Report on Structural Member Behavior, Bond, and Flexural Analysis
VerifiedAdded on 2020/03/04
|22
|9906
|432
Report
AI Summary
This report comprehensively analyzes the structural behavior of building components, focusing on the interaction between structural members, bond behavior, and flexural characteristics. It begins by categorizing structural members and explaining their behavior under various stresses, includi...
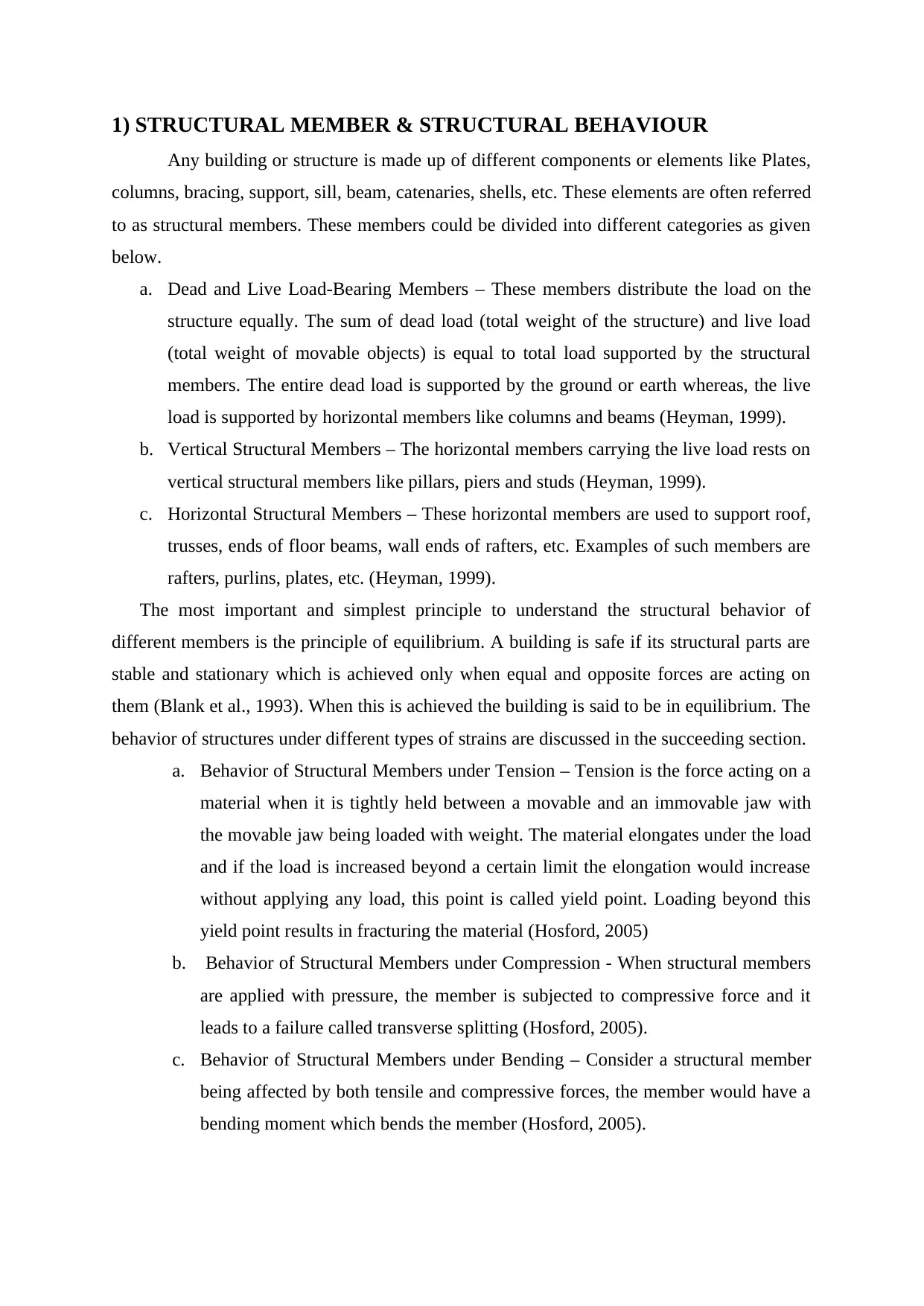
1) STRUCTURAL MEMBER & STRUCTURAL BEHAVIOUR
Any building or structure is made up of different components or elements like Plates,
columns, bracing, support, sill, beam, catenaries, shells, etc. These elements are often referred
to as structural members. These members could be divided into different categories as given
below.
a. Dead and Live Load-Bearing Members – These members distribute the load on the
structure equally. The sum of dead load (total weight of the structure) and live load
(total weight of movable objects) is equal to total load supported by the structural
members. The entire dead load is supported by the ground or earth whereas, the live
load is supported by horizontal members like columns and beams (Heyman, 1999).
b. Vertical Structural Members – The horizontal members carrying the live load rests on
vertical structural members like pillars, piers and studs (Heyman, 1999).
c. Horizontal Structural Members – These horizontal members are used to support roof,
trusses, ends of floor beams, wall ends of rafters, etc. Examples of such members are
rafters, purlins, plates, etc. (Heyman, 1999).
The most important and simplest principle to understand the structural behavior of
different members is the principle of equilibrium. A building is safe if its structural parts are
stable and stationary which is achieved only when equal and opposite forces are acting on
them (Blank et al., 1993). When this is achieved the building is said to be in equilibrium. The
behavior of structures under different types of strains are discussed in the succeeding section.
a. Behavior of Structural Members under Tension – Tension is the force acting on a
material when it is tightly held between a movable and an immovable jaw with
the movable jaw being loaded with weight. The material elongates under the load
and if the load is increased beyond a certain limit the elongation would increase
without applying any load, this point is called yield point. Loading beyond this
yield point results in fracturing the material (Hosford, 2005)
b. Behavior of Structural Members under Compression - When structural members
are applied with pressure, the member is subjected to compressive force and it
leads to a failure called transverse splitting (Hosford, 2005).
c. Behavior of Structural Members under Bending – Consider a structural member
being affected by both tensile and compressive forces, the member would have a
bending moment which bends the member (Hosford, 2005).
Any building or structure is made up of different components or elements like Plates,
columns, bracing, support, sill, beam, catenaries, shells, etc. These elements are often referred
to as structural members. These members could be divided into different categories as given
below.
a. Dead and Live Load-Bearing Members – These members distribute the load on the
structure equally. The sum of dead load (total weight of the structure) and live load
(total weight of movable objects) is equal to total load supported by the structural
members. The entire dead load is supported by the ground or earth whereas, the live
load is supported by horizontal members like columns and beams (Heyman, 1999).
b. Vertical Structural Members – The horizontal members carrying the live load rests on
vertical structural members like pillars, piers and studs (Heyman, 1999).
c. Horizontal Structural Members – These horizontal members are used to support roof,
trusses, ends of floor beams, wall ends of rafters, etc. Examples of such members are
rafters, purlins, plates, etc. (Heyman, 1999).
The most important and simplest principle to understand the structural behavior of
different members is the principle of equilibrium. A building is safe if its structural parts are
stable and stationary which is achieved only when equal and opposite forces are acting on
them (Blank et al., 1993). When this is achieved the building is said to be in equilibrium. The
behavior of structures under different types of strains are discussed in the succeeding section.
a. Behavior of Structural Members under Tension – Tension is the force acting on a
material when it is tightly held between a movable and an immovable jaw with
the movable jaw being loaded with weight. The material elongates under the load
and if the load is increased beyond a certain limit the elongation would increase
without applying any load, this point is called yield point. Loading beyond this
yield point results in fracturing the material (Hosford, 2005)
b. Behavior of Structural Members under Compression - When structural members
are applied with pressure, the member is subjected to compressive force and it
leads to a failure called transverse splitting (Hosford, 2005).
c. Behavior of Structural Members under Bending – Consider a structural member
being affected by both tensile and compressive forces, the member would have a
bending moment which bends the member (Hosford, 2005).
Secure Best Marks with AI Grader
Need help grading? Try our AI Grader for instant feedback on your assignments.
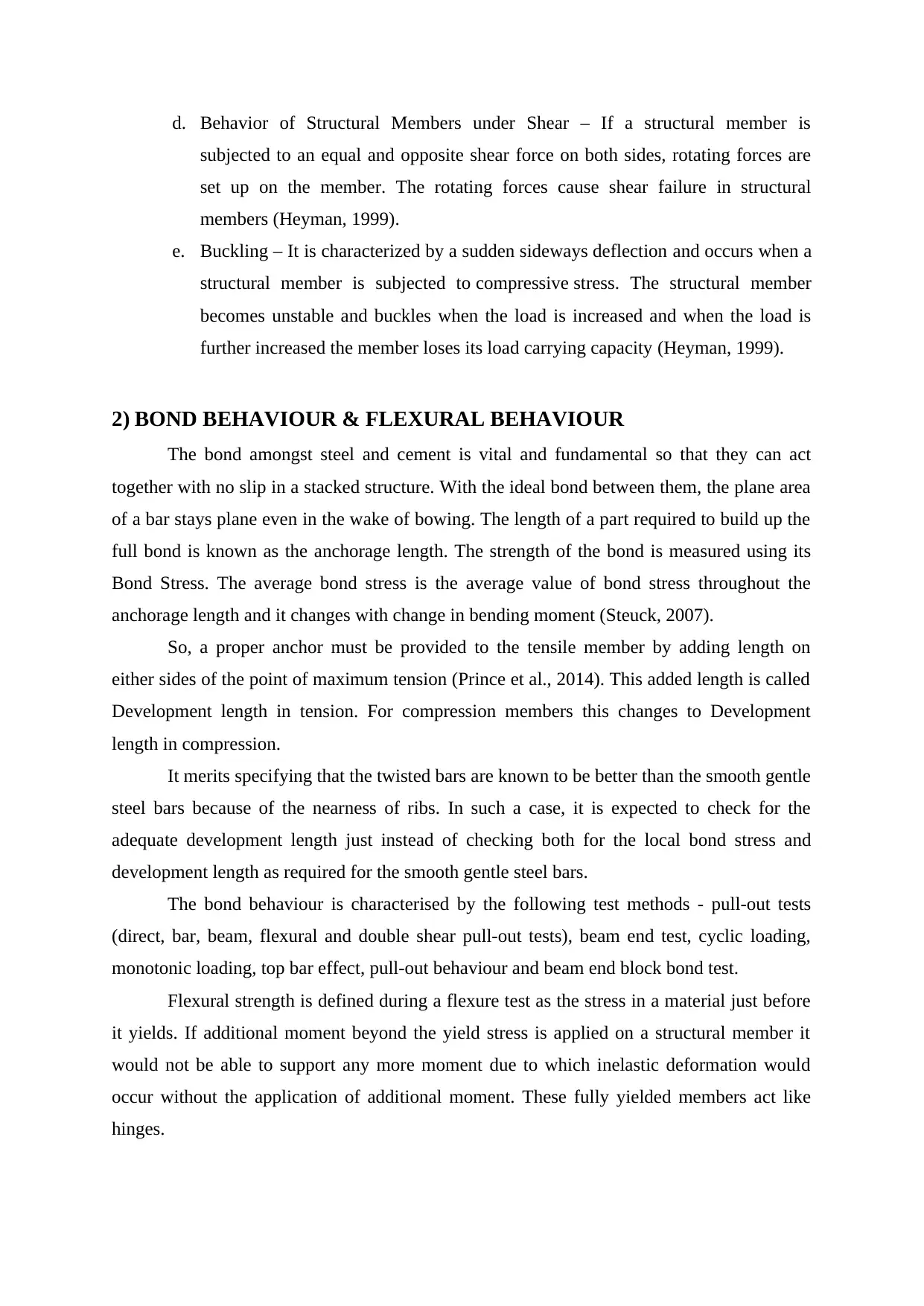
d. Behavior of Structural Members under Shear – If a structural member is
subjected to an equal and opposite shear force on both sides, rotating forces are
set up on the member. The rotating forces cause shear failure in structural
members (Heyman, 1999).
e. Buckling – It is characterized by a sudden sideways deflection and occurs when a
structural member is subjected to compressive stress. The structural member
becomes unstable and buckles when the load is increased and when the load is
further increased the member loses its load carrying capacity (Heyman, 1999).
2) BOND BEHAVIOUR & FLEXURAL BEHAVIOUR
The bond amongst steel and cement is vital and fundamental so that they can act
together with no slip in a stacked structure. With the ideal bond between them, the plane area
of a bar stays plane even in the wake of bowing. The length of a part required to build up the
full bond is known as the anchorage length. The strength of the bond is measured using its
Bond Stress. The average bond stress is the average value of bond stress throughout the
anchorage length and it changes with change in bending moment (Steuck, 2007).
So, a proper anchor must be provided to the tensile member by adding length on
either sides of the point of maximum tension (Prince et al., 2014). This added length is called
Development length in tension. For compression members this changes to Development
length in compression.
It merits specifying that the twisted bars are known to be better than the smooth gentle
steel bars because of the nearness of ribs. In such a case, it is expected to check for the
adequate development length just instead of checking both for the local bond stress and
development length as required for the smooth gentle steel bars.
The bond behaviour is characterised by the following test methods - pull-out tests
(direct, bar, beam, flexural and double shear pull-out tests), beam end test, cyclic loading,
monotonic loading, top bar effect, pull-out behaviour and beam end block bond test.
Flexural strength is defined during a flexure test as the stress in a material just before
it yields. If additional moment beyond the yield stress is applied on a structural member it
would not be able to support any more moment due to which inelastic deformation would
occur without the application of additional moment. These fully yielded members act like
hinges.
subjected to an equal and opposite shear force on both sides, rotating forces are
set up on the member. The rotating forces cause shear failure in structural
members (Heyman, 1999).
e. Buckling – It is characterized by a sudden sideways deflection and occurs when a
structural member is subjected to compressive stress. The structural member
becomes unstable and buckles when the load is increased and when the load is
further increased the member loses its load carrying capacity (Heyman, 1999).
2) BOND BEHAVIOUR & FLEXURAL BEHAVIOUR
The bond amongst steel and cement is vital and fundamental so that they can act
together with no slip in a stacked structure. With the ideal bond between them, the plane area
of a bar stays plane even in the wake of bowing. The length of a part required to build up the
full bond is known as the anchorage length. The strength of the bond is measured using its
Bond Stress. The average bond stress is the average value of bond stress throughout the
anchorage length and it changes with change in bending moment (Steuck, 2007).
So, a proper anchor must be provided to the tensile member by adding length on
either sides of the point of maximum tension (Prince et al., 2014). This added length is called
Development length in tension. For compression members this changes to Development
length in compression.
It merits specifying that the twisted bars are known to be better than the smooth gentle
steel bars because of the nearness of ribs. In such a case, it is expected to check for the
adequate development length just instead of checking both for the local bond stress and
development length as required for the smooth gentle steel bars.
The bond behaviour is characterised by the following test methods - pull-out tests
(direct, bar, beam, flexural and double shear pull-out tests), beam end test, cyclic loading,
monotonic loading, top bar effect, pull-out behaviour and beam end block bond test.
Flexural strength is defined during a flexure test as the stress in a material just before
it yields. If additional moment beyond the yield stress is applied on a structural member it
would not be able to support any more moment due to which inelastic deformation would
occur without the application of additional moment. These fully yielded members act like
hinges.
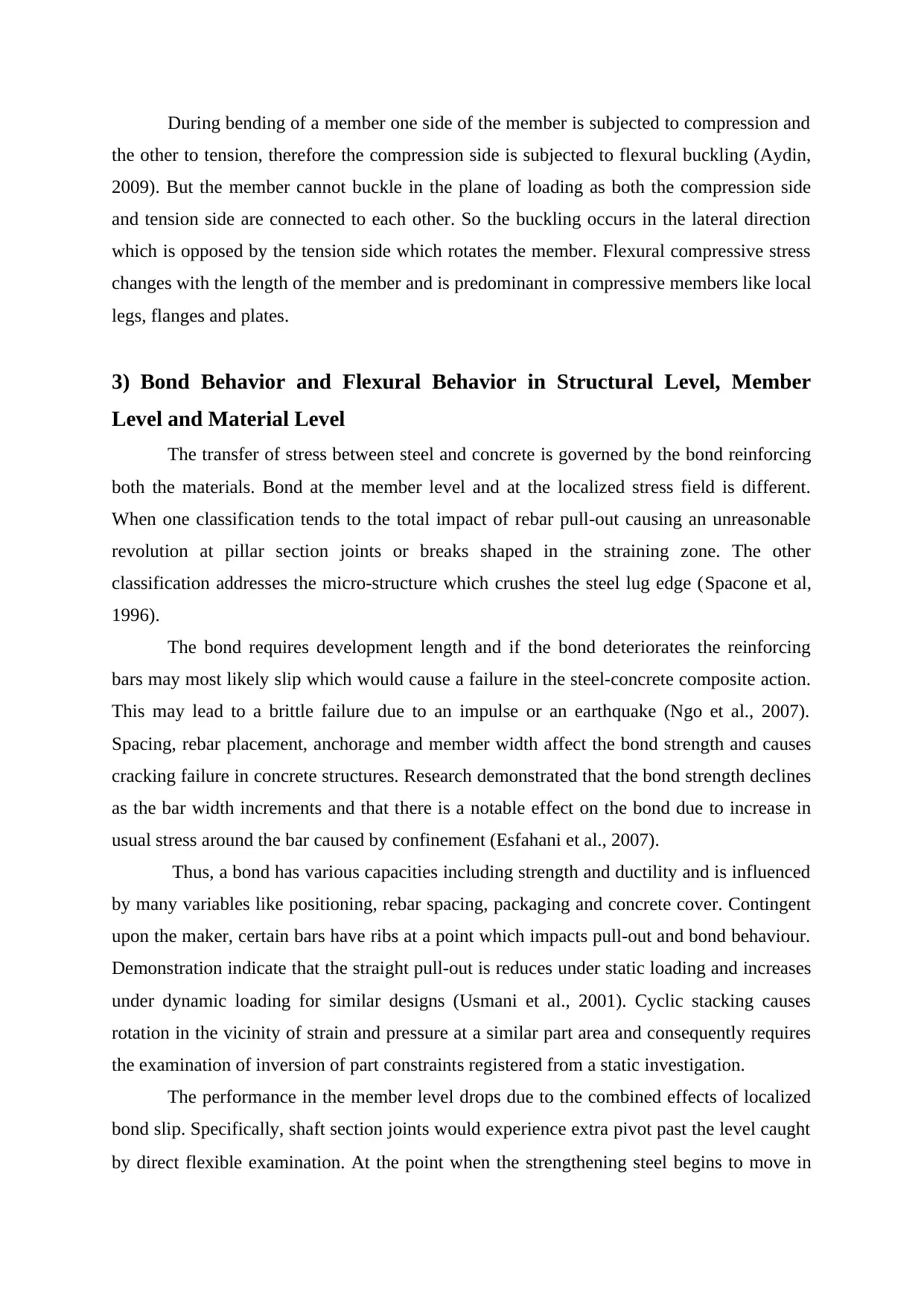
During bending of a member one side of the member is subjected to compression and
the other to tension, therefore the compression side is subjected to flexural buckling (Aydin,
2009). But the member cannot buckle in the plane of loading as both the compression side
and tension side are connected to each other. So the buckling occurs in the lateral direction
which is opposed by the tension side which rotates the member. Flexural compressive stress
changes with the length of the member and is predominant in compressive members like local
legs, flanges and plates.
3) Bond Behavior and Flexural Behavior in Structural Level, Member
Level and Material Level
The transfer of stress between steel and concrete is governed by the bond reinforcing
both the materials. Bond at the member level and at the localized stress field is different.
When one classification tends to the total impact of rebar pull-out causing an unreasonable
revolution at pillar section joints or breaks shaped in the straining zone. The other
classification addresses the micro-structure which crushes the steel lug edge (Spacone et al,
1996).
The bond requires development length and if the bond deteriorates the reinforcing
bars may most likely slip which would cause a failure in the steel-concrete composite action.
This may lead to a brittle failure due to an impulse or an earthquake (Ngo et al., 2007).
Spacing, rebar placement, anchorage and member width affect the bond strength and causes
cracking failure in concrete structures. Research demonstrated that the bond strength declines
as the bar width increments and that there is a notable effect on the bond due to increase in
usual stress around the bar caused by confinement (Esfahani et al., 2007).
Thus, a bond has various capacities including strength and ductility and is influenced
by many variables like positioning, rebar spacing, packaging and concrete cover. Contingent
upon the maker, certain bars have ribs at a point which impacts pull-out and bond behaviour.
Demonstration indicate that the straight pull-out is reduces under static loading and increases
under dynamic loading for similar designs (Usmani et al., 2001). Cyclic stacking causes
rotation in the vicinity of strain and pressure at a similar part area and consequently requires
the examination of inversion of part constraints registered from a static investigation.
The performance in the member level drops due to the combined effects of localized
bond slip. Specifically, shaft section joints would experience extra pivot past the level caught
by direct flexible examination. At the point when the strengthening steel begins to move in
the other to tension, therefore the compression side is subjected to flexural buckling (Aydin,
2009). But the member cannot buckle in the plane of loading as both the compression side
and tension side are connected to each other. So the buckling occurs in the lateral direction
which is opposed by the tension side which rotates the member. Flexural compressive stress
changes with the length of the member and is predominant in compressive members like local
legs, flanges and plates.
3) Bond Behavior and Flexural Behavior in Structural Level, Member
Level and Material Level
The transfer of stress between steel and concrete is governed by the bond reinforcing
both the materials. Bond at the member level and at the localized stress field is different.
When one classification tends to the total impact of rebar pull-out causing an unreasonable
revolution at pillar section joints or breaks shaped in the straining zone. The other
classification addresses the micro-structure which crushes the steel lug edge (Spacone et al,
1996).
The bond requires development length and if the bond deteriorates the reinforcing
bars may most likely slip which would cause a failure in the steel-concrete composite action.
This may lead to a brittle failure due to an impulse or an earthquake (Ngo et al., 2007).
Spacing, rebar placement, anchorage and member width affect the bond strength and causes
cracking failure in concrete structures. Research demonstrated that the bond strength declines
as the bar width increments and that there is a notable effect on the bond due to increase in
usual stress around the bar caused by confinement (Esfahani et al., 2007).
Thus, a bond has various capacities including strength and ductility and is influenced
by many variables like positioning, rebar spacing, packaging and concrete cover. Contingent
upon the maker, certain bars have ribs at a point which impacts pull-out and bond behaviour.
Demonstration indicate that the straight pull-out is reduces under static loading and increases
under dynamic loading for similar designs (Usmani et al., 2001). Cyclic stacking causes
rotation in the vicinity of strain and pressure at a similar part area and consequently requires
the examination of inversion of part constraints registered from a static investigation.
The performance in the member level drops due to the combined effects of localized
bond slip. Specifically, shaft section joints would experience extra pivot past the level caught
by direct flexible examination. At the point when the strengthening steel begins to move in
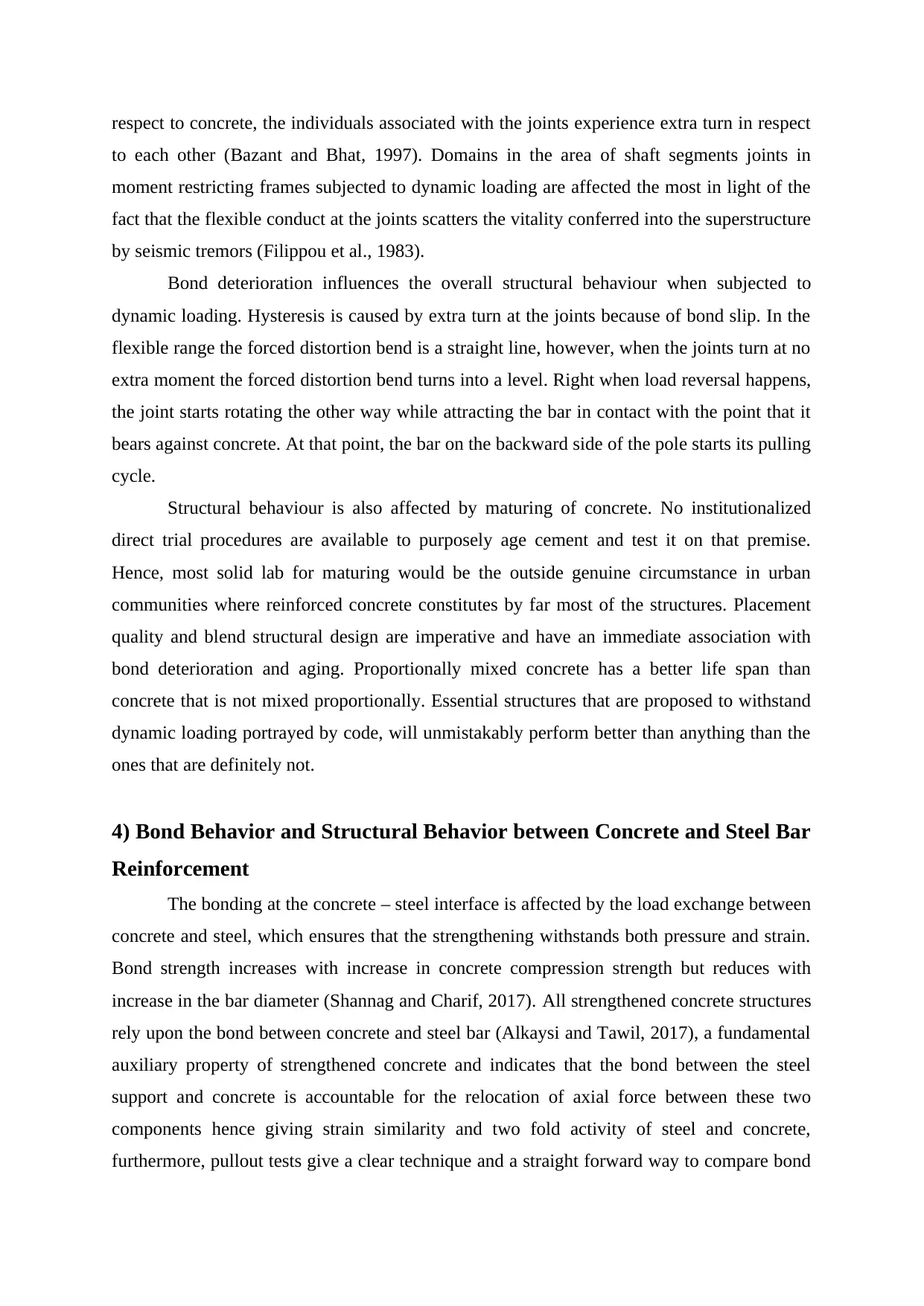
respect to concrete, the individuals associated with the joints experience extra turn in respect
to each other (Bazant and Bhat, 1997). Domains in the area of shaft segments joints in
moment restricting frames subjected to dynamic loading are affected the most in light of the
fact that the flexible conduct at the joints scatters the vitality conferred into the superstructure
by seismic tremors (Filippou et al., 1983).
Bond deterioration influences the overall structural behaviour when subjected to
dynamic loading. Hysteresis is caused by extra turn at the joints because of bond slip. In the
flexible range the forced distortion bend is a straight line, however, when the joints turn at no
extra moment the forced distortion bend turns into a level. Right when load reversal happens,
the joint starts rotating the other way while attracting the bar in contact with the point that it
bears against concrete. At that point, the bar on the backward side of the pole starts its pulling
cycle.
Structural behaviour is also affected by maturing of concrete. No institutionalized
direct trial procedures are available to purposely age cement and test it on that premise.
Hence, most solid lab for maturing would be the outside genuine circumstance in urban
communities where reinforced concrete constitutes by far most of the structures. Placement
quality and blend structural design are imperative and have an immediate association with
bond deterioration and aging. Proportionally mixed concrete has a better life span than
concrete that is not mixed proportionally. Essential structures that are proposed to withstand
dynamic loading portrayed by code, will unmistakably perform better than anything than the
ones that are definitely not.
4) Bond Behavior and Structural Behavior between Concrete and Steel Bar
Reinforcement
The bonding at the concrete – steel interface is affected by the load exchange between
concrete and steel, which ensures that the strengthening withstands both pressure and strain.
Bond strength increases with increase in concrete compression strength but reduces with
increase in the bar diameter (Shannag and Charif, 2017). All strengthened concrete structures
rely upon the bond between concrete and steel bar (Alkaysi and Tawil, 2017), a fundamental
auxiliary property of strengthened concrete and indicates that the bond between the steel
support and concrete is accountable for the relocation of axial force between these two
components hence giving strain similarity and two fold activity of steel and concrete,
furthermore, pullout tests give a clear technique and a straight forward way to compare bond
to each other (Bazant and Bhat, 1997). Domains in the area of shaft segments joints in
moment restricting frames subjected to dynamic loading are affected the most in light of the
fact that the flexible conduct at the joints scatters the vitality conferred into the superstructure
by seismic tremors (Filippou et al., 1983).
Bond deterioration influences the overall structural behaviour when subjected to
dynamic loading. Hysteresis is caused by extra turn at the joints because of bond slip. In the
flexible range the forced distortion bend is a straight line, however, when the joints turn at no
extra moment the forced distortion bend turns into a level. Right when load reversal happens,
the joint starts rotating the other way while attracting the bar in contact with the point that it
bears against concrete. At that point, the bar on the backward side of the pole starts its pulling
cycle.
Structural behaviour is also affected by maturing of concrete. No institutionalized
direct trial procedures are available to purposely age cement and test it on that premise.
Hence, most solid lab for maturing would be the outside genuine circumstance in urban
communities where reinforced concrete constitutes by far most of the structures. Placement
quality and blend structural design are imperative and have an immediate association with
bond deterioration and aging. Proportionally mixed concrete has a better life span than
concrete that is not mixed proportionally. Essential structures that are proposed to withstand
dynamic loading portrayed by code, will unmistakably perform better than anything than the
ones that are definitely not.
4) Bond Behavior and Structural Behavior between Concrete and Steel Bar
Reinforcement
The bonding at the concrete – steel interface is affected by the load exchange between
concrete and steel, which ensures that the strengthening withstands both pressure and strain.
Bond strength increases with increase in concrete compression strength but reduces with
increase in the bar diameter (Shannag and Charif, 2017). All strengthened concrete structures
rely upon the bond between concrete and steel bar (Alkaysi and Tawil, 2017), a fundamental
auxiliary property of strengthened concrete and indicates that the bond between the steel
support and concrete is accountable for the relocation of axial force between these two
components hence giving strain similarity and two fold activity of steel and concrete,
furthermore, pullout tests give a clear technique and a straight forward way to compare bond
Secure Best Marks with AI Grader
Need help grading? Try our AI Grader for instant feedback on your assignments.
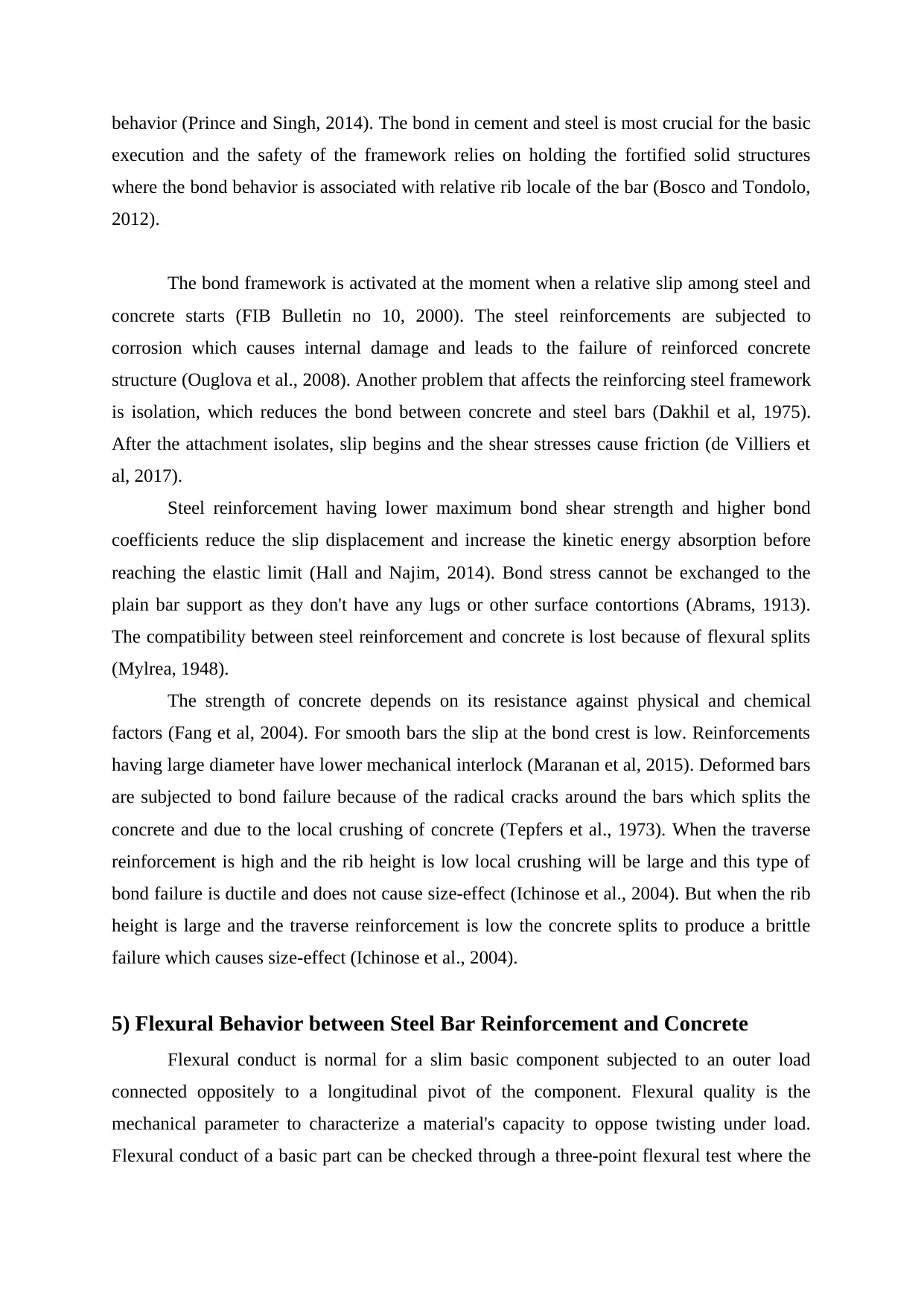
behavior (Prince and Singh, 2014). The bond in cement and steel is most crucial for the basic
execution and the safety of the framework relies on holding the fortified solid structures
where the bond behavior is associated with relative rib locale of the bar (Bosco and Tondolo,
2012).
The bond framework is activated at the moment when a relative slip among steel and
concrete starts (FIB Bulletin no 10, 2000). The steel reinforcements are subjected to
corrosion which causes internal damage and leads to the failure of reinforced concrete
structure (Ouglova et al., 2008). Another problem that affects the reinforcing steel framework
is isolation, which reduces the bond between concrete and steel bars (Dakhil et al, 1975).
After the attachment isolates, slip begins and the shear stresses cause friction (de Villiers et
al, 2017).
Steel reinforcement having lower maximum bond shear strength and higher bond
coefficients reduce the slip displacement and increase the kinetic energy absorption before
reaching the elastic limit (Hall and Najim, 2014). Bond stress cannot be exchanged to the
plain bar support as they don't have any lugs or other surface contortions (Abrams, 1913).
The compatibility between steel reinforcement and concrete is lost because of flexural splits
(Mylrea, 1948).
The strength of concrete depends on its resistance against physical and chemical
factors (Fang et al, 2004). For smooth bars the slip at the bond crest is low. Reinforcements
having large diameter have lower mechanical interlock (Maranan et al, 2015). Deformed bars
are subjected to bond failure because of the radical cracks around the bars which splits the
concrete and due to the local crushing of concrete (Tepfers et al., 1973). When the traverse
reinforcement is high and the rib height is low local crushing will be large and this type of
bond failure is ductile and does not cause size-effect (Ichinose et al., 2004). But when the rib
height is large and the traverse reinforcement is low the concrete splits to produce a brittle
failure which causes size-effect (Ichinose et al., 2004).
5) Flexural Behavior between Steel Bar Reinforcement and Concrete
Flexural conduct is normal for a slim basic component subjected to an outer load
connected oppositely to a longitudinal pivot of the component. Flexural quality is the
mechanical parameter to characterize a material's capacity to oppose twisting under load.
Flexural conduct of a basic part can be checked through a three-point flexural test where the
execution and the safety of the framework relies on holding the fortified solid structures
where the bond behavior is associated with relative rib locale of the bar (Bosco and Tondolo,
2012).
The bond framework is activated at the moment when a relative slip among steel and
concrete starts (FIB Bulletin no 10, 2000). The steel reinforcements are subjected to
corrosion which causes internal damage and leads to the failure of reinforced concrete
structure (Ouglova et al., 2008). Another problem that affects the reinforcing steel framework
is isolation, which reduces the bond between concrete and steel bars (Dakhil et al, 1975).
After the attachment isolates, slip begins and the shear stresses cause friction (de Villiers et
al, 2017).
Steel reinforcement having lower maximum bond shear strength and higher bond
coefficients reduce the slip displacement and increase the kinetic energy absorption before
reaching the elastic limit (Hall and Najim, 2014). Bond stress cannot be exchanged to the
plain bar support as they don't have any lugs or other surface contortions (Abrams, 1913).
The compatibility between steel reinforcement and concrete is lost because of flexural splits
(Mylrea, 1948).
The strength of concrete depends on its resistance against physical and chemical
factors (Fang et al, 2004). For smooth bars the slip at the bond crest is low. Reinforcements
having large diameter have lower mechanical interlock (Maranan et al, 2015). Deformed bars
are subjected to bond failure because of the radical cracks around the bars which splits the
concrete and due to the local crushing of concrete (Tepfers et al., 1973). When the traverse
reinforcement is high and the rib height is low local crushing will be large and this type of
bond failure is ductile and does not cause size-effect (Ichinose et al., 2004). But when the rib
height is large and the traverse reinforcement is low the concrete splits to produce a brittle
failure which causes size-effect (Ichinose et al., 2004).
5) Flexural Behavior between Steel Bar Reinforcement and Concrete
Flexural conduct is normal for a slim basic component subjected to an outer load
connected oppositely to a longitudinal pivot of the component. Flexural quality is the
mechanical parameter to characterize a material's capacity to oppose twisting under load.
Flexural conduct of a basic part can be checked through a three-point flexural test where the
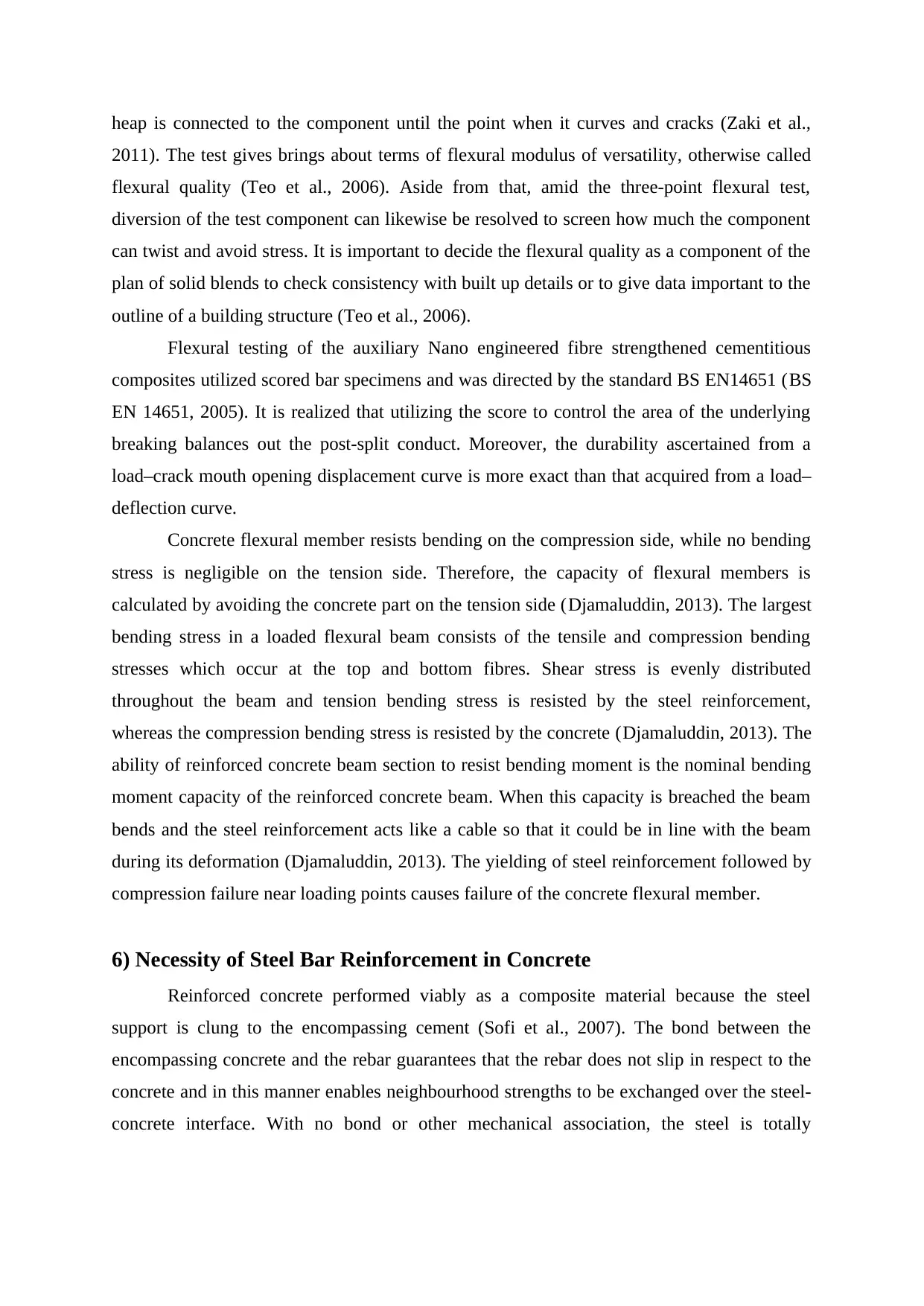
heap is connected to the component until the point when it curves and cracks (Zaki et al.,
2011). The test gives brings about terms of flexural modulus of versatility, otherwise called
flexural quality (Teo et al., 2006). Aside from that, amid the three-point flexural test,
diversion of the test component can likewise be resolved to screen how much the component
can twist and avoid stress. It is important to decide the flexural quality as a component of the
plan of solid blends to check consistency with built up details or to give data important to the
outline of a building structure (Teo et al., 2006).
Flexural testing of the auxiliary Nano engineered fibre strengthened cementitious
composites utilized scored bar specimens and was directed by the standard BS EN14651 (BS
EN 14651, 2005). It is realized that utilizing the score to control the area of the underlying
breaking balances out the post-split conduct. Moreover, the durability ascertained from a
load–crack mouth opening displacement curve is more exact than that acquired from a load–
deflection curve.
Concrete flexural member resists bending on the compression side, while no bending
stress is negligible on the tension side. Therefore, the capacity of flexural members is
calculated by avoiding the concrete part on the tension side (Djamaluddin, 2013). The largest
bending stress in a loaded flexural beam consists of the tensile and compression bending
stresses which occur at the top and bottom fibres. Shear stress is evenly distributed
throughout the beam and tension bending stress is resisted by the steel reinforcement,
whereas the compression bending stress is resisted by the concrete (Djamaluddin, 2013). The
ability of reinforced concrete beam section to resist bending moment is the nominal bending
moment capacity of the reinforced concrete beam. When this capacity is breached the beam
bends and the steel reinforcement acts like a cable so that it could be in line with the beam
during its deformation (Djamaluddin, 2013). The yielding of steel reinforcement followed by
compression failure near loading points causes failure of the concrete flexural member.
6) Necessity of Steel Bar Reinforcement in Concrete
Reinforced concrete performed viably as a composite material because the steel
support is clung to the encompassing cement (Sofi et al., 2007). The bond between the
encompassing concrete and the rebar guarantees that the rebar does not slip in respect to the
concrete and in this manner enables neighbourhood strengths to be exchanged over the steel-
concrete interface. With no bond or other mechanical association, the steel is totally
2011). The test gives brings about terms of flexural modulus of versatility, otherwise called
flexural quality (Teo et al., 2006). Aside from that, amid the three-point flexural test,
diversion of the test component can likewise be resolved to screen how much the component
can twist and avoid stress. It is important to decide the flexural quality as a component of the
plan of solid blends to check consistency with built up details or to give data important to the
outline of a building structure (Teo et al., 2006).
Flexural testing of the auxiliary Nano engineered fibre strengthened cementitious
composites utilized scored bar specimens and was directed by the standard BS EN14651 (BS
EN 14651, 2005). It is realized that utilizing the score to control the area of the underlying
breaking balances out the post-split conduct. Moreover, the durability ascertained from a
load–crack mouth opening displacement curve is more exact than that acquired from a load–
deflection curve.
Concrete flexural member resists bending on the compression side, while no bending
stress is negligible on the tension side. Therefore, the capacity of flexural members is
calculated by avoiding the concrete part on the tension side (Djamaluddin, 2013). The largest
bending stress in a loaded flexural beam consists of the tensile and compression bending
stresses which occur at the top and bottom fibres. Shear stress is evenly distributed
throughout the beam and tension bending stress is resisted by the steel reinforcement,
whereas the compression bending stress is resisted by the concrete (Djamaluddin, 2013). The
ability of reinforced concrete beam section to resist bending moment is the nominal bending
moment capacity of the reinforced concrete beam. When this capacity is breached the beam
bends and the steel reinforcement acts like a cable so that it could be in line with the beam
during its deformation (Djamaluddin, 2013). The yielding of steel reinforcement followed by
compression failure near loading points causes failure of the concrete flexural member.
6) Necessity of Steel Bar Reinforcement in Concrete
Reinforced concrete performed viably as a composite material because the steel
support is clung to the encompassing cement (Sofi et al., 2007). The bond between the
encompassing concrete and the rebar guarantees that the rebar does not slip in respect to the
concrete and in this manner enables neighbourhood strengths to be exchanged over the steel-
concrete interface. With no bond or other mechanical association, the steel is totally
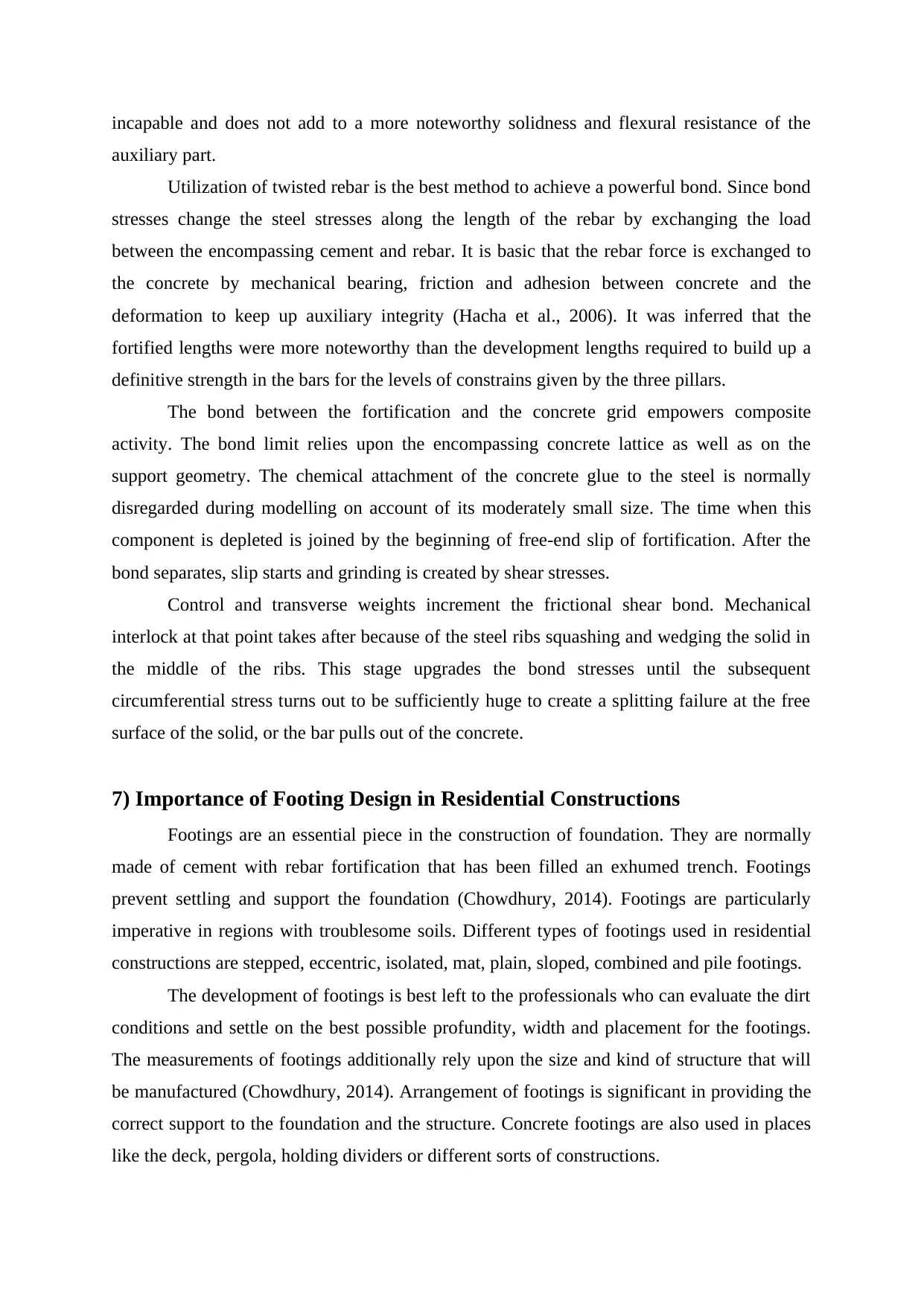
incapable and does not add to a more noteworthy solidness and flexural resistance of the
auxiliary part.
Utilization of twisted rebar is the best method to achieve a powerful bond. Since bond
stresses change the steel stresses along the length of the rebar by exchanging the load
between the encompassing cement and rebar. It is basic that the rebar force is exchanged to
the concrete by mechanical bearing, friction and adhesion between concrete and the
deformation to keep up auxiliary integrity (Hacha et al., 2006). It was inferred that the
fortified lengths were more noteworthy than the development lengths required to build up a
definitive strength in the bars for the levels of constrains given by the three pillars.
The bond between the fortification and the concrete grid empowers composite
activity. The bond limit relies upon the encompassing concrete lattice as well as on the
support geometry. The chemical attachment of the concrete glue to the steel is normally
disregarded during modelling on account of its moderately small size. The time when this
component is depleted is joined by the beginning of free-end slip of fortification. After the
bond separates, slip starts and grinding is created by shear stresses.
Control and transverse weights increment the frictional shear bond. Mechanical
interlock at that point takes after because of the steel ribs squashing and wedging the solid in
the middle of the ribs. This stage upgrades the bond stresses until the subsequent
circumferential stress turns out to be sufficiently huge to create a splitting failure at the free
surface of the solid, or the bar pulls out of the concrete.
7) Importance of Footing Design in Residential Constructions
Footings are an essential piece in the construction of foundation. They are normally
made of cement with rebar fortification that has been filled an exhumed trench. Footings
prevent settling and support the foundation (Chowdhury, 2014). Footings are particularly
imperative in regions with troublesome soils. Different types of footings used in residential
constructions are stepped, eccentric, isolated, mat, plain, sloped, combined and pile footings.
The development of footings is best left to the professionals who can evaluate the dirt
conditions and settle on the best possible profundity, width and placement for the footings.
The measurements of footings additionally rely upon the size and kind of structure that will
be manufactured (Chowdhury, 2014). Arrangement of footings is significant in providing the
correct support to the foundation and the structure. Concrete footings are also used in places
like the deck, pergola, holding dividers or different sorts of constructions.
auxiliary part.
Utilization of twisted rebar is the best method to achieve a powerful bond. Since bond
stresses change the steel stresses along the length of the rebar by exchanging the load
between the encompassing cement and rebar. It is basic that the rebar force is exchanged to
the concrete by mechanical bearing, friction and adhesion between concrete and the
deformation to keep up auxiliary integrity (Hacha et al., 2006). It was inferred that the
fortified lengths were more noteworthy than the development lengths required to build up a
definitive strength in the bars for the levels of constrains given by the three pillars.
The bond between the fortification and the concrete grid empowers composite
activity. The bond limit relies upon the encompassing concrete lattice as well as on the
support geometry. The chemical attachment of the concrete glue to the steel is normally
disregarded during modelling on account of its moderately small size. The time when this
component is depleted is joined by the beginning of free-end slip of fortification. After the
bond separates, slip starts and grinding is created by shear stresses.
Control and transverse weights increment the frictional shear bond. Mechanical
interlock at that point takes after because of the steel ribs squashing and wedging the solid in
the middle of the ribs. This stage upgrades the bond stresses until the subsequent
circumferential stress turns out to be sufficiently huge to create a splitting failure at the free
surface of the solid, or the bar pulls out of the concrete.
7) Importance of Footing Design in Residential Constructions
Footings are an essential piece in the construction of foundation. They are normally
made of cement with rebar fortification that has been filled an exhumed trench. Footings
prevent settling and support the foundation (Chowdhury, 2014). Footings are particularly
imperative in regions with troublesome soils. Different types of footings used in residential
constructions are stepped, eccentric, isolated, mat, plain, sloped, combined and pile footings.
The development of footings is best left to the professionals who can evaluate the dirt
conditions and settle on the best possible profundity, width and placement for the footings.
The measurements of footings additionally rely upon the size and kind of structure that will
be manufactured (Chowdhury, 2014). Arrangement of footings is significant in providing the
correct support to the foundation and the structure. Concrete footings are also used in places
like the deck, pergola, holding dividers or different sorts of constructions.
Paraphrase This Document
Need a fresh take? Get an instant paraphrase of this document with our AI Paraphraser
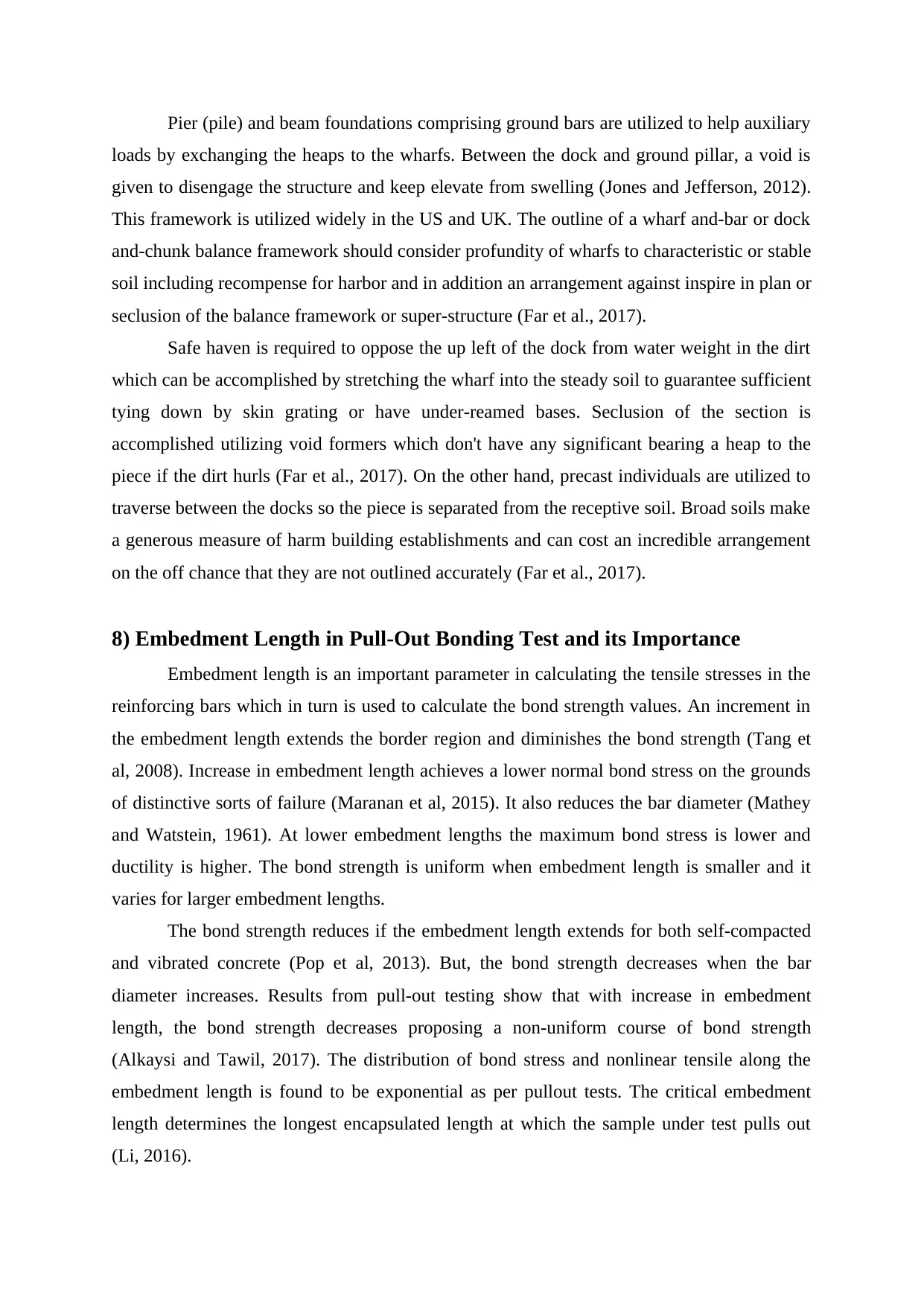
Pier (pile) and beam foundations comprising ground bars are utilized to help auxiliary
loads by exchanging the heaps to the wharfs. Between the dock and ground pillar, a void is
given to disengage the structure and keep elevate from swelling (Jones and Jefferson, 2012).
This framework is utilized widely in the US and UK. The outline of a wharf and-bar or dock
and-chunk balance framework should consider profundity of wharfs to characteristic or stable
soil including recompense for harbor and in addition an arrangement against inspire in plan or
seclusion of the balance framework or super-structure (Far et al., 2017).
Safe haven is required to oppose the up left of the dock from water weight in the dirt
which can be accomplished by stretching the wharf into the steady soil to guarantee sufficient
tying down by skin grating or have under-reamed bases. Seclusion of the section is
accomplished utilizing void formers which don't have any significant bearing a heap to the
piece if the dirt hurls (Far et al., 2017). On the other hand, precast individuals are utilized to
traverse between the docks so the piece is separated from the receptive soil. Broad soils make
a generous measure of harm building establishments and can cost an incredible arrangement
on the off chance that they are not outlined accurately (Far et al., 2017).
8) Embedment Length in Pull-Out Bonding Test and its Importance
Embedment length is an important parameter in calculating the tensile stresses in the
reinforcing bars which in turn is used to calculate the bond strength values. An increment in
the embedment length extends the border region and diminishes the bond strength (Tang et
al, 2008). Increase in embedment length achieves a lower normal bond stress on the grounds
of distinctive sorts of failure (Maranan et al, 2015). It also reduces the bar diameter (Mathey
and Watstein, 1961). At lower embedment lengths the maximum bond stress is lower and
ductility is higher. The bond strength is uniform when embedment length is smaller and it
varies for larger embedment lengths.
The bond strength reduces if the embedment length extends for both self-compacted
and vibrated concrete (Pop et al, 2013). But, the bond strength decreases when the bar
diameter increases. Results from pull-out testing show that with increase in embedment
length, the bond strength decreases proposing a non-uniform course of bond strength
(Alkaysi and Tawil, 2017). The distribution of bond stress and nonlinear tensile along the
embedment length is found to be exponential as per pullout tests. The critical embedment
length determines the longest encapsulated length at which the sample under test pulls out
(Li, 2016).
loads by exchanging the heaps to the wharfs. Between the dock and ground pillar, a void is
given to disengage the structure and keep elevate from swelling (Jones and Jefferson, 2012).
This framework is utilized widely in the US and UK. The outline of a wharf and-bar or dock
and-chunk balance framework should consider profundity of wharfs to characteristic or stable
soil including recompense for harbor and in addition an arrangement against inspire in plan or
seclusion of the balance framework or super-structure (Far et al., 2017).
Safe haven is required to oppose the up left of the dock from water weight in the dirt
which can be accomplished by stretching the wharf into the steady soil to guarantee sufficient
tying down by skin grating or have under-reamed bases. Seclusion of the section is
accomplished utilizing void formers which don't have any significant bearing a heap to the
piece if the dirt hurls (Far et al., 2017). On the other hand, precast individuals are utilized to
traverse between the docks so the piece is separated from the receptive soil. Broad soils make
a generous measure of harm building establishments and can cost an incredible arrangement
on the off chance that they are not outlined accurately (Far et al., 2017).
8) Embedment Length in Pull-Out Bonding Test and its Importance
Embedment length is an important parameter in calculating the tensile stresses in the
reinforcing bars which in turn is used to calculate the bond strength values. An increment in
the embedment length extends the border region and diminishes the bond strength (Tang et
al, 2008). Increase in embedment length achieves a lower normal bond stress on the grounds
of distinctive sorts of failure (Maranan et al, 2015). It also reduces the bar diameter (Mathey
and Watstein, 1961). At lower embedment lengths the maximum bond stress is lower and
ductility is higher. The bond strength is uniform when embedment length is smaller and it
varies for larger embedment lengths.
The bond strength reduces if the embedment length extends for both self-compacted
and vibrated concrete (Pop et al, 2013). But, the bond strength decreases when the bar
diameter increases. Results from pull-out testing show that with increase in embedment
length, the bond strength decreases proposing a non-uniform course of bond strength
(Alkaysi and Tawil, 2017). The distribution of bond stress and nonlinear tensile along the
embedment length is found to be exponential as per pullout tests. The critical embedment
length determines the longest encapsulated length at which the sample under test pulls out
(Li, 2016).
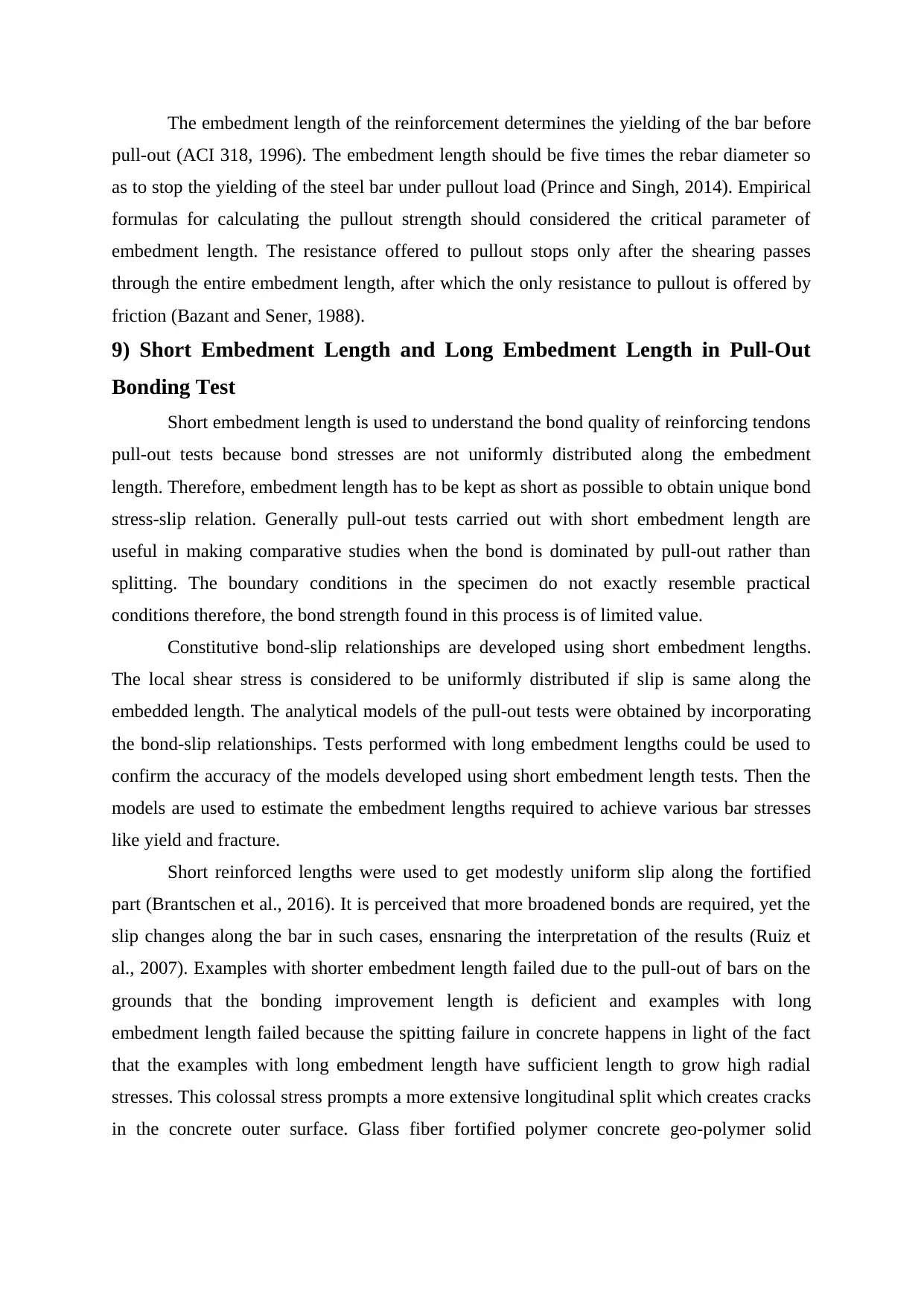
The embedment length of the reinforcement determines the yielding of the bar before
pull-out (ACI 318, 1996). The embedment length should be five times the rebar diameter so
as to stop the yielding of the steel bar under pullout load (Prince and Singh, 2014). Empirical
formulas for calculating the pullout strength should considered the critical parameter of
embedment length. The resistance offered to pullout stops only after the shearing passes
through the entire embedment length, after which the only resistance to pullout is offered by
friction (Bazant and Sener, 1988).
9) Short Embedment Length and Long Embedment Length in Pull-Out
Bonding Test
Short embedment length is used to understand the bond quality of reinforcing tendons
pull-out tests because bond stresses are not uniformly distributed along the embedment
length. Therefore, embedment length has to be kept as short as possible to obtain unique bond
stress-slip relation. Generally pull-out tests carried out with short embedment length are
useful in making comparative studies when the bond is dominated by pull-out rather than
splitting. The boundary conditions in the specimen do not exactly resemble practical
conditions therefore, the bond strength found in this process is of limited value.
Constitutive bond-slip relationships are developed using short embedment lengths.
The local shear stress is considered to be uniformly distributed if slip is same along the
embedded length. The analytical models of the pull-out tests were obtained by incorporating
the bond-slip relationships. Tests performed with long embedment lengths could be used to
confirm the accuracy of the models developed using short embedment length tests. Then the
models are used to estimate the embedment lengths required to achieve various bar stresses
like yield and fracture.
Short reinforced lengths were used to get modestly uniform slip along the fortified
part (Brantschen et al., 2016). It is perceived that more broadened bonds are required, yet the
slip changes along the bar in such cases, ensnaring the interpretation of the results (Ruiz et
al., 2007). Examples with shorter embedment length failed due to the pull-out of bars on the
grounds that the bonding improvement length is deficient and examples with long
embedment length failed because the spitting failure in concrete happens in light of the fact
that the examples with long embedment length have sufficient length to grow high radial
stresses. This colossal stress prompts a more extensive longitudinal split which creates cracks
in the concrete outer surface. Glass fiber fortified polymer concrete geo-polymer solid
pull-out (ACI 318, 1996). The embedment length should be five times the rebar diameter so
as to stop the yielding of the steel bar under pullout load (Prince and Singh, 2014). Empirical
formulas for calculating the pullout strength should considered the critical parameter of
embedment length. The resistance offered to pullout stops only after the shearing passes
through the entire embedment length, after which the only resistance to pullout is offered by
friction (Bazant and Sener, 1988).
9) Short Embedment Length and Long Embedment Length in Pull-Out
Bonding Test
Short embedment length is used to understand the bond quality of reinforcing tendons
pull-out tests because bond stresses are not uniformly distributed along the embedment
length. Therefore, embedment length has to be kept as short as possible to obtain unique bond
stress-slip relation. Generally pull-out tests carried out with short embedment length are
useful in making comparative studies when the bond is dominated by pull-out rather than
splitting. The boundary conditions in the specimen do not exactly resemble practical
conditions therefore, the bond strength found in this process is of limited value.
Constitutive bond-slip relationships are developed using short embedment lengths.
The local shear stress is considered to be uniformly distributed if slip is same along the
embedded length. The analytical models of the pull-out tests were obtained by incorporating
the bond-slip relationships. Tests performed with long embedment lengths could be used to
confirm the accuracy of the models developed using short embedment length tests. Then the
models are used to estimate the embedment lengths required to achieve various bar stresses
like yield and fracture.
Short reinforced lengths were used to get modestly uniform slip along the fortified
part (Brantschen et al., 2016). It is perceived that more broadened bonds are required, yet the
slip changes along the bar in such cases, ensnaring the interpretation of the results (Ruiz et
al., 2007). Examples with shorter embedment length failed due to the pull-out of bars on the
grounds that the bonding improvement length is deficient and examples with long
embedment length failed because the spitting failure in concrete happens in light of the fact
that the examples with long embedment length have sufficient length to grow high radial
stresses. This colossal stress prompts a more extensive longitudinal split which creates cracks
in the concrete outer surface. Glass fiber fortified polymer concrete geo-polymer solid
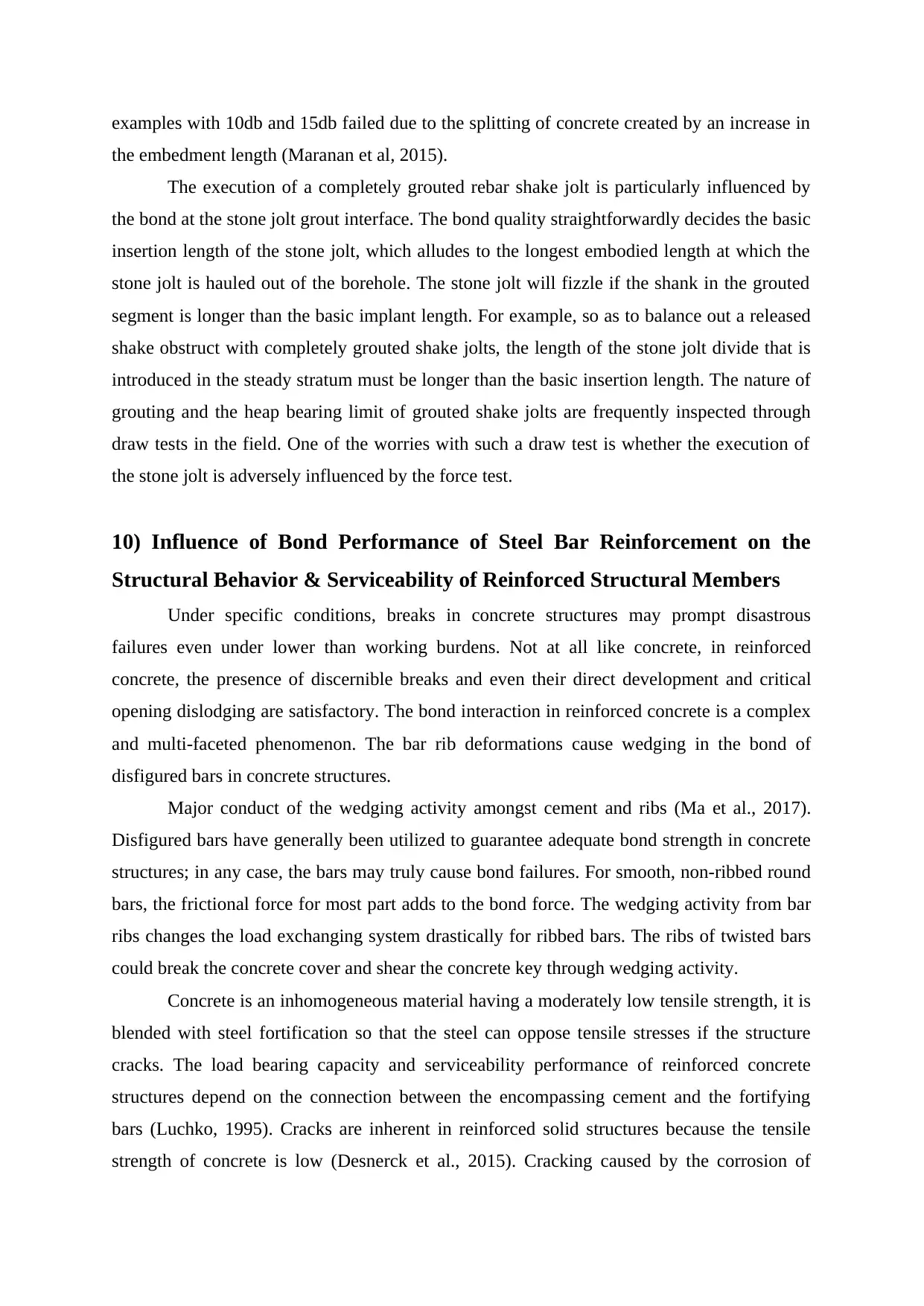
examples with 10db and 15db failed due to the splitting of concrete created by an increase in
the embedment length (Maranan et al, 2015).
The execution of a completely grouted rebar shake jolt is particularly influenced by
the bond at the stone jolt grout interface. The bond quality straightforwardly decides the basic
insertion length of the stone jolt, which alludes to the longest embodied length at which the
stone jolt is hauled out of the borehole. The stone jolt will fizzle if the shank in the grouted
segment is longer than the basic implant length. For example, so as to balance out a released
shake obstruct with completely grouted shake jolts, the length of the stone jolt divide that is
introduced in the steady stratum must be longer than the basic insertion length. The nature of
grouting and the heap bearing limit of grouted shake jolts are frequently inspected through
draw tests in the field. One of the worries with such a draw test is whether the execution of
the stone jolt is adversely influenced by the force test.
10) Influence of Bond Performance of Steel Bar Reinforcement on the
Structural Behavior & Serviceability of Reinforced Structural Members
Under specific conditions, breaks in concrete structures may prompt disastrous
failures even under lower than working burdens. Not at all like concrete, in reinforced
concrete, the presence of discernible breaks and even their direct development and critical
opening dislodging are satisfactory. The bond interaction in reinforced concrete is a complex
and multi-faceted phenomenon. The bar rib deformations cause wedging in the bond of
disfigured bars in concrete structures.
Major conduct of the wedging activity amongst cement and ribs (Ma et al., 2017).
Disfigured bars have generally been utilized to guarantee adequate bond strength in concrete
structures; in any case, the bars may truly cause bond failures. For smooth, non-ribbed round
bars, the frictional force for most part adds to the bond force. The wedging activity from bar
ribs changes the load exchanging system drastically for ribbed bars. The ribs of twisted bars
could break the concrete cover and shear the concrete key through wedging activity.
Concrete is an inhomogeneous material having a moderately low tensile strength, it is
blended with steel fortification so that the steel can oppose tensile stresses if the structure
cracks. The load bearing capacity and serviceability performance of reinforced concrete
structures depend on the connection between the encompassing cement and the fortifying
bars (Luchko, 1995). Cracks are inherent in reinforced solid structures because the tensile
strength of concrete is low (Desnerck et al., 2015). Cracking caused by the corrosion of
the embedment length (Maranan et al, 2015).
The execution of a completely grouted rebar shake jolt is particularly influenced by
the bond at the stone jolt grout interface. The bond quality straightforwardly decides the basic
insertion length of the stone jolt, which alludes to the longest embodied length at which the
stone jolt is hauled out of the borehole. The stone jolt will fizzle if the shank in the grouted
segment is longer than the basic implant length. For example, so as to balance out a released
shake obstruct with completely grouted shake jolts, the length of the stone jolt divide that is
introduced in the steady stratum must be longer than the basic insertion length. The nature of
grouting and the heap bearing limit of grouted shake jolts are frequently inspected through
draw tests in the field. One of the worries with such a draw test is whether the execution of
the stone jolt is adversely influenced by the force test.
10) Influence of Bond Performance of Steel Bar Reinforcement on the
Structural Behavior & Serviceability of Reinforced Structural Members
Under specific conditions, breaks in concrete structures may prompt disastrous
failures even under lower than working burdens. Not at all like concrete, in reinforced
concrete, the presence of discernible breaks and even their direct development and critical
opening dislodging are satisfactory. The bond interaction in reinforced concrete is a complex
and multi-faceted phenomenon. The bar rib deformations cause wedging in the bond of
disfigured bars in concrete structures.
Major conduct of the wedging activity amongst cement and ribs (Ma et al., 2017).
Disfigured bars have generally been utilized to guarantee adequate bond strength in concrete
structures; in any case, the bars may truly cause bond failures. For smooth, non-ribbed round
bars, the frictional force for most part adds to the bond force. The wedging activity from bar
ribs changes the load exchanging system drastically for ribbed bars. The ribs of twisted bars
could break the concrete cover and shear the concrete key through wedging activity.
Concrete is an inhomogeneous material having a moderately low tensile strength, it is
blended with steel fortification so that the steel can oppose tensile stresses if the structure
cracks. The load bearing capacity and serviceability performance of reinforced concrete
structures depend on the connection between the encompassing cement and the fortifying
bars (Luchko, 1995). Cracks are inherent in reinforced solid structures because the tensile
strength of concrete is low (Desnerck et al., 2015). Cracking caused by the corrosion of
Secure Best Marks with AI Grader
Need help grading? Try our AI Grader for instant feedback on your assignments.
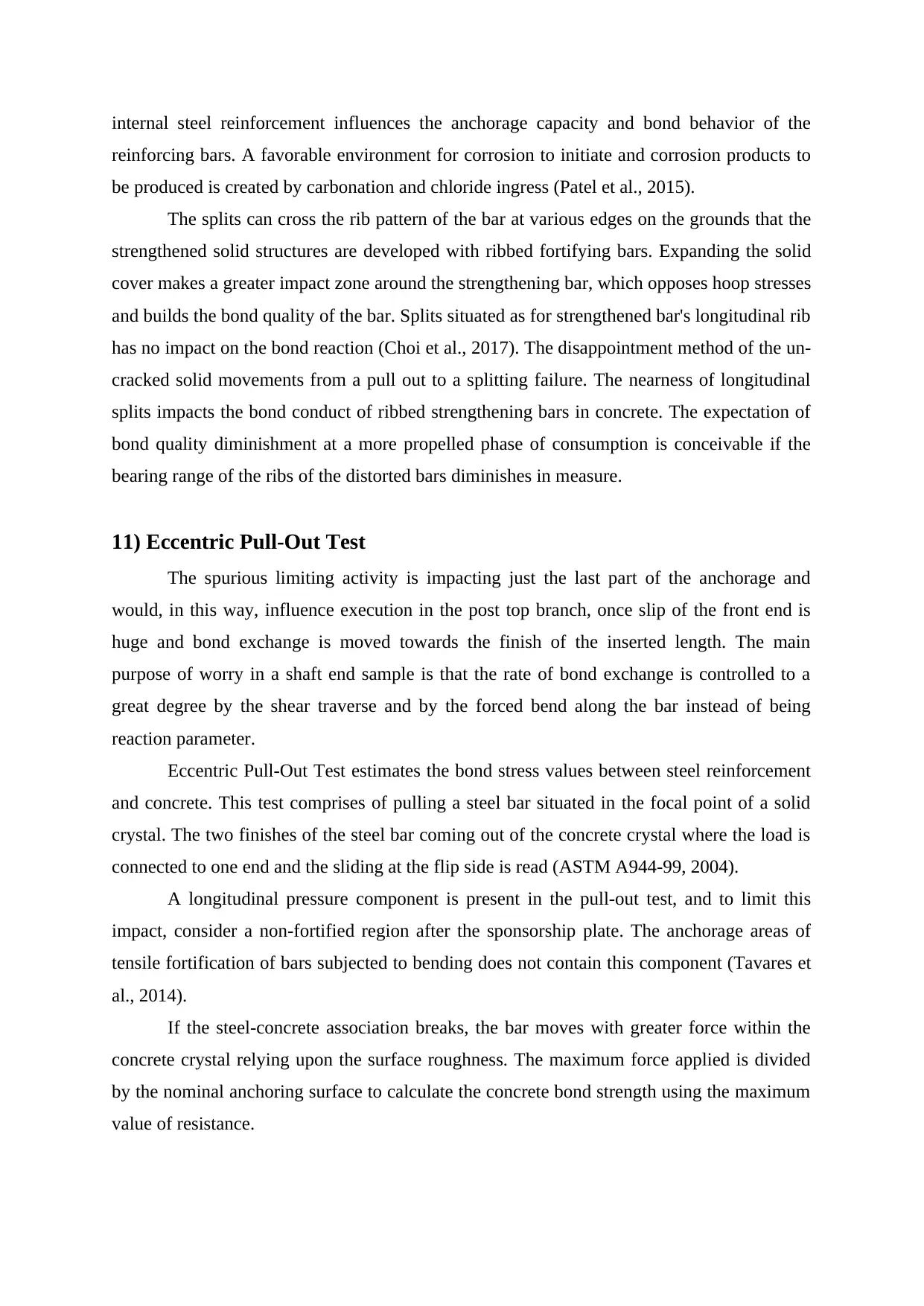
internal steel reinforcement influences the anchorage capacity and bond behavior of the
reinforcing bars. A favorable environment for corrosion to initiate and corrosion products to
be produced is created by carbonation and chloride ingress (Patel et al., 2015).
The splits can cross the rib pattern of the bar at various edges on the grounds that the
strengthened solid structures are developed with ribbed fortifying bars. Expanding the solid
cover makes a greater impact zone around the strengthening bar, which opposes hoop stresses
and builds the bond quality of the bar. Splits situated as for strengthened bar's longitudinal rib
has no impact on the bond reaction (Choi et al., 2017). The disappointment method of the un-
cracked solid movements from a pull out to a splitting failure. The nearness of longitudinal
splits impacts the bond conduct of ribbed strengthening bars in concrete. The expectation of
bond quality diminishment at a more propelled phase of consumption is conceivable if the
bearing range of the ribs of the distorted bars diminishes in measure.
11) Eccentric Pull-Out Test
The spurious limiting activity is impacting just the last part of the anchorage and
would, in this way, influence execution in the post top branch, once slip of the front end is
huge and bond exchange is moved towards the finish of the inserted length. The main
purpose of worry in a shaft end sample is that the rate of bond exchange is controlled to a
great degree by the shear traverse and by the forced bend along the bar instead of being
reaction parameter.
Eccentric Pull-Out Test estimates the bond stress values between steel reinforcement
and concrete. This test comprises of pulling a steel bar situated in the focal point of a solid
crystal. The two finishes of the steel bar coming out of the concrete crystal where the load is
connected to one end and the sliding at the flip side is read (ASTM A944-99, 2004).
A longitudinal pressure component is present in the pull-out test, and to limit this
impact, consider a non-fortified region after the sponsorship plate. The anchorage areas of
tensile fortification of bars subjected to bending does not contain this component (Tavares et
al., 2014).
If the steel-concrete association breaks, the bar moves with greater force within the
concrete crystal relying upon the surface roughness. The maximum force applied is divided
by the nominal anchoring surface to calculate the concrete bond strength using the maximum
value of resistance.
reinforcing bars. A favorable environment for corrosion to initiate and corrosion products to
be produced is created by carbonation and chloride ingress (Patel et al., 2015).
The splits can cross the rib pattern of the bar at various edges on the grounds that the
strengthened solid structures are developed with ribbed fortifying bars. Expanding the solid
cover makes a greater impact zone around the strengthening bar, which opposes hoop stresses
and builds the bond quality of the bar. Splits situated as for strengthened bar's longitudinal rib
has no impact on the bond reaction (Choi et al., 2017). The disappointment method of the un-
cracked solid movements from a pull out to a splitting failure. The nearness of longitudinal
splits impacts the bond conduct of ribbed strengthening bars in concrete. The expectation of
bond quality diminishment at a more propelled phase of consumption is conceivable if the
bearing range of the ribs of the distorted bars diminishes in measure.
11) Eccentric Pull-Out Test
The spurious limiting activity is impacting just the last part of the anchorage and
would, in this way, influence execution in the post top branch, once slip of the front end is
huge and bond exchange is moved towards the finish of the inserted length. The main
purpose of worry in a shaft end sample is that the rate of bond exchange is controlled to a
great degree by the shear traverse and by the forced bend along the bar instead of being
reaction parameter.
Eccentric Pull-Out Test estimates the bond stress values between steel reinforcement
and concrete. This test comprises of pulling a steel bar situated in the focal point of a solid
crystal. The two finishes of the steel bar coming out of the concrete crystal where the load is
connected to one end and the sliding at the flip side is read (ASTM A944-99, 2004).
A longitudinal pressure component is present in the pull-out test, and to limit this
impact, consider a non-fortified region after the sponsorship plate. The anchorage areas of
tensile fortification of bars subjected to bending does not contain this component (Tavares et
al., 2014).
If the steel-concrete association breaks, the bar moves with greater force within the
concrete crystal relying upon the surface roughness. The maximum force applied is divided
by the nominal anchoring surface to calculate the concrete bond strength using the maximum
value of resistance.
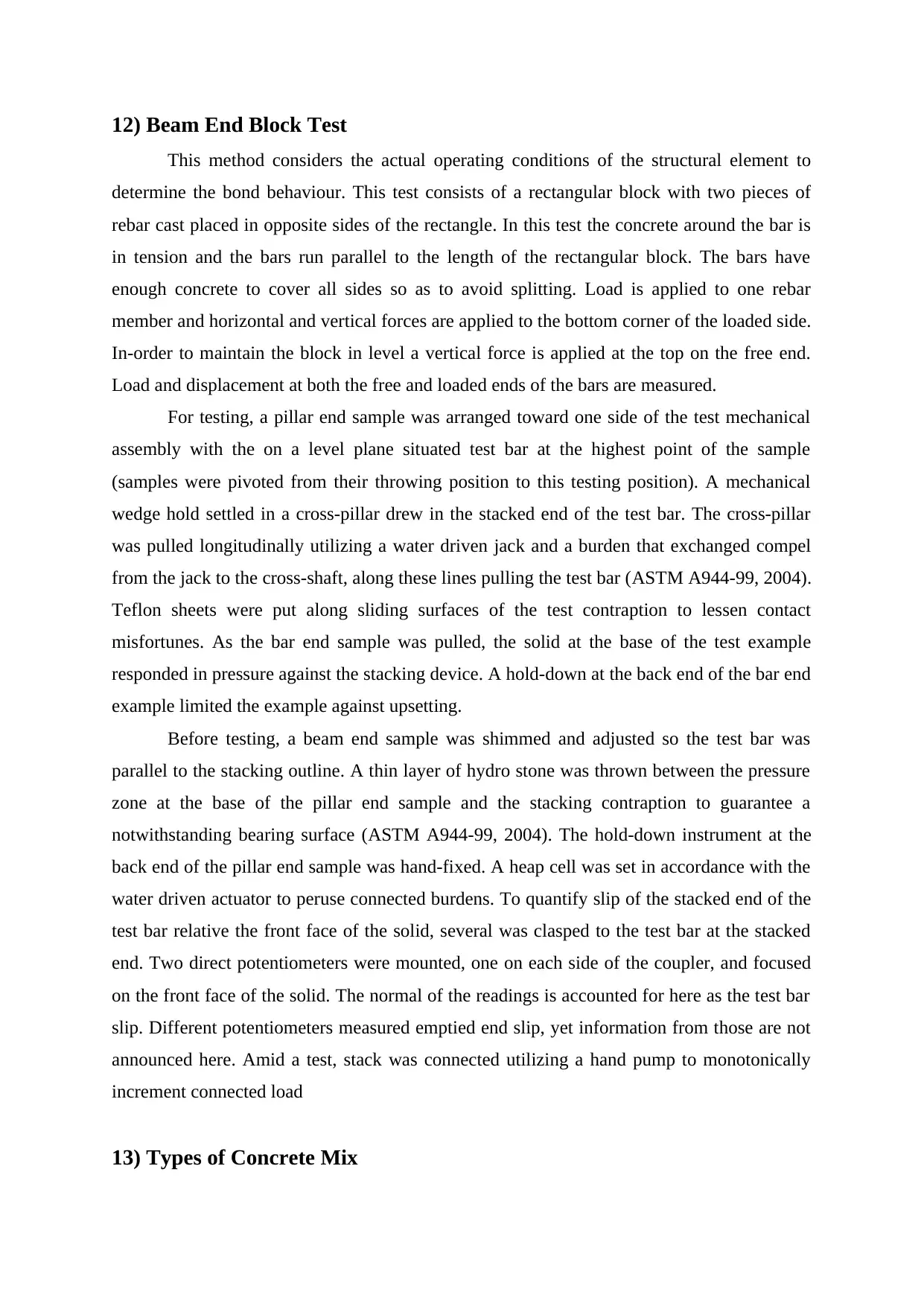
12) Beam End Block Test
This method considers the actual operating conditions of the structural element to
determine the bond behaviour. This test consists of a rectangular block with two pieces of
rebar cast placed in opposite sides of the rectangle. In this test the concrete around the bar is
in tension and the bars run parallel to the length of the rectangular block. The bars have
enough concrete to cover all sides so as to avoid splitting. Load is applied to one rebar
member and horizontal and vertical forces are applied to the bottom corner of the loaded side.
In-order to maintain the block in level a vertical force is applied at the top on the free end.
Load and displacement at both the free and loaded ends of the bars are measured.
For testing, a pillar end sample was arranged toward one side of the test mechanical
assembly with the on a level plane situated test bar at the highest point of the sample
(samples were pivoted from their throwing position to this testing position). A mechanical
wedge hold settled in a cross-pillar drew in the stacked end of the test bar. The cross-pillar
was pulled longitudinally utilizing a water driven jack and a burden that exchanged compel
from the jack to the cross-shaft, along these lines pulling the test bar (ASTM A944-99, 2004).
Teflon sheets were put along sliding surfaces of the test contraption to lessen contact
misfortunes. As the bar end sample was pulled, the solid at the base of the test example
responded in pressure against the stacking device. A hold-down at the back end of the bar end
example limited the example against upsetting.
Before testing, a beam end sample was shimmed and adjusted so the test bar was
parallel to the stacking outline. A thin layer of hydro stone was thrown between the pressure
zone at the base of the pillar end sample and the stacking contraption to guarantee a
notwithstanding bearing surface (ASTM A944-99, 2004). The hold-down instrument at the
back end of the pillar end sample was hand-fixed. A heap cell was set in accordance with the
water driven actuator to peruse connected burdens. To quantify slip of the stacked end of the
test bar relative the front face of the solid, several was clasped to the test bar at the stacked
end. Two direct potentiometers were mounted, one on each side of the coupler, and focused
on the front face of the solid. The normal of the readings is accounted for here as the test bar
slip. Different potentiometers measured emptied end slip, yet information from those are not
announced here. Amid a test, stack was connected utilizing a hand pump to monotonically
increment connected load
13) Types of Concrete Mix
This method considers the actual operating conditions of the structural element to
determine the bond behaviour. This test consists of a rectangular block with two pieces of
rebar cast placed in opposite sides of the rectangle. In this test the concrete around the bar is
in tension and the bars run parallel to the length of the rectangular block. The bars have
enough concrete to cover all sides so as to avoid splitting. Load is applied to one rebar
member and horizontal and vertical forces are applied to the bottom corner of the loaded side.
In-order to maintain the block in level a vertical force is applied at the top on the free end.
Load and displacement at both the free and loaded ends of the bars are measured.
For testing, a pillar end sample was arranged toward one side of the test mechanical
assembly with the on a level plane situated test bar at the highest point of the sample
(samples were pivoted from their throwing position to this testing position). A mechanical
wedge hold settled in a cross-pillar drew in the stacked end of the test bar. The cross-pillar
was pulled longitudinally utilizing a water driven jack and a burden that exchanged compel
from the jack to the cross-shaft, along these lines pulling the test bar (ASTM A944-99, 2004).
Teflon sheets were put along sliding surfaces of the test contraption to lessen contact
misfortunes. As the bar end sample was pulled, the solid at the base of the test example
responded in pressure against the stacking device. A hold-down at the back end of the bar end
example limited the example against upsetting.
Before testing, a beam end sample was shimmed and adjusted so the test bar was
parallel to the stacking outline. A thin layer of hydro stone was thrown between the pressure
zone at the base of the pillar end sample and the stacking contraption to guarantee a
notwithstanding bearing surface (ASTM A944-99, 2004). The hold-down instrument at the
back end of the pillar end sample was hand-fixed. A heap cell was set in accordance with the
water driven actuator to peruse connected burdens. To quantify slip of the stacked end of the
test bar relative the front face of the solid, several was clasped to the test bar at the stacked
end. Two direct potentiometers were mounted, one on each side of the coupler, and focused
on the front face of the solid. The normal of the readings is accounted for here as the test bar
slip. Different potentiometers measured emptied end slip, yet information from those are not
announced here. Amid a test, stack was connected utilizing a hand pump to monotonically
increment connected load
13) Types of Concrete Mix
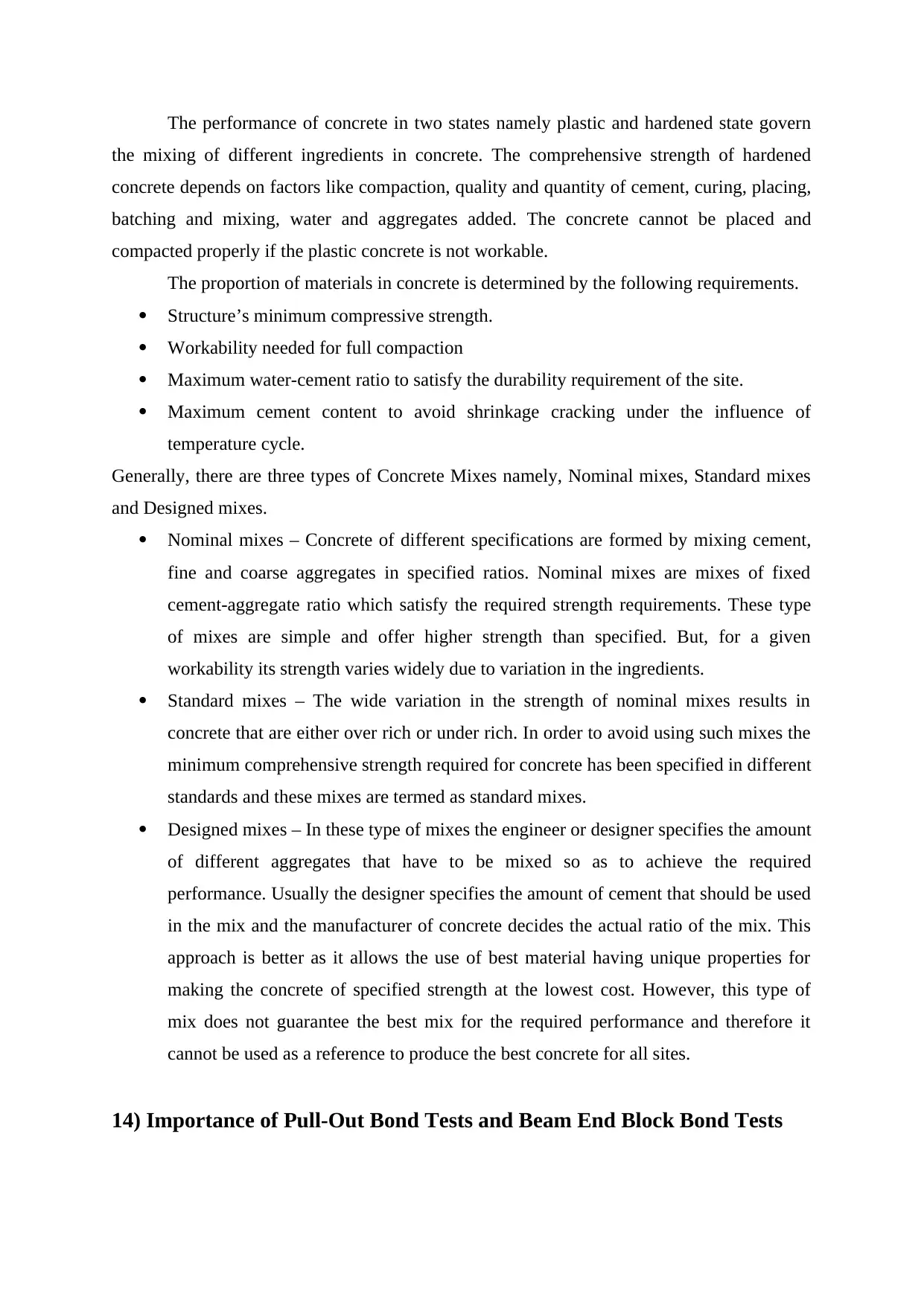
The performance of concrete in two states namely plastic and hardened state govern
the mixing of different ingredients in concrete. The comprehensive strength of hardened
concrete depends on factors like compaction, quality and quantity of cement, curing, placing,
batching and mixing, water and aggregates added. The concrete cannot be placed and
compacted properly if the plastic concrete is not workable.
The proportion of materials in concrete is determined by the following requirements.
Structure’s minimum compressive strength.
Workability needed for full compaction
Maximum water-cement ratio to satisfy the durability requirement of the site.
Maximum cement content to avoid shrinkage cracking under the influence of
temperature cycle.
Generally, there are three types of Concrete Mixes namely, Nominal mixes, Standard mixes
and Designed mixes.
Nominal mixes – Concrete of different specifications are formed by mixing cement,
fine and coarse aggregates in specified ratios. Nominal mixes are mixes of fixed
cement-aggregate ratio which satisfy the required strength requirements. These type
of mixes are simple and offer higher strength than specified. But, for a given
workability its strength varies widely due to variation in the ingredients.
Standard mixes – The wide variation in the strength of nominal mixes results in
concrete that are either over rich or under rich. In order to avoid using such mixes the
minimum comprehensive strength required for concrete has been specified in different
standards and these mixes are termed as standard mixes.
Designed mixes – In these type of mixes the engineer or designer specifies the amount
of different aggregates that have to be mixed so as to achieve the required
performance. Usually the designer specifies the amount of cement that should be used
in the mix and the manufacturer of concrete decides the actual ratio of the mix. This
approach is better as it allows the use of best material having unique properties for
making the concrete of specified strength at the lowest cost. However, this type of
mix does not guarantee the best mix for the required performance and therefore it
cannot be used as a reference to produce the best concrete for all sites.
14) Importance of Pull-Out Bond Tests and Beam End Block Bond Tests
the mixing of different ingredients in concrete. The comprehensive strength of hardened
concrete depends on factors like compaction, quality and quantity of cement, curing, placing,
batching and mixing, water and aggregates added. The concrete cannot be placed and
compacted properly if the plastic concrete is not workable.
The proportion of materials in concrete is determined by the following requirements.
Structure’s minimum compressive strength.
Workability needed for full compaction
Maximum water-cement ratio to satisfy the durability requirement of the site.
Maximum cement content to avoid shrinkage cracking under the influence of
temperature cycle.
Generally, there are three types of Concrete Mixes namely, Nominal mixes, Standard mixes
and Designed mixes.
Nominal mixes – Concrete of different specifications are formed by mixing cement,
fine and coarse aggregates in specified ratios. Nominal mixes are mixes of fixed
cement-aggregate ratio which satisfy the required strength requirements. These type
of mixes are simple and offer higher strength than specified. But, for a given
workability its strength varies widely due to variation in the ingredients.
Standard mixes – The wide variation in the strength of nominal mixes results in
concrete that are either over rich or under rich. In order to avoid using such mixes the
minimum comprehensive strength required for concrete has been specified in different
standards and these mixes are termed as standard mixes.
Designed mixes – In these type of mixes the engineer or designer specifies the amount
of different aggregates that have to be mixed so as to achieve the required
performance. Usually the designer specifies the amount of cement that should be used
in the mix and the manufacturer of concrete decides the actual ratio of the mix. This
approach is better as it allows the use of best material having unique properties for
making the concrete of specified strength at the lowest cost. However, this type of
mix does not guarantee the best mix for the required performance and therefore it
cannot be used as a reference to produce the best concrete for all sites.
14) Importance of Pull-Out Bond Tests and Beam End Block Bond Tests
Paraphrase This Document
Need a fresh take? Get an instant paraphrase of this document with our AI Paraphraser
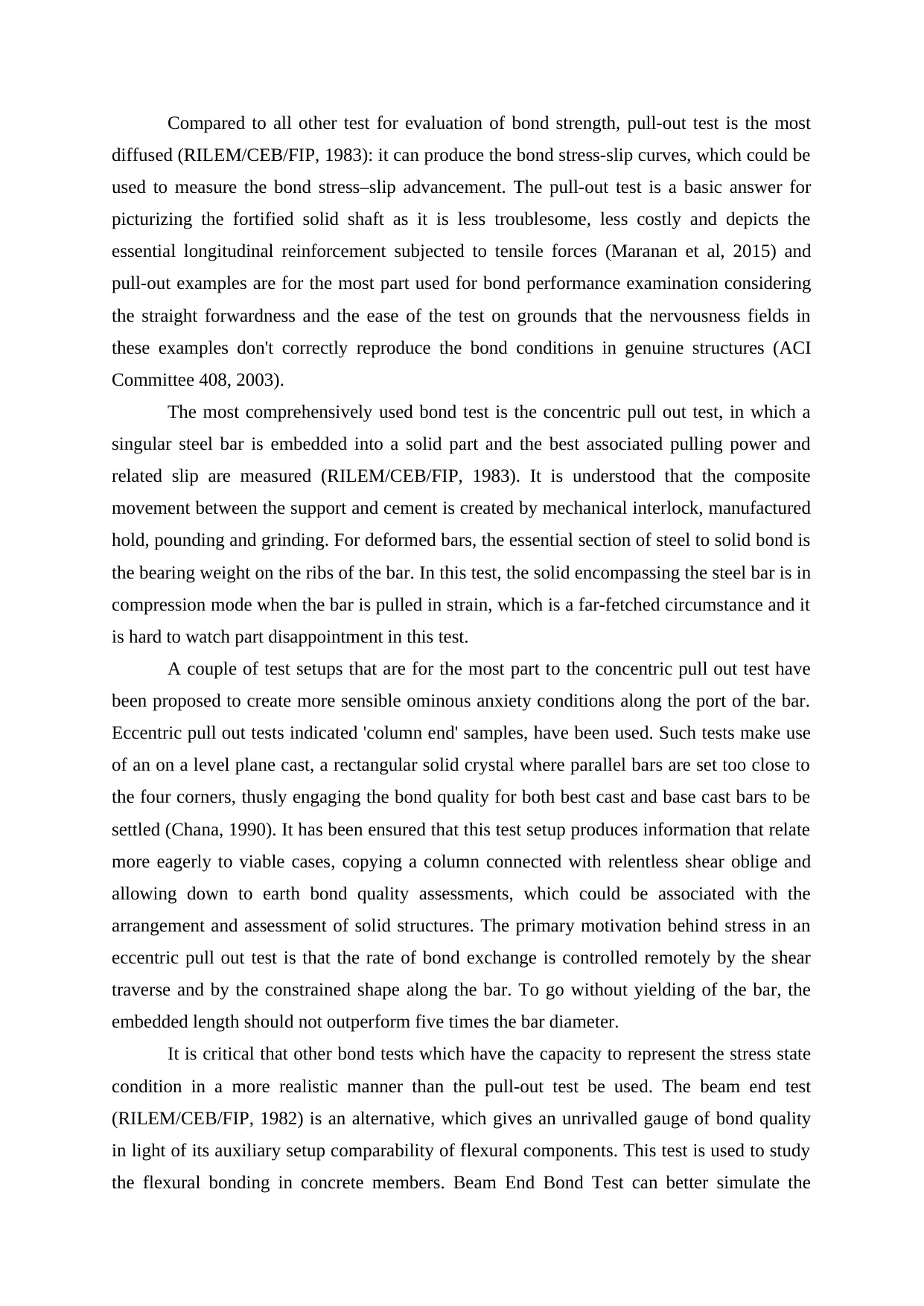
Compared to all other test for evaluation of bond strength, pull-out test is the most
diffused (RILEM/CEB/FIP, 1983): it can produce the bond stress-slip curves, which could be
used to measure the bond stress–slip advancement. The pull-out test is a basic answer for
picturizing the fortified solid shaft as it is less troublesome, less costly and depicts the
essential longitudinal reinforcement subjected to tensile forces (Maranan et al, 2015) and
pull-out examples are for the most part used for bond performance examination considering
the straight forwardness and the ease of the test on grounds that the nervousness fields in
these examples don't correctly reproduce the bond conditions in genuine structures (ACI
Committee 408, 2003).
The most comprehensively used bond test is the concentric pull out test, in which a
singular steel bar is embedded into a solid part and the best associated pulling power and
related slip are measured (RILEM/CEB/FIP, 1983). It is understood that the composite
movement between the support and cement is created by mechanical interlock, manufactured
hold, pounding and grinding. For deformed bars, the essential section of steel to solid bond is
the bearing weight on the ribs of the bar. In this test, the solid encompassing the steel bar is in
compression mode when the bar is pulled in strain, which is a far-fetched circumstance and it
is hard to watch part disappointment in this test.
A couple of test setups that are for the most part to the concentric pull out test have
been proposed to create more sensible ominous anxiety conditions along the port of the bar.
Eccentric pull out tests indicated 'column end' samples, have been used. Such tests make use
of an on a level plane cast, a rectangular solid crystal where parallel bars are set too close to
the four corners, thusly engaging the bond quality for both best cast and base cast bars to be
settled (Chana, 1990). It has been ensured that this test setup produces information that relate
more eagerly to viable cases, copying a column connected with relentless shear oblige and
allowing down to earth bond quality assessments, which could be associated with the
arrangement and assessment of solid structures. The primary motivation behind stress in an
eccentric pull out test is that the rate of bond exchange is controlled remotely by the shear
traverse and by the constrained shape along the bar. To go without yielding of the bar, the
embedded length should not outperform five times the bar diameter.
It is critical that other bond tests which have the capacity to represent the stress state
condition in a more realistic manner than the pull-out test be used. The beam end test
(RILEM/CEB/FIP, 1982) is an alternative, which gives an unrivalled gauge of bond quality
in light of its auxiliary setup comparability of flexural components. This test is used to study
the flexural bonding in concrete members. Beam End Bond Test can better simulate the
diffused (RILEM/CEB/FIP, 1983): it can produce the bond stress-slip curves, which could be
used to measure the bond stress–slip advancement. The pull-out test is a basic answer for
picturizing the fortified solid shaft as it is less troublesome, less costly and depicts the
essential longitudinal reinforcement subjected to tensile forces (Maranan et al, 2015) and
pull-out examples are for the most part used for bond performance examination considering
the straight forwardness and the ease of the test on grounds that the nervousness fields in
these examples don't correctly reproduce the bond conditions in genuine structures (ACI
Committee 408, 2003).
The most comprehensively used bond test is the concentric pull out test, in which a
singular steel bar is embedded into a solid part and the best associated pulling power and
related slip are measured (RILEM/CEB/FIP, 1983). It is understood that the composite
movement between the support and cement is created by mechanical interlock, manufactured
hold, pounding and grinding. For deformed bars, the essential section of steel to solid bond is
the bearing weight on the ribs of the bar. In this test, the solid encompassing the steel bar is in
compression mode when the bar is pulled in strain, which is a far-fetched circumstance and it
is hard to watch part disappointment in this test.
A couple of test setups that are for the most part to the concentric pull out test have
been proposed to create more sensible ominous anxiety conditions along the port of the bar.
Eccentric pull out tests indicated 'column end' samples, have been used. Such tests make use
of an on a level plane cast, a rectangular solid crystal where parallel bars are set too close to
the four corners, thusly engaging the bond quality for both best cast and base cast bars to be
settled (Chana, 1990). It has been ensured that this test setup produces information that relate
more eagerly to viable cases, copying a column connected with relentless shear oblige and
allowing down to earth bond quality assessments, which could be associated with the
arrangement and assessment of solid structures. The primary motivation behind stress in an
eccentric pull out test is that the rate of bond exchange is controlled remotely by the shear
traverse and by the constrained shape along the bar. To go without yielding of the bar, the
embedded length should not outperform five times the bar diameter.
It is critical that other bond tests which have the capacity to represent the stress state
condition in a more realistic manner than the pull-out test be used. The beam end test
(RILEM/CEB/FIP, 1982) is an alternative, which gives an unrivalled gauge of bond quality
in light of its auxiliary setup comparability of flexural components. This test is used to study
the flexural bonding in concrete members. Beam End Bond Test can better simulate the
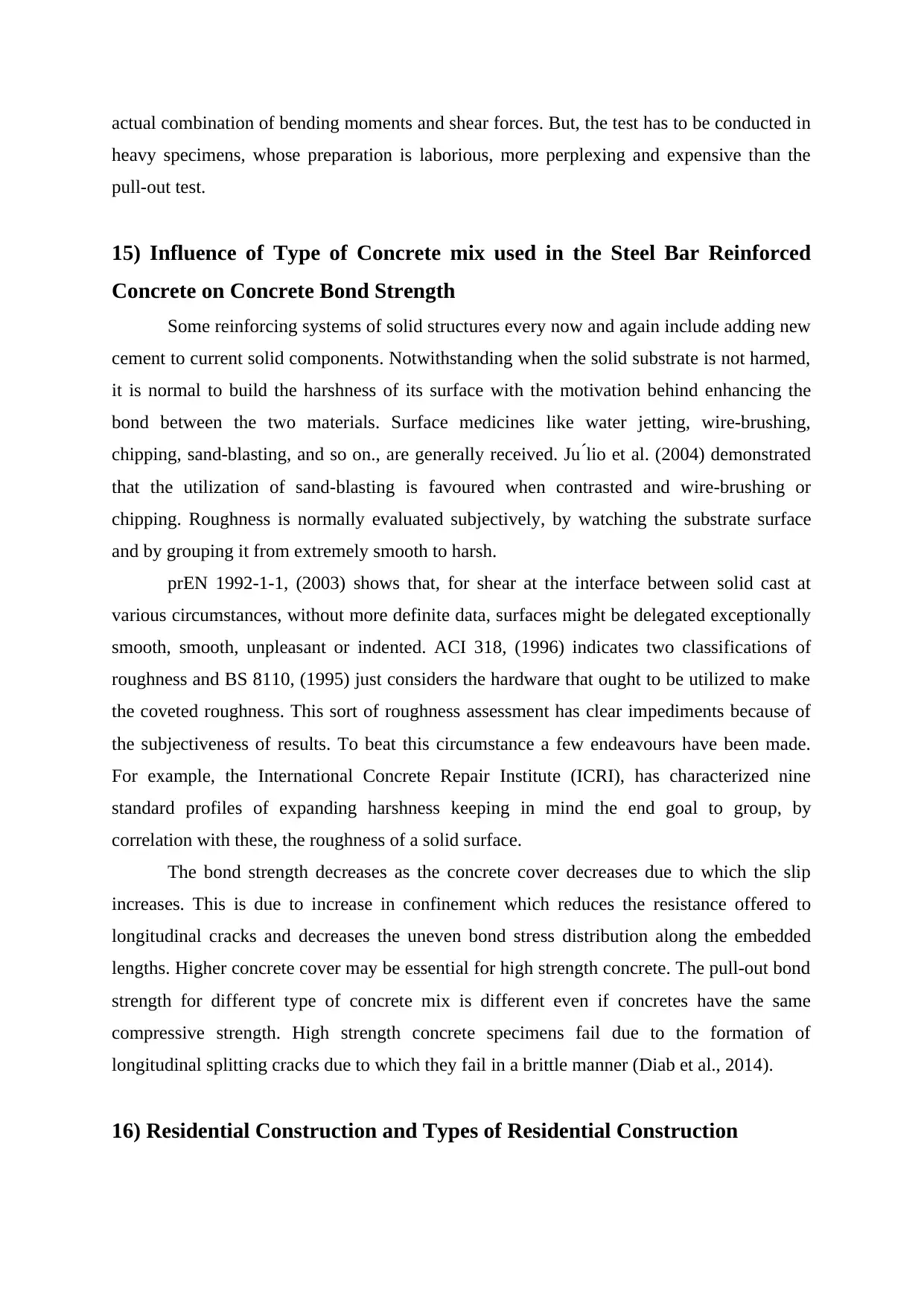
actual combination of bending moments and shear forces. But, the test has to be conducted in
heavy specimens, whose preparation is laborious, more perplexing and expensive than the
pull-out test.
15) Influence of Type of Concrete mix used in the Steel Bar Reinforced
Concrete on Concrete Bond Strength
Some reinforcing systems of solid structures every now and again include adding new
cement to current solid components. Notwithstanding when the solid substrate is not harmed,
it is normal to build the harshness of its surface with the motivation behind enhancing the
bond between the two materials. Surface medicines like water jetting, wire-brushing,
chipping, sand-blasting, and so on., are generally received. Ju ́ lio et al. (2004) demonstrated
that the utilization of sand-blasting is favoured when contrasted and wire-brushing or
chipping. Roughness is normally evaluated subjectively, by watching the substrate surface
and by grouping it from extremely smooth to harsh.
prEN 1992-1-1, (2003) shows that, for shear at the interface between solid cast at
various circumstances, without more definite data, surfaces might be delegated exceptionally
smooth, smooth, unpleasant or indented. ACI 318, (1996) indicates two classifications of
roughness and BS 8110, (1995) just considers the hardware that ought to be utilized to make
the coveted roughness. This sort of roughness assessment has clear impediments because of
the subjectiveness of results. To beat this circumstance a few endeavours have been made.
For example, the International Concrete Repair Institute (ICRI), has characterized nine
standard profiles of expanding harshness keeping in mind the end goal to group, by
correlation with these, the roughness of a solid surface.
The bond strength decreases as the concrete cover decreases due to which the slip
increases. This is due to increase in confinement which reduces the resistance offered to
longitudinal cracks and decreases the uneven bond stress distribution along the embedded
lengths. Higher concrete cover may be essential for high strength concrete. The pull-out bond
strength for different type of concrete mix is different even if concretes have the same
compressive strength. High strength concrete specimens fail due to the formation of
longitudinal splitting cracks due to which they fail in a brittle manner (Diab et al., 2014).
16) Residential Construction and Types of Residential Construction
heavy specimens, whose preparation is laborious, more perplexing and expensive than the
pull-out test.
15) Influence of Type of Concrete mix used in the Steel Bar Reinforced
Concrete on Concrete Bond Strength
Some reinforcing systems of solid structures every now and again include adding new
cement to current solid components. Notwithstanding when the solid substrate is not harmed,
it is normal to build the harshness of its surface with the motivation behind enhancing the
bond between the two materials. Surface medicines like water jetting, wire-brushing,
chipping, sand-blasting, and so on., are generally received. Ju ́ lio et al. (2004) demonstrated
that the utilization of sand-blasting is favoured when contrasted and wire-brushing or
chipping. Roughness is normally evaluated subjectively, by watching the substrate surface
and by grouping it from extremely smooth to harsh.
prEN 1992-1-1, (2003) shows that, for shear at the interface between solid cast at
various circumstances, without more definite data, surfaces might be delegated exceptionally
smooth, smooth, unpleasant or indented. ACI 318, (1996) indicates two classifications of
roughness and BS 8110, (1995) just considers the hardware that ought to be utilized to make
the coveted roughness. This sort of roughness assessment has clear impediments because of
the subjectiveness of results. To beat this circumstance a few endeavours have been made.
For example, the International Concrete Repair Institute (ICRI), has characterized nine
standard profiles of expanding harshness keeping in mind the end goal to group, by
correlation with these, the roughness of a solid surface.
The bond strength decreases as the concrete cover decreases due to which the slip
increases. This is due to increase in confinement which reduces the resistance offered to
longitudinal cracks and decreases the uneven bond stress distribution along the embedded
lengths. Higher concrete cover may be essential for high strength concrete. The pull-out bond
strength for different type of concrete mix is different even if concretes have the same
compressive strength. High strength concrete specimens fail due to the formation of
longitudinal splitting cracks due to which they fail in a brittle manner (Diab et al., 2014).
16) Residential Construction and Types of Residential Construction
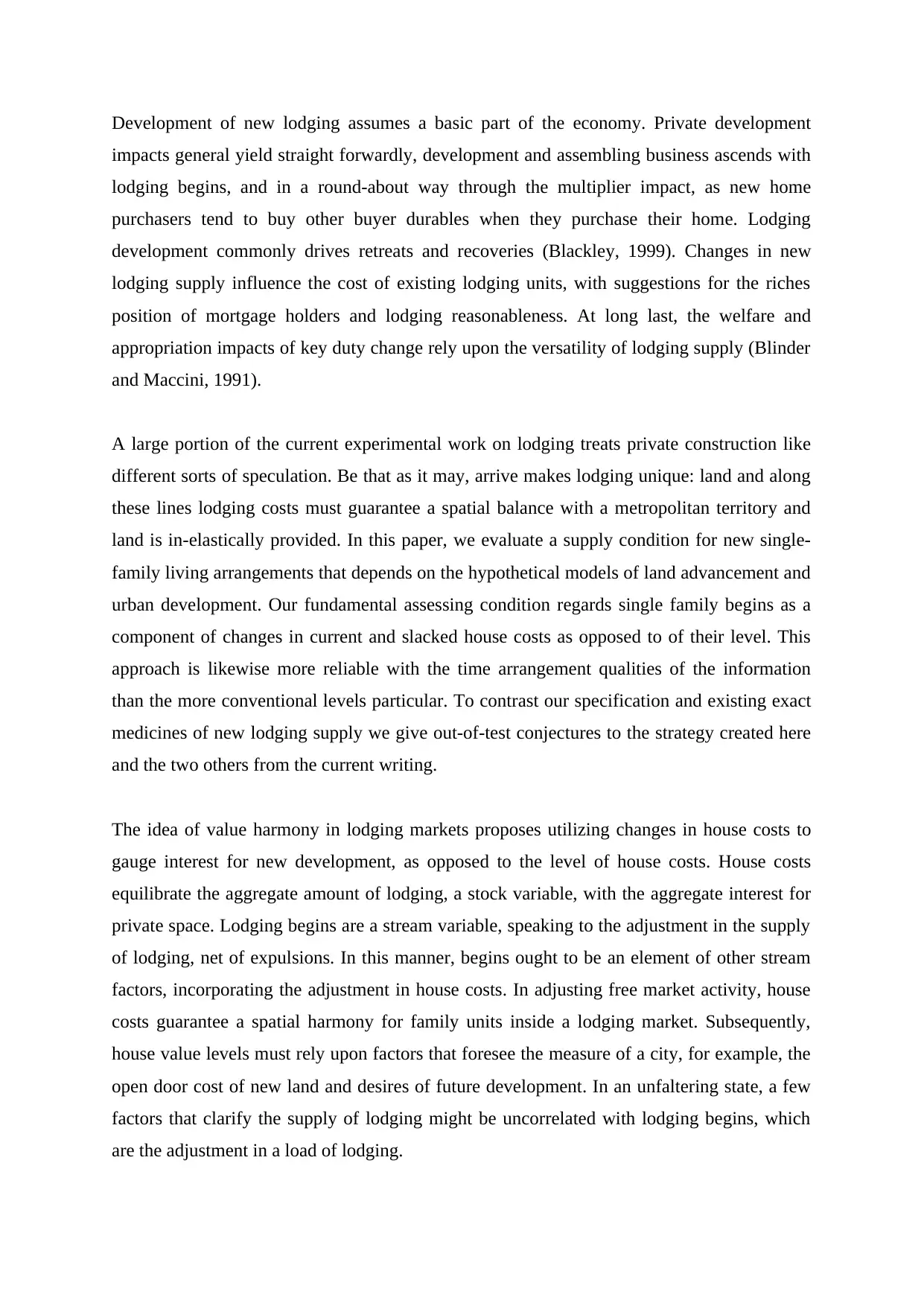
Development of new lodging assumes a basic part of the economy. Private development
impacts general yield straight forwardly, development and assembling business ascends with
lodging begins, and in a round-about way through the multiplier impact, as new home
purchasers tend to buy other buyer durables when they purchase their home. Lodging
development commonly drives retreats and recoveries (Blackley, 1999). Changes in new
lodging supply influence the cost of existing lodging units, with suggestions for the riches
position of mortgage holders and lodging reasonableness. At long last, the welfare and
appropriation impacts of key duty change rely upon the versatility of lodging supply (Blinder
and Maccini, 1991).
A large portion of the current experimental work on lodging treats private construction like
different sorts of speculation. Be that as it may, arrive makes lodging unique: land and along
these lines lodging costs must guarantee a spatial balance with a metropolitan territory and
land is in-elastically provided. In this paper, we evaluate a supply condition for new single-
family living arrangements that depends on the hypothetical models of land advancement and
urban development. Our fundamental assessing condition regards single family begins as a
component of changes in current and slacked house costs as opposed to of their level. This
approach is likewise more reliable with the time arrangement qualities of the information
than the more conventional levels particular. To contrast our specification and existing exact
medicines of new lodging supply we give out-of-test conjectures to the strategy created here
and the two others from the current writing.
The idea of value harmony in lodging markets proposes utilizing changes in house costs to
gauge interest for new development, as opposed to the level of house costs. House costs
equilibrate the aggregate amount of lodging, a stock variable, with the aggregate interest for
private space. Lodging begins are a stream variable, speaking to the adjustment in the supply
of lodging, net of expulsions. In this manner, begins ought to be an element of other stream
factors, incorporating the adjustment in house costs. In adjusting free market activity, house
costs guarantee a spatial harmony for family units inside a lodging market. Subsequently,
house value levels must rely upon factors that foresee the measure of a city, for example, the
open door cost of new land and desires of future development. In an unfaltering state, a few
factors that clarify the supply of lodging might be uncorrelated with lodging begins, which
are the adjustment in a load of lodging.
impacts general yield straight forwardly, development and assembling business ascends with
lodging begins, and in a round-about way through the multiplier impact, as new home
purchasers tend to buy other buyer durables when they purchase their home. Lodging
development commonly drives retreats and recoveries (Blackley, 1999). Changes in new
lodging supply influence the cost of existing lodging units, with suggestions for the riches
position of mortgage holders and lodging reasonableness. At long last, the welfare and
appropriation impacts of key duty change rely upon the versatility of lodging supply (Blinder
and Maccini, 1991).
A large portion of the current experimental work on lodging treats private construction like
different sorts of speculation. Be that as it may, arrive makes lodging unique: land and along
these lines lodging costs must guarantee a spatial balance with a metropolitan territory and
land is in-elastically provided. In this paper, we evaluate a supply condition for new single-
family living arrangements that depends on the hypothetical models of land advancement and
urban development. Our fundamental assessing condition regards single family begins as a
component of changes in current and slacked house costs as opposed to of their level. This
approach is likewise more reliable with the time arrangement qualities of the information
than the more conventional levels particular. To contrast our specification and existing exact
medicines of new lodging supply we give out-of-test conjectures to the strategy created here
and the two others from the current writing.
The idea of value harmony in lodging markets proposes utilizing changes in house costs to
gauge interest for new development, as opposed to the level of house costs. House costs
equilibrate the aggregate amount of lodging, a stock variable, with the aggregate interest for
private space. Lodging begins are a stream variable, speaking to the adjustment in the supply
of lodging, net of expulsions. In this manner, begins ought to be an element of other stream
factors, incorporating the adjustment in house costs. In adjusting free market activity, house
costs guarantee a spatial harmony for family units inside a lodging market. Subsequently,
house value levels must rely upon factors that foresee the measure of a city, for example, the
open door cost of new land and desires of future development. In an unfaltering state, a few
factors that clarify the supply of lodging might be uncorrelated with lodging begins, which
are the adjustment in a load of lodging.
Secure Best Marks with AI Grader
Need help grading? Try our AI Grader for instant feedback on your assignments.
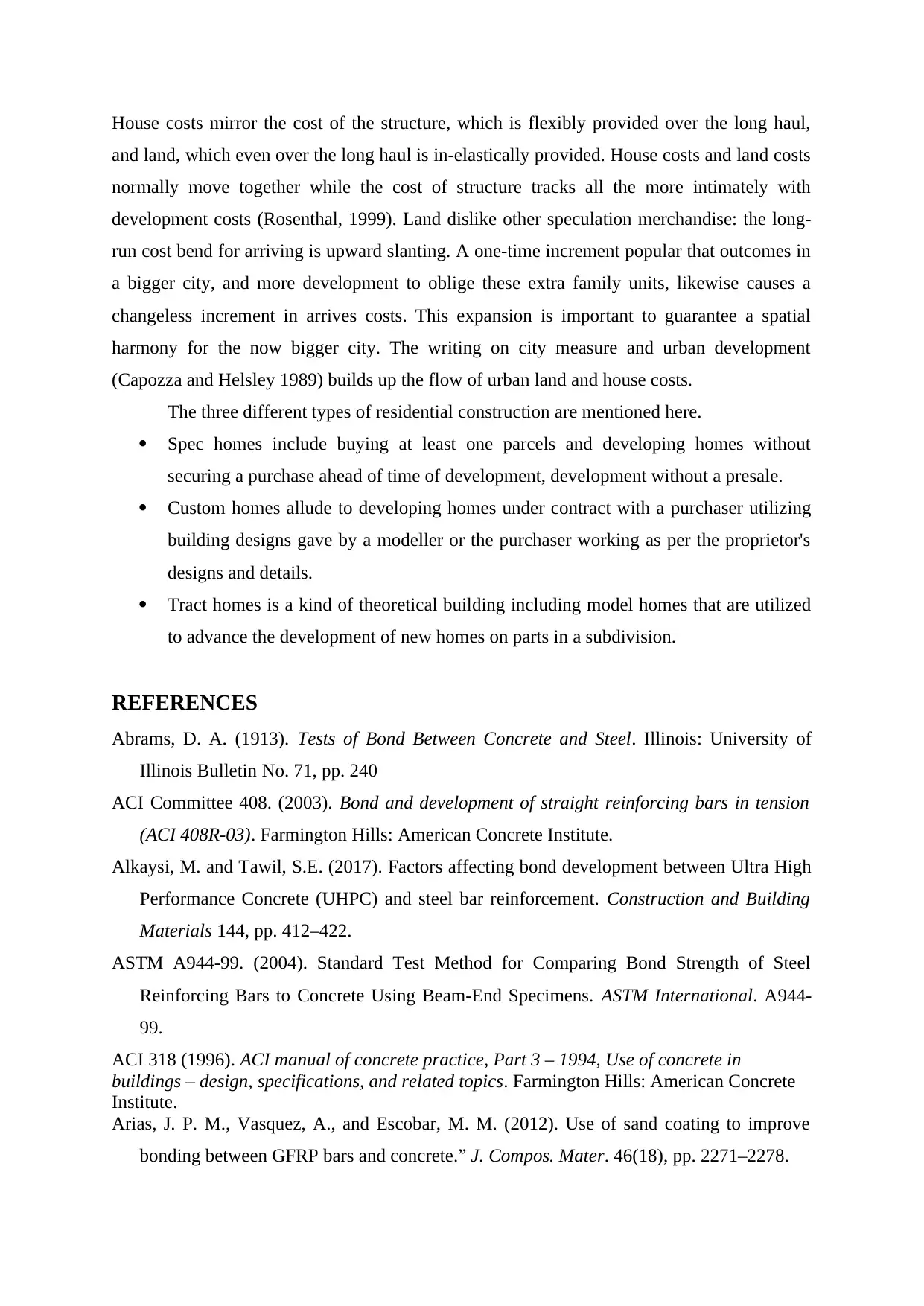
House costs mirror the cost of the structure, which is flexibly provided over the long haul,
and land, which even over the long haul is in-elastically provided. House costs and land costs
normally move together while the cost of structure tracks all the more intimately with
development costs (Rosenthal, 1999). Land dislike other speculation merchandise: the long-
run cost bend for arriving is upward slanting. A one-time increment popular that outcomes in
a bigger city, and more development to oblige these extra family units, likewise causes a
changeless increment in arrives costs. This expansion is important to guarantee a spatial
harmony for the now bigger city. The writing on city measure and urban development
(Capozza and Helsley 1989) builds up the flow of urban land and house costs.
The three different types of residential construction are mentioned here.
Spec homes include buying at least one parcels and developing homes without
securing a purchase ahead of time of development, development without a presale.
Custom homes allude to developing homes under contract with a purchaser utilizing
building designs gave by a modeller or the purchaser working as per the proprietor's
designs and details.
Tract homes is a kind of theoretical building including model homes that are utilized
to advance the development of new homes on parts in a subdivision.
REFERENCES
Abrams, D. A. (1913). Tests of Bond Between Concrete and Steel. Illinois: University of
Illinois Bulletin No. 71, pp. 240
ACI Committee 408. (2003). Bond and development of straight reinforcing bars in tension
(ACI 408R-03). Farmington Hills: American Concrete Institute.
Alkaysi, M. and Tawil, S.E. (2017). Factors affecting bond development between Ultra High
Performance Concrete (UHPC) and steel bar reinforcement. Construction and Building
Materials 144, pp. 412–422.
ASTM A944-99. (2004). Standard Test Method for Comparing Bond Strength of Steel
Reinforcing Bars to Concrete Using Beam-End Specimens. ASTM International. A944-
99.
ACI 318 (1996). ACI manual of concrete practice, Part 3 – 1994, Use of concrete in
buildings – design, specifications, and related topics. Farmington Hills: American Concrete
Institute.
Arias, J. P. M., Vasquez, A., and Escobar, M. M. (2012). Use of sand coating to improve
bonding between GFRP bars and concrete.” J. Compos. Mater. 46(18), pp. 2271–2278.
and land, which even over the long haul is in-elastically provided. House costs and land costs
normally move together while the cost of structure tracks all the more intimately with
development costs (Rosenthal, 1999). Land dislike other speculation merchandise: the long-
run cost bend for arriving is upward slanting. A one-time increment popular that outcomes in
a bigger city, and more development to oblige these extra family units, likewise causes a
changeless increment in arrives costs. This expansion is important to guarantee a spatial
harmony for the now bigger city. The writing on city measure and urban development
(Capozza and Helsley 1989) builds up the flow of urban land and house costs.
The three different types of residential construction are mentioned here.
Spec homes include buying at least one parcels and developing homes without
securing a purchase ahead of time of development, development without a presale.
Custom homes allude to developing homes under contract with a purchaser utilizing
building designs gave by a modeller or the purchaser working as per the proprietor's
designs and details.
Tract homes is a kind of theoretical building including model homes that are utilized
to advance the development of new homes on parts in a subdivision.
REFERENCES
Abrams, D. A. (1913). Tests of Bond Between Concrete and Steel. Illinois: University of
Illinois Bulletin No. 71, pp. 240
ACI Committee 408. (2003). Bond and development of straight reinforcing bars in tension
(ACI 408R-03). Farmington Hills: American Concrete Institute.
Alkaysi, M. and Tawil, S.E. (2017). Factors affecting bond development between Ultra High
Performance Concrete (UHPC) and steel bar reinforcement. Construction and Building
Materials 144, pp. 412–422.
ASTM A944-99. (2004). Standard Test Method for Comparing Bond Strength of Steel
Reinforcing Bars to Concrete Using Beam-End Specimens. ASTM International. A944-
99.
ACI 318 (1996). ACI manual of concrete practice, Part 3 – 1994, Use of concrete in
buildings – design, specifications, and related topics. Farmington Hills: American Concrete
Institute.
Arias, J. P. M., Vasquez, A., and Escobar, M. M. (2012). Use of sand coating to improve
bonding between GFRP bars and concrete.” J. Compos. Mater. 46(18), pp. 2271–2278.
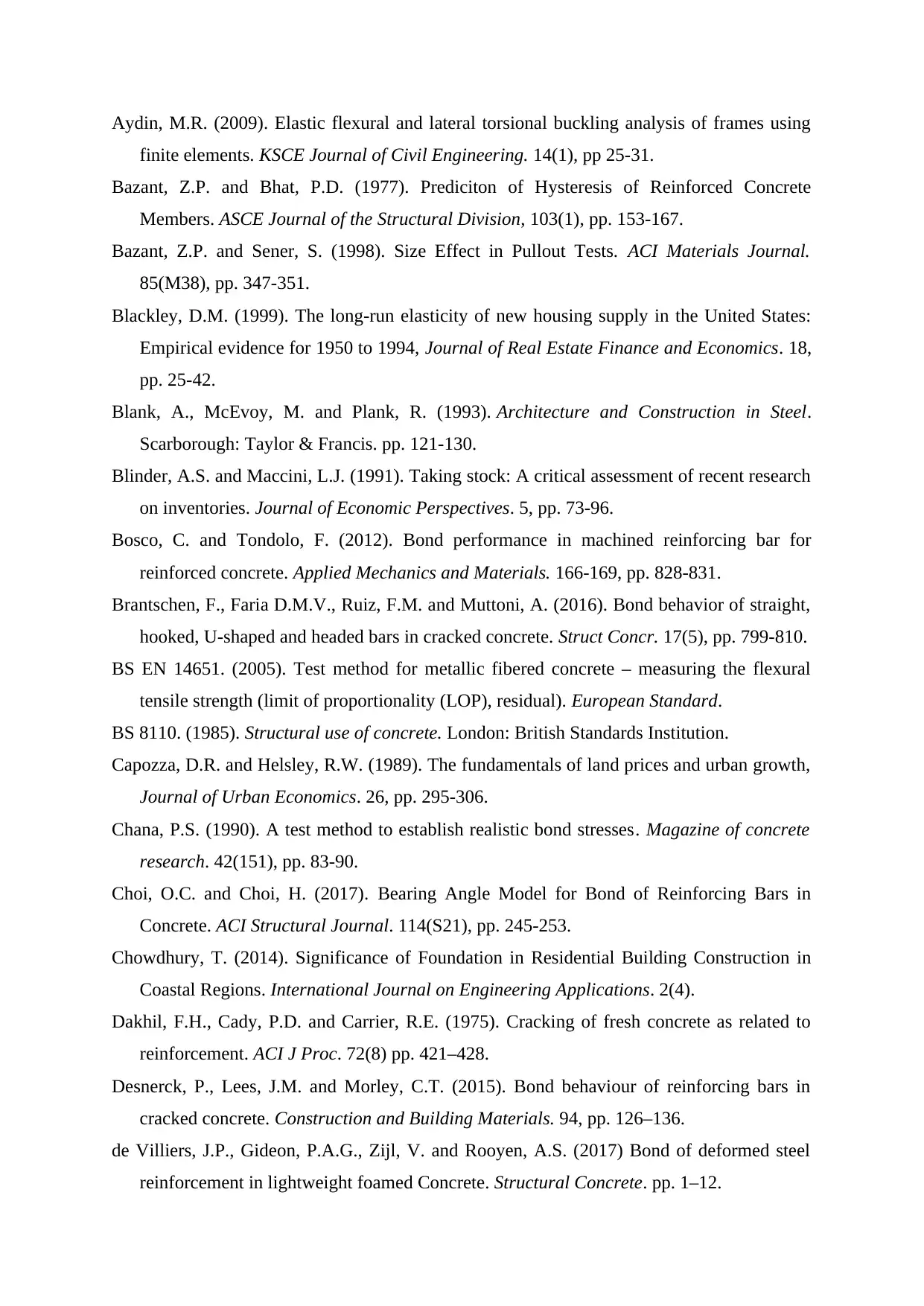
Aydin, M.R. (2009). Elastic flexural and lateral torsional buckling analysis of frames using
finite elements. KSCE Journal of Civil Engineering. 14(1), pp 25-31.
Bazant, Z.P. and Bhat, P.D. (1977). Prediciton of Hysteresis of Reinforced Concrete
Members. ASCE Journal of the Structural Division, 103(1), pp. 153-167.
Bazant, Z.P. and Sener, S. (1998). Size Effect in Pullout Tests. ACI Materials Journal.
85(M38), pp. 347-351.
Blackley, D.M. (1999). The long-run elasticity of new housing supply in the United States:
Empirical evidence for 1950 to 1994, Journal of Real Estate Finance and Economics. 18,
pp. 25-42.
Blank, A., McEvoy, M. and Plank, R. (1993). Architecture and Construction in Steel.
Scarborough: Taylor & Francis. pp. 121-130.
Blinder, A.S. and Maccini, L.J. (1991). Taking stock: A critical assessment of recent research
on inventories. Journal of Economic Perspectives. 5, pp. 73-96.
Bosco, C. and Tondolo, F. (2012). Bond performance in machined reinforcing bar for
reinforced concrete. Applied Mechanics and Materials. 166-169, pp. 828-831.
Brantschen, F., Faria D.M.V., Ruiz, F.M. and Muttoni, A. (2016). Bond behavior of straight,
hooked, U-shaped and headed bars in cracked concrete. Struct Concr. 17(5), pp. 799-810.
BS EN 14651. (2005). Test method for metallic fibered concrete – measuring the flexural
tensile strength (limit of proportionality (LOP), residual). European Standard.
BS 8110. (1985). Structural use of concrete. London: British Standards Institution.
Capozza, D.R. and Helsley, R.W. (1989). The fundamentals of land prices and urban growth,
Journal of Urban Economics. 26, pp. 295-306.
Chana, P.S. (1990). A test method to establish realistic bond stresses. Magazine of concrete
research. 42(151), pp. 83-90.
Choi, O.C. and Choi, H. (2017). Bearing Angle Model for Bond of Reinforcing Bars in
Concrete. ACI Structural Journal. 114(S21), pp. 245-253.
Chowdhury, T. (2014). Significance of Foundation in Residential Building Construction in
Coastal Regions. International Journal on Engineering Applications. 2(4).
Dakhil, F.H., Cady, P.D. and Carrier, R.E. (1975). Cracking of fresh concrete as related to
reinforcement. ACI J Proc. 72(8) pp. 421–428.
Desnerck, P., Lees, J.M. and Morley, C.T. (2015). Bond behaviour of reinforcing bars in
cracked concrete. Construction and Building Materials. 94, pp. 126–136.
de Villiers, J.P., Gideon, P.A.G., Zijl, V. and Rooyen, A.S. (2017) Bond of deformed steel
reinforcement in lightweight foamed Concrete. Structural Concrete. pp. 1–12.
finite elements. KSCE Journal of Civil Engineering. 14(1), pp 25-31.
Bazant, Z.P. and Bhat, P.D. (1977). Prediciton of Hysteresis of Reinforced Concrete
Members. ASCE Journal of the Structural Division, 103(1), pp. 153-167.
Bazant, Z.P. and Sener, S. (1998). Size Effect in Pullout Tests. ACI Materials Journal.
85(M38), pp. 347-351.
Blackley, D.M. (1999). The long-run elasticity of new housing supply in the United States:
Empirical evidence for 1950 to 1994, Journal of Real Estate Finance and Economics. 18,
pp. 25-42.
Blank, A., McEvoy, M. and Plank, R. (1993). Architecture and Construction in Steel.
Scarborough: Taylor & Francis. pp. 121-130.
Blinder, A.S. and Maccini, L.J. (1991). Taking stock: A critical assessment of recent research
on inventories. Journal of Economic Perspectives. 5, pp. 73-96.
Bosco, C. and Tondolo, F. (2012). Bond performance in machined reinforcing bar for
reinforced concrete. Applied Mechanics and Materials. 166-169, pp. 828-831.
Brantschen, F., Faria D.M.V., Ruiz, F.M. and Muttoni, A. (2016). Bond behavior of straight,
hooked, U-shaped and headed bars in cracked concrete. Struct Concr. 17(5), pp. 799-810.
BS EN 14651. (2005). Test method for metallic fibered concrete – measuring the flexural
tensile strength (limit of proportionality (LOP), residual). European Standard.
BS 8110. (1985). Structural use of concrete. London: British Standards Institution.
Capozza, D.R. and Helsley, R.W. (1989). The fundamentals of land prices and urban growth,
Journal of Urban Economics. 26, pp. 295-306.
Chana, P.S. (1990). A test method to establish realistic bond stresses. Magazine of concrete
research. 42(151), pp. 83-90.
Choi, O.C. and Choi, H. (2017). Bearing Angle Model for Bond of Reinforcing Bars in
Concrete. ACI Structural Journal. 114(S21), pp. 245-253.
Chowdhury, T. (2014). Significance of Foundation in Residential Building Construction in
Coastal Regions. International Journal on Engineering Applications. 2(4).
Dakhil, F.H., Cady, P.D. and Carrier, R.E. (1975). Cracking of fresh concrete as related to
reinforcement. ACI J Proc. 72(8) pp. 421–428.
Desnerck, P., Lees, J.M. and Morley, C.T. (2015). Bond behaviour of reinforcing bars in
cracked concrete. Construction and Building Materials. 94, pp. 126–136.
de Villiers, J.P., Gideon, P.A.G., Zijl, V. and Rooyen, A.S. (2017) Bond of deformed steel
reinforcement in lightweight foamed Concrete. Structural Concrete. pp. 1–12.
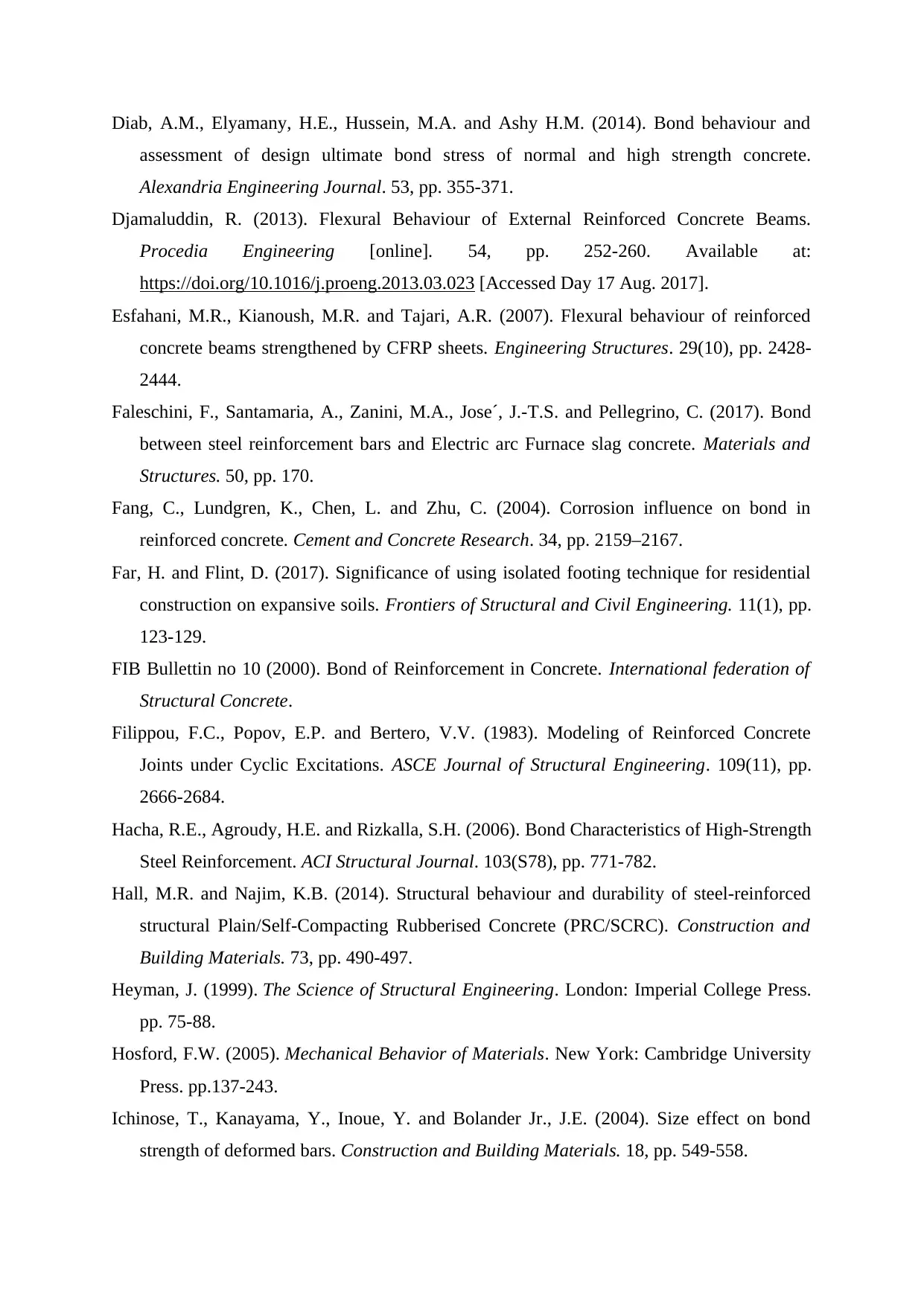
Diab, A.M., Elyamany, H.E., Hussein, M.A. and Ashy H.M. (2014). Bond behaviour and
assessment of design ultimate bond stress of normal and high strength concrete.
Alexandria Engineering Journal. 53, pp. 355-371.
Djamaluddin, R. (2013). Flexural Behaviour of External Reinforced Concrete Beams.
Procedia Engineering [online]. 54, pp. 252-260. Available at:
https://doi.org/10.1016/j.proeng.2013.03.023 [Accessed Day 17 Aug. 2017].
Esfahani, M.R., Kianoush, M.R. and Tajari, A.R. (2007). Flexural behaviour of reinforced
concrete beams strengthened by CFRP sheets. Engineering Structures. 29(10), pp. 2428-
2444.
Faleschini, F., Santamaria, A., Zanini, M.A., Jose´, J.-T.S. and Pellegrino, C. (2017). Bond
between steel reinforcement bars and Electric arc Furnace slag concrete. Materials and
Structures. 50, pp. 170.
Fang, C., Lundgren, K., Chen, L. and Zhu, C. (2004). Corrosion influence on bond in
reinforced concrete. Cement and Concrete Research. 34, pp. 2159–2167.
Far, H. and Flint, D. (2017). Significance of using isolated footing technique for residential
construction on expansive soils. Frontiers of Structural and Civil Engineering. 11(1), pp.
123-129.
FIB Bullettin no 10 (2000). Bond of Reinforcement in Concrete. International federation of
Structural Concrete.
Filippou, F.C., Popov, E.P. and Bertero, V.V. (1983). Modeling of Reinforced Concrete
Joints under Cyclic Excitations. ASCE Journal of Structural Engineering. 109(11), pp.
2666-2684.
Hacha, R.E., Agroudy, H.E. and Rizkalla, S.H. (2006). Bond Characteristics of High-Strength
Steel Reinforcement. ACI Structural Journal. 103(S78), pp. 771-782.
Hall, M.R. and Najim, K.B. (2014). Structural behaviour and durability of steel-reinforced
structural Plain/Self-Compacting Rubberised Concrete (PRC/SCRC). Construction and
Building Materials. 73, pp. 490-497.
Heyman, J. (1999). The Science of Structural Engineering. London: Imperial College Press.
pp. 75-88.
Hosford, F.W. (2005). Mechanical Behavior of Materials. New York: Cambridge University
Press. pp.137-243.
Ichinose, T., Kanayama, Y., Inoue, Y. and Bolander Jr., J.E. (2004). Size effect on bond
strength of deformed bars. Construction and Building Materials. 18, pp. 549-558.
assessment of design ultimate bond stress of normal and high strength concrete.
Alexandria Engineering Journal. 53, pp. 355-371.
Djamaluddin, R. (2013). Flexural Behaviour of External Reinforced Concrete Beams.
Procedia Engineering [online]. 54, pp. 252-260. Available at:
https://doi.org/10.1016/j.proeng.2013.03.023 [Accessed Day 17 Aug. 2017].
Esfahani, M.R., Kianoush, M.R. and Tajari, A.R. (2007). Flexural behaviour of reinforced
concrete beams strengthened by CFRP sheets. Engineering Structures. 29(10), pp. 2428-
2444.
Faleschini, F., Santamaria, A., Zanini, M.A., Jose´, J.-T.S. and Pellegrino, C. (2017). Bond
between steel reinforcement bars and Electric arc Furnace slag concrete. Materials and
Structures. 50, pp. 170.
Fang, C., Lundgren, K., Chen, L. and Zhu, C. (2004). Corrosion influence on bond in
reinforced concrete. Cement and Concrete Research. 34, pp. 2159–2167.
Far, H. and Flint, D. (2017). Significance of using isolated footing technique for residential
construction on expansive soils. Frontiers of Structural and Civil Engineering. 11(1), pp.
123-129.
FIB Bullettin no 10 (2000). Bond of Reinforcement in Concrete. International federation of
Structural Concrete.
Filippou, F.C., Popov, E.P. and Bertero, V.V. (1983). Modeling of Reinforced Concrete
Joints under Cyclic Excitations. ASCE Journal of Structural Engineering. 109(11), pp.
2666-2684.
Hacha, R.E., Agroudy, H.E. and Rizkalla, S.H. (2006). Bond Characteristics of High-Strength
Steel Reinforcement. ACI Structural Journal. 103(S78), pp. 771-782.
Hall, M.R. and Najim, K.B. (2014). Structural behaviour and durability of steel-reinforced
structural Plain/Self-Compacting Rubberised Concrete (PRC/SCRC). Construction and
Building Materials. 73, pp. 490-497.
Heyman, J. (1999). The Science of Structural Engineering. London: Imperial College Press.
pp. 75-88.
Hosford, F.W. (2005). Mechanical Behavior of Materials. New York: Cambridge University
Press. pp.137-243.
Ichinose, T., Kanayama, Y., Inoue, Y. and Bolander Jr., J.E. (2004). Size effect on bond
strength of deformed bars. Construction and Building Materials. 18, pp. 549-558.
Paraphrase This Document
Need a fresh take? Get an instant paraphrase of this document with our AI Paraphraser
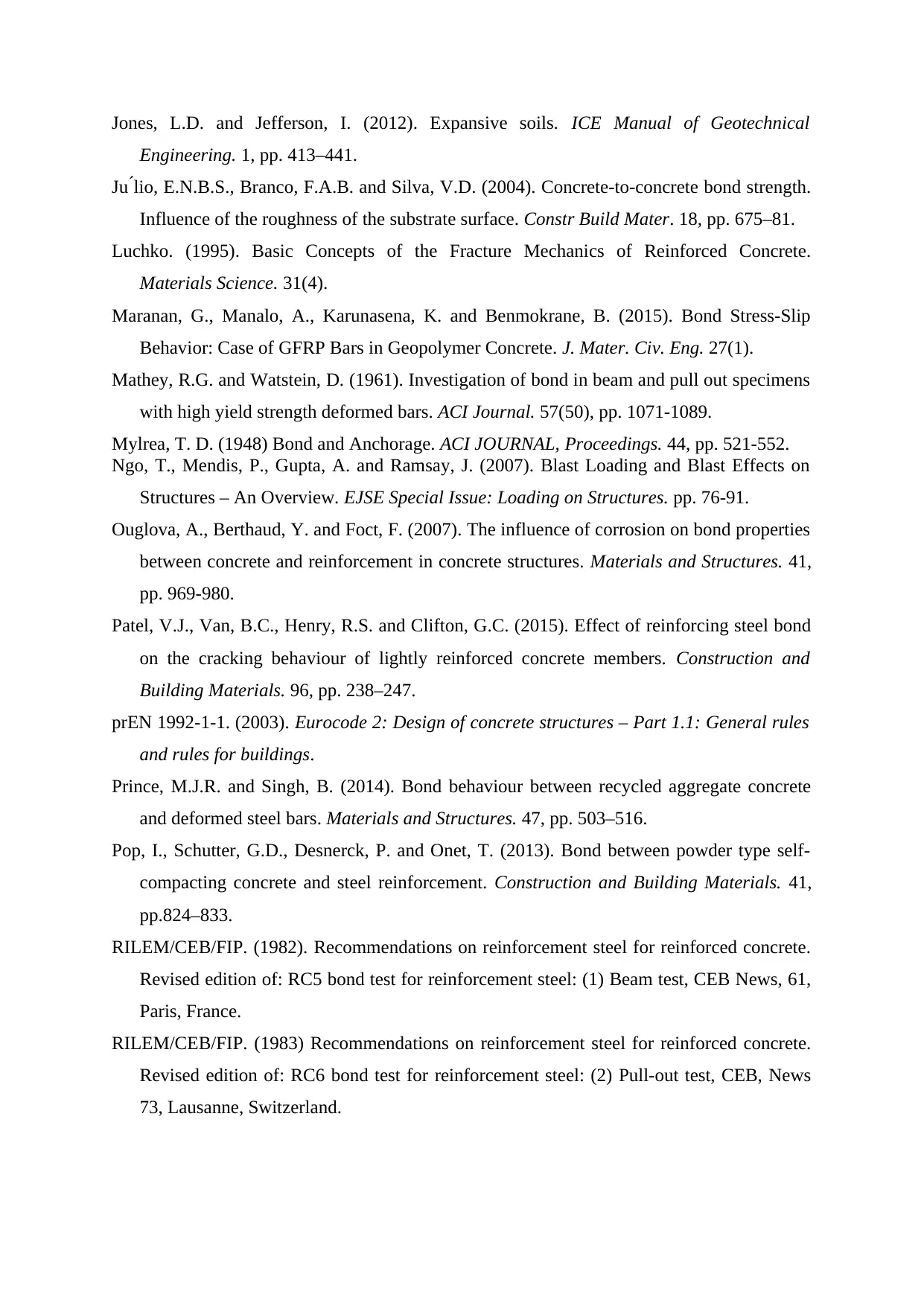
Jones, L.D. and Jefferson, I. (2012). Expansive soils. ICE Manual of Geotechnical
Engineering. 1, pp. 413–441.
Ju ́ lio, E.N.B.S., Branco, F.A.B. and Silva, V.D. (2004). Concrete-to-concrete bond strength.
Influence of the roughness of the substrate surface. Constr Build Mater. 18, pp. 675–81.
Luchko. (1995). Basic Concepts of the Fracture Mechanics of Reinforced Concrete.
Materials Science. 31(4).
Maranan, G., Manalo, A., Karunasena, K. and Benmokrane, B. (2015). Bond Stress-Slip
Behavior: Case of GFRP Bars in Geopolymer Concrete. J. Mater. Civ. Eng. 27(1).
Mathey, R.G. and Watstein, D. (1961). Investigation of bond in beam and pull out specimens
with high yield strength deformed bars. ACI Journal. 57(50), pp. 1071-1089.
Mylrea, T. D. (1948) Bond and Anchorage. ACI JOURNAL, Proceedings. 44, pp. 521-552.
Ngo, T., Mendis, P., Gupta, A. and Ramsay, J. (2007). Blast Loading and Blast Effects on
Structures – An Overview. EJSE Special Issue: Loading on Structures. pp. 76-91.
Ouglova, A., Berthaud, Y. and Foct, F. (2007). The influence of corrosion on bond properties
between concrete and reinforcement in concrete structures. Materials and Structures. 41,
pp. 969-980.
Patel, V.J., Van, B.C., Henry, R.S. and Clifton, G.C. (2015). Effect of reinforcing steel bond
on the cracking behaviour of lightly reinforced concrete members. Construction and
Building Materials. 96, pp. 238–247.
prEN 1992-1-1. (2003). Eurocode 2: Design of concrete structures – Part 1.1: General rules
and rules for buildings.
Prince, M.J.R. and Singh, B. (2014). Bond behaviour between recycled aggregate concrete
and deformed steel bars. Materials and Structures. 47, pp. 503–516.
Pop, I., Schutter, G.D., Desnerck, P. and Onet, T. (2013). Bond between powder type self-
compacting concrete and steel reinforcement. Construction and Building Materials. 41,
pp.824–833.
RILEM/CEB/FIP. (1982). Recommendations on reinforcement steel for reinforced concrete.
Revised edition of: RC5 bond test for reinforcement steel: (1) Beam test, CEB News, 61,
Paris, France.
RILEM/CEB/FIP. (1983) Recommendations on reinforcement steel for reinforced concrete.
Revised edition of: RC6 bond test for reinforcement steel: (2) Pull-out test, CEB, News
73, Lausanne, Switzerland.
Engineering. 1, pp. 413–441.
Ju ́ lio, E.N.B.S., Branco, F.A.B. and Silva, V.D. (2004). Concrete-to-concrete bond strength.
Influence of the roughness of the substrate surface. Constr Build Mater. 18, pp. 675–81.
Luchko. (1995). Basic Concepts of the Fracture Mechanics of Reinforced Concrete.
Materials Science. 31(4).
Maranan, G., Manalo, A., Karunasena, K. and Benmokrane, B. (2015). Bond Stress-Slip
Behavior: Case of GFRP Bars in Geopolymer Concrete. J. Mater. Civ. Eng. 27(1).
Mathey, R.G. and Watstein, D. (1961). Investigation of bond in beam and pull out specimens
with high yield strength deformed bars. ACI Journal. 57(50), pp. 1071-1089.
Mylrea, T. D. (1948) Bond and Anchorage. ACI JOURNAL, Proceedings. 44, pp. 521-552.
Ngo, T., Mendis, P., Gupta, A. and Ramsay, J. (2007). Blast Loading and Blast Effects on
Structures – An Overview. EJSE Special Issue: Loading on Structures. pp. 76-91.
Ouglova, A., Berthaud, Y. and Foct, F. (2007). The influence of corrosion on bond properties
between concrete and reinforcement in concrete structures. Materials and Structures. 41,
pp. 969-980.
Patel, V.J., Van, B.C., Henry, R.S. and Clifton, G.C. (2015). Effect of reinforcing steel bond
on the cracking behaviour of lightly reinforced concrete members. Construction and
Building Materials. 96, pp. 238–247.
prEN 1992-1-1. (2003). Eurocode 2: Design of concrete structures – Part 1.1: General rules
and rules for buildings.
Prince, M.J.R. and Singh, B. (2014). Bond behaviour between recycled aggregate concrete
and deformed steel bars. Materials and Structures. 47, pp. 503–516.
Pop, I., Schutter, G.D., Desnerck, P. and Onet, T. (2013). Bond between powder type self-
compacting concrete and steel reinforcement. Construction and Building Materials. 41,
pp.824–833.
RILEM/CEB/FIP. (1982). Recommendations on reinforcement steel for reinforced concrete.
Revised edition of: RC5 bond test for reinforcement steel: (1) Beam test, CEB News, 61,
Paris, France.
RILEM/CEB/FIP. (1983) Recommendations on reinforcement steel for reinforced concrete.
Revised edition of: RC6 bond test for reinforcement steel: (2) Pull-out test, CEB, News
73, Lausanne, Switzerland.
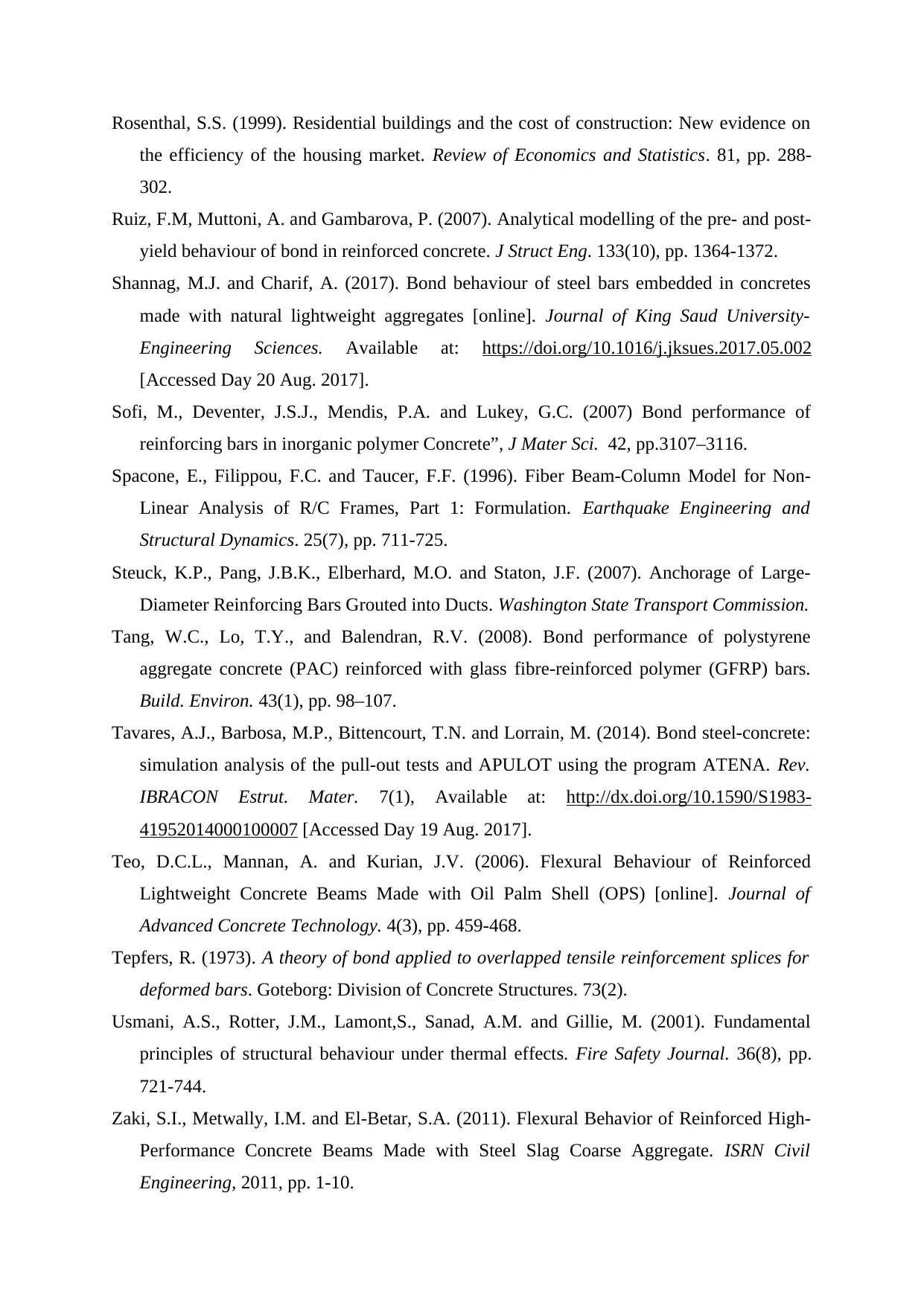
Rosenthal, S.S. (1999). Residential buildings and the cost of construction: New evidence on
the efficiency of the housing market. Review of Economics and Statistics. 81, pp. 288-
302.
Ruiz, F.M, Muttoni, A. and Gambarova, P. (2007). Analytical modelling of the pre- and post-
yield behaviour of bond in reinforced concrete. J Struct Eng. 133(10), pp. 1364-1372.
Shannag, M.J. and Charif, A. (2017). Bond behaviour of steel bars embedded in concretes
made with natural lightweight aggregates [online]. Journal of King Saud University-
Engineering Sciences. Available at: https://doi.org/10.1016/j.jksues.2017.05.002
[Accessed Day 20 Aug. 2017].
Sofi, M., Deventer, J.S.J., Mendis, P.A. and Lukey, G.C. (2007) Bond performance of
reinforcing bars in inorganic polymer Concrete”, J Mater Sci. 42, pp.3107–3116.
Spacone, E., Filippou, F.C. and Taucer, F.F. (1996). Fiber Beam-Column Model for Non-
Linear Analysis of R/C Frames, Part 1: Formulation. Earthquake Engineering and
Structural Dynamics. 25(7), pp. 711-725.
Steuck, K.P., Pang, J.B.K., Elberhard, M.O. and Staton, J.F. (2007). Anchorage of Large-
Diameter Reinforcing Bars Grouted into Ducts. Washington State Transport Commission.
Tang, W.C., Lo, T.Y., and Balendran, R.V. (2008). Bond performance of polystyrene
aggregate concrete (PAC) reinforced with glass fibre-reinforced polymer (GFRP) bars.
Build. Environ. 43(1), pp. 98–107.
Tavares, A.J., Barbosa, M.P., Bittencourt, T.N. and Lorrain, M. (2014). Bond steel-concrete:
simulation analysis of the pull-out tests and APULOT using the program ATENA. Rev.
IBRACON Estrut. Mater. 7(1), Available at: http://dx.doi.org/10.1590/S1983-
41952014000100007 [Accessed Day 19 Aug. 2017].
Teo, D.C.L., Mannan, A. and Kurian, J.V. (2006). Flexural Behaviour of Reinforced
Lightweight Concrete Beams Made with Oil Palm Shell (OPS) [online]. Journal of
Advanced Concrete Technology. 4(3), pp. 459-468.
Tepfers, R. (1973). A theory of bond applied to overlapped tensile reinforcement splices for
deformed bars. Goteborg: Division of Concrete Structures. 73(2).
Usmani, A.S., Rotter, J.M., Lamont,S., Sanad, A.M. and Gillie, M. (2001). Fundamental
principles of structural behaviour under thermal effects. Fire Safety Journal. 36(8), pp.
721-744.
Zaki, S.I., Metwally, I.M. and El-Betar, S.A. (2011). Flexural Behavior of Reinforced High-
Performance Concrete Beams Made with Steel Slag Coarse Aggregate. ISRN Civil
Engineering, 2011, pp. 1-10.
the efficiency of the housing market. Review of Economics and Statistics. 81, pp. 288-
302.
Ruiz, F.M, Muttoni, A. and Gambarova, P. (2007). Analytical modelling of the pre- and post-
yield behaviour of bond in reinforced concrete. J Struct Eng. 133(10), pp. 1364-1372.
Shannag, M.J. and Charif, A. (2017). Bond behaviour of steel bars embedded in concretes
made with natural lightweight aggregates [online]. Journal of King Saud University-
Engineering Sciences. Available at: https://doi.org/10.1016/j.jksues.2017.05.002
[Accessed Day 20 Aug. 2017].
Sofi, M., Deventer, J.S.J., Mendis, P.A. and Lukey, G.C. (2007) Bond performance of
reinforcing bars in inorganic polymer Concrete”, J Mater Sci. 42, pp.3107–3116.
Spacone, E., Filippou, F.C. and Taucer, F.F. (1996). Fiber Beam-Column Model for Non-
Linear Analysis of R/C Frames, Part 1: Formulation. Earthquake Engineering and
Structural Dynamics. 25(7), pp. 711-725.
Steuck, K.P., Pang, J.B.K., Elberhard, M.O. and Staton, J.F. (2007). Anchorage of Large-
Diameter Reinforcing Bars Grouted into Ducts. Washington State Transport Commission.
Tang, W.C., Lo, T.Y., and Balendran, R.V. (2008). Bond performance of polystyrene
aggregate concrete (PAC) reinforced with glass fibre-reinforced polymer (GFRP) bars.
Build. Environ. 43(1), pp. 98–107.
Tavares, A.J., Barbosa, M.P., Bittencourt, T.N. and Lorrain, M. (2014). Bond steel-concrete:
simulation analysis of the pull-out tests and APULOT using the program ATENA. Rev.
IBRACON Estrut. Mater. 7(1), Available at: http://dx.doi.org/10.1590/S1983-
41952014000100007 [Accessed Day 19 Aug. 2017].
Teo, D.C.L., Mannan, A. and Kurian, J.V. (2006). Flexural Behaviour of Reinforced
Lightweight Concrete Beams Made with Oil Palm Shell (OPS) [online]. Journal of
Advanced Concrete Technology. 4(3), pp. 459-468.
Tepfers, R. (1973). A theory of bond applied to overlapped tensile reinforcement splices for
deformed bars. Goteborg: Division of Concrete Structures. 73(2).
Usmani, A.S., Rotter, J.M., Lamont,S., Sanad, A.M. and Gillie, M. (2001). Fundamental
principles of structural behaviour under thermal effects. Fire Safety Journal. 36(8), pp.
721-744.
Zaki, S.I., Metwally, I.M. and El-Betar, S.A. (2011). Flexural Behavior of Reinforced High-
Performance Concrete Beams Made with Steel Slag Coarse Aggregate. ISRN Civil
Engineering, 2011, pp. 1-10.
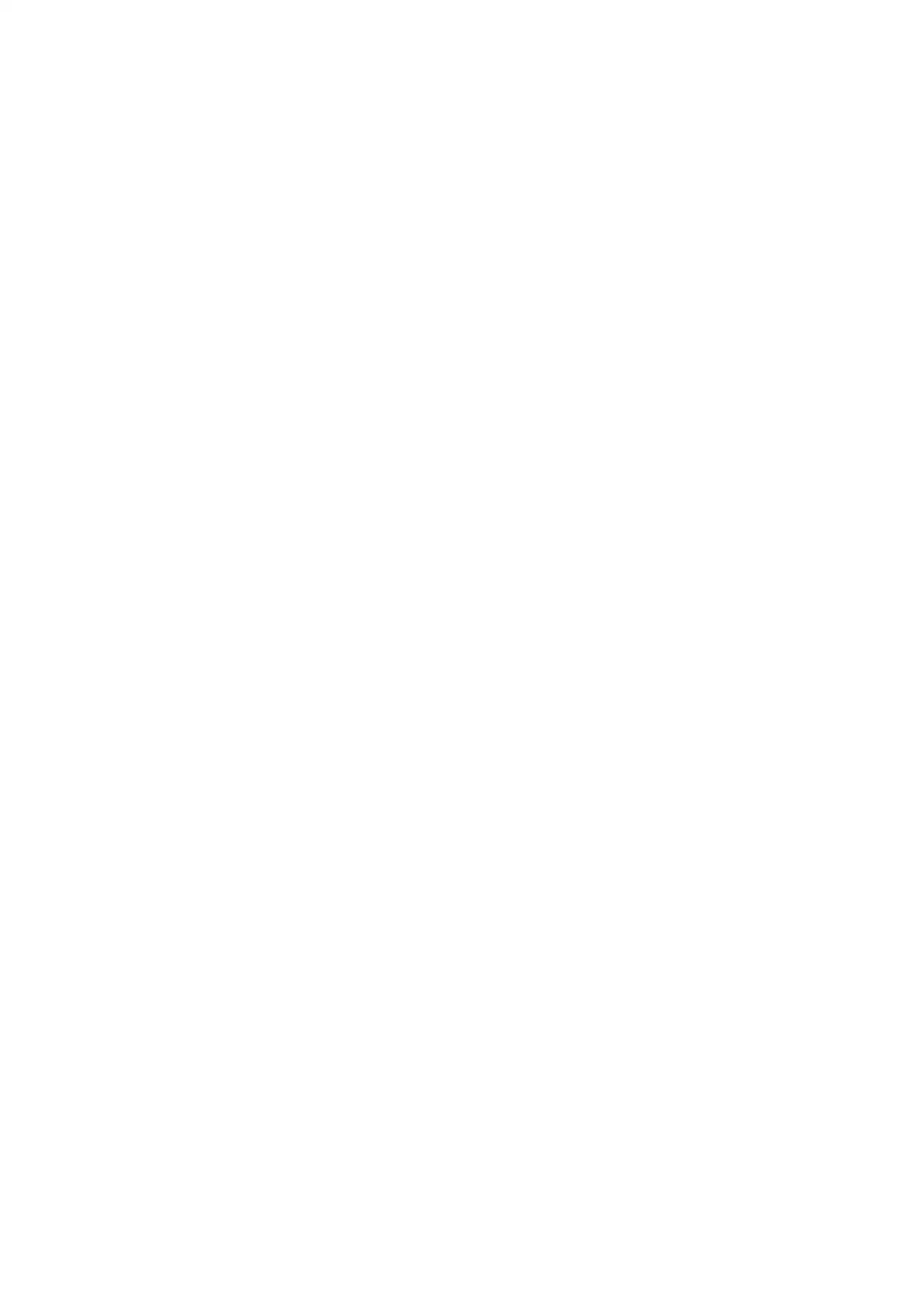
1 out of 22
Related Documents

Your All-in-One AI-Powered Toolkit for Academic Success.
+13062052269
info@desklib.com
Available 24*7 on WhatsApp / Email
Unlock your academic potential
© 2024 | Zucol Services PVT LTD | All rights reserved.