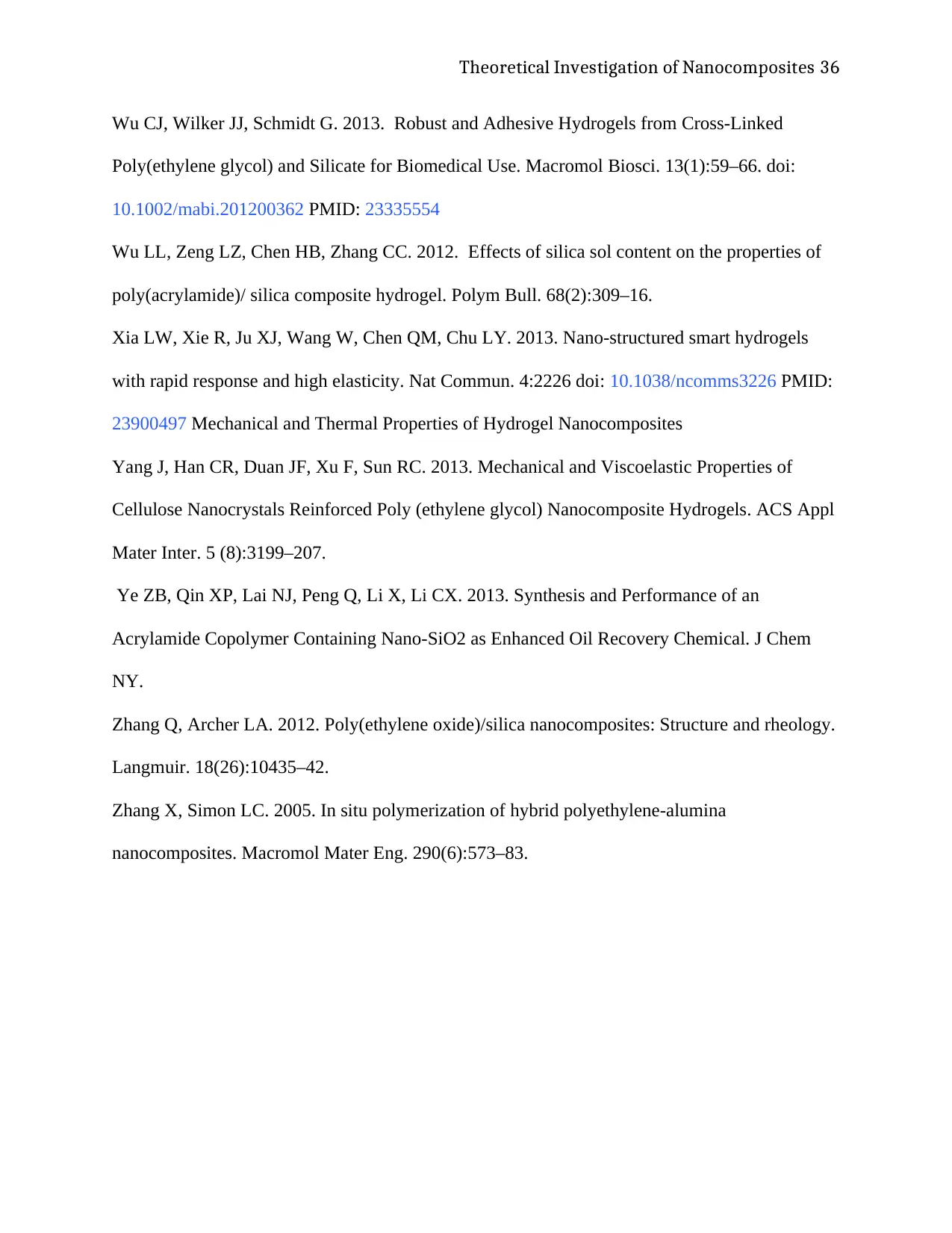
Theoretical Investigation of Nanocomposites 36
Wu CJ, Wilker JJ, Schmidt G. 2013. Robust and Adhesive Hydrogels from Cross-Linked
Poly(ethylene glycol) and Silicate for Biomedical Use. Macromol Biosci. 13(1):59–66. doi:
10.1002/mabi.201200362 PMID: 23335554
Wu LL, Zeng LZ, Chen HB, Zhang CC. 2012. Effects of silica sol content on the properties of
poly(acrylamide)/ silica composite hydrogel. Polym Bull. 68(2):309–16.
Xia LW, Xie R, Ju XJ, Wang W, Chen QM, Chu LY. 2013. Nano-structured smart hydrogels
with rapid response and high elasticity. Nat Commun. 4:2226 doi: 10.1038/ncomms3226 PMID:
23900497 Mechanical and Thermal Properties of Hydrogel Nanocomposites
Yang J, Han CR, Duan JF, Xu F, Sun RC. 2013. Mechanical and Viscoelastic Properties of
Cellulose Nanocrystals Reinforced Poly (ethylene glycol) Nanocomposite Hydrogels. ACS Appl
Mater Inter. 5 (8):3199–207.
Ye ZB, Qin XP, Lai NJ, Peng Q, Li X, Li CX. 2013. Synthesis and Performance of an
Acrylamide Copolymer Containing Nano-SiO2 as Enhanced Oil Recovery Chemical. J Chem
NY.
Zhang Q, Archer LA. 2012. Poly(ethylene oxide)/silica nanocomposites: Structure and rheology.
Langmuir. 18(26):10435–42.
Zhang X, Simon LC. 2005. In situ polymerization of hybrid polyethylene-alumina
nanocomposites. Macromol Mater Eng. 290(6):573–83.
Wu CJ, Wilker JJ, Schmidt G. 2013. Robust and Adhesive Hydrogels from Cross-Linked
Poly(ethylene glycol) and Silicate for Biomedical Use. Macromol Biosci. 13(1):59–66. doi:
10.1002/mabi.201200362 PMID: 23335554
Wu LL, Zeng LZ, Chen HB, Zhang CC. 2012. Effects of silica sol content on the properties of
poly(acrylamide)/ silica composite hydrogel. Polym Bull. 68(2):309–16.
Xia LW, Xie R, Ju XJ, Wang W, Chen QM, Chu LY. 2013. Nano-structured smart hydrogels
with rapid response and high elasticity. Nat Commun. 4:2226 doi: 10.1038/ncomms3226 PMID:
23900497 Mechanical and Thermal Properties of Hydrogel Nanocomposites
Yang J, Han CR, Duan JF, Xu F, Sun RC. 2013. Mechanical and Viscoelastic Properties of
Cellulose Nanocrystals Reinforced Poly (ethylene glycol) Nanocomposite Hydrogels. ACS Appl
Mater Inter. 5 (8):3199–207.
Ye ZB, Qin XP, Lai NJ, Peng Q, Li X, Li CX. 2013. Synthesis and Performance of an
Acrylamide Copolymer Containing Nano-SiO2 as Enhanced Oil Recovery Chemical. J Chem
NY.
Zhang Q, Archer LA. 2012. Poly(ethylene oxide)/silica nanocomposites: Structure and rheology.
Langmuir. 18(26):10435–42.
Zhang X, Simon LC. 2005. In situ polymerization of hybrid polyethylene-alumina
nanocomposites. Macromol Mater Eng. 290(6):573–83.
1 out of 36
![[object Object]](/_next/image/?url=%2F_next%2Fstatic%2Fmedia%2Flogo.6d15ce61.png&w=640&q=75)
Your All-in-One AI-Powered Toolkit for Academic Success.
+13062052269
info@desklib.com
Available 24*7 on WhatsApp / Email
Unlock your academic potential
© 2024 | Zucol Services PVT LTD | All rights reserved.