Ultra-Wideband Communication-Transceiver Design | Desklib
VerifiedAdded on 2023/06/03
|56
|14878
|118
AI Summary
This project report discusses the Ultra-Wideband Communication-Transceiver Design submitted to the University of Nairobi. It covers the advantages and challenges of UWB technology and its high data rate communication capability. The report includes a software radio transceiver design for impulse-based UWB with the ability to transmit a raw data rate of 100 Mbps yet encompasses the adaptability of a reconfigurable digital receiver.
Contribute Materials
Your contribution can guide someone’s learning journey. Share your
documents today.

UNIVERSITY OF NAIROBI
COLLEGE OF ARCHITECTURE AND ENGINEERING
DEPARTMENT OF ELECTRICAL AND ELECTRONIC ENGINEERING
FIFTH YEAR PROJECT REPORT
PROJECT CODE: PRJ 092
TITLE:
ULTRA-WIDEBAND COMMUNICATION-TRANSCEIVER
DESIGN
AUTHOR:
WAFULA WANJALA GEORGE
REG. NO: F17/8255/2004
DATE OF SUBMISSION: 28-05-2009
SUPERVISOR: Dr. Vitalice Oduol
EXAMINER: Dr. M. K. Gakuru
COLLEGE OF ARCHITECTURE AND ENGINEERING
DEPARTMENT OF ELECTRICAL AND ELECTRONIC ENGINEERING
FIFTH YEAR PROJECT REPORT
PROJECT CODE: PRJ 092
TITLE:
ULTRA-WIDEBAND COMMUNICATION-TRANSCEIVER
DESIGN
AUTHOR:
WAFULA WANJALA GEORGE
REG. NO: F17/8255/2004
DATE OF SUBMISSION: 28-05-2009
SUPERVISOR: Dr. Vitalice Oduol
EXAMINER: Dr. M. K. Gakuru
Secure Best Marks with AI Grader
Need help grading? Try our AI Grader for instant feedback on your assignments.
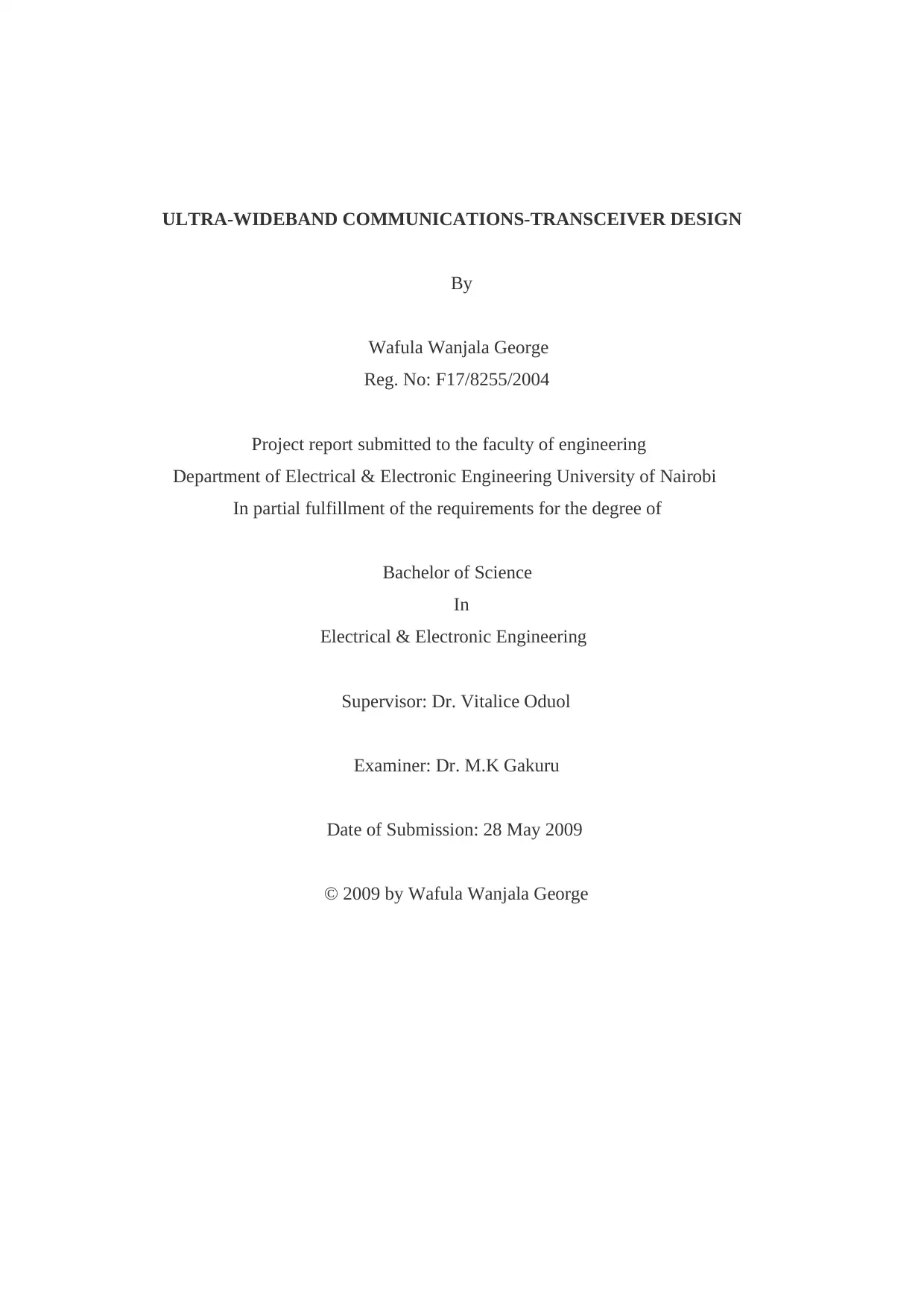
ULTRA-WIDEBAND COMMUNICATIONS-TRANSCEIVER DESIGN
By
Wafula Wanjala George
Reg. No: F17/8255/2004
Project report submitted to the faculty of engineering
Department of Electrical & Electronic Engineering University of Nairobi
In partial fulfillment of the requirements for the degree of
Bachelor of Science
In
Electrical & Electronic Engineering
Supervisor: Dr. Vitalice Oduol
Examiner: Dr. M.K Gakuru
Date of Submission: 28 May 2009
© 2009 by Wafula Wanjala George
By
Wafula Wanjala George
Reg. No: F17/8255/2004
Project report submitted to the faculty of engineering
Department of Electrical & Electronic Engineering University of Nairobi
In partial fulfillment of the requirements for the degree of
Bachelor of Science
In
Electrical & Electronic Engineering
Supervisor: Dr. Vitalice Oduol
Examiner: Dr. M.K Gakuru
Date of Submission: 28 May 2009
© 2009 by Wafula Wanjala George

Acknowledgements
I take this opportunity to thank my parents Mr. Peter Wafula Ichudi and my mother
Victoria Yaola for your love, guidance, advice, instruction and financial support. I wish
to thank my mother who has been with me all the way; though you do not understand
engineering, you do understand me. Thank you.
Am indebted to Florida Simiyu, she made her room and computer available for this
project. Your proof reading, encouragement and emotional support was a much-needed
tool in the quest to fulfil these project goals and objectives. It has been much more than
an honour to be by your side and I hope to continue our relationship for many years to
come. Thank you.
I acknowledge my friends and colleagues in the Electrical Engineering department, am
convinced that you have professionally influenced this project and my life in more than
one way. Thank you.
Last but not least, my professional thanks to my project supervisor Dr. Vitalice
Oduol. Your guidance and coaching skills have made this project a reality. I highly
appreciate your commitment and availability, always addressing issues relating to this
project. Thank you. Dr. Mwema our project co-coordinator, thank you for your lectures
on report writing and creating a good environment for experimentation and verification
of this project.
Everyday am more convinced and persuaded that I would have not reached thus
far, if it were not for The Lord God Almighty. Truly, words will fail to reflect and
reciprocate what you are to me and to what extent you have affected my life. It is
amazing. In more than many ways, you have given me the strength, agility and ability to
achieve my goals. I love you God. Thank you.
ii
I take this opportunity to thank my parents Mr. Peter Wafula Ichudi and my mother
Victoria Yaola for your love, guidance, advice, instruction and financial support. I wish
to thank my mother who has been with me all the way; though you do not understand
engineering, you do understand me. Thank you.
Am indebted to Florida Simiyu, she made her room and computer available for this
project. Your proof reading, encouragement and emotional support was a much-needed
tool in the quest to fulfil these project goals and objectives. It has been much more than
an honour to be by your side and I hope to continue our relationship for many years to
come. Thank you.
I acknowledge my friends and colleagues in the Electrical Engineering department, am
convinced that you have professionally influenced this project and my life in more than
one way. Thank you.
Last but not least, my professional thanks to my project supervisor Dr. Vitalice
Oduol. Your guidance and coaching skills have made this project a reality. I highly
appreciate your commitment and availability, always addressing issues relating to this
project. Thank you. Dr. Mwema our project co-coordinator, thank you for your lectures
on report writing and creating a good environment for experimentation and verification
of this project.
Everyday am more convinced and persuaded that I would have not reached thus
far, if it were not for The Lord God Almighty. Truly, words will fail to reflect and
reciprocate what you are to me and to what extent you have affected my life. It is
amazing. In more than many ways, you have given me the strength, agility and ability to
achieve my goals. I love you God. Thank you.
ii

Abstract
Federal Communications Commission (FCC) of the United States of America
approval of the unlicensed frequency band between 3.1 GHz and 10.6 GHz (7500 MHz)
for indoor UWB wireless communication systems in 2002; sparked newfound
excitement about UWB communications. Ultra-Wideband Impulse Radio (UWB-IR)
with its huge advantages has been recognized as a great solution for future Wireless
Sensor Networks (WSN) and Radio Frequency Identification (RFID). UWB-IR
technique has the possibility of achieving Gbps of data rate, hundreds of meter
operation range, picoJoules energy per bit, centimetre accuracy of positioning, and low
cost implementation.
This project is focussed specifically on a software radio transceiver design for impulse-
based UWB with the ability to transmit a raw data rate of 100 Mbps yet encompasses
the adaptability of a reconfigurable digital receiver.
A 500 picoseconds wide Gaussian pulse is generated at the transmitter utilizing
the fast-switching characteristics of a step recovery diode. Critical components at the
receiver consist of a bank of ADCs performing parallel sampling and an FPGA
employed for data processing. Using a software radio design, BPSK modulation scheme
and digital receiver topology of Digital Leading Edge Detection (DLED) was used
along with a vast number of algorithms for data demodulation methods. Verification for
the design is accomplished through transmitter and receiver design simulation.
Ultimately, the transceiver design demonstrates the advantages and challenges of UWB
technology while boasting high data rate communication capability and providing the
flexibility of a research.
This project presentation in the last academic year was without simulating the
transceiver; a MATLAB SIMULINK simulation was carried out in order to analyse
baseband processing of the transceiver. Each of the corresponding transceiver blocks,
that is, the transmitter, channel and receiver simulations were performed separately. The
transceiver simulation used an AWGN channel for a 30dB signal-to-noise performance.
It is from the software approach of the transceiver that further implementation of
various case scenarios are affected by making changes in software prior to the actual
fabrication.
iii
Federal Communications Commission (FCC) of the United States of America
approval of the unlicensed frequency band between 3.1 GHz and 10.6 GHz (7500 MHz)
for indoor UWB wireless communication systems in 2002; sparked newfound
excitement about UWB communications. Ultra-Wideband Impulse Radio (UWB-IR)
with its huge advantages has been recognized as a great solution for future Wireless
Sensor Networks (WSN) and Radio Frequency Identification (RFID). UWB-IR
technique has the possibility of achieving Gbps of data rate, hundreds of meter
operation range, picoJoules energy per bit, centimetre accuracy of positioning, and low
cost implementation.
This project is focussed specifically on a software radio transceiver design for impulse-
based UWB with the ability to transmit a raw data rate of 100 Mbps yet encompasses
the adaptability of a reconfigurable digital receiver.
A 500 picoseconds wide Gaussian pulse is generated at the transmitter utilizing
the fast-switching characteristics of a step recovery diode. Critical components at the
receiver consist of a bank of ADCs performing parallel sampling and an FPGA
employed for data processing. Using a software radio design, BPSK modulation scheme
and digital receiver topology of Digital Leading Edge Detection (DLED) was used
along with a vast number of algorithms for data demodulation methods. Verification for
the design is accomplished through transmitter and receiver design simulation.
Ultimately, the transceiver design demonstrates the advantages and challenges of UWB
technology while boasting high data rate communication capability and providing the
flexibility of a research.
This project presentation in the last academic year was without simulating the
transceiver; a MATLAB SIMULINK simulation was carried out in order to analyse
baseband processing of the transceiver. Each of the corresponding transceiver blocks,
that is, the transmitter, channel and receiver simulations were performed separately. The
transceiver simulation used an AWGN channel for a 30dB signal-to-noise performance.
It is from the software approach of the transceiver that further implementation of
various case scenarios are affected by making changes in software prior to the actual
fabrication.
iii
Secure Best Marks with AI Grader
Need help grading? Try our AI Grader for instant feedback on your assignments.

Table of Contents
Acknowledgements ...................................................................................................... ii
Abstract ...................................................................................................................... iii
Table of Figures ......................................................................................................... vii
List of Abbreviations ................................................................................................ viii
Chapter 1 UWB Introduction .................................................................................... 1
1.1 What is Ultra Wideband ................................................................................... 1
1.2 Why Ultra Wideband ....................................................................................... 1
1.3 What is Transceiver ......................................................................................... 2
1.4 Project Objectives ............................................................................................ 2
1.5 Project Overview ............................................................................................. 3
Chapter 2 Issues Unique to UWB .............................................................................. 6
2.1 Introduction ..................................................................................................... 6
2.2 History ............................................................................................................ 6
2.3 Regulatory ....................................................................................................... 6
2.4 Antennas .......................................................................................................... 6
2.5 Propagation & Channel Model ......................................................................... 7
2.6 Modulations ..................................................................................................... 8
2.7 Timing acquisitions ......................................................................................... 9
2.8 Receiver Structures .......................................................................................... 9
2.9 Multiple Access ............................................................................................. 10
Chapter 3 UWB Transmitter Design Principles ...................................................... 11
3.1 Introduction ................................................................................................... 11
3.2 Impulse Analysis ........................................................................................... 11
3.2.1 Gaussian Pulse ........................................................................................ 12
3.2.2 Windowed Gaussian Pulse ...................................................................... 12
3.2.3 Calculating Power of Repetitively Sent Pulses ........................................ 13
3.3 UWB Transmitter Architecture....................................................................... 14
Acknowledgements ...................................................................................................... ii
Abstract ...................................................................................................................... iii
Table of Figures ......................................................................................................... vii
List of Abbreviations ................................................................................................ viii
Chapter 1 UWB Introduction .................................................................................... 1
1.1 What is Ultra Wideband ................................................................................... 1
1.2 Why Ultra Wideband ....................................................................................... 1
1.3 What is Transceiver ......................................................................................... 2
1.4 Project Objectives ............................................................................................ 2
1.5 Project Overview ............................................................................................. 3
Chapter 2 Issues Unique to UWB .............................................................................. 6
2.1 Introduction ..................................................................................................... 6
2.2 History ............................................................................................................ 6
2.3 Regulatory ....................................................................................................... 6
2.4 Antennas .......................................................................................................... 6
2.5 Propagation & Channel Model ......................................................................... 7
2.6 Modulations ..................................................................................................... 8
2.7 Timing acquisitions ......................................................................................... 9
2.8 Receiver Structures .......................................................................................... 9
2.9 Multiple Access ............................................................................................. 10
Chapter 3 UWB Transmitter Design Principles ...................................................... 11
3.1 Introduction ................................................................................................... 11
3.2 Impulse Analysis ........................................................................................... 11
3.2.1 Gaussian Pulse ........................................................................................ 12
3.2.2 Windowed Gaussian Pulse ...................................................................... 12
3.2.3 Calculating Power of Repetitively Sent Pulses ........................................ 13
3.3 UWB Transmitter Architecture....................................................................... 14

3.4 SRD Pulse Generators .................................................................................... 15
3.5 BPSK Modulation .......................................................................................... 17
3.6 Antennas ........................................................................................................ 17
Chapter 4 UWB Receiver Design Principles ............................................................ 19
4.1 Introduction ................................................................................................... 19
4.2 Receiver Design Architecture ....................................................................... 19
4.2.1 RF Front End ........................................................................................... 19
4.2.2 ADC/ Clock Distribution ......................................................................... 20
4.2.3 Digital Processing .................................................................................. 21
4.3 Digital Leading Edge Detection Receiver Topology ....................................... 22
4.4 Data demodulation ......................................................................................... 24
Chapter 5 UWB Transceiver Design Simulation ..................................................... 25
5.1 Introduction ................................................................................................... 25
5.2 QAM/BPSK Modulation ................................................................................ 25
5.3 UWB Transmitter ............................................................................................ 27
5.3.1 Bernoulli Binary Generator ..................................................................... 27
5.3.2 Buffer ..................................................................................................... 27
5.3.3 BPSK Modulator Baseband ..................................................................... 27
5.3.4 Discrete Time Scatter Plot Scope ............................................................ 28
5.3.5 Pad Block ............................................................................................... 28
5.3.6 IFFT Block ............................................................................................. 28
5.3.7 Unbuffer Block ....................................................................................... 29
5.3.8 Spectrum Scope ...................................................................................... 29
5.4 UWB Channel ................................................................................................ 29
5.5 UWB Receiver ............................................................................................... 30
5.5.1 FFT Block ............................................................................................... 31
5.5.2 Selector ................................................................................................... 31
5.5.3 To Frame Conversion .............................................................................. 31
3.5 BPSK Modulation .......................................................................................... 17
3.6 Antennas ........................................................................................................ 17
Chapter 4 UWB Receiver Design Principles ............................................................ 19
4.1 Introduction ................................................................................................... 19
4.2 Receiver Design Architecture ....................................................................... 19
4.2.1 RF Front End ........................................................................................... 19
4.2.2 ADC/ Clock Distribution ......................................................................... 20
4.2.3 Digital Processing .................................................................................. 21
4.3 Digital Leading Edge Detection Receiver Topology ....................................... 22
4.4 Data demodulation ......................................................................................... 24
Chapter 5 UWB Transceiver Design Simulation ..................................................... 25
5.1 Introduction ................................................................................................... 25
5.2 QAM/BPSK Modulation ................................................................................ 25
5.3 UWB Transmitter ............................................................................................ 27
5.3.1 Bernoulli Binary Generator ..................................................................... 27
5.3.2 Buffer ..................................................................................................... 27
5.3.3 BPSK Modulator Baseband ..................................................................... 27
5.3.4 Discrete Time Scatter Plot Scope ............................................................ 28
5.3.5 Pad Block ............................................................................................... 28
5.3.6 IFFT Block ............................................................................................. 28
5.3.7 Unbuffer Block ....................................................................................... 29
5.3.8 Spectrum Scope ...................................................................................... 29
5.4 UWB Channel ................................................................................................ 29
5.5 UWB Receiver ............................................................................................... 30
5.5.1 FFT Block ............................................................................................... 31
5.5.2 Selector ................................................................................................... 31
5.5.3 To Frame Conversion .............................................................................. 31

5.5.4 BPSK Demodulator ................................................................................. 31
5.6 Discussion And Analysis ................................................................................ 32
Chapter 6 UWB Applications .................................................................................. 33
6.1 Introduction .................................................................................................. 33
6.2 Radar Applications ....................................................................................... 33
6.3 Communications ............................................................................................ 34
6.4 Location Aware Communications................................................................... 35
Chapter 7 Future Work and Recommendations ...................................................... 38
7.1 Conclusion and Recommendations ................................................................. 38
7.2 Future Work ................................................................................................... 38
References .................................................................................................................. 40
Appendix A Summary of FCC Regulations on UWB .............................................. 41
Appendix B Circuit Design for UWB Transceiver .................................................. 43
Appendix C Transceiver System Simulation in MATLAB ...................................... 47
5.6 Discussion And Analysis ................................................................................ 32
Chapter 6 UWB Applications .................................................................................. 33
6.1 Introduction .................................................................................................. 33
6.2 Radar Applications ....................................................................................... 33
6.3 Communications ............................................................................................ 34
6.4 Location Aware Communications................................................................... 35
Chapter 7 Future Work and Recommendations ...................................................... 38
7.1 Conclusion and Recommendations ................................................................. 38
7.2 Future Work ................................................................................................... 38
References .................................................................................................................. 40
Appendix A Summary of FCC Regulations on UWB .............................................. 41
Appendix B Circuit Design for UWB Transceiver .................................................. 43
Appendix C Transceiver System Simulation in MATLAB ...................................... 47
Paraphrase This Document
Need a fresh take? Get an instant paraphrase of this document with our AI Paraphraser

Table of Figures
Figure 1.1 UWB Signal Design points .......................................................................... 1
Figure 1.2 Design Flowchart for the project ................................................................. 4
Figure 2.1 FCC Spectral mask for indoor applications .................................................. 7
Figure 2.2 UWB Modulation points ........................................................................... 8,9
Figure 3.1 Windowed Cosine Pulse ............................................................................ 13
Figure 3.2 Power available in the UWB Band and in the signal .................................. 14
Figure 3.3 UWB generic transmitter Architecture ....................................................... 14
Figure 3.4 SRD current and stored charge ............................................................. 15,16
Figure 3.5 Comparison between transmitted & Radiated Pulse ................................... 18
Figure 4.1 UWB Receiver Design ............................................................................... 19
Figure 4.2 Block diagram of Parallel Time Sampling technique .................................. 21
Figure 4.3 Threshold detection in Gaussian Noise ...................................................... 24
Figure 5.1 QAM/BPSK Modulation Model ................................................................. 26
Figure 5.2 QAM/BPSK Modulation Scope Display .................................................... 26
Figure 5.3 UWB Transmitter design block in MATLAB ............................................. 27
Figure 5.4 Transmitted Waveform In MATLAB .......................................................... 29
Figure 5.5 UWB Channel with AGWN ....................................................................... 30
Figure 5.6 UWB Receiver design block in MATLAB ................................................. 30
Figure 5.7 UWB Received Waveform in MATLAB .................................................... 32
Figure 6.1 Communication Applications ..................................................................... 35
Figure B.1 UWB Schematic of the Input .................................................................... 35
Figure B.2 UWB Schematic of the SRD Pulse Generator ............................................ 35
Figure B.3 UWB Receiver Circuit design ................................................................... 35
Figure C.1 UWB Transceiver Design in SIMULINK ................................................. 35
vii
Figure 1.1 UWB Signal Design points .......................................................................... 1
Figure 1.2 Design Flowchart for the project ................................................................. 4
Figure 2.1 FCC Spectral mask for indoor applications .................................................. 7
Figure 2.2 UWB Modulation points ........................................................................... 8,9
Figure 3.1 Windowed Cosine Pulse ............................................................................ 13
Figure 3.2 Power available in the UWB Band and in the signal .................................. 14
Figure 3.3 UWB generic transmitter Architecture ....................................................... 14
Figure 3.4 SRD current and stored charge ............................................................. 15,16
Figure 3.5 Comparison between transmitted & Radiated Pulse ................................... 18
Figure 4.1 UWB Receiver Design ............................................................................... 19
Figure 4.2 Block diagram of Parallel Time Sampling technique .................................. 21
Figure 4.3 Threshold detection in Gaussian Noise ...................................................... 24
Figure 5.1 QAM/BPSK Modulation Model ................................................................. 26
Figure 5.2 QAM/BPSK Modulation Scope Display .................................................... 26
Figure 5.3 UWB Transmitter design block in MATLAB ............................................. 27
Figure 5.4 Transmitted Waveform In MATLAB .......................................................... 29
Figure 5.5 UWB Channel with AGWN ....................................................................... 30
Figure 5.6 UWB Receiver design block in MATLAB ................................................. 30
Figure 5.7 UWB Received Waveform in MATLAB .................................................... 32
Figure 6.1 Communication Applications ..................................................................... 35
Figure B.1 UWB Schematic of the Input .................................................................... 35
Figure B.2 UWB Schematic of the SRD Pulse Generator ............................................ 35
Figure B.3 UWB Receiver Circuit design ................................................................... 35
Figure C.1 UWB Transceiver Design in SIMULINK ................................................. 35
vii

List of Abbreviations
FCC – Federal Communications Commissions
UWB – Ultra Wideband
Mbps – Million Bits per Second
SRD – Step Recovery Diode
PSD- Power Spectral Density
Pdf - Probability density function
dB - Decibels
BPSK – Binary Phase Shift Key
RF – Radio Frequency
ADC – Analogue Digital Converter
I-UWB, IR-UWB – Impulse Ultra Wideband
GHz – Gigahertz
DC – Direct Current
DSP – Digital Signal Processing
TH-PAM – Time Hopping Pulse Amplitude Modulation
PRF – Pulse Repetition Frequency
HPA – High Pass Amplifier
BPF- Band Pass Filter
LNA – Low Noise Amplifier
SNR – Signal-to-Noise Ratio
QAM – Quadrature Amplitude Modulation
FPGA – Field Programmable Gate Array
PBER – Probability Bit Error Rate
PD – Probability Of Detection
CFAR – Constant False Alarm Rate
EIRP – Effective Isotropically Radiated Power
LPI – Low Probability of Intercept
DLED – Digital Leading Edge Detection
vii
FCC – Federal Communications Commissions
UWB – Ultra Wideband
Mbps – Million Bits per Second
SRD – Step Recovery Diode
PSD- Power Spectral Density
Pdf - Probability density function
dB - Decibels
BPSK – Binary Phase Shift Key
RF – Radio Frequency
ADC – Analogue Digital Converter
I-UWB, IR-UWB – Impulse Ultra Wideband
GHz – Gigahertz
DC – Direct Current
DSP – Digital Signal Processing
TH-PAM – Time Hopping Pulse Amplitude Modulation
PRF – Pulse Repetition Frequency
HPA – High Pass Amplifier
BPF- Band Pass Filter
LNA – Low Noise Amplifier
SNR – Signal-to-Noise Ratio
QAM – Quadrature Amplitude Modulation
FPGA – Field Programmable Gate Array
PBER – Probability Bit Error Rate
PD – Probability Of Detection
CFAR – Constant False Alarm Rate
EIRP – Effective Isotropically Radiated Power
LPI – Low Probability of Intercept
DLED – Digital Leading Edge Detection
vii

Chapter 1 Introduction
1.1 What is Ultra-Wideband
A UWB system is defined as any radio system that has a 10-db bandwidth larger
than 20% of its center frequency or has a 10-db bandwidth equal to and larger than
500MHz. In other words, a UWB system is also defined by the FCC as any wireless
scheme that occupies a fractional bandwidth W/f c≥ 20% where W is the transmission
bandwidth and f c is the band center, or more than 500MHz of absolute bandwidth. The
formula proposed for calculating fractional bandwidth is 2(f H - fL)/ (f H + fL) where f H
represents the upper frequency of the 10-db emission limit and f L represents the lower
frequency limit of the 10-db emission limit. The approval of UWB technology made by
the FCC of the United States in 2002 reserves the unlimited frequency band between
3.1GHz and 10.6GHz for indoor UWB wireless communication system.
Figure 1.1 UWB signal design points [1].
1.2 Why Ultra wideband
UWB has clearly shown various advantages in both indoor and industrial applications,
which encourage its use as a promising solution for high data rate short-range and
moderate range wireless communications and ranging. Its centimeter accuracy in
ranging and communications provide unique solutions to applications including
logistics, security applications, medical applications, control of home appliances, search
and rescue, family communications, supervision of children and military applications.
UWB has several features that differentiate it from conventional narrowband systems.
This includes:
i) Large instantaneous bandwidth enables fine time resolution for network time
distribution, precision location capability, or use as radar.
1
1.1 What is Ultra-Wideband
A UWB system is defined as any radio system that has a 10-db bandwidth larger
than 20% of its center frequency or has a 10-db bandwidth equal to and larger than
500MHz. In other words, a UWB system is also defined by the FCC as any wireless
scheme that occupies a fractional bandwidth W/f c≥ 20% where W is the transmission
bandwidth and f c is the band center, or more than 500MHz of absolute bandwidth. The
formula proposed for calculating fractional bandwidth is 2(f H - fL)/ (f H + fL) where f H
represents the upper frequency of the 10-db emission limit and f L represents the lower
frequency limit of the 10-db emission limit. The approval of UWB technology made by
the FCC of the United States in 2002 reserves the unlimited frequency band between
3.1GHz and 10.6GHz for indoor UWB wireless communication system.
Figure 1.1 UWB signal design points [1].
1.2 Why Ultra wideband
UWB has clearly shown various advantages in both indoor and industrial applications,
which encourage its use as a promising solution for high data rate short-range and
moderate range wireless communications and ranging. Its centimeter accuracy in
ranging and communications provide unique solutions to applications including
logistics, security applications, medical applications, control of home appliances, search
and rescue, family communications, supervision of children and military applications.
UWB has several features that differentiate it from conventional narrowband systems.
This includes:
i) Large instantaneous bandwidth enables fine time resolution for network time
distribution, precision location capability, or use as radar.
1
Secure Best Marks with AI Grader
Need help grading? Try our AI Grader for instant feedback on your assignments.

ii) Short duration pulses are able to provide robust performance in dense multipath
environments by exploiting paths that are more resolvable. This allows better
immunity to multi-path propagation.
iii) Low power spectral density allows co-existence with existing users and has a
low probability of intercept (LPI). This permits low interference.
iv) Data may be traded for power spectral density and multipath performance.
v) Availability of low cost transceivers, low transmit power.
Taken together these features give UWB systems a clear technical advantage over the
more conventional approaches in high multipath environments at low to medium data
rates. These arguments prove why UWB is the preferred choice in high multipath
environments at low to medium data rates.
1.3 What is a UWB Transceiver
A transceiver is a device that has both a transmitter and receiver, which are combined to
share common circuitry or a single housing, e.g. a walkie-talkie. Hence, a UWB
transceiver incorporates both the UWB transmitter and receiver in a single housing.
Trends in UWB transceiver design methods have been proposed to overcome various
unresolved issues in low complexity channel acquisitions transceiver design. The
methods have been classified into two primary categories:-Digital and mixed
analog/digital (solutions) schemes.
1.4 Project Objectives
The main aim of this project is:
• To study Ultra-Wideband Communications System
• To design a transceiver system based UWB
• To demonstrate that the UWB transceiver works
With the objectives in hand, the corresponding experiments, investigations, discussions
and solutions were performed to design UWB systems. UWB has proven to be a
stimulating, exciting, rejuvenating and brain storming communication system that is
wide in scope in terms of knowledge and experimentation. It has been proven that in
order to design any transceiver system based on UWB several factors have to be
evaluated, namely [1]:
i) What is permissible under the rules & regulations?
ii) What level of co-existence with other services in the frequency band is desirable
iii) What technological constraints there are from the feasibility, cost and
marketability points of view?
2
environments by exploiting paths that are more resolvable. This allows better
immunity to multi-path propagation.
iii) Low power spectral density allows co-existence with existing users and has a
low probability of intercept (LPI). This permits low interference.
iv) Data may be traded for power spectral density and multipath performance.
v) Availability of low cost transceivers, low transmit power.
Taken together these features give UWB systems a clear technical advantage over the
more conventional approaches in high multipath environments at low to medium data
rates. These arguments prove why UWB is the preferred choice in high multipath
environments at low to medium data rates.
1.3 What is a UWB Transceiver
A transceiver is a device that has both a transmitter and receiver, which are combined to
share common circuitry or a single housing, e.g. a walkie-talkie. Hence, a UWB
transceiver incorporates both the UWB transmitter and receiver in a single housing.
Trends in UWB transceiver design methods have been proposed to overcome various
unresolved issues in low complexity channel acquisitions transceiver design. The
methods have been classified into two primary categories:-Digital and mixed
analog/digital (solutions) schemes.
1.4 Project Objectives
The main aim of this project is:
• To study Ultra-Wideband Communications System
• To design a transceiver system based UWB
• To demonstrate that the UWB transceiver works
With the objectives in hand, the corresponding experiments, investigations, discussions
and solutions were performed to design UWB systems. UWB has proven to be a
stimulating, exciting, rejuvenating and brain storming communication system that is
wide in scope in terms of knowledge and experimentation. It has been proven that in
order to design any transceiver system based on UWB several factors have to be
evaluated, namely [1]:
i) What is permissible under the rules & regulations?
ii) What level of co-existence with other services in the frequency band is desirable
iii) What technological constraints there are from the feasibility, cost and
marketability points of view?
2

These and many other factors formed the foundational basis for the questions that
needed to be answered for any UWB transceiver, design to be viable. The regulations
define the broad rules & conditions for UWB communications systems to access &
share a 7.5GHz swath of spectrum extending from 3.1GHz to 10.6GHz. To gain market
acceptance, UWB radios will need to be cheaper, flexible and to co-exist with, share
and even perhaps interoperate with other radio services.
Furthermore, UWB technology will need to be physically implemented in cost-effective
integrated circuits. With adequate knowledge and foresight in hand, an impulse-UWB
communication system was design based on software-defined radio. A UWB transceiver
system had the following specifications:
• Data rate of 100 Mbps
• Transmitter design:
• Modulation Schemes: BPSK
• Receiver Design: Digital Leading Edge Detection
Simulation in MATLAB version 7.6.0.324 (R2008a) was to essentially show and proof
that these design objectives were tested, experimented and proved. The system
simulation was necessary to uncover any design flaws and calculate system performance
statistics based on the final system design. Finally, the optimistic results from these
simulations help support the overall goal of producing a UWB transceiver capable of
high data rate.
1.5 Project Overview
This project report is organised in such a way that each design objectives has been
experimented, investigated, researched, discussed and interpreted with relevant
conclusions and summaries being finalised. It is through these incentives and
innovations that the project has been classified into seven major topics namely
introduction to UWB, transmitter design, receiver design, system simulation,
applications and future work. Similarly, each topic has two sections, the introduction,
and main text. A flowchart maps the direction to realizing these project goals and
objectives as shown on figure 1.2.
3
needed to be answered for any UWB transceiver, design to be viable. The regulations
define the broad rules & conditions for UWB communications systems to access &
share a 7.5GHz swath of spectrum extending from 3.1GHz to 10.6GHz. To gain market
acceptance, UWB radios will need to be cheaper, flexible and to co-exist with, share
and even perhaps interoperate with other radio services.
Furthermore, UWB technology will need to be physically implemented in cost-effective
integrated circuits. With adequate knowledge and foresight in hand, an impulse-UWB
communication system was design based on software-defined radio. A UWB transceiver
system had the following specifications:
• Data rate of 100 Mbps
• Transmitter design:
• Modulation Schemes: BPSK
• Receiver Design: Digital Leading Edge Detection
Simulation in MATLAB version 7.6.0.324 (R2008a) was to essentially show and proof
that these design objectives were tested, experimented and proved. The system
simulation was necessary to uncover any design flaws and calculate system performance
statistics based on the final system design. Finally, the optimistic results from these
simulations help support the overall goal of producing a UWB transceiver capable of
high data rate.
1.5 Project Overview
This project report is organised in such a way that each design objectives has been
experimented, investigated, researched, discussed and interpreted with relevant
conclusions and summaries being finalised. It is through these incentives and
innovations that the project has been classified into seven major topics namely
introduction to UWB, transmitter design, receiver design, system simulation,
applications and future work. Similarly, each topic has two sections, the introduction,
and main text. A flowchart maps the direction to realizing these project goals and
objectives as shown on figure 1.2.
3

Figure 1.2 Design Flowchart for the project
Each communication system has its relevant protocols that are unique to that particular
system. Chapter 2 discusses the issues (protocols) which are specific and unique to
UWB such as regulations, modulation, transmitter, receiver and the UWB signal. The
Federal Communications Commission (FCC) in the United States the equivalent of the
Communications Council of Kenya (CCK) sets down these rules and regulations.
Chapter 3 gives a thorough discussion of the transmitter design, which comprises of
impulse analysis, data generation, SRD generator, modulation and antennas. Details of
the transmitter architecture were discussed at length with emphasis on the specifications
of each design block being evaluated.
4
Each communication system has its relevant protocols that are unique to that particular
system. Chapter 2 discusses the issues (protocols) which are specific and unique to
UWB such as regulations, modulation, transmitter, receiver and the UWB signal. The
Federal Communications Commission (FCC) in the United States the equivalent of the
Communications Council of Kenya (CCK) sets down these rules and regulations.
Chapter 3 gives a thorough discussion of the transmitter design, which comprises of
impulse analysis, data generation, SRD generator, modulation and antennas. Details of
the transmitter architecture were discussed at length with emphasis on the specifications
of each design block being evaluated.
4
Paraphrase This Document
Need a fresh take? Get an instant paraphrase of this document with our AI Paraphraser

The receiver design in chapter 4 has schematics such as RF Front End, ADC clock and
digital processing. There being various receiver design topologies only the digital
leading edge detection proved efficient for the design. This is so because it less
complex, easy to understand and does not require any synchronization. Hence, the
receiver design structure is less as no synchronization between the transmitter and
receiver is necessary.
Science as a study comprises of hypothesis, theory and experiments. Chapter 5 is the
experimental part of this project; it seeks to justify the theoretical and hypothetical
approach of the previous chapters. Simulation was in MATLAB 7.6.0.324 (R2008a)
using its numerous design tools and block sets in SIMULINK. MATLAB performed the
system simulation by inserting data and performing the digital processing, each of these
procedures along with channel details described herein.
Any system design is gauged on, not only its performance, but also its applications and
relevance in providing solutions in the physical and natural world. Chapter 6 answers
questions to UWB importance in solving communication and engineering problems.
Future work and recommendations in chapter 7 focuses on UWB transceiver design
implementations, additions and changes that could effect.
5
digital processing. There being various receiver design topologies only the digital
leading edge detection proved efficient for the design. This is so because it less
complex, easy to understand and does not require any synchronization. Hence, the
receiver design structure is less as no synchronization between the transmitter and
receiver is necessary.
Science as a study comprises of hypothesis, theory and experiments. Chapter 5 is the
experimental part of this project; it seeks to justify the theoretical and hypothetical
approach of the previous chapters. Simulation was in MATLAB 7.6.0.324 (R2008a)
using its numerous design tools and block sets in SIMULINK. MATLAB performed the
system simulation by inserting data and performing the digital processing, each of these
procedures along with channel details described herein.
Any system design is gauged on, not only its performance, but also its applications and
relevance in providing solutions in the physical and natural world. Chapter 6 answers
questions to UWB importance in solving communication and engineering problems.
Future work and recommendations in chapter 7 focuses on UWB transceiver design
implementations, additions and changes that could effect.
5

Chapter 2 Issues Unique to UWB
2.1 Introduction
UWB has a unique set of design requirements, and attempting to apply the principles for
traditional narrowband or even broadband communications to the design of I-UWB
systems can be misleading. This chapter sheds light on protocols that are unique to
UWB systems and design
2.2 History
Although, often considered as a recent breakthrough in wireless communications, UWB
has actually experienced well over 40 years of technological developments. The
physical cornerstone for understanding UWB pulse propagation established by
Somerfield a century ago (1901) when he attacked the diffraction of a time-domain
pulse by a perfectly conducting wedge [2]. In fact, one may reasonably argue that UWB
actually had its origins in the spark-gap transmission design of Marconi and Hertz in the
late 1890s [4]. In other words, the first wireless communication system was based on
UWB. Owing to the technical limitations, narrowband communication was preferred to
UWB; UWB followed a similar path with early systems designed for military covert
radar and communication. After the accelerating development since 1994 when some of
the research activities were declassified [3], UWB picked up momentum after the FCC
notice of inquiry in 1998. The interest in UWB was ‘sparked’ since the FCC issued a
Report and Order allowing its commercial deployment with a given spectral-mask
requirement for both indoor and outdoor applications
2.3 Regulatory
UWB technology is defined by the FCC as any wireless scheme that occupies a
fractional bandwidth W/fc ≥ 20 %, where W is the transmission bandwidth and fc is the
band centre, or more than 500 MHz of absolute bandwidth. The FCC approved [5] the
deployment of UWB on an unlicensed basis in the 3.1–10.6 GHz band subject to a
modified version of Part 15.209 rules. The essence of the rulings is that power spectral
density (PSD) of the modulated UWB signal must satisfy the spectral masks specified
by spectrum regulating agencies. The spectral mask for indoor applications specified by
the FCC in the United States is in Figure 1.1.
2.4 Antennas
UWB antennas are modelled as the front-end pulse shaping filters that affect the
baseband detection.
6
2.1 Introduction
UWB has a unique set of design requirements, and attempting to apply the principles for
traditional narrowband or even broadband communications to the design of I-UWB
systems can be misleading. This chapter sheds light on protocols that are unique to
UWB systems and design
2.2 History
Although, often considered as a recent breakthrough in wireless communications, UWB
has actually experienced well over 40 years of technological developments. The
physical cornerstone for understanding UWB pulse propagation established by
Somerfield a century ago (1901) when he attacked the diffraction of a time-domain
pulse by a perfectly conducting wedge [2]. In fact, one may reasonably argue that UWB
actually had its origins in the spark-gap transmission design of Marconi and Hertz in the
late 1890s [4]. In other words, the first wireless communication system was based on
UWB. Owing to the technical limitations, narrowband communication was preferred to
UWB; UWB followed a similar path with early systems designed for military covert
radar and communication. After the accelerating development since 1994 when some of
the research activities were declassified [3], UWB picked up momentum after the FCC
notice of inquiry in 1998. The interest in UWB was ‘sparked’ since the FCC issued a
Report and Order allowing its commercial deployment with a given spectral-mask
requirement for both indoor and outdoor applications
2.3 Regulatory
UWB technology is defined by the FCC as any wireless scheme that occupies a
fractional bandwidth W/fc ≥ 20 %, where W is the transmission bandwidth and fc is the
band centre, or more than 500 MHz of absolute bandwidth. The FCC approved [5] the
deployment of UWB on an unlicensed basis in the 3.1–10.6 GHz band subject to a
modified version of Part 15.209 rules. The essence of the rulings is that power spectral
density (PSD) of the modulated UWB signal must satisfy the spectral masks specified
by spectrum regulating agencies. The spectral mask for indoor applications specified by
the FCC in the United States is in Figure 1.1.
2.4 Antennas
UWB antennas are modelled as the front-end pulse shaping filters that affect the
baseband detection.
6
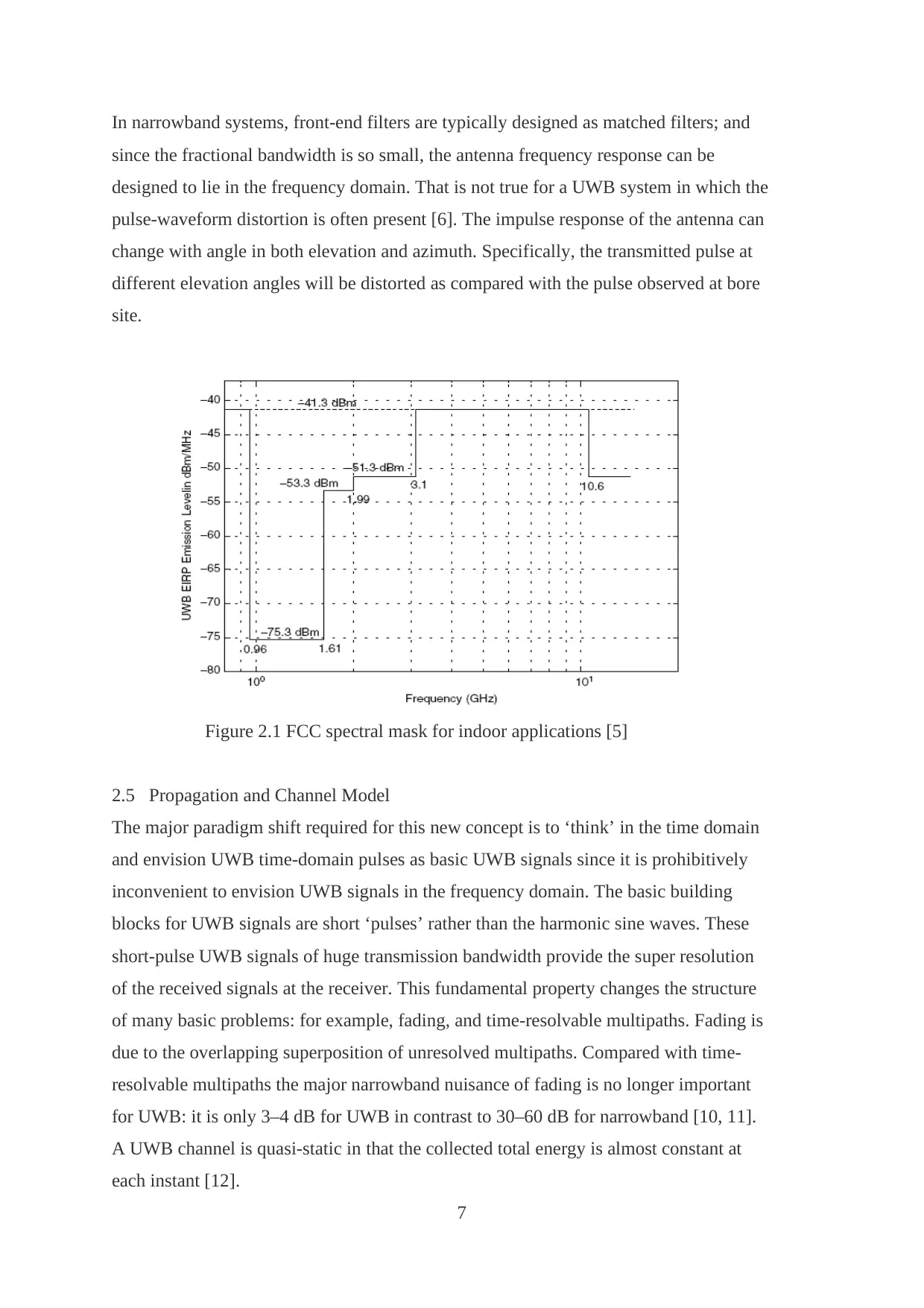
In narrowband systems, front-end filters are typically designed as matched filters; and
since the fractional bandwidth is so small, the antenna frequency response can be
designed to lie in the frequency domain. That is not true for a UWB system in which the
pulse-waveform distortion is often present [6]. The impulse response of the antenna can
change with angle in both elevation and azimuth. Specifically, the transmitted pulse at
different elevation angles will be distorted as compared with the pulse observed at bore
site.
Figure 2.1 FCC spectral mask for indoor applications [5]
2.5 Propagation and Channel Model
The major paradigm shift required for this new concept is to ‘think’ in the time domain
and envision UWB time-domain pulses as basic UWB signals since it is prohibitively
inconvenient to envision UWB signals in the frequency domain. The basic building
blocks for UWB signals are short ‘pulses’ rather than the harmonic sine waves. These
short-pulse UWB signals of huge transmission bandwidth provide the super resolution
of the received signals at the receiver. This fundamental property changes the structure
of many basic problems: for example, fading, and time-resolvable multipaths. Fading is
due to the overlapping superposition of unresolved multipaths. Compared with time-
resolvable multipaths the major narrowband nuisance of fading is no longer important
for UWB: it is only 3–4 dB for UWB in contrast to 30–60 dB for narrowband [10, 11].
A UWB channel is quasi-static in that the collected total energy is almost constant at
each instant [12].
7
since the fractional bandwidth is so small, the antenna frequency response can be
designed to lie in the frequency domain. That is not true for a UWB system in which the
pulse-waveform distortion is often present [6]. The impulse response of the antenna can
change with angle in both elevation and azimuth. Specifically, the transmitted pulse at
different elevation angles will be distorted as compared with the pulse observed at bore
site.
Figure 2.1 FCC spectral mask for indoor applications [5]
2.5 Propagation and Channel Model
The major paradigm shift required for this new concept is to ‘think’ in the time domain
and envision UWB time-domain pulses as basic UWB signals since it is prohibitively
inconvenient to envision UWB signals in the frequency domain. The basic building
blocks for UWB signals are short ‘pulses’ rather than the harmonic sine waves. These
short-pulse UWB signals of huge transmission bandwidth provide the super resolution
of the received signals at the receiver. This fundamental property changes the structure
of many basic problems: for example, fading, and time-resolvable multipaths. Fading is
due to the overlapping superposition of unresolved multipaths. Compared with time-
resolvable multipaths the major narrowband nuisance of fading is no longer important
for UWB: it is only 3–4 dB for UWB in contrast to 30–60 dB for narrowband [10, 11].
A UWB channel is quasi-static in that the collected total energy is almost constant at
each instant [12].
7
Secure Best Marks with AI Grader
Need help grading? Try our AI Grader for instant feedback on your assignments.

Thus, time-resolvable multipaths will become the dominant mechanism for energy
transmission and captures, since a huge number (hundreds) of paths are resolved in
contrast to several paths (in a 5 MHz system). The transmitted energy is carried through
these time-resolvable multipaths. The UWB pulse signals associated with those
individual paths are the basic building blocks of a UWB system and require a careful
treatment. Another conceptual difference is in the necessity to consider the per-path
pulse distortion [2] that is neglected in Turin’s multipath channel model (Turin 1958)
widely used in wireless communications. Turin’s model is based on empirical
experimental data obtained using a typical narrowband measurement system. The
generalized multipath model [2] is based on the transient physical mechanisms obtained
directly from Maxwell’s equations.
2.6 Modulations
In choosing modulation schemes for UWB systems [2], one must consider a number of
aspects such as data rate, transceiver complexity, spectral characteristics, robustness
against narrowband interference, inter symbol interference, error performance, and so
on. For pulsed UWB systems, the widely used forms of modulation schemes include
pulse amplitude modulation (PAM), on off keying (OOK), and pulse position
modulation (PPM). To satisfy the FCC spectral mask, passband pulses are used to
transmit information in pulsed UWB systems. Although these passband pulses could be
obtained by modulating a baseband pulse using a sinusoidal carrier signal, the term
binary phase-shift keying (BPSK) is still somewhat imprecise in the context of pulsed
UWB signalling as the carrier-modulated baseband pulse is usually treated as a single
entity – the UWB pulse shape. The three modulation schemes mentioned above are
illustrated in Figure 2.2. For a single-user, system with binary PPM signalling, bit ‘1’ is
represented by a pulse without any delay and bit ‘0’ is represented by a pulse with a
delay τ relative to the time reference. For binary PAM signalling, information bits
modulate the pulse polarity. For OOK signalling, information bit ‘1’ is represented by
the presence of a pulse and no pulse is sent for bit ‘0’. Binary PAM and PPM schemes
have similar performances.
8
transmission and captures, since a huge number (hundreds) of paths are resolved in
contrast to several paths (in a 5 MHz system). The transmitted energy is carried through
these time-resolvable multipaths. The UWB pulse signals associated with those
individual paths are the basic building blocks of a UWB system and require a careful
treatment. Another conceptual difference is in the necessity to consider the per-path
pulse distortion [2] that is neglected in Turin’s multipath channel model (Turin 1958)
widely used in wireless communications. Turin’s model is based on empirical
experimental data obtained using a typical narrowband measurement system. The
generalized multipath model [2] is based on the transient physical mechanisms obtained
directly from Maxwell’s equations.
2.6 Modulations
In choosing modulation schemes for UWB systems [2], one must consider a number of
aspects such as data rate, transceiver complexity, spectral characteristics, robustness
against narrowband interference, inter symbol interference, error performance, and so
on. For pulsed UWB systems, the widely used forms of modulation schemes include
pulse amplitude modulation (PAM), on off keying (OOK), and pulse position
modulation (PPM). To satisfy the FCC spectral mask, passband pulses are used to
transmit information in pulsed UWB systems. Although these passband pulses could be
obtained by modulating a baseband pulse using a sinusoidal carrier signal, the term
binary phase-shift keying (BPSK) is still somewhat imprecise in the context of pulsed
UWB signalling as the carrier-modulated baseband pulse is usually treated as a single
entity – the UWB pulse shape. The three modulation schemes mentioned above are
illustrated in Figure 2.2. For a single-user, system with binary PPM signalling, bit ‘1’ is
represented by a pulse without any delay and bit ‘0’ is represented by a pulse with a
delay τ relative to the time reference. For binary PAM signalling, information bits
modulate the pulse polarity. For OOK signalling, information bit ‘1’ is represented by
the presence of a pulse and no pulse is sent for bit ‘0’. Binary PAM and PPM schemes
have similar performances.
8

Figure 2.2 UWB Modulation options
OOK and PPM signals have discrete spectral lines. These spectral lines could cause
severe interference to existing narrowband radios, and various techniques such as
random dithering could be applied in PPM to lower these discrete spectral lines and
smoothen the spectrum. Because of the random polarities of the information symbols,
the PAM scheme inherently offers a smooth PSD when averaged over a number of
symbol intervals. In this sense, PAM signalling is attractive.
2.7 Timing Acquisition
Timing acquisition is the first operating stage in any communication system. It is a
difficult task in the UWB system owing to its limited transmitted power and a high-
resolution multipath. Low transmitted power implies a long search time to obtain
reliable timing, while a received signal with multipath components can result in a set of
‘good’ signal phases within the search window, making the decision much more
complex.
2.8 Transmitter and Receiver Design
RF design for UWB systems is distinct from traditional narrowband or broadband
systems in several ways. The extremely wide bandwidth of a UWB necessitates RF
components that have flat frequency responses. Significant deviation, or ripple, in the
frequency response of RF components as well as the nonlinearities present in all RF
devices will introduce distortion to the UWB signal. UWB transmitted signals also have
a very high peak-to-average power ratio (PAPR). As RF components are peak power
limited, it becomes important to ensure that all RF devices have a power handling
capacity at least as great as the peak power in the UWB signal.
9
OOK and PPM signals have discrete spectral lines. These spectral lines could cause
severe interference to existing narrowband radios, and various techniques such as
random dithering could be applied in PPM to lower these discrete spectral lines and
smoothen the spectrum. Because of the random polarities of the information symbols,
the PAM scheme inherently offers a smooth PSD when averaged over a number of
symbol intervals. In this sense, PAM signalling is attractive.
2.7 Timing Acquisition
Timing acquisition is the first operating stage in any communication system. It is a
difficult task in the UWB system owing to its limited transmitted power and a high-
resolution multipath. Low transmitted power implies a long search time to obtain
reliable timing, while a received signal with multipath components can result in a set of
‘good’ signal phases within the search window, making the decision much more
complex.
2.8 Transmitter and Receiver Design
RF design for UWB systems is distinct from traditional narrowband or broadband
systems in several ways. The extremely wide bandwidth of a UWB necessitates RF
components that have flat frequency responses. Significant deviation, or ripple, in the
frequency response of RF components as well as the nonlinearities present in all RF
devices will introduce distortion to the UWB signal. UWB transmitted signals also have
a very high peak-to-average power ratio (PAPR). As RF components are peak power
limited, it becomes important to ensure that all RF devices have a power handling
capacity at least as great as the peak power in the UWB signal.
9

Furthermore, the coexistence of UWB and existing services means that the receiver will
detect narrow-band interfering signals. These narrowband signals can either corrupt the
pulse or saturate the RF front-end, decreasing the receiver's dynamic range and
effectively limiting the range of the UWB system. Introducing notch filters at the
receiver is a potential solution; pulse-shaping techniques, such as those described in,
provide an alternative method for mitigating narrowband interferers without distorting
the UWB waveform. Most UWB receiver techniques require highly accurate
synchronization with the transmitter as well as stable oscillators to maintain
synchronization. With certain I-UWB modulation schemes, conveying of data is by the
precise position or timing of the pulse and a loss of precise synchronization could result
in a loss of data.
2.9 Multiple Access
Various multiple-access schemes and their performances have been reported in the
literature. Time hopping (TH) has been found to be a good multiple access technique for
pulsed UWB systems. Direct sequence (DS) spreading is also an attractive method for
multiple access in UWB systems. Since pulsed UWB systems are inherently spread-
spectrum systems, the use of spreading codes in DS-UWB systems is solely for
accommodating multiple users. Firstly, in TH pulsed UWB systems, the pulse duty
cycle is very small. Thus, the transmitter is gated off for the bulk of a symbol period.
Multiple access implementation is by employing appropriately chosen hopping
sequences for different users to minimize the probability of collisions due to multiple
accessing. Secondly, DS can also be used for multiple access in a PAM-UWB or PPM-
UWB system. In such a system, each symbol is represented by a series of pulses that are
pulse-amplitude-modulated by a chip sequence. Input symbols are modulated onto
either the amplitude or the relative positions of each sequence of pulses.
10
detect narrow-band interfering signals. These narrowband signals can either corrupt the
pulse or saturate the RF front-end, decreasing the receiver's dynamic range and
effectively limiting the range of the UWB system. Introducing notch filters at the
receiver is a potential solution; pulse-shaping techniques, such as those described in,
provide an alternative method for mitigating narrowband interferers without distorting
the UWB waveform. Most UWB receiver techniques require highly accurate
synchronization with the transmitter as well as stable oscillators to maintain
synchronization. With certain I-UWB modulation schemes, conveying of data is by the
precise position or timing of the pulse and a loss of precise synchronization could result
in a loss of data.
2.9 Multiple Access
Various multiple-access schemes and their performances have been reported in the
literature. Time hopping (TH) has been found to be a good multiple access technique for
pulsed UWB systems. Direct sequence (DS) spreading is also an attractive method for
multiple access in UWB systems. Since pulsed UWB systems are inherently spread-
spectrum systems, the use of spreading codes in DS-UWB systems is solely for
accommodating multiple users. Firstly, in TH pulsed UWB systems, the pulse duty
cycle is very small. Thus, the transmitter is gated off for the bulk of a symbol period.
Multiple access implementation is by employing appropriately chosen hopping
sequences for different users to minimize the probability of collisions due to multiple
accessing. Secondly, DS can also be used for multiple access in a PAM-UWB or PPM-
UWB system. In such a system, each symbol is represented by a series of pulses that are
pulse-amplitude-modulated by a chip sequence. Input symbols are modulated onto
either the amplitude or the relative positions of each sequence of pulses.
10
Paraphrase This Document
Need a fresh take? Get an instant paraphrase of this document with our AI Paraphraser

Chapter 3 Transmitter Design
3.1 Introduction
This chapter on UWB transmitter design is thoroughly detailed in explaining the
generation of UWB signals, modulation and the impulse based UWB technique.
Generally, the first step in a radio communication link involves the generation of a
suitable signal, which is then modulated with the desired information and then
transmission using appropriate circuitry. The response of the antenna is also included.
The circuits and techniques described in this section are typical of transmitters used for
impulse UWB communications and ranging.
3.2 Impulse Analysis
There are two common forms of UWB: one based on sending very short duration pulses
to convey information and another approach using multiple simultaneous carriers. Pure
impulse radio, unlike classic communications, does not use a modulated sinusoidal
carrier to convey information, instead, the transmit signal is a series of baseband pulses
[5]. Because the pulses are extremely short (commonly in the nanosecond range or
shorter), the transmit signal bandwidth is in the order of gigahertz. Note that the
fractional bandwidth is greater than 20%. This means that:
i) we need to use as much bandwidth as possible to maximize the emitted power;
ii) because of the antenna aperture scaling, the lower frequencies in the
UWB band are the most valuable for our purposes. Design of signals is by shaping their
properties as a function of time. There is a one-to-one mapping between what a signal
looks like in time and its frequency spectrum. Mathematically, this relationship is by the
Fourier transform. In signal design, synthesis of UWB signals was with precision in
both the signal shape and its placement in the frequency spectrum. To that end, signals
shaping was at baseband then frequency shifted to the desired location in the spectrum
using Armstrong’s heterodyning technique. Band shaping was much easier to
accomplish at the baseband than it is at higher radio RFs. In the signal pulse design
examples a UWB signal that occupies the desired 3.1GHz to 5.1GHz segment was
chosen. Hence, a 2GHz bandwidth as measured between the points on either side of the
centre frequency and are 20db below the peak level. The spectrum centred at 4.1GHz. In
the current studies, Gaussian pulse modelling is the most used [3] but a windowed
cosine model seemed to be a better candidate for our restricted band.
11
3.1 Introduction
This chapter on UWB transmitter design is thoroughly detailed in explaining the
generation of UWB signals, modulation and the impulse based UWB technique.
Generally, the first step in a radio communication link involves the generation of a
suitable signal, which is then modulated with the desired information and then
transmission using appropriate circuitry. The response of the antenna is also included.
The circuits and techniques described in this section are typical of transmitters used for
impulse UWB communications and ranging.
3.2 Impulse Analysis
There are two common forms of UWB: one based on sending very short duration pulses
to convey information and another approach using multiple simultaneous carriers. Pure
impulse radio, unlike classic communications, does not use a modulated sinusoidal
carrier to convey information, instead, the transmit signal is a series of baseband pulses
[5]. Because the pulses are extremely short (commonly in the nanosecond range or
shorter), the transmit signal bandwidth is in the order of gigahertz. Note that the
fractional bandwidth is greater than 20%. This means that:
i) we need to use as much bandwidth as possible to maximize the emitted power;
ii) because of the antenna aperture scaling, the lower frequencies in the
UWB band are the most valuable for our purposes. Design of signals is by shaping their
properties as a function of time. There is a one-to-one mapping between what a signal
looks like in time and its frequency spectrum. Mathematically, this relationship is by the
Fourier transform. In signal design, synthesis of UWB signals was with precision in
both the signal shape and its placement in the frequency spectrum. To that end, signals
shaping was at baseband then frequency shifted to the desired location in the spectrum
using Armstrong’s heterodyning technique. Band shaping was much easier to
accomplish at the baseband than it is at higher radio RFs. In the signal pulse design
examples a UWB signal that occupies the desired 3.1GHz to 5.1GHz segment was
chosen. Hence, a 2GHz bandwidth as measured between the points on either side of the
centre frequency and are 20db below the peak level. The spectrum centred at 4.1GHz. In
the current studies, Gaussian pulse modelling is the most used [3] but a windowed
cosine model seemed to be a better candidate for our restricted band.
11

3.2.1 Gaussian pulse
A zero-order Gaussian pulse can be time-defined by:
(1)
where, σ is the standard deviation of the Gaussian signal. Successive derivations of (1)
obtained by recurrence with:
(2)
Moreover, the Fourier Transform of (2) is:
(3)
3.2.2 Windowed cosine impulse
Another type of pulse model is the windowed cosine pulse. Its temporal expression is:
(4)
where σ is the standard deviation of the zero-order Gaussian envelop and f 0 the central
frequency of the sinusoidal function. Fourier Transform of (4) is:
(5)
With this pulse model, parameters were selected independently in order to adjust pulse
width with frequency boundaries. Most of pulse generators try to approximate Gaussian
shapes but generations are difficult above n = 2 due to the number of oscillations
required [8]. To achieve these complex shapes, we decided to generate oscillations
firstly and secondly filter them to shape a desired pulse. The idea of using a windowed
cosine shape comes from the possibility of controlling oscillations and envelop
separately. With this model, we generate an UWB pulse by multiplying a sinusoidal
signal with an envelop close to a zero order Gaussian shape.
12
A zero-order Gaussian pulse can be time-defined by:
(1)
where, σ is the standard deviation of the Gaussian signal. Successive derivations of (1)
obtained by recurrence with:
(2)
Moreover, the Fourier Transform of (2) is:
(3)
3.2.2 Windowed cosine impulse
Another type of pulse model is the windowed cosine pulse. Its temporal expression is:
(4)
where σ is the standard deviation of the zero-order Gaussian envelop and f 0 the central
frequency of the sinusoidal function. Fourier Transform of (4) is:
(5)
With this pulse model, parameters were selected independently in order to adjust pulse
width with frequency boundaries. Most of pulse generators try to approximate Gaussian
shapes but generations are difficult above n = 2 due to the number of oscillations
required [8]. To achieve these complex shapes, we decided to generate oscillations
firstly and secondly filter them to shape a desired pulse. The idea of using a windowed
cosine shape comes from the possibility of controlling oscillations and envelop
separately. With this model, we generate an UWB pulse by multiplying a sinusoidal
signal with an envelop close to a zero order Gaussian shape.
12

Figure 3.1 Windowed cosine impulse [8].
3.2.3 Calculating Power for Repetitively Sent Pulses
A single isolated pulse delivers energy, measured in joules. Energy pulses are delivered
at an average signal pulse repetition frequency (PRF), so they appear as energy per unit
time (joules/s), or power. The emission rules and regulations specify limits on both
average power emissions and peak power. Measuring the average power emission as
effective isotropically radiated power (EIRP) – that is, power radiated by an antenna
with a gain of 1. The absolute peak power is measured over the duration of the pulses
sent repetitively. However, for purposes of conforming to regulations, peak power is the
power measured in a 1-MHz bandwidth. The total emitted power for a repetitively sent
signal S (f) whose peak PSD exactly equals the limit −41.3 dBm/MHz is found by
(6)
The peak amplitude value of S (f) had been set to one, and the frequency f is in hertz.
The closer S (f) conforms to the area under the PSD limits, the more power in the signal
is transmitted. If all available spectra from 3.1 to 10.6 GHz were perfectly filled with
the maximum allowed signal PSD, the total EIRP would amount to (10,600–3,100)
10−41.3/10 = 0.556 mW, equivalent to −2.55 dBm as shown in Figure 4.10. This
represents the absolute maximum possible EIRP limit for UWB under these particular
regulations. The figure also shows the average power, −10.6 dBm, represented by 2-
GHz wide pulses sent at some continuous PRF.
13
3.2.3 Calculating Power for Repetitively Sent Pulses
A single isolated pulse delivers energy, measured in joules. Energy pulses are delivered
at an average signal pulse repetition frequency (PRF), so they appear as energy per unit
time (joules/s), or power. The emission rules and regulations specify limits on both
average power emissions and peak power. Measuring the average power emission as
effective isotropically radiated power (EIRP) – that is, power radiated by an antenna
with a gain of 1. The absolute peak power is measured over the duration of the pulses
sent repetitively. However, for purposes of conforming to regulations, peak power is the
power measured in a 1-MHz bandwidth. The total emitted power for a repetitively sent
signal S (f) whose peak PSD exactly equals the limit −41.3 dBm/MHz is found by
(6)
The peak amplitude value of S (f) had been set to one, and the frequency f is in hertz.
The closer S (f) conforms to the area under the PSD limits, the more power in the signal
is transmitted. If all available spectra from 3.1 to 10.6 GHz were perfectly filled with
the maximum allowed signal PSD, the total EIRP would amount to (10,600–3,100)
10−41.3/10 = 0.556 mW, equivalent to −2.55 dBm as shown in Figure 4.10. This
represents the absolute maximum possible EIRP limit for UWB under these particular
regulations. The figure also shows the average power, −10.6 dBm, represented by 2-
GHz wide pulses sent at some continuous PRF.
13
Secure Best Marks with AI Grader
Need help grading? Try our AI Grader for instant feedback on your assignments.

Figure 3.2 Power available in the UWB band and in the signal [1].
3.3 UWB Transmitter Architecture
It is composed of a pulse generator followed by a modulator, all driven by a digital
baseband. The modulator's connection is directly to an amplifier to achieve required
power, a filter to reduce spurious emissions and a wideband antenna to radiate
information. Critical blocks of this architecture are:
• Pulse generator we have to find a tradeoff between generated pulses complexity,
consumption and integration.
• Antenna –We have to find a wideband antenna that minimises distortions and
ringing.
Figure 3.3 UWB generic transmitter architecture [8].
14
3.3 UWB Transmitter Architecture
It is composed of a pulse generator followed by a modulator, all driven by a digital
baseband. The modulator's connection is directly to an amplifier to achieve required
power, a filter to reduce spurious emissions and a wideband antenna to radiate
information. Critical blocks of this architecture are:
• Pulse generator we have to find a tradeoff between generated pulses complexity,
consumption and integration.
• Antenna –We have to find a wideband antenna that minimises distortions and
ringing.
Figure 3.3 UWB generic transmitter architecture [8].
14

3.4 SRD Pulse Generator
The heart of any I-UWB system is some type of fast-rise time step or pulse generator.
These pulse generators are used for both transmitting and receiving. I-UWB transmitters
convert data bits directly to fast-rise time pulses. Matched filter correlation receivers
must generate a template pulse that matches the incoming waveform. To generate ultra
short pulses for a UWB, historically two devices have been in use. A tunnel diode was
the original method in early UWB transmitters including the system built by Gerald F.
Ross. A tunnel diode is very successful in fast switching applications due to its negative
resistance region over part of its operating point; however, primary drawbacks of low
impedance, low voltage output and the fact that they have two terminals rend it
inefficient. Furthermore, tunnel diodes have not been in mainstream production since
the 1960s.
With tunnel diodes being difficult to find, step recovery diodes (SRDs) have become the
common source of generating UWB pulses. Step Recovery Diodes (SRD) or "snap off"
diodes can be used to make very fast-rise time pulses. Conventional diodes conduct
when forward biased and turn off when reverse biased. SRDs have a P-I-N structure,
and charge is stored in the intrinsic layer when the SRD is forward biased. This allows
the SRD to continue to conduct when the device is reverse biased. This reverse
conduction continues until the charge is swept out of the intrinsic layer; with the charge
gone, the diode abruptly stops conducting and "snaps off". The SRD presents low
impedance during the forward/ reverse conduction and transitions to high impedance
when it snaps off. Figure 3.4 plots charge versus time when a current is applied. During
the forward bias condition, minority carriers are injected into the junction. Due to the
intrinsic layer, recombination requires a finite amount of time so charge is stored in the
junction.
15
The heart of any I-UWB system is some type of fast-rise time step or pulse generator.
These pulse generators are used for both transmitting and receiving. I-UWB transmitters
convert data bits directly to fast-rise time pulses. Matched filter correlation receivers
must generate a template pulse that matches the incoming waveform. To generate ultra
short pulses for a UWB, historically two devices have been in use. A tunnel diode was
the original method in early UWB transmitters including the system built by Gerald F.
Ross. A tunnel diode is very successful in fast switching applications due to its negative
resistance region over part of its operating point; however, primary drawbacks of low
impedance, low voltage output and the fact that they have two terminals rend it
inefficient. Furthermore, tunnel diodes have not been in mainstream production since
the 1960s.
With tunnel diodes being difficult to find, step recovery diodes (SRDs) have become the
common source of generating UWB pulses. Step Recovery Diodes (SRD) or "snap off"
diodes can be used to make very fast-rise time pulses. Conventional diodes conduct
when forward biased and turn off when reverse biased. SRDs have a P-I-N structure,
and charge is stored in the intrinsic layer when the SRD is forward biased. This allows
the SRD to continue to conduct when the device is reverse biased. This reverse
conduction continues until the charge is swept out of the intrinsic layer; with the charge
gone, the diode abruptly stops conducting and "snaps off". The SRD presents low
impedance during the forward/ reverse conduction and transitions to high impedance
when it snaps off. Figure 3.4 plots charge versus time when a current is applied. During
the forward bias condition, minority carriers are injected into the junction. Due to the
intrinsic layer, recombination requires a finite amount of time so charge is stored in the
junction.
15

Figure 3.4 SRD current and stored charge
Let i d be the instantaneous diode current, Q the charge stored, and τ the carrier lifetime.
We have the following
(7)
When a constant forward bias current is applied to the diode, charge is stored in the
junction. The charge for a forward constant bias current is given by
(8)
where Q f is the charge stored due to forward current and t F is the time that forward
current is applied. If the forward current is applied for a time significantly longer than
the carrier lifetime, τ, the stored charge can be approximated by
(9)
When the reverse current is applied, the stored charge is depleted. The depletion time is
given by
(10)
where t s is the time to remove the stored charge and IR is the reverse current. Typical
SRD circuits operate with so (5.4) can be further simplified as
(11)
16
Let i d be the instantaneous diode current, Q the charge stored, and τ the carrier lifetime.
We have the following
(7)
When a constant forward bias current is applied to the diode, charge is stored in the
junction. The charge for a forward constant bias current is given by
(8)
where Q f is the charge stored due to forward current and t F is the time that forward
current is applied. If the forward current is applied for a time significantly longer than
the carrier lifetime, τ, the stored charge can be approximated by
(9)
When the reverse current is applied, the stored charge is depleted. The depletion time is
given by
(10)
where t s is the time to remove the stored charge and IR is the reverse current. Typical
SRD circuits operate with so (5.4) can be further simplified as
(11)
16
Paraphrase This Document
Need a fresh take? Get an instant paraphrase of this document with our AI Paraphraser
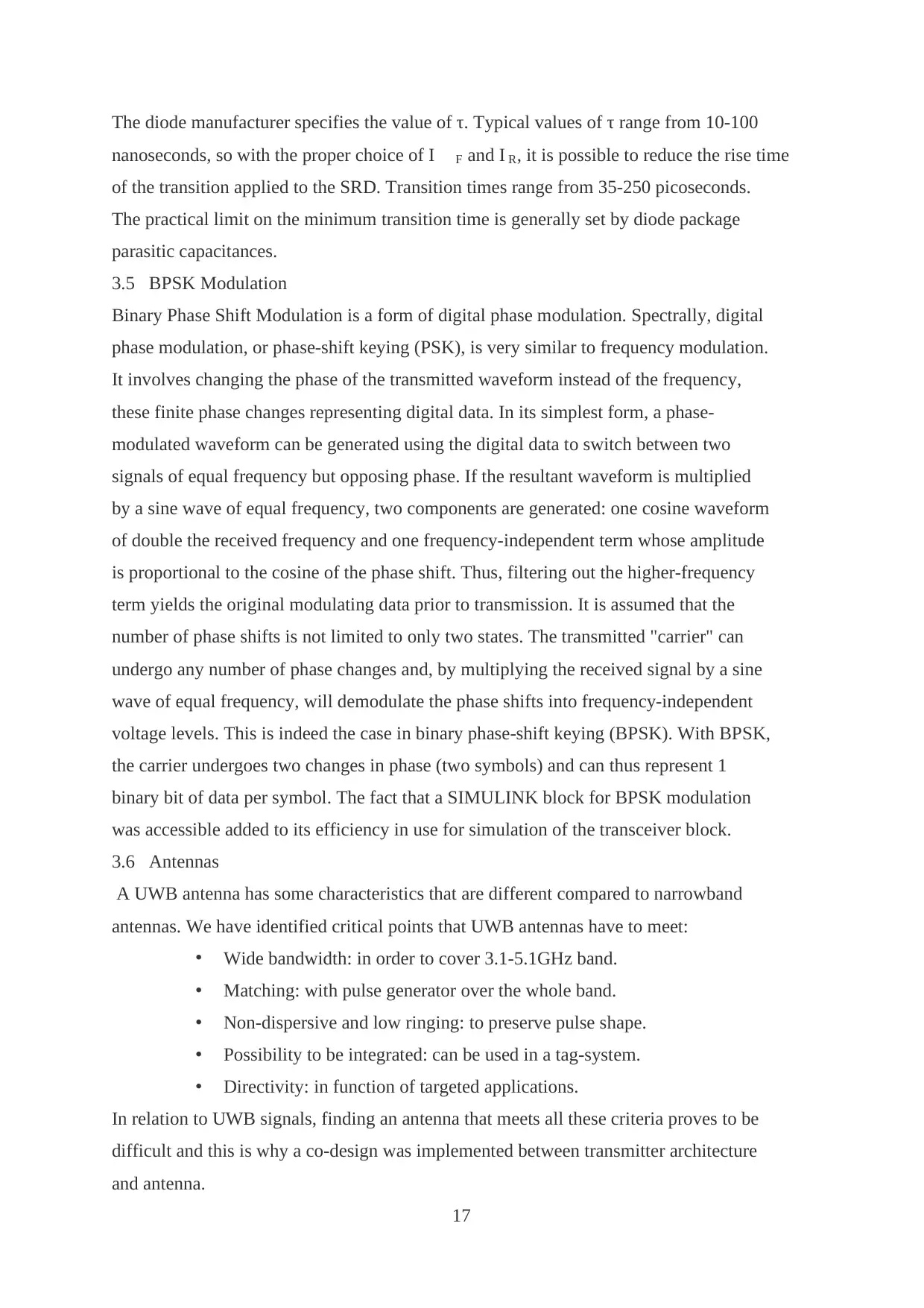
The diode manufacturer specifies the value of τ. Typical values of τ range from 10-100
nanoseconds, so with the proper choice of I F and I R, it is possible to reduce the rise time
of the transition applied to the SRD. Transition times range from 35-250 picoseconds.
The practical limit on the minimum transition time is generally set by diode package
parasitic capacitances.
3.5 BPSK Modulation
Binary Phase Shift Modulation is a form of digital phase modulation. Spectrally, digital
phase modulation, or phase-shift keying (PSK), is very similar to frequency modulation.
It involves changing the phase of the transmitted waveform instead of the frequency,
these finite phase changes representing digital data. In its simplest form, a phase-
modulated waveform can be generated using the digital data to switch between two
signals of equal frequency but opposing phase. If the resultant waveform is multiplied
by a sine wave of equal frequency, two components are generated: one cosine waveform
of double the received frequency and one frequency-independent term whose amplitude
is proportional to the cosine of the phase shift. Thus, filtering out the higher-frequency
term yields the original modulating data prior to transmission. It is assumed that the
number of phase shifts is not limited to only two states. The transmitted "carrier" can
undergo any number of phase changes and, by multiplying the received signal by a sine
wave of equal frequency, will demodulate the phase shifts into frequency-independent
voltage levels. This is indeed the case in binary phase-shift keying (BPSK). With BPSK,
the carrier undergoes two changes in phase (two symbols) and can thus represent 1
binary bit of data per symbol. The fact that a SIMULINK block for BPSK modulation
was accessible added to its efficiency in use for simulation of the transceiver block.
3.6 Antennas
A UWB antenna has some characteristics that are different compared to narrowband
antennas. We have identified critical points that UWB antennas have to meet:
• Wide bandwidth: in order to cover 3.1-5.1GHz band.
• Matching: with pulse generator over the whole band.
• Non-dispersive and low ringing: to preserve pulse shape.
• Possibility to be integrated: can be used in a tag-system.
• Directivity: in function of targeted applications.
In relation to UWB signals, finding an antenna that meets all these criteria proves to be
difficult and this is why a co-design was implemented between transmitter architecture
and antenna.
17
nanoseconds, so with the proper choice of I F and I R, it is possible to reduce the rise time
of the transition applied to the SRD. Transition times range from 35-250 picoseconds.
The practical limit on the minimum transition time is generally set by diode package
parasitic capacitances.
3.5 BPSK Modulation
Binary Phase Shift Modulation is a form of digital phase modulation. Spectrally, digital
phase modulation, or phase-shift keying (PSK), is very similar to frequency modulation.
It involves changing the phase of the transmitted waveform instead of the frequency,
these finite phase changes representing digital data. In its simplest form, a phase-
modulated waveform can be generated using the digital data to switch between two
signals of equal frequency but opposing phase. If the resultant waveform is multiplied
by a sine wave of equal frequency, two components are generated: one cosine waveform
of double the received frequency and one frequency-independent term whose amplitude
is proportional to the cosine of the phase shift. Thus, filtering out the higher-frequency
term yields the original modulating data prior to transmission. It is assumed that the
number of phase shifts is not limited to only two states. The transmitted "carrier" can
undergo any number of phase changes and, by multiplying the received signal by a sine
wave of equal frequency, will demodulate the phase shifts into frequency-independent
voltage levels. This is indeed the case in binary phase-shift keying (BPSK). With BPSK,
the carrier undergoes two changes in phase (two symbols) and can thus represent 1
binary bit of data per symbol. The fact that a SIMULINK block for BPSK modulation
was accessible added to its efficiency in use for simulation of the transceiver block.
3.6 Antennas
A UWB antenna has some characteristics that are different compared to narrowband
antennas. We have identified critical points that UWB antennas have to meet:
• Wide bandwidth: in order to cover 3.1-5.1GHz band.
• Matching: with pulse generator over the whole band.
• Non-dispersive and low ringing: to preserve pulse shape.
• Possibility to be integrated: can be used in a tag-system.
• Directivity: in function of targeted applications.
In relation to UWB signals, finding an antenna that meets all these criteria proves to be
difficult and this is why a co-design was implemented between transmitter architecture
and antenna.
17

Due to the shape of the generated pulse, the transmitted signal is similar to an
amplitude modulated signal than to an impulse signal some antenna constraints like
dispersivity were reduced. In order to illustrate that idea, a diamond dipole that is well
matched in 3.1-5.1GHz band was used. We observe that we have a good matching
between transmitted pulse and radiated field
Figure 3.8 Comparison between transmitted and radiated pulse.
Diamond antenna is a compact and planar one with a maximum gain of 2.7dBi on z-
axis. It is thus a good candidate for low data rate applications like tags.
18
amplitude modulated signal than to an impulse signal some antenna constraints like
dispersivity were reduced. In order to illustrate that idea, a diamond dipole that is well
matched in 3.1-5.1GHz band was used. We observe that we have a good matching
between transmitted pulse and radiated field
Figure 3.8 Comparison between transmitted and radiated pulse.
Diamond antenna is a compact and planar one with a maximum gain of 2.7dBi on z-
axis. It is thus a good candidate for low data rate applications like tags.
18

Chapter 4 Receiver Design Principles
4.1 Introduction
Signals once generated, transmitted, propagated are received to be understood and
decoded as relevant information. In that, efficient signal recovery and information
detection are primary to any successful communications receiver. This chapter provides
a comprehensive review of the UWB receiver architecture, listing the blocks, which
constitute it, namely: RF Front End, ADC clock and digital processing by the FPGA.
Digital Leading Edge Detection, being one of the many digital receiver topologies is
discussed mainly because of its low complexity, easy demodulation, faster retrieval and
the fact that no synchronisation is required. Binary PSK demodulation is highlighted in
the view of decoding the message signal sent at the transmitter end, focus was also
shifted to UWB demodulation mechanisms, optimally in the sense of reducing the
Probability Bit Error Rate.
4.2 Receiver Design Architecture
The UWB receiver design was in such a way that it had three major components as will
be discussed in each sub section. Figure 4.3 shows the overall block as depicted below.
The basic receiver guidelines used in this project was an impulse based UWB wideband
3.1GHz to 5.1 GHz with a centre frequency of 4.1 GHz.
Figure 4.1 UWB Receiver Design Architecture [9].
4.2.1 RF Front End
The RF Front End is composed of a series of broadband amplifiers, a bandlimiting filter,
a broadband variable gain amplifier/variable attenuator, and a power divider. The low-
noise amplifier (LNA) ensures both the longest possible range of the communication
link by minimizing the amount of noise and distortion added to the received signal.
19
4.1 Introduction
Signals once generated, transmitted, propagated are received to be understood and
decoded as relevant information. In that, efficient signal recovery and information
detection are primary to any successful communications receiver. This chapter provides
a comprehensive review of the UWB receiver architecture, listing the blocks, which
constitute it, namely: RF Front End, ADC clock and digital processing by the FPGA.
Digital Leading Edge Detection, being one of the many digital receiver topologies is
discussed mainly because of its low complexity, easy demodulation, faster retrieval and
the fact that no synchronisation is required. Binary PSK demodulation is highlighted in
the view of decoding the message signal sent at the transmitter end, focus was also
shifted to UWB demodulation mechanisms, optimally in the sense of reducing the
Probability Bit Error Rate.
4.2 Receiver Design Architecture
The UWB receiver design was in such a way that it had three major components as will
be discussed in each sub section. Figure 4.3 shows the overall block as depicted below.
The basic receiver guidelines used in this project was an impulse based UWB wideband
3.1GHz to 5.1 GHz with a centre frequency of 4.1 GHz.
Figure 4.1 UWB Receiver Design Architecture [9].
4.2.1 RF Front End
The RF Front End is composed of a series of broadband amplifiers, a bandlimiting filter,
a broadband variable gain amplifier/variable attenuator, and a power divider. The low-
noise amplifier (LNA) ensures both the longest possible range of the communication
link by minimizing the amount of noise and distortion added to the received signal.
19
Secure Best Marks with AI Grader
Need help grading? Try our AI Grader for instant feedback on your assignments.

The LNA amplifies small input signals, is made of a low noise figure (NF), high
transition frequency, with an amplification capability of 4 GHz, and provides at least a
60dB of total voltage gain. To limit the reflections, a 3 dB attenuator was placed as an
isolation device. The bandpass filter bandlimits the incoming signal and limits the input
noise. It has the following properties of a centre frequency of 4 GHz, 10dB and a
bandwidth greater than 500 MHz Furthermore, the variable gain amplifier/variable
attenuator allows the receiver to reduce the overall gain of the RF Front End to avoid
overdriving the ADCs. A square law detector, which is a tunnel diode, is selected due to
its sharp I-V slope, small capacitance and fast execution. Its function is usable in DC
signals from extremely small RF input. The low pass filter that was used was a 3 rd order
Butterworth design with a 600 MHz cut-off frequency whose work was to filter the
carrier frequency to retrieve the base band pulses. Once the base band pulses were
retrieved a baseband Op-Amp was used to provide gain for the pulses. The Op-Amp
properties included a non-inverting type, high slew rate and operating with a single
supply voltage (+ 5 DC).
4.2.2 ADC/ Clock Distribution
The RF Front block is followed by a limiter, the Receiver design is a Colonics Company
design, in which the limiter is replaced by a MAX 108 by Maxim. The limiter acts as a
power divider that sends the signal through a series of delay lines to the ADC bank. In
addition, it also has an ADC integrated within this chip. These delay lines will likely be
made out of hard-line coaxial cable, the same form of signal transmission used by many
digital oscilloscopes. They will be cut to the specific length of the time delay needed
based on their propagation delay properties. To perform the parallel sampling, the delay
lines present each ADC with a slightly delayed version of the input waveform. Delaying
each input signal with respect to the previous one in the chain is essentially identical to
successively delaying each ADC’s clock signal, but removes the hassle of precision
clock distribution and routing. The bank of ADCs and clock distribution network which
will be responsible for parallel sampling the received UWB pulse. The Maxim 108 ADC
was found to offer an input bandwidth of 2.2 GHz, a maximum sampling rate of 1 GHz
with 8-bit quantization, an aperture delay of 100 ps with part-to-part variation of less
than 30%. With 8 of the Maxim 104 ADCs operating in parallel at an individual
sampling rate of 1 GHz, each successive ADC’s samples the signal at 125 picoseconds
increments, yielding an effective sampling rate of 8 GHz.
20
transition frequency, with an amplification capability of 4 GHz, and provides at least a
60dB of total voltage gain. To limit the reflections, a 3 dB attenuator was placed as an
isolation device. The bandpass filter bandlimits the incoming signal and limits the input
noise. It has the following properties of a centre frequency of 4 GHz, 10dB and a
bandwidth greater than 500 MHz Furthermore, the variable gain amplifier/variable
attenuator allows the receiver to reduce the overall gain of the RF Front End to avoid
overdriving the ADCs. A square law detector, which is a tunnel diode, is selected due to
its sharp I-V slope, small capacitance and fast execution. Its function is usable in DC
signals from extremely small RF input. The low pass filter that was used was a 3 rd order
Butterworth design with a 600 MHz cut-off frequency whose work was to filter the
carrier frequency to retrieve the base band pulses. Once the base band pulses were
retrieved a baseband Op-Amp was used to provide gain for the pulses. The Op-Amp
properties included a non-inverting type, high slew rate and operating with a single
supply voltage (+ 5 DC).
4.2.2 ADC/ Clock Distribution
The RF Front block is followed by a limiter, the Receiver design is a Colonics Company
design, in which the limiter is replaced by a MAX 108 by Maxim. The limiter acts as a
power divider that sends the signal through a series of delay lines to the ADC bank. In
addition, it also has an ADC integrated within this chip. These delay lines will likely be
made out of hard-line coaxial cable, the same form of signal transmission used by many
digital oscilloscopes. They will be cut to the specific length of the time delay needed
based on their propagation delay properties. To perform the parallel sampling, the delay
lines present each ADC with a slightly delayed version of the input waveform. Delaying
each input signal with respect to the previous one in the chain is essentially identical to
successively delaying each ADC’s clock signal, but removes the hassle of precision
clock distribution and routing. The bank of ADCs and clock distribution network which
will be responsible for parallel sampling the received UWB pulse. The Maxim 108 ADC
was found to offer an input bandwidth of 2.2 GHz, a maximum sampling rate of 1 GHz
with 8-bit quantization, an aperture delay of 100 ps with part-to-part variation of less
than 30%. With 8 of the Maxim 104 ADCs operating in parallel at an individual
sampling rate of 1 GHz, each successive ADC’s samples the signal at 125 picoseconds
increments, yielding an effective sampling rate of 8 GHz.
20

Another benefit of the Maxim 104 is a demultiplexer feature that allows for two output
data paths at half of the sampling rate. This will allow digital data to be processed at a
clock frequency of 500 MHz instead of 1 GHz, relaxing the input timing requirements
of the FPGA. The configuration of the ADC/ Clock distribution is depicted in the figure
below.
Figure 4.2 Block Diagram of the Parallel Time-Domain Sampling Technique
The performance of the Parallel Time-Domain Sampling technique is highly dependent
on sampling the received signal at precisely spaced intervals. Thus, an ultra-low skew
clock distribution network is required so that to be precise to ensure that each ADC is
clocked at exactly the right instant in time.
4.2.3 Digital Processing
The digital processing part of UWB receiver proves to be a vital functional block in the
receiver architecture; this is so because it must be capable of handling multiple data
streams from the ADCs and then demodulating the data in real time. In order to meet the
design objectives, the digital processing must also be reconfigurable, in order to provide
the flexibility demanded by a software radio design.
21
data paths at half of the sampling rate. This will allow digital data to be processed at a
clock frequency of 500 MHz instead of 1 GHz, relaxing the input timing requirements
of the FPGA. The configuration of the ADC/ Clock distribution is depicted in the figure
below.
Figure 4.2 Block Diagram of the Parallel Time-Domain Sampling Technique
The performance of the Parallel Time-Domain Sampling technique is highly dependent
on sampling the received signal at precisely spaced intervals. Thus, an ultra-low skew
clock distribution network is required so that to be precise to ensure that each ADC is
clocked at exactly the right instant in time.
4.2.3 Digital Processing
The digital processing part of UWB receiver proves to be a vital functional block in the
receiver architecture; this is so because it must be capable of handling multiple data
streams from the ADCs and then demodulating the data in real time. In order to meet the
design objectives, the digital processing must also be reconfigurable, in order to provide
the flexibility demanded by a software radio design.
21

A powerful FPGA is the optimal choice for the digital processing block on the receiver.
Current top-of-the-line FPGAs are capable of handling multiple high data rate input
streams, have several embedded processor cores for any DSP operations which may be
required, and may be easily reconfigured for a variety of different modulation/
demodulation schemes. Practical FPGAs are limited by the rate at which they can input
data; and the maximum achievable data rate of the wireless link is limited by the time
required to process and demodulate each received pulse. FPGAs capable of inputting
data at the rates used in the design presented in this project, as well as demodulating the
proposed data rate of 100 Mbps were used. As discussed above, a software-defined
radio implementation of the receiver architecture provides a tremendous amount of
flexibility over hardware-based implementations. For the UWB receiver design
presented in this project, data demodulation is performed by reconfigurable logic—in
the form of an FPGA—in the digital domain. This approach allows the user to choose
the modulation scheme in this case a binary PSK as well the Digital Leading Edge
Detection receiver topologies. FPGAs are crucial, in that, the project is not tied down to
a particular modulation and receiver topologies as additional receiver topologies and/or
Rake combining algorithms can easily be generated and downloaded into the FPGA—
without making a single hardware change. Thus, future work can utilise the ability to
match schemes and topologies based on application requirements or research interests.
The FPGA must be able to handle the 32 ADC outputs (16 differential pairs from each
ADC using the demultiplexer feature), at an individual data rate of 500 MHz and 8
bits/sample—an aggregate data rate of 64 Gbps. Furthermore, assuming a target raw
data rate of 100 Mbps (using a pulse rate of 100 MHz), for real-time data transfer, the
FPGA must be capable of demodulating data in under 10 ns.
4.3 Digital Leading Edge Detection Receiver Topology
Threshold detectors, also known as leading edge detection (LED) receivers, were some
of the earliest and probably the simplest of all I-UWB receivers. The LED receiver sets
a threshold at the receiver, and any incoming pulse that crosses the threshold is detected
and demodulated. The problem with the threshold reception technique is that noise
spikes, which happen to cross the threshold, will also be erroneously detected as a data
pulse (known as a "false alarm" or "false detection"). To mitigate the problem of false
detections, the receiver must continuously monitor the input noise signal and adaptively
set a threshold such that only a small percentage of false detections will occur, similar
to the constant false alarm rate (CFAR) used in radar.
22
Current top-of-the-line FPGAs are capable of handling multiple high data rate input
streams, have several embedded processor cores for any DSP operations which may be
required, and may be easily reconfigured for a variety of different modulation/
demodulation schemes. Practical FPGAs are limited by the rate at which they can input
data; and the maximum achievable data rate of the wireless link is limited by the time
required to process and demodulate each received pulse. FPGAs capable of inputting
data at the rates used in the design presented in this project, as well as demodulating the
proposed data rate of 100 Mbps were used. As discussed above, a software-defined
radio implementation of the receiver architecture provides a tremendous amount of
flexibility over hardware-based implementations. For the UWB receiver design
presented in this project, data demodulation is performed by reconfigurable logic—in
the form of an FPGA—in the digital domain. This approach allows the user to choose
the modulation scheme in this case a binary PSK as well the Digital Leading Edge
Detection receiver topologies. FPGAs are crucial, in that, the project is not tied down to
a particular modulation and receiver topologies as additional receiver topologies and/or
Rake combining algorithms can easily be generated and downloaded into the FPGA—
without making a single hardware change. Thus, future work can utilise the ability to
match schemes and topologies based on application requirements or research interests.
The FPGA must be able to handle the 32 ADC outputs (16 differential pairs from each
ADC using the demultiplexer feature), at an individual data rate of 500 MHz and 8
bits/sample—an aggregate data rate of 64 Gbps. Furthermore, assuming a target raw
data rate of 100 Mbps (using a pulse rate of 100 MHz), for real-time data transfer, the
FPGA must be capable of demodulating data in under 10 ns.
4.3 Digital Leading Edge Detection Receiver Topology
Threshold detectors, also known as leading edge detection (LED) receivers, were some
of the earliest and probably the simplest of all I-UWB receivers. The LED receiver sets
a threshold at the receiver, and any incoming pulse that crosses the threshold is detected
and demodulated. The problem with the threshold reception technique is that noise
spikes, which happen to cross the threshold, will also be erroneously detected as a data
pulse (known as a "false alarm" or "false detection"). To mitigate the problem of false
detections, the receiver must continuously monitor the input noise signal and adaptively
set a threshold such that only a small percentage of false detections will occur, similar
to the constant false alarm rate (CFAR) used in radar.
22
Paraphrase This Document
Need a fresh take? Get an instant paraphrase of this document with our AI Paraphraser

To operate properly, the LED receiver must use a device that is capable of responding to
a very sharp change in received voltage in a very short time span. An analysis of
threshold receiver performance is given by Harmuth [21]. Estimates of the probability
of detection, PD, and the probability of false alarm, P fa, are necessary in order to
estimate threshold receiver performance. The noise and the signal-pulse- noise
distributions are assumed Gaussian. The zero mean noise distribution is given by
(11)
where
(12)
and
(13)
Because the received signal is the sum of the noise voltage and the signal voltage, the
normalized signal plus noise voltage is given by
(14)
Thus, the sum of the noise voltage and the signal voltage is also Gaussian distributed
whose mean value is shifted by the signal. This results in a pdf for the signal, plus the
superimposed noise given by
(15)
The normalized probability density functions for W N (x) and W N+S (x) are plotted in
Figure 6.10. Suppose the threshold is given by F times σ G or F σ G. Any value of the
noise pdf that exceeds the threshold is a false alarm, and any value of the signal plus
noise pdf below the threshold is an error. By integrating the pdfs over the proper
regions, the probability of detection, PD, and probability of false alarm, P fa, can be
computed as given by the following equations
(16)
23
a very sharp change in received voltage in a very short time span. An analysis of
threshold receiver performance is given by Harmuth [21]. Estimates of the probability
of detection, PD, and the probability of false alarm, P fa, are necessary in order to
estimate threshold receiver performance. The noise and the signal-pulse- noise
distributions are assumed Gaussian. The zero mean noise distribution is given by
(11)
where
(12)
and
(13)
Because the received signal is the sum of the noise voltage and the signal voltage, the
normalized signal plus noise voltage is given by
(14)
Thus, the sum of the noise voltage and the signal voltage is also Gaussian distributed
whose mean value is shifted by the signal. This results in a pdf for the signal, plus the
superimposed noise given by
(15)
The normalized probability density functions for W N (x) and W N+S (x) are plotted in
Figure 6.10. Suppose the threshold is given by F times σ G or F σ G. Any value of the
noise pdf that exceeds the threshold is a false alarm, and any value of the signal plus
noise pdf below the threshold is an error. By integrating the pdfs over the proper
regions, the probability of detection, PD, and probability of false alarm, P fa, can be
computed as given by the following equations
(16)
23

(17)
Figure 4.3 Threshold Detection in Gaussian Noise [5].
A 15 dB signal-to-noise ratio (SNR) is required for a 0.99 probability of detection with
a 10-8false alarm rate. Increasing the threshold decreases the false alarm rate but
decreases the probability of detection for a given signal-to-noise ratio.
4.4 Data Demodulation
Data demodulation using the D-LED method is performed by using the start of the data
index and comparing it to threshold value. This value is based on the received pulse
amplitude and the standard deviation of the noise. The data index will be incremented
based on the number of samples per pulse period and the expected number of samples
between a “1” and a “0” if using binary PSK. Bit decisions are made based on the case
of binary PSK, two sample values for each pulse are taken and they are compared to
each other. The higher sampled value will be chosen as the correct bit.
24
Figure 4.3 Threshold Detection in Gaussian Noise [5].
A 15 dB signal-to-noise ratio (SNR) is required for a 0.99 probability of detection with
a 10-8false alarm rate. Increasing the threshold decreases the false alarm rate but
decreases the probability of detection for a given signal-to-noise ratio.
4.4 Data Demodulation
Data demodulation using the D-LED method is performed by using the start of the data
index and comparing it to threshold value. This value is based on the received pulse
amplitude and the standard deviation of the noise. The data index will be incremented
based on the number of samples per pulse period and the expected number of samples
between a “1” and a “0” if using binary PSK. Bit decisions are made based on the case
of binary PSK, two sample values for each pulse are taken and they are compared to
each other. The higher sampled value will be chosen as the correct bit.
24
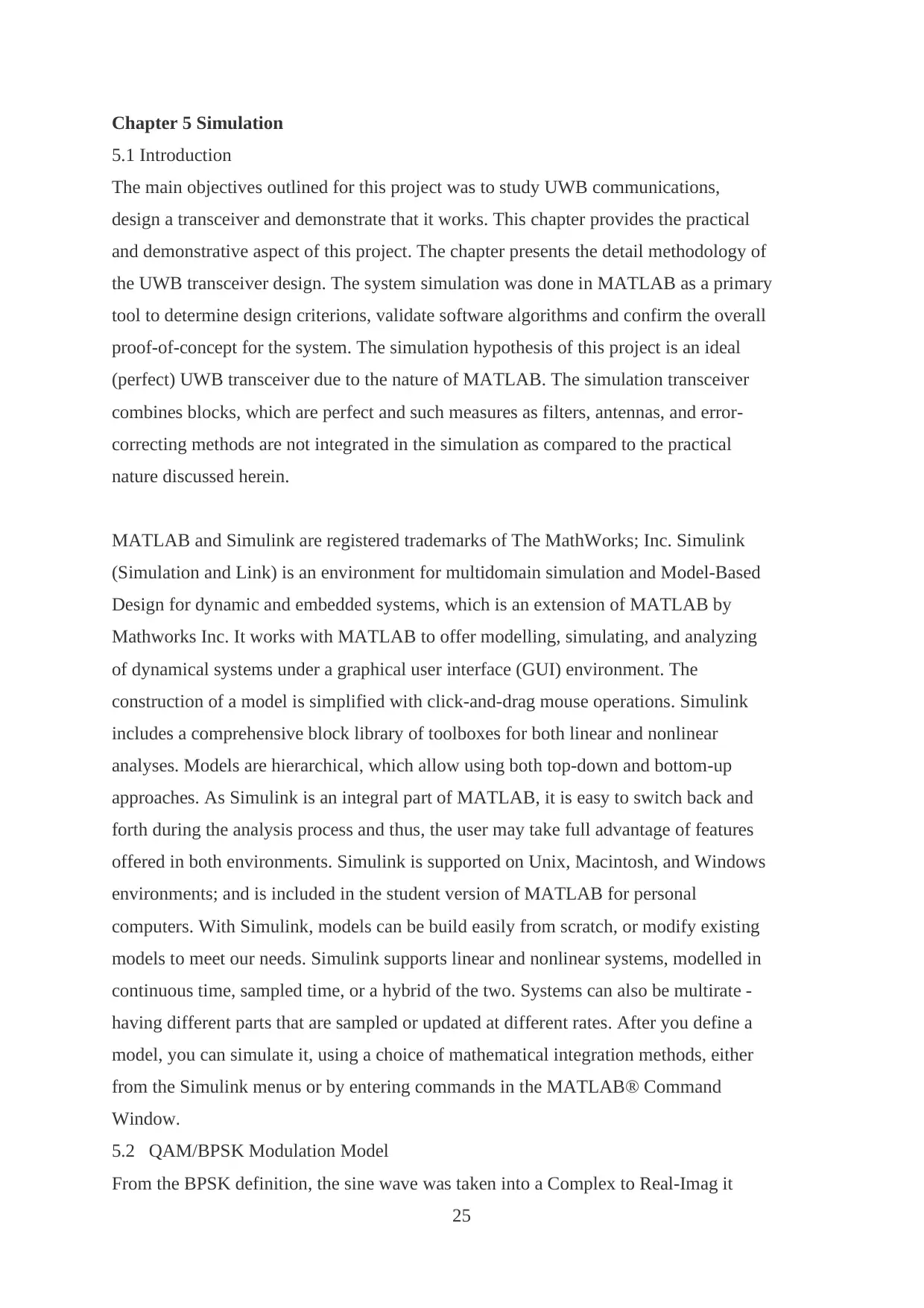
Chapter 5 Simulation
5.1 Introduction
The main objectives outlined for this project was to study UWB communications,
design a transceiver and demonstrate that it works. This chapter provides the practical
and demonstrative aspect of this project. The chapter presents the detail methodology of
the UWB transceiver design. The system simulation was done in MATLAB as a primary
tool to determine design criterions, validate software algorithms and confirm the overall
proof-of-concept for the system. The simulation hypothesis of this project is an ideal
(perfect) UWB transceiver due to the nature of MATLAB. The simulation transceiver
combines blocks, which are perfect and such measures as filters, antennas, and error-
correcting methods are not integrated in the simulation as compared to the practical
nature discussed herein.
MATLAB and Simulink are registered trademarks of The MathWorks; Inc. Simulink
(Simulation and Link) is an environment for multidomain simulation and Model-Based
Design for dynamic and embedded systems, which is an extension of MATLAB by
Mathworks Inc. It works with MATLAB to offer modelling, simulating, and analyzing
of dynamical systems under a graphical user interface (GUI) environment. The
construction of a model is simplified with click-and-drag mouse operations. Simulink
includes a comprehensive block library of toolboxes for both linear and nonlinear
analyses. Models are hierarchical, which allow using both top-down and bottom-up
approaches. As Simulink is an integral part of MATLAB, it is easy to switch back and
forth during the analysis process and thus, the user may take full advantage of features
offered in both environments. Simulink is supported on Unix, Macintosh, and Windows
environments; and is included in the student version of MATLAB for personal
computers. With Simulink, models can be build easily from scratch, or modify existing
models to meet our needs. Simulink supports linear and nonlinear systems, modelled in
continuous time, sampled time, or a hybrid of the two. Systems can also be multirate -
having different parts that are sampled or updated at different rates. After you define a
model, you can simulate it, using a choice of mathematical integration methods, either
from the Simulink menus or by entering commands in the MATLAB® Command
Window.
5.2 QAM/BPSK Modulation Model
From the BPSK definition, the sine wave was taken into a Complex to Real-Imag it
25
5.1 Introduction
The main objectives outlined for this project was to study UWB communications,
design a transceiver and demonstrate that it works. This chapter provides the practical
and demonstrative aspect of this project. The chapter presents the detail methodology of
the UWB transceiver design. The system simulation was done in MATLAB as a primary
tool to determine design criterions, validate software algorithms and confirm the overall
proof-of-concept for the system. The simulation hypothesis of this project is an ideal
(perfect) UWB transceiver due to the nature of MATLAB. The simulation transceiver
combines blocks, which are perfect and such measures as filters, antennas, and error-
correcting methods are not integrated in the simulation as compared to the practical
nature discussed herein.
MATLAB and Simulink are registered trademarks of The MathWorks; Inc. Simulink
(Simulation and Link) is an environment for multidomain simulation and Model-Based
Design for dynamic and embedded systems, which is an extension of MATLAB by
Mathworks Inc. It works with MATLAB to offer modelling, simulating, and analyzing
of dynamical systems under a graphical user interface (GUI) environment. The
construction of a model is simplified with click-and-drag mouse operations. Simulink
includes a comprehensive block library of toolboxes for both linear and nonlinear
analyses. Models are hierarchical, which allow using both top-down and bottom-up
approaches. As Simulink is an integral part of MATLAB, it is easy to switch back and
forth during the analysis process and thus, the user may take full advantage of features
offered in both environments. Simulink is supported on Unix, Macintosh, and Windows
environments; and is included in the student version of MATLAB for personal
computers. With Simulink, models can be build easily from scratch, or modify existing
models to meet our needs. Simulink supports linear and nonlinear systems, modelled in
continuous time, sampled time, or a hybrid of the two. Systems can also be multirate -
having different parts that are sampled or updated at different rates. After you define a
model, you can simulate it, using a choice of mathematical integration methods, either
from the Simulink menus or by entering commands in the MATLAB® Command
Window.
5.2 QAM/BPSK Modulation Model
From the BPSK definition, the sine wave was taken into a Complex to Real-Imag it
25
Secure Best Marks with AI Grader
Need help grading? Try our AI Grader for instant feedback on your assignments.

outputs the real and/or imaginary part of the input signal, depending on the setting of
the parameters. The real outputs are of the same data type as the complex input. The
real array contains the real parts of the corresponding complex sine input elements. The
imaginary output similarly contains the imaginary parts of the input elements. The
product waveforms were then added to the show the modulated waveform. The sine
wave became a cosine wave by an input of pi/2 in the phase parameter field; otherwise,
all the other blocks used in their default settings as in the figure.
Figure 5.1 QAM/BPSK Modulation Model
The QAM/BPSK model was simulated and the corresponding display in the scope as
shown in the figure below.
Figure 5.2 QAM/BPSK Modulation Scope Display
5.3 UWB Transmitter
The following SIMULINK model was used for simulating the UWB transmitter as
shown in the figure below. Each of the corresponding blocks used is discussed in detail
in terms of parameter settings and block functionalities.
26
the parameters. The real outputs are of the same data type as the complex input. The
real array contains the real parts of the corresponding complex sine input elements. The
imaginary output similarly contains the imaginary parts of the input elements. The
product waveforms were then added to the show the modulated waveform. The sine
wave became a cosine wave by an input of pi/2 in the phase parameter field; otherwise,
all the other blocks used in their default settings as in the figure.
Figure 5.1 QAM/BPSK Modulation Model
The QAM/BPSK model was simulated and the corresponding display in the scope as
shown in the figure below.
Figure 5.2 QAM/BPSK Modulation Scope Display
5.3 UWB Transmitter
The following SIMULINK model was used for simulating the UWB transmitter as
shown in the figure below. Each of the corresponding blocks used is discussed in detail
in terms of parameter settings and block functionalities.
26

Figure 5.3 UWB Transmitter Design block in MATLAB
5.3.1 Bernoulli Binary Generator
The Bernoulli Binary Generator block generates random binary numbers using a
Bernoulli distribution. The Bernoulli distribution with parameter p produces zero with
probability p and one with probability 1-p. The Bernoulli distribution has mean value 1-
p and variance p (1-p). The Probability of a zero parameter specifies p, and can be any
real number between zero and one [9]. This block was used to generate the binary data
by adjusting the output attributes of the sample time field to 1.0ns. This is in accordance
with UWB criterion that the pulses should be of short duration in the order of
nanoseconds, in addition to fulfilling of the project’s objective of 100MHz bandwidth.
5.3.2 Buffer
The Buffer block redistributes the input samples to a new frame size. Buffering to a
larger frame size yields an output with a slower frame rate than the input. The block
coordinates the output frame size and frame rate of non-overlapping buffers such that
the sample period of the signal is the same at both the input and output. This block
supports triggered subsystems when the block's input and output rates are the same [9].
The buffer’s functionality is to create an area or limit within which the UWB signal will
work within and this set as 96 in the output buffer size (per channel) field in the
parameter settings. It acts as a guard to restrict the UWB signal into the desired
frequency band.
5.3.3 BPSK Modulator Baseband
The BPSK Modulator Baseband block modulates using binary phase shift keying
method. The output is a baseband representation of the modulated signal. The input
must be a discrete-time binary-valued signal. If the input bit is 0 or 1, respectively, then
the modulated symbol is exp (jθ) or –exp (jθ) respectively, where θ is the Phase offset
parameter [9]. The block is used in its default parameter settings.
27
5.3.1 Bernoulli Binary Generator
The Bernoulli Binary Generator block generates random binary numbers using a
Bernoulli distribution. The Bernoulli distribution with parameter p produces zero with
probability p and one with probability 1-p. The Bernoulli distribution has mean value 1-
p and variance p (1-p). The Probability of a zero parameter specifies p, and can be any
real number between zero and one [9]. This block was used to generate the binary data
by adjusting the output attributes of the sample time field to 1.0ns. This is in accordance
with UWB criterion that the pulses should be of short duration in the order of
nanoseconds, in addition to fulfilling of the project’s objective of 100MHz bandwidth.
5.3.2 Buffer
The Buffer block redistributes the input samples to a new frame size. Buffering to a
larger frame size yields an output with a slower frame rate than the input. The block
coordinates the output frame size and frame rate of non-overlapping buffers such that
the sample period of the signal is the same at both the input and output. This block
supports triggered subsystems when the block's input and output rates are the same [9].
The buffer’s functionality is to create an area or limit within which the UWB signal will
work within and this set as 96 in the output buffer size (per channel) field in the
parameter settings. It acts as a guard to restrict the UWB signal into the desired
frequency band.
5.3.3 BPSK Modulator Baseband
The BPSK Modulator Baseband block modulates using binary phase shift keying
method. The output is a baseband representation of the modulated signal. The input
must be a discrete-time binary-valued signal. If the input bit is 0 or 1, respectively, then
the modulated symbol is exp (jθ) or –exp (jθ) respectively, where θ is the Phase offset
parameter [9]. The block is used in its default parameter settings.
27

The reason as to why this block is used is that UWB radio, unlike classic
communications, does not use a modulated sinusoidal carrier to convey information.
Instead, the transmitted signal is a series of baseband pulses generated by the BPSK
block.
5.3.4 Discrete Time Scatter Plot Scope
The Discrete-Time Scatter Plot Scope block displays scatter plots of a modulated
signal, to reveal the modulation characteristics, such as pulse shaping or channel
distortions of the signal. The Discrete-Time Scatter Plot Scope block has one input port.
The input signal must be complex. The block accepts signal of type double, single, base
integer, and fixed-point for input, but will cast it as double. The input signal must be a
sample-based scalar in sample-based mode. The input must be a frame-based column
vector or a scalar in frame-based mode [9]. This block was used to display the
transmitted UWB signal in Gray code. It was relevant to use this scope, since it can
display the baseband pulses. The parameter settings of this scope were set at -1.5 and
1.5 on the x-axis and y-axis respectively.
5.3.5 Pad Block
The Pad block extends or crops the dimensions of the input by padding or truncating
along its columns, rows, columns and rows, or any dimension(s) you specify. Truncation
occurs when you specify output dimensions that are shorter than the corresponding
input dimensions. If the input and output lengths are the same, the block is a pass-
through [9]. The pad block is used to append the signal for proper inverse fast fourier
transformation. This is so because the IFFT block cannot perform the inversion without
appending or truncation. The parameters were modified as pad signal field was placed at
the beginning and the padding being done along the columns with specified output rows
at a value of one.
5.3.6 IFFT Block
The IFFT block computes the inverse fast Fourier transform (IFFT) of each channel of a
P-by-N or length-P input, u. When the Inherit FFT length from input dimensions check
box is selected, the input length P must be an integer power of two and the FFT length
M is equal to P. When the check box is not selected, P can be any length, and the value
of the FFT length parameter must be a positive integer power of two. For user-specified
FFT lengths, when M is not equal to P, zero padding or modulo-M data wrapping
happens before the IFFT operation [9].
28
communications, does not use a modulated sinusoidal carrier to convey information.
Instead, the transmitted signal is a series of baseband pulses generated by the BPSK
block.
5.3.4 Discrete Time Scatter Plot Scope
The Discrete-Time Scatter Plot Scope block displays scatter plots of a modulated
signal, to reveal the modulation characteristics, such as pulse shaping or channel
distortions of the signal. The Discrete-Time Scatter Plot Scope block has one input port.
The input signal must be complex. The block accepts signal of type double, single, base
integer, and fixed-point for input, but will cast it as double. The input signal must be a
sample-based scalar in sample-based mode. The input must be a frame-based column
vector or a scalar in frame-based mode [9]. This block was used to display the
transmitted UWB signal in Gray code. It was relevant to use this scope, since it can
display the baseband pulses. The parameter settings of this scope were set at -1.5 and
1.5 on the x-axis and y-axis respectively.
5.3.5 Pad Block
The Pad block extends or crops the dimensions of the input by padding or truncating
along its columns, rows, columns and rows, or any dimension(s) you specify. Truncation
occurs when you specify output dimensions that are shorter than the corresponding
input dimensions. If the input and output lengths are the same, the block is a pass-
through [9]. The pad block is used to append the signal for proper inverse fast fourier
transformation. This is so because the IFFT block cannot perform the inversion without
appending or truncation. The parameters were modified as pad signal field was placed at
the beginning and the padding being done along the columns with specified output rows
at a value of one.
5.3.6 IFFT Block
The IFFT block computes the inverse fast Fourier transform (IFFT) of each channel of a
P-by-N or length-P input, u. When the Inherit FFT length from input dimensions check
box is selected, the input length P must be an integer power of two and the FFT length
M is equal to P. When the check box is not selected, P can be any length, and the value
of the FFT length parameter must be a positive integer power of two. For user-specified
FFT lengths, when M is not equal to P, zero padding or modulo-M data wrapping
happens before the IFFT operation [9].
28
Paraphrase This Document
Need a fresh take? Get an instant paraphrase of this document with our AI Paraphraser

IFFT is a form of digital signal processing (dsp) and is relevant to allow for easy
transmission of the generated signals across the channel to the receiver end of the
transceiver.
5.3.7 Unbuffer block
The Unbuffer block unbuffers an Mi-by-N frame-based input into a 1-by-N sample-
based output. That is, inputs are unbuffered row-wise so that each matrix row becomes
an independent time-sample in the output. The rate at which the block receives inputs is
generally less than the rate at which the block produces outputs [9]. The Unbuffer
block’s seeks to integrate the IFFT output from the frame based aspect to the waveform
(sample based) display on the spectrum scope.
5.3.8 Spectrum Scope
The Spectrum Scope block computes and displays the periodogram of the input. The
input can be a sample-based or frame-based vector or a frame-based matrix. The
spectrum scope is used to display the transmitted UWB signal as desired in figure 5.4.
Figure 5.4 Transmitted UWB Waveform in MATLAB
5.4 UWB Channel
A channel is a physical or logical path for a signal transmission and forms the
relationship between the received signal and the transmitted signal. In the UWB system
model, we designed the received signal as a sum of the transmitted signal and additive
white Gaussian noise.
29
transmission of the generated signals across the channel to the receiver end of the
transceiver.
5.3.7 Unbuffer block
The Unbuffer block unbuffers an Mi-by-N frame-based input into a 1-by-N sample-
based output. That is, inputs are unbuffered row-wise so that each matrix row becomes
an independent time-sample in the output. The rate at which the block receives inputs is
generally less than the rate at which the block produces outputs [9]. The Unbuffer
block’s seeks to integrate the IFFT output from the frame based aspect to the waveform
(sample based) display on the spectrum scope.
5.3.8 Spectrum Scope
The Spectrum Scope block computes and displays the periodogram of the input. The
input can be a sample-based or frame-based vector or a frame-based matrix. The
spectrum scope is used to display the transmitted UWB signal as desired in figure 5.4.
Figure 5.4 Transmitted UWB Waveform in MATLAB
5.4 UWB Channel
A channel is a physical or logical path for a signal transmission and forms the
relationship between the received signal and the transmitted signal. In the UWB system
model, we designed the received signal as a sum of the transmitted signal and additive
white Gaussian noise.
29

The AWGN Channel block adds white Gaussian noise to a real or complex input signal.
When the input signal is real, this block adds real Gaussian noise and produces a real
output signal. When the input signal is complex, this block adds complex Gaussian
noise and produces a complex output signal. This block inherits its sample time from
the input signal [9]. With the AWGN channel being available in MATLAB, thus,
simulation to model the UWB channel effects of noise and propagation. Although this
forms an ideal situation for channel modelling, in that, a particular signal-to-noise ratio
is chosen which is different in practical areas. Overall, choosing an AWGN channel to
experiment and simulate the behaviour of UWB signal as in figure. The AWGN model is
set up to allow variations in the SNR, which was the parameters within the model were
set up with the SNR equal to 30, number of bits per symbol equal to 2, input signal
power equal to one watt, and symbol period equal to 2 nanoseconds in Figure 5.5
Figure 5.5 UWB Channel with AGWN
5.5 UWB Receiver
The following SIMULINK model as shown in figure was used to simulate the UWB
receiver in accordance with the project objectives. The receiver is similar in design to
the transmitter model but in this case, each of the blocks performing the reverse
operations as compared to the transmitter block.
Figure 5.6 UWB Receiver design Block in MATLAB
30
When the input signal is real, this block adds real Gaussian noise and produces a real
output signal. When the input signal is complex, this block adds complex Gaussian
noise and produces a complex output signal. This block inherits its sample time from
the input signal [9]. With the AWGN channel being available in MATLAB, thus,
simulation to model the UWB channel effects of noise and propagation. Although this
forms an ideal situation for channel modelling, in that, a particular signal-to-noise ratio
is chosen which is different in practical areas. Overall, choosing an AWGN channel to
experiment and simulate the behaviour of UWB signal as in figure. The AWGN model is
set up to allow variations in the SNR, which was the parameters within the model were
set up with the SNR equal to 30, number of bits per symbol equal to 2, input signal
power equal to one watt, and symbol period equal to 2 nanoseconds in Figure 5.5
Figure 5.5 UWB Channel with AGWN
5.5 UWB Receiver
The following SIMULINK model as shown in figure was used to simulate the UWB
receiver in accordance with the project objectives. The receiver is similar in design to
the transmitter model but in this case, each of the blocks performing the reverse
operations as compared to the transmitter block.
Figure 5.6 UWB Receiver design Block in MATLAB
30

5.5.1 FFT Block
The FFT block computes the fast Fourier transform (FFT) of each channel of a P-by-N
or length-P input, u. When the Inherit FFT length from input dimensions check box is
selected, the input length P must be an integer power of two and the FFT length M is
equal to P. When the check box is not selected, P can be any length, and the value of the
FFT length parameter must be a positive integer power of two. For user-specified FFT
lengths, when M is not equal to P, zero padding or modulo-M data wrapping happens
before the FFT operation [9]. The parameter settings were set at table lookup in the
twiddle factor computation field and speed in the optimise table field. Its functionality
is to get the fast fourier transform of the received signal for proper demodulation of the
signal.
5.5.2 Selector
The Selector block generates as output selected or reordered elements of an input
vector, matrix, or multidimensional signal. A Selector block accepts vector, matrix, or
multidimensional signals as input. The parameter dialog box and the block's appearance
change to reflect the number of dimensions of the input [9]. The Input type parameter
was set to the type of signal “Matrix”. The parameter dialog box and the block's
appearance changed to reflect the type of input that was selected.
5.5.3 To Frame Conversion
The Frame Conversion block passes the input through to the output and sets the output-
sampling mode to the value of the Sampling mode of output signal parameter, which can
be either Frame-based or Sample-based. The output sampling mode can also be
inherited from the signal at the Ref (reference) input port, which you make visible by
selecting the Inherit output sampling mode from <Ref> input port check box. The
Frame Conversion block does not make any changes to the input signal other than the
sampling mode. In particular, the block does not rebuffer or resize 2-D inputs. Because
1-D vectors cannot be frame based, when the input is a length-M 1-D vector and the
block is in Frame-based mode, the output is a frame-based M-by-1 matrix — that is, a
single channel [9]. The block was used in the default settings with no changes being
affected.
5.5.4 BPSK Demodulator
The BPSK Demodulator Baseband block demodulates a signal that was modulated using
the binary phase shift keying method. The input is a baseband representation of the
modulated signal. The input can be either a scalar or a frame-based column vector.
31
The FFT block computes the fast Fourier transform (FFT) of each channel of a P-by-N
or length-P input, u. When the Inherit FFT length from input dimensions check box is
selected, the input length P must be an integer power of two and the FFT length M is
equal to P. When the check box is not selected, P can be any length, and the value of the
FFT length parameter must be a positive integer power of two. For user-specified FFT
lengths, when M is not equal to P, zero padding or modulo-M data wrapping happens
before the FFT operation [9]. The parameter settings were set at table lookup in the
twiddle factor computation field and speed in the optimise table field. Its functionality
is to get the fast fourier transform of the received signal for proper demodulation of the
signal.
5.5.2 Selector
The Selector block generates as output selected or reordered elements of an input
vector, matrix, or multidimensional signal. A Selector block accepts vector, matrix, or
multidimensional signals as input. The parameter dialog box and the block's appearance
change to reflect the number of dimensions of the input [9]. The Input type parameter
was set to the type of signal “Matrix”. The parameter dialog box and the block's
appearance changed to reflect the type of input that was selected.
5.5.3 To Frame Conversion
The Frame Conversion block passes the input through to the output and sets the output-
sampling mode to the value of the Sampling mode of output signal parameter, which can
be either Frame-based or Sample-based. The output sampling mode can also be
inherited from the signal at the Ref (reference) input port, which you make visible by
selecting the Inherit output sampling mode from <Ref> input port check box. The
Frame Conversion block does not make any changes to the input signal other than the
sampling mode. In particular, the block does not rebuffer or resize 2-D inputs. Because
1-D vectors cannot be frame based, when the input is a length-M 1-D vector and the
block is in Frame-based mode, the output is a frame-based M-by-1 matrix — that is, a
single channel [9]. The block was used in the default settings with no changes being
affected.
5.5.4 BPSK Demodulator
The BPSK Demodulator Baseband block demodulates a signal that was modulated using
the binary phase shift keying method. The input is a baseband representation of the
modulated signal. The input can be either a scalar or a frame-based column vector.
31
Secure Best Marks with AI Grader
Need help grading? Try our AI Grader for instant feedback on your assignments.

The block accepts the data types double, single, and signed fixed-point. The input must
be a discrete-time complex signal. The block maps the point’s exp (jθ) and -exp (jθ) to 0
and 1, respectively, where θ is the Phase offset parameter [9]. The UWB signal
demodulation was as required and displayed on the discrete scatter plot. The BPSK
Demodulator parameter output type was set at bit and the constellation ordering was set
to gray code. The demodulated signal is as in figure 5.7.
Figure 5.7 Received UWB waveform plus AGWN in MATLAB
5.6 Discussion and Analysis
The transceiver design is as implemented herein. It is from the simulation results of the
spectrum scope at the transmitter and receiver side that they showed the actual
functioning of the transceiver. The waveform from the transmitter is the one actually
received at the receiver side plus an AWGN, as theory dictated. The simulated and
theoretical discussions agree, thus the project being a success.
32
be a discrete-time complex signal. The block maps the point’s exp (jθ) and -exp (jθ) to 0
and 1, respectively, where θ is the Phase offset parameter [9]. The UWB signal
demodulation was as required and displayed on the discrete scatter plot. The BPSK
Demodulator parameter output type was set at bit and the constellation ordering was set
to gray code. The demodulated signal is as in figure 5.7.
Figure 5.7 Received UWB waveform plus AGWN in MATLAB
5.6 Discussion and Analysis
The transceiver design is as implemented herein. It is from the simulation results of the
spectrum scope at the transmitter and receiver side that they showed the actual
functioning of the transceiver. The waveform from the transmitter is the one actually
received at the receiver side plus an AWGN, as theory dictated. The simulated and
theoretical discussions agree, thus the project being a success.
32
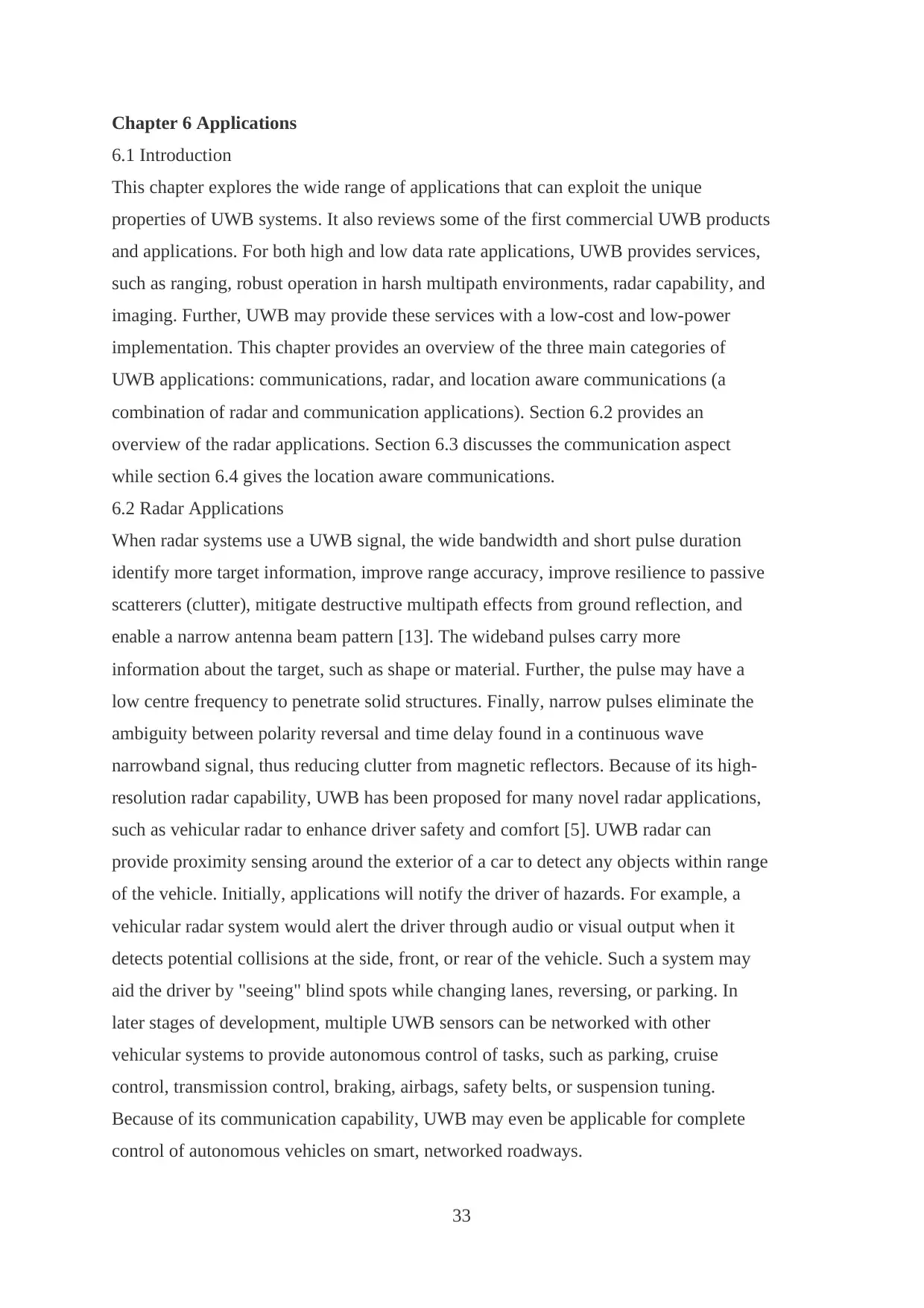
Chapter 6 Applications
6.1 Introduction
This chapter explores the wide range of applications that can exploit the unique
properties of UWB systems. It also reviews some of the first commercial UWB products
and applications. For both high and low data rate applications, UWB provides services,
such as ranging, robust operation in harsh multipath environments, radar capability, and
imaging. Further, UWB may provide these services with a low-cost and low-power
implementation. This chapter provides an overview of the three main categories of
UWB applications: communications, radar, and location aware communications (a
combination of radar and communication applications). Section 6.2 provides an
overview of the radar applications. Section 6.3 discusses the communication aspect
while section 6.4 gives the location aware communications.
6.2 Radar Applications
When radar systems use a UWB signal, the wide bandwidth and short pulse duration
identify more target information, improve range accuracy, improve resilience to passive
scatterers (clutter), mitigate destructive multipath effects from ground reflection, and
enable a narrow antenna beam pattern [13]. The wideband pulses carry more
information about the target, such as shape or material. Further, the pulse may have a
low centre frequency to penetrate solid structures. Finally, narrow pulses eliminate the
ambiguity between polarity reversal and time delay found in a continuous wave
narrowband signal, thus reducing clutter from magnetic reflectors. Because of its high-
resolution radar capability, UWB has been proposed for many novel radar applications,
such as vehicular radar to enhance driver safety and comfort [5]. UWB radar can
provide proximity sensing around the exterior of a car to detect any objects within range
of the vehicle. Initially, applications will notify the driver of hazards. For example, a
vehicular radar system would alert the driver through audio or visual output when it
detects potential collisions at the side, front, or rear of the vehicle. Such a system may
aid the driver by "seeing" blind spots while changing lanes, reversing, or parking. In
later stages of development, multiple UWB sensors can be networked with other
vehicular systems to provide autonomous control of tasks, such as parking, cruise
control, transmission control, braking, airbags, safety belts, or suspension tuning.
Because of its communication capability, UWB may even be applicable for complete
control of autonomous vehicles on smart, networked roadways.
33
6.1 Introduction
This chapter explores the wide range of applications that can exploit the unique
properties of UWB systems. It also reviews some of the first commercial UWB products
and applications. For both high and low data rate applications, UWB provides services,
such as ranging, robust operation in harsh multipath environments, radar capability, and
imaging. Further, UWB may provide these services with a low-cost and low-power
implementation. This chapter provides an overview of the three main categories of
UWB applications: communications, radar, and location aware communications (a
combination of radar and communication applications). Section 6.2 provides an
overview of the radar applications. Section 6.3 discusses the communication aspect
while section 6.4 gives the location aware communications.
6.2 Radar Applications
When radar systems use a UWB signal, the wide bandwidth and short pulse duration
identify more target information, improve range accuracy, improve resilience to passive
scatterers (clutter), mitigate destructive multipath effects from ground reflection, and
enable a narrow antenna beam pattern [13]. The wideband pulses carry more
information about the target, such as shape or material. Further, the pulse may have a
low centre frequency to penetrate solid structures. Finally, narrow pulses eliminate the
ambiguity between polarity reversal and time delay found in a continuous wave
narrowband signal, thus reducing clutter from magnetic reflectors. Because of its high-
resolution radar capability, UWB has been proposed for many novel radar applications,
such as vehicular radar to enhance driver safety and comfort [5]. UWB radar can
provide proximity sensing around the exterior of a car to detect any objects within range
of the vehicle. Initially, applications will notify the driver of hazards. For example, a
vehicular radar system would alert the driver through audio or visual output when it
detects potential collisions at the side, front, or rear of the vehicle. Such a system may
aid the driver by "seeing" blind spots while changing lanes, reversing, or parking. In
later stages of development, multiple UWB sensors can be networked with other
vehicular systems to provide autonomous control of tasks, such as parking, cruise
control, transmission control, braking, airbags, safety belts, or suspension tuning.
Because of its communication capability, UWB may even be applicable for complete
control of autonomous vehicles on smart, networked roadways.
33

Another high-resolution radar application is Ground Penetrating Radar (GPR), which
detects objects buried underground [5]. The crowded infrastructure of cities places a
crisscrossed web of water pipes, electrical lines, communications links, and other
obstacles underground. Heavy construction equipment can damage these structures and
even cause injury or loss of life. GPR provides precise location information for such
obstacles, and it does not rely on records, which can be incomplete, inaccurate,
inaccessible, or missing. Another valuable use of GPR is to detect abandoned land
mines and unexploded ordinance [5]. GPR operates mostly in the low frequency band
below 960 MHz because low frequencies penetrate substances such as soil and sand
better than high frequencies. The operation of GPR is restricted to construction, law
enforcement, fire and rescue, commercial mining, and scientific research in the United
States of America. Through-walls imaging is based on the same principle as GPR;
however, signal energy is now directed horizontally. Therefore, through-walls imaging
has considerable potential to interfere with existing systems, and the FCC restricts it to
the public safety sector for use by fire fighters and law enforcement officials. UWB can
provide resolution fine enough to identify the presence of humans through walls, and it
can even detect the small, involuntary motions of respiration or heartbeats. Finally,
UWB offers promise for medical imaging to enable health care professionals to look
inside the body of a human or animal [5]. For example, UWB can detect movements of
the heart, lungs, vocal cords, vessels, bowels, chest, bladder, or a foetus. Because UWB
radar can resolve images with safe levels of radiation, it can be used even in sensitive
patients, such as mothers in the final stages of pregnancy. With the radiated field of
UWB, the patient can be anywhere, and the imaging device moves while the patient
remains in a comfortable position. Because UWB radar may function a few meters from
the target, it can provide remote monitoring of patients through blankets and clothing.
6.3 Communications
Applications that are more recent focus on wireless personal area networks (WPANs),
which connect a limited number of devices in a small coverage area (within 10 m).
Current UWB radios for WPANs must meet the FCC power limits; hence, they radiate
much less power than earlier systems. Taking full advantage of the high data rate of
UWB, the IEEE 802.15.3a standard endeavours to define medium access and physical
layers with the highest data rate possible for WPAN applications. UWB promises to
deliver extremely high data rates (up to Gbps range) for multimedia applications and
quick download times for large data files.
34
detects objects buried underground [5]. The crowded infrastructure of cities places a
crisscrossed web of water pipes, electrical lines, communications links, and other
obstacles underground. Heavy construction equipment can damage these structures and
even cause injury or loss of life. GPR provides precise location information for such
obstacles, and it does not rely on records, which can be incomplete, inaccurate,
inaccessible, or missing. Another valuable use of GPR is to detect abandoned land
mines and unexploded ordinance [5]. GPR operates mostly in the low frequency band
below 960 MHz because low frequencies penetrate substances such as soil and sand
better than high frequencies. The operation of GPR is restricted to construction, law
enforcement, fire and rescue, commercial mining, and scientific research in the United
States of America. Through-walls imaging is based on the same principle as GPR;
however, signal energy is now directed horizontally. Therefore, through-walls imaging
has considerable potential to interfere with existing systems, and the FCC restricts it to
the public safety sector for use by fire fighters and law enforcement officials. UWB can
provide resolution fine enough to identify the presence of humans through walls, and it
can even detect the small, involuntary motions of respiration or heartbeats. Finally,
UWB offers promise for medical imaging to enable health care professionals to look
inside the body of a human or animal [5]. For example, UWB can detect movements of
the heart, lungs, vocal cords, vessels, bowels, chest, bladder, or a foetus. Because UWB
radar can resolve images with safe levels of radiation, it can be used even in sensitive
patients, such as mothers in the final stages of pregnancy. With the radiated field of
UWB, the patient can be anywhere, and the imaging device moves while the patient
remains in a comfortable position. Because UWB radar may function a few meters from
the target, it can provide remote monitoring of patients through blankets and clothing.
6.3 Communications
Applications that are more recent focus on wireless personal area networks (WPANs),
which connect a limited number of devices in a small coverage area (within 10 m).
Current UWB radios for WPANs must meet the FCC power limits; hence, they radiate
much less power than earlier systems. Taking full advantage of the high data rate of
UWB, the IEEE 802.15.3a standard endeavours to define medium access and physical
layers with the highest data rate possible for WPAN applications. UWB promises to
deliver extremely high data rates (up to Gbps range) for multimedia applications and
quick download times for large data files.
34
Paraphrase This Document
Need a fresh take? Get an instant paraphrase of this document with our AI Paraphraser

To support these applications, the UWB physical layer for IEEE 802.15.3a offers data
rates of 110 Mbps at 10meters, 200 Mbps at 4 meters, and, optionally, 480 Mbps or
higher at a shorter distance. Such data rates allow high-quality multimedia services and
comfortable download times (seconds as opposed to minutes) for large media files [5].
Currently, the most common application for UWB WPAN communications is cable
replacement for high-speed devices. Cabling is a bulky and aesthetically displeasing
means of connecting devices, and it tethers devices together to limit device mobility.
XtremeSpectrum Inc. [5] proposed cable replacement for home networking [5], and
Intel Corporation proposed cable replacement for office environments [5]. In fact, there
are proposals for wireless Universal Serial Bus (USB) and wireless IEEE 1394 for
consumer electronic (CE) devices [5]. These standards would connect peripherals, such
as digital video players, projectors, MP3 players, portable disk drives, camcorders,
high-resolution digital cameras, PDAs, printers, scanners, web cams, home theatres, CD
players, keyboards, or mice [5]. UWB could even provide a standard interface for
wireless docking of laptops [5] or wireless interchip connections.
Figure 6.1 Communication Applications in UWB
6.4 Location Aware Communications
UWB offers a unique blend of radar and communications applications known as
Location Aware Communications. The upcoming IEEE 802.15.4a standard takes
advantage of that combination in a UWB physical layer that emphasizes low data rate,
low power, and location awareness instead of high throughput.
35
rates of 110 Mbps at 10meters, 200 Mbps at 4 meters, and, optionally, 480 Mbps or
higher at a shorter distance. Such data rates allow high-quality multimedia services and
comfortable download times (seconds as opposed to minutes) for large media files [5].
Currently, the most common application for UWB WPAN communications is cable
replacement for high-speed devices. Cabling is a bulky and aesthetically displeasing
means of connecting devices, and it tethers devices together to limit device mobility.
XtremeSpectrum Inc. [5] proposed cable replacement for home networking [5], and
Intel Corporation proposed cable replacement for office environments [5]. In fact, there
are proposals for wireless Universal Serial Bus (USB) and wireless IEEE 1394 for
consumer electronic (CE) devices [5]. These standards would connect peripherals, such
as digital video players, projectors, MP3 players, portable disk drives, camcorders,
high-resolution digital cameras, PDAs, printers, scanners, web cams, home theatres, CD
players, keyboards, or mice [5]. UWB could even provide a standard interface for
wireless docking of laptops [5] or wireless interchip connections.
Figure 6.1 Communication Applications in UWB
6.4 Location Aware Communications
UWB offers a unique blend of radar and communications applications known as
Location Aware Communications. The upcoming IEEE 802.15.4a standard takes
advantage of that combination in a UWB physical layer that emphasizes low data rate,
low power, and location awareness instead of high throughput.
35

The goal of the standard is to provide simple, pervasive, and seamless wireless
connectivity among devices. Suggested application spaces include home automation,
industrial automation, and tracking of people, assets, or geographically localized
phenomena. Many parties desire to use UWB to provide communications devices that
can locate people. In a burning building, heavy smoke and darkness impair both audio
and visual communications. Samsung and Staccato Communications have proposed
smart home applications that manage almost all aspects of the home, including doors,
keys, TV, radio, and computers [5].
The system keeps track of individual users and their preferences. As individuals move
throughout the smart home, a tracking system could automatically adjust room
temperatures and activate entertainment devices to a particular television channel or
website. General Atomics offers proximity sensors that provide security for cars,
computers, or homes, which automatically lock and unlock as the owner travels in and
out of range [5]. LB & J consulting suggests that UWB could be used to track students
and personnel in school buildings [5]. In schools, the tracking system could control
access to school buildings, conduct instant roll call, produce reports to analyze incident
patterns, monitor school bus transportation, and engage parents in student supervision.
Ubisense Ltd. Proposes to increase productivity in office environments by locating key
personnel [5].
Location-aware communications capability is used to manage assets. UWB expedites
the inventory control process because the network can automatically scan individual
containers and report status information without physical examination of the container's
contents. Inforange offers a similar suggested application for tracking packages during
shipment to reduce the amount of lost and misrouted packages. They propose a
transmit-only device to save power in the tags.
General Atomics extends this idea to tracking for inventory control in warehouses and
retail shops [5]. A similar technology could be used as small, discrete security tags for
high-valued items, such as leather jackets in retail stores [5]. Both Ubisense and MSSI
have suggested tracking life-saving equipment in hospitals, as it is not always possible
to locate equipment quickly in an emergency.
Tracking mobile objects is another application that uses UWB's radar capability. One
idea is a UWB network that provides a protective security "bubble" around a geographic
area.
36
connectivity among devices. Suggested application spaces include home automation,
industrial automation, and tracking of people, assets, or geographically localized
phenomena. Many parties desire to use UWB to provide communications devices that
can locate people. In a burning building, heavy smoke and darkness impair both audio
and visual communications. Samsung and Staccato Communications have proposed
smart home applications that manage almost all aspects of the home, including doors,
keys, TV, radio, and computers [5].
The system keeps track of individual users and their preferences. As individuals move
throughout the smart home, a tracking system could automatically adjust room
temperatures and activate entertainment devices to a particular television channel or
website. General Atomics offers proximity sensors that provide security for cars,
computers, or homes, which automatically lock and unlock as the owner travels in and
out of range [5]. LB & J consulting suggests that UWB could be used to track students
and personnel in school buildings [5]. In schools, the tracking system could control
access to school buildings, conduct instant roll call, produce reports to analyze incident
patterns, monitor school bus transportation, and engage parents in student supervision.
Ubisense Ltd. Proposes to increase productivity in office environments by locating key
personnel [5].
Location-aware communications capability is used to manage assets. UWB expedites
the inventory control process because the network can automatically scan individual
containers and report status information without physical examination of the container's
contents. Inforange offers a similar suggested application for tracking packages during
shipment to reduce the amount of lost and misrouted packages. They propose a
transmit-only device to save power in the tags.
General Atomics extends this idea to tracking for inventory control in warehouses and
retail shops [5]. A similar technology could be used as small, discrete security tags for
high-valued items, such as leather jackets in retail stores [5]. Both Ubisense and MSSI
have suggested tracking life-saving equipment in hospitals, as it is not always possible
to locate equipment quickly in an emergency.
Tracking mobile objects is another application that uses UWB's radar capability. One
idea is a UWB network that provides a protective security "bubble" around a geographic
area.
36
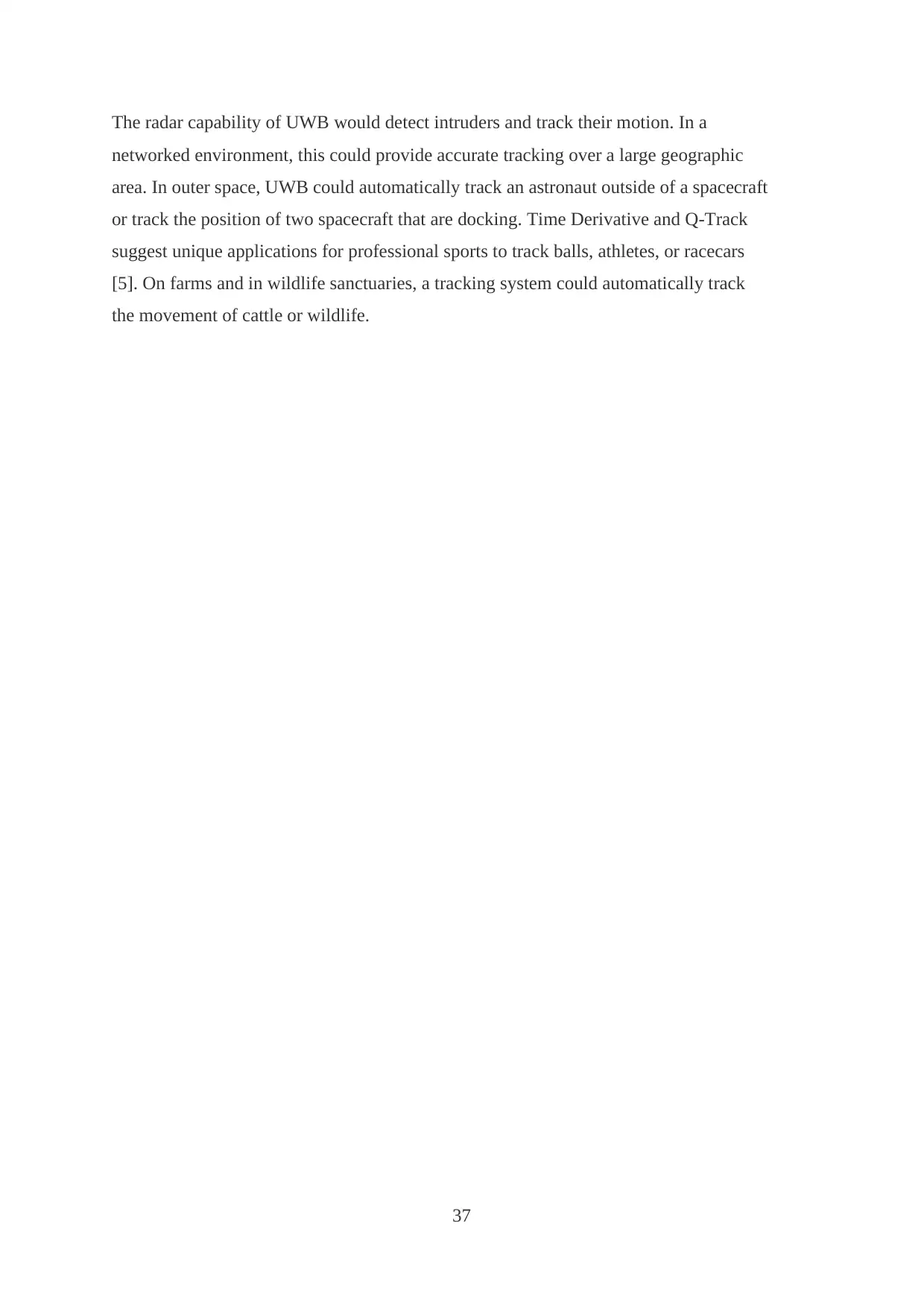
The radar capability of UWB would detect intruders and track their motion. In a
networked environment, this could provide accurate tracking over a large geographic
area. In outer space, UWB could automatically track an astronaut outside of a spacecraft
or track the position of two spacecraft that are docking. Time Derivative and Q-Track
suggest unique applications for professional sports to track balls, athletes, or racecars
[5]. On farms and in wildlife sanctuaries, a tracking system could automatically track
the movement of cattle or wildlife.
37
networked environment, this could provide accurate tracking over a large geographic
area. In outer space, UWB could automatically track an astronaut outside of a spacecraft
or track the position of two spacecraft that are docking. Time Derivative and Q-Track
suggest unique applications for professional sports to track balls, athletes, or racecars
[5]. On farms and in wildlife sanctuaries, a tracking system could automatically track
the movement of cattle or wildlife.
37
Secure Best Marks with AI Grader
Need help grading? Try our AI Grader for instant feedback on your assignments.

Chapter 7 Future Work and Recommendations
7.1 Conclusion and Recommendations
With increasing demand for spectrum allocation to accommodate new commercial
wireless services, UWB has proven to offer a win-win innovation that makes available
efficient use and re-use for critical spectrum. It was with the notion that an impulse-
based software radio UWB transceiver design was presented to accomplish goals such
as a raw data rate of 100 Mbps, flexibility in terms of modulation schemes and receiver
topologies, and a method that can incorporate multipath energy in dense environments.
Another benefit of this implementation is the capability to upgrade performance of
system characteristics with a software change instead of building a new hardware
configuration, making the system ideal for a UWB test simulation. Each of these
advantages was demonstrated in this project, along with system verification through
simulation in MATLAB. Throughout the design presented in this project, suggestions
have been made to make improvements to the design. In addition, the findings in this
project will lead to revisions made in the future, as more system specifications are
defined. As discussed previously, since the software radio UWB system was designed to
be flexible, many of the communication system and receiver algorithm designs can be
further implemented. Future design modifications not proposed in this project will be
made based on future simulation and implementation results.
7.2 Future Work
Technological progress and innovation occurs faster mainly in the electrical and
electronic fraternity. Advances in integrated circuits and fabrication of low power
consumptions evolve very quickly as technological ventures are pursued.
There is a large amount of future work left on the UWB system, as none of the
construction for the transceiver has begun. In addition, working towards creation of a
schematic and PCB layout, this is highly complex due to the strenuous layout criteria
necessary, such as determining trace lengths for the ADC clock. A mechanical design
for the heat sink must also be completed, as the dissipated heat on the digital boards at
the receiver is approximately 100 Watts per board. One of the last hardware
considerations is the implementation of the Rocket I/O transceivers to communicate
between the two digital circuit boards. Design of a high-speed data bus with the
capability to handle data at 2.5 Gbps is necessary.
38
7.1 Conclusion and Recommendations
With increasing demand for spectrum allocation to accommodate new commercial
wireless services, UWB has proven to offer a win-win innovation that makes available
efficient use and re-use for critical spectrum. It was with the notion that an impulse-
based software radio UWB transceiver design was presented to accomplish goals such
as a raw data rate of 100 Mbps, flexibility in terms of modulation schemes and receiver
topologies, and a method that can incorporate multipath energy in dense environments.
Another benefit of this implementation is the capability to upgrade performance of
system characteristics with a software change instead of building a new hardware
configuration, making the system ideal for a UWB test simulation. Each of these
advantages was demonstrated in this project, along with system verification through
simulation in MATLAB. Throughout the design presented in this project, suggestions
have been made to make improvements to the design. In addition, the findings in this
project will lead to revisions made in the future, as more system specifications are
defined. As discussed previously, since the software radio UWB system was designed to
be flexible, many of the communication system and receiver algorithm designs can be
further implemented. Future design modifications not proposed in this project will be
made based on future simulation and implementation results.
7.2 Future Work
Technological progress and innovation occurs faster mainly in the electrical and
electronic fraternity. Advances in integrated circuits and fabrication of low power
consumptions evolve very quickly as technological ventures are pursued.
There is a large amount of future work left on the UWB system, as none of the
construction for the transceiver has begun. In addition, working towards creation of a
schematic and PCB layout, this is highly complex due to the strenuous layout criteria
necessary, such as determining trace lengths for the ADC clock. A mechanical design
for the heat sink must also be completed, as the dissipated heat on the digital boards at
the receiver is approximately 100 Watts per board. One of the last hardware
considerations is the implementation of the Rocket I/O transceivers to communicate
between the two digital circuit boards. Design of a high-speed data bus with the
capability to handle data at 2.5 Gbps is necessary.
38

Finally, to perform the acquisition, synchronization, and data demodulation techniques
presented, software must be developed to process data within the FPGA. The software
must be optimized to reduce the amount of processing time for each algorithm, as it will
ultimately control the system’s data rate.
UWB is a promising study due to its flexibility while maintaining its character and
properties. Moreover, the applications state that future work for any UWB project
should proceed in a particular direction. There is a lot of untapped UWB potential, in
the construction and implementation of this UWB transceiver. Initial work on the
system simulation should encourage real time implementation of the circuits
Future work should concentrate on either building a UWB radar or a communicator or a
location aware as advocated by UWB applications. This would stimulate technological
advances in implementing real case scenarios such as: UWB circuits as RFIDs for
supermarkets, wireless mouse, keyboards and data streaming techniques. After all, the
society judges any innovation not on terms of its study but its relevance in elevating
societal problems, its viability and marketability in terms of cost, availability and
effects. Future UWB systems must take this approach.
39
presented, software must be developed to process data within the FPGA. The software
must be optimized to reduce the amount of processing time for each algorithm, as it will
ultimately control the system’s data rate.
UWB is a promising study due to its flexibility while maintaining its character and
properties. Moreover, the applications state that future work for any UWB project
should proceed in a particular direction. There is a lot of untapped UWB potential, in
the construction and implementation of this UWB transceiver. Initial work on the
system simulation should encourage real time implementation of the circuits
Future work should concentrate on either building a UWB radar or a communicator or a
location aware as advocated by UWB applications. This would stimulate technological
advances in implementing real case scenarios such as: UWB circuits as RFIDs for
supermarkets, wireless mouse, keyboards and data streaming techniques. After all, the
society judges any innovation not on terms of its study but its relevance in elevating
societal problems, its viability and marketability in terms of cost, availability and
effects. Future UWB systems must take this approach.
39

References
[1] Kazimierz Siwiak and Debra Mckeown, “Ultra wideband Radio technology,”
John Wiley & Sons Ltd. First Edition 2004
[2] X. Shen, M. Guizani and R.C. Qiu, “Ultra-Wideband Wireless Communications
and network,” John Wiley & Sons Ltd. 2006
[3] R. A. Scholtz, “Multiple Access with Time-hopping Impulse Modulator (invited
paper),” MILCOM ’93, Bedford, MA, Oct. 11–14, 1993.
[4] T. W. Barrett, “History of Ultra Wideband Communications and Radar: Part II,
UWB Radar and Sensors,” Microwave J., Euro-Global Edition, vol. 44, no. 2, pp.
22–52, Feb. 2001.
[5] Jeffrey H. Reed, “Introduction to Ultra wideband Communication systems,”
Prentice Hall Publications, First printing March 2005.
[6] R. C. Qiu, H. P. Liu, and X. Shen, “Ultra-Wideband for Multiple Access,” IEEE
Commun. Mag., vol. 43, no. 2, pp. 80–87, Feb. 2005.
[7] H. F. Harmuth, "Signal Processing and Transmission by Means of Walsh
Functions," U.S. Patent 3,678,204, dated July 18, 1972.
[8] David Marchaland, Martine Villegas et al, “System Concepts dedicated to UWB
Transmitter,” email: d.marchaland@esiee.fr.
[9] Matlab, Version 7.6.0.324 (R2008a), The Mathworks, Inc., Natick, MA.
[10] F. Ramirez-Mireles, “On the Performance of Ultra-Wideband Signals in
Gaussian Noise and Dense Multipath,”IEEE Trans. Veh. Technol., vol. 50, no. 1,
pp. 244–249, Jan. 2001.
[11] F. Ram´ırez-Mireles, “Signal Design for Ultra-wideband Communications in
Dense Multipath,” IEEE Trans. Veh. Technol., vol. 51, no. 6, pp. 1517–1521,
Nov. 2002.
[12] D. Porcino and W. Hirt, “Ultra-Wideband Radio Technology: Potential and
Challenges Ahead,” IEEE Commun. Mag., vol. 41, no. 7, pp. 66–74, July 2003.
[13] I. I. Immoreev and D. V. Fedotov, "Ultra Wideband Radar System: Advantages
and Disadvantages," Digest of Papers 2002 IEEE Conference on Ultra Wideband
Systems and Technologies, pp. 201-206, May 2002
[14] C. R. Anderson, A. M. Orndorff, R. M. Buehrer, and J. H. Reed, “An
Introduction and Overview of an Impulse-Radio Ultra wideband Communication
System Design,” tech. rep., MPRG, Virginia Polytechnic Institute and State
University, June 2004.
40
[1] Kazimierz Siwiak and Debra Mckeown, “Ultra wideband Radio technology,”
John Wiley & Sons Ltd. First Edition 2004
[2] X. Shen, M. Guizani and R.C. Qiu, “Ultra-Wideband Wireless Communications
and network,” John Wiley & Sons Ltd. 2006
[3] R. A. Scholtz, “Multiple Access with Time-hopping Impulse Modulator (invited
paper),” MILCOM ’93, Bedford, MA, Oct. 11–14, 1993.
[4] T. W. Barrett, “History of Ultra Wideband Communications and Radar: Part II,
UWB Radar and Sensors,” Microwave J., Euro-Global Edition, vol. 44, no. 2, pp.
22–52, Feb. 2001.
[5] Jeffrey H. Reed, “Introduction to Ultra wideband Communication systems,”
Prentice Hall Publications, First printing March 2005.
[6] R. C. Qiu, H. P. Liu, and X. Shen, “Ultra-Wideband for Multiple Access,” IEEE
Commun. Mag., vol. 43, no. 2, pp. 80–87, Feb. 2005.
[7] H. F. Harmuth, "Signal Processing and Transmission by Means of Walsh
Functions," U.S. Patent 3,678,204, dated July 18, 1972.
[8] David Marchaland, Martine Villegas et al, “System Concepts dedicated to UWB
Transmitter,” email: d.marchaland@esiee.fr.
[9] Matlab, Version 7.6.0.324 (R2008a), The Mathworks, Inc., Natick, MA.
[10] F. Ramirez-Mireles, “On the Performance of Ultra-Wideband Signals in
Gaussian Noise and Dense Multipath,”IEEE Trans. Veh. Technol., vol. 50, no. 1,
pp. 244–249, Jan. 2001.
[11] F. Ram´ırez-Mireles, “Signal Design for Ultra-wideband Communications in
Dense Multipath,” IEEE Trans. Veh. Technol., vol. 51, no. 6, pp. 1517–1521,
Nov. 2002.
[12] D. Porcino and W. Hirt, “Ultra-Wideband Radio Technology: Potential and
Challenges Ahead,” IEEE Commun. Mag., vol. 41, no. 7, pp. 66–74, July 2003.
[13] I. I. Immoreev and D. V. Fedotov, "Ultra Wideband Radar System: Advantages
and Disadvantages," Digest of Papers 2002 IEEE Conference on Ultra Wideband
Systems and Technologies, pp. 201-206, May 2002
[14] C. R. Anderson, A. M. Orndorff, R. M. Buehrer, and J. H. Reed, “An
Introduction and Overview of an Impulse-Radio Ultra wideband Communication
System Design,” tech. rep., MPRG, Virginia Polytechnic Institute and State
University, June 2004.
40
Paraphrase This Document
Need a fresh take? Get an instant paraphrase of this document with our AI Paraphraser

Appendix A Summary of FCC Regulations on UWB
This Appendix contains the excerpts from the Introduction and Executive Summary of
the FCC First Report and Order, ET Docket 98–153 adopted February 14, 2002 and
released April 22, 2002 by the Federal Communications Commission (FCC). The Report
and Order establishes the basis and justification for the FCC rules.
On September 1, 1998, the FCC issued a Notice of Inquiry pertaining to the revision of
Part 15 rules to allow the unlicensed use of UWB devices [5]. The FCC was motivated
by the potential for a host of new applications for UWB technology: high-precision
radar, through-wall imaging, medical imaging, remote sensors, and secure voice and
data communications. Investigating the potential use of UWB devices presented a very
different mode of operation for the FCC. Instead of dividing the spectrum into distinct
bands that were then allocated to specific users/services, UWB devices would be
allowed to operate overlaid with existing services. Essentially, the UWB device would
be allowed to interfere with existing services, ideally at a low enough power level that
existing services would not experience performance degradation. The operation of UWB
devices in tandem with existing users is a significantly different approach to spectral
efficiency than achieving the highest possible data rates in a channel with precisely
defined bandwidths. In fact, many have questioned whether the operation of UWB
devices is "efficient" in the strict sense of the word, or if it is instead an exercise in
interference tolerance.
Specifically, the FCC was concerned about the potential interference from UWB
transmissions on Global Positioning System (GPS) signals and commercial/military
avionics signals. On February 14, 2002, the FCC issued a First Report and Order [5],
which classified UWB operation into three separate categories:
1. Communication and Measurement Systems
2. Vehicular Radar Systems
3. Imaging Systems, including Ground Penetrating Radar, Through-Wall Imaging
and Surveillance Systems, and Medical Imaging.
Table A.1 summarizes the various UWB operational categories and their allocated
bandwidths, along with restrictions on organizations that are allowed to operate in that
particular mode.
41
This Appendix contains the excerpts from the Introduction and Executive Summary of
the FCC First Report and Order, ET Docket 98–153 adopted February 14, 2002 and
released April 22, 2002 by the Federal Communications Commission (FCC). The Report
and Order establishes the basis and justification for the FCC rules.
On September 1, 1998, the FCC issued a Notice of Inquiry pertaining to the revision of
Part 15 rules to allow the unlicensed use of UWB devices [5]. The FCC was motivated
by the potential for a host of new applications for UWB technology: high-precision
radar, through-wall imaging, medical imaging, remote sensors, and secure voice and
data communications. Investigating the potential use of UWB devices presented a very
different mode of operation for the FCC. Instead of dividing the spectrum into distinct
bands that were then allocated to specific users/services, UWB devices would be
allowed to operate overlaid with existing services. Essentially, the UWB device would
be allowed to interfere with existing services, ideally at a low enough power level that
existing services would not experience performance degradation. The operation of UWB
devices in tandem with existing users is a significantly different approach to spectral
efficiency than achieving the highest possible data rates in a channel with precisely
defined bandwidths. In fact, many have questioned whether the operation of UWB
devices is "efficient" in the strict sense of the word, or if it is instead an exercise in
interference tolerance.
Specifically, the FCC was concerned about the potential interference from UWB
transmissions on Global Positioning System (GPS) signals and commercial/military
avionics signals. On February 14, 2002, the FCC issued a First Report and Order [5],
which classified UWB operation into three separate categories:
1. Communication and Measurement Systems
2. Vehicular Radar Systems
3. Imaging Systems, including Ground Penetrating Radar, Through-Wall Imaging
and Surveillance Systems, and Medical Imaging.
Table A.1 summarizes the various UWB operational categories and their allocated
bandwidths, along with restrictions on organizations that are allowed to operate in that
particular mode.
41

Table A.1. Summary of FCC Restrictions on UWB Operation.
Application Frequency Band for
Operation at Part 15
Limits
User Restrictions
Communications and Measurement
Systems (sensors)
3.1-10.6 GHz (different
emission limits for
indoor and outdoor
systems)
None
Vehicular Radar for collision
avoidance, airbag activation, and
suspension system control
24-29 GHz None
Ground Penetrating Radar to see or
detect buried objects
3.1-10.6 GHz and
below 960 MHz
Law enforcement, fire
and rescue, research
institutions, mining,
construction
Wall Imaging Systems to detect
objects contained in walls
3.1-10.6 GHz and
below 960 MHz
Law enforcement, fire
and rescue, mining,
construction
Through-wall Imaging Systems to
detect location or movement of
objects located on the other side of
a wall
1.99-10.6 GHz and
below 960 MHz
Law enforcement, fire
and rescue
Medical Systems for imaging
inside people and animals
3.1-10.6 GHz Medical personnel
Surveillance Systems for intrusion
detection
1.99-10.6 GHz Law enforcement, fire
and rescue, public
utilities, and industry
42
Application Frequency Band for
Operation at Part 15
Limits
User Restrictions
Communications and Measurement
Systems (sensors)
3.1-10.6 GHz (different
emission limits for
indoor and outdoor
systems)
None
Vehicular Radar for collision
avoidance, airbag activation, and
suspension system control
24-29 GHz None
Ground Penetrating Radar to see or
detect buried objects
3.1-10.6 GHz and
below 960 MHz
Law enforcement, fire
and rescue, research
institutions, mining,
construction
Wall Imaging Systems to detect
objects contained in walls
3.1-10.6 GHz and
below 960 MHz
Law enforcement, fire
and rescue, mining,
construction
Through-wall Imaging Systems to
detect location or movement of
objects located on the other side of
a wall
1.99-10.6 GHz and
below 960 MHz
Law enforcement, fire
and rescue
Medical Systems for imaging
inside people and animals
3.1-10.6 GHz Medical personnel
Surveillance Systems for intrusion
detection
1.99-10.6 GHz Law enforcement, fire
and rescue, public
utilities, and industry
42
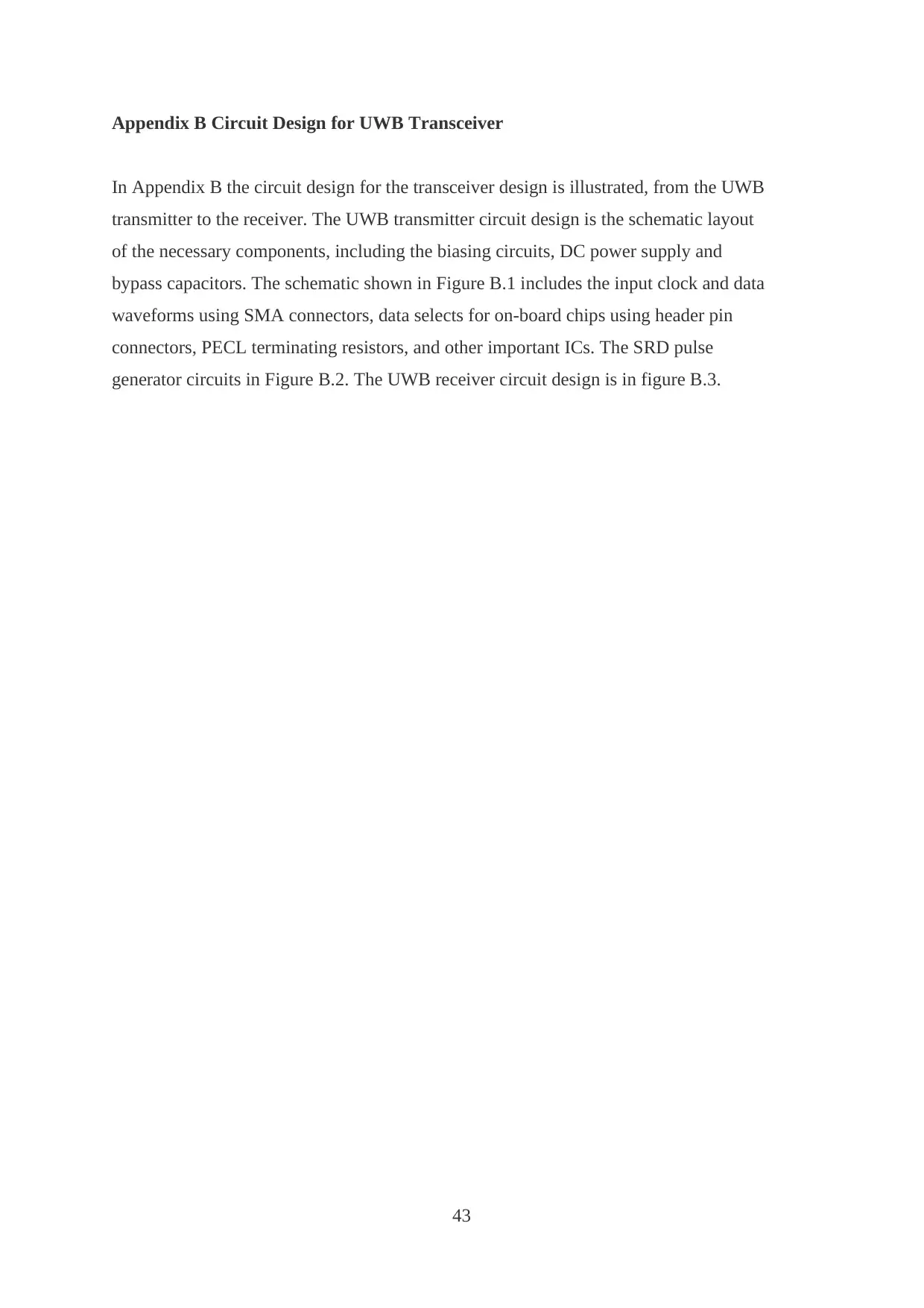
Appendix B Circuit Design for UWB Transceiver
In Appendix B the circuit design for the transceiver design is illustrated, from the UWB
transmitter to the receiver. The UWB transmitter circuit design is the schematic layout
of the necessary components, including the biasing circuits, DC power supply and
bypass capacitors. The schematic shown in Figure B.1 includes the input clock and data
waveforms using SMA connectors, data selects for on-board chips using header pin
connectors, PECL terminating resistors, and other important ICs. The SRD pulse
generator circuits in Figure B.2. The UWB receiver circuit design is in figure B.3.
43
In Appendix B the circuit design for the transceiver design is illustrated, from the UWB
transmitter to the receiver. The UWB transmitter circuit design is the schematic layout
of the necessary components, including the biasing circuits, DC power supply and
bypass capacitors. The schematic shown in Figure B.1 includes the input clock and data
waveforms using SMA connectors, data selects for on-board chips using header pin
connectors, PECL terminating resistors, and other important ICs. The SRD pulse
generator circuits in Figure B.2. The UWB receiver circuit design is in figure B.3.
43
Secure Best Marks with AI Grader
Need help grading? Try our AI Grader for instant feedback on your assignments.

Figure B.1 UWB Schematic of the Input [14].
44
44

Figure B.2 UWB Schematic of the SRD Pulse generator [14].
45
45

Figure B.3 UWB Receiver Circuit Design [14]
46
46
Paraphrase This Document
Need a fresh take? Get an instant paraphrase of this document with our AI Paraphraser

Appendix C Transceiver System Simulation in MATLAB
The appendix shows the whole transceiver block as simulated in MATLAB SIMULINK.
Note this is the simulated transceiver with the transmitter, channel and receiver all
together in figure C.1.
Figure C.1 UWB Transceiver Design in SIMULINK
47
The appendix shows the whole transceiver block as simulated in MATLAB SIMULINK.
Note this is the simulated transceiver with the transmitter, channel and receiver all
together in figure C.1.
Figure C.1 UWB Transceiver Design in SIMULINK
47
1 out of 56
![[object Object]](/_next/image/?url=%2F_next%2Fstatic%2Fmedia%2Flogo.6d15ce61.png&w=640&q=75)
Your All-in-One AI-Powered Toolkit for Academic Success.
+13062052269
info@desklib.com
Available 24*7 on WhatsApp / Email
Unlock your academic potential
© 2024 | Zucol Services PVT LTD | All rights reserved.