Mechanism of Action of Botox: A Cellular Perspective
VerifiedAdded on 2023/05/30
|5
|1205
|421
AI Summary
This article explains the mechanism of action of Botox from a cellular perspective. It describes how Botox prevents the release of acetylcholine and cleaves key proteins necessary for nerve activation. It also discusses how Botox is useful in spasticity and other disorders. The article includes an experimental plan for elucidating the action of Botox using Fluorescence Correlation Spectroscopy (FCS).
Contribute Materials
Your contribution can guide someone’s learning journey. Share your
documents today.
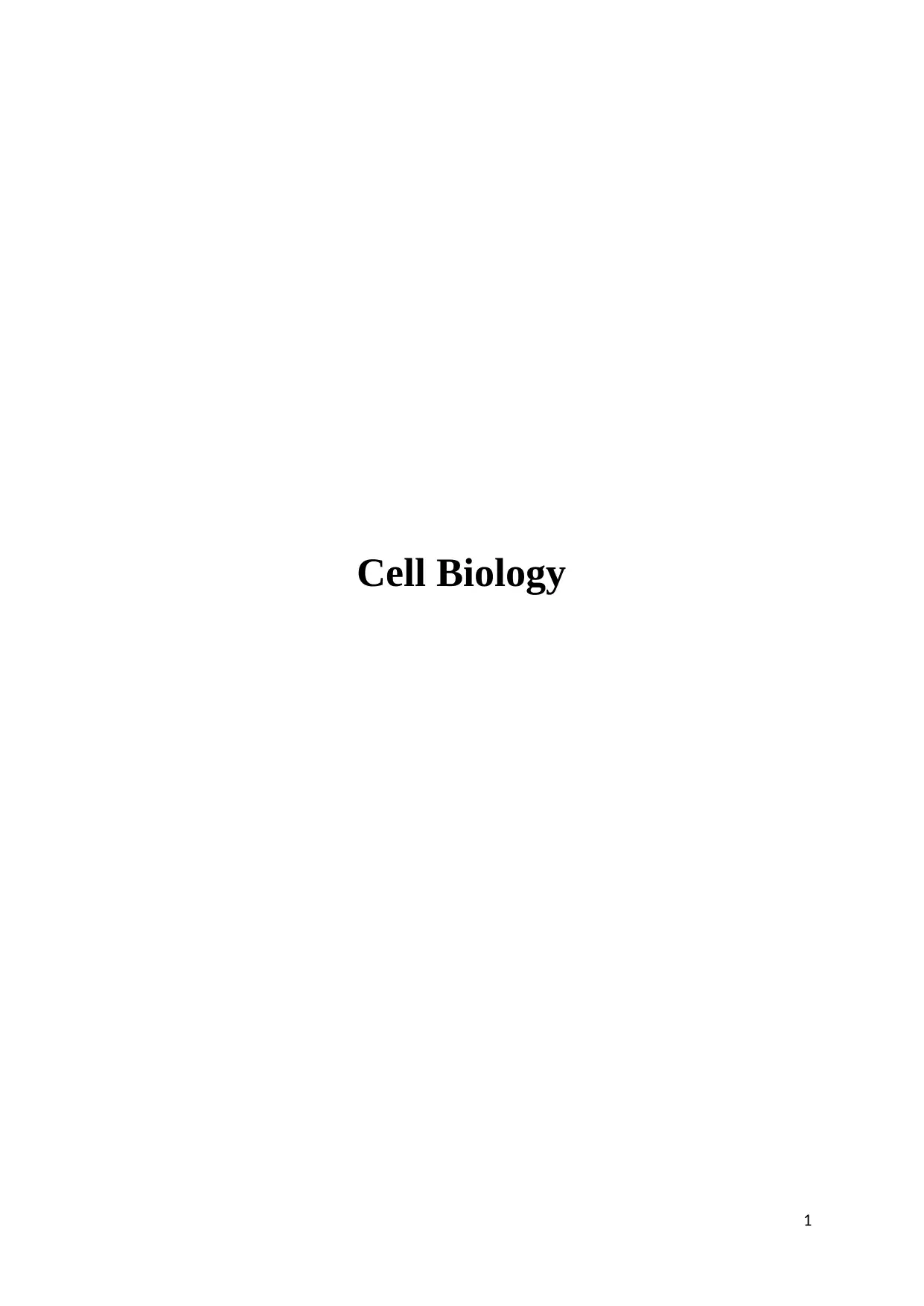
Cell Biology
1
1
Secure Best Marks with AI Grader
Need help grading? Try our AI Grader for instant feedback on your assignments.
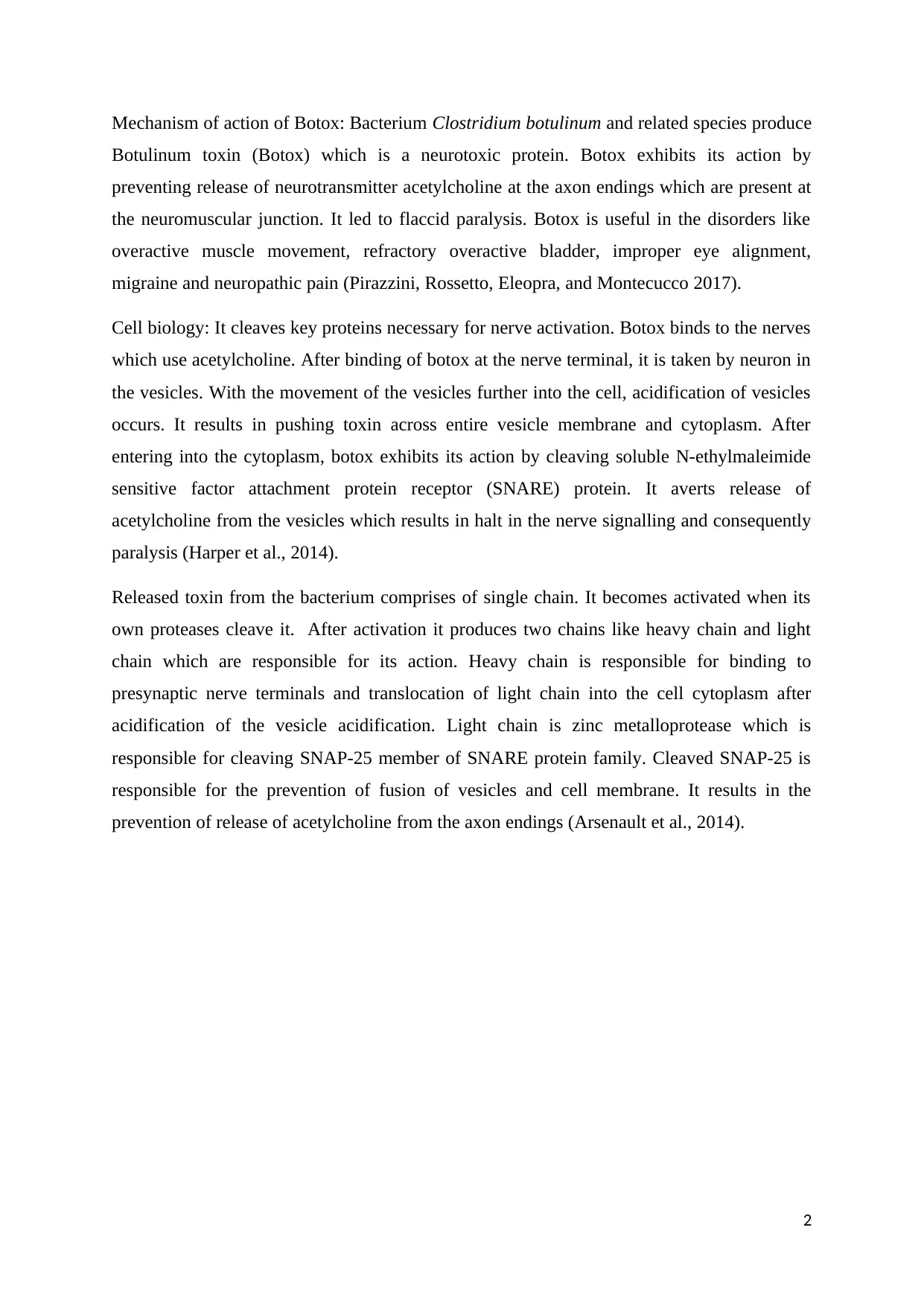
Mechanism of action of Botox: Bacterium Clostridium botulinum and related species produce
Botulinum toxin (Botox) which is a neurotoxic protein. Botox exhibits its action by
preventing release of neurotransmitter acetylcholine at the axon endings which are present at
the neuromuscular junction. It led to flaccid paralysis. Botox is useful in the disorders like
overactive muscle movement, refractory overactive bladder, improper eye alignment,
migraine and neuropathic pain (Pirazzini, Rossetto, Eleopra, and Montecucco 2017).
Cell biology: It cleaves key proteins necessary for nerve activation. Botox binds to the nerves
which use acetylcholine. After binding of botox at the nerve terminal, it is taken by neuron in
the vesicles. With the movement of the vesicles further into the cell, acidification of vesicles
occurs. It results in pushing toxin across entire vesicle membrane and cytoplasm. After
entering into the cytoplasm, botox exhibits its action by cleaving soluble N-ethylmaleimide
sensitive factor attachment protein receptor (SNARE) protein. It averts release of
acetylcholine from the vesicles which results in halt in the nerve signalling and consequently
paralysis (Harper et al., 2014).
Released toxin from the bacterium comprises of single chain. It becomes activated when its
own proteases cleave it. After activation it produces two chains like heavy chain and light
chain which are responsible for its action. Heavy chain is responsible for binding to
presynaptic nerve terminals and translocation of light chain into the cell cytoplasm after
acidification of the vesicle acidification. Light chain is zinc metalloprotease which is
responsible for cleaving SNAP-25 member of SNARE protein family. Cleaved SNAP-25 is
responsible for the prevention of fusion of vesicles and cell membrane. It results in the
prevention of release of acetylcholine from the axon endings (Arsenault et al., 2014).
2
Botulinum toxin (Botox) which is a neurotoxic protein. Botox exhibits its action by
preventing release of neurotransmitter acetylcholine at the axon endings which are present at
the neuromuscular junction. It led to flaccid paralysis. Botox is useful in the disorders like
overactive muscle movement, refractory overactive bladder, improper eye alignment,
migraine and neuropathic pain (Pirazzini, Rossetto, Eleopra, and Montecucco 2017).
Cell biology: It cleaves key proteins necessary for nerve activation. Botox binds to the nerves
which use acetylcholine. After binding of botox at the nerve terminal, it is taken by neuron in
the vesicles. With the movement of the vesicles further into the cell, acidification of vesicles
occurs. It results in pushing toxin across entire vesicle membrane and cytoplasm. After
entering into the cytoplasm, botox exhibits its action by cleaving soluble N-ethylmaleimide
sensitive factor attachment protein receptor (SNARE) protein. It averts release of
acetylcholine from the vesicles which results in halt in the nerve signalling and consequently
paralysis (Harper et al., 2014).
Released toxin from the bacterium comprises of single chain. It becomes activated when its
own proteases cleave it. After activation it produces two chains like heavy chain and light
chain which are responsible for its action. Heavy chain is responsible for binding to
presynaptic nerve terminals and translocation of light chain into the cell cytoplasm after
acidification of the vesicle acidification. Light chain is zinc metalloprotease which is
responsible for cleaving SNAP-25 member of SNARE protein family. Cleaved SNAP-25 is
responsible for the prevention of fusion of vesicles and cell membrane. It results in the
prevention of release of acetylcholine from the axon endings (Arsenault et al., 2014).
2
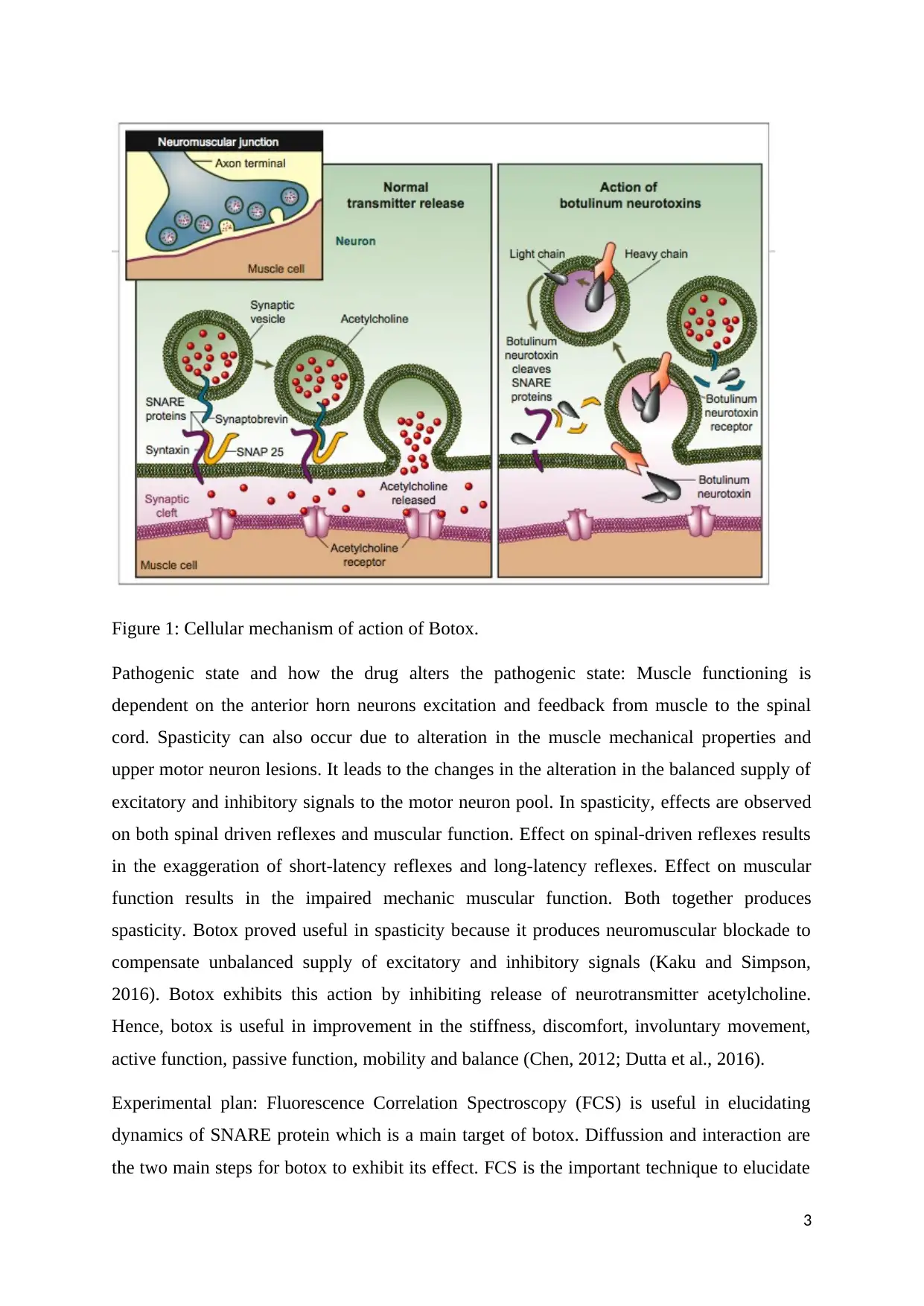
Figure 1: Cellular mechanism of action of Botox.
Pathogenic state and how the drug alters the pathogenic state: Muscle functioning is
dependent on the anterior horn neurons excitation and feedback from muscle to the spinal
cord. Spasticity can also occur due to alteration in the muscle mechanical properties and
upper motor neuron lesions. It leads to the changes in the alteration in the balanced supply of
excitatory and inhibitory signals to the motor neuron pool. In spasticity, effects are observed
on both spinal driven reflexes and muscular function. Effect on spinal-driven reflexes results
in the exaggeration of short-latency reflexes and long-latency reflexes. Effect on muscular
function results in the impaired mechanic muscular function. Both together produces
spasticity. Botox proved useful in spasticity because it produces neuromuscular blockade to
compensate unbalanced supply of excitatory and inhibitory signals (Kaku and Simpson,
2016). Botox exhibits this action by inhibiting release of neurotransmitter acetylcholine.
Hence, botox is useful in improvement in the stiffness, discomfort, involuntary movement,
active function, passive function, mobility and balance (Chen, 2012; Dutta et al., 2016).
Experimental plan: Fluorescence Correlation Spectroscopy (FCS) is useful in elucidating
dynamics of SNARE protein which is a main target of botox. Diffussion and interaction are
the two main steps for botox to exhibit its effect. FCS is the important technique to elucidate
3
Pathogenic state and how the drug alters the pathogenic state: Muscle functioning is
dependent on the anterior horn neurons excitation and feedback from muscle to the spinal
cord. Spasticity can also occur due to alteration in the muscle mechanical properties and
upper motor neuron lesions. It leads to the changes in the alteration in the balanced supply of
excitatory and inhibitory signals to the motor neuron pool. In spasticity, effects are observed
on both spinal driven reflexes and muscular function. Effect on spinal-driven reflexes results
in the exaggeration of short-latency reflexes and long-latency reflexes. Effect on muscular
function results in the impaired mechanic muscular function. Both together produces
spasticity. Botox proved useful in spasticity because it produces neuromuscular blockade to
compensate unbalanced supply of excitatory and inhibitory signals (Kaku and Simpson,
2016). Botox exhibits this action by inhibiting release of neurotransmitter acetylcholine.
Hence, botox is useful in improvement in the stiffness, discomfort, involuntary movement,
active function, passive function, mobility and balance (Chen, 2012; Dutta et al., 2016).
Experimental plan: Fluorescence Correlation Spectroscopy (FCS) is useful in elucidating
dynamics of SNARE protein which is a main target of botox. Diffussion and interaction are
the two main steps for botox to exhibit its effect. FCS is the important technique to elucidate
3
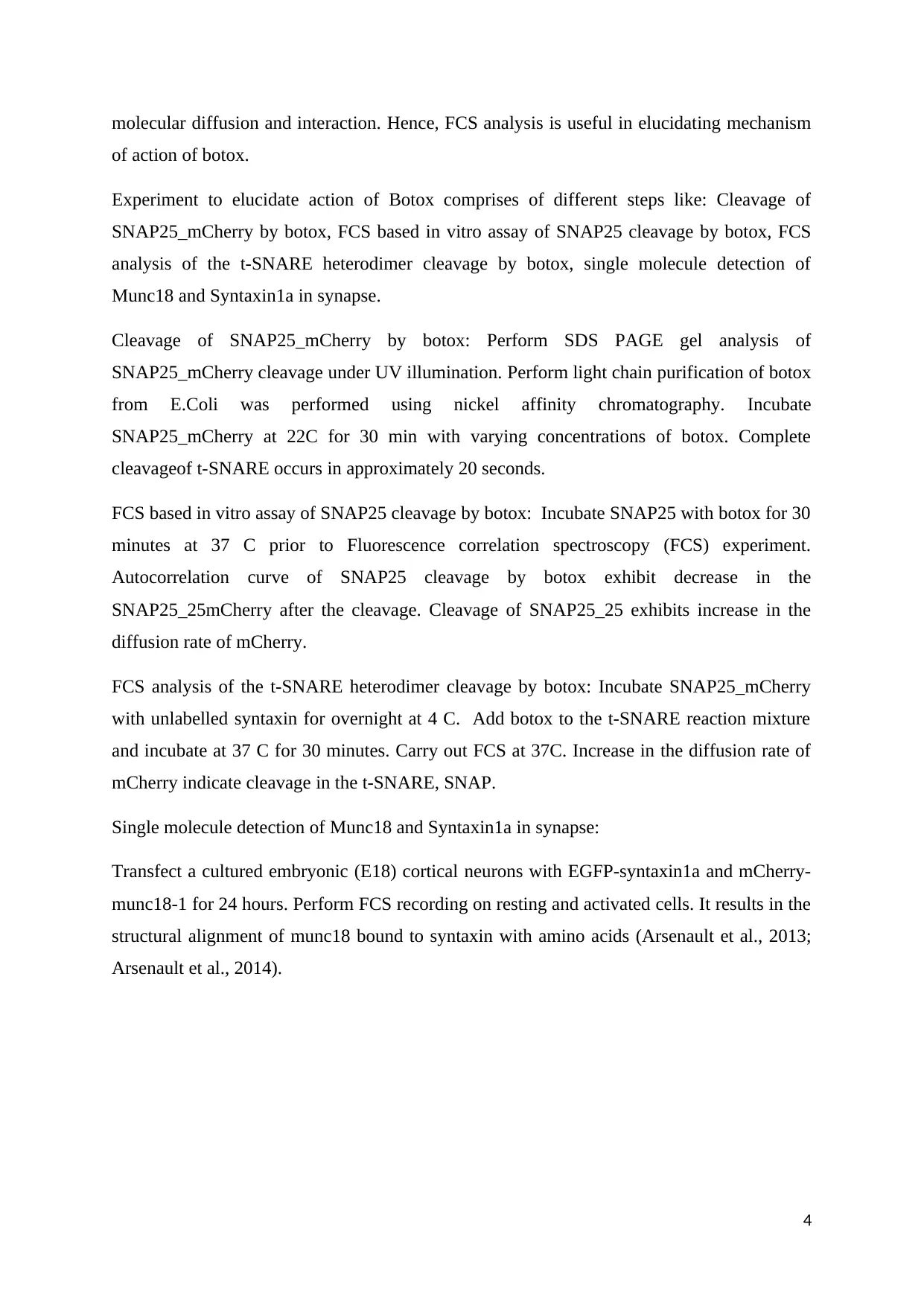
molecular diffusion and interaction. Hence, FCS analysis is useful in elucidating mechanism
of action of botox.
Experiment to elucidate action of Botox comprises of different steps like: Cleavage of
SNAP25_mCherry by botox, FCS based in vitro assay of SNAP25 cleavage by botox, FCS
analysis of the t-SNARE heterodimer cleavage by botox, single molecule detection of
Munc18 and Syntaxin1a in synapse.
Cleavage of SNAP25_mCherry by botox: Perform SDS PAGE gel analysis of
SNAP25_mCherry cleavage under UV illumination. Perform light chain purification of botox
from E.Coli was performed using nickel affinity chromatography. Incubate
SNAP25_mCherry at 22C for 30 min with varying concentrations of botox. Complete
cleavageof t-SNARE occurs in approximately 20 seconds.
FCS based in vitro assay of SNAP25 cleavage by botox: Incubate SNAP25 with botox for 30
minutes at 37 C prior to Fluorescence correlation spectroscopy (FCS) experiment.
Autocorrelation curve of SNAP25 cleavage by botox exhibit decrease in the
SNAP25_25mCherry after the cleavage. Cleavage of SNAP25_25 exhibits increase in the
diffusion rate of mCherry.
FCS analysis of the t-SNARE heterodimer cleavage by botox: Incubate SNAP25_mCherry
with unlabelled syntaxin for overnight at 4 C. Add botox to the t-SNARE reaction mixture
and incubate at 37 C for 30 minutes. Carry out FCS at 37C. Increase in the diffusion rate of
mCherry indicate cleavage in the t-SNARE, SNAP.
Single molecule detection of Munc18 and Syntaxin1a in synapse:
Transfect a cultured embryonic (E18) cortical neurons with EGFP-syntaxin1a and mCherry-
munc18-1 for 24 hours. Perform FCS recording on resting and activated cells. It results in the
structural alignment of munc18 bound to syntaxin with amino acids (Arsenault et al., 2013;
Arsenault et al., 2014).
4
of action of botox.
Experiment to elucidate action of Botox comprises of different steps like: Cleavage of
SNAP25_mCherry by botox, FCS based in vitro assay of SNAP25 cleavage by botox, FCS
analysis of the t-SNARE heterodimer cleavage by botox, single molecule detection of
Munc18 and Syntaxin1a in synapse.
Cleavage of SNAP25_mCherry by botox: Perform SDS PAGE gel analysis of
SNAP25_mCherry cleavage under UV illumination. Perform light chain purification of botox
from E.Coli was performed using nickel affinity chromatography. Incubate
SNAP25_mCherry at 22C for 30 min with varying concentrations of botox. Complete
cleavageof t-SNARE occurs in approximately 20 seconds.
FCS based in vitro assay of SNAP25 cleavage by botox: Incubate SNAP25 with botox for 30
minutes at 37 C prior to Fluorescence correlation spectroscopy (FCS) experiment.
Autocorrelation curve of SNAP25 cleavage by botox exhibit decrease in the
SNAP25_25mCherry after the cleavage. Cleavage of SNAP25_25 exhibits increase in the
diffusion rate of mCherry.
FCS analysis of the t-SNARE heterodimer cleavage by botox: Incubate SNAP25_mCherry
with unlabelled syntaxin for overnight at 4 C. Add botox to the t-SNARE reaction mixture
and incubate at 37 C for 30 minutes. Carry out FCS at 37C. Increase in the diffusion rate of
mCherry indicate cleavage in the t-SNARE, SNAP.
Single molecule detection of Munc18 and Syntaxin1a in synapse:
Transfect a cultured embryonic (E18) cortical neurons with EGFP-syntaxin1a and mCherry-
munc18-1 for 24 hours. Perform FCS recording on resting and activated cells. It results in the
structural alignment of munc18 bound to syntaxin with amino acids (Arsenault et al., 2013;
Arsenault et al., 2014).
4
Secure Best Marks with AI Grader
Need help grading? Try our AI Grader for instant feedback on your assignments.
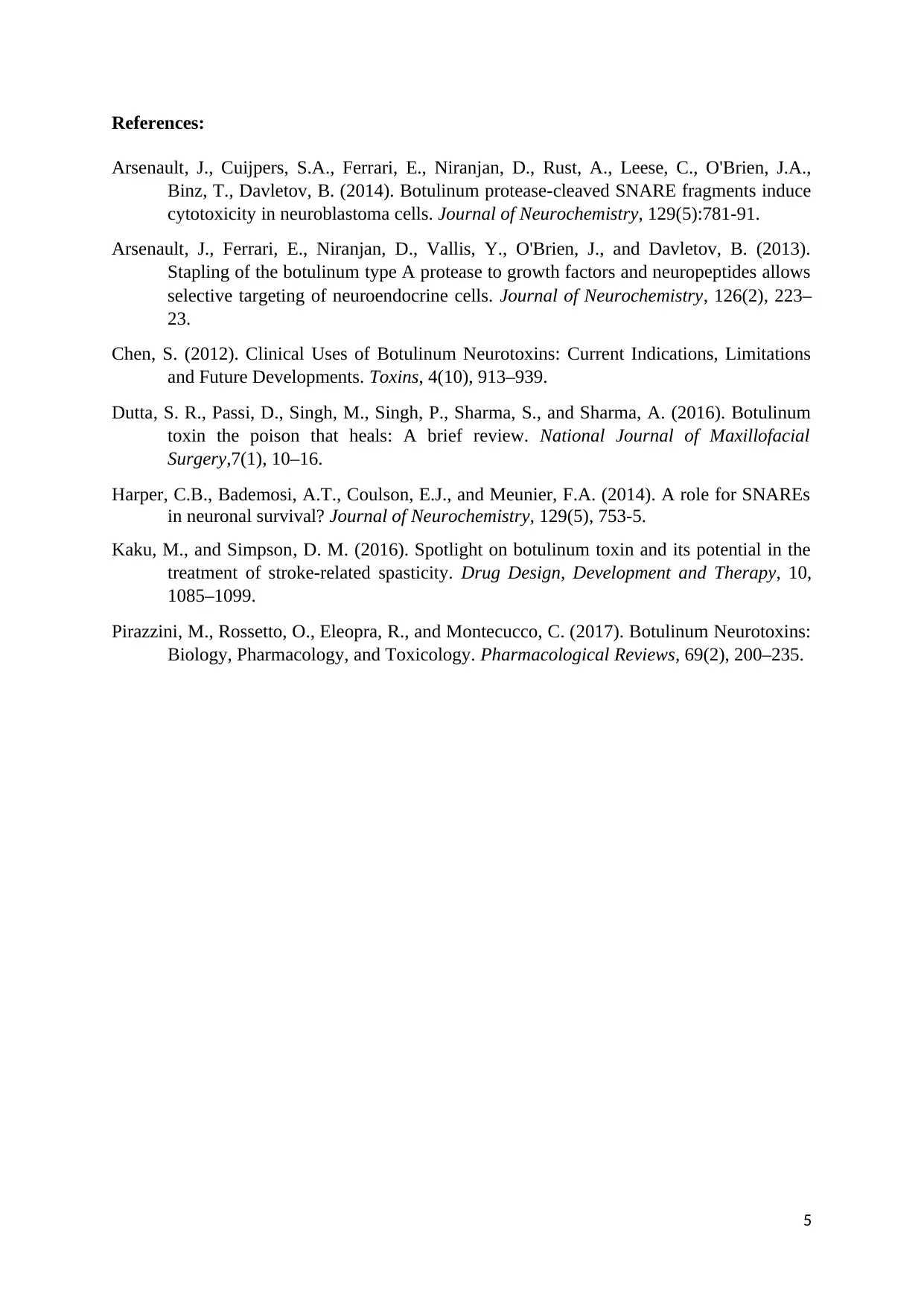
References:
Arsenault, J., Cuijpers, S.A., Ferrari, E., Niranjan, D., Rust, A., Leese, C., O'Brien, J.A.,
Binz, T., Davletov, B. (2014). Botulinum protease-cleaved SNARE fragments induce
cytotoxicity in neuroblastoma cells. Journal of Neurochemistry, 129(5):781-91.
Arsenault, J., Ferrari, E., Niranjan, D., Vallis, Y., O'Brien, J., and Davletov, B. (2013).
Stapling of the botulinum type A protease to growth factors and neuropeptides allows
selective targeting of neuroendocrine cells. Journal of Neurochemistry, 126(2), 223–
23.
Chen, S. (2012). Clinical Uses of Botulinum Neurotoxins: Current Indications, Limitations
and Future Developments. Toxins, 4(10), 913–939.
Dutta, S. R., Passi, D., Singh, M., Singh, P., Sharma, S., and Sharma, A. (2016). Botulinum
toxin the poison that heals: A brief review. National Journal of Maxillofacial
Surgery,7(1), 10–16.
Harper, C.B., Bademosi, A.T., Coulson, E.J., and Meunier, F.A. (2014). A role for SNAREs
in neuronal survival? Journal of Neurochemistry, 129(5), 753-5.
Kaku, M., and Simpson, D. M. (2016). Spotlight on botulinum toxin and its potential in the
treatment of stroke-related spasticity. Drug Design, Development and Therapy, 10,
1085–1099.
Pirazzini, M., Rossetto, O., Eleopra, R., and Montecucco, C. (2017). Botulinum Neurotoxins:
Biology, Pharmacology, and Toxicology. Pharmacological Reviews, 69(2), 200–235.
5
Arsenault, J., Cuijpers, S.A., Ferrari, E., Niranjan, D., Rust, A., Leese, C., O'Brien, J.A.,
Binz, T., Davletov, B. (2014). Botulinum protease-cleaved SNARE fragments induce
cytotoxicity in neuroblastoma cells. Journal of Neurochemistry, 129(5):781-91.
Arsenault, J., Ferrari, E., Niranjan, D., Vallis, Y., O'Brien, J., and Davletov, B. (2013).
Stapling of the botulinum type A protease to growth factors and neuropeptides allows
selective targeting of neuroendocrine cells. Journal of Neurochemistry, 126(2), 223–
23.
Chen, S. (2012). Clinical Uses of Botulinum Neurotoxins: Current Indications, Limitations
and Future Developments. Toxins, 4(10), 913–939.
Dutta, S. R., Passi, D., Singh, M., Singh, P., Sharma, S., and Sharma, A. (2016). Botulinum
toxin the poison that heals: A brief review. National Journal of Maxillofacial
Surgery,7(1), 10–16.
Harper, C.B., Bademosi, A.T., Coulson, E.J., and Meunier, F.A. (2014). A role for SNAREs
in neuronal survival? Journal of Neurochemistry, 129(5), 753-5.
Kaku, M., and Simpson, D. M. (2016). Spotlight on botulinum toxin and its potential in the
treatment of stroke-related spasticity. Drug Design, Development and Therapy, 10,
1085–1099.
Pirazzini, M., Rossetto, O., Eleopra, R., and Montecucco, C. (2017). Botulinum Neurotoxins:
Biology, Pharmacology, and Toxicology. Pharmacological Reviews, 69(2), 200–235.
5
1 out of 5
Related Documents
![[object Object]](/_next/image/?url=%2F_next%2Fstatic%2Fmedia%2Flogo.6d15ce61.png&w=640&q=75)
Your All-in-One AI-Powered Toolkit for Academic Success.
+13062052269
info@desklib.com
Available 24*7 on WhatsApp / Email
Unlock your academic potential
© 2024 | Zucol Services PVT LTD | All rights reserved.