Additive Manufacturing and Thermal Analysis of Ti-6Al-4V using ANSYS Software
VerifiedAdded on 2023/04/11
|18
|4150
|394
AI Summary
This paper discusses the use of additive manufacturing in the production of complex-shaped components of metals, with a focus on the development of a model for selective laser melting of Ti-6Al-4V using ANSYS software. The study aims to predict the distribution of temperature and induced residual stresses in the process. The research strategy involves in situ measurements of temperature and distortion, as well as comparison of different scanning strategies. The resource requirements include the use of ANSYS software and a heat source model. The paper also includes a literature review on the properties and applications of Ti-6Al-4V alloy.
Contribute Materials
Your contribution can guide someone’s learning journey. Share your
documents today.
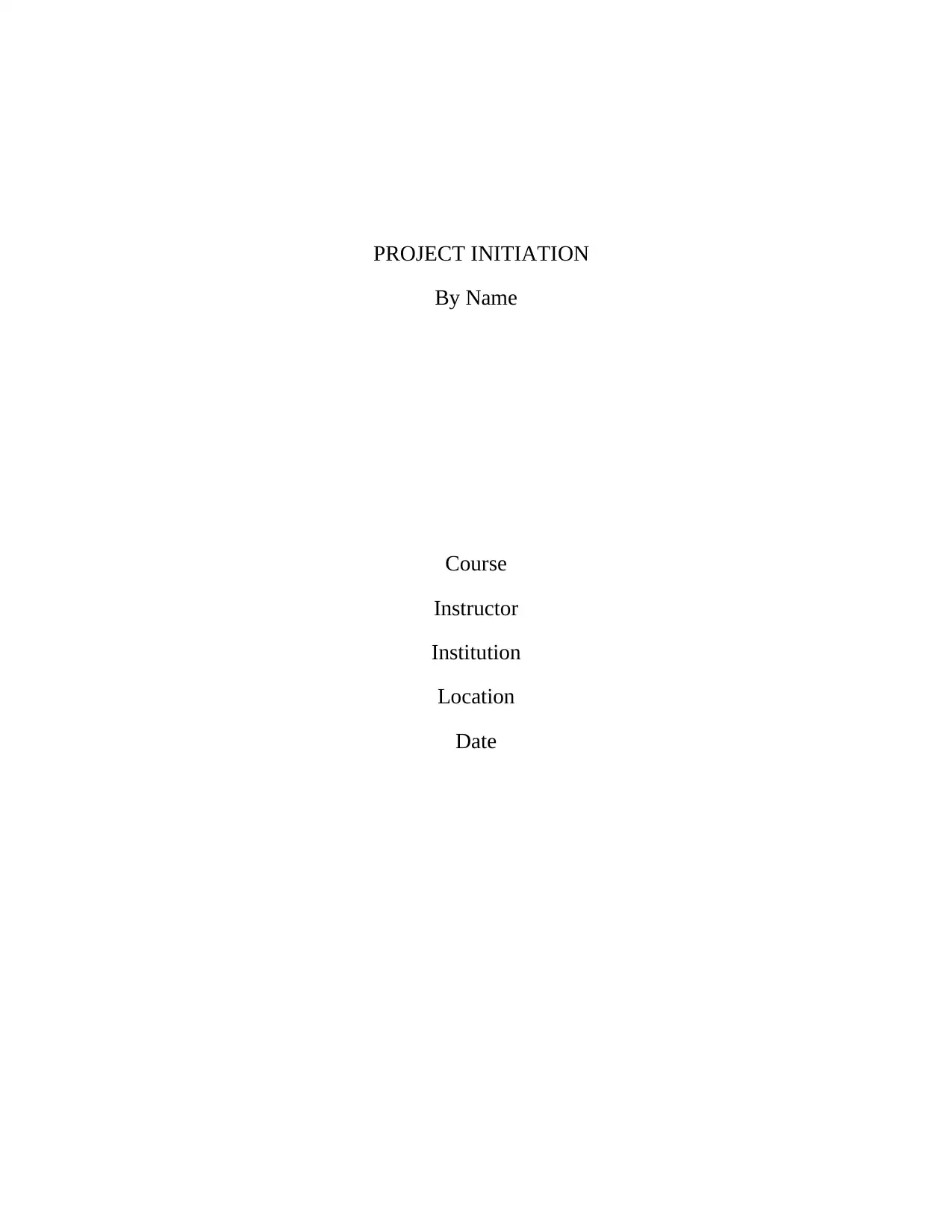
PROJECT INITIATION
By Name
Course
Instructor
Institution
Location
Date
By Name
Course
Instructor
Institution
Location
Date
Secure Best Marks with AI Grader
Need help grading? Try our AI Grader for instant feedback on your assignments.
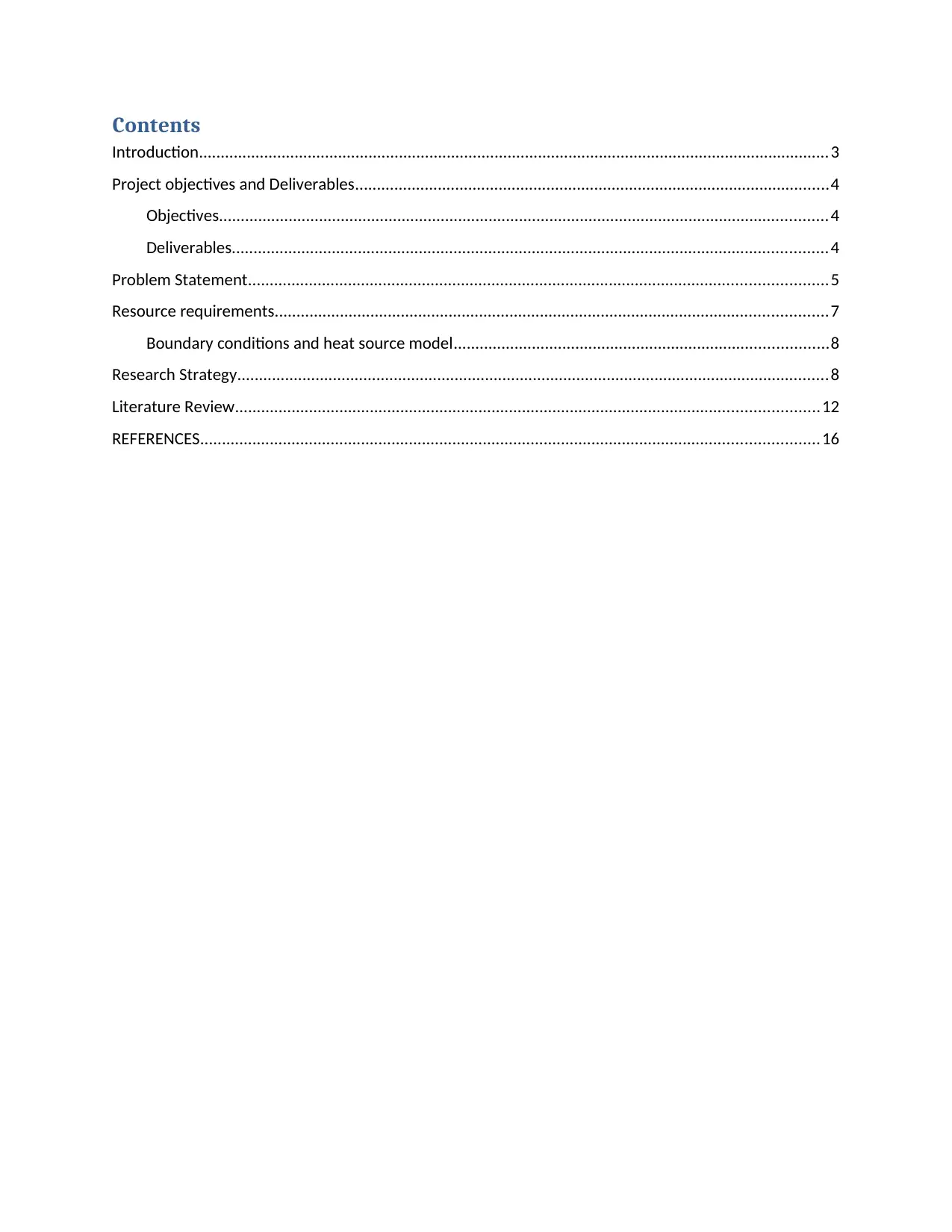
Contents
Introduction.................................................................................................................................................3
Project objectives and Deliverables.............................................................................................................4
Objectives............................................................................................................................................4
Deliverables.........................................................................................................................................4
Problem Statement.....................................................................................................................................5
Resource requirements...............................................................................................................................7
Boundary conditions and heat source model......................................................................................8
Research Strategy........................................................................................................................................8
Literature Review......................................................................................................................................12
REFERENCES..............................................................................................................................................16
Introduction.................................................................................................................................................3
Project objectives and Deliverables.............................................................................................................4
Objectives............................................................................................................................................4
Deliverables.........................................................................................................................................4
Problem Statement.....................................................................................................................................5
Resource requirements...............................................................................................................................7
Boundary conditions and heat source model......................................................................................8
Research Strategy........................................................................................................................................8
Literature Review......................................................................................................................................12
REFERENCES..............................................................................................................................................16
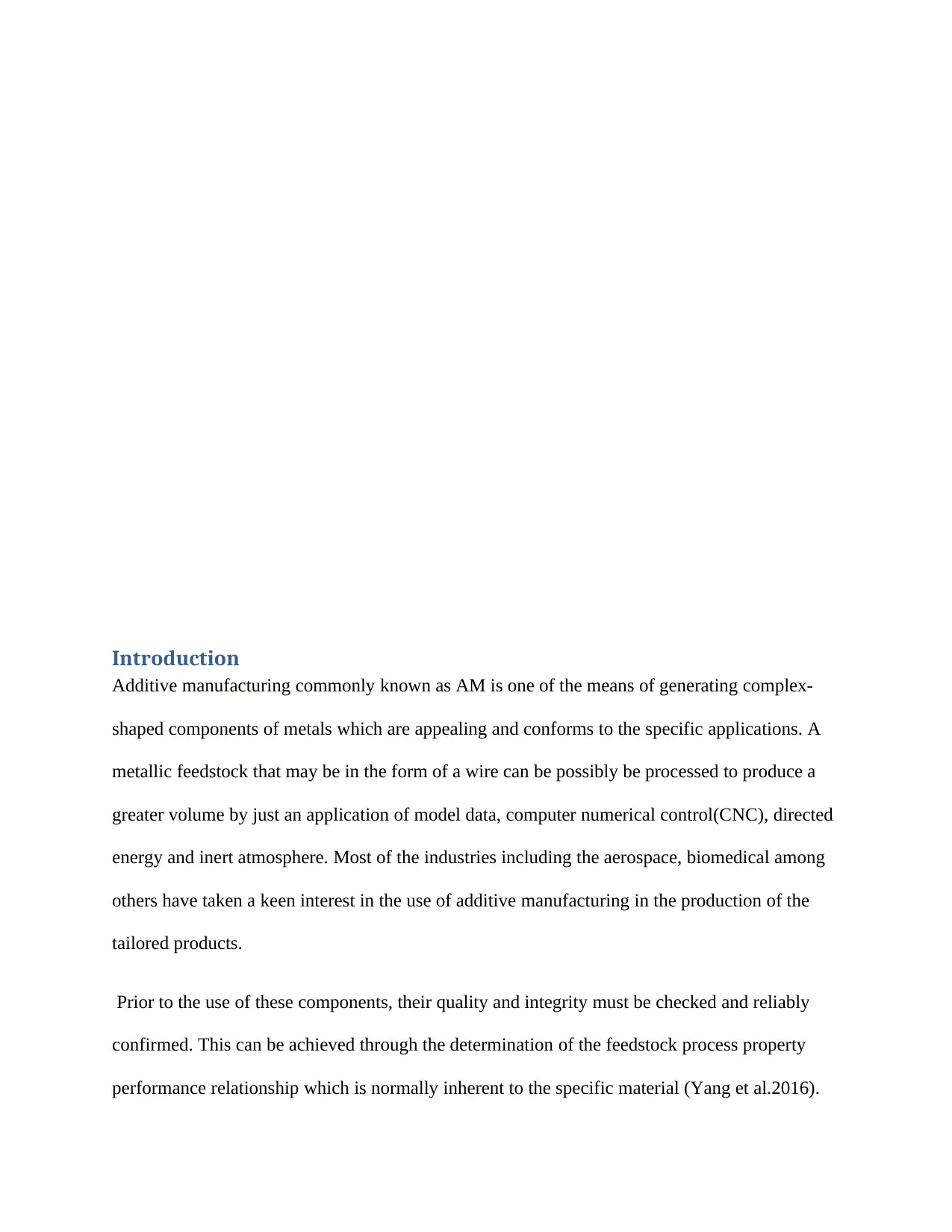
Introduction
Additive manufacturing commonly known as AM is one of the means of generating complex-
shaped components of metals which are appealing and conforms to the specific applications. A
metallic feedstock that may be in the form of a wire can be possibly be processed to produce a
greater volume by just an application of model data, computer numerical control(CNC), directed
energy and inert atmosphere. Most of the industries including the aerospace, biomedical among
others have taken a keen interest in the use of additive manufacturing in the production of the
tailored products.
Prior to the use of these components, their quality and integrity must be checked and reliably
confirmed. This can be achieved through the determination of the feedstock process property
performance relationship which is normally inherent to the specific material (Yang et al.2016).
Additive manufacturing commonly known as AM is one of the means of generating complex-
shaped components of metals which are appealing and conforms to the specific applications. A
metallic feedstock that may be in the form of a wire can be possibly be processed to produce a
greater volume by just an application of model data, computer numerical control(CNC), directed
energy and inert atmosphere. Most of the industries including the aerospace, biomedical among
others have taken a keen interest in the use of additive manufacturing in the production of the
tailored products.
Prior to the use of these components, their quality and integrity must be checked and reliably
confirmed. This can be achieved through the determination of the feedstock process property
performance relationship which is normally inherent to the specific material (Yang et al.2016).
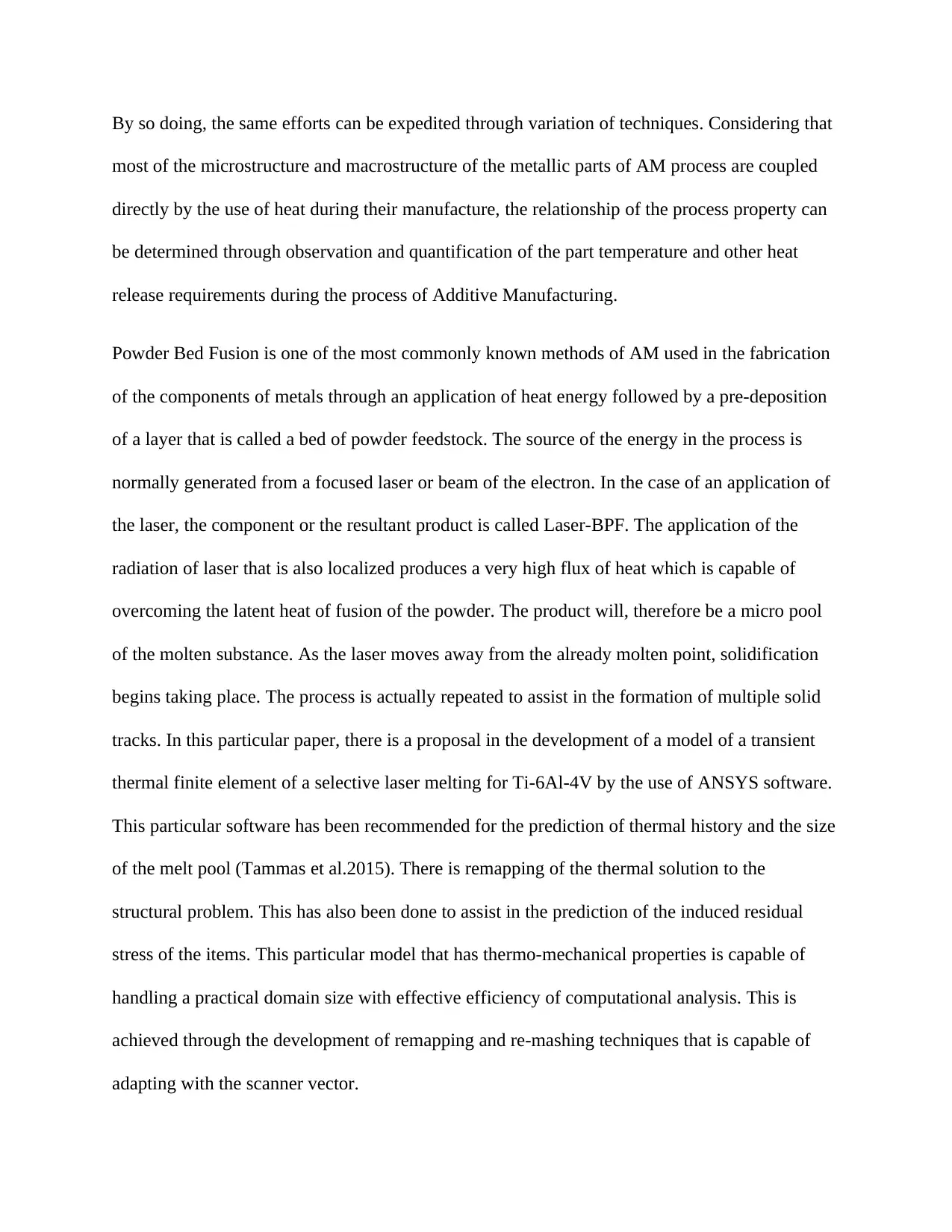
By so doing, the same efforts can be expedited through variation of techniques. Considering that
most of the microstructure and macrostructure of the metallic parts of AM process are coupled
directly by the use of heat during their manufacture, the relationship of the process property can
be determined through observation and quantification of the part temperature and other heat
release requirements during the process of Additive Manufacturing.
Powder Bed Fusion is one of the most commonly known methods of AM used in the fabrication
of the components of metals through an application of heat energy followed by a pre-deposition
of a layer that is called a bed of powder feedstock. The source of the energy in the process is
normally generated from a focused laser or beam of the electron. In the case of an application of
the laser, the component or the resultant product is called Laser-BPF. The application of the
radiation of laser that is also localized produces a very high flux of heat which is capable of
overcoming the latent heat of fusion of the powder. The product will, therefore be a micro pool
of the molten substance. As the laser moves away from the already molten point, solidification
begins taking place. The process is actually repeated to assist in the formation of multiple solid
tracks. In this particular paper, there is a proposal in the development of a model of a transient
thermal finite element of a selective laser melting for Ti-6Al-4V by the use of ANSYS software.
This particular software has been recommended for the prediction of thermal history and the size
of the melt pool (Tammas et al.2015). There is remapping of the thermal solution to the
structural problem. This has also been done to assist in the prediction of the induced residual
stress of the items. This particular model that has thermo-mechanical properties is capable of
handling a practical domain size with effective efficiency of computational analysis. This is
achieved through the development of remapping and re-mashing techniques that is capable of
adapting with the scanner vector.
most of the microstructure and macrostructure of the metallic parts of AM process are coupled
directly by the use of heat during their manufacture, the relationship of the process property can
be determined through observation and quantification of the part temperature and other heat
release requirements during the process of Additive Manufacturing.
Powder Bed Fusion is one of the most commonly known methods of AM used in the fabrication
of the components of metals through an application of heat energy followed by a pre-deposition
of a layer that is called a bed of powder feedstock. The source of the energy in the process is
normally generated from a focused laser or beam of the electron. In the case of an application of
the laser, the component or the resultant product is called Laser-BPF. The application of the
radiation of laser that is also localized produces a very high flux of heat which is capable of
overcoming the latent heat of fusion of the powder. The product will, therefore be a micro pool
of the molten substance. As the laser moves away from the already molten point, solidification
begins taking place. The process is actually repeated to assist in the formation of multiple solid
tracks. In this particular paper, there is a proposal in the development of a model of a transient
thermal finite element of a selective laser melting for Ti-6Al-4V by the use of ANSYS software.
This particular software has been recommended for the prediction of thermal history and the size
of the melt pool (Tammas et al.2015). There is remapping of the thermal solution to the
structural problem. This has also been done to assist in the prediction of the induced residual
stress of the items. This particular model that has thermo-mechanical properties is capable of
handling a practical domain size with effective efficiency of computational analysis. This is
achieved through the development of remapping and re-mashing techniques that is capable of
adapting with the scanner vector.
Paraphrase This Document
Need a fresh take? Get an instant paraphrase of this document with our AI Paraphraser
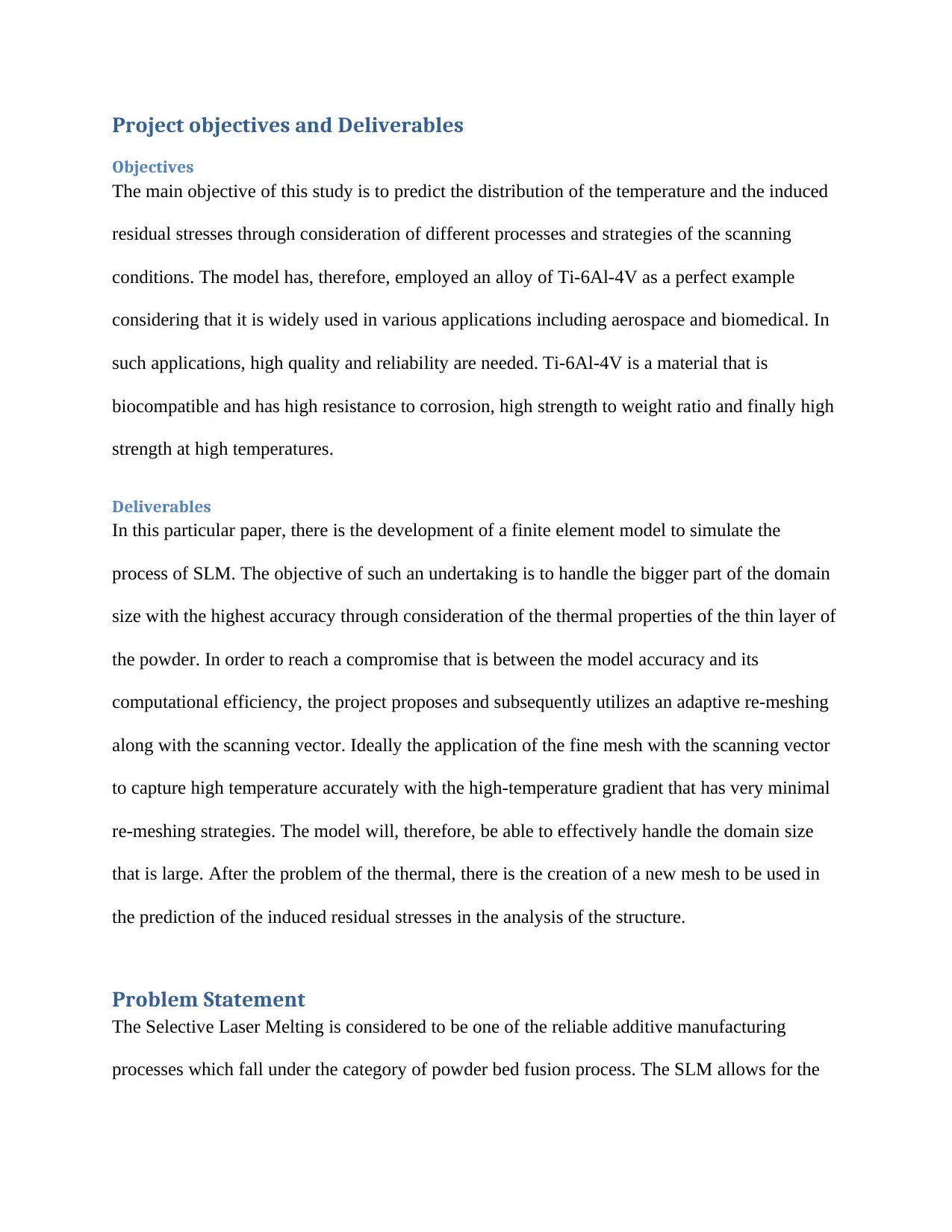
Project objectives and Deliverables
Objectives
The main objective of this study is to predict the distribution of the temperature and the induced
residual stresses through consideration of different processes and strategies of the scanning
conditions. The model has, therefore, employed an alloy of Ti-6Al-4V as a perfect example
considering that it is widely used in various applications including aerospace and biomedical. In
such applications, high quality and reliability are needed. Ti-6Al-4V is a material that is
biocompatible and has high resistance to corrosion, high strength to weight ratio and finally high
strength at high temperatures.
Deliverables
In this particular paper, there is the development of a finite element model to simulate the
process of SLM. The objective of such an undertaking is to handle the bigger part of the domain
size with the highest accuracy through consideration of the thermal properties of the thin layer of
the powder. In order to reach a compromise that is between the model accuracy and its
computational efficiency, the project proposes and subsequently utilizes an adaptive re-meshing
along with the scanning vector. Ideally the application of the fine mesh with the scanning vector
to capture high temperature accurately with the high-temperature gradient that has very minimal
re-meshing strategies. The model will, therefore, be able to effectively handle the domain size
that is large. After the problem of the thermal, there is the creation of a new mesh to be used in
the prediction of the induced residual stresses in the analysis of the structure.
Problem Statement
The Selective Laser Melting is considered to be one of the reliable additive manufacturing
processes which fall under the category of powder bed fusion process. The SLM allows for the
Objectives
The main objective of this study is to predict the distribution of the temperature and the induced
residual stresses through consideration of different processes and strategies of the scanning
conditions. The model has, therefore, employed an alloy of Ti-6Al-4V as a perfect example
considering that it is widely used in various applications including aerospace and biomedical. In
such applications, high quality and reliability are needed. Ti-6Al-4V is a material that is
biocompatible and has high resistance to corrosion, high strength to weight ratio and finally high
strength at high temperatures.
Deliverables
In this particular paper, there is the development of a finite element model to simulate the
process of SLM. The objective of such an undertaking is to handle the bigger part of the domain
size with the highest accuracy through consideration of the thermal properties of the thin layer of
the powder. In order to reach a compromise that is between the model accuracy and its
computational efficiency, the project proposes and subsequently utilizes an adaptive re-meshing
along with the scanning vector. Ideally the application of the fine mesh with the scanning vector
to capture high temperature accurately with the high-temperature gradient that has very minimal
re-meshing strategies. The model will, therefore, be able to effectively handle the domain size
that is large. After the problem of the thermal, there is the creation of a new mesh to be used in
the prediction of the induced residual stresses in the analysis of the structure.
Problem Statement
The Selective Laser Melting is considered to be one of the reliable additive manufacturing
processes which fall under the category of powder bed fusion process. The SLM allows for the
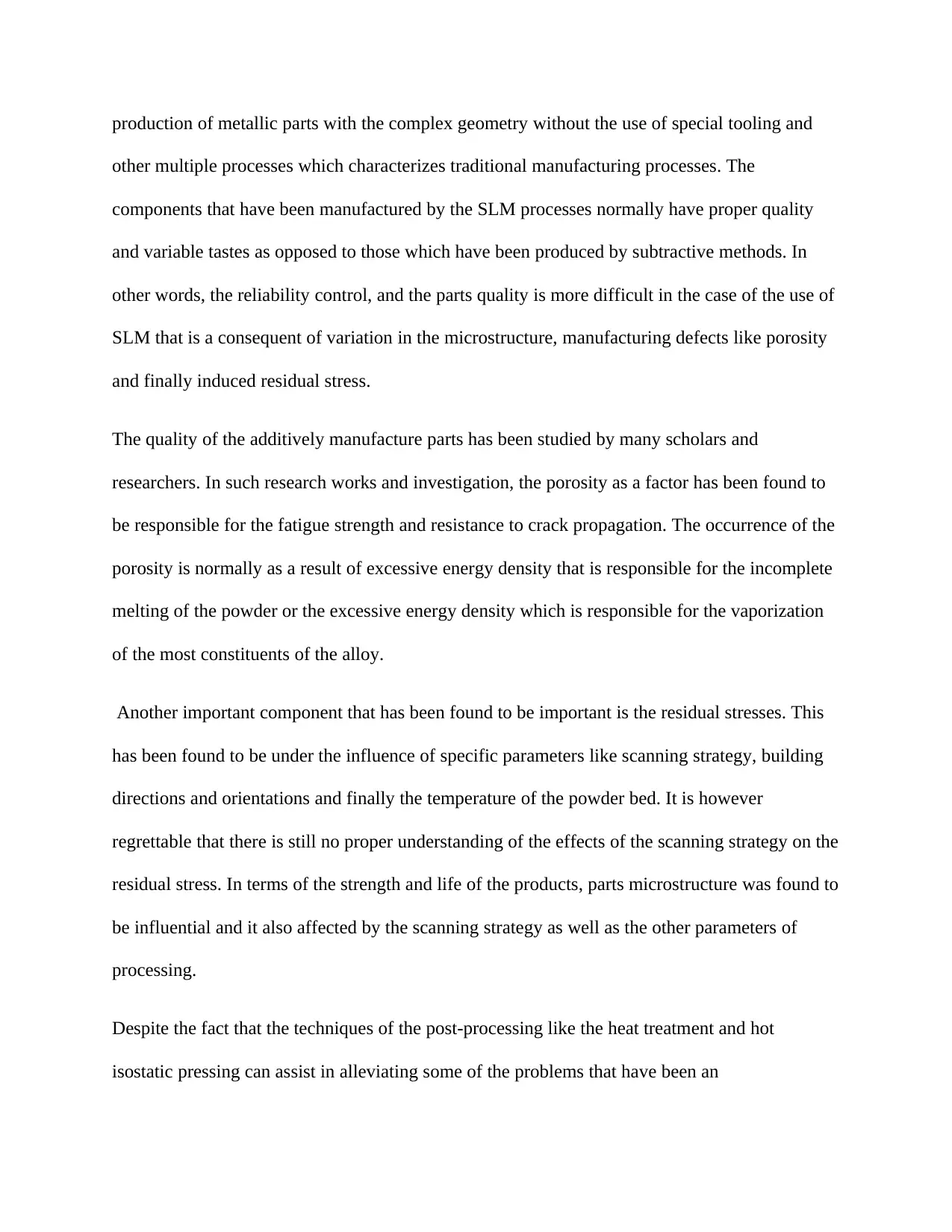
production of metallic parts with the complex geometry without the use of special tooling and
other multiple processes which characterizes traditional manufacturing processes. The
components that have been manufactured by the SLM processes normally have proper quality
and variable tastes as opposed to those which have been produced by subtractive methods. In
other words, the reliability control, and the parts quality is more difficult in the case of the use of
SLM that is a consequent of variation in the microstructure, manufacturing defects like porosity
and finally induced residual stress.
The quality of the additively manufacture parts has been studied by many scholars and
researchers. In such research works and investigation, the porosity as a factor has been found to
be responsible for the fatigue strength and resistance to crack propagation. The occurrence of the
porosity is normally as a result of excessive energy density that is responsible for the incomplete
melting of the powder or the excessive energy density which is responsible for the vaporization
of the most constituents of the alloy.
Another important component that has been found to be important is the residual stresses. This
has been found to be under the influence of specific parameters like scanning strategy, building
directions and orientations and finally the temperature of the powder bed. It is however
regrettable that there is still no proper understanding of the effects of the scanning strategy on the
residual stress. In terms of the strength and life of the products, parts microstructure was found to
be influential and it also affected by the scanning strategy as well as the other parameters of
processing.
Despite the fact that the techniques of the post-processing like the heat treatment and hot
isostatic pressing can assist in alleviating some of the problems that have been an
other multiple processes which characterizes traditional manufacturing processes. The
components that have been manufactured by the SLM processes normally have proper quality
and variable tastes as opposed to those which have been produced by subtractive methods. In
other words, the reliability control, and the parts quality is more difficult in the case of the use of
SLM that is a consequent of variation in the microstructure, manufacturing defects like porosity
and finally induced residual stress.
The quality of the additively manufacture parts has been studied by many scholars and
researchers. In such research works and investigation, the porosity as a factor has been found to
be responsible for the fatigue strength and resistance to crack propagation. The occurrence of the
porosity is normally as a result of excessive energy density that is responsible for the incomplete
melting of the powder or the excessive energy density which is responsible for the vaporization
of the most constituents of the alloy.
Another important component that has been found to be important is the residual stresses. This
has been found to be under the influence of specific parameters like scanning strategy, building
directions and orientations and finally the temperature of the powder bed. It is however
regrettable that there is still no proper understanding of the effects of the scanning strategy on the
residual stress. In terms of the strength and life of the products, parts microstructure was found to
be influential and it also affected by the scanning strategy as well as the other parameters of
processing.
Despite the fact that the techniques of the post-processing like the heat treatment and hot
isostatic pressing can assist in alleviating some of the problems that have been an
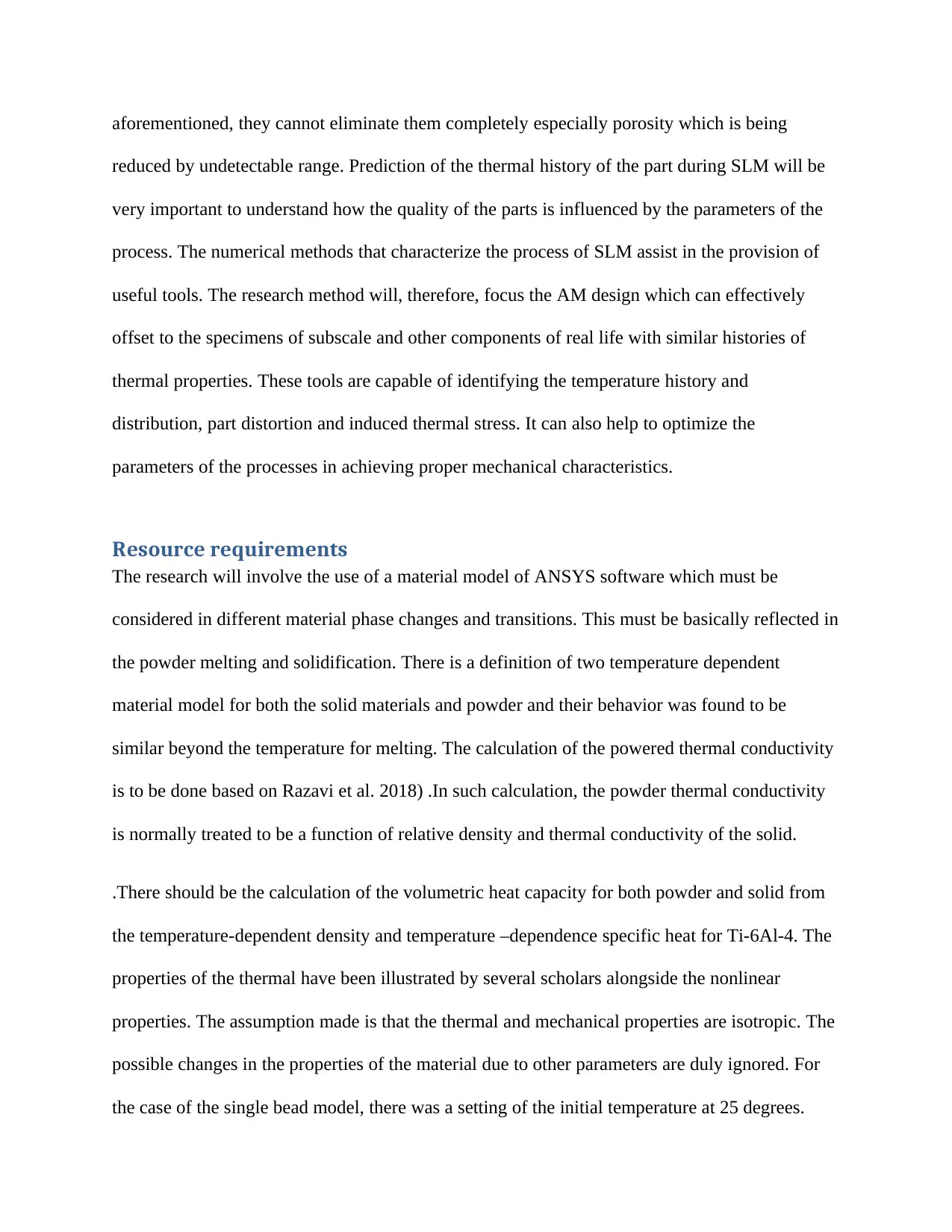
aforementioned, they cannot eliminate them completely especially porosity which is being
reduced by undetectable range. Prediction of the thermal history of the part during SLM will be
very important to understand how the quality of the parts is influenced by the parameters of the
process. The numerical methods that characterize the process of SLM assist in the provision of
useful tools. The research method will, therefore, focus the AM design which can effectively
offset to the specimens of subscale and other components of real life with similar histories of
thermal properties. These tools are capable of identifying the temperature history and
distribution, part distortion and induced thermal stress. It can also help to optimize the
parameters of the processes in achieving proper mechanical characteristics.
Resource requirements
The research will involve the use of a material model of ANSYS software which must be
considered in different material phase changes and transitions. This must be basically reflected in
the powder melting and solidification. There is a definition of two temperature dependent
material model for both the solid materials and powder and their behavior was found to be
similar beyond the temperature for melting. The calculation of the powered thermal conductivity
is to be done based on Razavi et al. 2018) .In such calculation, the powder thermal conductivity
is normally treated to be a function of relative density and thermal conductivity of the solid.
.There should be the calculation of the volumetric heat capacity for both powder and solid from
the temperature-dependent density and temperature –dependence specific heat for Ti-6Al-4. The
properties of the thermal have been illustrated by several scholars alongside the nonlinear
properties. The assumption made is that the thermal and mechanical properties are isotropic. The
possible changes in the properties of the material due to other parameters are duly ignored. For
the case of the single bead model, there was a setting of the initial temperature at 25 degrees.
reduced by undetectable range. Prediction of the thermal history of the part during SLM will be
very important to understand how the quality of the parts is influenced by the parameters of the
process. The numerical methods that characterize the process of SLM assist in the provision of
useful tools. The research method will, therefore, focus the AM design which can effectively
offset to the specimens of subscale and other components of real life with similar histories of
thermal properties. These tools are capable of identifying the temperature history and
distribution, part distortion and induced thermal stress. It can also help to optimize the
parameters of the processes in achieving proper mechanical characteristics.
Resource requirements
The research will involve the use of a material model of ANSYS software which must be
considered in different material phase changes and transitions. This must be basically reflected in
the powder melting and solidification. There is a definition of two temperature dependent
material model for both the solid materials and powder and their behavior was found to be
similar beyond the temperature for melting. The calculation of the powered thermal conductivity
is to be done based on Razavi et al. 2018) .In such calculation, the powder thermal conductivity
is normally treated to be a function of relative density and thermal conductivity of the solid.
.There should be the calculation of the volumetric heat capacity for both powder and solid from
the temperature-dependent density and temperature –dependence specific heat for Ti-6Al-4. The
properties of the thermal have been illustrated by several scholars alongside the nonlinear
properties. The assumption made is that the thermal and mechanical properties are isotropic. The
possible changes in the properties of the material due to other parameters are duly ignored. For
the case of the single bead model, there was a setting of the initial temperature at 25 degrees.
Secure Best Marks with AI Grader
Need help grading? Try our AI Grader for instant feedback on your assignments.
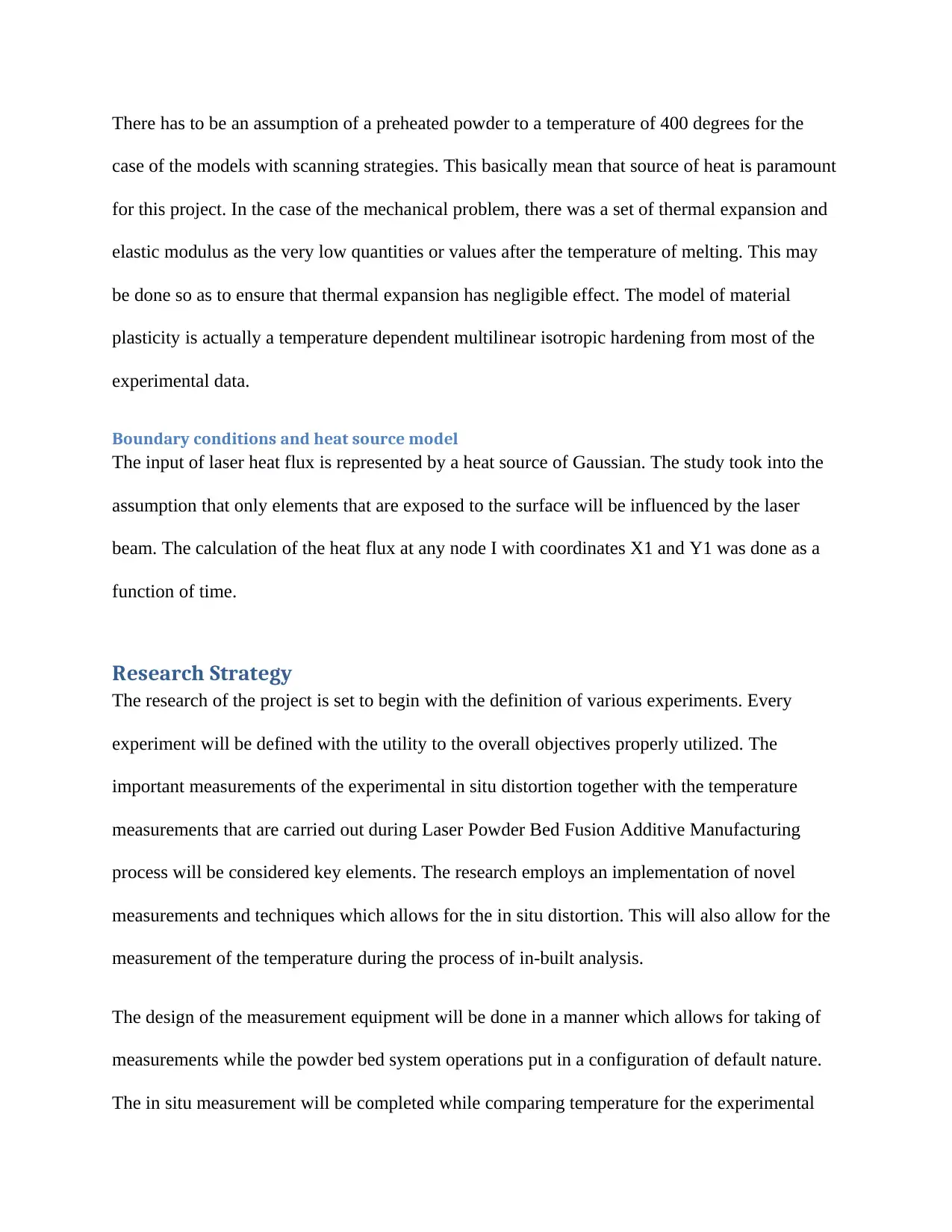
There has to be an assumption of a preheated powder to a temperature of 400 degrees for the
case of the models with scanning strategies. This basically mean that source of heat is paramount
for this project. In the case of the mechanical problem, there was a set of thermal expansion and
elastic modulus as the very low quantities or values after the temperature of melting. This may
be done so as to ensure that thermal expansion has negligible effect. The model of material
plasticity is actually a temperature dependent multilinear isotropic hardening from most of the
experimental data.
Boundary conditions and heat source model
The input of laser heat flux is represented by a heat source of Gaussian. The study took into the
assumption that only elements that are exposed to the surface will be influenced by the laser
beam. The calculation of the heat flux at any node I with coordinates X1 and Y1 was done as a
function of time.
Research Strategy
The research of the project is set to begin with the definition of various experiments. Every
experiment will be defined with the utility to the overall objectives properly utilized. The
important measurements of the experimental in situ distortion together with the temperature
measurements that are carried out during Laser Powder Bed Fusion Additive Manufacturing
process will be considered key elements. The research employs an implementation of novel
measurements and techniques which allows for the in situ distortion. This will also allow for the
measurement of the temperature during the process of in-built analysis.
The design of the measurement equipment will be done in a manner which allows for taking of
measurements while the powder bed system operations put in a configuration of default nature.
The in situ measurement will be completed while comparing temperature for the experimental
case of the models with scanning strategies. This basically mean that source of heat is paramount
for this project. In the case of the mechanical problem, there was a set of thermal expansion and
elastic modulus as the very low quantities or values after the temperature of melting. This may
be done so as to ensure that thermal expansion has negligible effect. The model of material
plasticity is actually a temperature dependent multilinear isotropic hardening from most of the
experimental data.
Boundary conditions and heat source model
The input of laser heat flux is represented by a heat source of Gaussian. The study took into the
assumption that only elements that are exposed to the surface will be influenced by the laser
beam. The calculation of the heat flux at any node I with coordinates X1 and Y1 was done as a
function of time.
Research Strategy
The research of the project is set to begin with the definition of various experiments. Every
experiment will be defined with the utility to the overall objectives properly utilized. The
important measurements of the experimental in situ distortion together with the temperature
measurements that are carried out during Laser Powder Bed Fusion Additive Manufacturing
process will be considered key elements. The research employs an implementation of novel
measurements and techniques which allows for the in situ distortion. This will also allow for the
measurement of the temperature during the process of in-built analysis.
The design of the measurement equipment will be done in a manner which allows for taking of
measurements while the powder bed system operations put in a configuration of default nature.
The in situ measurement will be completed while comparing temperature for the experimental
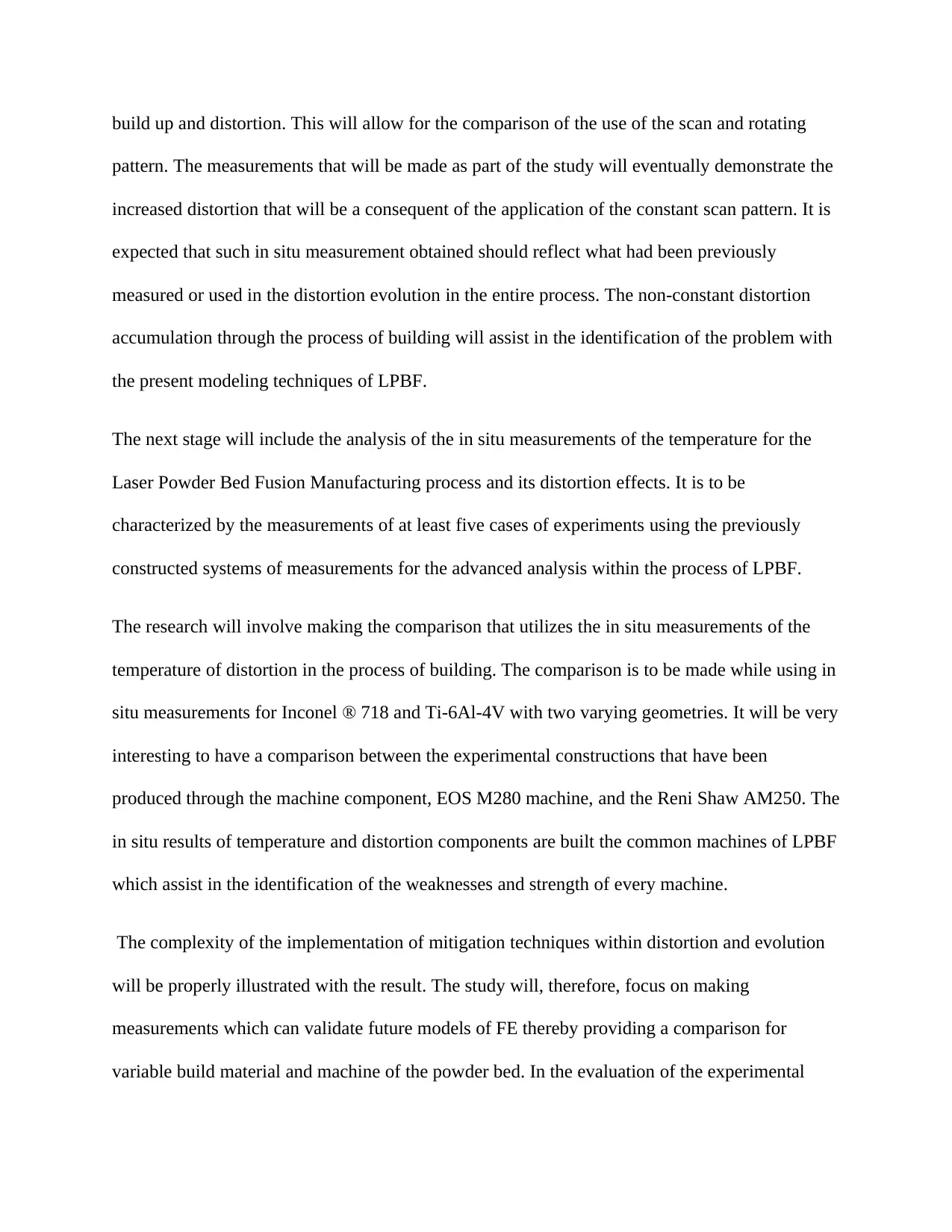
build up and distortion. This will allow for the comparison of the use of the scan and rotating
pattern. The measurements that will be made as part of the study will eventually demonstrate the
increased distortion that will be a consequent of the application of the constant scan pattern. It is
expected that such in situ measurement obtained should reflect what had been previously
measured or used in the distortion evolution in the entire process. The non-constant distortion
accumulation through the process of building will assist in the identification of the problem with
the present modeling techniques of LPBF.
The next stage will include the analysis of the in situ measurements of the temperature for the
Laser Powder Bed Fusion Manufacturing process and its distortion effects. It is to be
characterized by the measurements of at least five cases of experiments using the previously
constructed systems of measurements for the advanced analysis within the process of LPBF.
The research will involve making the comparison that utilizes the in situ measurements of the
temperature of distortion in the process of building. The comparison is to be made while using in
situ measurements for Inconel ® 718 and Ti-6Al-4V with two varying geometries. It will be very
interesting to have a comparison between the experimental constructions that have been
produced through the machine component, EOS M280 machine, and the Reni Shaw AM250. The
in situ results of temperature and distortion components are built the common machines of LPBF
which assist in the identification of the weaknesses and strength of every machine.
The complexity of the implementation of mitigation techniques within distortion and evolution
will be properly illustrated with the result. The study will, therefore, focus on making
measurements which can validate future models of FE thereby providing a comparison for
variable build material and machine of the powder bed. In the evaluation of the experimental
pattern. The measurements that will be made as part of the study will eventually demonstrate the
increased distortion that will be a consequent of the application of the constant scan pattern. It is
expected that such in situ measurement obtained should reflect what had been previously
measured or used in the distortion evolution in the entire process. The non-constant distortion
accumulation through the process of building will assist in the identification of the problem with
the present modeling techniques of LPBF.
The next stage will include the analysis of the in situ measurements of the temperature for the
Laser Powder Bed Fusion Manufacturing process and its distortion effects. It is to be
characterized by the measurements of at least five cases of experiments using the previously
constructed systems of measurements for the advanced analysis within the process of LPBF.
The research will involve making the comparison that utilizes the in situ measurements of the
temperature of distortion in the process of building. The comparison is to be made while using in
situ measurements for Inconel ® 718 and Ti-6Al-4V with two varying geometries. It will be very
interesting to have a comparison between the experimental constructions that have been
produced through the machine component, EOS M280 machine, and the Reni Shaw AM250. The
in situ results of temperature and distortion components are built the common machines of LPBF
which assist in the identification of the weaknesses and strength of every machine.
The complexity of the implementation of mitigation techniques within distortion and evolution
will be properly illustrated with the result. The study will, therefore, focus on making
measurements which can validate future models of FE thereby providing a comparison for
variable build material and machine of the powder bed. In the evaluation of the experimental
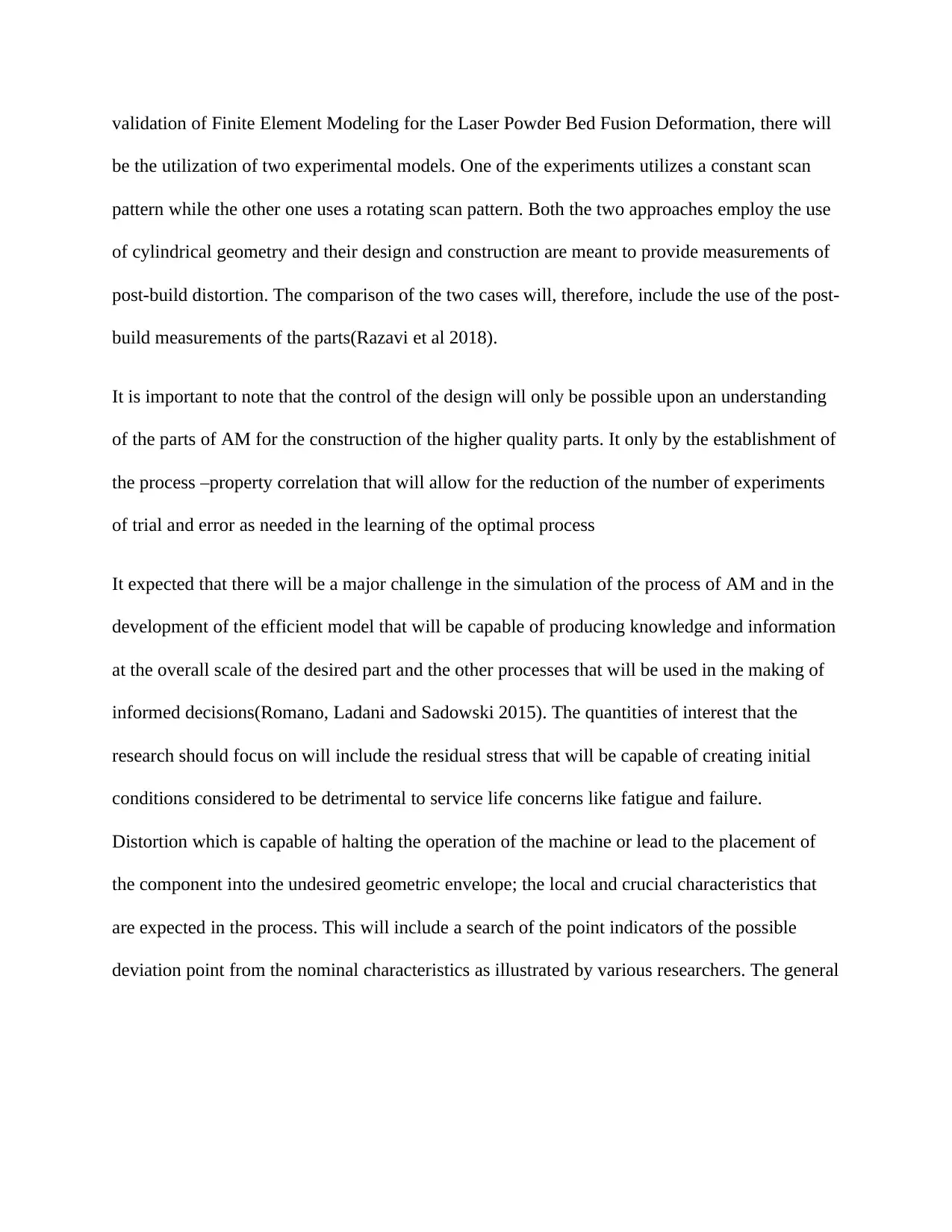
validation of Finite Element Modeling for the Laser Powder Bed Fusion Deformation, there will
be the utilization of two experimental models. One of the experiments utilizes a constant scan
pattern while the other one uses a rotating scan pattern. Both the two approaches employ the use
of cylindrical geometry and their design and construction are meant to provide measurements of
post-build distortion. The comparison of the two cases will, therefore, include the use of the post-
build measurements of the parts(Razavi et al 2018).
It is important to note that the control of the design will only be possible upon an understanding
of the parts of AM for the construction of the higher quality parts. It only by the establishment of
the process –property correlation that will allow for the reduction of the number of experiments
of trial and error as needed in the learning of the optimal process
It expected that there will be a major challenge in the simulation of the process of AM and in the
development of the efficient model that will be capable of producing knowledge and information
at the overall scale of the desired part and the other processes that will be used in the making of
informed decisions(Romano, Ladani and Sadowski 2015). The quantities of interest that the
research should focus on will include the residual stress that will be capable of creating initial
conditions considered to be detrimental to service life concerns like fatigue and failure.
Distortion which is capable of halting the operation of the machine or lead to the placement of
the component into the undesired geometric envelope; the local and crucial characteristics that
are expected in the process. This will include a search of the point indicators of the possible
deviation point from the nominal characteristics as illustrated by various researchers. The general
be the utilization of two experimental models. One of the experiments utilizes a constant scan
pattern while the other one uses a rotating scan pattern. Both the two approaches employ the use
of cylindrical geometry and their design and construction are meant to provide measurements of
post-build distortion. The comparison of the two cases will, therefore, include the use of the post-
build measurements of the parts(Razavi et al 2018).
It is important to note that the control of the design will only be possible upon an understanding
of the parts of AM for the construction of the higher quality parts. It only by the establishment of
the process –property correlation that will allow for the reduction of the number of experiments
of trial and error as needed in the learning of the optimal process
It expected that there will be a major challenge in the simulation of the process of AM and in the
development of the efficient model that will be capable of producing knowledge and information
at the overall scale of the desired part and the other processes that will be used in the making of
informed decisions(Romano, Ladani and Sadowski 2015). The quantities of interest that the
research should focus on will include the residual stress that will be capable of creating initial
conditions considered to be detrimental to service life concerns like fatigue and failure.
Distortion which is capable of halting the operation of the machine or lead to the placement of
the component into the undesired geometric envelope; the local and crucial characteristics that
are expected in the process. This will include a search of the point indicators of the possible
deviation point from the nominal characteristics as illustrated by various researchers. The general
Paraphrase This Document
Need a fresh take? Get an instant paraphrase of this document with our AI Paraphraser
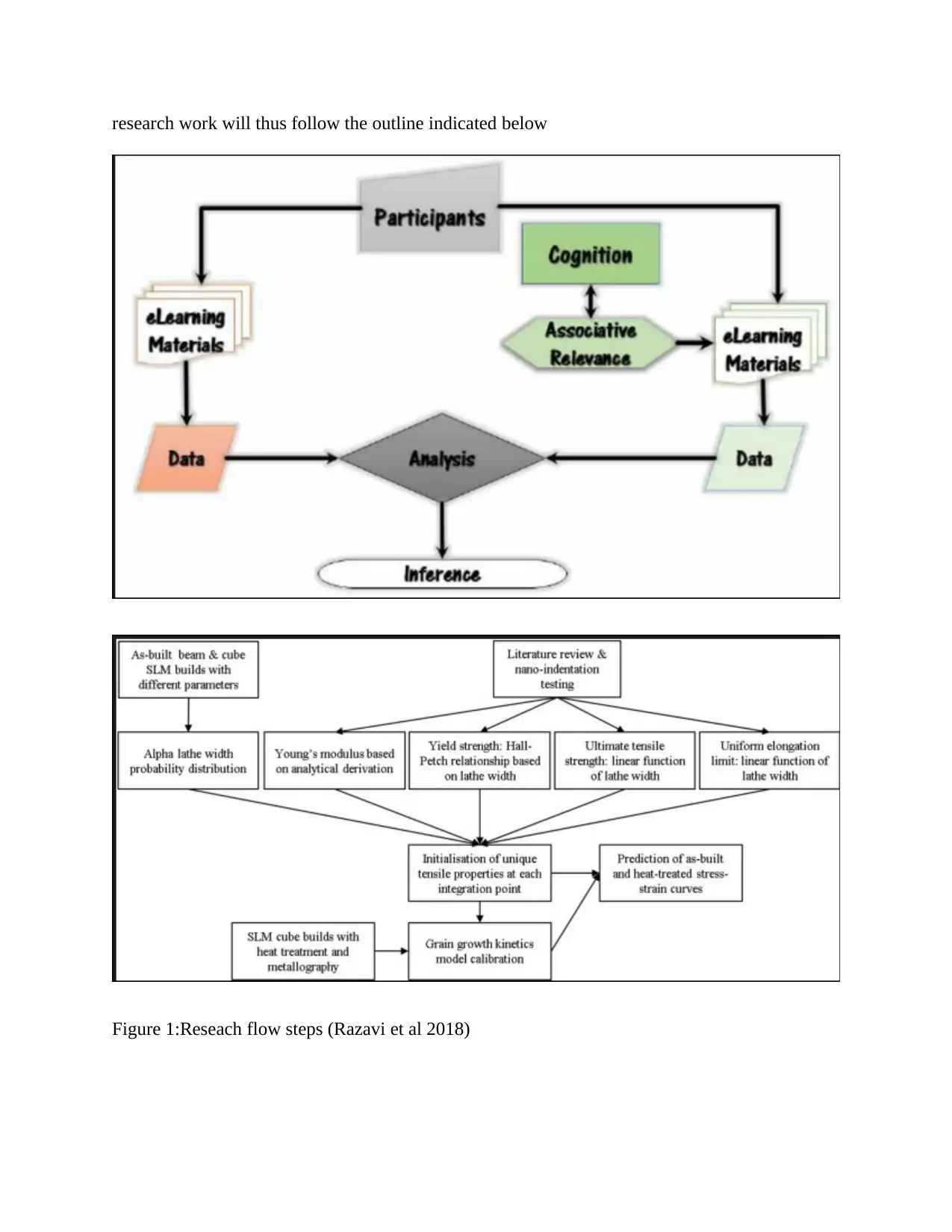
research work will thus follow the outline indicated below
Figure 1:Reseach flow steps (Razavi et al 2018)
Figure 1:Reseach flow steps (Razavi et al 2018)
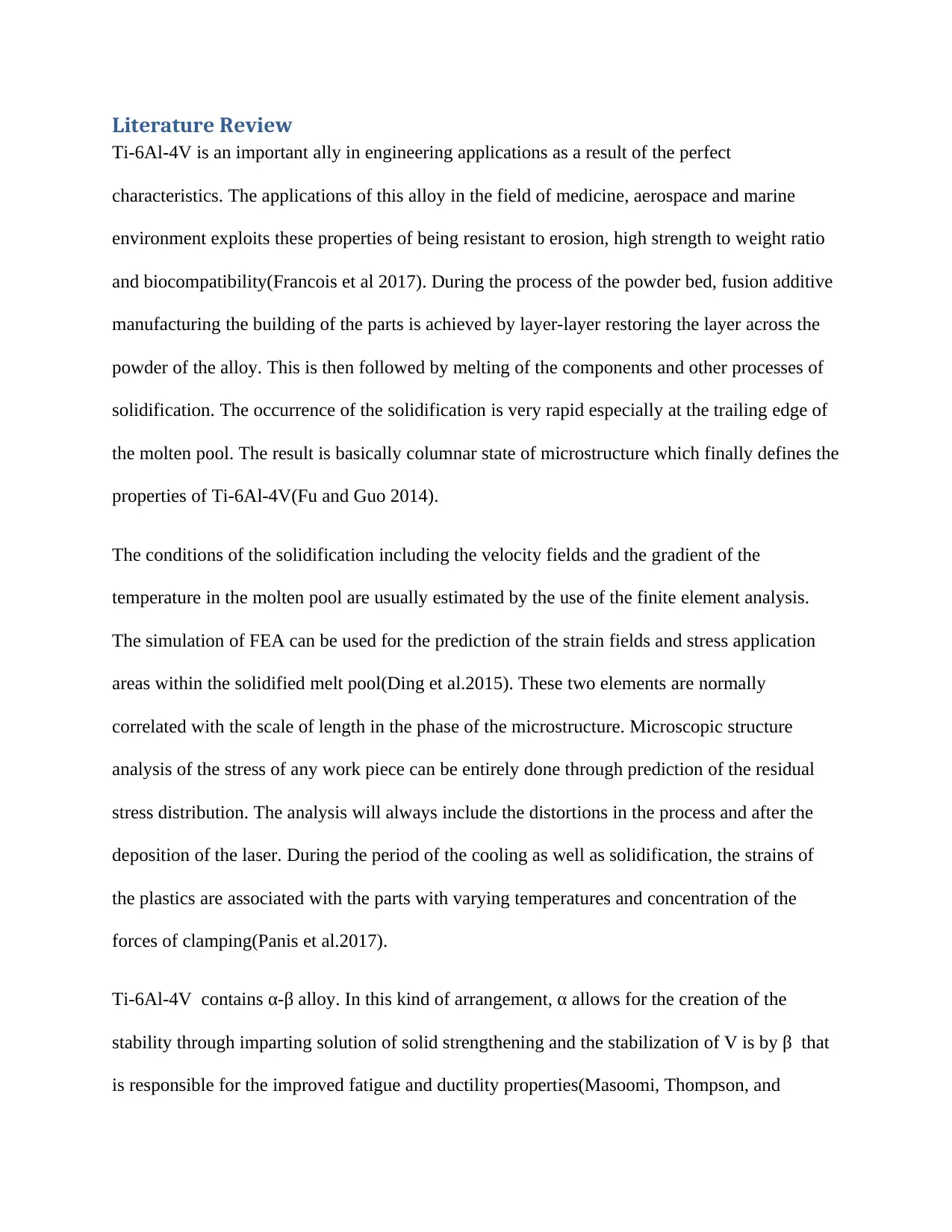
Literature Review
Ti-6Al-4V is an important ally in engineering applications as a result of the perfect
characteristics. The applications of this alloy in the field of medicine, aerospace and marine
environment exploits these properties of being resistant to erosion, high strength to weight ratio
and biocompatibility(Francois et al 2017). During the process of the powder bed, fusion additive
manufacturing the building of the parts is achieved by layer-layer restoring the layer across the
powder of the alloy. This is then followed by melting of the components and other processes of
solidification. The occurrence of the solidification is very rapid especially at the trailing edge of
the molten pool. The result is basically columnar state of microstructure which finally defines the
properties of Ti-6Al-4V(Fu and Guo 2014).
The conditions of the solidification including the velocity fields and the gradient of the
temperature in the molten pool are usually estimated by the use of the finite element analysis.
The simulation of FEA can be used for the prediction of the strain fields and stress application
areas within the solidified melt pool(Ding et al.2015). These two elements are normally
correlated with the scale of length in the phase of the microstructure. Microscopic structure
analysis of the stress of any work piece can be entirely done through prediction of the residual
stress distribution. The analysis will always include the distortions in the process and after the
deposition of the laser. During the period of the cooling as well as solidification, the strains of
the plastics are associated with the parts with varying temperatures and concentration of the
forces of clamping(Panis et al.2017).
Ti-6Al-4V contains α-β alloy. In this kind of arrangement, α allows for the creation of the
stability through imparting solution of solid strengthening and the stabilization of V is by β that
is responsible for the improved fatigue and ductility properties(Masoomi, Thompson, and
Ti-6Al-4V is an important ally in engineering applications as a result of the perfect
characteristics. The applications of this alloy in the field of medicine, aerospace and marine
environment exploits these properties of being resistant to erosion, high strength to weight ratio
and biocompatibility(Francois et al 2017). During the process of the powder bed, fusion additive
manufacturing the building of the parts is achieved by layer-layer restoring the layer across the
powder of the alloy. This is then followed by melting of the components and other processes of
solidification. The occurrence of the solidification is very rapid especially at the trailing edge of
the molten pool. The result is basically columnar state of microstructure which finally defines the
properties of Ti-6Al-4V(Fu and Guo 2014).
The conditions of the solidification including the velocity fields and the gradient of the
temperature in the molten pool are usually estimated by the use of the finite element analysis.
The simulation of FEA can be used for the prediction of the strain fields and stress application
areas within the solidified melt pool(Ding et al.2015). These two elements are normally
correlated with the scale of length in the phase of the microstructure. Microscopic structure
analysis of the stress of any work piece can be entirely done through prediction of the residual
stress distribution. The analysis will always include the distortions in the process and after the
deposition of the laser. During the period of the cooling as well as solidification, the strains of
the plastics are associated with the parts with varying temperatures and concentration of the
forces of clamping(Panis et al.2017).
Ti-6Al-4V contains α-β alloy. In this kind of arrangement, α allows for the creation of the
stability through imparting solution of solid strengthening and the stabilization of V is by β that
is responsible for the improved fatigue and ductility properties(Masoomi, Thompson, and
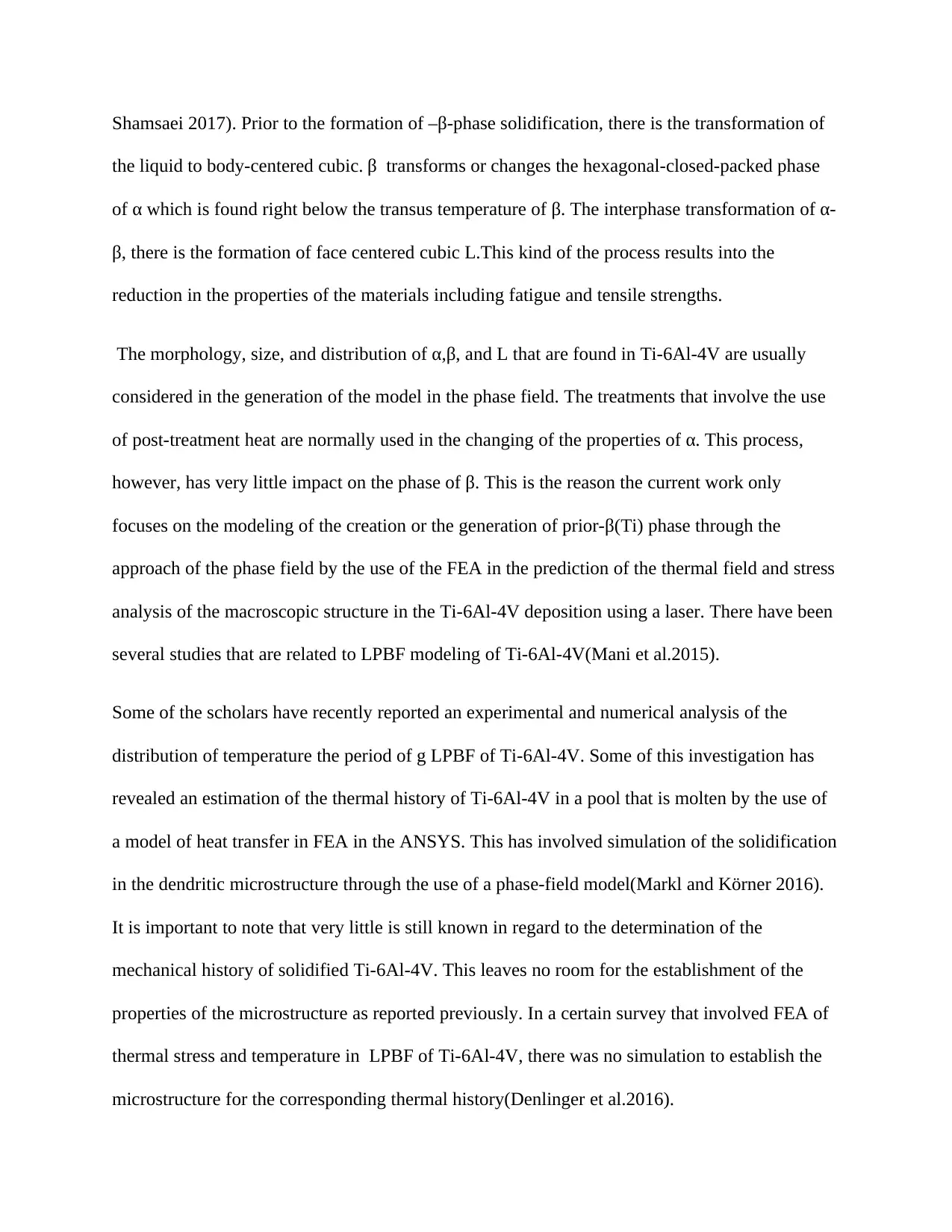
Shamsaei 2017). Prior to the formation of –β-phase solidification, there is the transformation of
the liquid to body-centered cubic. β transforms or changes the hexagonal-closed-packed phase
of α which is found right below the transus temperature of β. The interphase transformation of α-
β, there is the formation of face centered cubic L.This kind of the process results into the
reduction in the properties of the materials including fatigue and tensile strengths.
The morphology, size, and distribution of α,β, and L that are found in Ti-6Al-4V are usually
considered in the generation of the model in the phase field. The treatments that involve the use
of post-treatment heat are normally used in the changing of the properties of α. This process,
however, has very little impact on the phase of β. This is the reason the current work only
focuses on the modeling of the creation or the generation of prior-β(Ti) phase through the
approach of the phase field by the use of the FEA in the prediction of the thermal field and stress
analysis of the macroscopic structure in the Ti-6Al-4V deposition using a laser. There have been
several studies that are related to LPBF modeling of Ti-6Al-4V(Mani et al.2015).
Some of the scholars have recently reported an experimental and numerical analysis of the
distribution of temperature the period of g LPBF of Ti-6Al-4V. Some of this investigation has
revealed an estimation of the thermal history of Ti-6Al-4V in a pool that is molten by the use of
a model of heat transfer in FEA in the ANSYS. This has involved simulation of the solidification
in the dendritic microstructure through the use of a phase-field model(Markl and Körner 2016).
It is important to note that very little is still known in regard to the determination of the
mechanical history of solidified Ti-6Al-4V. This leaves no room for the establishment of the
properties of the microstructure as reported previously. In a certain survey that involved FEA of
thermal stress and temperature in LPBF of Ti-6Al-4V, there was no simulation to establish the
microstructure for the corresponding thermal history(Denlinger et al.2016).
the liquid to body-centered cubic. β transforms or changes the hexagonal-closed-packed phase
of α which is found right below the transus temperature of β. The interphase transformation of α-
β, there is the formation of face centered cubic L.This kind of the process results into the
reduction in the properties of the materials including fatigue and tensile strengths.
The morphology, size, and distribution of α,β, and L that are found in Ti-6Al-4V are usually
considered in the generation of the model in the phase field. The treatments that involve the use
of post-treatment heat are normally used in the changing of the properties of α. This process,
however, has very little impact on the phase of β. This is the reason the current work only
focuses on the modeling of the creation or the generation of prior-β(Ti) phase through the
approach of the phase field by the use of the FEA in the prediction of the thermal field and stress
analysis of the macroscopic structure in the Ti-6Al-4V deposition using a laser. There have been
several studies that are related to LPBF modeling of Ti-6Al-4V(Mani et al.2015).
Some of the scholars have recently reported an experimental and numerical analysis of the
distribution of temperature the period of g LPBF of Ti-6Al-4V. Some of this investigation has
revealed an estimation of the thermal history of Ti-6Al-4V in a pool that is molten by the use of
a model of heat transfer in FEA in the ANSYS. This has involved simulation of the solidification
in the dendritic microstructure through the use of a phase-field model(Markl and Körner 2016).
It is important to note that very little is still known in regard to the determination of the
mechanical history of solidified Ti-6Al-4V. This leaves no room for the establishment of the
properties of the microstructure as reported previously. In a certain survey that involved FEA of
thermal stress and temperature in LPBF of Ti-6Al-4V, there was no simulation to establish the
microstructure for the corresponding thermal history(Denlinger et al.2016).
Secure Best Marks with AI Grader
Need help grading? Try our AI Grader for instant feedback on your assignments.
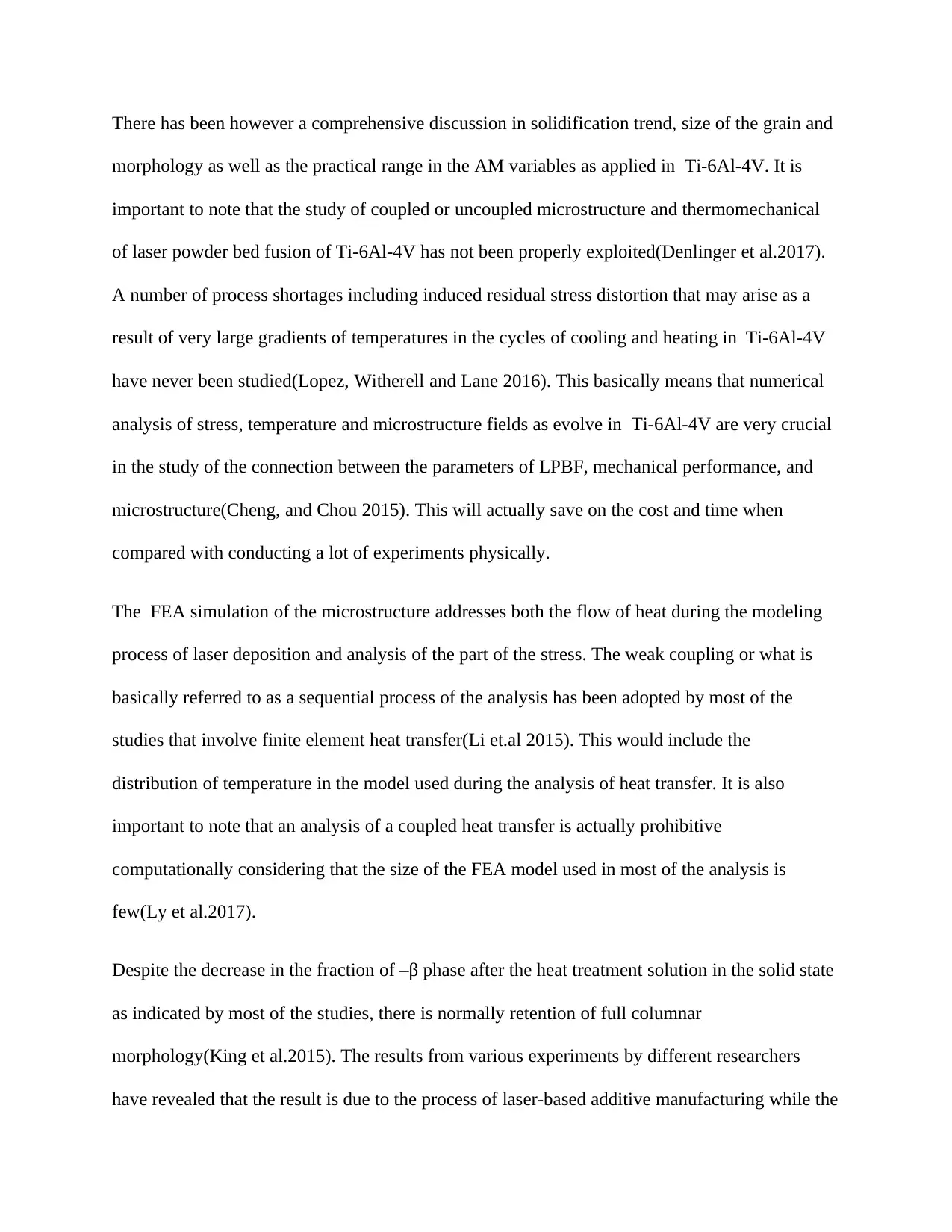
There has been however a comprehensive discussion in solidification trend, size of the grain and
morphology as well as the practical range in the AM variables as applied in Ti-6Al-4V. It is
important to note that the study of coupled or uncoupled microstructure and thermomechanical
of laser powder bed fusion of Ti-6Al-4V has not been properly exploited(Denlinger et al.2017).
A number of process shortages including induced residual stress distortion that may arise as a
result of very large gradients of temperatures in the cycles of cooling and heating in Ti-6Al-4V
have never been studied(Lopez, Witherell and Lane 2016). This basically means that numerical
analysis of stress, temperature and microstructure fields as evolve in Ti-6Al-4V are very crucial
in the study of the connection between the parameters of LPBF, mechanical performance, and
microstructure(Cheng, and Chou 2015). This will actually save on the cost and time when
compared with conducting a lot of experiments physically.
The FEA simulation of the microstructure addresses both the flow of heat during the modeling
process of laser deposition and analysis of the part of the stress. The weak coupling or what is
basically referred to as a sequential process of the analysis has been adopted by most of the
studies that involve finite element heat transfer(Li et.al 2015). This would include the
distribution of temperature in the model used during the analysis of heat transfer. It is also
important to note that an analysis of a coupled heat transfer is actually prohibitive
computationally considering that the size of the FEA model used in most of the analysis is
few(Ly et al.2017).
Despite the decrease in the fraction of –β phase after the heat treatment solution in the solid state
as indicated by most of the studies, there is normally retention of full columnar
morphology(King et al.2015). The results from various experiments by different researchers
have revealed that the result is due to the process of laser-based additive manufacturing while the
morphology as well as the practical range in the AM variables as applied in Ti-6Al-4V. It is
important to note that the study of coupled or uncoupled microstructure and thermomechanical
of laser powder bed fusion of Ti-6Al-4V has not been properly exploited(Denlinger et al.2017).
A number of process shortages including induced residual stress distortion that may arise as a
result of very large gradients of temperatures in the cycles of cooling and heating in Ti-6Al-4V
have never been studied(Lopez, Witherell and Lane 2016). This basically means that numerical
analysis of stress, temperature and microstructure fields as evolve in Ti-6Al-4V are very crucial
in the study of the connection between the parameters of LPBF, mechanical performance, and
microstructure(Cheng, and Chou 2015). This will actually save on the cost and time when
compared with conducting a lot of experiments physically.
The FEA simulation of the microstructure addresses both the flow of heat during the modeling
process of laser deposition and analysis of the part of the stress. The weak coupling or what is
basically referred to as a sequential process of the analysis has been adopted by most of the
studies that involve finite element heat transfer(Li et.al 2015). This would include the
distribution of temperature in the model used during the analysis of heat transfer. It is also
important to note that an analysis of a coupled heat transfer is actually prohibitive
computationally considering that the size of the FEA model used in most of the analysis is
few(Ly et al.2017).
Despite the decrease in the fraction of –β phase after the heat treatment solution in the solid state
as indicated by most of the studies, there is normally retention of full columnar
morphology(King et al.2015). The results from various experiments by different researchers
have revealed that the result is due to the process of laser-based additive manufacturing while the
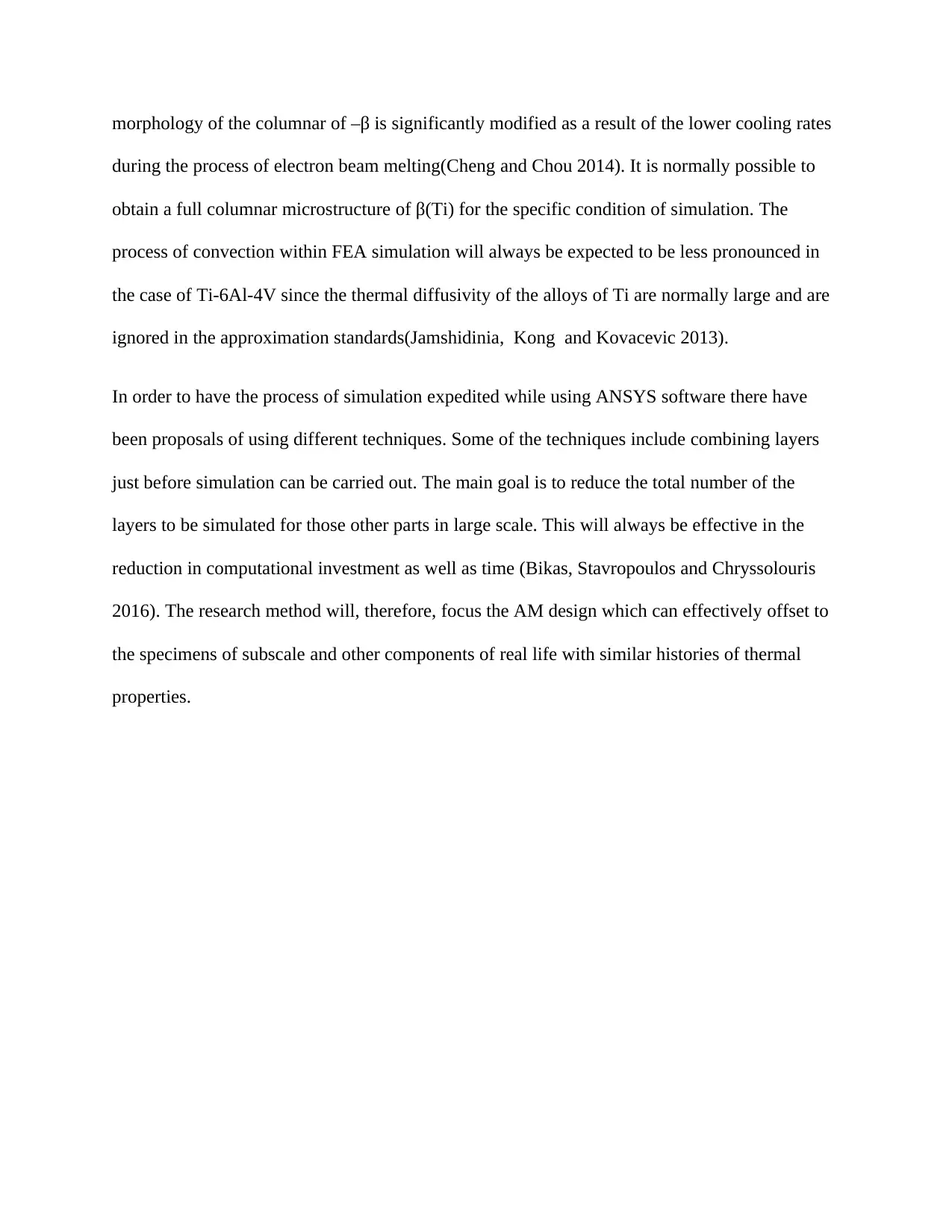
morphology of the columnar of –β is significantly modified as a result of the lower cooling rates
during the process of electron beam melting(Cheng and Chou 2014). It is normally possible to
obtain a full columnar microstructure of β(Ti) for the specific condition of simulation. The
process of convection within FEA simulation will always be expected to be less pronounced in
the case of Ti-6Al-4V since the thermal diffusivity of the alloys of Ti are normally large and are
ignored in the approximation standards(Jamshidinia, Kong and Kovacevic 2013).
In order to have the process of simulation expedited while using ANSYS software there have
been proposals of using different techniques. Some of the techniques include combining layers
just before simulation can be carried out. The main goal is to reduce the total number of the
layers to be simulated for those other parts in large scale. This will always be effective in the
reduction in computational investment as well as time (Bikas, Stavropoulos and Chryssolouris
2016). The research method will, therefore, focus the AM design which can effectively offset to
the specimens of subscale and other components of real life with similar histories of thermal
properties.
during the process of electron beam melting(Cheng and Chou 2014). It is normally possible to
obtain a full columnar microstructure of β(Ti) for the specific condition of simulation. The
process of convection within FEA simulation will always be expected to be less pronounced in
the case of Ti-6Al-4V since the thermal diffusivity of the alloys of Ti are normally large and are
ignored in the approximation standards(Jamshidinia, Kong and Kovacevic 2013).
In order to have the process of simulation expedited while using ANSYS software there have
been proposals of using different techniques. Some of the techniques include combining layers
just before simulation can be carried out. The main goal is to reduce the total number of the
layers to be simulated for those other parts in large scale. This will always be effective in the
reduction in computational investment as well as time (Bikas, Stavropoulos and Chryssolouris
2016). The research method will, therefore, focus the AM design which can effectively offset to
the specimens of subscale and other components of real life with similar histories of thermal
properties.
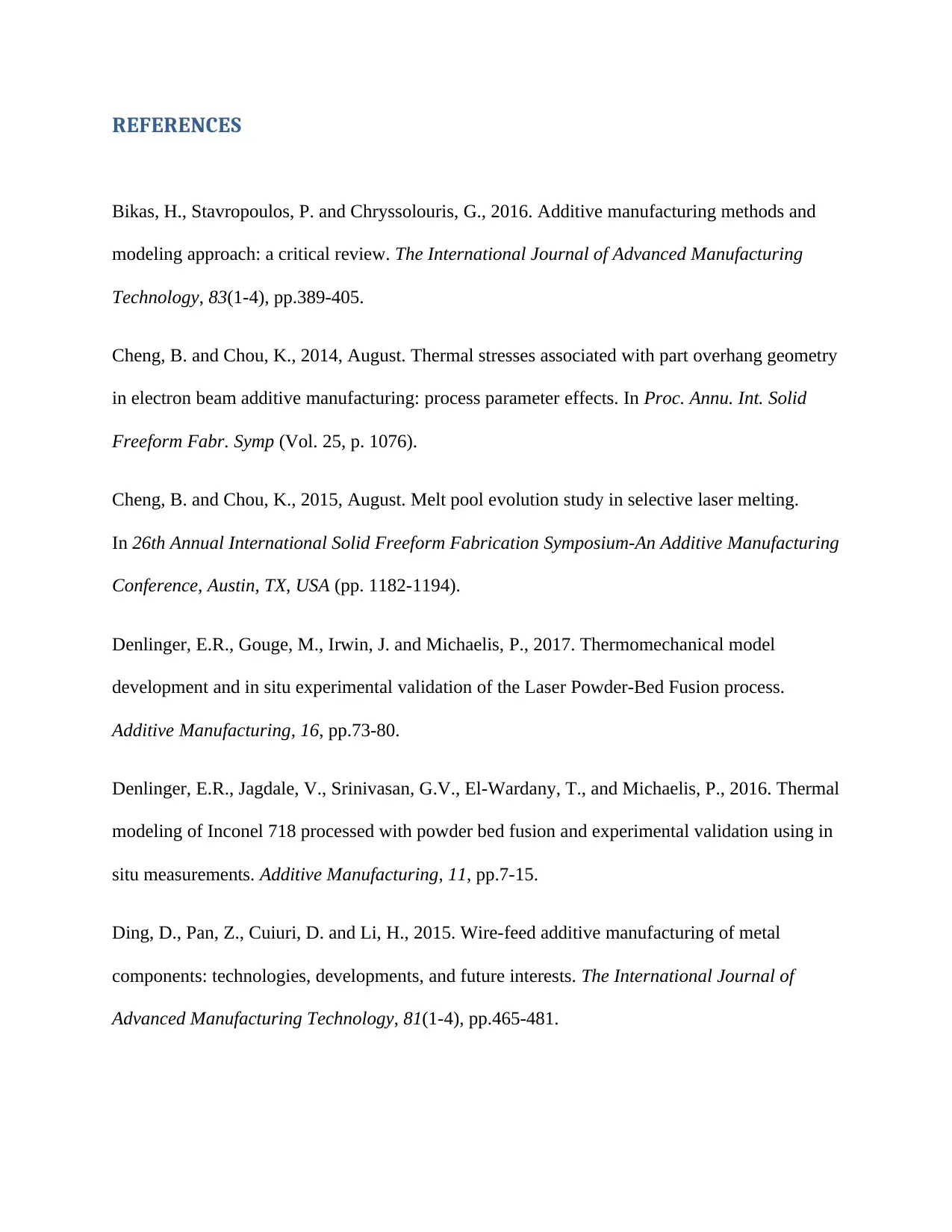
REFERENCES
Bikas, H., Stavropoulos, P. and Chryssolouris, G., 2016. Additive manufacturing methods and
modeling approach: a critical review. The International Journal of Advanced Manufacturing
Technology, 83(1-4), pp.389-405.
Cheng, B. and Chou, K., 2014, August. Thermal stresses associated with part overhang geometry
in electron beam additive manufacturing: process parameter effects. In Proc. Annu. Int. Solid
Freeform Fabr. Symp (Vol. 25, p. 1076).
Cheng, B. and Chou, K., 2015, August. Melt pool evolution study in selective laser melting.
In 26th Annual International Solid Freeform Fabrication Symposium-An Additive Manufacturing
Conference, Austin, TX, USA (pp. 1182-1194).
Denlinger, E.R., Gouge, M., Irwin, J. and Michaelis, P., 2017. Thermomechanical model
development and in situ experimental validation of the Laser Powder-Bed Fusion process.
Additive Manufacturing, 16, pp.73-80.
Denlinger, E.R., Jagdale, V., Srinivasan, G.V., El-Wardany, T., and Michaelis, P., 2016. Thermal
modeling of Inconel 718 processed with powder bed fusion and experimental validation using in
situ measurements. Additive Manufacturing, 11, pp.7-15.
Ding, D., Pan, Z., Cuiuri, D. and Li, H., 2015. Wire-feed additive manufacturing of metal
components: technologies, developments, and future interests. The International Journal of
Advanced Manufacturing Technology, 81(1-4), pp.465-481.
Bikas, H., Stavropoulos, P. and Chryssolouris, G., 2016. Additive manufacturing methods and
modeling approach: a critical review. The International Journal of Advanced Manufacturing
Technology, 83(1-4), pp.389-405.
Cheng, B. and Chou, K., 2014, August. Thermal stresses associated with part overhang geometry
in electron beam additive manufacturing: process parameter effects. In Proc. Annu. Int. Solid
Freeform Fabr. Symp (Vol. 25, p. 1076).
Cheng, B. and Chou, K., 2015, August. Melt pool evolution study in selective laser melting.
In 26th Annual International Solid Freeform Fabrication Symposium-An Additive Manufacturing
Conference, Austin, TX, USA (pp. 1182-1194).
Denlinger, E.R., Gouge, M., Irwin, J. and Michaelis, P., 2017. Thermomechanical model
development and in situ experimental validation of the Laser Powder-Bed Fusion process.
Additive Manufacturing, 16, pp.73-80.
Denlinger, E.R., Jagdale, V., Srinivasan, G.V., El-Wardany, T., and Michaelis, P., 2016. Thermal
modeling of Inconel 718 processed with powder bed fusion and experimental validation using in
situ measurements. Additive Manufacturing, 11, pp.7-15.
Ding, D., Pan, Z., Cuiuri, D. and Li, H., 2015. Wire-feed additive manufacturing of metal
components: technologies, developments, and future interests. The International Journal of
Advanced Manufacturing Technology, 81(1-4), pp.465-481.
Paraphrase This Document
Need a fresh take? Get an instant paraphrase of this document with our AI Paraphraser
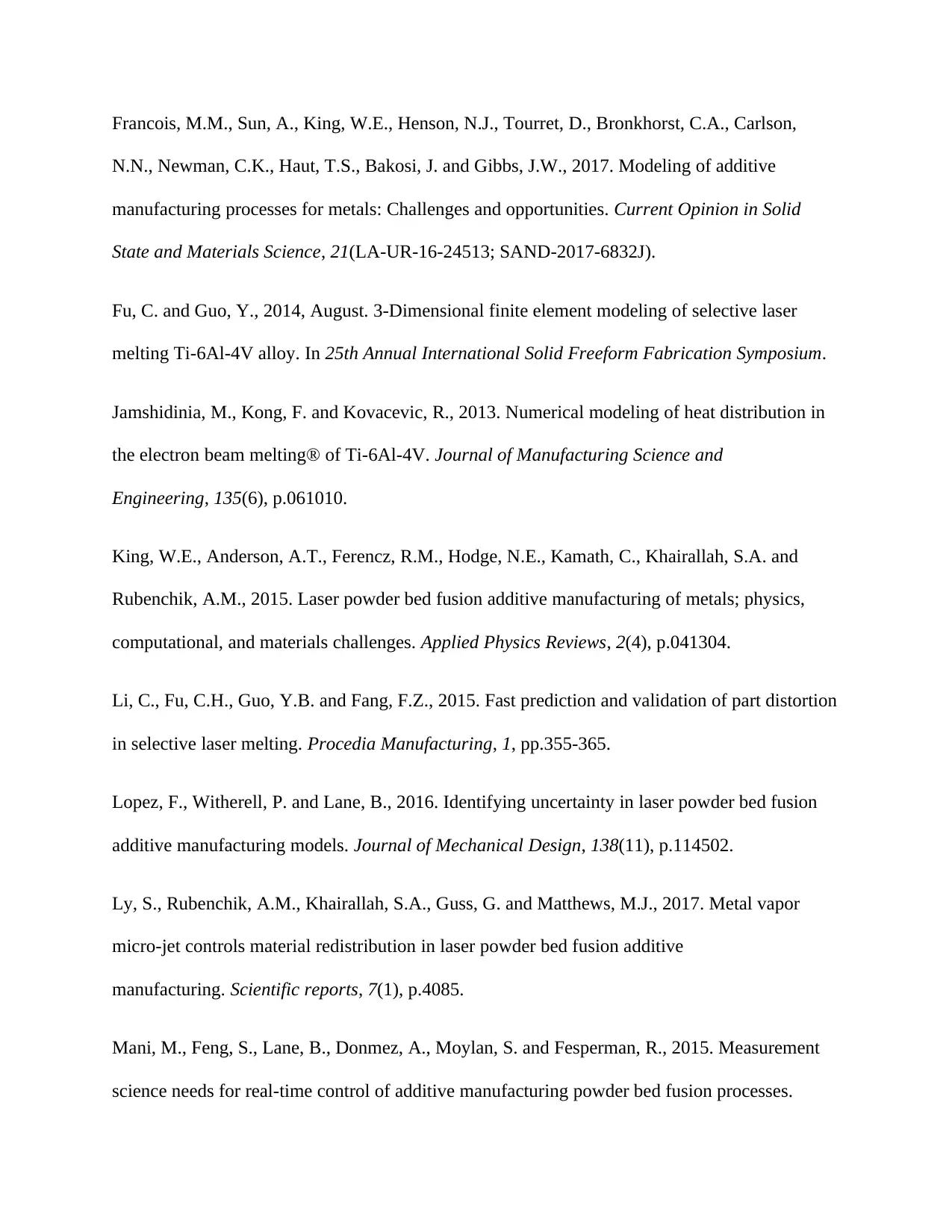
Francois, M.M., Sun, A., King, W.E., Henson, N.J., Tourret, D., Bronkhorst, C.A., Carlson,
N.N., Newman, C.K., Haut, T.S., Bakosi, J. and Gibbs, J.W., 2017. Modeling of additive
manufacturing processes for metals: Challenges and opportunities. Current Opinion in Solid
State and Materials Science, 21(LA-UR-16-24513; SAND-2017-6832J).
Fu, C. and Guo, Y., 2014, August. 3-Dimensional finite element modeling of selective laser
melting Ti-6Al-4V alloy. In 25th Annual International Solid Freeform Fabrication Symposium.
Jamshidinia, M., Kong, F. and Kovacevic, R., 2013. Numerical modeling of heat distribution in
the electron beam melting® of Ti-6Al-4V. Journal of Manufacturing Science and
Engineering, 135(6), p.061010.
King, W.E., Anderson, A.T., Ferencz, R.M., Hodge, N.E., Kamath, C., Khairallah, S.A. and
Rubenchik, A.M., 2015. Laser powder bed fusion additive manufacturing of metals; physics,
computational, and materials challenges. Applied Physics Reviews, 2(4), p.041304.
Li, C., Fu, C.H., Guo, Y.B. and Fang, F.Z., 2015. Fast prediction and validation of part distortion
in selective laser melting. Procedia Manufacturing, 1, pp.355-365.
Lopez, F., Witherell, P. and Lane, B., 2016. Identifying uncertainty in laser powder bed fusion
additive manufacturing models. Journal of Mechanical Design, 138(11), p.114502.
Ly, S., Rubenchik, A.M., Khairallah, S.A., Guss, G. and Matthews, M.J., 2017. Metal vapor
micro-jet controls material redistribution in laser powder bed fusion additive
manufacturing. Scientific reports, 7(1), p.4085.
Mani, M., Feng, S., Lane, B., Donmez, A., Moylan, S. and Fesperman, R., 2015. Measurement
science needs for real-time control of additive manufacturing powder bed fusion processes.
N.N., Newman, C.K., Haut, T.S., Bakosi, J. and Gibbs, J.W., 2017. Modeling of additive
manufacturing processes for metals: Challenges and opportunities. Current Opinion in Solid
State and Materials Science, 21(LA-UR-16-24513; SAND-2017-6832J).
Fu, C. and Guo, Y., 2014, August. 3-Dimensional finite element modeling of selective laser
melting Ti-6Al-4V alloy. In 25th Annual International Solid Freeform Fabrication Symposium.
Jamshidinia, M., Kong, F. and Kovacevic, R., 2013. Numerical modeling of heat distribution in
the electron beam melting® of Ti-6Al-4V. Journal of Manufacturing Science and
Engineering, 135(6), p.061010.
King, W.E., Anderson, A.T., Ferencz, R.M., Hodge, N.E., Kamath, C., Khairallah, S.A. and
Rubenchik, A.M., 2015. Laser powder bed fusion additive manufacturing of metals; physics,
computational, and materials challenges. Applied Physics Reviews, 2(4), p.041304.
Li, C., Fu, C.H., Guo, Y.B. and Fang, F.Z., 2015. Fast prediction and validation of part distortion
in selective laser melting. Procedia Manufacturing, 1, pp.355-365.
Lopez, F., Witherell, P. and Lane, B., 2016. Identifying uncertainty in laser powder bed fusion
additive manufacturing models. Journal of Mechanical Design, 138(11), p.114502.
Ly, S., Rubenchik, A.M., Khairallah, S.A., Guss, G. and Matthews, M.J., 2017. Metal vapor
micro-jet controls material redistribution in laser powder bed fusion additive
manufacturing. Scientific reports, 7(1), p.4085.
Mani, M., Feng, S., Lane, B., Donmez, A., Moylan, S. and Fesperman, R., 2015. Measurement
science needs for real-time control of additive manufacturing powder bed fusion processes.
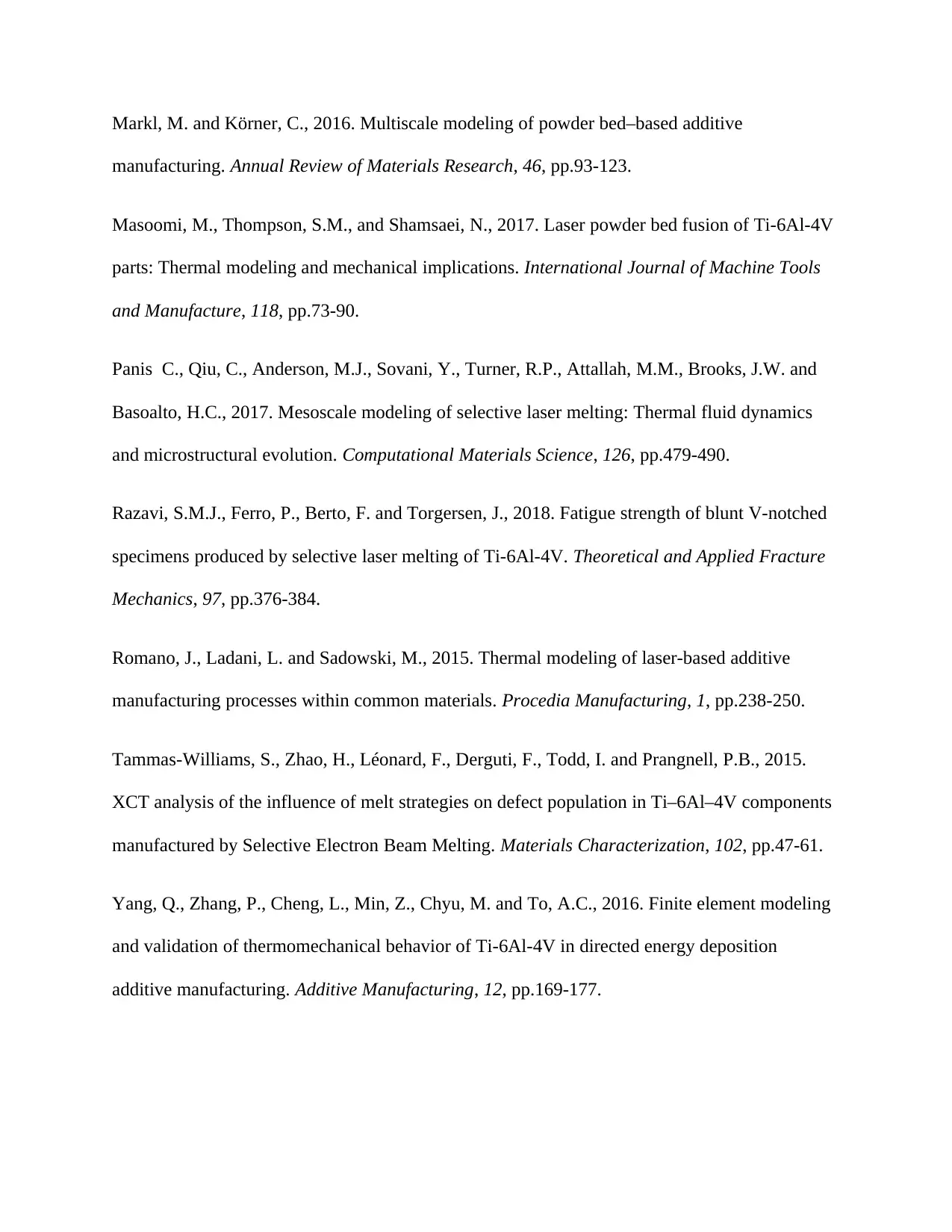
Markl, M. and Körner, C., 2016. Multiscale modeling of powder bed–based additive
manufacturing. Annual Review of Materials Research, 46, pp.93-123.
Masoomi, M., Thompson, S.M., and Shamsaei, N., 2017. Laser powder bed fusion of Ti-6Al-4V
parts: Thermal modeling and mechanical implications. International Journal of Machine Tools
and Manufacture, 118, pp.73-90.
Panis C., Qiu, C., Anderson, M.J., Sovani, Y., Turner, R.P., Attallah, M.M., Brooks, J.W. and
Basoalto, H.C., 2017. Mesoscale modeling of selective laser melting: Thermal fluid dynamics
and microstructural evolution. Computational Materials Science, 126, pp.479-490.
Razavi, S.M.J., Ferro, P., Berto, F. and Torgersen, J., 2018. Fatigue strength of blunt V-notched
specimens produced by selective laser melting of Ti-6Al-4V. Theoretical and Applied Fracture
Mechanics, 97, pp.376-384.
Romano, J., Ladani, L. and Sadowski, M., 2015. Thermal modeling of laser-based additive
manufacturing processes within common materials. Procedia Manufacturing, 1, pp.238-250.
Tammas-Williams, S., Zhao, H., Léonard, F., Derguti, F., Todd, I. and Prangnell, P.B., 2015.
XCT analysis of the influence of melt strategies on defect population in Ti–6Al–4V components
manufactured by Selective Electron Beam Melting. Materials Characterization, 102, pp.47-61.
Yang, Q., Zhang, P., Cheng, L., Min, Z., Chyu, M. and To, A.C., 2016. Finite element modeling
and validation of thermomechanical behavior of Ti-6Al-4V in directed energy deposition
additive manufacturing. Additive Manufacturing, 12, pp.169-177.
manufacturing. Annual Review of Materials Research, 46, pp.93-123.
Masoomi, M., Thompson, S.M., and Shamsaei, N., 2017. Laser powder bed fusion of Ti-6Al-4V
parts: Thermal modeling and mechanical implications. International Journal of Machine Tools
and Manufacture, 118, pp.73-90.
Panis C., Qiu, C., Anderson, M.J., Sovani, Y., Turner, R.P., Attallah, M.M., Brooks, J.W. and
Basoalto, H.C., 2017. Mesoscale modeling of selective laser melting: Thermal fluid dynamics
and microstructural evolution. Computational Materials Science, 126, pp.479-490.
Razavi, S.M.J., Ferro, P., Berto, F. and Torgersen, J., 2018. Fatigue strength of blunt V-notched
specimens produced by selective laser melting of Ti-6Al-4V. Theoretical and Applied Fracture
Mechanics, 97, pp.376-384.
Romano, J., Ladani, L. and Sadowski, M., 2015. Thermal modeling of laser-based additive
manufacturing processes within common materials. Procedia Manufacturing, 1, pp.238-250.
Tammas-Williams, S., Zhao, H., Léonard, F., Derguti, F., Todd, I. and Prangnell, P.B., 2015.
XCT analysis of the influence of melt strategies on defect population in Ti–6Al–4V components
manufactured by Selective Electron Beam Melting. Materials Characterization, 102, pp.47-61.
Yang, Q., Zhang, P., Cheng, L., Min, Z., Chyu, M. and To, A.C., 2016. Finite element modeling
and validation of thermomechanical behavior of Ti-6Al-4V in directed energy deposition
additive manufacturing. Additive Manufacturing, 12, pp.169-177.
1 out of 18
Related Documents
![[object Object]](/_next/image/?url=%2F_next%2Fstatic%2Fmedia%2Flogo.6d15ce61.png&w=640&q=75)
Your All-in-One AI-Powered Toolkit for Academic Success.
+13062052269
info@desklib.com
Available 24*7 on WhatsApp / Email
Unlock your academic potential
© 2024 | Zucol Services PVT LTD | All rights reserved.